- 1Division of Marine Science and Conservation, Nicholas School of the Environment, Duke University, Beaufort, NC, United States
- 2Department of Geology and Geophysics, University of Wyoming, Laramie, WY, United States
- 3Department of Geology and Geophysics, Woods Hole Oceanographic Institution, Woods Hole, MA, United States
When hydrothermal activity ceases at black-smoker chimneys on mid-ocean ridges, populations of associated invertebrates hosting chemoautotrophic endosymbionts decline and then disappear, but the chimneys can persist on the seabed as relicts. Suspension-feeding brisingid seastars colonize hydrothermally inactive (relict) chimneys on the East Pacific Rise (EPR), though their distribution relative to available hard substrata and proximity to hydrothermal activity is poorly documented. In this study, brisingid abundance on sulfide and basalt substrata was assessed along an ∼3,700 m ROV Jason II transect at the summit of Pito Seamount (SE Pacific; ∼2,275 m). Brisingids were non-randomly distributed, with highest densities (up to ∼300 m–2) on relict sulfides chimneys near active black smokers. Brisingids were relatively uncommon on basalt substrata, and absent on black smokers. We infer that both relict sulfide structures and proximity to black smokers play key roles in the maintenance of dense brisingid populations on Pito Seamount and in similar environments on the EPR. Our observations suggest that experimental introduction of “artificial” relict chimneys providing microtopographic relief could test whether such an approach might mitigate potential impacts of mineral extraction on populations of suspension-feeding invertebrates.
Introduction
High-temperature hydrothermal activity (black smokers) in the deep sea typically forms meters- to 10 s-of-meters-high, 3-dimensional structures (chimneys) composed of precipitated sulfide minerals rich in copper, iron, zinc, and other metals (Petersen et al., 2016). Chemosynthetic primary productivity is intense at black smokers (Van Dover, 2000) and is exported beyond the immediate community of endemic invertebrate and fish taxa associated with venting fluids (Levin et al., 2016). Indeed, 10–33% of the particulate organic carbon reaching the entire deep ocean floor worldwide may be stimulated by activity at hydrothermal vents (German et al., 2015).
Black smokers ultimately wane and expire as conduits are clogged through mineralization, as flow is redirected due to tectonic activity, and as volcanic eruptions repave the ocean floor (Fustec et al., 2013; Van Dover, 2019; Jamieson and Gartman, 2020). Relict, hydrothermally inactive sulfide structures may remain as chimneys and mounds, or collapse into blocks and rubble. Where high-temperature hydrothermal activity persists for long periods (thousands of years or more; Lalou et al., 1985, 1995), polymetallic sulfides may accumulate as deposits of sufficient size and mineral quality to be of interest to an emergent deep-sea mining industry (Hoagland et al., 2010; Hannington et al., 2011; Cherkashov, 2017). Long-duration hydrothermal activity and large accumulations of mineral precipitates at relatively low spatial frequencies are known from intermediate-, slow-, and ultraslow- spreading mid-ocean ridges (Rona et al., 1993; Halbach et al., 1998; Karson et al., 2015), as well as off-axis sites on the fast-spreading East Pacific Rise (EPR) (Hekinian and Fouquet, 1985; Fouquet et al., 1996). On the EPR itself, the spatial frequency of vent fields with high-temperature (∼350°C) black smokers is relatively high, on the order of ∼4 fields per 100 km (Chen et al., 2021), and the duration of venting activity is short (decadal scale or less; Lalou and Brichet, 1982; Karson et al., 2015).
Because of the relatively short life cycle for black smokers on the EPR, sulfide accumulations are small (<3,000 tons for a given vent field; Hannington et al., 2011). Further, accumulations of mineral precipitates along the EPR are composed primarily of iron sulfides with little to no economic value at present (Petersen et al., 2016). To date, no contracts for polymetallic sulfide mineral exploration along fast-spreading mid-ocean ridges of the seabed beyond national jurisdictions in the eastern Pacific (i.e., along the EPR, Galapagos Spreading Center, Pacific-Antarctic Ridge) have been awarded by the International Seabed Authority. Nevertheless, study of the biology of hydrothermally inactive sulfide accumulations in this region adds to our understanding of the role of seabed sulfide occurrences as habitat and may inform environmental management of future seabed mining in this area.
The fauna associated with relict sulfide accumulations is in general poorly known (reviewed in Van Dover, 2019; Van Dover et al., 2020). Small macrofaunal and meiofaunal organisms such as nematodes, gastropods, copepods, and amphipods occur in association with inactive sulfides on the EPR (Gollner et al., 2020). Suspension-feeding brisingid seastars have also been reported on relict sulfide structures on the EPR (Desbruyeres et al., 2006) and elsewhere from peripheral zones of active vent fields (Kim and Hammerstrom, 2012). Deep-sea seastars in the family Brisingidae are common benthic organisms found worldwide (Emson and Young, 1994), often associated with hard substrata such as drop stones (Lacharité and Metaxas, 2017), corals (Mah, 2016), or manmade objects (Mordecai et al., 2011; Edwards et al., 2016). Brisingids rely on deep-ocean currents for delivery of prey (e.g., copepods and other small planktonic organisms) that is captured by raptorial pedicellariae and transferred to the mouth (Emson and Young, 1994; Howell et al., 2003; Gale et al., 2013). Brisingid seastars on relict sulfide structures are not endemic to active hydrothermal vents or other deep-sea chemosynthetic ecosystems (Mah et al., 2015), but likely benefit from primary productivity associated with chemosynthetic production (Carney, 2010; Levin et al., 2016).
To increase our understanding of the distribution of brisingid seastars in the vicinity of deep-sea hydrothermal vents and the potential importance of relict sulfides as habitat, we undertook a census of brisingid distributions relative to substratum type along a geological transect at Pito Seamount (2,240–2,325 m; Naar et al., 2004; Cheadle et al., 2017), which lies at the northern tip of the propagating EPR axis on the eastern side of the Easter Microplate (Figure 1). Hydrothermal activity was first discovered on Pito Seamount in 1993 by scientists in the submersible Nautile (Naar et al., 2004). In 2017, more than 50 black smokers and relict sulfide chimneys comprising the Nautile Hydrothermal Field were detected using high-resolution mapping with the Autonomous Underwater Vehicle (AUV) Sentry. Black smoker and relict sulfide chimney targets were further explored using the Remotely Operated Vehicle (ROV) Jason II during the 2017 geological expedition.
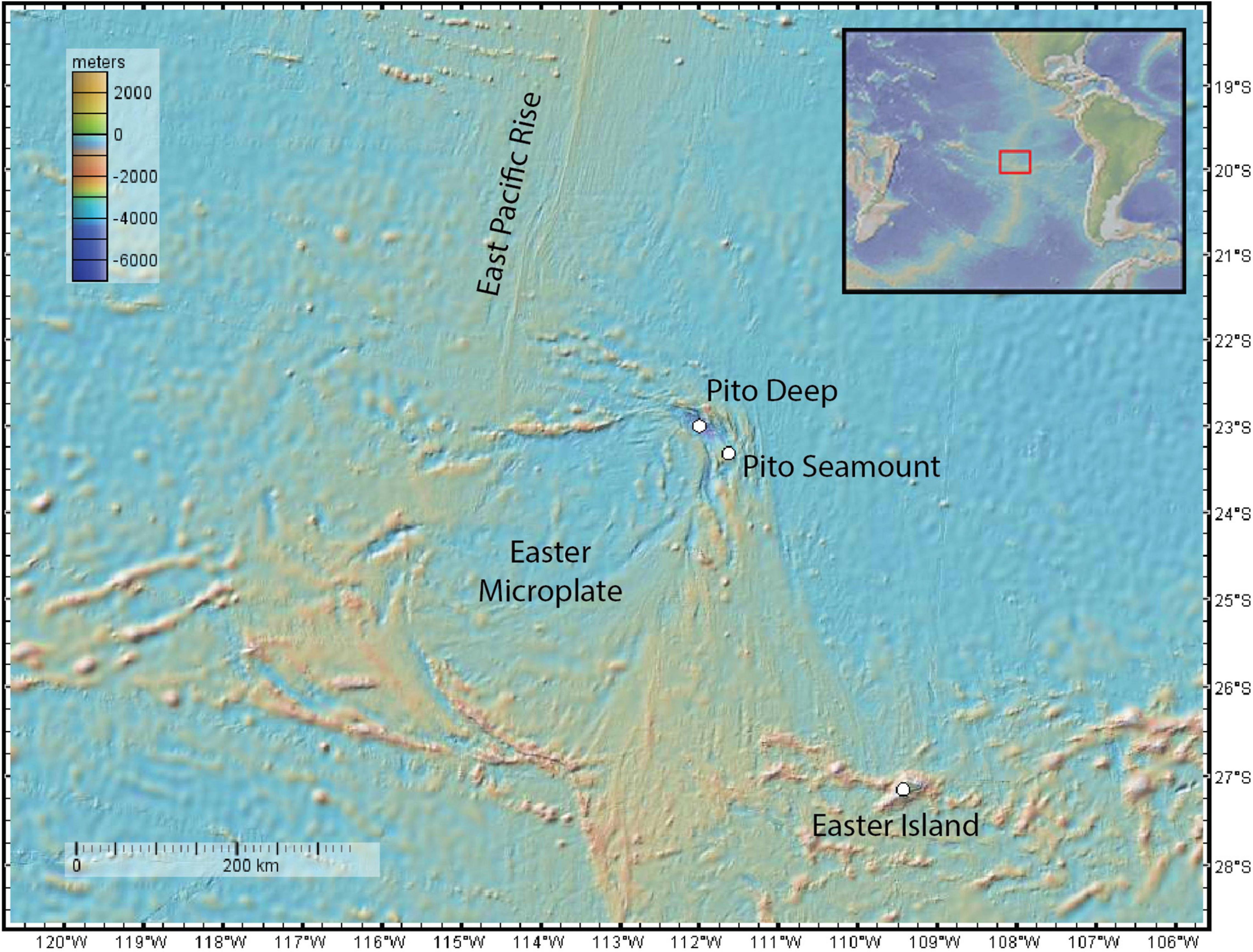
Figure 1. Study locations: Pito Seamount, Pito Deep. GeoMapApp (www.geomapapp.org).
