- 1Department of Marine and Coastal Environmental Science, Texas A&M University at Galveston, Galveston, TX, United States
- 2Okaloosa County, Board of County Commissioners, Destin-Fort Walton Beach, FL, United States
- 3National Oceanic and Atmospheric Administration, Flower Garden Banks National Marine Sanctuary, Galveston, TX, United States
- 4Fisheries Ecology and Ecosystem Research Lab, Department of Marine Biology, Texas A&M University at Galveston, Galveston, TX, United States
- 5Department of Ecology and Conservation Biology, Texas A&M University, College Station, TX, United States
Indo-Pacific lionfish (Pterois volitans and P. miles) are the first marine teleost to have successfully invaded and become established in the Western Atlantic Ocean of the United States, Gulf of Mexico (GoM), and Caribbean Sea. Pterois volitans were first reported in the Flower Garden Banks National Marine Sanctuary (FGBNMS), a protected coral reef system in the northwestern GoM, in 2011. Little is understood about the life history characteristics of lionfish in this ecosystem. This study assessed population characteristics (size, density, age and growth) and removal efforts of lionfish (n = 1,665) at two coral reef sites within FGBNMS for 2015, 2016, and 2018. The annual increment formation in sagittal otoliths was examined to assess the age and growth of lionfish collected in 2018 (n = 100). Lionfish ranged in size from 75 to 444 mm total length (TL) and 4–1,153 g in total weight (TW). Six hundred and ten fish were randomly dissected for sex determination (females = 256, males = 354), females ranged in size from 137 to 348 mm TL and 21–586 g, while males ranged from 118 to 444 mm TL and 18–1,153 g. Interannual variation in mean lionfish density ranged from 26.7 individuals per hectare (ind ha–1) in 2016 to 81.1 ind ha–1 in 2018, while removal effort significantly increased ranging from 1.92 to 5.42 kg diver h–1. Lionfish age ranged from 0 to 10 years, with a mean age of 3.9 years. The observed values of the asymptotic maximum total length (L∞) and Brody’s growth coefficient (K) were 345 mm and 0.30 for females and 415 mm and 0.18 for males. Results suggest lionfish from FGBNMS exhibit markedly lower mean densities, a lower L∞ and growth rate, but attain older ages than lionfish in the Caribbean Sea, Western Atlantic Ocean, and other ecoregions in the northern GoM. This study describes the first key life history parameters and removal efforts for lionfish in a protected, healthy coral reef system in the northwestern GoM that may provide insight into environmental population controls (e.g., ecological resilience). Metrics from this study could be integrated into mechanistic ecological models to determine if FGBNMS is in fact exhibiting natural resilience to the lionfish invasion.
Introduction
Indo-Pacific lionfish (Pterois volitans and P. miles, herein referred to as lionfish) are non-indigenous marine fish that were introduced in the mid-1980s along the Western Atlantic Coast of the United States (U.S.), most likely through release from aquaria (Semmens et al., 2004; Hunter et al., 2021). Their distribution has since expanded into the Caribbean Sea, throughout the Gulf of Mexico (GoM), and into the Southwest Atlantic Ocean along South America (Aguilar-Perera and Tuz-Sulub, 2010; Schofield, 2010; Ferreira et al., 2015; Campbell et al., 2022). More recently, lionfish have invaded the Mediterranean Sea (Azzurro et al., 2017; Bariche et al., 2017) via the Suez Canal. Lionfish appear to be thriving in the warm temperate, and subtropical, waters of the Western Atlantic Ocean, Caribbean Sea, and GoM, as their densities are an order of magnitude higher than observed in their native range in the Indo-Pacific (Grubich et al., 2009; Kulbicki et al., 2012; Elise et al., 2015). Several biological characteristics of lionfish have been well documented to explain their rapid expansion and sustained population levels in invaded ranges. Life history characteristics facilitating the population expansion of lionfish are rapid growth, early sexual maturity, and frequent spawning capabilities (Betancur-R et al., 2011; Cote et al., 2013a; Gardner et al., 2015; Fogg et al., 2017). Lionfish exhibit physiological tolerances to a breadth of habitat and environmental conditions (Kimball et al., 2004; Biggs and Olden, 2011; Nuttall et al., 2014; Jud et al., 2015) and are opportunistic generalist feeders (Peake et al., 2018). Finally, lionfish appear to be resistance to disease and parasites (Simmons, 2014; Sellers et al., 2015; Fogg et al., 2016; Montoya-Mendoza et al., 2017; Aguilar-Perera et al., 2018; Harris et al., 2020) and lack natural predators (Maljkovic et al., 2008; Ulman et al., 2021), rendering their populations virtually unchecked. Lionfish have the potential to alter marine communities by consuming herbivorous fishes responsible for controlling algal production (Hughes, 1994; Albins and Hixon, 2008; Barbour et al., 2011), competing for resources with native meso-predators (Raymond et al., 2015; Marshak et al., 2018), and reducing local reef-fish populations (Arias-Gonzalez et al., 2011; Green et al., 2012a; Albins, 2013; Cote et al., 2013b).
It is important to gather regional baseline demographic data to inform management of this invasive species. Population dynamics and age-specific life history parameters are fundamental to understanding factors affecting recruitment success and are essential inputs in population models to evaluate efficacy of fishery management policies (Campana, 1999; Mendoza, 2006; Pope et al., 2010; Kindsvater et al., 2016). These data become particularly important when non-native species establish in a new region, as this can inform management and assess the effects they have on native species and the environment they invaded. Changes in size, age structure, and sex ratio of fishes are common indicators of overfishing (Berkeley et al., 2004; Cope, 2013), but in the case of invasive species, may be used to determine the success of management strategies used to reduce their populations (e.g., targeted removals; Frazer et al., 2012) or whether natural control may be occurring (Harris et al., 2020).
The northwestern Gulf of Mexico (generally recognized as the area from the Texas-Mexico border to the state line between Louisiana and Mississippi; New World Encyclopedia, 2021) is marked by well-established coral communities and banks that encompass biologically and ecologically important features along the outer continental shelf (Schmahl et al., 2008). The Flower Garden Banks National Marine Sanctuary (FGBNMS) represents the northernmost coral reef system in the Gulf of Mexico (GoM) and was designated a national marine sanctuary by the Office of National Marine Sanctuaries, within the National Oceanic and Atmospheric Administration (NOAA), in 1992 (Federal Register, 1991). Originally comprised of three banks (East Flower Garden Bank (EFGB), West Flower Garden Bank (WFGB), and Stetson Bank), this marine sanctuary was expanded in 2021 to encompass 17 bank systems located 110–304 km offshore from Galveston, Texas (Federal Register, 2021). EFGB and WFGB are populated by a rugous, complex reef structure and are arguably some of the healthiest coral reefs in the Caribbean region, with 55–60% living scleractinian coral cover on the reef caps (16–40 m) that has remained stable or increased significantly over the last 30 years (Johnston et al., 2021). Lionfish were first observed at EFGB and WFGB in 2011 (Johnston et al., 2016b), though recent records estimate their invasion may have begun as early as 2008 (Blakeway et al., 2021). This study reports life history characteristics and removal efforts of lionfish collected from EFGB and WFGB across 3 years.
Lionfish density, weight-length relationship, age, and growth have been suggested to differ among invaded regions likely attributed to invasion chronology, environmental influences (e.g., resource availability and accessibility, and climate), and removal efforts (Barbour et al., 2011; Farquhar, 2016; Fogg, 2017). The aim of this study was to document key life history attributes of lionfish on a protected coral reef system (i.e., FGBNMS) in the northwestern GoM, and to determine if differences were evident for age at length and age structure composition among invaded ecosystems. In addition, catch per unit effort (CPUE) was estimated for each year as another mechanism to describe trends in the lionfish population.
Materials and Methods
Study Area
EFGB and WFGB are prominent geological features located near the edge of the continental shelf and were created by the uplift of underlying salt domes (Hickerson and Schmahl, 2005; Figure 1). The sanctuary boundaries of EFGB encompass approximately 72 km2, while WFGB comprises approximately 96.3 km2 (Figure 1). Well-developed scleractinian corals (e.g., Orbicella franksi, Psuedodiploria strigosa, and Porites astreoides) comprise 55 and 60% of the benthic cover at EFGB and WFGB, respectively, in water depths ranging from 16 to 40 m. On reef slopes, coral cover increases to as high as 94% at both banks, where the dominant species transition to O. franksi and Montastraea cavernosa (Johnston et al., 2018, 2021). Mooring buoys are present at EFGB (n = 7) and WFGB (n = 5), anchored to the seafloor with permanent stainless-steel U-bolts.
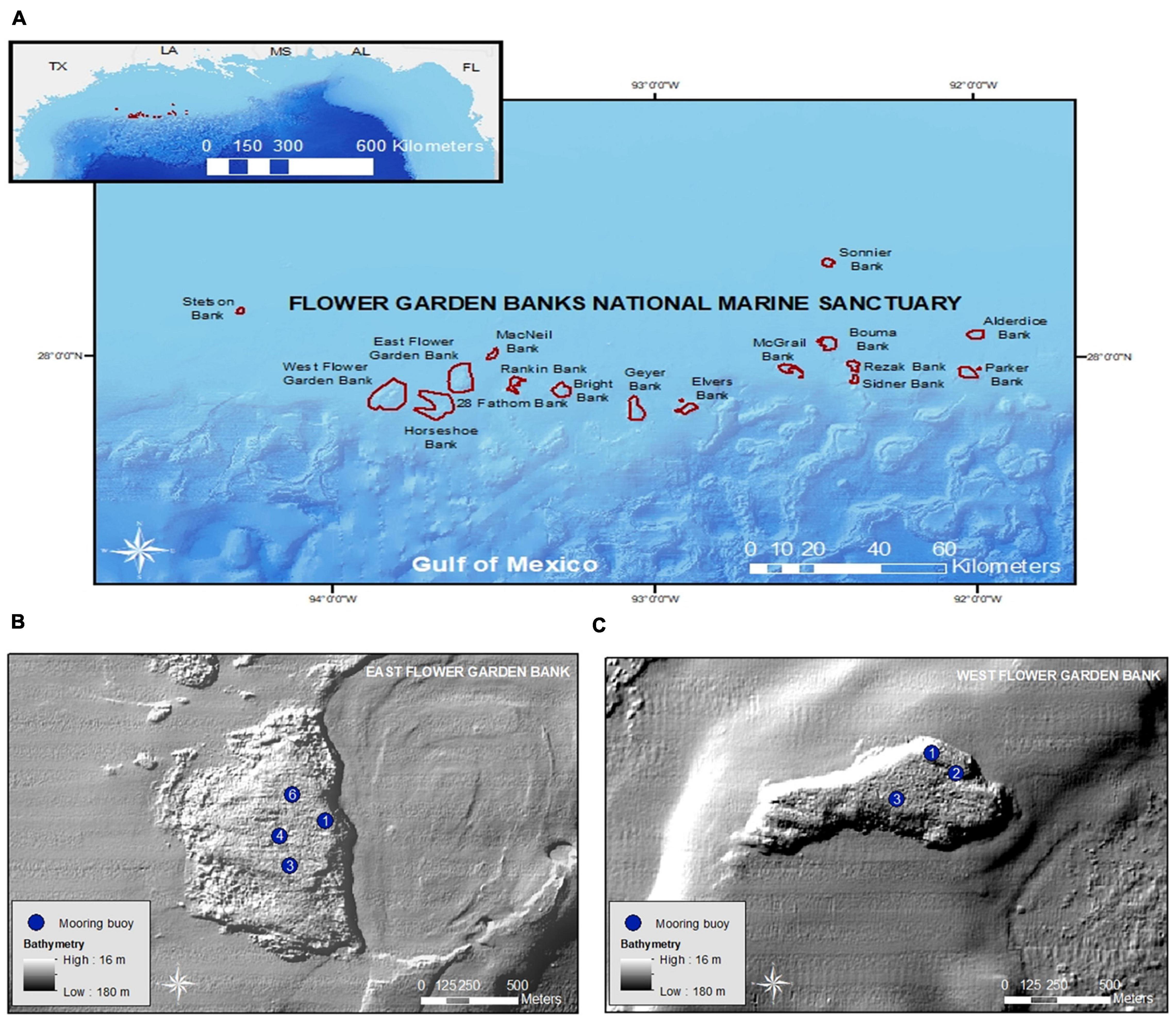
Figure 1. (A) Flower Garden Banks National Marine Sanctuary (FGBNMS) in the northwestern Gulf of Mexico (inset). (B) Buoyed sampling sites at East Flower Garden Bank (EFGB) and (C) West Flower Garden Bank (WFGB). Buoys at EFGB1 and EFGB6 are at 19.5 m water depth, EFGB3 at 19.8 m, and EFGB4 at 18.6 m. WFGB1 is at 23.8 m, while WFGB2 and WFGB3 are in 24.4 m water depth. Bathymetry data obtained from USGS, while boundary shapefiles were obtained from FGBNMS.
In 2015, 2016, and 2018, research at EFGB and WFGB was conducted at buoyed sites that ranged in depth from 18.6 to 19.5 m and 23.8–24.4 m, respectively (Supplementary Table 1), to survey lionfish densities, collect samples for age-growth and size analyses, and calculate removal effort (Figure 1). Four sampling efforts occurred across multiple days over the following research cruises: 31 August–3 September 2015, 29 August–1 September 2016, 26–28 June 2018, and 27–30 August 2018. Each research expedition constituted one cruise. Research cruises visited the same buoys when possible. If buoys were missing, similar alternate sites were visited if time allowed, but some cruises were cut short due to poor weather and/or sea conditions. Percent coral cover, coral composition, depth range, and habitat complexity are comparable for all sites. However, it is important to note buoy 1 at EFGB (i.e., EFGB1), WFGB1, and WFGB3 are located at the edge of the reef cap which transitions into deeper reef area (>30.1 m) marked by coral cover as high as 94% (Johnston et al., 2021).
