- 1School of Science, Walailak University, Nakhon Si Thammarat, Thailand
- 2Reef Biology Research Group, Department of Marine Science, Faculty of Science, Chulalongkorn University, Bangkok, Thailand
- 3Department of Pharmacognosy, Faculty of Pharmaceutical Sciences, Chulalongkorn University, Bangkok, Thailand
- 4Aquatic Resources Research Institute, Chulalongkorn University, Bangkok, Thailand
- 5Center of Excellence for Marine Biotechnology, Department of Marine Science, Faculty of Science, Chulalongkorn University, Bangkok, Thailand
Allelopathy is commonly found in marine sponges. However, information on allelopathic activities of sponge extracts is limited. In this study, variations of nutritional values of Xestospongia sp. were investigated. Xestospongia did not unequally distribute its nutritional values for predatory defense. Carbon–nitrogen contents and renieramycin M concentrations were not significantly different among the areas of Xestospongia sp. (edge, inner, and outer). However, carbon–nitrogen contents and renieramycin M concentrations of Xestospongia sp. coexisting with different organisms were not significantly different. In the laboratory, bleaching and necrosis of coral nubbins were not observed in every treatment, and renieramycin M did not show any allelopathic effect on its coexisting organisms (Porites lutea and Palythoa caesia). However, renieramycin M inhibited settlement of acorn barnacle (Semibalanus balanoides) but did not inhibit the settlement of pelecypods. When testing for antimicrobial effect, renieramycin M did not inhibit the growth of aerobic bacteria in any treatments. This study provides evidence that renieramycin M found in Xestospongia was uniform; however, it can possess strong inhibitory activities against some marine organisms.
Introduction
Sponges are one of the common marine organisms in tropical areas and are known to produce diverse secondary metabolites (Faulkner, 1984). Some secondary metabolites and chemical compounds produced by sponges have been intensively screened as marine natural products (Frincke and Faulkner, 1982; Nakao et al., 2004). They are also known as inhibitors of bacteria and antileishmania (Frincke and Faulkner, 1982; Nakao et al., 2004). The blue sponge, Xestospongia sp., can be found worldwide (Pulitzer—Finali, 1996; Kritsanapuntu et al., 2001; Darumas et al., 2007). In Thailand, Xestospongia sp. is found commonly in both the Gulf of Thailand and the Andaman Sea coexisting with other reef organisms (e.g., corals, algae, and other sponges) and settling on rock beds and dead coral rubble (Darumas et al., 2007). This sponge differs from renierid, cribrochalinid, and neopetrosid sponges by its ectosomal skeleton with a tangential disordered network and no specialized structure (Darumas et al., 2007). In coral reef communities, sponge species have been found to successfully compete with corals (Vicente, 1990). They are also well-known space competitors in the reefs (Aerts and Van Soest, 1997). Factors influencing the outcomes of the competitive interactions include distances between species and chemical defense mechanisms (Lang and Chornesky, 1990; Nakao et al., 2004). The chemical defense of secondary metabolites can serve as antipredatory, antimicrobial, antifouling, and allelopathy agents (Wang et al., 2008).
Allelopathy is the beneficial or harmful effect of one organism on another organism by the release of chemicals from body part(s) by leaching, root exudation (in plants), volatilization, residue decomposition, and other processes (Folt and Goldman, 1981; Willis, 2007). The term “allelopathy” has been borrowed by zoologists to describe chemical-based interactions among invertebrates, especially sponges and corals (Willis, 2007). Allelopathy is also common in marine habitats. Some cyanobacteria produce chemicals to inhibit the growth of algae (Suikkanen et al., 2004). The same multifunctional roles of allelopathic chemicals were observed in marine habitats as well (Kubanek et al., 2002). For example, hexanic extracted from soft coral, Stereonephthya aff. curvata, caused serious necrosis on gorgonians and deterred fish feeding (Lages et al., 2006). The allelochemicals that prevented space settlement of either intra or interspecific larva were known as antifouling agents. These inhibited larval settlement (pre-settlement allelopathic) or killed the new settlers (post-settlement allelopathic) (Sears et al., 1990; Hirota et al., 1998). However, more complex antifouling activity was reported. Some chemicals prevent settlement of specific species, while some species benefit from settlement of others on them, thus producing the chemicals promotes settlement of specific species (Henrikson and Pawlik, 1995).
One of chemical compounds that Xestospongia produced is renieramycin (Suwanborirux et al., 2003). Renieramycins are also produced by several species of sponge such as Reniera sp., Haliclona cribricutis cribricutis, Cribrochalina sp., and Neopetrosia sp./spp. (Frincke and Faulkner, 1982; Parameswaran et al., 1998; Pettit et al., 2000; Oku et al., 2003; Nakao et al., 2004; Van Soest et al., 2005). They have potent cytotoxicities against cancer cell lines (Suwanborirux et al., 2003; Amnuoypol et al., 2004). Structures (Types) of renieramycins have been modified and documented (Amnuoypol et al., 2004). However, the natural structure, but easily degraded, was renieramycin E because the hydroxyl function group causes this compound to be unstable (Suwanborirux et al., 2003). The protocol to make renieramycin E more stable is to replace the hydroxyl function group with a cyanide functional group to become renieramycin M (Suwanborirux et al., 2003). This protocol allowed the high-yield extraction of renieramycin (Suwanborirux et al., 2003). However, the ecological roles of these compounds are less well understood.
The present study aimed to investigate the variation of nutritional values [carbon (C), hydrogen (H), and nitrogen (N)] and renieramycin M concentrations in different areas within the individuals of Xestospongia coexisting with Porites and Palythoa. Xestospongia was expected to face intensive predatory and competitive pressures because of the high diversity in the coral reef community. Thus, nutritional values that are essential sources of energy and growth of Xestospongia may exhibit differently in different parts of Xestospongia. In addition, the necrotic effect of renieramycin M on Xestospongia’s coexisting species, Porites, and its effect on settlement of fouling organisms were investigated.
Materials and Methods
In this study, C, H, and N contents and renieramycin M concentrations were investigated in different areas within the individuals of Xestospongia. The allelopathic effects of renieramycin M on Portes bleaching, larval settlement, and aerobic bacteria were examined.
Carbon, Hydrogen, Nitrogen Contents and Allocations of Renieramycin M in Xestospongia
Sample Collection
Fifteen specimens of Xestospongia coexisting with Porites lutea (thereafter Porites) (Phylum Cnidaria; Class Anthozoa; Family Poritidae) and 15 sponge specimens coexisting with Palythoa caesia (thereafter Palythoa) (Phylum Cnidaria; Class Anthozoa; Family Zoanthidae) were collected from Ran Dok Mai Island (13°09′05.35″N, 100°50′01.41″E) by scuba diving. Each sponge specimen was separated into three areas: the outer area (blue color); the inner area (yellowish-gray color), and the edge of the sponge. The definition of each area was as follows: the outer area was about 1 mm thick of the sponge’s upper surface that was totally blue in color; the inner area was about 1 mm thick of the sponge’s surface attached to the substrate that was totally yellowish-gray; the edge was about 1 mm thick of the sponge’s surface attached to the living tissue of the coexisting organisms (herein were Porites and Palythoa) that was located at the edge of the sponges and mostly were bluish-gray in color (Figure 1). To reduce the amount of saltwater, each specimen was cut into 2-cm2-sized pieces and dried with tissue paper for a minute, twice. The semidried specimens were placed in plastic bags in an icebox during transportation to the lab. The specimens were then stored at -20°C until extraction.