Here we provide a descriptive account of brisingid distributions, using tests of a posteriori hypotheses to assess whether brisingid seastars (i) aggregate on relict sulfides, especially sulfide chimneys with microscale relief (∼0.5–15 m), (ii) are relatively uncommon on basalt substrata, (iii) are absent on black smokers, and (iv) are scarce in the nearby Pito Deep, where hydrothermal activity is absent (51 km northwest of Pito Seamount, Figure 1).
Materials and Methods
Mapping
R/V Atlantis Cruise AT37-08 was devoted to mapping and understanding volcanic processes in Pito Deep and rifting of Pito Seamount [PaleoMagnetism and Gabbro Cruise (PMaG); M Cheadle, Chief Scientist]. The cruise included a 1-dive subcomponent to assess the extent of the hydrothermal field reported by Naar et al. (2004) on Pito Seamount. AUV Sentry Dive 427 (6 February 2017) mapped the summit of Pito Seamount (0.5–1 m resolution) using a Reson 7125 multibeam sonar system at a nominal altitude of 80 m above the seafloor, with 85 m line spacing to ensure > 100% overlapping multibeam coverage between adjacent survey lines. The multibeam coverage angle was reduced to 70° from the standard 120° to accommodate the increased altitude and reduced track-line spacing. Fledermaus 7.7.4 was used to render 3-D perspectives of the terrain. Multibeam data was used to identify sulfide targets on the seafloor.
Image Collection, Archive, and Analysis
Time-stamped and georeferenced (latitude, longitude, depth) images were captured during ROV Jason II Dive 961 at the Nautile Hydrothermal Field on Pito Seamount (23.3275°S, 111.635°W; 10 February 2017) during the PMaG Cruise to document the terrain and geological sampling efforts. During this dive transect, most of the sulfide features identified during the multibeam mapping effort were visited. SciCam images (frame grabs) were automatically collected every 30 s, augmented by manual image captures at the discretion of scientists on watch at the time. Due to the nature of the geological exploration, vehicle speed, heading, and altitude were variable, as was stand-off distance from vertical surfaces. The frequency of image collection was also variable, increasing in the vicinity of black smokers and hydrothermally inactive sulfides occurrences and when geological samples were collected. Thumbnail images from the SciCam are available on the Jason Virtual Van System1 under “2017: at37–08”; images and metadata are archived at the Woods Hole Oceanographic Institution. There are evident limitations to the dataset obtained in this study, including an inability (i) to assess total abundance of brisingids on any given vertical surface, since only one face of a structure was in the field of view on the transect, (ii) to reliably measure brisingid densities, since stand-off distances from vertical surfaces were variable and not measured, or (iii) to capture a quantitative estimate of sampling effort for each substratum type. Nevertheless, we could reliably quantify the abundance of brisingid seastars in each photo and the nature of the substratum on which each brisingid was located. Duplicate counting of individuals was avoided by attention to overlapping fields of view between images. Because lighting, water clarity, and scale varied among photos and likely contributed to color variation in the images, no quantitative data was collected on the relative abundance of two different color morphs (orange and white) of Pito Seamount brisingids.
The substratum was broadly classified as basalt or sulfide. Following Karson et al. (2015), basalt substrata were further classified as hackly, rubble, edge (e.g., scarp edge), pillow, sheet, ropey, and lobate (Figure 2). Hydrothermally inactive (relict) sulfide substrata (i.e., visually devoid of shimmering water or other indication of fluid flux) were further classified as chimney, boulder, ledge, and debris (Figure 3). Locations of black smokers observed along the Dive 961 transect were also recorded (Table 1).
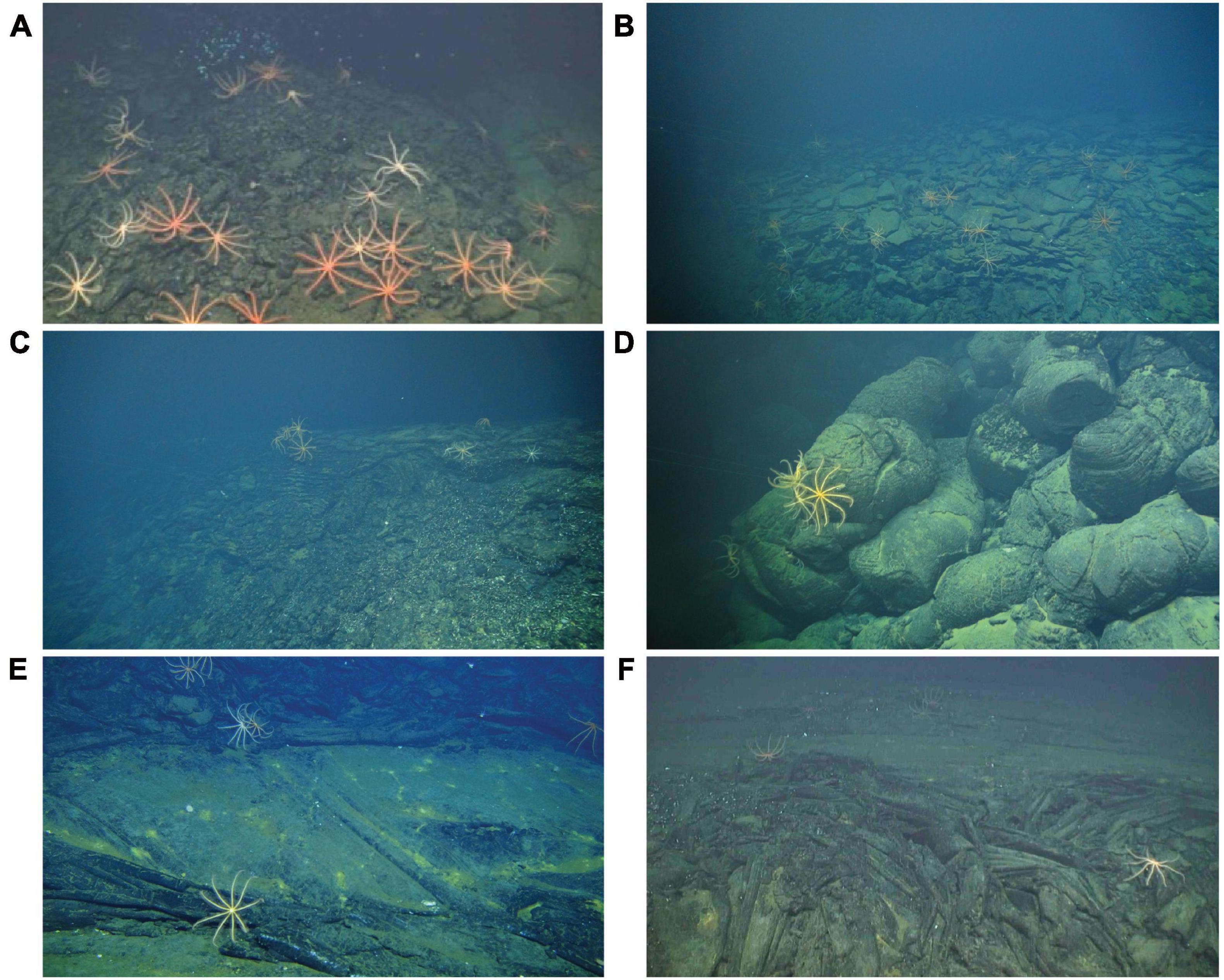
Figure 2. Examples of basalt substrata hosting brisingids at Pito Seamount: (A) hackly and sheet; (B) edge and rubble; (C) lobate and edge; (D) pillow; (E) sheet; (F) ropey and sheet.
