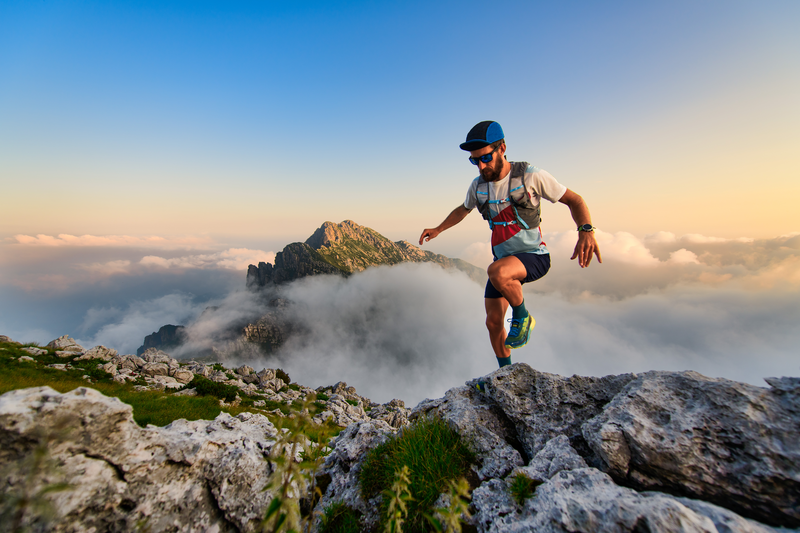
94% of researchers rate our articles as excellent or good
Learn more about the work of our research integrity team to safeguard the quality of each article we publish.
Find out more
ORIGINAL RESEARCH article
Front. Mar. Sci. , 20 May 2022
Sec. Coral Reef Research
Volume 9 - 2022 | https://doi.org/10.3389/fmars.2022.725778
Thermal stress is now considered the major recent cause of coral reef degradation; yet few studies have been conducted describing those effects on Southwestern Atlantic (SWA) reefs. The SWA represents a coral endemism hotspot with low-functional redundancy and therefore high extinction risk. Recent research has suggested a “thermal refuge” potential for SWA; however, evidence could suggest a different trend. We report herein an unprecedented coral mortality on the largest coastal Brazilian Marine Protected Area (MPA) following the worst thermal stress event since 1985. Degree Heating Week (DHW) values over 4.0 were observed for 107 days, averaging 8.70 for the period, with a maximum of 12.1. Average live coral cover was reduced by 18.1% while average turf algae cover increase by 19.3%. Mortality was highest for three coral species, with a mean mortality of 50.8% per transect for Millepora braziliensis, 32.6% for Mussismilia harttii and 16.6% for Millepora alcicornis. Our unique data for SWA indicates that the populations of two Brazilian endemic species (Millepora braziliensis and Mussismilia harttii) are under severe threat from global warming and that overall coral cover has been dramatically reduced. Hence, the idea of a possible “thermal” refugia within the SWA must be taken with caution for this coral endemism hotspot.
Global warming is now the main cause of coral reef degradation and has resulted in increased frequency and magnitude of mass coral bleaching and mortality events (Hughes et al., 2017; Sully et al., 2019). Increased temperatures lead corals to lose or expel symbiont zooxanthellate and associated microbiota, increasing their vulnerability to mortality (Baker et al., 2008; Spalding and Brown, 2015). These physiological alterations can lead to dramatic coral mortality, losses of coral cover, changes in structural complexity and reef functioning (Donner and Carilli, 2019; Magel et al., 2019). Conversely, corals can be resilient, resisting or fully recovering their pigmented symbionts and health after milder bleaching episodes (Loya et al., 2001; Graham et al., 2015). Results depend on the intensity and duration of thermal stress and local environmental conditions, which determine bleaching and mortality thresholds (Glynn, 1996; Baker et al., 2008; Hughes et al., 2018a; Soares et al., 2021). The threshold for bleaching can be higher when coral species have associations to tolerant clades of symbionts, such as Symbiodiniaceae (Marshall and Baird, 2000; Loya et al., 2001; Jiang et al., 2021) and predominant heterotrophic feeding which may increase resistance to mortality (Anthony, 2006).
Reefs dominated by branching coral species (e.g., Acropora spp.), such as Indo-Pacific and Caribbean reefs, have demonstrated low resistance, suffering high mortality rates after mass coral bleaching episodes (Hughes et al., 2018a; Eakin et al., 2019). However, fast-growing branching/tabular corals can also contribute to reef recovery in some cases, yet this is a long-term process that can require up to a decade (Pisapia et al., 2016). In contrast with Indo-Pacific and Caribbean reefs, Southwestern Atlantic (SWA) reefs have previously shown only infrequent bleaching, usually followed by recovery with low coral mortality (no coral mortality was observed in 80% of bleaching episodes) (Mies et al., 2020) after bleaching events such as those reported between 1993 and 2018 (Migotto, 1995; Castro and Pires, 1999; Kikuchi et al., 2003; Leão et al., 2003; Krug et al., 2012, 2013; Ferreira et al., 2013; Miranda et al., 2013; Soares and Rabelo, 2014; Banha et al., 2019; Teixeira et al., 2019; see Soares et al., 2021 for a review). Hence, recent studies have indicated peculiar characteristics of the SWA coral species, such as deeper bathymetric distribution, higher tolerance to turbidity, higher morphological resistance and more flexible symbiotic associations, that would supposedly make them less susceptible to coral bleaching and subsequent mortality, so that SWA reefs could act as “thermal refugia” (Mies et al., 2020). However, this has not been empirically demonstrated during the latest SWA thermal stress events, and current evidence from Brazilian reefs elsewhere suggest a different trend over the last few years (Teixeira et al., 2019; Duarte et al., 2020; Soares et al., 2021). The key point is that we need to distinguish between the corals being more resistant and the conditions less challenging, as recently suggested by Dixon et al. (2022).