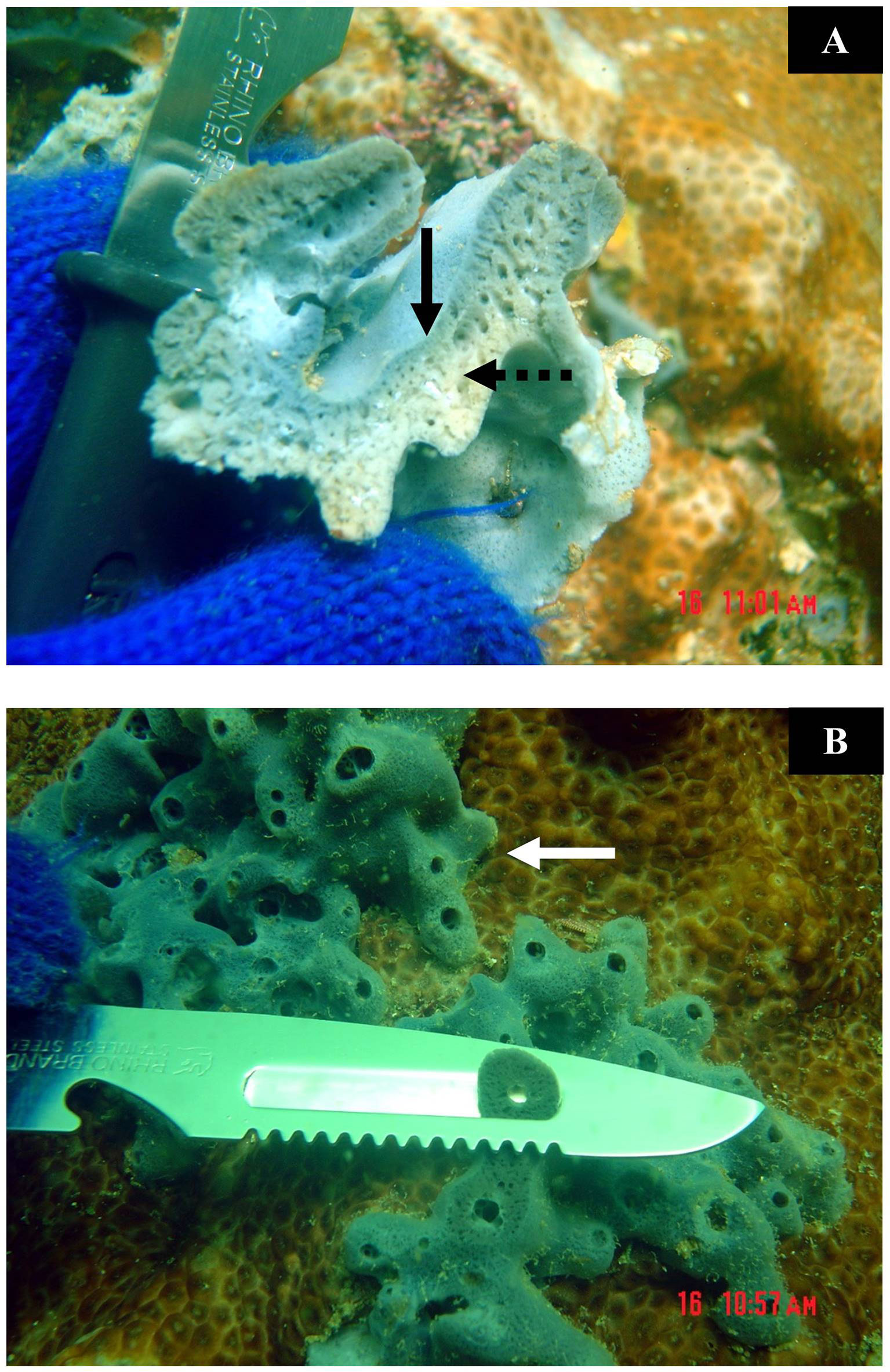
Figure 1 (A) The outer area (solid arrow) and the inner area (dot arrow) of Xestospongia. (B) The edge (solid arrow) of Xestospongia and the cutting method.
Elemental Analysis
The samples were analyzed for C, H, and N contents (percent of dry weight) by Dynamic Flash Combustion Technique. The instrument used was the CE Instruments Flash 1112 Series EA CHNS-O Analyzer at the Scientific Equipment Center, Prince of Songkla University. The analyzing condition was as follows: furnace temperature: 900°C; oven temperature: 65°C; carrier flow: 130 ml/min; reference flow: 100 ml/min; oxygen flow: 250 ml/min. Before the analysis, the samples were freeze-dried and then fumed with hydrochloric acid to remove inorganic C.
Renieramycin M Measurement
For crude extract preparation, all samples were lyophilized for 12 h using a freeze dryer. Each sample was accurately weighed at 100.0 mg for extraction. The sample was macerated with 10 mM potassium cyanide in phosphate buffer solution (6 ml, pH 7.0) for 5 h. Then, the suspension was extracted with methanol (24 ml) for 1 h. After centrifugation at 5,000 rpm for 5 min, the supernatant (3 ml) was partitioned with ethyl acetate (9 ml) and a brine solution (6 ml). The ethyl acetate layer (3 ml) was evaporated until dry. The dried residue was dissolved in methanol (1 ml) containing 300 ng acenapthene as an internal standard. The sample solutions were filtered through 0.45-nm nylon syringe tip filters before the high-performance liquid chromatographic (HPLC) analysis. All procedures were conducted by following the university safety protocol.
To calibrate the standard solution, standard renieramycin M derived from Xestospongia sp. in the Gulf of Thailand was obtained from Dr. Khanit Suwanborirux, Center of Bioactive Natural Products from Marine Organisms and Endophytic Fungi (BNPME). A stock standard solution of renieramycin M at a concentration of 1 mg/ml was prepared in methanol. Calibration solutions containing 2.4, 4.8, 9.0, 180, 375, 750, and 1,500 ng/ml were prepared by appropriate dilutions of the stock solution with methanol containing 800 ng acetonaphthone as an internal standard.
For HPLC conditions, a Waters 2690 Controller was used with a Waters 996 Photodiode Array (PDA) Detector operating at 270 nm. The separation was performed on a LiChrospher®100RP-18 reversed phase column (5 µm, spherical, 4 mm × 125 mm) with methanol–water (7:3) as the mobile phase at a flow rate of 1.00 ml/min.
Bleaching and Necrotic Effects of Renieramycin M on Porites
A colony of live massive coral, Porites, was collected from the intertidal zone of Sichon (9°00′16″N, 99°55′20″E). It was then broken into small pieces. There were about 10–15 corallites in each piece (nubbins). A nubbin was attached on top of a plastic rod using a very small amount of ethyl-2-cyanoacrylate glue and then left in the air for several seconds for the hardening of the glue. All coral nubbins were vertically set onto the plastic net (approximate mesh size 5 mm). The plastic net (culture frame) with coral nubbins was hung in the ocean for 2 months. The survival rate was monitored weekly.
Thirty coral nubbins were collected from the culture frame and then were acclimatized in the lab for a week prior to the experiment. There were five treatments of different renieramycin M concentrations: 0.00 ppm (control), 0.02 ppm, 0.20 ppm (natural concentration), 1.00 ppm, and 10.00 ppm. One hundred microliters of dimethyl sulfoxide (DMSO), which was preliminarily tested and showed no effects on Porites, was used as a solvent for each treatment, including the control. The total volume was made to be 50.0 ml by 30 ppt of filtered seawater with natural salinity. For each treatment, 5 coral nubbins were submerged in the solution with air bubbles for 8 h. After that, coral nubbins from each treatment were separately placed into transparent containers, each of them containing 500 ml of seawater. To control the growth of algae during the experiment, every container was left under approximately 40% of natural light. Bleaching and necrosis were observed daily for 2 weeks.