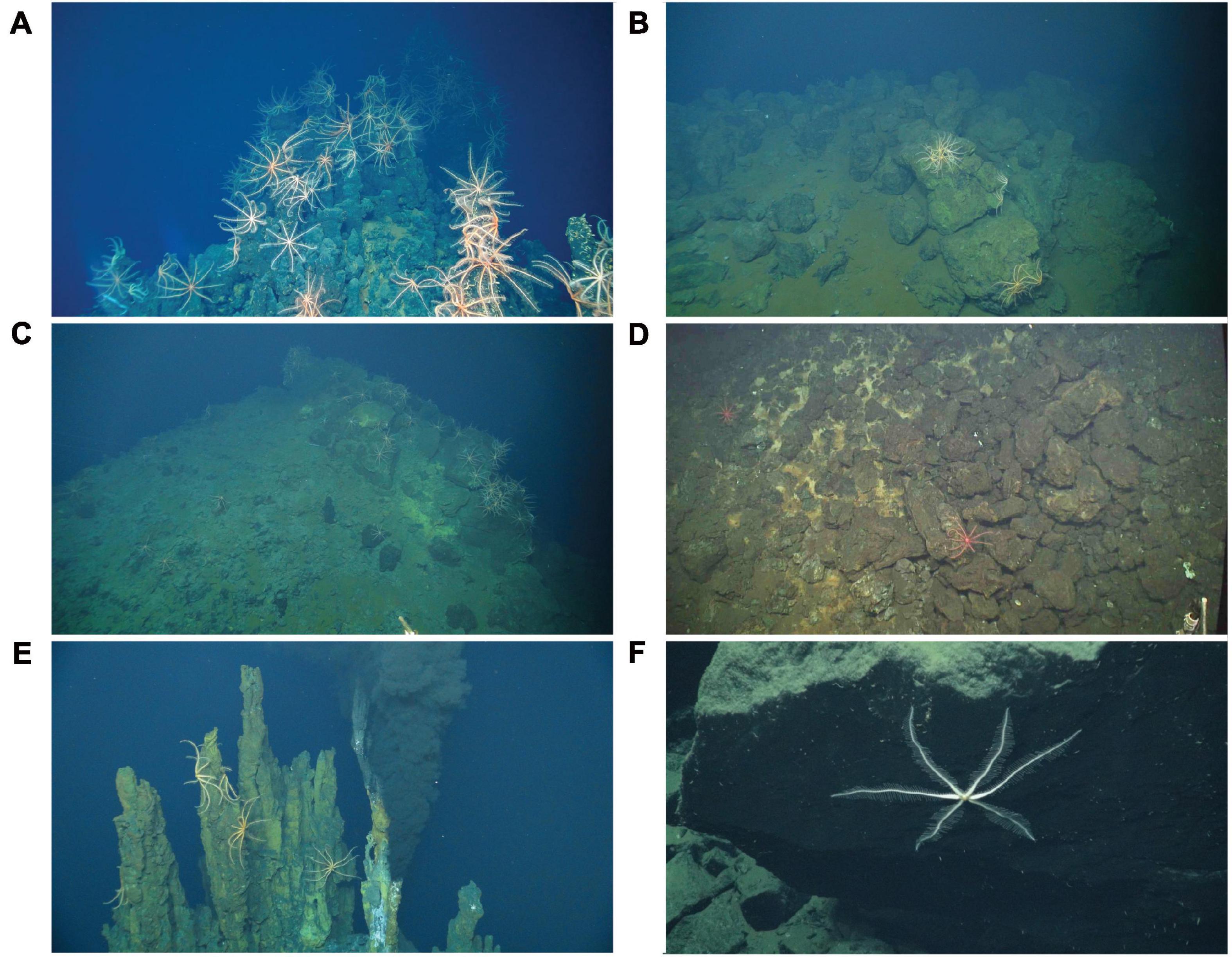
Figure 3. Examples of hydrothermally inactive (relict) sulfide substrata at Pito Seamount: (A) chimney; (B) boulders; (C) ledge; (D) debris; (E) example of brisingids on relict chimneys close to Scotty’s Castle black smoker; (F) six-armed brisingid on olivine gabbro talus at 5,200 m in Pito Deep.
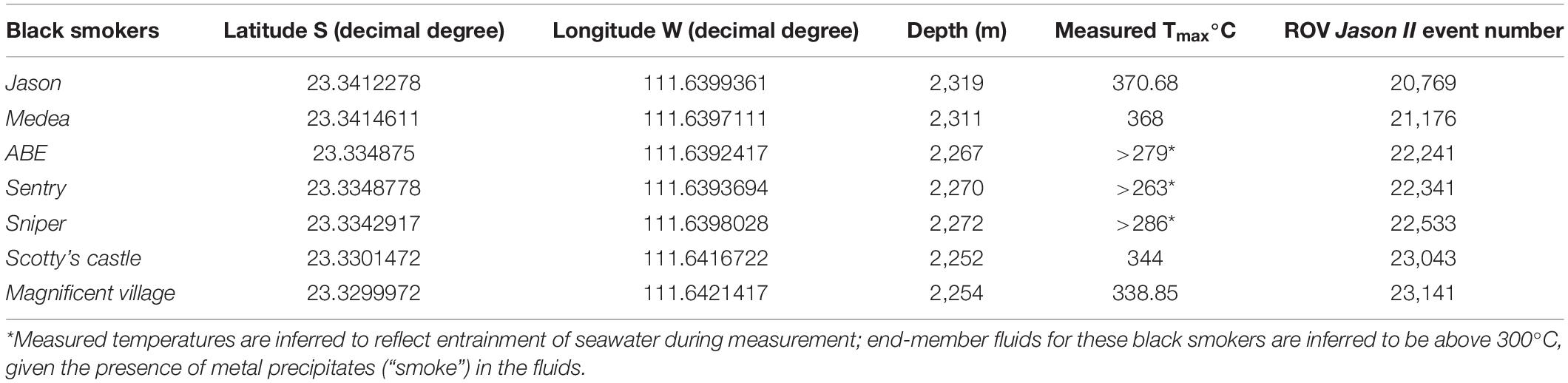
Table 1. Pito Seamount black smoker coordinates, depth, maximum temperature, and ROV Jason II event number.
To supplement observations of brisingid distributions on the Pito Seamount transect, Virtual Van SciCam thumbnail images from ROV Jason II Dives 953 through 960 from the walls of nearby Pito Deep (max depth of 5,260 m; PMaG cruise; 22.97°S, 111.95°W; Figure 1) were reviewed. Pito Deep provides a cross section through rifted crust of the EPR, is dominated by hard rock substrata, and is devoid of hydrothermal activity and sulfide features.
Data Analysis
Brisingid data analyses and visualizations were undertaken using R (R Core Team, 2020) and ggplot2 (Wickham, 2016). To test for significant differences among brisingid median abundances and substratum types, a Kruskal-Wallis rank sum test followed by a Dunn post-hoc test was undertaken using “rcompanion” (Mangiafico, 2016).
Results
Pito Seamount: Transect Effort and Brisingid Seastar Distributions
At Pito Seamount, the seabed was visible in 2,773 photos collected during a 15.6-h, non-linear transect that covered ∼3,700 m. Depth varied by < 100 m, from 2,240 to 2,325 m. Seven black smoker complexes (Figure 4) plus ∼57 discrete hydrothermally inactive, relict sulfide chimneys (ranging in height from 0.15 to ∼15 m) were visited during the transect. About a third of the photos (963) included black smokers, another third (886) were of basalt substrata, and the remainder (924) were of hydrothermally inactive sulfides (Table 2). Distributions of substratum types among photos, while indicative, are not quantitative measures of sampling effort given the limitations of the survey strategy as described in section “Materials and Methods.”