Lionfish Surveys and Collections
Lionfish surveys and collections were conducted in 16–30 m water depth by two separate dive teams: scientific divers who were experts in reef fish field identification and techniques completed surveys to count lionfish, while removal divers collected lionfish on a separate, subsequent dive. Surveys were conducted using SCUBA during daylight hours between 0800 and 1600 h, with underwater visibility ranging from 80 to 100 m. Starting from the buoy U-bolt, scientific divers laid transect tapes 100 m along the reef bottom in each cardinal direction for lionfish collections by removal divers (Supplementary Figure 1). On each transect, a lionfish survey following techniques described in Green et al. (2012c) was conducted within a 50 m × 20 m area, covering a total of 4,000 m2 per buoy. Scientific divers waited 5 min after a transect line was laid, then began a survey at the 75 m mark on the transect tape and swam toward the U-bolt in a sinusoidal pattern hovering above the transect tape, surveying under ledges and over coral heads to count lionfish. Lionfish surveys ended at the 25 m mark on the transect tape. Each lionfish survey was considered one sample.
Following the survey, lionfish were collected using pole spears and retained within a hard containment unit on two dives separated by a 2-h surface interval. One survey dive and two removal dives were conducted at each buoyed site visited. In total, 12 samples across three buoys were conducted at EFGB and WFGB in 2015 (n = 24 surveys). In 2016, 12 samples were conducted at three buoys at WFGB and 8 samples from two buoys at EFGB (n = 20 surveys). In 2018, eight samples were collected from two buoys at each bank in June (n = 16 surveys), while 12 samples were conducted at three buoys at each bank in August (n = 24 surveys). Fewer lionfish surveys and removals were completed in 2016 and June 2018 due to inclement weather.
Density
Visual survey data from transects were entered following each dive and all data underwent assurance for quality control (QA/QC) by a second party, to ensure consistency between data recorded in the field and data entered into the database.
Survey area was computed by multiplying the transect length and transect width, for a total area of 1,000 m2 surveyed per sample. Lionfish counts recorded in each cardinal direction (i.e., four samples per buoy; Supplementary Figure 1) were divided by total area surveyed to determine density per sample (ind. m–2). Mean density for each buoy was calculated by averaging lionfish density across the four samples and extrapolated to density per 1-ha (ind. ha–1). Mean density per bank was calculated by averaging lionfish density across all samples at the bank in a sampling period and extrapolated to ind. ha–1.
A Welch’s two-sample t-test was used to contrast mean density between banks to identify if significant differences existed.
Catch per Unit Effort
Catch per unit effort (CPUE) for removal divers was standarized by restricting teams to two divers with a 45 min total bottom time for each dive. The total catch for each removal team was computed by summing the TW of all lionfish removed (kg) during one cruise. CPUE (kg diver h–1) was calculated by:
where TWkg is the total weight captured, n is the number of divers (n = 2), and t is the total dive time (number of dives*bottom time). An ANOVA was used to determine if there were significant differences in CPUE between cruises and banks. If differences were detected, a Tukey-post hoc analysis was conducted to determine homogenious subsets.
Weight-Length Relationship
At the end of each day, lionfish total length (TL, mm) was measured on the vessel and fish were retained in labeled bags and later transported to the NOAA Galveston Lab to measure total weight (TW, g) and other metrics. A random subset of lionfish (37% of total collected) were thawed and dissected to determine sex, following methods found in Green et al. (2012b). Not all lionfish could be dissected because of time and personnel limitations. In some instances, lionfish could not be retained for additional examination. All lionfish collected in 2015 (n = 304) and 2016 (n = 376) were processed in the NOAA Galveston Lab. In 2018, lionfish exceeded the available freezer capacity on the vessel, thus some had to be discarded after TL measurements were collected. In total, 985 lionfish were collected in 2018 and 684 had to be discarded before arrival at the lab.
For discarded lionfish, TW was calculated using an allometric weight-length-relationship (WLR) developed from other lionfish collected at FGBNMS. TL-TW values for lionfish measured in the NOAA Galveston Lab were log transformed to derive parameters for the WLR calcuations. An Analysis of Covariance (ANCOVA) was used to determine if these weight-length relationships differed by sex, cruise, and bank with TL as a continuous variable. A Tukey-post hoc analysis tested for the differences among categorical groups (e.g., cruise) if significant differences were detected in the ANCOVA. If differences were detected, WLR was calculated by categorical group, rather than being pooled.
Lionfish weight-length-relationship was calculated by:
where W = whole body wet weight in grams (g), L = length in centimeters, and a and b are parameters (Switzer et al., 2015). A linear regression of log transformed weight and length values failed tests for heteroskedasticity (i.e., error terms were heteroskedastic). Therefore, coefficients a and b were estimated by a linear regression with robust standard erros of logarithms from the TL and TW measurements of lionfish retained each year:
with parameters defined above, whereby a is the intercept and b is the regression coefficient (slope).
Age and Growth Parameters
Sagittal otoliths were removed from a random subset sample of lionfish in June (n = 51) and August (n = 71) 2018 from EFGB (n = 65) and WFGB (n = 57) across five size bins (0–100 mm, 101–200 mm, 201–300 mm, 301–400 mm, ≥ 401 mm), following methods described in Green et al. (2012b). Otoliths were rinsed with fresh water, dried, and stored in labeled vials. Single otoliths were placed dorsal-ventrally in the center of 22 x 22 x 20 mm embedding molds, filled with West System 105 resin and 206 hardener mixture and left to dry for 24 h (h). When possible, left otoliths were sectioned along the transverse plane using a Buehler IsoMet saw with diamond wafering blade to expose the primordium and growth increments (i.e., annuli), mounted onto glass slides using Crystal Bond, allowed to dry for 24 h, then ground and polished to the vertical mid-sagittal plane with a graded series of Buehler silicon carbide paper (600, 800, and 1,200 grit) and Buehler micropolish alumina 0.3 μm. If the left otolith was not available (e.g., damaged), the right otolith was used for age analysis.
Annuli were viewed under a Leica compound microscope at 45x magnification, and sections were enumerated using the Leica LAZ EZ image analysis software. Otoliths were examined independently by two readers to determine age. Upon completion, readers compared ages and if a consensus could not be made, the otolith was discarded from further analysis. Daily increments were not enumerated in this study.
Age and length data were used to model growth by estimating parameters for the von Bertalanffy (VB) growth equation (von Bertalanffy, 1957):
where Lt is the length at age (t), L∞ is the asymptotic maximum total length, K is the Brody growth coefficient or rate of growth toward L∞, and t0 is the theoretical age at which a fish would be 0 mm in length. The scaling factor, t0, is a modeling artifact used to adjust the model for the initial size of the fish by defining the age at which the organism would be of zero length if its growth were constant over-time (Beverton, 1954; Schnute and Fournier, 1980).
An ANCOVA was used to compare TL between sex and bank while controlling for age to identify if significant differences were evident. If no significant differences were found, all data were pooled. If significant differences existed, a Tukey post-hoc test was used to determine homogeneous subsets. Additionally, a Welch’s two-sample t-test was used to compare mean age by sex and bank to identify if significant differences existed. Estimates from VB growth parameters from this study were compared to previous studies and other regions.
Results
Densities
Mean lionfish densities (individual ha–1) were computed for each buoy at each bank by cruise (Supplementary Table 1). Additionally, mean densities for each bank per cruise were calculated, showing a general increase over time (Table 1). Mean density at EFGB ranged from 26.7 ind. ha–1 to 81.1 ind. ha–1, while mean density at WFGB ranged from 34.1 ind. ha–1 to 104.8 ind. ha–1. Lionfish density consistently increased at EFGB across the cruises (Table 1). Density at WFGB decreased from 2015 to 2016, but then increased from 2016 to June 2018, and nearly doubled from June 2018 to August 2018. The minimum mean density recorded at a buoy was 0 ind. ha–1 and 10 ind. ha–1 at EFGB4 and WFGB1, respectively, in June 2018. The maximum mean density recorded was 102.6 ind. ha–1 and 117 ind. ha–1 at EFGB1 and WFGB3, respectively, in August 2018. The highest lionfish densities were observed at EFGB1, WFGB1, and WFGB3 in August 2018 (Supplementary Table 1). The Welch’s t-test showed significant differences in mean density between banks, in which greater mean lionfish densities were observed at WFGB [t (184.43) = –3.31), p < 0.001].
Mean lionfish densities at FGBNMS appear to be markedly lower than in other invaded regions of the southern GoM, Caribbean Sea, and Western Atlantic Ocean, but slighlty higher than other natural reefs in the northeastern GoM (Table 2).
Catch per Unit Effort
A total of 1,665 lionfish were collected from FGBNMS during the four sampling efforts.
Mean CPUE significantly increased with each cruise [Table 3; ANOVA: F(3, 1688) = 212.30, p < 0.001, Supplementary Table 2], but not by bank (p = 0.376). Results of the Tukey-post hoc analysis indicated CPUE was significantly higher in August 2018 than in June 2018 (p < 0.001), 2016 (p < 0.001), and 2015 (p < 0.001; Supplementary Table 3). Additionally, CPUE was significantly higher in June 2018 than 2016 (p < 0.001) and 2015 (p < 0.001). Mean CPUE was not significantly different between 2015 and 2016 (p = 0.997; Supplementary Table 3).
Size Frequency and Weight Length Relationship
The total number of lionfish collected for each cruise ranged from from 281 to 704 fish (Table 3). Lionfish TL ranged from 75 to 444 mm with mean 281.9 mm ± 53.2 SD. Size frequency of lionfish was generally normally distributed with a clear temporal shift toward larger size classes (Figure 2). Males represented 58.0% (n = 354) of lionfish sexed (n = 610) and females comprised 42.0% (n = 256). This differed by cruise with males representing 44.9–63.4% of lionfish collected, while females comprised 36.6–55.1 % (Table 4). Males TL ranged from 118 to 444 mm with mean 299.0 mm ± 60.7 SD, while females ranged from 137 to 348 mm (mean 266.2 ± 37.7 SD; Table 4).
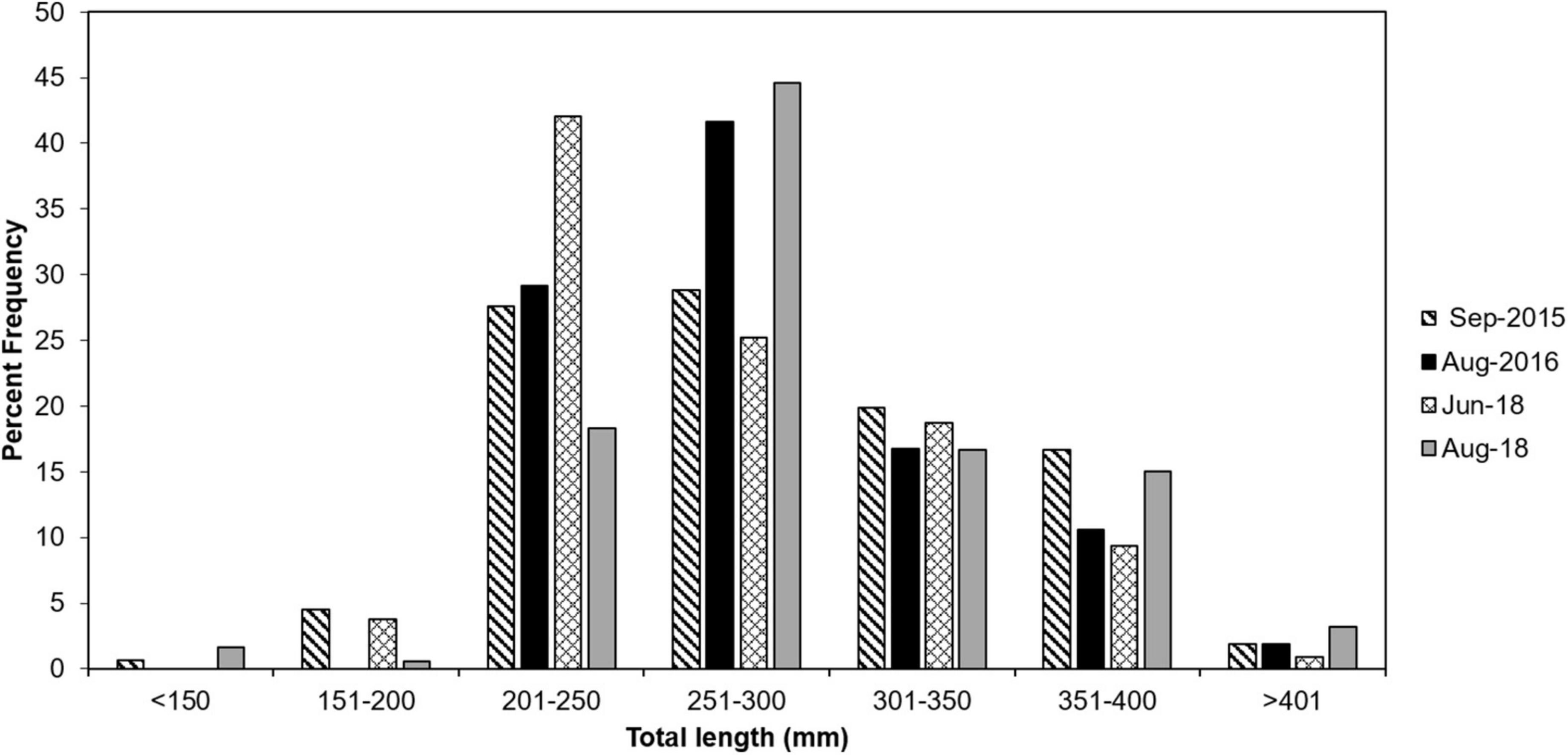
Figure 2. Total length distributions of lionfish collected from EFGB and WFGB in 2015 (striped bar), 2016 (solid black bar), June 2018 (hashed bar), and August 2018 (solid gray bar).