Antifouling Effect of Renieramycin M on Larval Settlement
This method was modified from Maida et al. (2006). Several dead coral rocks of Porites sp. with approximately 20 × 30 × 20 cm3 (W × L × H) were collected from an intertidal zone of Sichon (9°00′16″N, 99°55′20″E). They were then cut into approximately 4 × 4 × 1 cm3 (W × L × H) cubes. Each coral cube was desalinated by submerging it in distilled water several times. After that, the coral cubes were dried in an oven at 50°C for 2 days. In all of the cubes, a hole (6.35 mm in diameter) was made at the center by a drilling machine.
Five experiments were prepared with 0.00 ppm (control), 0.05 ppm (natural concentration), 0.10 ppm, 0.20 ppm, and 2.00 ppm renieramycin M in 500 ml of methanol. Three cubes were randomly assigned for each experimental unit. The cubes were submerged into the methanolic solutions for 24 h. Then, they were hung and dried at room temperature (28°C). The experiment contained four experimental sets. Each set consisted of a control unit and four treatment units with different renieramycin M concentrations as described above. There were three replicates of each unit. All experimental sets were then hung in the sea (approximately 1 m below the low tide level) at the same coral reef habitat where the dead coral rocks were collected (Figure 2). Each replicate was approximately 10 cm deep apart from each other. Thus, the depths did not have any effects. The first experimental set was collected from the sea 5 days after the experiment started and the second, third, and fourth experimental sets on days 10, 15, and 20, respectively. Only the upper and lower surfaces of every cube were investigated for fouling organisms under a binocular stereoscope. The number of sessile organisms was counted and grouped into class taxa level. Species diversity index (H’) was used to determine the fouling effect of renieramycin M as well.
Antimicrobial Effect of Renieramycin M on Aerobic Bacteria
Fresh seawater, collected from a coral reef habitat from Sichon (9°00′16″N, 99°55′20″E), was aerated 24 h prior to the experiment. Then, 1.00 ml of this water was pipetted using a micropipette and was poured onto an aerobic count plate (petrifilm sheet) (3M Petrifilm Aerobic Count Plate, 3M) for each treatment. A petrifilm sheet was unmoved for a couple of minutes to let the gel solidify. Paper disks (5.0-mm diameter) (MF-Millipore Membrane Filter, Merck) have 200, 100, 10, or 1 µg of renieramycin M. As a control, paper disks bearing 0.0 µg of renieramycin M were prepared (Figure 3). The petrifilm sheets were placed and incubated at 30°C for 24 h. In one plate, both a control disk and a treatment disk (a single concentration of renieramycin M in each plate) were placed. After 24 h, the diameter of the zone of inhibition in each paper disk was recorded. The number of bacterial colonies growing on the grid areas containing the paper disks was also counted by using an illuminated magnifier and a hand tally counter.
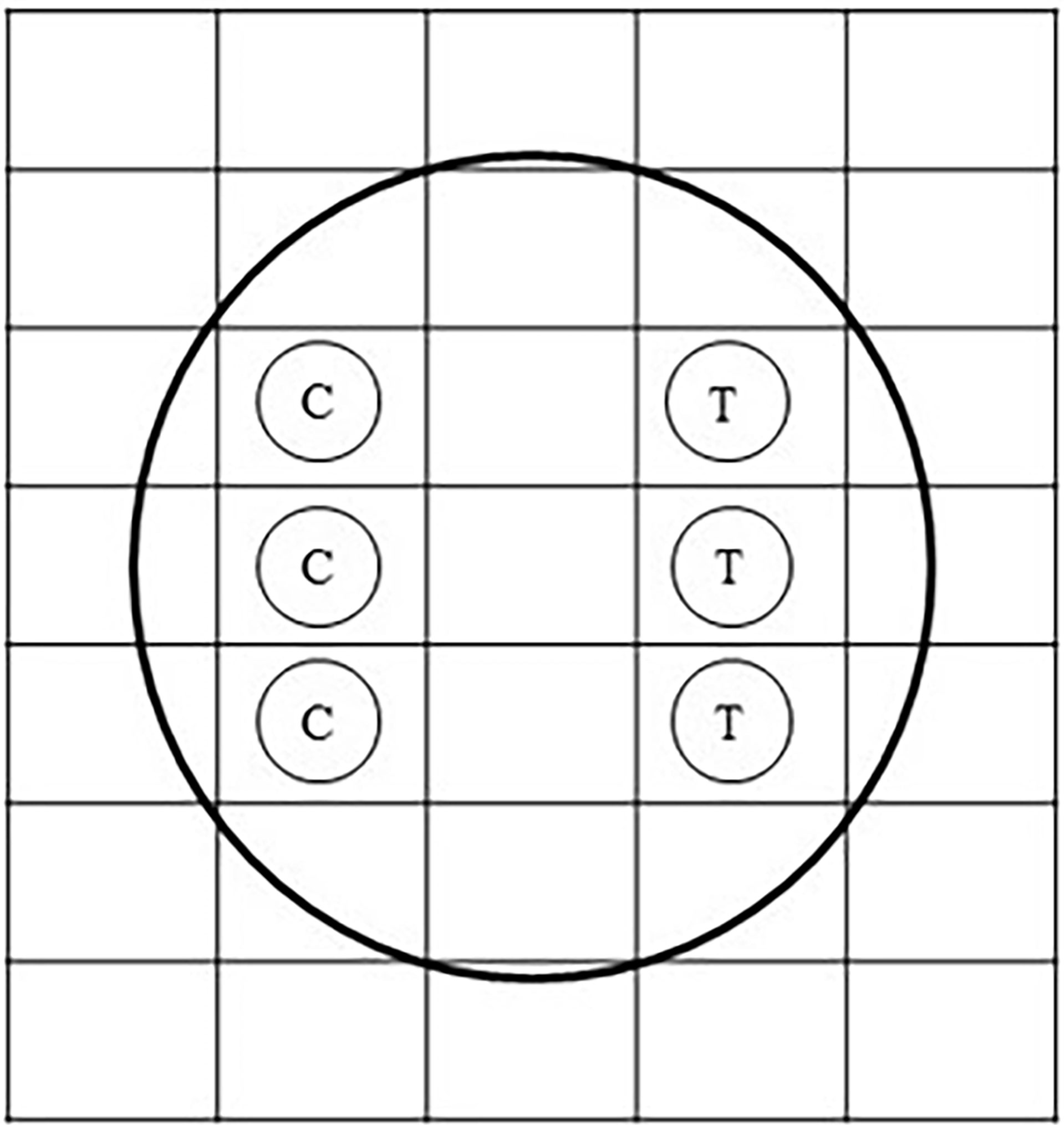
Figure 3 Diagram of the paper disk technique on an aerobic count plate. C stands for the control bearing only 3 µl of dimethyl sulfoxide (DMSO). T stands for the treatment bearing renieramycin M at 200, 100, 10, or 1 µg with 3 µl of DMSO.