Coral reefs in the SWA occur in marginal conditions in turbid waters, where stress-tolerant species may exhibit high bleaching tolerance due to local adaptations and conditions (e.g., shading by turbidity and nutrient enrichment) (Teixeira et al., 2019; Soares et al., 2021). Furthermore, flexible association with symbiont generalists, massive-form coral dominance and deeper bathymetric distribution could facilitate coral resilience post-thermal stress as suggested by Mies et al. (2020). However, recent empirical studies assessing SWA reefs post-bleaching events are scarce. Additionally, coral bleaching and mortality thresholds are sensitive to thermal stress intensity and frequency, which are increasing in last decade (Sully et al., 2019). This has caused recent mass mortality in SWA reefs, especially for the most common branching species Millepora alcicornis (Duarte et al., 2020).
Here, we document an unprecedented coral mortality episode that occurred during the 2020 COVID-19 pandemic, which coincided with the worst thermal stress event reported in SWA coral reefs since 1985, that resulted in a reduction in cover of live coral. We monitored endemic/threatened branching and massive corals for several years on coral reefs in the largest coastal Brazilian Marine Protected Area (MPA) and related this data with 30-year sea temperature modeling series. Additionally, remote sensing imagery of the study area during pre- and post-bleaching period was accessed to infer on detection of coral bleaching in the SWA.
The present study was conducted in the municipality of Maragogi, in the state of Alagoas, Brazil (Figure 1), whitin the largest multiple-use Brazilian coastal (MPA Costa dos Corais, APACC in the Portuguese acronym), created in 1997 to protect coral reef systems on Brazilian waters. This MPA stretches for 120 km along northeastern Brazil, including two states (Pernambuco and Alagoas) and 12 municipalities. MPA Costa dos Corais covers a large range of different ecosystems, including shallow reefs, mangroves, seagrass beds, rhodolith and sponge beds and mesophotic reefs, extending from the coast to the break of the continental shelf (Maida and Ferreira, 1997; Pereira et al., 2018, 2021a,b). Live coral cover and coral mortality at Maragogi were recorded at three different sites: Pedra do Meio, Aquário and Mãe da Galé, located around 5 km from the coast and with a depth ranging from 3 to 8 m (Figure 1 and Supplementary Material 1). These sites were chosen since they are among the long-term monitoring sites selected by the MPA management team, from among high coral cover areas within the MPA. This study was conducted with the full approval of the Sistema Nacional de Informação sobre Biodiversidade (SISBIO) by Brazilian Government, permit #67684-1.
Figure 1. Study area for monitoring bleaching, highlighting sampling sites (red stars in the right hand map) at marine protected area (MPA) Costa dos Corais (APACC, black line in lower left map) and NOAA’s Coral Reef Watch greater Costa dos Corais Regional Virtual Station (CCRVS, red line in lower left map). The red star on the bottom left map marks the coastal center of the degree heating week (DHW) anomaly.
To compare coral cover and mortality to Sea Surface Temperature (SST) and its effects, we use Degree Heating Week (DHW) from NOAA’s Coral Reef Watch database, a well-established method that allows for global comparison (Wellington et al., 2001; Kayanne, 2017). Basically, the DHW product for Regional Virtual Stations is an accumulation of the 90th percentile Coral Bleaching Hot Spot values within each station; this figure provides a cumulative measurement of both the intensity and duration of the heat stress. Considering it is an accumulation over a 12-week period, there cannot be a DHW value until 12 weeks of Hot Spots have initiated. According to Duarte et al. (2020), corals exposed to values above 4 DHW bleach at a percentage of 30–40%, whereas corals exposed to DHW values above 8 results in bleaching of over 70%, with subsequent higher rates of coral mortality.
We have downloaded the available time series data file for the Regional Virtual Station “Costa dos Corais” in ASCII text format from https://coralreefwatch.noaa.gov/product/vs/data/costa_dos_corais.txt (NOAA Coral Reef Watch, 2020). We truncated the data, available since 1985, for the study period, February 8 to July 30, 2020, but we have also analyzed the complete series in order to provide a broader context.
The area analyzed and compiled for the MPA Costa dos Corais Regional Virtual Station (CCRVS) is about 2.5 × larger than our study area (Figure 1), extending both North and South from the MPA Costa dos Corais. In order to understand whether this has any effects in our comparisons, we have compiled DHW maps produced for the CCRVS (available at)1 for the duration of our detailed analysis and produced a short animation for quick reference (Supplementary Material 2). These maps show a more detailed SST and derived data (e.g., DHW) distribution for the CCRVS polygon, in 5 km2 pixel resolution. We were thus able to check the synchronicity between data for the entire CCRVS and for the MPA Costa dos Corais.
To better analyze the extent of the bleaching event in the MPA Costa dos Corais area, Sentinel-2A images were used dating from September 10, 2019 and June 1, 2020 (coinciding with values above 8.0 DHW in the region). Coral bleaching monitoring sites (Pedra do Meio, Aquário and Mãe Galé) served as the basis for image selection. Imagery was processed using the SNAP 8.0 software, and the Sen2Coral plugin based on Hedley et al. (2018). Changes in bottom reflectance data were taken to indicate a bleaching event.
Sentinel-2 images are made available by the ESA (European Space Agency) with a revisit rate of 5 days and spatial resolutions of 10, 20 and 60 m. Images with low incidence of clouds and high rate of return are required to detect bleaching. Previous research points to the potential of Sentinel-2 images for bleaching detection (Hedley et al., 2012, 2018; Collin et al., 2016; Wouthuyzen et al., 2019).