The ANCOVA analysis revealed weight-length relationships differed significanlty by sex [ANCOVA: F(1, 597) = 14.31, p < 0.001] and cruise [ANCOVA: F(3, 929) = 10.48, p < 0.001], but not bank (p = 0.986; Supplementary Table 4). Males were significantly larger than females across all years [ANOVA: F(1, 597) = 14.31, p < 0.001; Supplementary Table 5]. Tukey-post hoc test indicated lionfish collected in August 2018 were significantly larger than those collected in June 2018 (p = 0.02) and in 2016 (p < 0.001; Supplementary Table 6). Additionally, lionfish were significantly larger in 2015 than in 2016 (p = 0.006). Separate WLR equations were calculated for lionfish by cruise, because sex information was not gathered for all fish collected. WLR equations were as follows: –2.02 + 3.10*LogL (2015), –1.60 + 2.79*LogL (2016), –2.17 + 3.19*LogL (June 2018), and –2.17 + 3.20*LogL (August 2018).
Lionfish TW ranged from 12 to 1,153 g with mean 321.4 g ± 196.5 SD. Males TW ranged from 18 to 1,153 g (mean 397.1 g ± 245.9 SD) and females 21–586 g (mean 255.2 g ± 111.5 SD; Table 4).
Age and Growth Parameters
Otolith pairs were removed from lionfish ranging in TL 125–444 mm and TW 18–1,153 g. Lionfish were sexed, of which 84 were male, 35 were female, and 3 were undetermined. Of the 122 otoliths collected for aging, 12 were discarded due to poor cuts or over-polished sections. Two readers separately aged otoliths, in which 51% agreement was reached. Following this, readers collaboratively aged the otolith sections and agreed on 91% of the sample. Lionfish ages ranged from 0 to 10 years, with a mean of 3.9 years. Female lionfish ranged in age from 1 to 9 (mean 3.6 ± 2.3), while males ranged from 0 to 10 years old (yo; mean 3.9 ± 2.1; Table 5).
ANCOVA analysis indicated significant differences in TL between males and females [ANCOVA: F(1, 97) = 10.57, p = 0.002], but not by bank (p = 0.894; Supplementary Table 7) when controlling for age. As a result of this difference, otolith-based age data were pooled by bank to generate sex-specific von Bertalanffy (VB) growth equations (Figure 3). Age-0 lionfish were removed from the sample (n = 2) as to not influence the VB equations by misrepresenting the growth curve from lack of daily increment estimations. Male lionfish had lower Brody growth coefficients (K), higher asymptotic maximum length (L∞), and reached a greater length-at-age than females (Table 5). Mean size-at-age data shows males were larger than females for every age class, except age-2 lionfish (Figure 4). For example, age-1 male lionfish were on average 266 mm, but as large as 313 mm; age-1 females were on average 213 mm and as large as 273 mm (Figure 4).
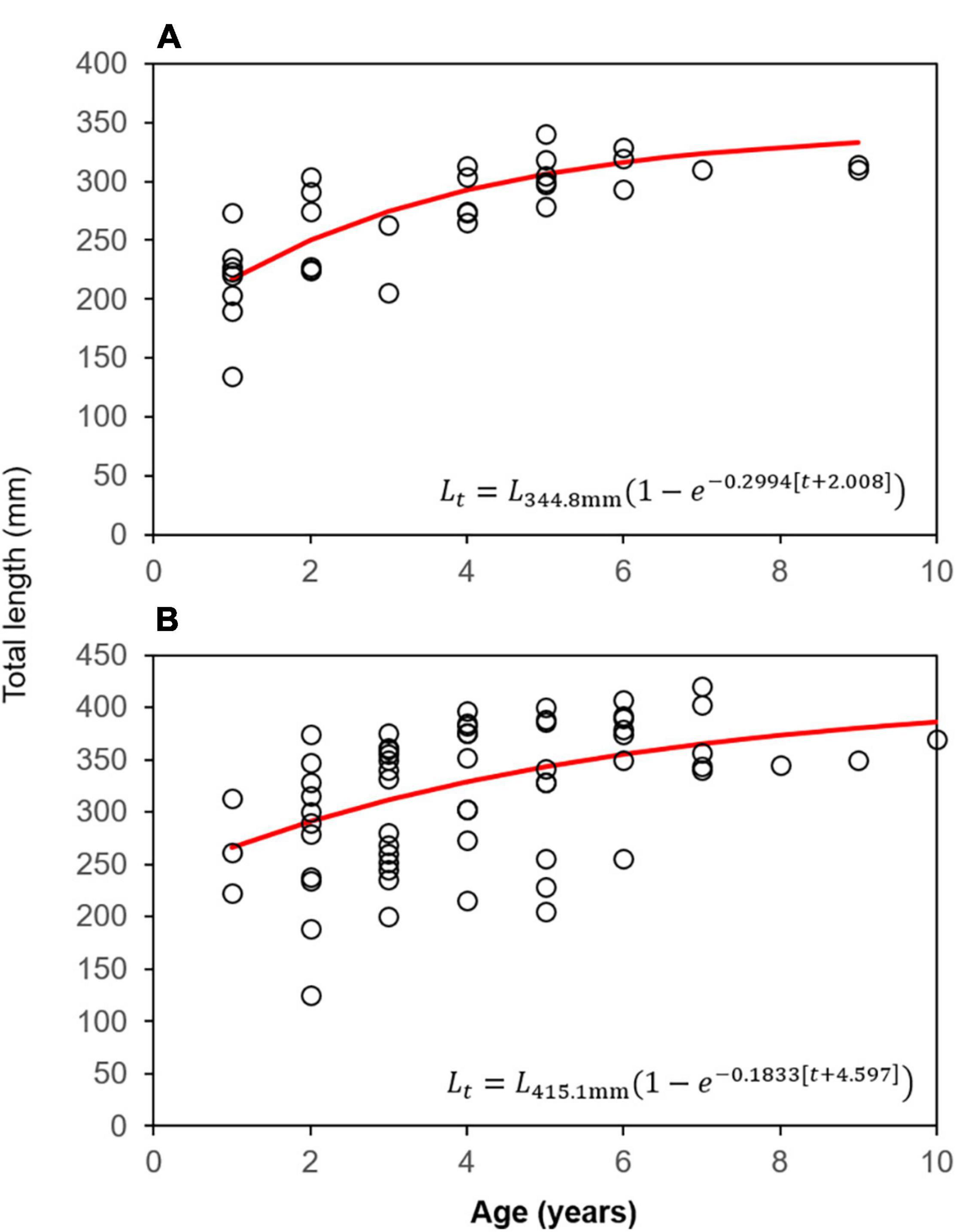
Figure 3. von Bertalanffy growth curves and associated equations for (A) female and (B) male lionfish from FGBNMS.
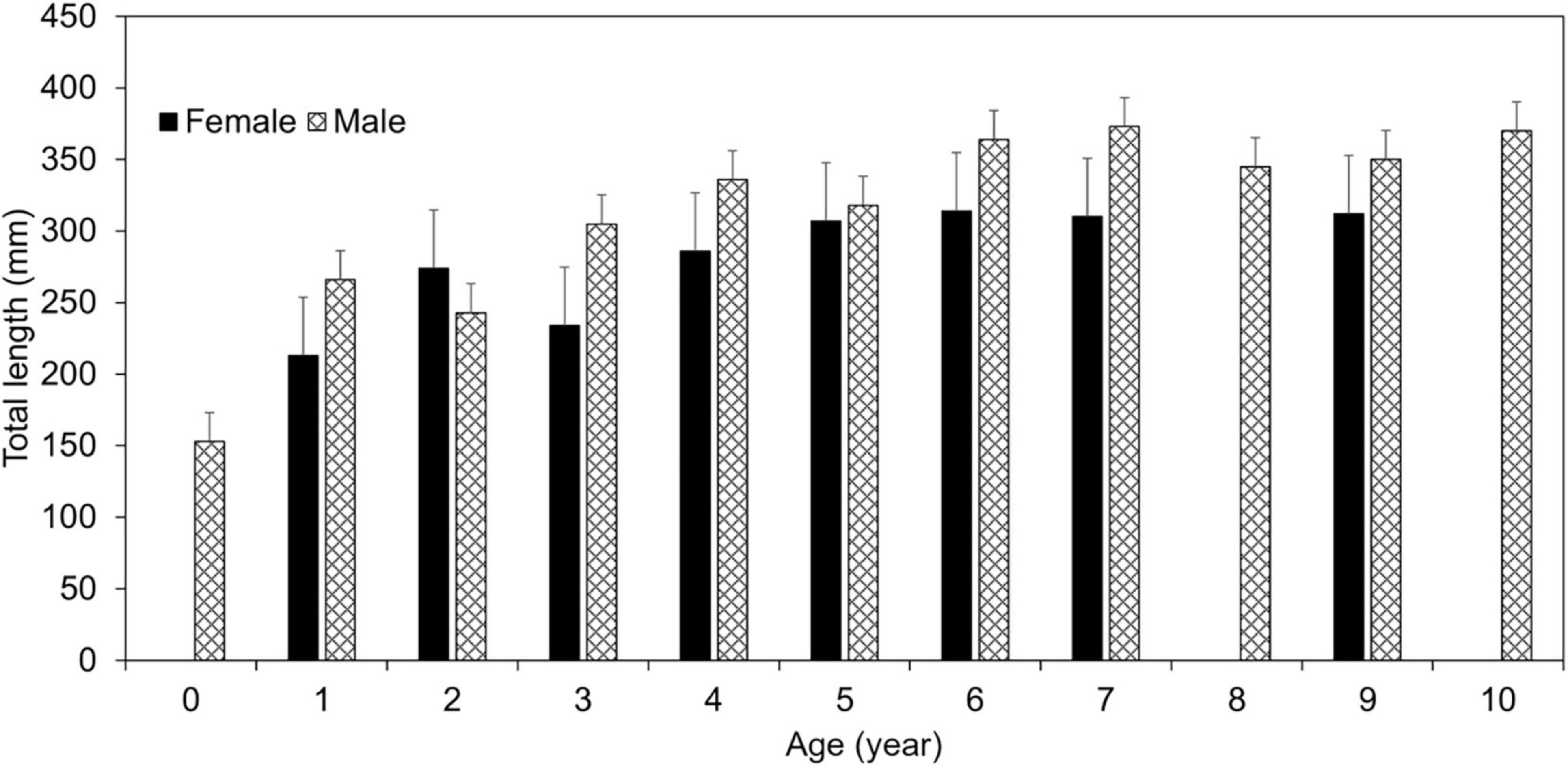
Figure 4. Mean size-at-age of lionfish by sex with standard error bars. Females are shown in solid bars, while males are shown in hashed bars.
Welch’s two-sample t-test revealed no significant difference in the mean age between males and females [t (60.5) = –0.79, p = 0.22]. However, the Welch’s t-test showed significant differences in mean age between EFGB (mean = 3.4, SE = 0.3) and WFGB (mean = 4.3, SE = 0.3), in which WFGB lionfish were older [t (97.8) = –1.92), p = 0.03]. Age frequency distribution shows WFGB is marked by more older individuals than EFGB, particularly from 5 to 10 yo lionfish (Figure 5). Males constituted 54% (n = 26) of lionfish aged from EFGB, while 78% (n = 39) were males from WFGB.