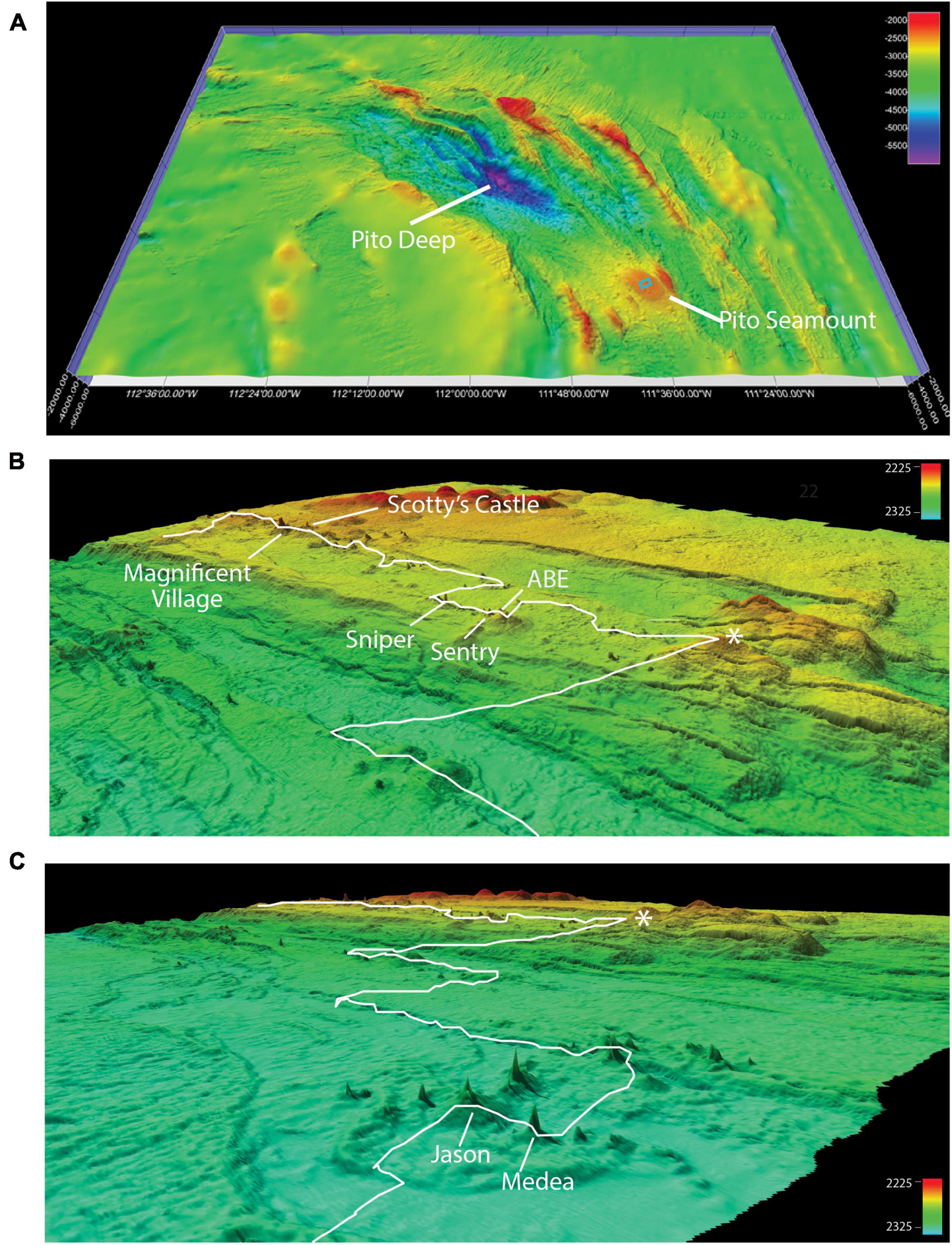
Figure 4. Geomorphological context of the ROV Jason II Dive 961 transect. (A) Ship multibeam bathymetry (60-m resolution) from R/V Atlantis cruise 11–33 (2005) infilled with satellite-derived bathymetry to show the location of Pito Seamount and the study area detailed in (B,C) (blue rectangle) relative to Pito Deep. (B,C) Overlapping Fledermaus-rendered perspectives, Pito Seamount study site. Approximate location of ROV Jason II Dive 961 transect (white line) is overlain on high-resolution bathymetry (0.5–1 m resolution) collected during AUV Sentry Dive 427 at the summit of Pito Seamount. Asterisks in B and C mark the same geographical locale. Active hydrothermal vents visited during the transect are labeled (see also Table 1). All images produced in Fledermaus v 7.7.4.
Of the photos collected, 301 (∼10%; excluding overlapping images) included one or more 9-armed, orange or white/cream-colored (Figure 2A) brisingid seastars (Supplementary Table 1). The total number of brisingids recorded was 4,005 individuals. Apart from an occasional gorgonian whip coral (n = ∼11 total), two seastars (2 different species), and invertebrate taxa associated with black smoker complexes, other benthic megafauna were inconspicuous along the transect.
Brisingid seastars were observed at intervals along the entire ROV Jason II Dive 961 transect, occurring occasionally as solitary individuals and most often in aggregations (Figures 2, 3). Most brisingids (∼90%) occurred on relict sulfide substrata (Table 2 and Figure 5). Highest abundances of brisingids on relict sulfide substrata were observed near the Sniper, Sentry, and ABE black smokers and on relict sulfide chimneys 120 m to the NE of the Jason and Medea vents (Figure 6). Highest abundances of brisingids on basalt were observed near the Jason and Medea black smokers (Figure 6). There was a single example of brisingids on hydrothermally inactive chimneys within just a few to tens of centimeters of a black smoker at Scotty’s Castle (Figure 3E). Eight relict sulfide chimneys (of 57; < 15%) were devoid of brisingids. Two chimney-scale structures were also devoid of brisingids, but the photos were not of sufficient quality to distinguish whether the substratum was basalt or sulfide. In addition, one basalt pillar was observed, also devoid of brisingid seastars.
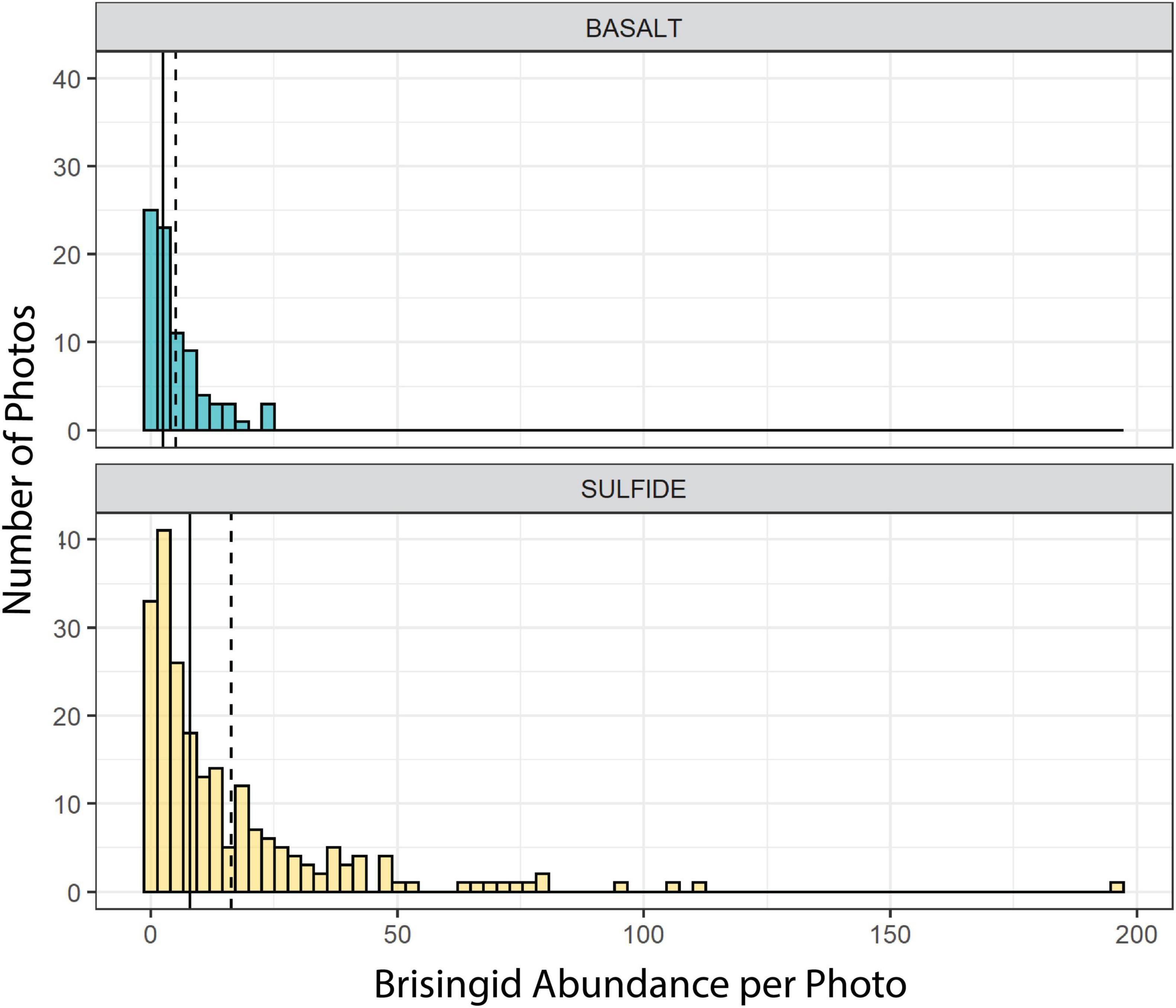
Figure 5. Frequency distributions of brisingid abundances per photo on basalt and hydrothermally inactive sulfide substrata. Solid lines: median; dashed lines: mean.