Coral community data collection was performed in 2018, 2019 and in February and July 2020 (pre- and post-bleaching periods) within the MPA Costa dos Corais at three different sites: Pedra do Meio, Aquário and Mãe da Galé (Figure 1). SCUBA diving was used to survey a 20 m × 5 m belt transect (2.5 m on the right and 2.5 m on the left) with four transects surveyed per site. Transects were performed at permanent transects points (e.g., surveys were conducted in the same general area), parallel to the coast line and ranging from 3 to 6 m depth. To measure the coral cover and categorize the substrate (benthic community), an adaptation of the Point Intercept Transect (PIT) was used where data was record along the central transect line. One caveat of concern with the PIT methodology is that line methods can yield a slightly higher coral cover, especially under conditions of high surge, because the line will tend to hang up on coral heads as well as sway in the current and consequently bias the result (Jokiel et al., 2015). At the same time the full width of belt transect method (Hill and Wilkinson, 2004; Leão et al., 2016) was used to assess the percentage of bleached corals and hydrocorals by number as well as to visually estimate coral mortality. Coral mortality was also estimated per belt transect (20 m × 5 m) corresponding to the proportion of colonies per transect per species for the most impacted and ecologically relevant coral species: Millepora braziliensis, Mussismilia harttii, and Millepora alcicornis.
Monitoring of the bleaching processes during the event was not possible due to restrictions on fieldwork resulting from the COVID-19 epidemic. Brazilian Environmental Ministry prohibited all visits and research activities in the MPA; also, due to team health and safety concerns, no fieldwork was performed from March to June 2020.
Analysis of variance “ANOVA” was used to test for differences in the benthic communities (“live coral cover”/“algal turf cover”) between the different surveys in 2018, 2019 and February and July 2020. Two-way ANOVA was used and “year” was used as a fixed factor and “live coral cover” and “algal turf cover” as dependent variables. Analysis of variance “ANOVA” was used following test of normality of the data tested by Shapiro–Wilk test. All statistical analyses were performed in R software with a significance level of P < 0.05.
In 2020, DHW values equal to or >4.0 started on March 14th and lasted until June 28th (107 days, mean 8.70), while DHW values above 8.0 started occurring on April 5th and lasted until June 7th (63 days, mean 10.63). DHW values above 10 lasted for 43 days (mean 11.41 DHW for the period) and reached a maximum value of 12.07 on May 7th, 2020. SST remained above the historic monthly mean for the whole period (Figure 2).
Figure 2. Sea surface temperature (SST) and degree heating week (DHW) for the marine protected area (MPA) Costa dos Corais Regional Virtual Station (CCRVS) from February 8 to July 30, 2020.
NOAA historical data spans 35 years, starting from 1985, when records became available for the CCRVS. Of the 15 highest daily SST values recorded, ranging from 29.8 to 30.0°C, 14 were in 2020. The exception occurred on March 26th, 1988 (29.8°C) occupying seventh place together with four others occurrences. Most extreme days occurred in the March-April 2020 period, including 7 extreme events measured on consecutive days in March (8–14) and 5 in April (11–15).
We illustrate in Figure 3 the ten largest DHW anomalies since 1985; all are above 2.0 DHW, with four very close to that threshold (below). Of the other six, two were between 4.0 and 6.0 DHW, and four were 8.0 or more. These four strongest anomalies occurred in 1998, 2010, 2019, and 2020. Eight out of the 10 years with the greatest DHW anomalies occurred in the last 15 years, which is less than half of the analyzed period.
Figure 3. Sea surface temperature (SST) and degree heating week (DHW) series for the ten largest anomalies since 1985, when records became available for the Costa dos Corais Regional Virtual Station (CCRVS). Black line highlights the 2020 thermal stress event.
Values above 4.0, which indicate potential coral bleaching and mortality, were recorded in the years 1988, 1998, 2010, 2017, 2019 and 2020, with increasing intensity. Values above 10.0 had not been previously recorded by the CCRVS. The 2020 DHW anomaly was both the highest and the most enduring, while the 2020 SST being, on average, the furthest from the mean monthly climatology.
Values higher than 8.0 DHW in 2020 occurred at APACC for 2 months, between April 7th and June 5th. First located about 20 km south of Maragogi (our field work location), these areas were also the last to have high DHW values (please refer to Figure 1 and the animation in the Supplementary Material for location). NOAA data recorded by CCRVS refers to a larger area (∼2.5 the MPA area), and average values above 8.0 DHW started 2 days earlier and lasted an additional day (please refer to the NOAA bulk data in the Supplementary Material). This similarity in behavior allows us to extrapolate the data from the entire CCRVS polygon as a proxy for the MPA Costa dos Corais.
It was possible to detect the extent of the bleaching event close to the DHW peak on June 1, 2020 by comparison with an image from September 10, 2019 for the same region (Figure 4). An increase in bottom reflectance from 0.139 (September 2019) to 0.340 (June 2020) indicate a bleaching event (Table 1). According to the images, 28% (0.17 km2) of the selected reef area (0.62 km2) had bleached. Coral mortality at the same site was confirmed through field research on July 21, 2020.
Figure 4. Visible bleaching on Sentinel-2A images at the Aquario reef in June 2020, compared to September 2019. The two smaller images are those which reflectance was compared and show a zoomed area as represented by a black rectangle in the upper left panel.