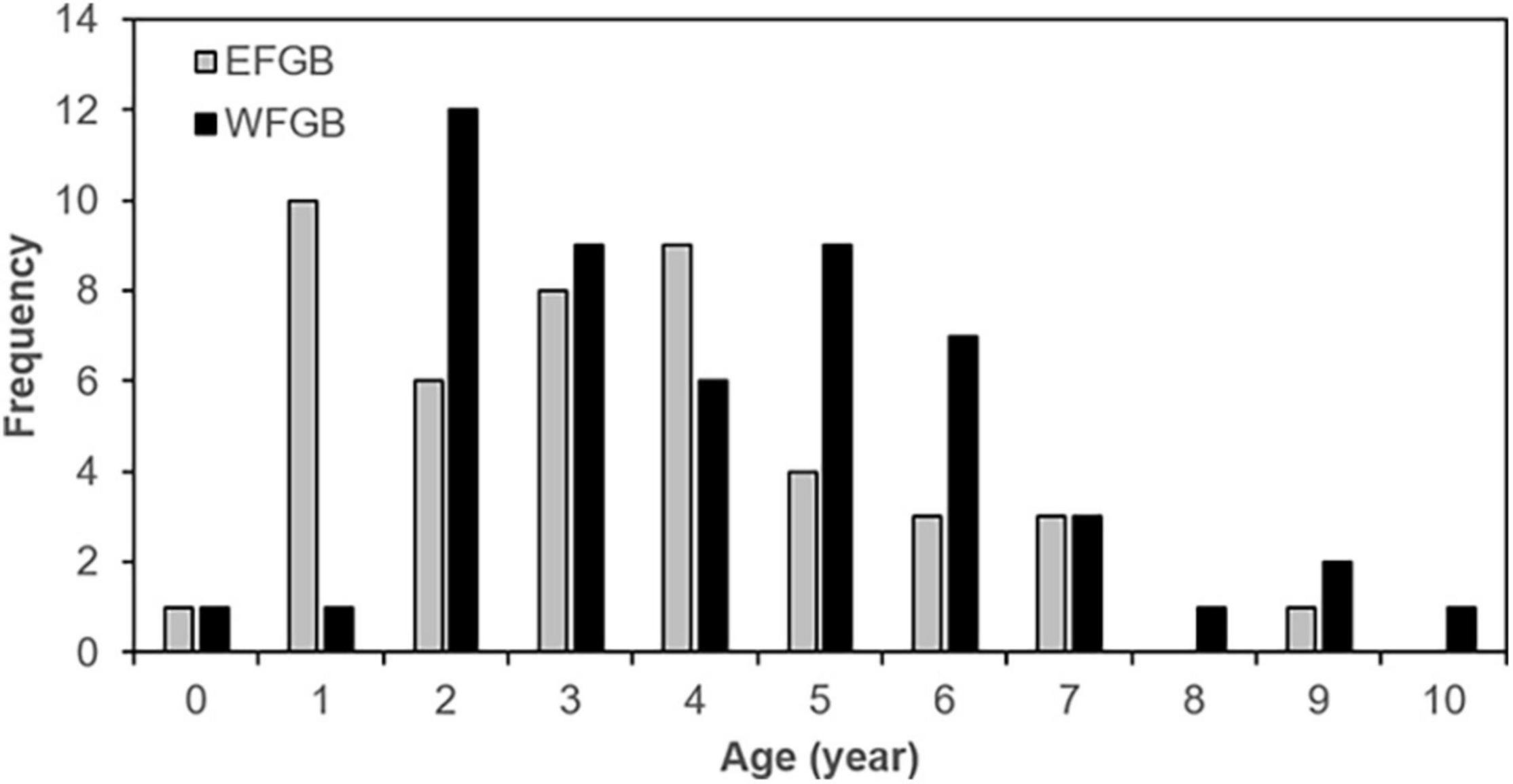
Figure 5. Age frequency distribution of lionfish collected in 2018 by bank at FGBNMS. EFGB is represented by solid gray bars and WFGB is solid black bars.
Discussion
Our study is the first to provide population demographic and CPUE data for lionfish in FGBNMS, a nationally significant marine system marked by some of the healthiest coral reefs in the U.S. Lionfish were observed at both banks on all four research cruises. The results of our study revealed significant differences in density, size, and age of lionfish at FGBNMS, as well as CPUE, between sampling periods.
Main Findings
Lionfish densities increased fourfold at EFGB from 2015 to August 2018, but remained nearly constant at WFGB in 2015, 2016, and June 2018, followed by a twofold increase from June 2018 to August 2018. This was surprising given the similarities in habitat structure, prey availability, and nearly absent fishing-mortality for both reefs. Survey effort was consistent across the banks for all years apart from 2016, in which, four additional samples were collected at WFGB than at EFGB. This, however, would not account for the difference in density trends recorded between these two banks. Lionfish larvae are likely distributed to EFGB and WFGB via the Loop Current from southern GoM reefs in the Yucután Peninsula (Kitchens et al., 2017); however, retention and distribution of lionfish larvae between the two reefs have not been investigated. High local retention of larvae from dominant coral species (e.g., P. strigosa) has been recorded for EFGB and WFGB (Davies et al., 2017; Garavelli et al., 2018), largerly influenced by eddies known to spin off from the Loop Current (Limer et al., 2020). Limer et al. (2020) noted between-bank exchange of coral larvae, with an eastward transport, whereby WFGB contributed coral larve to EFGB. Although this is highly influenced by variation in eddy movement, it could lead to a disproportionate mid-range larval dispersion, in which EFGB receives more larval input than WFGB. This is not to suggest that a direct comparison between inter-bank connectedness, retention, and transport of coral larvae can be made for lionfish larval exchange; however, it is worth noting that WFGB could act as an additional source for lionfish larvae at EFGB. This exchange needs to be confirmed using mesocale current modeling of lionfish larvae, but would likely provide insight as to why lionfish densities continually increase at EFGB while they remain nearly constant at WFGB. Fishing mortality is negligible at both banks and the fish community and benthic habitat composition are similar, which suggests there are other factors contributing to this disparity in densities.
EFGB1, WFGB1, and WFGB3 exhibited some of the highest mean densities documented across the sampling period. All three buoys are located near the edge of the reef cap which slopes to deeper water rather rapidly (Figure 1). These slopes and deeper areas of the reef (>30.1 m) are marked by more expansive coverage of scleractinian coral, as high as 94%, offering a more continuous and complex habitat than the bank crest. Lionfish have been noted to occur in higher densities on reef ecosystems lying 30–60 m, with higher abundances associated with greater structural complexity (Lesser and Slattery, 2011; Claydon et al., 2012; Nuttall et al., 2014; Andradi-Brown, 2019). On Honduran reefs, lionfish in depths > 30 m exhibited behavior consistent with diver wariness that was observed in the shallow reef areas (<30 m), suggesting potential exposure to culling. This does not explicitly suggest lionfish travel between the shallow reef cap to deeper areas; however, is notable given the lack of culling efforts that occur below 30 m (Andradi-Brown et al., 2017). Because of the high continuous coral coverage between the shallow (16–24 m) and deep reef (30–60 m) at the three buoys, and that lionfish have been recorded to travel up to 2 km (Dahl and Patterson, 2020), it is not unrealistic to assume that lionfish could be transiting along the reef slope at these locations. It is plausible this transition to deep reef plays a role in the higher densities observed at these three buoyed areas, as has been previously recorded in Little Cayman (Bejarano et al., 2014).
Overall, mean CPUE significantly increased with each sampling period, but did not differ between banks. In particular, mean CPUE nearly doubled between June 2018 and August 2018 cruises. It is not apparant why CPUE increased so drastically between these two periods. Removal divers were thoroughly vetted for these cruises, with preference given to those with removal experience and those who had been to FGBNMS before. We do not suspect this difference in CPUE can be attributed to differences in diver ability. CPUE and lionfish density increased overtime concurrently, suggesting the lionfish population is expanding at EFGB and WFGB. We would expect that an increase in effort would reduce lionfish densities if fishing mortality was having a measurable impact on the population; however, this was not evident in our study.
We note, in general, lionfish shifted toward larger size classes at EFGB and WFGB during our study period. This is contradictory to previous studies in the northeastern GoM, in which lionfish mean size appeared to shift to smaller size classes (Dahl et al., 2019). Targeted fishing efforts can have a pronounced effect on the size structure of marine fish species, with a general shift toward smaller size classes with increasing fishing pressure (e.g., Bianchi, 2000; Tu et al., 2018). Lionfish are predominantly removed through spearfishing as they have low vulnerability to conventional hook-and-line techniques (Morris and Whitfield, 2009). Spearfishing is a restricted activity in FGBNMS, thus, fishing-mortality is nearly negligible at EFGB and WFGB and likely does not effect lionfish size class variation. Lionfish at EFGB and WFGB showed sexually dimorphic size variation consistent with previous research (Morris and Whitfield, 2009; Morris et al., 2011; Edwards et al., 2014; Dahl et al., 2019; Eddy et al., 2019; Fogg et al., 2019), where males were significanlty larger than females and comprised a greater percentage of the larger size classes across all sampling periods. Evidence of adult mediated density-dependent growth has been reported for lionfish on artificial reefs in the northeastern GoM (Dahl et al., 2019); however, we do not find evidence to support this phenomenon at FGBNMS. FGBNMS is absent of the ecological (e.g., warm temperatures, low resource availability) and biological (e.g., high density, disease) constraints described to influence density-dependent traits in adult lionfish Dahl et al. (2019). If lionfish densities at EFGB and WFGB increase to carrying capacity, density-dependence may affect the population structure in the future; however, this would need to be monitored to determine if this occurred.
Results of this study revealed significant differences in weight-length relationships between sex and cruise, but not by bank. Males were heavier per length than females for all sampling periods, consistent with results from other regions in the northern GoM (Dahl and Patterson, 2014; Fogg et al., 2019). Mean size was significantly different between cruises, generally increasing with each year. Similarly, Dahl and Patterson (2014) reported significant inter-annual increases in mean size for lionfish in the northeastern GoM on artificial and natural reefs. Lionfish weight-length relationships did not differ by bank; EFGB and WFGB are comparable in habitat and food resources which may allow for similar body conditions at the two reefs. Lionfish populations likely have not reached their peak at FGBNMS, as density and mean size increased throughout the study period.
Mean age of lionfish were significantly different between banks, but not by sex. Lionfish were older at WFGB than EFGB by approximately 1-year and WFGB was marked by more, older individuals. Males constituted more of the lionfish aged at WFGB than EFGB (78% vs. 54%) and likely contributes to the observed differences. Female lionfish tend to grow faster than males, but attain a smaller maximum size and length at maturity throughout their invaded range (Edwards et al., 2014; Dahl et al., 2019; Eddy et al., 2019; Fogg et al., 2019), which was also true for this study. A disproportion in larval exchange could contribute to the difference in lionfish age between the two banks, if WFGB in fact acts as a larval source for EFGB. If this holds true, we would expect to see more, older individuals at WFGB and a predominatly younger population at EFGB. Lionfish from FGBNMS were notably comprised of more, older individuals which may be attributed, in part, to fewer collections of smaller lionfish. Small lionfish are harder to detect in complex natural habitats (Harris et al., 2019); EFGB and WFGB are notably complex reef habitats that could provide refuge for smaller lionfish, rendering their detection and removal challenging. Lionfish at FGBNMS exhibited one of the slowest growth rates than in any of the other invaded regions. Daily increment analyses were not conducted in this study, and thus, removes the effect of juvenile growth characteristics in the VB growth curve estimates, which may lead to an under-estimation of these values (Pusack et al., 2016; Dahl et al., 2019; Eddy et al., 2019; Fogg et al., 2019). Additionally, the sample size for the age analysis in this study was limited and may further skew the VB growth curve estimates. Future studies should prioritize collection of smaller lionfish to include juveniles and increase the sample size in age-based analyses to better quantify these parameters for FGBNMS.
Lionfish age structure at EFGB and WFGB most closely matched the age structure of lionfish collected in Bermuda (Eddy et al., 2019), which is interesting given the difference in invasion chronology. However, we note FGBNMS and Bermuda have similar habitat types and are both northern latitude coral reefs with seasonal temperature fluctuations, which may contribute to the observed similarities. The first reported observation of lionfish in Bermuda occurred in 2000, while the age and size analysis were on lionfish collected between 2012 and 2016 (Eddy et al., 2019). Here, we report age structure for lionfish from FGBNMS in which observations were first reported in 2011 and analysis conducted in 2018. Results from this study suggest lionfish invaded EFGB and WFGB 3 years prior to initials observations (Blakeway et al., 2021), but also shows lionfish can successfully attain older ages in their invaded region when fishing-induced mortality and predation are negligible. Lionfish grow considerably slower at EFGB and WFGB than Bermuda for pooled data and males, exhibiting two-fold lower Brody growth coefficients. However, male lionfish from FGBNMS attain similar asymptotic maximum total length as males in Bermuda (415 mm vs. 418 mm, respectively). We report a larger L∞ for females at FGBNMS as compared to Bermuda (345 mm vs. 332 mm; Eddy et al., 2019), though this is likely a result of under-sampling for the age analysis. It is not obvious from our analysis whether FGBNMS has a greater proportion of larger, older lionfish than other invaded regions, or if this is an artifact of a small sample size. A more robust sample size would afford for more confidence in interpreting growth parameter values for lionfish from FGBNMS.
It should also be noted that it is not clear whether this differentiation between regional age and size structure is due to differences in species, in that, both species of lionfish (P. volitans, P. miles) have been confirmed in the Caribbean Sea and Western Atlantic Ocean, while only one species (P. volitans) has been confirmed in the GoM (Betancur-R et al., 2011; Johnson et al., 2016). There are conflicting reports on whether the distribution and expansion of the two species of lionfish have been homogeneous throughout their invasion of these regions (Perez-Portela et al., 2018) or if a genetic founder effect occurred (Betancur-R et al., 2011; Toledo-Hernández, 2014; Johnson et al., 2016). Nevertheless, genetic analyses may afford the ability to determine if differences reported in age/size and growth demographics are species-specific, and thus should be included in future research. Lionfish life history parameters characterized in this study have illustrated a successful invasion at both EFGB and WFGB in the northwestern GoM. When compared with lionfish population structure in other invaded reefs in the GoM, Western Atlantic Ocean, and Caribbean Sea, striking differences are evident.