Statistical Analyses
The differences on C, H, and N contents and renieramycin M concentrations with Porites or Palythoa were examined using the nested analysis of variance (ANOVA) to examine both differences between coexisting species and among the areas, as the factor of the areas is nested in the factor of the coral species. In addition, the numbers of bacterial colonies on paper disks (3 disks for control and 3 disks for treatment) of each treatment petrifilm sheet were statistically compared using t-test. A significant level applied in this study was 0.05.
Results
Carbon, Hydrogen, and Nitrogen Contents and Allocations of Renieramycin M in Xestospongia
The results showed that C, H, N contents and renieramycin M concentration were not significantly different (P > 0.05) among areas of Xestospongia coexisting with Porites and Palythoa (Table 1). The nutrition values (C/N ratio) at different areas of Xestospongia coexisting with Porites and of those coexisting with Palythoa were also not significant (P > 0.05) (Table 1). However, 8 out of 15 Xestospongia individuals that coexisted with Porites had their highest renieramycin M concentrations in the edge area, whereas 7 out of 15 Xestospongia individuals coexisting with Palythoa had the highest concentrations in the outer area (Table 2).
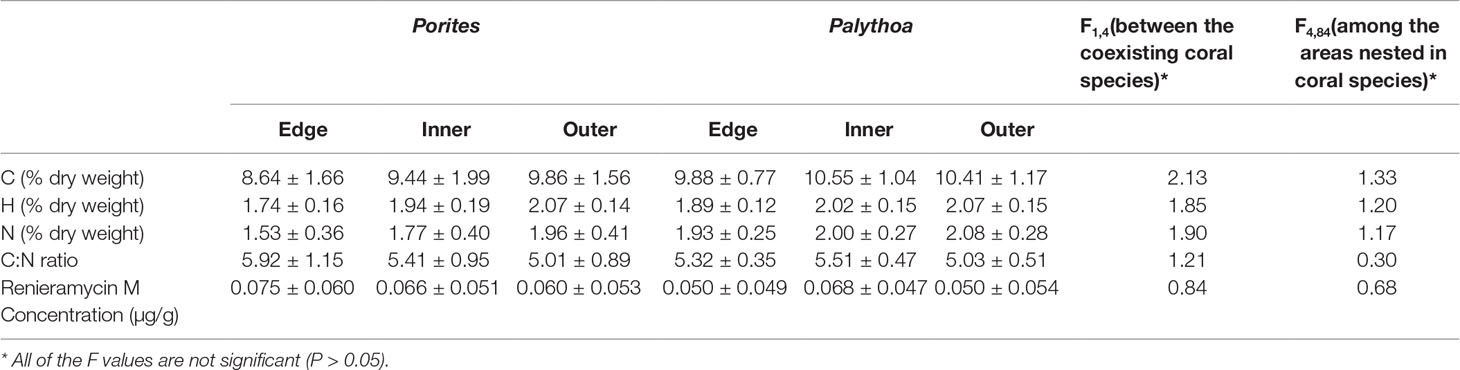
Table 1 The percentage (mean ± SE) of carbon (C), hydrogen (H), and nitrogen (N) contents, C:N ratio, and renieramycin M concentration in different areas of Xestospongia coexisting with different organisms.

Table 2 The proportions of Xestospongia individuals that contain the highest renieramycin M concentrations at different areas when they coexist with Porites and Palythoa.
Bleaching and Necrosis Effects of Renieramycin M on Porites
Bleaching and necrosis of coral nubbins were not observed during 2 weeks in every treatment. Coral nubbins were still brown in color in both control and treatments and sometimes covered by a mucous sheet. During nighttime or low light intensity, corallites of some coral nubbins protruded and waved their trunks and their tentacles into the water column.
Antifouling Effect of Renieramycin M on Larval Settlement
Renieramycin M was found to inhibit the settlement of some sessile organisms. However, the duration of the antifouling effect could be found on the first 5 days (Figure 4). There were three groups of sessile organisms found on the control plates, pelecypoda, polychaeta, and barnacle (Semibalanus balanoides), whereas only pelecypoda was found on the treatment plates during the 5-day period. After 10 days, pelecypods were the major group of sessile organisms on control and treatment plates. Polychaeta was found settled on the treatment plates of 0.05–2.0 ppm concentrations (Figure 4). After a 15-day period, S. balanoides was found again on the settlement plates (control, 0.05 ppm, 0.1 ppm, and 2.0 ppm), whereas at the 5-day period, S. balanoides was found only on the control plates (Figure 4). The composition of sessile organisms on every treatment was the same as the composition of sessile organisms on the control after 20 days (Figure 4). The diversity index also supported the effect on antifouling of renieramycin M (Figure 5). After 5 days, the highest diversity index was for the control plates, whereas other treatment plates had a zero diversity index. After longer periods (days 15 and 20), the diversity index values were high in all treatments.
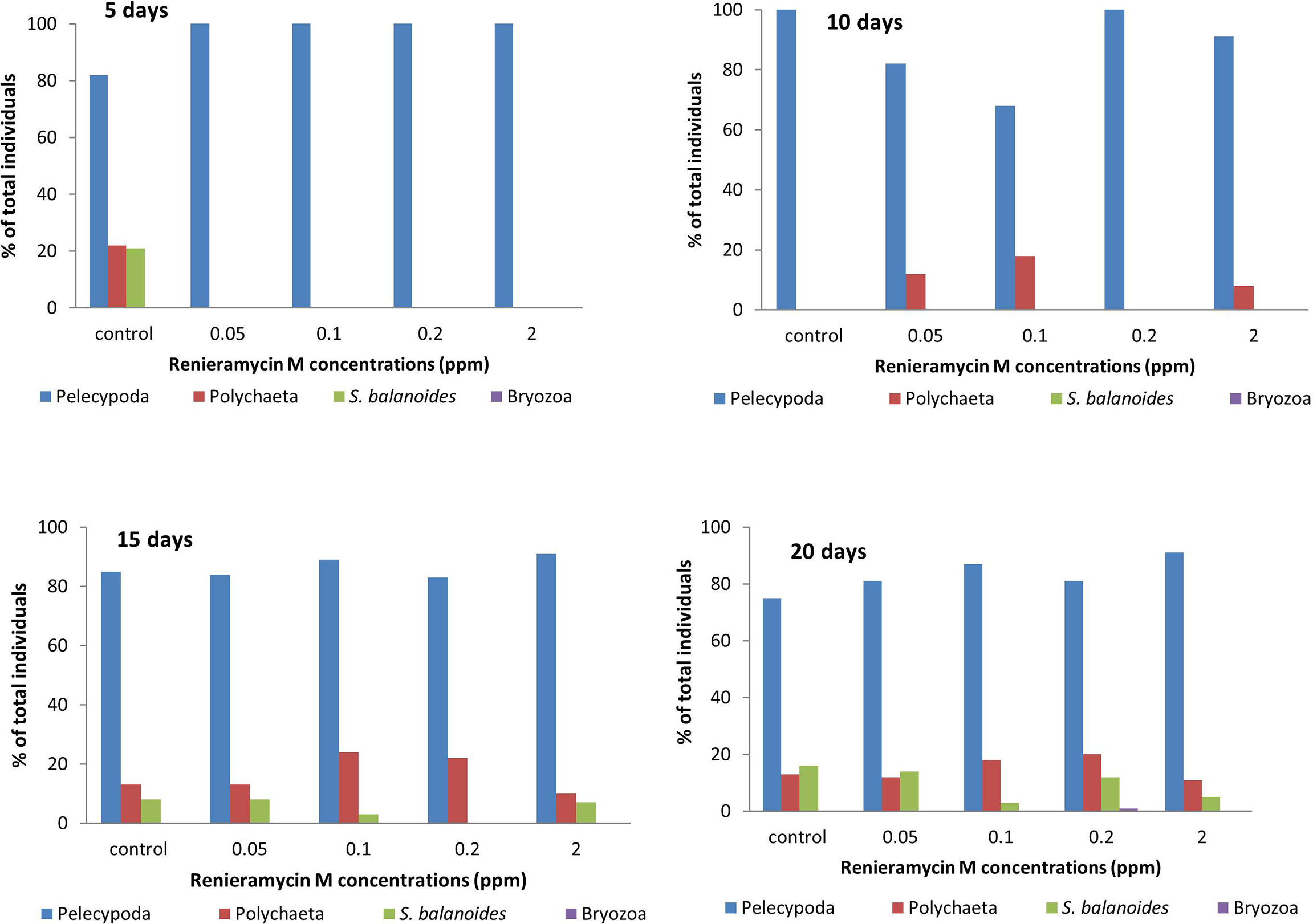
Figure 4 The percentage of total individuals of sessile organisms on different experimental plates after 5, 10, 15, and 20 days.