Live coral cover in 2018 was 50.2 ± 9.1% (mean ± SD), and in 2019 was 44.1 ± 12.2%, and in the 2020 pre-bleaching period 48.1 ± 8.75%, with no significant difference recorded among those records (F = 0.18; p = 0.83) (Figure 5A). In contrast, in 2020 post-bleaching, live coral cover was 30.1 ± 7.1% (mean ± SD), a statistically significant reduction on live coral cover compared to previous surveys (F = 7.12; p < 0.05). This amounted to a mean reduction of 18.1% on live coral cover comparing the 2020 pre- and post-bleaching records (Figure 5A).
Figure 5. Live coral cover (%) (A) and algal turf cover (B) on marine protected area (MPA) Costa dos Corais with temporal data and pre/post bleaching period. *Means significant differences.
Algal turf cover in 2018 was 25.1 ± 8.5% (mean ± SD), in 2019 20.8 ± 12.4%, and in the 2020 pre-bleaching period 26.9 ± 6.6%, with no statistically significant differences between those records (F = 1.08; p = 0.34) (Figure 5B). In contrast, in 2020 post-bleaching, algal turf cover was 46.2 ± 10.1% (average ± SD) a statistically significant increase compared to previous surveys (F = 8.16; p < 0.05). This amounted to a mean increase of 19.3% on algal turf cover comparing the 2020 pre and post-bleaching records (Figure 5B).
Coral mortality at the post-bleaching survey was significantly greater for three species, Millepora alcicornis, Millepora braziliensis, and Mussismilia harttii, the last two being endemic species. Mean mortality per transect was 50.8 ± 35.9% (average ± SD) for Millepora braziliensis, 32.6 ± 11.6% for Mussismilia harttii and 16.6 ± 8.1% for Millepora alcicornis (Figures 6, 7).
Figure 6. Coral mortality per transect at marine protected area (MPA) Costa dos Corais during post-bleaching period for Mussismilia harttii, Millepora braziliensis, and Millepora alcicornis,
Figure 7. Coral mortality records for Millepora alcicornis (A,B) and for the endemic Mussismilia harttii (C–F) at marine protected area (MPA) Costa dos Corais during post-bleaching period. Dead corals are brownish and covered by algae for (A,B) and coral polyps are pale and covered by sediment for (C–F).
Our findings demonstrate unprecedented coral mortality following the most severe thermal stress event recorded at the largest Brazilian coastal MPA, originally designated to protect one of the largest coral reef systems on the SWA Ocean. DHW values went above 10 for 43 days, the greatest and the most enduring thermal stress event recorded since DHW values were first determined in 1985. Following this thermal stress, a mean reduction of 18.1% in live coral cover and a mean increase in algal turf of 19.3% compared to previous years was recorded in our study area. Mortality was high for three species, with up to 100% mortality on some belt transect for Millepora braziliensis, 55% for Mussismilia harttii and 27% for Millepora alcicornis (Figure 7) and Suplementary Material 3 (coral mortality video). Our data indicate that the populations of two Brazilian endemic coral species are under severe threat and suggest that the concept of the SWA serving as a “thermal” refugia (Mies et al., 2020) should be taken with caution. Coral mortality associated with thermal stress has already been recorded for most other coral reefs regions, including the Great Barrier Reef and Caribbean reefs (Neal et al., 2017; Hughes et al., 2018a; Duarte et al., 2020; Soares et al., 2021). For instance, a major decline in mean coral cover from 28.0 to 13.8% (0.53% y–1), a loss of 50.7% of the initial coral cover, has been recorded for the Great Barrier Reef (De’ath et al., 2012).
Unfortunately, due to the COVID-19 pandemic and consequent health and safety procedures adopted in Brazil, our team was not able to survey the coral reef area during the peak of the thermal stress event (April to June 2020). However, our pre-bleaching survey (February 2020) and the post-bleaching data (July 2020) clearly demonstrate that, even though bleaching percentage was not evaluated, mortality was high for the three species monitored: M. harttii, M. braziliensis, and M. alcicornis. These species represent ecologically relevant species (e.g., reef bio constructors and habitat for fish/invertebrates) as well as Brazilian endemics (Leal et al., 2015; Soares et al., 2021). Hence, population reduction would likely also compromise SWA coral reef ecosystem and ecosystem functioning.
High coral mortality due to thermal stress at SWA has been only recently demonstrated (Banha et al., 2019; Duarte et al., 2020; Teixeira and Creed, 2020; Ferreira et al., 2021; Soares et al., 2021). Two recent studies recorded high mortality levels for the hydrocoral Millepora alcicornis of up to 89.1% (Duarte et al., 2020) and 90% (Ferreira et al., 2021). Yet, our study also recorded one of the highest coral mortally observed following thermal stress in the SWA, with the endemic and endangered Brazilian brain coral (Mussismilia harttii) averaging a 32.6% mortality, compared to the 12.9% of mortality recorded by Duarte et al. (2020). Leão et al. (2008) suggested that more than 10% of a local M. harttii population bleached in Bahia State with a thermal stress of up to 0.25°C over a week. Recent modeling predicts further impact on the species leading to a reduction of its coastal distribution, and possibly local extinction in the next decade, with temperature being the main predictive variable (De Oliveira et al., 2019).
It is possible that additional impacts at MPA Costa dos Corais could have intensified coral mortality during the period of the 2020 bleaching event. The largest oil spill to have affected SWA coral reefs occurred at the end of 2019 and extended into early 2020, with at least 5 k tons of crude oil reaching several ecosystems, including mangroves, seagrass and algae beds as well as coral reefs (Magalhães et al., 2020; Miranda et al., 2020; Soares et al., 2020). One of the worst affected areas was our study area, with oil recorded at around 80 sites within the MPA. Metagenomic analyses are currently ongoing to better understand the role of the microbiome under such circumstances, and whether such an additional impact would be expected to have a synergistic effect.