Seven years after the invasion, lionfish densities at EFGB and WFGB remain twofold lower than in Mexico and Belize (Hackerott et al., 2013; Sosa-Cordero et al., 2013), two to sevenfold lower than in the Bahamas and North Carolina (Morris and Whitfield, 2009; Darling et al., 2011; Green et al., 2012b), twofold lower than in the southern Caribbean Sea, and 10-fold lower than on artificial reefs in the northeastern GoM (Dahl and Patterson, 2014; Dahl et al., 2019). The lionfish invasion chronology was similar among these regions, in terms of time between first reported observation and date of survey, which suggests a slower colonization and growth rate of lionfish at EFGB and WFGB. Lionfish in higher latitudes (i.e., Bermuda, northern GoM) have shown seasonal spawning patterns, with peak spawning activity in the summer and fall and a subsequent decline or absence in the winter months (Fogg et al., 2017; Eddy et al., 2019). This seasonality in spawning could result in seasonal fluctuations in density. However, the limited surveys, predominantly conducted the same time of year, may yield skewed density estimates at EFGB and WFGB. More surveys that span a greater temporal scale would be necessary to determine if density exhibits a seasonal pattern at FGBNMS, particularly one that correlates with spawning seasonality.
At FGBNMS, 31% of lionfish were ≤ 2 yr, 58% ranged from 3 to 6 yo, and 11% from 7 to 10 yo. Age structure between males and females were identical, with 37% being ≥ 5 years of age and 63% < 5 years old. This age structure is much different from lionfish collected in the Caribbean Sea (Edwards et al., 2014), Western Atlantic Ocean (Barbour et al., 2011; Johnson and Swenarton, 2016), and northern GoM (Fogg et al., 2019), where 90% of their sampled fish were ≤ 3 years of age. Additionally, mean lionfish age at FGBNMS was older at 3.9 yo, while most other regions reported mean ages < 3 yo (Barbour et al., 2011; Edwards et al., 2014; Rodríquez-Cortés et al., 2015; Johnson and Swenarton, 2016; Dahl et al., 2019; Eddy et al., 2019; Fogg et al., 2019) (Table 6).
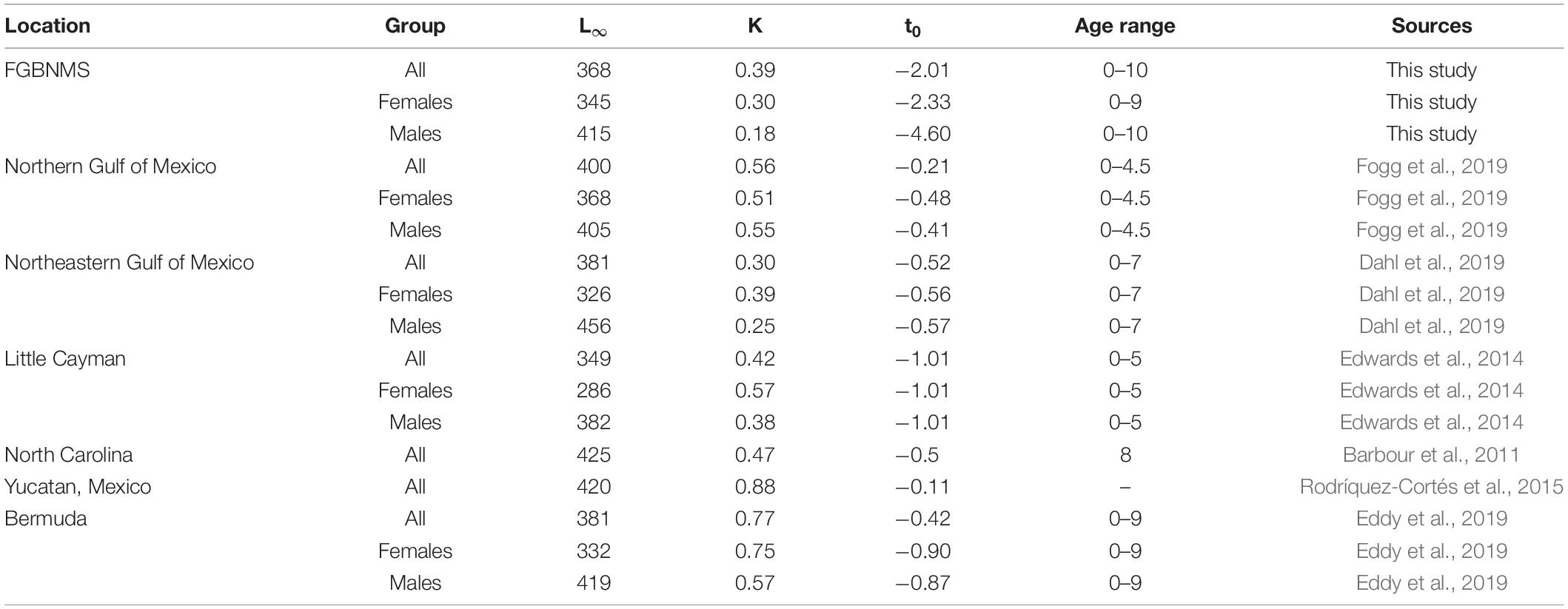
Table 6. Comparison of age and growth parameters and maximum age reported for lionfish from invaded regions.
Lionfish at EFGB and WFGB appeared to grow slower (K = 0.39) than lionfish from other areas in the northern GoM (0.56, Fogg et al., 2019), southern GoM (0.88, Rodríquez-Cortés et al., 2015), Western Atlantic Ocean (0.47, Barbour et al., 2011; 0.77, Eddy et al., 2019), and Caribbean Sea (0.42, Edwards et al., 2014). In every location, including FGBNMS, lionfish demonstrated sexually dimorphic growth, in which females typically grew faster but attained a smaller L∞ (Edwards et al., 2014; Dahl et al., 2019; Eddy et al., 2019; Fogg et al., 2019). Male lionfish exhibited the slowest growth at FGBNMS of all the invaded regions, in most cases growing two times slower than other areas in the northern GoM (Dahl et al., 2019; Fogg et al., 2019), Western Atlantic Ocean (Barbour et al., 2011; Eddy et al., 2019), and Caribbean Sea (Edwards et al., 2014).
Lionfish were smaller on average at FGBNMS (mean TL 281.9 mm ± 53.2) than those collected in Bermuda (mean TL 339 mm ± 1.6; Eddy et al., 2019) and North Carolina (mean TL 305 mm; Whitfield et al., 2007). The differences in the size structure between FGBNMS and the Western Atlantic Ocean may be attributed to different environmental factors (e.g., temperature) or regional differences in prey availability, sampling techniques (i.e. fewer smaller, younger lionfish sampled in the Atlantic), or that marine teleosts are typically larger in temperate regions (Luckhurst et al., 1992; Robertson et al., 2005).
The size classes of lionfish at EFGB and WFGB showed a continual shift toward having more, larger fish, particularly with respect to males. This differs from reports in the northeastern GoM, which showed lionfish shifting to smaller size classes (Dahl et al., 2019). Density-dependent growth processes may have acted as an important population-level regulator of lionfish on reefs in the northeastern GoM (Dahl et al., 2019); however, it is not apparent that this is occurring with lionfish populations at FGBNMS. Ecological conditions (e.g., rugous, complex reef structure, high species richness and prey biomass) are much different at EFGB and WFGB than on artificial reefs (Rooker et al., 1997; Dance et al., 2011; Patterson et al., 2014; Dahl et al., 2016, 2019; Johnston et al., 2021), for example, and we suspect that lionfish are not as susceptible to competition for food and habitat resources that would lead to density-dependent regulation of adults in this system. This suggests there are likely other mechanisms controlling lionfish populations at FGBNMS.
Invasion Impacts and Implications for Resilience and Management
Lionfish are subject to negligible fishing-induced mortality, lack observations of predation by native predators, do not exhibit traits of population-level regulation (i.e., density-dependent growth), and lacked signs of disease at FGBNMS. Lionfish are larger in the invaded range as compared to their native range in the Indo-Pacific (Pusack et al., 2016), which may lessen predation by native predators. Additionally, native predators may be wary of consuming lionfish because of the venomous dorsal spines that are uncharacteristic of other native prey species. Density-dependent population control is not evident at FGBNMS, as lionfish exhibited lower densities and were characterized by larger, older fish. Fewer or shorter recruitment events may, in part, be responsible for lower lionfish densities at EFGB and WFGB affected by seasonal spawning. Lionfish showed seasonal spawning in northern latitudes where ambient water temperature fluctuations followed a seasonal pattern (Fogg et al., 2017; Eddy et al., 2019). EFGB and WFGB also reveal seasonal variation in water temperature, with a 10°C difference between maximum and minimum temperatures in the summer and winter months (Johnston et al., 2021). Although it cannot be deduced from data presented in this study if seasonal spawning or intermittent recruitment are leading to lower densities, given other similarites between lionfish in FGBNMS and Bermuda and that FGBNMS is a remote reef system, it could be assumed these are individually or collectively contributing factors. Future research would need to investigate this phenomenon to determine if recruitment or spawning is reduced at these banks. An ulcerative disease led to considerable declines in northern GoM lionfish populations in 2018 (Harris et al., 2020); however, evidence of this disease was not observed during surveys/removals of lionfish at FGBNMS during this study period. Although this may have impacted lionfish populations at EFGB and WFGB at some point, it was not a contributor to observations for this study. The population characterisitcs at these reefs may also indicate there are other environmental or ecological controls contributing to the observed differences between FGBNMS and other invaded regions.
Our study may support the idea that lionfish have been incorporated functionally into the existing ecosystem processes at EFGB and WFGB (e.g., lower densities, slower growth, older invidivuals) because few alterations, if any, have been observed to the extant ecosystem dynamics of these reefs (Johnston et al., 2016a,2021). This could be attributed in part, to ecological resilience, in that the overall reef health improves the capacity to absorb the lionfish invasion with very little impact. Ecological resilience is the capacity of a system to withstand change while maintaining processess and structures (Holling, 1973), and offers a theoretical frame for understanding ecosystem-level controls for biological invasions. For example, EFGB and WFGB possess slightly different reef fish assemblages than other reef systems in the Caribbean Sea and GoM, maintaining stable resident populations dominated by pomacentrids and labrids (Wetmore et al., 2020; Johnston et al., 2021), with a limited presence of important predators such as lutjanids and haemulids (Rooker et al., 1997; Johnston et al., 2021). Lionfish are a known generalist meso-predator with a diet consisting primarily of small-bodied teleosts and crustaceans (Morris and Akins, 2009; Cote et al., 2013b; Eddy et al., 2016). Thus far, the presence of lionfish at EFGB and WFGB has not correlated with any measurable decrease or negative effect to biomass of prey fishes (Johnston et al., 2016a), as has been reported to have rapidly occurred in other invaded regions (e.g., Albins and Hixon, 2008; Green et al., 2012a; Benkwitt, 2014; Ballew et al., 2016), even with a diet that is broadly similar in composition and quantity (Johnston, unpublished data). This may suggest lionfish provide the same functional role as other important predators at EFGB and WFGB without reducing the ecological funtion of prey species that could otherwise lead to ecosystem-level impacts (Albins and Hixon, 2013). Despite the addition and increasing densities of lionfish at EFGB and WFGB, the fundamental ecological functions provided by reef fish seems to be conserved across the ecosystem.
EFGB and WFGB have shown evidence of ecological resilience to perturbations for several decades, being one of the healthiest coral reefs in the GoM and Caribbean regions (Gil-Agudelo et al., 2020; Johnston et al., 2021). In the early 1990’s, a phase shift in coral reefs was described referring to a drastic change from a coral to algal dominance, particularly with coral reefs throughout the Caribbean Sea (Done, 1992; Hughes, 1994; Mumby et al., 2013). However, this has not occurred at EFGB and WFGB, rather it has remained a coral dominated reef over the last three decades even with significant increases in macroalgae cover, again suggesting resilience to disturbance (Schmahl et al., 2008; Johnston et al., 2021). Additionally, EFGB and WFGB appear to be resilient, in part, in their apparent recovery, with very minimal mortality, from major bleaching events (Johnston et al., 2019), albeit the significant warming water temperature trend documented over the last 30 s (Johnston et al., 2021). Given the reefs’ ability to respond favorably following extreme and longwithstanding disturbances, it may be the same has been true for the lionfish invasion. It is not clear whether the lionfish invasion will have negative impacts in the future, but thus far, their presence has not contributed to apparent degredation of the reef systems or ecosystem function (Johnston et al., 2016a,2021). The presence and/or level of ecological resilience the lionfish population characteristcs confer cannot be deduced from our study; however, it may prove itself overtime with longer temporal observations. Other regions have revealed the lionfish invasion did not lead to signifcant negative impacts to prey fish communities, and though a definitive explanation was unclear, the health of reef systems and ecological parameters were suggested as potential contributors (Valdivia et al., 2014; Elise et al., 2015; Hackerott et al., 2017; Goodbody-Gringley et al., 2019). It cannot be ignored that ecological resilience of EFGB and WFGB may be a mechanism for controlling lionfish at these reefs, whereby managing the populations lie with the environment itself rather than through human intervention alone. However, it must also be understood that more time, information, and data are needed to evaluate whether or not ecological resilience is evident at FGBNMS.
Our present study of life history characteristics and removal effort of lionfish at EFGB and WFGB may suggest biological (e.g., seasonal recruitment) and/or ecological (e.g., resilience) mechanisms are contributing to controlling the invasion at this protected reef. This has the potential for very different management of lionfish at these reefs, because to date, most strategies have been reactionary and focused on control through mitigation and removal. Here, we argue, that gaining an understanding of the lionfish invasion, seasonality in recruitment and spawning, and population characteristics as it relates to ecological resilience will allow for more deliberate engagement with resilience-based approaches to governing (Cook et al., 2010; Foxcroft and McGeoch, 2011; Garmestani and Benson, 2013) their presence in reef ecosystems. In this sense, we argue lionfish management may be more successful if governance takes a dynamic approach that considers ecological systems jointly to achieve a strategy that accommodates adaptation. As such, we recommend resource managers employ resilience-based management practices that “use knowledge of current and future drivers influencing ecosystem function to prioritize, implement, and adapt management actions that sustain ecosystems and human wellbeing” (McLeod et al., 2019) for established invasive species. The data provided in this study are an example of empirical-based metrics that can be used to identify desirable characteristics that support resilience in a reef system, which can later be integrated into mechanistic ecological models to understand the processes of resilience and/or predict future coral reef trajectories under multiple stressors.