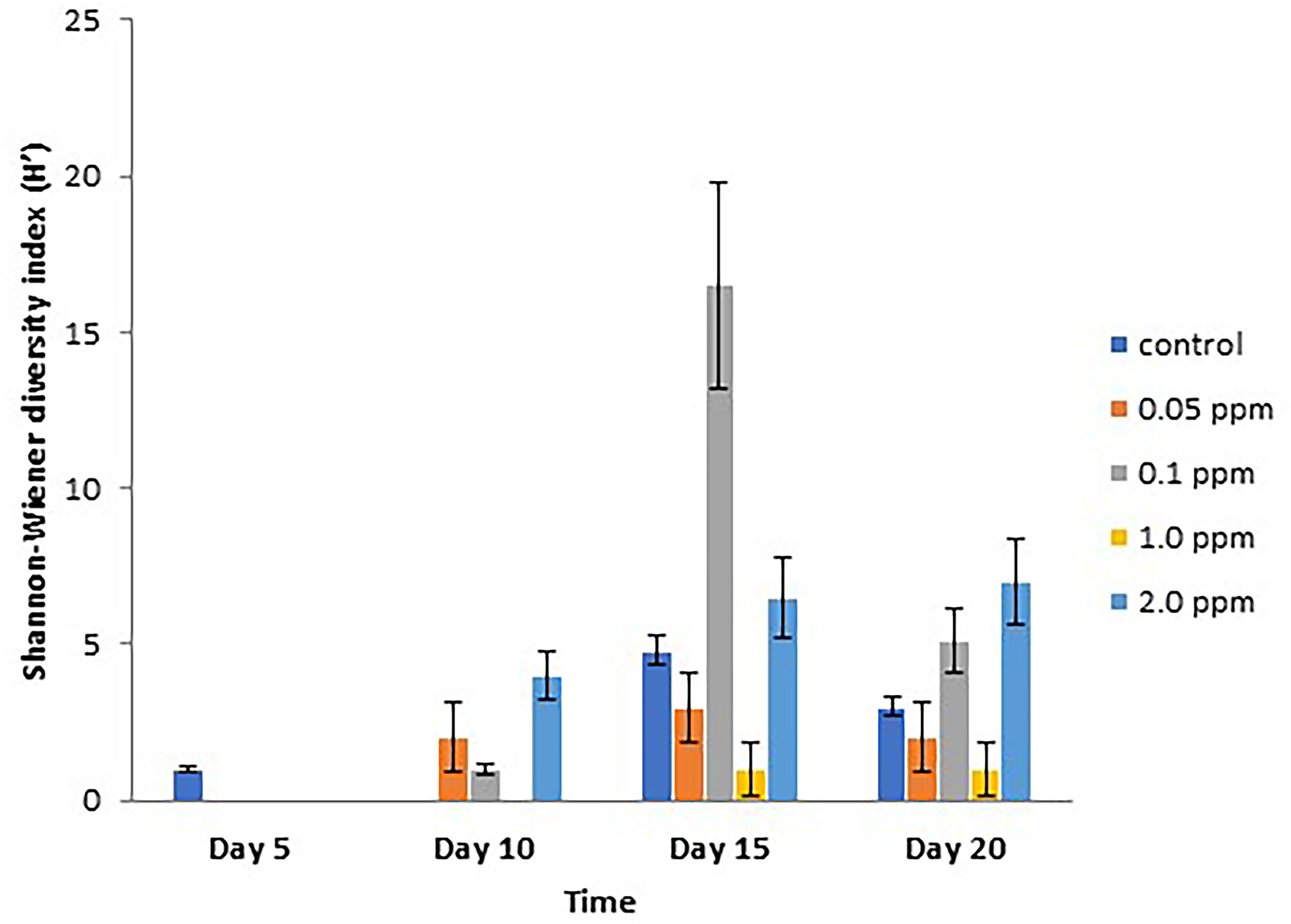
Figure 5 Shannon–Wiener diversity index (H’) (mean ± SE) on different experimental plates at different concentrations of renieramycin M and different periods.
Antimicrobial Effect of Renieramycin M on Aerobic Bacteria
There was no significant difference (P > 0.05) in the number of aerobic bacterial colonies in all contents of renieramycin M (Figure 6). The average number of bacterial colonies on the control paper disks ranged from 30 to 62 colonies per 50 µl, whereas the average number of colonies on treatment paper disks ranged from 45 to 61 colonies per 50 µl (Figure 6). The zone of colonial growth inhibition was not observed on any control or any treatment paper disks. The statistical analysis of antimicrobial effects between different concentrations of renieramycin M compared with control was shown in Table S1.
Discussion
Allocations of Carbon and Nitrogen Contents and Renieramycin M in Xestospongia
In this study, the C, H, and N contents were uniformly allocated in the body of Xestospongia coexisting with Porites, even though there were no significant differences on C, H, or N contents in same areas of Xestospongia when it coexisted with different competitors (Porites or Palythoa) (Table 1). However, the varieties of nutrition, chemical contents, and morphological and physiological changes were commonly found in plants, sponges, and other sessile organisms (Haukioja et al., 1998; Swearingen and Pawlik, 1998; Puglisi et al., 2000; Furrow et al., 2003; Meroz-Fine et al., 2005). For example, for plant species in boreal forests, plants balance their C/N utilizations to survive in the intensely competitive and herbivory pressured habitats (Bryant et al., 1983). In addition, some N-based chemicals, produced by organisms, were varied by N-limited habitats (Bryant et al., 1983).
There were no significant distributions of renieramycin M concentrations along the body parts of Xestospongia (Table 1). On the other hand, other sponge species, Oceanapia spp. and Crambe crambe, have been proven to significantly distribute their toxicity and secondary metabolites within specimens such as at fistule, capitum, basal, or even different cell types (Uriz et al., 1996; Schupp et al., 1999; Salomon et al., 2001). By comparison, renieramycin M concentrations at the edges were higher in Porites-coexisted Xestospongia than Palythoa-coexisted ones. These results might be due to the allocation of chemicals on different spatial competitors of Xestospongia. Although there were a number of studies on the effects of allelochemicals and secondary metabolites of marine sponges on potential spatial competitors, particularly corals, the comparison of chemicals produced by sponges when they coexist with different potential spatial competitors was less available (see examples in Porter and Targett, 1988; Aerts and Van Soest, 1997; Aerts, 1998; Nishiyama and Bakus, 1999; Engel and Pawlik, 2000; Pawlik et al., 2007).