Mies et al. (2020) recently suggested that the SWA might be able to provide corals with refugia from ocean warming because of such factors as deeper bathymetric distribution, higher tolerance to turbidity, higher tolerance to nutrient enrichment and a more resistant morphology of species. Additionally, no recent mass coral mortality episodes associated with the three global mass bleaching events had been reported for the SWA (Mies et al., 2020). However, the recent and intense heat waves of 2019 and 2020 caused high mortality rates in three structural coral species (e.g., Millepora alcicornis, Millepora braziliensis, and Mussismilia harttii) (Soares et al., 2021) suggesting that recent thermal stress levels in our part of the SWA were above the levels required for the area to serve as a thermal refugium.
Our findings (a mean reduction of 18.1% in live coral cover, and up to 100% of coral mortality of selected species on some transects) suggest that caution should be taken into considering the SWA as a region able to provide thermal refugia for corals more effectively than the Caribbean or Indo-Pacific. Regional ocean warming appears to have intensified in recent years, and the 2020 heat wave seems to have exposed SWA reefs to unprecedented levels of thermal stress, causing the worst recorded coral mortality in the area (Duarte et al., 2020; Teixeira and Creed, 2020; Ferreira et al., 2021; Soares et al., 2021). Mies et al. (2020) assumptions could be based on previous thermal stress models and should be revisited. If considered thermal refugium, a governmental reduction on the priority of coral conservation strategies and climate change mitigation for SWA could jeopardize these unique coral reef ecosystems.
The long-term significance of the impact on the community, structure, and function of Costa dos Corais coral reefs, caused by the mortality documented here, and the implications for the future of Brazilian reef ecosystem, remain unclear. Hence, continued spatial-temporal monitoring will be critical to understand if these reefs can recover after this mortality episode (Gilmour et al., 2013). In contrast to Caribbean and Indo-Pacific reefs, often dominated by branching corals with higher growth rates and eventual recovery (Pisapia et al., 2016), Atlantic reefs are dominated by massive coral forms with low growth rates and limited capacity to recover after mortality events. Considering the warming of ocean surface temperatures by at least 2°C predicted for the near future (Hoegh-Guldberg et al., 2007), we could face an irreversible loss of endemic species such as Millepora braziliensis and Mussismilia hartii, followed by functional extinction of Northern Brazilian coral reefs in the next few decades. The local extinction of endemic coral species is extremely relevant to coral reef conservation and is a challenge to be properly evaluated by future research. Our results should be considered in environmental policy-making, directed toward a strategic plan for managing SWA coral reefs in the face of global warming. Dixon et al. (2022) has shown that climate change will overwhelm current local-scale refugia, with declines in global thermal refugia from 84% of global coral reef pixels in the present-day climate to 0.2% at 1.5°C, and 0% at 2.0°C of global warming. Hence, focusing management efforts on thermal refugia may be effective only in the short-term We reinforce the view that strong and urgent actions to reduce carbon emission, the root cause of coral reef decline, must be immediately implemented (Hughes et al., 2018b, 2019; Bruno et al., 2019).
The raw data supporting the conclusions of this article will be made available by the authors, without undue reservation.
PP, GL, AP, LC, and EG conceived the experiment and conducted the experiment(s). JA and JS curated remote sensing data. PP, GVL, AP, LC, EG, JA, and JS analyzed the results. All authors wrote and reviewed the manuscript.
This study was financed by Rufford Small Grants, Conservation Leadership Program (CLP), Marine Conservation Action Fund, and Mohamed Bin Zayed Species Conservation grants for PHCP and PCR.
The authors declare that the research was conducted in the absence of any commercial or financial relationships that could be construed as a potential conflict of interest.
All claims expressed in this article are solely those of the authors and do not necessarily represent those of their affiliated organizations, or those of the publisher, the editors and the reviewers. Any product that may be evaluated in this article, or claim that may be made by its manufacturer, is not guaranteed or endorsed by the publisher.
We would like to thank MPA Costa dos Corais (APACC) management team and Projeto Conservação Recifal staff for support. This study was conducted under the full approval of the Sistema Nacional de Informação sobre Biodiversidade (SISBIO) by the Brazilian Government, permit #67684-1. We thank Fundação SOS Mata Atlântica and Fundação Toyota for support during MPA Costa dos Corais field trips. We also acknowledge public data providers from NOAA Coral Reef Watch and the European Space Agency Copernicus program.
The Supplementary Material for this article can be found online at: https://www.frontiersin.org/articles/10.3389/fmars.2022.725778/full#supplementary-material
Anthony, K. R. N. (2006). Enhanced energy status of corals on coastal, high-turbidity reefs. Mar. Ecology-Prog. Series 319, 111–116. doi: 10.3354/meps319111
Baker, A. C., Glynn, P. W., and Riegl, B. (2008). Climate change and coral reef bleaching: an ecological assessment of long-term impacts, recovery trends and future outlook. Estuarine Coastal Shelf Sci. 80, 435–471. doi: 10.1016/j.ecss.2008.09.003
Banha, T. N. S., Capel, K. C. C., Kitahara, M. V., Francini-Filho, R. B., Francini, C. L. B., Sumida, P. Y. G., et al. (2019). Low coral mortality during the most intense bleaching event ever recorded in subtropical Southwestern Atlantic reefs. Coral Reefs 39, 515–521. doi: 10.1007/s00338-019-01856-y
Bruno, J. F., Côté, I. M., and Toth, L. T. (2019). Climate change, coral loss, and the curious case of the parrotfish paradigm: why don’t marine protected areas improve reef resilience? Ann. Rev. Mar. Sci. 11, 307–334. doi: 10.1146/annurev-marine-010318-095300
Castro, C. B., and Pires, D. O. (1999). A bleaching event on a Brazilian coral reef. Rev. Brasileira Oceanografia 47, 87–90. doi: 10.1007/s00248-012-0095-x
Collin, A., Laporte, J., Koetz, B., Martin-Lauzer, F. R., and Desnos, Y. L. (2016). “Mapping bathymetry, habitat, and potential bleaching of coral reefs using Sentinel-2,” in Proceedings of the 13th International Coral Reef Symposium, (Honolulu).