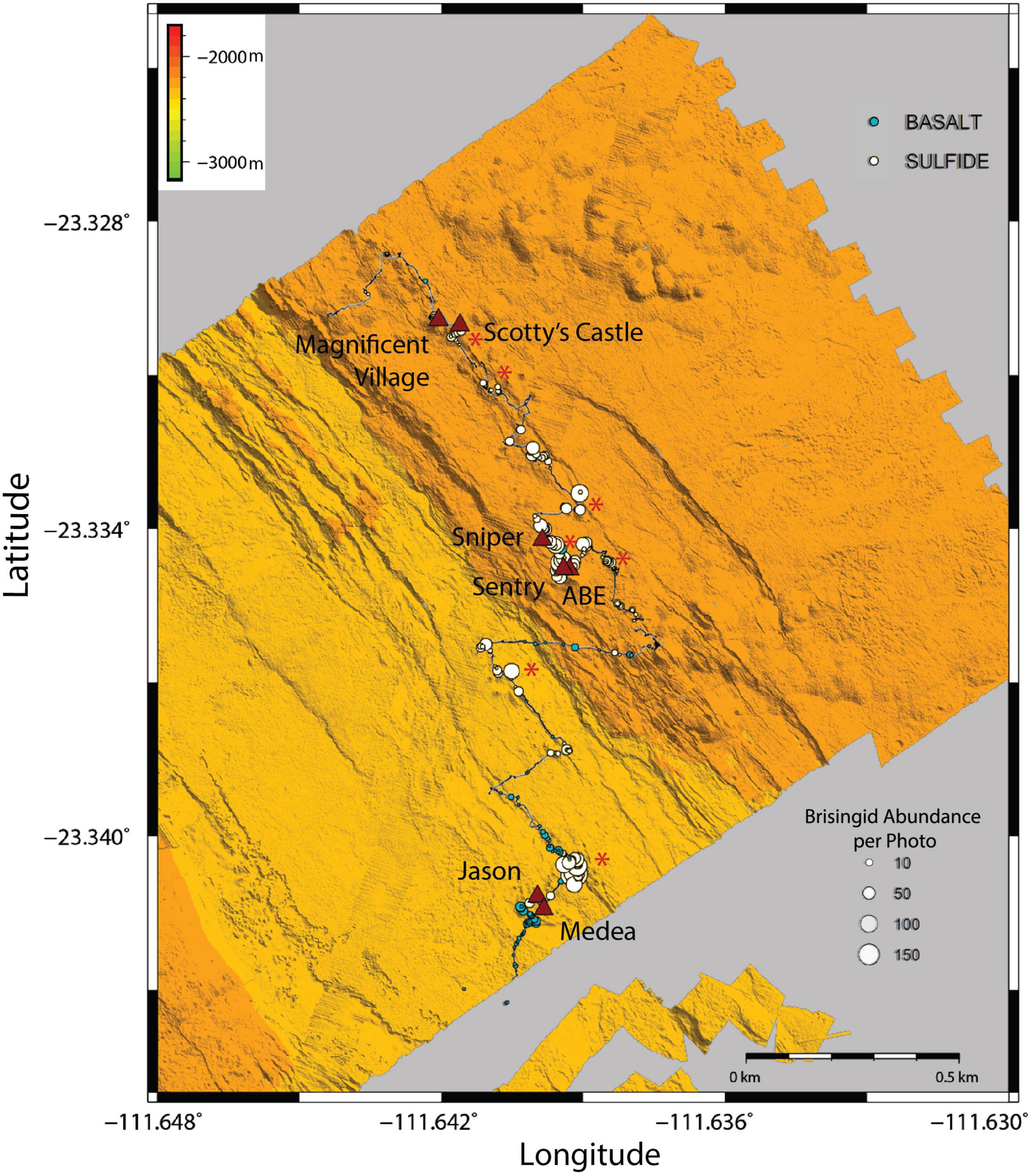
Figure 6. ROV Jason II Dive 961 navigation track overlain with brisingid abundance (per photo) bubble plots for basalt and hydrothermally inactive sulfide substrata. Labeled triangles indicate locations of active black smokers; asterisks indicate aggregations of relict sulfide chimneys.
Median brisingid abundances were greatest on sulfide substrata classified as relict sulfide chimneys (Table 2 and Figure 7; see also Supplementary Figure 1). Relict sulfide chimneys were also the substratum type that had the greatest variability in brisingid abundance per photo and the greatest number of outlier abundances in boxplots (i.e., lying more than 1.5× the length of the box from either end). One image included nearly 200 brisingids (estimated maximum density on the order of 300 individuals per m2). Brisingids were scarce on low-relief basalt substrata (Figure 7) and absent on black-smoker chimneys. Brisingid abundances for the sulfide substratum type “chimney” and the basalt substratum type “hackly” differed significantly from those of all other basalt and sulfide substratum types [Kruskal-Wallis rank sum test; chi-squared (H) = 45.1, d.f. = 10, p < 0.001, followed by a Dunn test (p < 0.001); Figure 7].
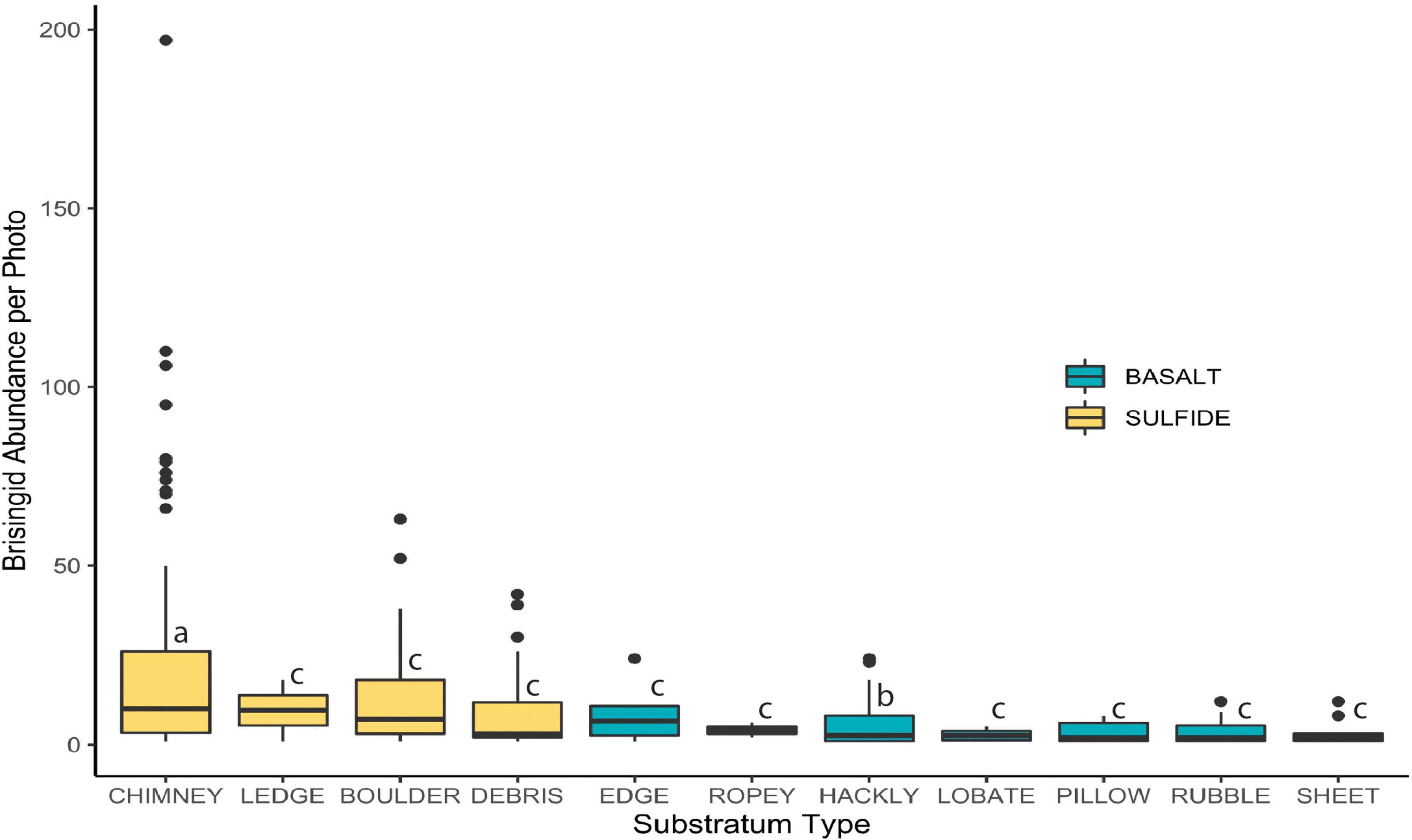
Figure 7. Brisingid abundance per photo on sulfide and basalt, ordered by median values. Horizontal line: median; filled area: 25th through 75th percentiles; whiskers: maximum and minimum values; symbols: outliers (i.e., more than 1.5 × the length of the box from either end). Letters indicate significant differences (p ≤ 0.001).