Allelopathic Effects of Renieramycin M
The effects of allelochemicals of sponges on corals and on other sponge species were necrotic effects, bleaching, and the effect on the photosynthetic ability of coral’s symbionts (Porter and Targett, 1988; Engel and Pawlik, 2000; Pawlik et al., 2007). However, renieramycin M exhibited neither bleaching nor necrosis effects on Porites.
During the surveys, the necrotic scars or the dead zones of Porites were observed only on the tissue that contacted Xestospongia (in this study; Darumas et al., 2007). Therefore, the dead zones or necrotic areas might be caused by the synergy of physical damage (by spicules) and other allelochemicals. In addition, sessile organisms were known to compete for space. The strategies to compete with their competitors include overgrowth, physical damage, allelochemicals, and waterborne substances (Porter and Targett, 1988; Paul, 1992; Aerts, 1998; Nishiyama and Bakus, 1999). According to the field surveys of this study, the interactions between Xestospongia and the massive coral, Porites, were of the single category, sponge overgrowing coral. The sponge/coral interactions were classified into four categories: overgrowth, peripheral contact, tissue contact, and non-contact (Aerts and Van Soest, 1997). Their study showed that 46.6% of Xestospongia spp. (X. caminata, X. muta, X. proxima) had tissue contact with corals, and 32.6% had non-contact with corals, while 2.5% of Xestospongia overgrew corals. On the other hand, just 2.2%, 14.3%, and 33.8% of massive coral, Porites astreoides, had overgrown, peripheral contact, and tissue contact by sponges, respectively. In addition, the major proportion of the interaction (49.7%) of this coral was non-contact to sponges (Aerts and Van Soest, 1997). Although, the effect of overgrowth by sponges on corals was not directly documented, one study showed that the overgrowth of sponge on introduced mussels in the Great Lakes (USA) caused mortality or at least loss in the mussels (Ricciardi et al., 1995).Renieramycin M had no antifouling effect on pelecypods but on polychaetes and barnacles (S. balanoides) during the first 5 days of the experiments. The antifouling chemicals produced by pre-settlement organisms were not universally against newcomers (Porter and Targett, 1988; Paul, 1992; Nishiyama and Bakus, 1999). Antifouling was the active competitive defense of sessile organisms. This strategy prevented interspecific larva from settling on surfaces of the previous one (Jackson and Buss, 1975; Nishiyama and Bakus, 1999; Kubanek et al., 2002). The antifouling process operates through mechanical and chemical mechanisms. Some soft corals were swept out and were damaged by sweeper tentacles and nematocysts of hard corals (Paul, 1992). Some sessile organisms used at least two mechanisms to inhibit interspecific larval settlement (Bak and Borsboom, 1984; Coll et al., 1987; Sears et al., 1990; Henrikson and Pawlik, 1995; Hirota et al., 1998; Nishiyama and Bakus, 1999). First was a waterborne substance production, which was released into the water column to affect the competitor’s fitness. Second was the allelochemical that affected the competitors when the tissues were in contact.
Renieramycins were tested to inhibit Staphylococcus aureus, Bacillus subtilis, and Vibrio angularium but not Escherichia coli, Candida albicans, Pseudomonas aeruginosa, and Enterobacter aerogenes (Frincke and Faulkner, 1982; Kelman et al., 2001). In this study, renieramycin M did not inhibit the growth of aerobic bacteria in any treatment. However, antibiotic chemicals produced by sessile organisms or the symbionts were effective against specific bacteria rather than a broad spectrum (Frincke and Faulkner, 1982; Kelman et al., 2001). A number of studies elaborated more about the interaction between bacteria vs. sponge and bacteria vs. macroalgae such as anti-non-symbiotic species and antifouling (Imhoff and Trüper, 1976; Wilkinson, 1978; Lemos et al., 1985; Althoff et al., 1998; Webster and Hill, 2001; Thacker and Starnes, 2003; Armstrong et al., 2006). Sponges of the genus Dysidea were a specific host of cyanobacterium (Thacker and Starnes, 2003). Sometimes, the chemicals produced by microsymbionts were beneficial to the host (Lemos et al., 1985; Webster and Hill, 2001). Epiphytic bacteria attached to seaweed produced antimicrobial chemicals for the host (Lemos et al., 1985). α-Proteobacteria, surrounding the choanocyte chamber of Rhopaloeides sponge, was expected to play the role of nutrient uptake in the host sponge (Webster and Hill, 2001). In some cases, microorganisms may be harmful to sessile organisms. This can be inferred by the varieties of antibiotic substances produced by sessile organisms or their symbionts (Frincke and Faulkner, 1982; Lemos et al., 1985; Kelman et al., 2001; Kubanek et al., 2002; Müller et al., 2004).
Conclusion
This study provides evidence that renieramycin M found in Xestospongia was uniform; however, it can possess strong inhibitory activities against some marine organisms. From our study, there were variations of nutritional values of Xestospongia. Xestospongia did not unequally distribute its nutritional values for predatory defense. The overall contents of C, H, and N in every area of Xestospongia coexisting with Porites were not different from those of Xestospongia coexisting with Palythoa. In the laboratory, renieramycin M did not show any allelopathic effect on its coexisting organisms (Porites). Furthermore, it did not inhibit the growth of aerobic bacteria. However, renieramycin M inhibited the settlement of acorn barnacle. However, it did not inhibit the settlement of pelecypods.
Data Availability Statement
The original contributions presented in the study are included in the article/Supplementary Material. Further inquiries can be directed to the corresponding author.
Author Contributions
UD, KS, and SC conceived the ideas and designed the study. UD and SC carried out the field work and sampling quantification. US, KS, VV, and SC contributed to the data analysis and interpretation. UD, GS, VV, and SC drafted the manuscript. All authors contributed to the article and approved the submitted version.
Funding
This work was supported by Second Century Fund (C2F) Postdoctoral Scholarship of Chulalongkorn University, NRCT-JSPS Core to Core Program, CREPSUM JPJSCCB20200009, Thailand Science research and Innovation Fund Chulalongkorn University (CU_FRB65_dis (3)_091_23_21)), National Research Council of Thailand and Chulalongkorn University (N42A650257), and Mubadala Petroleum (Thailand) Limited. Mubadal Petrolem (Thailand) was not involved in the study design, collection analysis, interpretation of data, the writing of this article or the decision to submit it for publication.
Conflict of Interest
The authors declare that the research was conducted in the absence of any commercial or financial relationships that could be construed as a potential conflict of interest.
Publisher’s Note
All claims expressed in this article are solely those of the authors and do not necessarily represent those of their affiliated organizations, or those of the publisher, the editors and the reviewers. Any product that may be evaluated in this article, or claim that may be made by its manufacturer, is not guaranteed or endorsed by the publisher.
Acknowledgments
We acknowledged Dr. John Hooper, Dr. Larry Harris, Dr. Pitiwong Tantichodok, editor and reviewers for their valuable comments and suggestions. We also thank UNESCO/IOC-WESTPAC for the support.