De Oliveira, U. D. R., Gomes, P. B., Silva Cordeiro, R. T., de Lima, G. V., and Pérez, C. D. (2019). Modeling impacts of climate change on the potential habitat of an endangered Brazilian endemic coral: discussion about deep sea refugia. PLoS One 14:e0211171. doi: 10.1371/journal.pone.0211171
De’ath, G., Fabricius, K. E., Sweatman, H., and Puotinen, M. (2012). The 27-year decline of coral cover on the Great Barrier Reef and its causes. Proc. Natl. Acad. Sci. U S A. 109, 17995–17999. doi: 10.1073/pnas.1208909109
Dixon, A. M., Forster, P. M., Heron, S. F., Stoner, A. M., and Beger, M. (2022). Future loss of local-scale thermal refugia in coral reef ecosystems. PLoS Climate 1:e0000004. doi: 10.1371/journal.pclm.0000004
Donner, S. D., and Carilli, J. (2019). Resilience of Central Pacific reefs subject to frequent heat stress and human disturbance. Sci. Rep. 9:3484. doi: 10.1038/s41598-019-40150-40153
Duarte, G. A. S., Villela, H. D. M., Deocleciano, M., Silva, D., Barno, A., Cardoso, P. M., et al. (2020). Heat waves are a major threat to turbid coral reefs in Brazil. Front. Mar. Sci. 7:179. doi: 10.3389/fmars.2020.00179
Eakin, C. M., Sweatman, H. P. A., and Brainard, R. E. (2019). The 2014–2017 global-scale coral bleaching event: insights and impacts. Coral Reef. 38, 539–545.
Ferreira, B. P., Costa, M. B. S. F., Coxey, M. S., Gaspar, A. L. B., Veleda, D., and Araujo, M. (2013). The effects of sea surface temperatures anomalies on oceanic coral reef systems in the southwestern tropical Atlantic. Coral Reefs 32, 441–454. doi: 10.1007/s00338-012-0992-y
Ferreira, L. C. L., Grillo, A. C., Repinaldo Filho, F. P. M., Souza, F. N. R., and Longo, G. O. (2021). Different responses of massive and branching corals to a major heatwave at the largest and richest reef complex in South Atlantic. Mar. Biol. 168:54.
Gilmour, J. P., Smith, L. D., Heyward, A. J., Baird, A. H., and Pratchett, M. S. (2013). Recovery of an isolated coral reef system following severe disturbance. Science 340, 69–71. doi: 10.1126/science.1232310
Glynn, P. W. (1996). Coral reef bleaching: facts, hypotheses and implications. Global Change Biol. 2, 495–509. doi: 10.1111/j.1365-2486.1996.tb00063.x
Graham, N. A., Jennings, S., MacNeil, M. A., Mouillot, D., and Wilson, S. K. (2015). Predicting climate-driven regime shifts versus rebound potential in coral reefs. Nature 518, 94–97. doi: 10.1038/nature14140
Hedley, J. D., Roelfsema, C., Brando, V., Giardino, C., Kutser, T., Phinn, S., et al. (2018). Coral reef applications of Sentinel-2: coverage, characteristics, bathymetry and benthic mapping with comparison to landsat 8. Remote Sensing Environ. 216, 598–614. doi: 10.1016/j.rse.2018.07.014
Hedley, J. D., Roelfsema, C., Koetz, B., and Phinn, S. (2012). Capability of Sentinel 2 mission for tropical coral reef mapping and coral bleaching detection. Rem. Sens. Environ. 120, 145–155. doi: 10.1016/j.rse.2011.06.028
Hill, J., and Wilkinson, C. L. I. V. E. (2004). Methods for Ecological Monitoring of Coral Reefs. Townsville, QLD: Australian Institute of Marine Science.
Hoegh-Guldberg, O., Mumby, P. J., Hooten, A. J., Steneck, R. S., Greenfield, P., Gomez, E., et al. (2007). Coral reefs under rapid climate change and ocean acidification. Science 318, 1737–1742. doi: 10.1126/science.1152509
Hughes, T. P., Kerry, J. T., Álvarez-Noriega, M., Álvarez-Romero, J. G., Anderson, K. D., Baird, A. H., et al. (2017). Global warming and recurrent mass bleaching of corals. Nature 543, 373–377. doi: 10.1038/nature21707
Hughes, T. P., Anderson, K. D., Connoly, S. R., Heron, S. F., Kerry, J. T., Lough, J. M., et al. (2018a). Spatial and temporal patterns of mass bleaching of corals in the Anthropocene. Science 359, 80–83. doi: 10.1126/science.aan8048
Hughes, T. P., Kerry, J. T., Baird, A. H., Connolly, S. R., Dietzel, A., Eakin, C. M., et al. (2018b). Global warming transforms coral reef assemblages. Nature 556, 492–496. doi: 10.1038/s41586-018-0041-42
Hughes, T. P., Kerry, J. T., Baird, A. H., Connolly, S. R., Chase, T. J., Dietzel, A., et al. (2019). Global warming impairs stock-recruitment dynamics of corals. Nature 568, 387–390. doi: 10.1038/s41586-019-1081-y
Jiang, J., Wang, A., Deng, X., Zhou, W., Gan, Q., and Lu, Y. (2021). How Symbiodiniaceae meets the challenges of life during coral bleaching. Coral Reefs 40, 1339–1353.