Pito Deep: Transect Effort and Brisingid Seastar Distributions
During nine ROV Jason II dives in the Pito Deep locale, 20,544 images were collected during a total of 362 h, covering ∼22 km along the seafloor (transects not illustrated). Solitary brisingid seastars were observed in 21 of these photos (0.001%), from depths of 3,680 to 5,260 m (Supplementary Table 2). Pito Deep brisingids were 6-armed (Figure 3F), distinct from those of Pito Seamount, and inferred here to be a different species.
Discussion
Despite decades of scientific study on the EPR, surprisingly little is known about the distribution of benthic invertebrates (abundance, composition, functional traits, etc.) relative to black smokers and relict sulfide occurrences. This study is, to our knowledge, the only mapping of non-vent-endemic invertebrate distributions relative to substratum types in an EPR-like setting. Analytical limitations encountered during the opportunistic study described here (e.g., challenges in quantifying sampling effort) point to the need of “fit-for-purpose” surveys and attention to sampling design.
Less surprising is the finding that relict sulfides in the Nautile Hydrothermal Field were not colonized by large, long-lived (decades and more) corals and sponges. This contrasts with observations of relict sulfides covered by relatively dense and/or distinct assemblages of attached and sessile invertebrates, including those reported from Manus Basin back arc spreading centers (Erickson et al., 2009), New Zealand Seamounts (Boschen et al., 2015, 2016), and the Central Indian Ridge (Gerdes et al., 2019). While we do not know the age of the relict sulfides observed in this study, they may be too young (decadal or less) to have accumulated long-lived taxa, but other explanations can be imagined. Alternative (and non-exclusive) explanations for the absence of large, attached organisms include differences in predominant sulfide mineralogies and substratum instability or other geochemical conditions that render the substratum unsuitable for establishment and persistence of coral, sponge, or other attached, long-lived taxa.
Suspension-feeding brisingid seastars on Pito Seamount were the visually dominant organisms on hydrothermally inactive sulfide substrata in this study. They were most abundant on relict sulfide chimneys elevated above the seafloor by 10 s of cm to ∼15 meters or more and within meters to 100’s of meters of black smokers. Of the basalt substrata, the frequency of occurrence of brisingids was slightly elevated on hackly basalt adjacent to black smokers. Anecdotally, relief alone is not sufficient to attract brisingids, given their absence on a meters-tall basalt pillar captured in the photos. The absence of brisingids on black smokers at Pito Seamount suggests that hydrothermal fluids emanating from sulfide surfaces create a thermally and/or chemically noxious habitat for this taxon. Brisingid seastars (likely a different species from those on Pito Seamount) were scarce in Pito Deep, where there was no hydrothermal activity and where food delivery may be diminished due to the greater depth. Future assessments of controls on brisingid distributions would benefit from surveys of basalt substrata at Pito Seamount (i.e., conspecifics at similar depths) but further removed from hydrothermal activity.
Aggregations of brisingids on hydrothermally relict chimneys some distance (up to 300 m) away from black smokers suggests that additional factors other than proximity to hydrothermal activity may contribute to their observed distribution. These include (i) microtopographically modified currents that deliver enhanced particulate organic material (POM) from chemosynthetic activity within the hydrothermal field and from surface-derived photosynthetic activity to suspension feeders on relict sulfide chimneys (Tunnicliffe et al., 1986; Erickson et al., 2009; Washburn et al., 2019); (ii) diffuse low-temperature and low-toxicity fluid flux that may persist in what might visually appear to be “relict” sulfide chimneys and that supports primary production by free-living microorganisms, which in turn serves as food for brisingids (Van Dover, 2019); (iii) autotrophic production based on oxidation of sulfide minerals (rather than dissolved sulfide or other reduced compounds in hydrothermal fluids) that serves as a food source for brisingid seastars occupying relict chimneys (Kato et al., 2010; Li et al., 2017); and (iv) microbes associated with undetected areas of diffuse, low-temperature flow through faults and cracks in the basalt that contribute to chemoautotrophic food resources for brisingids.
Currents that deliver suspended particles are known to be modified in areas of microtopographic (meters) relief, with acceleration zones over and between structures and eddy dissipation in the wake of structures (Hench and Rosman, 2013). The observed higher abundances and densities of brisingids on relict chimneys proximal to black smokers is consistent with the hypothesis that brisingids benefit from such modified currents and from enhanced delivery of organic material produced at and exported from nearby areas of hydrothermal activity. Stable isotope analyses of suspension-feeding invertebrates in areas peripheral to active vents in Manus Basin (Erickson et al., 2009) provide indirect support of this food-enrichment and delivery hypothesis. However, where gradual mineralization leads to clogging and cessation of high-temperature fluid flux in a black smoker chimney, diffuse flow may continue to be emitted. Without sensitive temperature or fluid flux measures, we cannot state with certainty that relict sulfide chimneys on Pito Seamount lacked a diffuse flow component of hydrothermal activity, below what would be apparent from shimmer in the real-time video as our imagery was collected. Nor can we be certain there were no areas of diffuse flow through fissures or cracks in basalt outside the field of view of the ROV cameras. We also can offer no evidence that the sulfide substratum itself is essential to maintaining dense aggregations of brisingids, although chemoautotrophic microbial production based on oxidation of sulfide minerals of relict sulfide chimneys is well documented (Van Dover, 2019; Orcutt et al., 2020; Van Dover et al., 2020). The abundance and activity of microbial communities at relict sulfides may even be comparable to or greater than that of microbial communities on black smoker chimneys (Edwards et al., 2003; Kato et al., 2010; Li et al., 2017), but whether these microbes are available to suspension feeders remains to be determined.
A nutritional connection between brisingid abundance and chemosynthetic productivity exported from active hydrothermal vents on Pito Seamount and elsewhere on the EPR thus remains conjectural in the absence of direct evidence. Future studies may gather systematic data to refine the spatial “sphere of influence” (Levin et al., 2016) of hydrothermal activity and brisingid distributions. Such efforts might include undertaking more detailed characterization of the hydrothermal status of “relict” chimneys and using a combination of abundance data and stable isotope compositions of megafaunal tissues along transects approaching zones of hydrothermal activity to estimate the relative importance of photosynthetically and chemosynthetically derived organic material.
Reproductive benefits of aggregative behaviors may also underlie brisingid distributions near hydrothermal vents. While brisingids are sedentary, they are capable of some movement over the substratum. One species was observed to shift position by ∼1 cm in 10 min, presumably in response to shifting currents (Edwards et al., 2016). The extent to which dense aggregations of brisingids contribute to reproductive success of the species is unknown, but high densities may be important in the maintenance of local populations. Where eggs and sperm of a species are broadcast into the water column, as indicated for brisingid seastars, aggregation of adults that exploit enriched sources of food could mitigate reproductive Allee effects, where fertilization success is limited among more solitary individuals (Gascoigne and Lipcius, 2004).
While it seems unlikely that relict sulfide occurrences such as those observed at Pito Seamount will be the target of deep-sea mining activities, they do appear to be an important habitat for brisingid seastars. If conservation measures should be merited to conserve biodiversity in this region, area-based management interventions could be used to protect representative aggregations of brisingids (Boschen et al., 2016; Van Dover, 2019; Boschen-Rose et al., 2021). While it can be challenging to suggest effective mitigation or restoration actions in the deep sea (Van Dover et al., 2014; Da Ros et al., 2019), this study suggests that construction of “artificial” microtopographic features within 10–100’s of meters of hydrothermal activity might passively attract mobile taxa and/or new recruits, or might be repopulated through transplant of individuals from sites targeted for disturbance. Such an approach might be considered for aggregated taxa associated with relict sulfides elsewhere, as, for example, corals and urchins found on hydrothermally inactive sulfide occurrences associated with New Zealand seamounts (Boschen et al., 2015, 2016). Further research would be merited, however, to determine the design and efficacy of such artificial structures as mitigation interventions in any given region.
Conclusion
Suspension-feeding brisingid seastars were the visually dominant megafaunal invertebrate observed along the ROV Jason II Dive 961 transect at Pito Seamount and were most abundant on microtopographic relief provided by relict sulfide chimneys near black smokers. The sphere of influence of vent productivity on brisingid abundance may extend > 300 m from black smokers. Together, relict sulfide chimneys and proximity to black smokers likely play key roles in the maintenance of brisingid population density on Pito Seamount, though there may be other contributing factors. Experimental introduction of “artificial” microtopographic relief could test whether such an approach might mitigate potential impacts of mineral extraction on populations of suspension-feeding invertebrates.
Data Availability Statement
The original contributions presented in the study are included in the article/Supplementary Material, further inquiries can be directed to the corresponding author/s.