We suggest FGBNMS is uniquely positioned to evaluate ecological resilience and the level of control it can provide after an invasive marine fish species establishes in the ecosystem. Furthermore, we propose resource managers at FGBNMS, and other invaded areas with similar characteristics (e.g., remote offshore location, healthy system, few anthropogenic disturbances), redirect their approach to management of lionfish (and other invasive species) to focus on understanding ecosystem dynamics as opposed to controlling a single species (e.g., Angeler et al., 2015). Additionally, these efforts would also be useful in protected marine areas where consistent, physical removal may not be an effective or practical mitigation strategy. By gaining an understanding of biological invasions through ecosystem function, governance approaches can be resilience-based and coordinate management at scales relevant to ecosystem functions, rather than anthropocentric scales driven primarily by political and jurisdictional boundaries (Chaffin et al., 2016). It will be important that future studies prioritize ecosystem-based analyses that tailor to the understanding of ecosystem-scale functions that may be affected by the presence of lionfish. This is particularly critical for locations, like FGBNMS, that have minimal to no influence of fishery-induced mortality and will require other mechanisms of population-level control. With ecosystem-based analyses, resource managers and scientists may be able to better understand whether lionfish are having a negative impact to an invaded area or if in fact, the environment can transition into another functioning regime, whereby ecosystem resilience is the primary control mechanism.
Conclusion
The extent to which invasive lionfish may impact the unique offshore ecosystem of FGBNMS is relatively unknown, though no evidence suggests, to date, their presence has had any measurable impact on biodiversity, community structure, or ecosystem function (Johnston et al., 2016a,2018, 2021). This study describes key life history attributes and removal effort of lionfish on a protected coral reef system in the northwestern GoM. Despite minimal human removal of lionfish at FGBNMS, lionfish populations have shown slower population expansion as compared to other invaded regions of the Western Atlantic Ocean, Caribbean Sea, and northeastern GoM. In particular, this study demonstrated lionfish at FGBNMS exhibit lower densities with significantly increasing CPUE, indicating population expansion. Additionally, a temporal shift toward larger size classes suggests an expanding population. This study showed longevity of lionfish with more, older individuals and slower growth rates comparable to those aged in other temperate regions (i.e., North Carolina and Bermuda). EFGB showed an increasing lionfish density, while WFGB remained relatively stable over the study period, likely attributed to a slight unidirectional larval dispersal between the two banks. EFGB had predominately younger lionfish as compared to WFGB, further suggesting this unidirectional transfer of larvae. Given the absence of fishing mortality (because spearfishing is a restricted activity at FGBNMS), expanding population of lionfish over a decade (introduced in 2008, study occurred in 2015, 2016, and 2018), lack of observed predation from natural predators, absence of disease, and that lionfish did not exhibit traits of density-dependent growth (Dahl et al., 2019), our study suggests there may be an environmental or ecological control for the lionfish populations at FGBNMS. Lionfish densities may be lower due to seasonal spawning or intermittent recruitment which has impacted the expansion of lionfish populations in northern latitudes (i.e., Bermuda and northern GoM), mostly attributed to lower ambient water temperatures, of which are experienced seasonally at FGBNMS. In contrast, we suggest EFGB and WFGB may present ecological resilience to invasive lionfish, that affords these reef systems the ability to absorb impacts on the established populations. This phenomenon would have very different implications for management at this protected reef, where consistent, physical removal is not practical. Ecosystem-based analyses would afford a better understanding of whether lionfish are having a negative impact at FGBNMS and require directed intervention, or if the environment can act as the primary control mechanism for this reef system.
Data Availability Statement
The raw data supporting the conclusions of this article will be made available by the authors, without undue reservation.
Ethics Statement
The animal study was reviewed and approved by the Texas A&M University’s Animal Welfare Assurance Program Institutional Animal Care and Use Committee.
Author Contributions
RB, AF, MJ, JR, and GJ contributed to the conceptualization of this study. RB and GJ designed the experiments. RB and AF analyzed the data. JR contributed the reagents, materials, and analysis equipment. RB wrote the initial draft of the manuscript. All authors contributed to the article, revised the manuscript, and approved the submitted version.
Funding
Funding for sampling efforts were funded, in part, by Texas State Aquarium, Ripley’s Aquarium, the Georgia Aquarium, Texas Lionfish Control Unit, Reef Environmental Education Foundation, and the M/V Fling.
Conflict of Interest
The authors declare that the research was conducted in the absence of any commercial or financial relationships that could be construed as a potential conflict of interest.
Publisher’s Note
All claims expressed in this article are solely those of the authors and do not necessarily represent those of their affiliated organizations, or those of the publisher, the editors and the reviewers. Any product that may be evaluated in this article, or claim that may be made by its manufacturer, is not guaranteed or endorsed by the publisher.
Acknowledgments
We would like to thank the Texas State Aquarium, Ripley’s Aquarium, Texas Lionfish Control Unit, Reef Environmental Education Foundation, and the M/V Fling for contributing funds for these research expeditions. We also greatly appreciate all of the volunteer divers that participated, as well as the crew members of the M/V Fling. We appreciate all of the volunteers that helped with lionfish dissections and measurements, and we appreciate graduate and undergraduate students in JR.’s lab. Lionfish collections were a permitted activity in FGBNMS, under permit numbers FGBNMS-2015-001 (2015), FGBNMS-2016-006 (2016), and FGBNMS-2018-002 (2018). We appreciate FGBNMS granting the permits for these expeditions. No Animal Use Protocol (AUP) was required for this study, as determined by Texas A&M University’s Animal Welfare Assurance Program Institutional Animal Care and Use Committee.
Supplementary Material
The Supplementary Material for this article can be found online at: https://www.frontiersin.org/articles/10.3389/fmars.2022.774407/full#supplementary-material
Abbreviations
ANOVA, Analysis of Variance; ANCOVA, Analysis of Covariance; CPUE, Catch Per Unit Effort; EFGB, East Flower Garden Bank; FGBNMS, Flower Garden Banks National Marine Sanctuary; GoM, Gulf of Mexico; NOAA, National Oceanic and Atmospheric Administration; QA/QC, Quality assurance quality control; TL, Total Length; TW, Total Weight; VB, von Bertalanffy; WFGB, West Flower Garden Bank; WLR, Weight-Length Relationship.
References
Aguilar-Perera, A., and Tuz-Sulub, A. (2010). Non-native, invasive red lionfish (Pterois volitans [Linnaeus, 1758]: Scorpaenidae), is first recorded in the southern Gulf of Mexico, off the northern Yucatan Peninsula, Mexico. Aqu. Invasions 5(Suppl. 1) S9–S12. doi: 10.3391/ai.2010.5.S1.003
Aguilar-Perera, A., Valle-Nava, J., Williams, E. H., and Bunkley-Williams, L. (2018). Rocinela signata (Isopoda: Aegidae) parasitizing Indo-Pacific lionfish Pterois volitans (Scorpaeniformes: Scorpaenidae) in the Southern Gulf of Mexico. Mar. Biol. Res. 14:4. doi: 10.1080/17451000.2018.1528012
Albins, M. A. (2013). Effects of invasive Pacific red lionfish Pterois volitans versus a native predator on Bahamian coral-reef fish communities. Biol. Invasions 15, 29–43. doi: 10.1007/s10530-012-0266-1
Albins, M. A., and Hixon, M. A. (2008). Invasive Indo-Pacific lionfish Pterois volitans reduce recruitment of Atlantic coral-reef fishes. Mar. Ecol. Prog. Ser. 367, 233–238. doi: 10.3354/meps07620
Albins, M. A., and Hixon, M. A. (2013). Worst case scenario: potential long-term effects of invasive predatory lionfish (Pterois volitans) on Atlantic and Caribbean coral-reef communities. Environ. Biol. Fish. 96, 1151–1157. doi: 10.1007/s10641-011-9795-1
Andradi-Brown, D. A. (2019). “Invasive lionfish (Pterois volitans and P. miles): distribution, impact, and management,” in Mesophotic Coral Ecosystems, ed. T. Bridge (Cham: Springer), 931–941. doi: 10.1007/978-3-319-92735-0_48
Andradi-Brown, D. A., Grey, R., Hendrix, A., Hitchner, D., Hunt, C. L., Gress, E., et al. (2017). Depth-dependent effects of culling-do mesophotic lionfish populations undermine current management? R. Soc. Open Sci. 4:170027. doi: 10.1098/rsos.170027
Angeler, D. G., Allen, C. R., Barichievy, C., Eason, T., Garmestani, A. S., Graham, N. A. J., et al. (2015). Management applications of discontinuity theory. J. Appl. Ecol. 53, 688–698. doi: 10.1111/1365-2664.12494
Arias-Gonzalez, J. E., Gonzalez-Gandara, C., Cabrera, J. L., and Christensen, V. (2011). Predicted impact of the invasive lionfish Pterois volitans on the food web of a Caribbean coral reef. Environ. Res. 111, 917–925. doi: 10.1016/j.envres.2011.07.008
Azzurro, E., Stancanelli, B., Di Martino, V., and Bariche, M. (2017). Range expansion of the common lionfish Pterois miles (Bennett, 1828) in the Mediterranean Sea: an unwanted new guest for Italian waters. Bioinvasions Rec. 6, 95–98. doi: 10.3391/bir.2017.6.2.01
Ballew, N. G., Bacheler, N. M., Kellison, G. T., and Schueller, A. M. (2016). Invasive lionfish reduce native fish abundance on a regional scale. Sci. Rep. 6:32169. doi: 10.1038/srep32169
Barbour, A. B., Allen, M. S., Frazer, T. K., and Sherman, K. D. (2011). Evaluating the potential efficacy of invasive lionfish (Pterois volitans) removals. PLoS One 6:e19666. doi: 10.1371/journal.pone.0019666
Bariche, M., Kleitou, P., Kalogirou, S., and Bernardi, G. (2017). Genetics reveal the identity and origin of the lionfish invasion in the Mediterranean Sea. Sci. Rep. 7:6782. doi: 10.1038/s41598-017-07326-1
Bejarano, S., Lohr, K., Hamilton, S., and Manfrino, C. (2014). Relationships of invasive lionfish with topographic complexity, groupers, and native prey fishes in Little Cayman. Mar. Biol. 162, 253–266. doi: 10.1007/s00227-014-2595-3
Benkwitt, C. E. (2014). Non-linear effects of invasive lionfish density on native coral-reef fish communities. Biol. Invasions 17:12. doi: 10.1007/s10530-014-0801-3
Berkeley, S. A., Hixon, M., Larson, R. J., and Love, M. S. (2004). Fisheries sustainability via protection of age structure and spatial distribution of fish populations. Fisheries 29:9.
Betancur-R, R., Hines, A., Acero, A., Orti, G., Wilbur, A. E., and Freshwater, D. W. (2011). Reconstructing the lionfish invasion: insights into Greater Caribbean biogeography. J. Biogeogr. 38, 1281–1293. doi: 10.1111/j.1365-2699.2011.02496.x
Beverton, R. J. H. (1954). “Notes on the use of theoretical models in the study of the dynamics of exploited fish populations,” in Marine Fisheries Section, American Fisheries Society, Special Publication 1. (Beaufort, NC: U.S. Fishery Laboratory).
Bianchi, G. (2000). Impact of fishing on size composition and diversity of demersal fish communities. ICES J. Mar. Sci. 57, 558–571. doi: 10.1006/jmsc.2000.0727
Biggs, C. R., and Olden, J. D. (2011). Multi-scale habitat occupancy of invasive lionfish (Pterois volitans) in coral reef environments of Roatan, Honduras. Aqua. Invasions 6, 347–353. doi: 10.3391/ai.2011.6.3.11
Blakeway, R., Fogg, A., and Jones, G. (2021). Oldest Indo-Pacific lionfish (Pterois volitans/P. miles) recorded from the Northwestern Gulf of Mexico. Gulf Caribbean Res. 32, GCFI1–GCFI4. doi: 10.18785/gcr.3201.01
Campana, S. E. (1999). Chemistry and composition of fish otoliths: pathways, mechanisms, and applications. Mar. Ecol. Prog. Ser. 188:35.
Campbell, M. D., Pollack, A. G., Thompson, K., Switzer, T., Driggers, W. B., Hoffmayer, E. R., et al. (2022). Rapid spatial expansion and population increase of invasive lionfish (Pterois spp.) observed on natural habitats in the northern Gulf of Mexico. Biol. Invasions 24, 93–105. doi: 10.1007/s10530-021-02625-1
Chaffin, B. C., Garmestani, A. S., Angeler, D. G., Herrmann, D. L., Stow, C. A., Nystrom, M., et al. (2016). Biological invasions, ecological resilience and adaptive governance. J. Environ. Manage. 183(Pt 2) 399–407. doi: 10.1016/j.jenvman.2016.04.040
Claydon, J. A. B., Calosso, M. C., and Traiger, S. B. (2012). Progression of invasive lionfish in seagrass, mangrove and reef habitats. Mar. Ecol. Prog. Ser. 448, 119–129. doi: 10.3354/meps09534
Cook, D. C., Liu, S., Murphy, B., and Lonsdale, W. M. (2010). Adaptive approaches to biosecurity governance. Soc. Risk Anal. 30:11. doi: 10.1111/j.1539-6924.2010.01439.x
Cope, J. M. (2013). Implementing a statistical catch-at-age model (Stock Synthesis) as a tool for deriving overfishing limits in data-limited situations. Fish. Res. 142, 11.