Jokiel, P. L., Rodgers, K. S., Brown, E. K., Kenyon, J. C., Aeby, G., Smith, W. R., et al. (2015). Comparison of methods used to estimate coral cover in the Hawaiian Islands. PeerJ 3:e954. doi: 10.7717/peerj.954
Kayanne, H. (2017). Validation of degree heating weeks as a coral bleaching index in the northwestern Pacific. Coral Reefs 36, 63–70. doi: 10.1007/s00338-016-1524-y
Kikuchi, R. K. P., Leão, Z. M. A. N., Testa, V., Dutra, L. X. C., and Spanó, S. (2003). Rapid assessment of the Abrolhos Reefs. Eastern Brazil (Part 1: stony corals and algae). Atoll Res. Bull. 496, 172–187. doi: 10.5479/si.00775630.496-9.172
Krug, L. A., Gherardi, D. F. M., Stech, J. L., Leão, Z. M. A. N., and Kikuchi, R. K. P. (2012). Characterization of coral bleaching environments and their variation along the Bahia state coast, Brazil. Int. J. Remote Sens. 33, 4059–4074. doi: 10.1080/01431161.2011.639505
Krug, L. A., Gherardi, D. F. M., Stech, J. L., Leão, Z. M. A. N., Kikuchi, R. K. P., Hruschka-Júnior, E. R., et al. (2013). The construction of causal networks to estimate coral bleaching intensity. Environ. Model. Software 42, 157–167. doi: 10.1016/j.envsoft.2013.01.003
Leal, I. C. S., de Araújo, M. E., da Cunha, S. R., and Pereira, P. H. C. (2015). The influence of fire-coral colony size and agonistic behaviour of territorial damselfish on associated coral reef fish communities. Mar. Environ. Res. 108, 45–54. doi: 10.1016/j.marenvres.2015.04.009
Leão, Z. M. A. N., Kikuchi, R., and Testa, V. (2003). “Corals and coral reefs of Brazi,” in Latin American Coral Reefs, ed. J. Cortés (Amsterdam: Elsevier).
Leão, Z. M. N., Kikuchi, R. P. K., and Oliveira, M. D. M. (2008). Branqueamento de corais nos recifes da Bahia e sua relação com eventos de anomalias térmicas nas águas superficiais do oceano. Biota Neotroprica 8, 69–82. doi: 10.1590/s1676-06032008000300006
Leão, Z. M., Kikuchi, R. K., Ferreira, B. P., Neves, E. G., Sovierzoski, H. H., Oliveira, M. D., et al. (2016). Brazilian coral reefs in a period of global change: a synthesis. Brazilian J. Oceanography 64, 97–116. doi: 10.1590/s1679-875920160916064sp2
Loya, Y., Sakai, K., Yamazato, K., Nakano, Y., Sambali, H., and van Woesik, R. (2001). Coral bleaching: the winners and the losers. Ecol. Lett. 4, 122–131. doi: 10.1046/j.1461-0248.2001.00203.x
Magalhães, K. M., Barros, K. V. S., Lima, M. C. S., Barreira, C. C. A. R., RosaFilho, J. S., and Soares, M. O. (2020). OilSpill+COVID -19: a disastrous year for Brazilian Seagrass Conservation. Sci. Total Environ. 764:142872. doi: 10.1016/j.scitotenv.2020.142872
Magel, J. M. T., Burns, J. H. R., Gates, R. D., and Baum, J. K. (2019). Effects of bleaching-associated mass coral mortality on reef structural complexity across a gradient of local disturbance. Sci. Rep. 9:2512. doi: 10.1038/s41598-018-37713-1
Maida, M., and Ferreira, B. P. (1997). Coral Reefs of Brazil: overview and field guide. Proc. 8th Int Coral Reef Sym. 1:74.
Marshall, P., and Baird, A. (2000). Bleaching of corals on the Great Barrier Reef: differential susceptibilities among taxa. Coral Reefs 19, 155–163. doi: 10.1007/s003380000086
Mies, M., Francini-Filho, R. B., Zilberberg, C., Garrido, A. G., Longo, G. O., Laurentino, E., et al. (2020). South Atlantic coral reefs are major global warming refugia and less susceptible to bleaching. Front. Mar. Sci. 7:514. doi: 10.3389/fmars.2020.00514
Migotto, A. E. (1995). “Anthozoa bleaching on the southeastern coast of Brazil in the summer of 1994,” in Proceedings of the 6th International Conference on Coelenterate Biology, Leiden.
Miranda, R. J., Cruz, I. C. S., and Leão, Z. (2013). Coral bleaching in the Caramuanas reef (Todos os Santos Bay, Brazil) during the 2010 El Niño event. Latin Am. J. Aquatic Res. 41, 351–360.
Miranda, R. J., Malhado, A. C. M., Fabré, N., Batista, V., Santos, R., Campos-Silva, J., et al. (2020). A. Integrating long term ecological research (LTER) and marine protected area management: challenges and solutions. Oecol. Australis 24, 279–300.
Neal, B. P., Khen, A., Treibitz, T., Beijbom, O., O’Connor, G., Coffroth, M. A., et al. (2017). Caribbean massive corals not recovering from repeated thermal stress events during 2005-2013. Ecol. Evol. 7, 1339–1353. doi: 10.1002/ece3.2706
NOAA Coral Reef Watch (2020). Updated Daily. NOAA Coral Reef Watch Version 3.1 Daily Global 5-km Satellite Coral Bleaching Degree Heating Week Product, Feb. TypeCollege Park, MD: NOAA Coral Reef Watch.