Author Contributions
DW: image analysis, statistics, visualizations, and writing (original draft, review, and edit). MC, BJ, and CG: resources and writing (visualization, review, and edit). CV: conceptualization, funding acquisition, supervision, methodology, statistics, visualization, and writing (original draft, review, and edit). All authors contributed to the article and approved the submitted version.
Funding
This project was partially supported by the Global Ocean Biodiversity Initiative through the International Climate Initiative (IKI; grant no. 16_IV_049_Global_A_Global Ocean Biodiversity Initiative GOBI). The Federal Ministry for the Environment, Nature Conservation, and Nuclear Safety (BMU) supports IKI on the basis of a decision adopted by the German Bundestag. DW was supported by Duke University funds to CV. CG’s participation was funded through WHOI’s Deep Ocean Exploration Institute. The AT37-08 cruise was funded by NSF OCE-1459462 (MC and BJ) and OCE-1459387 (J Gee, Scripps Institution of Oceanography).
Conflict of Interest
The authors declare that the research was conducted in the absence of any commercial or financial relationships that could be construed as a potential conflict of interest.
The reviewer RB-R declared a past co-authorship with one of the authors CV to the handling editor.
Publisher’s Note
All claims expressed in this article are solely those of the authors and do not necessarily represent those of their affiliated organizations, or those of the publisher, the editors and the reviewers. Any product that may be evaluated in this article, or claim that may be made by its manufacturer, is not guaranteed or endorsed by the publisher.
Acknowledgments
We thank the Captain and crew of the R/V Atlantis, the ROV Jason II and AUV Sentry teams, as well as Jeff Gee (Scripps Institution of Oceanography) and the Pito Deep 2017 Scientific Party for their efforts collecting images used in this study. We also value the preliminary work of students in the 2018 Deep-Sea Science and Environmental Management course at Duke University: A. Alberini, K. Johnson-Sapp, S. Poulin, K. Kochvar, E. Olszewski, L. Breitkreutz, E. Darnell, and N. Jacobson. The manuscript benefitted from reviewers’ constructive comments.
Supplementary Material
The Supplementary Material for this article can be found online at: https://www.frontiersin.org/articles/10.3389/fmars.2022.774628/full#supplementary-material
Supplementary Figure 1 | Frequency distributions of brisingid abundances per photo on basalt and sulfide substrata.
Supplementary Table 1 | Brisingid abundances and substratum types, Pito Seamount.
Supplementary Table 2 | Brisingid abundances, Pito Deep.
Footnotes
References
Boschen, R. E., Rowden, A. A., Clark, M. R., Pallentin, A., and Gardner, J. P. A. (2016). Seafloor massive sulfide deposits support unique megafaunal assemblages: implications for seabed mining and conservation. Mar. Environ. Res. 115, 78–88. doi: 10.1016/j.marenvres.2016.02.005
Boschen, R., Rowden, A., Clark, M., Barton, S., Pallentin, A., and Gardner, J. (2015). Megabenthic assemblage structure on three New Zealand seamounts: implications for seafloor massive sulfide mining. Mar. Ecol. Prog. Ser. 523, 1–14. doi: 10.3354/meps11239
Boschen-Rose, R. E., Clark, M. R., Rowden, A. A., and Gardner, J. P. A. (2021). Assessing the ecological risk to deep-sea megafaunal assemblages from seafloor massive sulfide mining using a functional traits sensitivity approach. Ocean Coast. Manag. 210:105656. doi: 10.1016/j.ocecoaman.2021.105656
Carney, R. S. (2010). Stable isotope trophic patterns in echinoderm megafauna in close proximity to and remote from Gulf of Mexico lower slope hydrocarbon seeps. Deep Sea Res. Part II Top. Stud. Oceanogr. 57, 1965–1971. doi: 10.1016/j.dsr2.2010.09.027
Cheadle, M., John, B., German, C., Gee, J., Coogan, L., Gillis, K., et al. (2017). Pito Seamount revisited: the discovery and mapping of new black smoker vents. AGU Fall Meet. Abstr. 2017, 51D–0388D.
Chen, S., Tao, C., and German, C. R. (2021). Abundance of low-temperature axial venting at the equatorial East Pacific Rise. Deep. Res. Part I Oceanogr. Res. Pap. 167:103426. doi: 10.1016/j.dsr.2020.103426
Cherkashov, G. (2017). “Seafloor massive sulfide deposits: distribution and prospecting,” in Deep-Sea Mining, ed. R. Sharma (Berlin: Springer International Publishing), 143–164. doi: 10.1007/978-3-319-52557-0
Da Ros, Z., Dell’Anno, A., Morato, T., Sweetman, A. K., Carreiro-Silva, M., Smith, C. J., et al. (2019). The deep sea: the new frontier for ecological restoration. Mar. Policy 108:103642. doi: 10.1016/j.marpol.2019.103642
Desbruyeres, D., Segonzac, M., and Bright, M. (2006). Handbook of deep-sea hydrothermal vent fauna. Denisia 18, 1–434.
Edwards, K. J., Bach, W., and Rogers, D. R. (2003). Geomicrobiology of the ocean crust: a role for chemoautotrophic Fe-bacteria. Biol. Bull. 204, 180–185. doi: 10.2307/1543555
Edwards, M. H., Fornari, D. J., Rognstad, M. R., Kelley, C. D., Mah, C. L., Davis, L. K., et al. (2016). Time-lapse camera studies of sea-disposed chemical munitions in Hawaii. Deep. Res. Part II Top. Stud. Oceanogr. 128, 25–33. doi: 10.1016/j.dsr2.2015.03.003
Emson, R. H., and Young, C. M. (1994). Feeding mechanism of the brisingid starfish Novodinia antillensis. Mar. Biol. 118, 433–442. doi: 10.1007/BF00350300
Erickson, K. L., Macko, S. A., and Van Dover, C. L. (2009). Evidence for a chemoautotrophically based food web at inactive hydrothermal vents (Manus Basin). Deep Sea Res. Part II Top. Stud. Oceanogr. 56, 1577–1585. doi: 10.1016/j.dsr2.2009.05.002
Fouquet, Y., Knott, R., Cambon, P., Fallick, A., Rickard, D., and Desbruyeres, D. (1996). Formation of large sulfide mineral deposits along fast spreading ridges. Example from off-axial deposits at 12°43’N on the East Pacific Rise. Earth Planet. Sci. Lett. 144, 147–162. doi: 10.1016/0012-821x(96)00142-2
Fustec, A., Desbruyères, D., Juniper, S. K., Fustec, A., and Desbruyeres, D. (2013). Deep-sea hydrothermal vent communities at 13°N on the East Pacific Rise: microdistribution and temporal variations. Biol. Oceanogr. 4, 121–164. doi: 10.1080/01965581.1987.10749487
Gale, K. S. P., Hamel, J. F., and Mercier, A. (2013). Trophic ecology of deep-sea Asteroidea (Echinodermata) from eastern Canada. Deep. Res. Part I Oceanogr. Res. Pap. 80, 25–36. doi: 10.1016/j.dsr.2013.05.016
Gascoigne, J., and Lipcius, R. N. (2004). Allee effects in marine systems. Mar. Ecol. Prog. Ser. 269, 49–59. doi: 10.3354/meps269049
Gerdes, K. H., Martínez Arbizu, P., Schwentner, M., Freitag, R., Schwarz-Schampera, U., Brandt, A., et al. (2019). Megabenthic assemblages at the southern Central Indian Ridge – Spatial segregation of inactive hydrothermal vents from active-, periphery- and non-vent sites. Mar. Environ. Res. 151:104776. doi: 10.1016/j.marenvres.2019.104776
German, C. R., Legendre, L. L., Sander, S. G., Niquil, N., Luther, G. W., Bharati, L., et al. (2015). Hydrothermal Fe cycling and deep ocean organic carbon scavenging: model-based evidence for significant POC supply to seafloor sediments. Earth Planet. Sci. Lett. 419, 143–153. doi: 10.1016/j.epsl.2015.03.012
Gollner, S., Govenar, B., Arbizu, P. M., Mullineaux, L. S., Mills, S., Bris, N. L., et al. (2020). Animal community dynamics at senescent and active vents at the 9° N East Pacific Rise after a volcanic eruption. Front. Mar. Sci. 6:832. doi: 10.3389/fmars.2019.00832
Halbach, P., Blum, N., Münch, U., Plüger, W., Garbe-Schönberg, D., and Zimmer, M. (1998). Formation and decay of a modern massive sulfide deposit in the Indian Ocean. Miner. Depos. 33, 302–309. doi: 10.1007/s001260050149
Hannington, M., Jamieson, J., Monecke, T., Petersen, S., and Beaulieu, S. (2011). The abundance of seafloor massive sulfide deposits. Geology 39, 1155–1158. doi: 10.1130/G32468.1
Hekinian, R., and Fouquet, Y. (1985). Volcanism and metallogenesis of axial and off-axial structures on the East Pacific Rise near 13N. Econ. Geol. 80, 221–249.