Supplementary Material
The Supplementary Material for this article can be found online at: https://www.frontiersin.org/articles/10.3389/fmars.2022.735702/full#supplementary-material
References
Aerts L. A. M. (1998). Sponge/coral Interactions in Caribbean Reefs: Analysis of Overgrowth Patterns in Relation to Species Identity and Cover. Mar. Ecol. Prog. Ser. 175, 241–249. doi: 10.3354/meps175241
Aerts L. A. M., Van Soest R. W. M. (1997). Quantification of Sponge/Coral Interactions in a Physically Stressed Reef Community, NE Colombia. Mar. Ecol. Prog. Ser. 148, 125–134. doi: 10.3354/meps148125
Althoff K., Schütt C., Steffen R., Batel R., Müller W. E. G. (1998). Evidence for a Symbiosis Between Bacteria of the Genus Rhodobacter and the Marine Sponge Halichondria Panacea: Harbor Also for Putatively Toxic Bacteria? Mar. Biol. 130, 529–536. doi: 10.1007/s002270050273
Amnuoypol S., Suwanborirux K., Pummangura S., Kubo A., Tanaka C., Saito N. (2004). Chemistry of Renieramycins. Part 5. Structure Elucidation of Renieramycin-Type Derivatives O, Q, R, and S From Thai Marine Sponge Xestospongia Species Pretreated With Potassium Cyanide. J. Nat. Prod. 67, 1023–1028. doi: 10.1021/np030534o
Armstrong R. A., Singh H., Torres J., Nemeth R. S., Can A., Roman C., et al. (2006). Characterizing the Deep Insular Shelf Coral Reef Habitat of the Hind Bank Marine Conservation District (US Virgin Islands) Using the Seabed Autonomous Underwater Vehicle. Continent. Shelf. Res. 26, 194–205. doi: 10.1016/j.csr.2005.10.004
Bak R. P. M., Borsboom J. L. A. (1984). Allelopathic Interaction Between a Reef Coelenterate and Benthic Algae. Oecologia. 63, 194–198. doi: 10.1007/BF00379877
Bryant J. P., Chapin F.S.III., Klein D. R. (1983). Carbon/nutrient Balance of Boreal Plants in Relation to Vertebrate Herbivory. Oikos. 40, 357–368. doi: 10.2307/3544308
Coll J. C., Price I. R., König G. M., Bowden B. F. (1987). Algal Overgrowth Alcyonacean Soft Corals. Mar. Biol. 96, 129–135. doi: 10.1007/BF00394846
Darumas U., Chavanich S., Suwanborirux K. (2007). Distribution Patterns of the Renieramycin-Producing Sponge, Xestospongia Sp., and its Association With Other Reef Organisms in the Gulf of Thailand. Zoological Stud. 46 (6), 695–704.
Engel S., Pawlik J. R. (2000). Alleopathic Activities of Sponge Extracts. Mar. Ecol. Prog. Ser. 207, 273–281. doi: 10.3354/meps207273
Faulkner D. J. (1984). Marine Natural Products: Metabolites of Marine Invertebrates. Nat.Prod. Rep. 1, 551–598. doi: 10.1039/np9840100551
Folt C., Goldman C. (1981). Allelopathy Between Zooplankton: A Mechanism for Interference Competition. Science 213, 1133–1135. doi: 10.1126/science.213.4512.1133
Frincke J. M., Faulkner D. J. (1982). Antimicrobial Metabolites of the Sponge Reniera Sp. J. Am. Chem. Soc 104, 265–269. doi: 10.1021/ja00365a048
Furrow F. B., Amsler C. D., McClintock J. B., Baker B. J. (2003). Surface Sequestration of Chemical Feeding Deterrents in the Antarctic Sponge Latrunculia Apicalis as an Optimal Defense Against Sea Star Spongivory. Mar. Biol. 143, 443–449. doi: 10.1007/s00227-003-1109-5
Haukioja E., Ossipov V., Koricheva J., Honkanen T., Larsson S., Lempa K. (1998). Biosynthetic Origin of Carbon-Based Secondary Compounds: Cause of Variable Responses of Woody Plants to Fertilization? Chemoecol. 8, 133–139. doi: 10.1007/s000490050018
Henrikson A. A., Pawlik J. R. (1995). A New Antifouling Assay Method: Results From Field Experiments Using Extracts of Four Marine Organisms. J. Exp. Mar. Biol. Ecol. 194, 157–165. doi: 10.1016/0022-0981(95)00088-7
Hirota H., Okino T., Yoshimora E., Fusetani N. (1998). Five New Antifouling Sesquiterpenes From Two Marine Sponges of the Genus Axinyssa and the Nudibranch Phyllidia Pustulosa. Tetrahedron. 54, 13971–13980. doi: 10.1016/S0040-4020(98)00867-9
Imhoff J. F., Trüper H. G. (1976). Marine Sponges as Habitats of Anarobic Phototrophic Bacteria. Microb. Ecol. 3, 1–9. doi: 10.1007/BF02011449
Jackson J. B. C., Buss L. (1975). Allelopathy and Spatial Competition Among Coral Reef Invertebrates. Proc. Nat. Acad. Sci. 72 (12), 5160–5163. doi: 10.1073/pnas.72.12.5160
Kelman D., Kashman Y., Rosenberg E., Ilan M., Ifrach I., Loya Y. (2001). Antimicrobial Activity of the Reef Sponge Amphimedon Viridis From the Red Sea: Evidence for Selective Toxicity. Aquat. Microb. 24, 9–16. doi: 10.3354/ame024009
Kritsanapuntu S., Chaitanawisuti N., Yeemin T., Putchakan S. (2001). First Investigation on Biodiversity of Marine Sponges Associated With Reef Coral Habitats in the Eastern Gulf of Thailand. Asian Mar. Biol. 18, 105–115.
Kubanek J., Whalen K. E., Engle S., Kelly S. R., Henkel T. P., Fenical W., et al. (2002). Multiple Defensive Roles for Triterpene Glycosides From Two Caribbean Sponges. Oecologia. 131, 125–136. doi: 10.1007/s00442-001-0853-9
Lages B. G., Fleury B. G., Ferreira C. E. L., Pereira R. C. (2006). Chemical Defense of an Exotic Coral as Invasion Strategy. J. Exp. Mar. Biol. Ecol. 328, 127–135. doi: 10.1016/j.jembe.2005.07.011
Lang J. C., Chornesky E. A. (1990). “Competition Between Scleractinian Reef Corals: A Review of Mechanisms and Effects,” in Ecosystems of the World: Coral Reefs. Ed. Dubinsky Z. (Amsterdam: Elsevier Press).
Lemos M. L., Toranzo A. E., Barja J. L. (1985). Antibiotic Activity of Epiphytic Isolated From Intertidal Seaweeds. Microb. Ecol. 11, 149–163. doi: 10.1007/BF02010487
Maida M., Sammarco P. W., Coll J. C. (2006). A Diffusion Chamber for Assessing Efficacy of Natural Anti-Fouling Defenses in Marine Organisms. J. Exp. Mar. Biol. Ecol. 337, 59–64. doi: 10.1016/j.jembe.2006.06.008
Meroz-Fine E., Shefer S., Ilan M. (2005). Changes in Morphology and Physiology of an East Mediterranean Sponge in Different Habitats. Mar. Biol. 147, 243–250. doi: 10.1007/s00227-004-1532-2
Müller W. E. G., Klemt M., Thakur N. L., Schröder H. C., Aiello A., D’Esposito M., et al. (2004). Molecular/chemical Ecology in Sponges: Evidence for an Adaptive Antibacterial Response in Suberites Domancula. Mar. Biol. 144 (1), 19–29. doi: 10.1007/s00227-003-1184-7
Nakao Y., Shiroiwa T., Murayama S., Matsunaga S., Goto Y., Masumoto Y., et al. (2004). Identification of Renieramycin A as an Antileishmanial Substance in Marine Sponge Neopetrosia Sp. Mar. Drugs 2, 55–62. doi: 10.3390/md202055
Nishiyama G. K., Bakus G. J. (1999). Release of Allelochemicals by Three Tropical Sponges (Demospongiae) and Their Toxic Effects on Coral Substrate Competitors. Memoirs Queensland Museum. 44, 411–417.