Pereira, P. H. C., Macedo, C. H., Nunes, J. D. A. C., Marangoni, L. F. D. B., and Bianchini, A. (2018). Effects of depth on reef fish communities: insights of a “deep refuge hypothesis” from Southwestern Atlantic reefs. PLoS One 13:e0203072. doi: 10.1371/journal.pone.0203072
Pereira, P. H. C., Ternes, M. L. F., Nunes, J. A. C., and Giglio, V. J. (2021a). Overexploitation and behavioral changes of the largest South Atlantic parrotfish (Scarus trispinosus): evidence from fishers’ knowledge. Biol. Conserv. 254:108940. doi: 10.1016/j.biocon.2020.108940
Pereira, P. H. C., Côrtes, L. G. F., Lima, G. V., and Sampaio, C. L. S. (2021b). Reef fishes biodiversity and conservation at the largest Brazilian coastal Marine Protected Area (MPA Costa dos Corais). Neotropical Ichthyol. 19. doi: 10.1590/1982-0224-2021-0071
Pisapia, C., Burn, D., Yoosuf, R., Najeeb, A., Anderson, K. D., and Pratchett, M. S. (2016). Coral recovery in the central Maldives archipelago since the last major mass-bleaching, in 1998. Sci. Rep. 6:34720. doi: 10.1038/srep34720
Soares, M. O., and Rabelo, E. F. (2014). Primeiro registro de branqueamento de corais no litoral do Ceará (NE, Brasil): indicador das mudanças climáticas? Geociências 33, 1–10.
Soares, M. O., Rossi, S., Gurgel, A. R., Lucas, C. C., Tavares, T. C. L., Diniz, B., et al. (2021). Impacts of a changing environment on marginal coral reefs in the Tropical Southwestern Atlantic. Ocean Coastal Manag. 210:105692. doi: 10.1016/j.ocecoaman.2021.105692
Soares, M., Teixeira, C. E. P., Bezerra, L. E. A., Paiva, S. V., Tavares, T. C. L., Garcia, T. M., et al. (2020). Oil spill in South Atlantic (Brazil): environmental and governmental disaster. Mar. Policy 115:103879.
Spalding, M. D., and Brown, B. E. (2015). Warm-water coral reefs and climate change. Science 350, 769–771. doi: 10.1126/science.aad0349
Sully, S., Burkepile, D. E., Donovan, M. K., Hodgson, G., and van Woesik, R. (2019). A global analysis of coral bleaching over the past two decades. Nat. Commun. 10:1264. doi: 10.1038/s41467-019-09238-2
Teixeira, C. D., Leitão, R. L. L., Ribeiro, F. V., Moraes, F. C., Neves, L. M., Bastos, A. C., et al. (2019). Sustained mass coral bleaching (2016-2017) in Brazilian turbid-zone reefs: taxonomic, cross-shelf and habitat-related trends. Coral Reefs 38, 801–813. doi: 10.1007/s00338-019-01789-1786
Teixeira, L. M. P., and Creed, J. C. (2020). A decade on: an updated assessment of the status of marine non-indigenous species in Brazil. Aquatic Invasions 15, 30–43. doi: 10.3391/ai.2020.15.1.03
Wellington, G. M., Glynn, P. W., Strong, A. E., Navarrete, S. A., Wieters, E., and Hubbard, D. (2001). Crisis on coral reefs linked to climate change. EOS Trans. Am. Geophys. Union 82, 1–5. doi: 10.1038/srep08402
Wouthuyzen, S., Abrar, M., Corvianawatie, C., Salatalohi, A., Kusumo, S., Yanuar, Y., et al. (2019). “The potency of Sentinel-2 satellite for monitoring during and after coral bleaching events of 2016 in the some islands of Marine Recreation Park (TWP) of Pieh, West Sumatra,” in Proceedings of the IOP Conference Series: Earth and Environmental Science, (Bristol: IOP Publishing).
Keywords: coral reefs, coral bleaching, coral mortality, brazilian mpa, mpa costa dos corais
Citation: Pereira PHC, Lima GV, Pontes AVF, Côrtes LGF, Gomes E, Sampaio CLS, Pinto TK, Miranda RJ, Cardoso ATC, Araujo JC and Seoane JCS (2022) Unprecedented Coral Mortality on Southwestern Atlantic Coral Reefs Following Major Thermal Stress. Front. Mar. Sci. 9:725778. doi: 10.3389/fmars.2022.725778
Received: 15 June 2021; Accepted: 21 February 2022;
Published: 20 May 2022.
Edited by:
Stefano Goffredo, University of Bologna, ItalyReviewed by:
Cristiane Cassiolato Pires Hardoim, São Paulo State University, BrazilCopyright © 2022 Pereira, Lima, Pontes, Côrtes, Gomes, Sampaio, Pinto, Miranda, Cardoso, Araujo and Seoane. This is an open-access article distributed under the terms of the Creative Commons Attribution License (CC BY). The use, distribution or reproduction in other forums is permitted, provided the original author(s) and the copyright owner(s) are credited and that the original publication in this journal is cited, in accordance with accepted academic practice. No use, distribution or reproduction is permitted which does not comply with these terms.
*Correspondence: Pedro H. C. Pereira, cGVkcm9oY3AyQHlhaG9vLmNvbS5icg==
Disclaimer: All claims expressed in this article are solely those of the authors and do not necessarily represent those of their affiliated organizations, or those of the publisher, the editors and the reviewers. Any product that may be evaluated in this article or claim that may be made by its manufacturer is not guaranteed or endorsed by the publisher.
Research integrity at Frontiers
Learn more about the work of our research integrity team to safeguard the quality of each article we publish.