Hench, J. L., and Rosman, J. H. (2013). Observations of spatial flow patterns at the coral colony scale on a shallow reef flat. J. Geophys. Res. Ocean. 118, 1142–1156. doi: 10.1002/jgrc.20105
Hoagland, P., Beaulieu, S., Tivey, M. A., Eggert, R. G., German, C., Glowka, L., et al. (2010). Deep-sea mining of seafloor massive sulfides. Mar. Policy 34, 728–732. doi: 10.1016/j.marpol.2009.12.001
Howell, K., Pond, D., Billett, D., and Tyler, P. (2003). Feeding ecology of deep-sea seastars (Echinodermata: Asteroidea): a fatty-acid biomarker approach. Mar. Ecol. Prog. Ser. 255, 193–206. doi: 10.3354/meps255193
Jamieson, J., and Gartman, A. (2020). Defining active, inactive, and extinct seafloor massive sulfide deposits. Mar. Policy 117:103926. doi: 10.1016/j.marpol.2020.103926
Karson, J., Kelly, D., Fornari, D., Perfit, M., and Shank, T. (2015). Discovering the Deep: A Photographic Atlas of the Seafloor and Ocean Crust. Cambridge: Cambridge University Press.
Kato, S., Takano, Y., Kakegawa, T., Oba, H., Inoue, K., Kobayashi, C., et al. (2010). Biogeography and biodiversity in sulfide structures of active and inactive vents at deep-sea hydrothermal fields of the Southern Mariana Trough. Appl. Environ. Microbiol. 76, 2968–2979. doi: 10.1128/AEM.00478-10
Kim, S., and Hammerstrom, K. (2012). Hydrothermal vent community zonation along environmental gradients at the Lau back-arc spreading center. Deep. Res. Part I Oceanogr. Res. Pap. 62, 10–19. doi: 10.1016/j.dsr.2011.12.010
Lacharité, M., and Metaxas, A. (2017). Hard substrate in the deep ocean: how sediment features influence epibenthic megafauna on the eastern Canadian margin. Deep. Res. Part I Oceanogr. Res. Pap. 126, 50–61. doi: 10.1016/j.dsr.2017.05.013
Lalou, C., and Brichet, E. (1982). Ages and implications of East Pacific Rise sulphide deposits at 21 °N. Nature 300, 169–171. doi: 10.1038/300169a0
Lalou, C., Brichet, E., and Hekinian, R. (1985). Age dating of sulfide deposits from axial and off-axial structures on the East Pacific Rise near 12°50’N. Earth Planet. Sci. Lett. 75, 59–71. doi: 10.1016/0012-821X(85)90050-0
Lalou, C., Reyss, J.-L., Brichet, E., Rona, P. A., and Thompson, G. (1995). Hydrothermal activity on a 10^5-year scale at a slow-spreading ridge, TAG hydrothermal field, Mid-Atlantic Ridge 26oN. J. Geophys. Res. 100, 17855–17862.
Levin, L. A., Baco, A. R., Bowden, D. A., Colaco, A., Cordes, E. E., Cunha, M. R., et al. (2016). Hydrothermal vents and methane seeps: rethinking the sphere of influence. Front. Mar. Sci. 3:72. doi: 10.3389/fmars.2016.00072
Li, J., Cui, J., Yang, Q., Cui, G., Wei, B., Wu, Z., et al. (2017). Oxidative weathering and microbial diversity of an inactive seafloor hydrothermal sulfide chimney. Front. Microbiol. 8:1378. doi: 10.3389/fmicb.2017.01378
Mah, C. L. (2016). A new species of Brisingenes from the Hawaii undersea military munitions assessment area with an overview of Hawaiian brisingid in situ video observations and functional morphology of subambulacral spines (Forcipulatacea; Asteroidea). Deep. Res. Part II Top. Stud. Oceanogr. 128, 43–52. doi: 10.1016/j.dsr2.2014.06.003
Mah, C., Linse, K., Copley, J., Marsh, L., Rogers, A., Clague, D., et al. (2015). Description of a new family, new genus, and two new species of deep-sea Forcipulatacea (Asteroidea), including the first known sea star from hydrothermal vent habitats. Zool. J. Linn. Soc. 174, 93–113. doi: 10.1111/zoj.12229
Mordecai, G., Tyler, P. A., Masson, D. G., and Huvenne, V. A. I. (2011). Litter in submarine canyons off the west coast of Portugal. Deep. Res. Part II Top. Stud. Oceanogr. 58, 2489–2496. doi: 10.1016/j.dsr2.2011.08.009
Naar, D., Hekinian, R., Segonzac, M., and Francheteau, J., The Pito Dive Team. (2004). “Vigorous venting and biology at Pito Seamount, Easter Microplate,” in Mid-Ocean Ridges: Hydrothermal Interactions Between the Lithosphere and Ocean, eds C. German, J. Lin, and L. M. Parson (Washington, DC: American Geophysical Union), 305–318. doi: 10.1029/148gm13
Orcutt, B. N., Bradley, J. A., Brazelton, W. J., Estes, E. R., Goordial, J. M., Huber, J. A., et al. (2020). Impacts of deep-sea mining on microbial ecosystem services. Limnol. Oceanogr. 65, 1489–1510. doi: 10.1101/463992
Petersen, S., Krätschell, A., Augustin, N., Jamieson, J., Hein, J. R., and Hannington, M. D. (2016). News from the seabed – Geological characteristics and resource potential of deep-sea mineral resources. Mar. Policy 70, 175–187. doi: 10.1016/j.marpol.2016.03.012
Rona, P., Hannington, M. D., Tivey, K., and Lalou, C. (1993). Active and relict sea-floor hydrothermal mineralization at the TAG hydrothermal field, Mid-Atlantic Ridge. Econ. Geol. 88, 1989–2017.
Tunnicliffe, V., Botros, M., De Burgh, M. E., Dinet, A., Johnson, H. P., Juniper, S. K., et al. (1986). Hydrothermal vents of explorer ridge, northeast Pacific. Deep Sea Res. Part A Oceanogr. Res. Pap. 33, 401–412. doi: 10.1016/0198-0149(86)90100-7
Van Dover, C. L. (2000). The Ecology of Deep-Sea Hydrothermal Vents. Princeton, NJ: Princeton University Press, doi: 10.2307/177518
Van Dover, C. L. (2019). Inactive sulfide ecosystems in the deep sea: a review. Front. Mar. Sci. 6:461. doi: 10.3389/fmars.2019.00461
Van Dover, C. L., Aronson, J., Pendleton, L., Smith, S., Arnaud-Haond, S., Moreno-Mateos, D., et al. (2014). Ecological restoration in the deep sea: desiderata. Mar. Policy 44, 98–106. doi: 10.1016/j.marpol.2013.07.006
Van Dover, C. L., Colaço, A., Collins, P. C., Croot, P., Metaxas, A., Murton, B. J., et al. (2020). Research is needed to inform environmental management of hydrothermally inactive and extinct polymetallic sulfide (PMS) deposits. Mar. Policy 121:104183. doi: 10.1016/j.marpol.2020.104183
Washburn, T. W., Turner, P. J., Durden, J. M., Jones, D. O. B., Weaver, P., and Van Dover, C. L. (2019). Ecological risk assessment for deep-sea mining. Ocean Coast. Manag. 176, 24–39. doi: 10.1016/j.ocecoaman.2019.04.014
Keywords: brisingid seastar, East Pacific Rise (EPR), hydrothermal vent, Pito Seamount, Nautile Hydrothermal Field, deep-sea mining (DSM), black smoker, hydrothermally inactive sulfide
Citation: Woods D, Cheadle MJ, John BE, German CR and Van Dover CL (2022) Making Use of Relicts: Brisingid Seastars Aggregate on Hydrothermally Inactive Sulfide Chimneys Near Black Smokers. Front. Mar. Sci. 9:774628. doi: 10.3389/fmars.2022.774628
Received: 12 September 2021; Accepted: 25 January 2022;
Published: 17 February 2022.
Edited by:
Philip Weaver, Seascape Consultants Ltd., United KingdomReviewed by:
Helena Passeri Lavrado, Federal University of Rio de Janeiro, BrazilRachel Elizabeth Boschen-Rose, Marine Scotland, United Kingdom
Marie-Anne Cambon, Institut Français de Recherche pour l’Exploitation de la Mer (IFREMER), France
Copyright © 2022 Woods, Cheadle, John, German and Van Dover. This is an open-access article distributed under the terms of the Creative Commons Attribution License (CC BY). The use, distribution or reproduction in other forums is permitted, provided the original author(s) and the copyright owner(s) are credited and that the original publication in this journal is cited, in accordance with accepted academic practice. No use, distribution or reproduction is permitted which does not comply with these terms.
*Correspondence: Cindy L. Van Dover, Y2x2M0BkdWtlLmVkdQ==