Cote, I. M., Green, S. J., and Hixon, M. A. (2013a). Predatory fish invaders: Insights from Indo-Pacific lionfish in the western Atlantic and Caribbean. Biol. Conserv. 164, 50–61. doi: 10.1016/j.biocon.2013.04.014
Cote, I. M., Green, S. J., Morris, J. A., Akins, J. L., and Steinke, D. (2013b). Diet richness of invasive Indo-Pacific lionfish revealed by DNA barcoding. Mar. Ecol. Prog. Ser. 472, 249–256. doi: 10.3354/meps09992
Dahl, K. A., Edwards, M. A., and Patterson, W. F. (2019). Density-dependent condition and growth of invasive lionfish in the northern Gulf of Mexico. Mar. Ecol. Prog. Ser. 623, 145–159. doi: 10.3354/meps13028
Dahl, K. A., and Patterson, W. F. (2020). Movement, home range, and depredation of invasive lionfish revealed by fine-scale acoustic telemetry in the northern Gulf of Mexico. Mar. Biol. 167:111. doi: 10.1007/s00227-020-03728-4
Dahl, K. A., and Patterson, W. F. III (2014). Habitat-specific density and diet of rapidly expanding invasive red lionfish, Pterois volitans, populations in the northern Gulf of Mexico. PLoS One 9:e105852. doi: 10.1371/journal.pone.0105852
Dahl, K. A., Patterson, W. F., and Snyder, R. A. (2016). Experimental assessment of lionfish removals to mitigate reef fish community shifts on northern Gulf of Mexico artificial reefs. Mar. Ecol. Prog. Ser. 558, 207–221. doi: 10.3354/meps11898
Dance, M. A., Patterson Iii, W. F., and Addis, D. T. (2011). Fish community and trophic structure at artificial reef sites in the Northeastern Gulf of Mexico. Bull. Mar. Sci. 87, 301–324. doi: 10.5343/bms.2010.1040
Darling, E. S., Green, S. J., O’Leary, J. K., and Cote, I. M. (2011). Indo-Pacific lionfish are larger and more abundant on invaded reefs: a comparison of Kenyan and Bahamian lionfish populations. Biol. Invasions 13:7. doi: 10.1007/s10530-011-0020-0
Davies, S. W., Strader, M. E., Kool, J. T., Kenkel, C. D., and Matz, M. V. (2017). Modeled differences of coral life-history traits influence the refugium potential of a remote Caribbean reef. Coral Reefs 36:12. doi: 10.1007/s00338-017-1583-8
de León, R., Vane, K., Bertuol, P., Chamberland, V. C., Simal, F., Imms, E., et al. (2013). Effectiveness of lionfish removal efforts in the southern Caribbean. Endangered Species Res. 22, 175–182. doi: 10.3354/esr00542
Done, T. J. (1992). Phase shifts in coral reef communities and their ecological significance. Hydrobiologia 247:11. doi: 10.1007/BF00008211
Eddy, C., Pitt, J., Morris, J. A., Smith, S., Goodbody-Gringley, G., and Bernal, D. (2016). Diet of invasive lionfish (Pterois volitans and P. miles) in Bermuda. Mar. Ecol. Prog. Ser. 558, 193–206. doi: 10.3354/meps11838
Eddy, C., Pitt, J., Oliveira, K., Morris, J. A., Potts, J., and Bernal, D. (2019). The life history characteristics of invasive lionfish (Pterois volitans and P. miles) in Bermuda. Environ. Biol. Fish. 102, 887–900. doi: 10.1007/s10641-019-00877-4
Edwards, M. A., Frazer, T. K., and Jacoby, C. A. (2014). Age and growth of invasive lionfish (Pterois spp.) in the Caribbean Sea, with implications for management. Bull. Mar. Sci. 90, 953–966. doi: 10.5343/bms.2014.1022
Elise, S., Urbina-Barreto, I., Boadas-Gil, H., Galindo-Vivas, M., and Kulbicki, M. (2015). No detectable effect of lionfish (Pterois volitans and P. miles) invasion on a healthy reef fish assemblage in Archipelago Los Roques National Park, Venezuela. Mar. Biol. 162:11. doi: 10.1007/s00227-014-2571-y
New World Encyclopedia (2021). Gulf of Mexico [Online]. Available online at: https://www.newworldencyclopedia.org/entry/Gulf_of_Mexico (accessed October 22 2021).
Farquhar, S. D. (2016). “Age and growth of invasive lionfish: North Carolina, USA vs Bonaire, Dutch Caribbean,” in Proceedings of the 69th Gulf and Caribbean Fisheries Institute, Grand Cayman.
Ferreira, C. E., Luiz, O. J., Floeter, S. R., Lucena, M. B., Barbosa, M. C., Rocha, C. R., et al. (2015). First record of invasive lionfish (Pterois volitans) for the Brazilian coast. PLoS One 10:e0123002. doi: 10.1371/journal.pone.0123002
Fogg, A. Q. (2017). Life History of the Non-native Invasive Red Lionfish (Pterois volitans) in the Northern Gulf of Mexico. Master of Science. Hattiesburg, MS: University of Southern Mississippi.
Fogg, A. Q., Brown-Peterson, N. J., and Peterson, M. S. (2017). Reproductive life history characteristics of invasive red lionfish (Pterois volitans) in the northern Gulf of Mexico. Bull. Mar. Sci. 93:23. doi: 10.5343/bms.2016.1095
Fogg, A. Q., Evans, J. T., Peterson, M. S., Brown-Peterson, N. J., Hoffmayer, E. R., and Ingram, G. W. (2019). Comparison of age and growth parameters of invasive red lionfish (Pterois volitans) across the northern Gulf of Mexico. Fish. Bull. 117, 1–15. doi: 10.7755/fb.117.3.1
Fogg, A. Q., Ruiz, C. F., Curran, S. S., and Bullard, S. A. (2016). Parasites from the red lionfish, Pterois volitans from the Gulf of Mexico. Gulf Caribbean Res. 27:SC1–SC5. doi: 10.18785/gcr.2701.07
Foxcroft, L. C., and McGeoch, M. (2011). Implementing invasive species management in an adaptive management framework. Koedoe 53:1049. doi: 10.4102/koedoe.v53i2.1006
Frazer, T. K., Jacoby, C. A., Edwards, M. A., Barry, S. C., and Manfrino, C. M. (2012). Coping with the lionfish invasion: can targeted removals yield beneficial effects? Rev. Fish. Sci. 20, 185–191. doi: 10.1080/10641262.2012.700655
Garavelli, L., Studivan, M. S., Voss, J. D., Kuba, A., Figueiredo, J., and Chérubin, L. M. (2018). Assessment of mesophotic coral ecosystem connectivity for proposed expansion of a marine sanctuary in the Northwest Gulf of Mexico: larval dynamics. Front. Mar. Sci. 5:174. doi: 10.3389/fmars.2018.00174
Gardner, P. G., Frazer, T. K., Jacoby, C. A., and Yanong, R. P. E. (2015). Reproductive biology of invasive lionfish (Pterois spp.). Front. Mar. Sci. 2:7. doi: 10.3389/fmars.2015.00007
Garmestani, A. S., and Benson, M. H. (2013). A framework for resilience-based governance of social-ecological systems. Ecol. Soc. 18:9. doi: 10.5751/es-05180-180109
Gil-Agudelo, D. L., Cintra-Buenrostro, C. E., Brenner, J., González-Díaz, P., Kiene, W., Lustic, C., et al. (2020). Coral reefs in the Gulf of mexico large marine ecosystem: conservation status, challenges, and opportunities. Front. Mar. Sci. 6:807. doi: 10.3389/fmars.2019.00807
Goodbody-Gringley, G., Eddy, C., Pitt, J. M., Chequer, A. D., and Smith, S. R. (2019). Ecological drivers of invasive lionfish (Pterois volitans and Pterois miles) distribution across mesophotic reefs in Bermuda. Front. Mar. Sci. 6:258. doi: 10.3389/fmars.2019.00258
Green, S. J., Akins, J. L., Maljkovic, A., and Cote, I. M. (2012a). Invasive lionfish drive Atlantic coral reef fish declines. PLoS One 7:e32596. doi: 10.1371/journal.pone.0032596
Green, S. J., Tamburello, N., Miller, S. E., Akins, J. L., and Côté, I. M. (2012c). Habitat complexity and fish size affect the detection of Indo-Pacific lionfish on invaded coral reefs. Coral Reefs 32, 413–421. doi: 10.1007/s00338-012-0987-8
Green, S. J., Akins, J. L., and Morris, J. (2012b). Lionfish Dissection: Techniques and Applications. NOAA Technical Memorandum NOS NCCOS. Beaufort, NC: NOAA’s National Centers for Coastal Ocean Science.
Green, S. J., and Cote, I. M. (2009). Record densities of Indo-Pacific lionfish on Bahamian coral reefs. Coral Reefs 28:1. doi: 10.1007/s00338-008-0446-8
Grubich, J. R., Westneat, M. W., and McCord, C. L. (2009). Diversity of lionfishes (Pisces: Scorpaenidae) among remote coral reefs of the Palau Archipelago. Coral Reefs 28, 807–807. doi: 10.1007/s00338-009-0510-z
Hackerott, S., Valdivia, A., Cox, C. E., Silbiger, N. J., and Bruno, J. F. (2017). Invasive lionfish had no measurable effect on prey fish community structure across the Belizean Barrier Reef. PeerJ 5:e3270. doi: 10.7717/peerj.3270
Hackerott, S., Valdivia, A., Green, S. J., Cote, I. M., Cox, C. E., Akins, L., et al. (2013). Native predators do not influence invasion success of pacific lionfish on Caribbean reefs. PLoS One 8:e68259. doi: 10.1371/journal.pone.0068259
Harris, H. E., Fogg, A. Q., Allen, M. S., Ahrens, R. N. M., and Patterson, W. F. (2020). Precipitous declines in Northern Gulf of Mexico invasive lionfish populations following the emergence of an ulcerative skin disease. Sci. Rep. 10:1934. doi: 10.1038/s41598-020-58886-8
Harris, H. E., Patterson, W. F., Ahrens, R. N. M., and Allen, M. S. (2019). Detection and removal efficiency of invasive lionfish in the northern Gulf of Mexico. Fish. Res. 213, 22–32. doi: 10.1016/j.fishres.2019.01.002
Hickerson, E. L., and Schmahl, G. P. (2005). The State of Coral Reef Ecosystems of the Flower Garden Banks, Stetson Bank and Other Banks in the Northwestern Gulf of Mexico. Silver Spring, MD: NOAA Technical Memorandum NOS NCCOS.
Holling, C. S. (1973). Resilience and stability of ecological systems. Annu. Rev. Ecol. Syst. 4:24. doi: 10.1146/annurev.es.04.110173.000245
Hughes, T. P. (1994). Catastrophes, phase shifts, and large-scale degredation of a caribbean coral reef. Science 265:5. doi: 10.1126/science.265.5178.1547
Hunter, M. E., Beaver, C. E., Johnson, N. A., Bors, E. K., Mignucci-Giannoni, A. A., Silliman, B. R., et al. (2021). Genetic analysis of red lionfish Pterois volitans from Florida, USA, leads to alternative North Atlantic introduction scenarios. Mar. Ecol. Prog. Ser. 675, 133–151. doi: 10.3354/meps13841
Johnson, E. G., and Swenarton, M. K. (2016). Age, growth and population structure of invasive lionfish (Pterois volitans/miles) in northeast Florida using a length-based, age-structured population model. PeerJ 4:e2730. doi: 10.7717/peerj.2730
Johnson, J., Bird, C. E., Johnston, M. A., Fogg, A. Q., and Hogan, J. D. (2016). Regional genetic structure and genetic founder effects in the invasive lionfish: comparing the Gulf of Mexico, Caribbean and North Atlantic. Mar. Biol. 163:216. doi: 10.1007/s00227-016-2981-0
Johnston, M. A., Hickerson, E. L., Nuttall, M. F., Blakeway, R. D., Sterne, T. K., Eckert, R. J., et al. (2019). Coral bleaching and recovery from 2016 to 2017 at East and West Flower Garden Banks, Gulf of Mexico. Coral Reefs 38, 787–799. doi: 10.1007/s00338-019-01788-7
Johnston, M. A., Nuttall, M. F., Eckert, R. J., Embesi, J. A., Sterne, T. K., Hickerson, E. L., et al. (2016b). Rapid invasion of Indo-Pacific lionfishes Pterois volitans (Linnaeus, 1758) and P. miles (Bennett, 1828) in Flower Garden Banks National Marine Sanctuary, Gulf of Mexico, documented in multiple data sets. BioInvasions Rec. 5:7. doi: 10.3391/bir.2016.5.2.09
Johnston, M. A., Lemm, A., Hogan, D., Nuttall, M. F., Walker, R. D., Hickerson, E. L., et al. (2016a). “The effects of lionfish at Flower Garden Banks National Marine Sanctuary in the Northwest Gulf of Mexico,” in Proceedings of the 68th Annual Gulf of Caribbean Fisheries Institute (Panama), 386.