Oku N., Matsunaga S., van Soest R. W. M., Fusetani N. (2003). Renieramycin J, a Highly Cytotoxic Tetrahydroisoquinoline Alkaloid, From a Marine Sponge Neopetrosia Sp. J. Nat. Prod. 66, 1136–1139. doi: 10.1021/np030092g.
Parameswaran P. S., Naik C. G., Kamat S. Y. (1998). Renieramycins H and I, Two Novel Alkaloids From the Sponge Haliclona Cribricutis Dany. Indian J. Chem., 37B. 1258–1263.
Paul V. J. (1992). “Chemical Defenses of Benthic Marine Invertebrates,” in Ecological Roles of Marine Natural Products. Ed. Paul V. J. (London: Comstock Publishing Associates), 164–188. doi: 10.7591/9781501737435-009
Pawlik J. R., Steindler L., Henkel T. P., Beer S., Ilan M. (2007). Chemical Warfare on Coral Reefs: Sponge Metabolites Differently Affect Coral Symbiosis in Situ. Limnol. Oceanogr. 52 (2), 907–911. doi: 10.4319/lo.2007.52.2.0907
Pettit G. R., Knight J. C., Collin J. C., Herald D. L., Pettit R. K., Boyd M. R., et al. (2000). Antineoplastic Agent 430. Isolation and Structure of Cribrostatins 3, 4 and 5 From the Republic of Maldives Cribrochalina Species. J. Nat. Prod. 63, 793–798. doi: 10.1021/np990618q
Porter J. W., Targett N. M. (1988). Allelochemical Interactions Between Sponges and Corals. Biol. Bull. 175, 230–239. doi: 10.2307/1541563
Puglisi M. P., Paul V. J., Slattery M. (2000). Biogeographic Compositions of Chemical and Structural Defenses of the Pacific Gorgonians Annella Mollis and A. Reticulate. Mar. Ecol. Prog. Ser. 207, 263–272. doi: 10.3354/meps207263
Pulitzer—Finali G. (1996) Sponges from the Bismarck Sea.Bollettino dei Musei e degli Istituti Biologici della (R.) Università di Genova, 60–61, 101–138.
Ricciardi A., Snyder F. L., Kelch D. O., Reiswig H. M. (1995). Lethal and Sublethal Effects of Sponge Overgrowth on Introduced Dreissenid Mussels in the Great Lakes-St. Lawrence River System. Can. J. Fish. Aqua. Sci. 52, 2695–2703. doi: 10.1139/f95-858
Salomon C. E., Deerinck T., Ellisman M. H., Faulkner D. J. (2001). The Cellular Location of Dercitimide in the Palauan Sponge Oceanapia Sagittaria. Mar. Biol. 139, 313–319. doi: 10.1007/s002270100493
Schupp P., Eder C., Paul V., Proksch P. (1999). Distribution of Secondary Metabolites in Sponge Oceanapia Sp. And its Ecological Implications. Mar. Biol. 135, 573–580. doi: 10.1007/s002270050658
Sears M. A., Gerhart D. J., Rittschof D. (1990). Antifouling Agents From Marine Sponge Lissodendroxyx Isodictylis Carter. J. Chem. Ecol. 16 (3), 791–799. doi: 10.1007/BF01016489
Suikkanen S., Fistarol G. O., Granéli E. (2004). Allelopathic Effects of the Baltic Cyanobacteria Nodularia Spumigena, Aphanizomanon Flos-Aquae and Anabaena Lemmermannii on Algal Monocultures. J. Exp. Mar. Biol. Ecol. 308, 85–101. doi: 10.1016/j.jembe.2004.02.012
Suwanborirux K., Amnuoypol S., Plubrukarn A., Pummangura S., Kubo A., Tanaka C., et al. (2003). Chemistry of Renieramycins. Part 3. Isolation and Structure of Stabilized Renieramycin Type Derivatives Possessing Antitumor Activity From Thai Sponge Xestospongia Species, Pretreated With Potassium Cyanide. J. Nat. Prod. 66, 1441–1446. doi: 10.1021/np030262p
Swearingen D. C., Pawlik J. R. (1998). Variability in the Chemical Defense of the Sponge Chondrilla Nucula Against Predatory Reef Fishes. Mar. Biol. 131, 619–627. doi: 10.1007/s002270050354
Thacker R. W., Starnes S. (2003). Host Specificity of the Symbiotic Cyanobacterium Oscillatoria Spongiliae in Marine Sponges Dysidea Spp. Mar. Biol. 142, 643–648. doi: 10.1007/s00227-002-0971-x
Uriz M. J., Becerro M. A., Tur J. M., Turon X. (1996). Location of Toxicity Within the Mediterranean Sponge Crambe Crambe (Demospongiae: Poecilosclerida). Mar. Biol. 124, 583–590. doi: 10.1007/BF00351039
Van Soest R. W. M., Boury-Esnault N., Janussen D., Hooper J. (2005) World Porifera Database. Available at: http://www.vliz.be/vmdcdata/porifera.
Vicente V. P. (1990). Response of Sponges With Autotrophic Endosymbionts During the Coral-Bleaching Episode in Puerto Rico. Coral Reefs 8, 199–202. doi: 10.1007/BF00265011
Wang C. Y., Liu H. Y., Shao C. L., Wang Y., Li L., Guan H. S. (2008). Chemical Defensive Substances of Soft Corals and Gorgonians. Acta Ecologica Sin. 28, 2320–2328. doi: 10.1016/S1872-2032(08)60048-7
Webster N. S., Hill R. T. (2001). The Culturable Microbial Community of the Great Barrier Reef Sponge Rhopaloeides Odorabile is Dominated by an α-Proteobacterium. Mar. Biol. 138, 843–851. doi: 10.1007/s002270000503
Wilkinson C. R. (1978). Microbial Associations in Sponges. I. Ecology, Physiology and Microbial Populations of Coral Reef Sponges. Mar. Biol. 49, 161–167. doi: 10.1007/BF00387115
Keywords: Xestospongia sp., renieramycin M, sponge, allelopathy, Gulf of Thailand, bioactive compounds, coral
Citation: Darumas U, Salem GEM, Suwanborirux K, Viyakarn V and Chavanich S (2022) Variation of Carbon–Nitrogen Contents and Allelopathic Disruption of Renieramycin M–Producing Sponge Xestospongia sp. in the Gulf of Thailand. Front. Mar. Sci. 9:735702. doi: 10.3389/fmars.2022.735702
Received: 03 July 2021; Accepted: 21 June 2022;
Published: 22 July 2022.
Edited by:
Keryea Soong, National Sun Yat-sen University, TaiwanCopyright © 2022 Darumas, Salem, Suwanborirux, Viyakarn and Chavanich. This is an open-access article distributed under the terms of the Creative Commons Attribution License (CC BY). The use, distribution or reproduction in other forums is permitted, provided the original author(s) and the copyright owner(s) are credited and that the original publication in this journal is cited, in accordance with accepted academic practice. No use, distribution or reproduction is permitted which does not comply with these terms.
*Correspondence: Suchana Chavanich, suchana.c@chula.ac.th
†These authors have contributed equally to this work and share first authorship