Johnston, M. A., O’Connell, K., Blakeway, R. D., MacMillan, J., Nuttall, M. F., Hu, X., et al. (2021). Long-Term Monitoring at East and West Flower Garden Banks: 2019 Annual Report. Galveston, TX: National Marine Sanctuaries Conservation Series.
Johnston, M. A., Sterne, T. K., Blakeway, R. D., MacMillan, J., Nuttall, M. F., Hu, J. A., et al. (2018). Long-Term Monitoring at East and West Flower Garden Banks: 2017 Annual Report. Galveston, TX: Marine Sanctuaries Conservation Series.
Jud, Z. R., Nichols, P. K., and Layman, C. A. (2015). Broad salinity tolerance in the invasive lionfish Pterois spp. may facilitate estuarine colonization. Environ. Biol. Fish. 98, 135–143. doi: 10.1007/s10641-014-0242-y
Kimball, M. E., Miller, J. M., Whitfield, P. E., and Hare, J. A. (2004). Thermal tolerance and potential distribution of invasive lionfish (Pterois volitans/miles complex) on the east coast of the United States. Mar. Ecol. Prog. Ser. 283, 269–278. doi: 10.3354/meps283269
Kindsvater, H. K., Mangel, M., Reynolds, J. D., and Dulvy, N. K. (2016). Ten principles from evolutionary ecology essential for effective marine conservation. Ecol. Evol. 6, 2125–2138. doi: 10.1002/ece3.2012
Kitchens, L. L., Paris, C. B., Vaz, A. C., Ditty, J. G., Cornic, M., Cowan, J. H., et al. (2017). Occurrence of invasive lionfish (Pterois volitans) larvae in the northern Gulf of Mexico: characterization of dispersal pathways and spawning areas. Biol. Invasions 19, 1971–1979. doi: 10.1007/s10530-017-1417-1
Kulbicki, M., Beets, J., Chabanet, P., Cure, K., Darling, E., Floeter, S. R., et al. (2012). Distributions of Indo-Pacific lionfishes Pterois spp. in their native ranges: implications for the Atlantic invasion. Mar. Ecol. Prog. Ser. 446, 189–205. doi: 10.3354/meps09442
Lesser, M., and Slattery, M. (2011). Phase shift to algal dominated communities at mesophotic depths associated with lionfish (Pterois volitans) invasion on a Bahamian coral reef. Biol. Invasions 13:13. doi: 10.1007/s10530-011-0005-z
Limer, B. D., Bloomberg, J., and Holstein, D. M. (2020). The influence of eddies on coral larval retention in the flower garden banks. Front. Mar. Sci. 7:372. doi: 10.3389/fmars.2020.00372
Luckhurst, B. E., Barnes, J. A., and Sadovy, Y. (1992). Record of an unusually large Red Hind, Epinephelus guttatus (Pisces: Serranidae) from Bermuda with comments on its age. Bull. Mar. Sci. 51:4.
Maljkovic, A., van Leeuwen, T. E., and Cove, S. N. (2008). Predation on the invasive red lionfish, Pterois volitans (Pisces : Scorpaenidae), by native groupers in the Bahamas. Coral Reefs 27, 501–501. doi: 10.1007/s00338-008-0372-9
Marshak, A. R., Heck, K. L. Jr., and Jud, Z. R. (2018). Ecological interactions between Gulf of Mexico snappers (Teleostei: Lutjanidae) and invasive red lionfish (Pterois volitans). PLoS One 13:e0206749. doi: 10.1371/journal.pone.0206749
McLeod, E., Anthony, K. R. N., Mumby, P. J., Maynard, J., Beeden, R., Graham, N. A. J., et al. (2019). The future of resilience-based management in coral reef ecosystems. J. Environ. Manage. 233, 291–301. doi: 10.1016/j.jenvman.2018.11.034
Montoya-Mendoza, J., Camarena-Luhrs, T., Castañeda-Chávez, M. R., and Lango-Reynoso, F. (2017). Helminth parasites of Red Lionfish, Pterois volitans from the Veracruz coral reef system, Mexico, Southern Gulf of Mexico. J. Agric. Sci. 9:30. doi: 10.5539/jas.v9n11p30
Morris, J. A., and Akins, J. L. (2009). Feeding ecology of invasive lionfish (Pterois volitans) in the Bahamian archipelago. Environ. Biol. Fish. 86, 389–398. doi: 10.1007/s10641-009-9538-8
Morris, J. A., Shertzer, K. W., and Rice, J. A. (2011). A stage-based matrix population model of invasive lionfish with implications for control. Biol. Invasions 13:5. doi: 10.1007/s10530-010-9786-8
Morris, J. A., and Whitfield, P. E. (2009). Biology, Ecology, Control and Management of the Invasive Indo-Pacific Lionfish: An Updated Integrated Assessment. Washington, DC: NOAA.
Mumby, P. J., Steneck, R. S., and Hastings, A. (2013). Evidence for and against the existence of alternate attractors on coral reefs. Oikos 122, 481–491. doi: 10.1111/j.1600-0706.2012.00262.x
Nuttall, M. F., Johnston, M. A., Eckert, R. J., Embesi, J. A., Hickerson, E. L., and Schmahl, G. P. (2014). Lionfish (Pterois volitans [Linnaeus, 1758] and P. miles [Bennett, 1828]) records within mesophotic depth ranges on natural banks in the Northwestern Gulf of Mexico. Bioinvasions Rec. 3, 111–115. doi: 10.3391/bir.2014.3.2.09
Patterson, W. F., Tarnecki, J. H., Addis, D. T., and Barbieri, L. R. (2014). “Reef fish community structure at natural versus artificial reefs in the Northern Gulf of Mexico,” in Proceedings of the 66th Gulf and Caribbean Fisheries Institute (Corpus Christi, TX).
Peake, J., Bogdanoff, A. K., Layman, C. A., Castillo, B., Reale-Munroe, K., Chapman, J., et al. (2018). Feeding ecology of invasive lionfish (Pterois volitans and Pterois miles) in the temperate and tropical western Atlantic. Biol. Invasions 20, 2567–2597. doi: 10.1007/s10530-018-1720-5
Perez-Portela, R., Bumford, A., Coffman, B., Wedelich, S., Davenport, M., Fogg, A., et al. (2018). Genetic homogeneity of the invasive lionfish across the Northwestern Atlantic and the Gulf of Mexico based on single nucleotide polymorphisms. Sci. Rep. 8:5062. doi: 10.1038/s41598-018-23339-w
Pope, K. L., Lochman, S. E., and Young, M. K. (2010). “Methods for assessing fish populations,” in Inland Fisheries Management in North America, 3rd Edn, eds W. A. Hubert and M. C. Quist (Bethesda, MD: American Fisheries Society), 325–351.
Pusack, T. J., Benkwitt, C. E., Cure, K., and Kindinger, T. L. (2016). Invasive Red Lionfish (Pterois volitans) grow faster in the Atlantic Ocean than in their native Pacific range. Environ. Biol. Fish. 99:8. doi: 10.1007/s10641-016-0499-4
Raymond, W. W., Albins, M. A., and Pusack, T. J. (2015). Competitive interactions for shelter between invasive Pacific red lionfish and native Nassau grouper. Environ. Biol. Fishes 98, 57–65. doi: 10.1007/s10641-014-0236-9
Federal Register (1991). Flower Garden Banks National Marine Sanctuary Regulations. Washington, DC: NOAA.
Federal Register (2021). Expansion of Flower Garden Banks National Marine Sanctuary. Washington, DC: NOAA.
Robertson, D. R., Ackerman, J. L., Choat, J. H., Posada, J. M., and Pitt, J. (2005). Ocean surgeonfish Acanthurus bahianus. I. The geography of demography. Mar. Ecol. Prog. Ser. 295:16.
Rodríquez-Cortés, K. D., Aquilar-Perera, A., and Bonilla-Gómez, J. L. (2015). Growth and mortality of red lionfish, Pterois volitans (Actinopterygii: Scorpaeniformes: Scorpaenidae), in the Parque Nacional Arrecife Alacranes, southern Gulf of Mexico, as determined by size-frequency analysis. Acta Ichthyol. Piscatoria 45, 175–179. doi: 10.3750/aip2015.45.2.07
Rooker, J. R., Dokken, Q. R., Pattengill, C. V., and Holt, G. J. (1997). Fish assemblages on artificial and natural reefs in the Flower Garden Banks National Marine Sanctuary, USA. Coral Reefs 16:9. doi: 10.1007/s003380050062
Schmahl, G. P., Hickerson, E. L., and Precht, W. F. (2008). “Biology and ecology of coral reefs and coral communities in the flower garden banks region, Northwestern Gulf of Mexico,” in Coral Reefs of the USA, eds B. M. Riegl and R. E. Dodge (Berlin: Springer Science Business Media B.V), 811.
Schnute, J., and Fournier, D. (1980). A new approach to length-frequency analysis: Growth structure. Can. J. Fish. Aqu. Sci. 37:14.
Schofield, P. J. (2010). Update on geographic spread of invasive lionfishes (Pterois volitans [Linnaeus, 1758] and P. miles [Bennett, 1828]) in the Western North Atlantic Ocean, Caribbean Sea and Gulf of Mexico. Aqu. Invasions 5, S117–S122. doi: 10.3391/ai.2010.5.S1.024
Sellers, A. J., Ruiz, G. M., Leung, B., and Torchin, M. E. (2015). Regional variation in parasite species richness and abundance in the introduced range of the invasive lionfish, Pterois volitans. PLoS One 10, e0131075. doi: 10.1371/journal.pone.0131075
Semmens, B. X., Buhle, E. R., Salomon, A. K., and Pattengill-Semmens, C. V. (2004). A hotspot on non-native marine fishes: evidence for the aquarium trade as an invasion pathway. Mar. Ecol. Prog. Ser. 266:5.
Simmons, K. R. (2014). Evidence of the Enemy Release Hypothesis: Parasites of the Lionfish Complex (Pterois volitans and P.miles) in the Western North Atlantic, Gulf of Mexico, and Caribbean Sea. Master’s of Science. Hollywood, FL: Nova Southeastern University Oceanographic Center.
Sosa-Cordero, E., Malca, E., Brito, A., and Hernández, N. (2013). Torneos de Pesca: Estimando la Densidad del pez León. Miama, FL: Univeristy ofyb Miami-CIMAS.
Switzer, T. S., Tremain, D. M., Keenan, S. F., Stafford, C. J., Parks, S. L., and McMichael, R. H. (2015). Temporal and spatial dynamics of the lionfish invasion in the Eastern Gulf of Mexico: perspectives from a broadscale trawl survey. Mar. Coastal Fish. 7, 1–8. doi: 10.1080/19425120.2014.987888
Toledo-Hernández, C. (2014). Population ecology and genetics of the invasive lionfish in Puerto Rico. Aqu. Invasions 9, 227–237. doi: 10.3391/ai.2014.9.2.12
Tu, C. Y., Chen, K. T., and Hsieh, C. H. (2018). Fishing and temperature effects on the size structure of exploited fish stocks. Sci. Rep. 8:7132. doi: 10.1038/s41598-018-25403-x
Ulman, A., Harris, H. E., Doumpas, N., Deniz Akbora, H., Al Mabruk, S. A. A., Azzurro, E., et al. (2021). Low pufferfish and lionfish predation in their native and invaded ranges suggests human control mechanisms may be necessary to control their mediterranean abundances. Front. Mar. Sci. 8:413. doi: 10.3389/fmars.2021.670413
Valdivia, A., Bruno, J. F., Cox, C. E., Hackerott, S., and Green, S. J. (2014). Re-examining the relationship between invasive lionfish and native grouper in the Caribbean. PeerJ 2:e348. doi: 10.7717/peerj.348
von Bertalanffy, L. (1957). Quantitative laws in metabolism and growth. Q. Rev. Biol. 32:14. doi: 10.1086/401873
Wetmore, L. S., Dance, M. A., Hill, R. L., and Rooker, J. R. (2020). Community dynamics of fish assemblages on mid-shelf and outer-shelf coral reefs in the Northwestern Gulf of Mexico. Front. Mar. Sci. 7:152. doi: 10.3389/fmars.2020.00152
Keywords: invasive species, coral reef, age and growth, density, otolith, ecological resilience
Citation: Blakeway RD, Fogg AQ, Johnston MA, Rooker JR and Jones GA (2022) Key Life History Attributes and Removal Efforts of Invasive Lionfish (Pterois volitans) in the Flower Garden Banks National Marine Sanctuary, Northwestern Gulf of Mexico. Front. Mar. Sci. 9:774407. doi: 10.3389/fmars.2022.774407
Received: 11 September 2021; Accepted: 13 January 2022;
Published: 03 February 2022.
Edited by:
Pedro Morais, Florida International University, United StatesReviewed by:
Joanna Maria Pitt, The Department of Environment and Natural Resources, BermudaAlfonso Aguilar-Perera, Universidad Autónoma de Yucatán, Mexico
Copyright © 2022 Blakeway, Fogg, Johnston, Rooker and Jones. This is an open-access article distributed under the terms of the Creative Commons Attribution License (CC BY). The use, distribution or reproduction in other forums is permitted, provided the original author(s) and the copyright owner(s) are credited and that the original publication in this journal is cited, in accordance with accepted academic practice. No use, distribution or reproduction is permitted which does not comply with these terms.
*Correspondence: Raven D. Blakeway, cndhbGtlMDlAZW1haWwudGFtdS5lZHU=