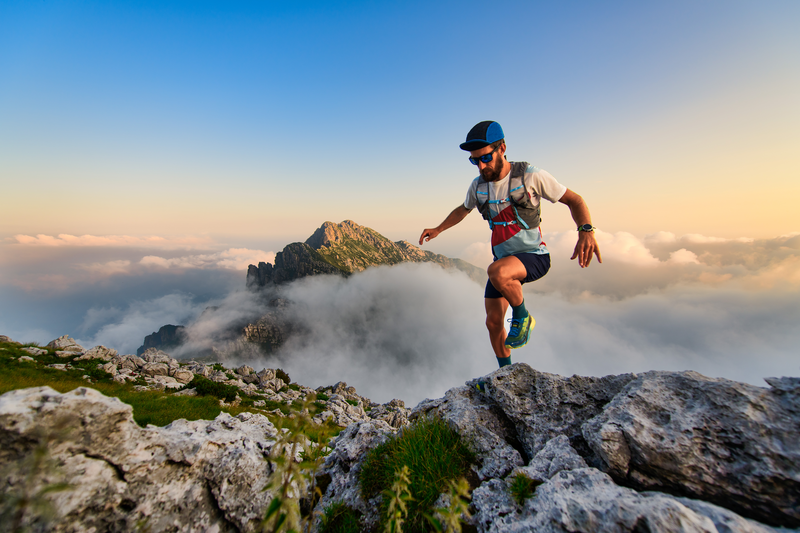
94% of researchers rate our articles as excellent or good
Learn more about the work of our research integrity team to safeguard the quality of each article we publish.
Find out more
ORIGINAL RESEARCH article
Front. Mar. Sci. , 09 December 2022
Sec. Marine Pollution
Volume 9 - 2022 | https://doi.org/10.3389/fmars.2022.1099984
This article is part of the Research Topic Toxicological Endpoints and Bioavailability of Emerging Contaminants and their Impacts on Marine Nutrient Cycles View all 6 articles
Organic ultraviolet filters (OUVFs) and nanoplastics (NPs) are two important new pollutants that coexist widely in freshwater and coastal habitats. Both OUVFs and MPs can be taken up by aquatic organisms and may be toxic or co-toxic. In this study, the neurotoxicity and interaction neurotoxicity of the typical OUVFs butyl methoxydibenzoyl methane (BM-DBM) and polystyrene nanoplastics (PS-NPs) on zebrafish at environmental concentrations were comprehensively investigated at the tissue and molecular levels using transgenic or non-transgenic zebrafish. Results showed that both BM-DBM and PS-NPs induced abnormal expression of developmental genes neurogenin 1, glial fibrillary acidic protein, Finkel-Biskis-Jinkins osteosarcoma oncogene, growth-associated protein 43, synapsin IIa, and apoptosis regulator a (Bcl2a), Bcl2 associated x and apoptosis-related cysteine peptidase a, neuronal loss, motor neuron axon deletion, neuronal apoptosis and behavioral abnormalities in larvae thus being neurotoxic. BM-DBM also specifically interferes with neurotransmitter systems and affects signaling between neurons. In addition, PS-NPs and BM-BDM exerted synergistic and antagonistic effects in inducing apoptosis in neuronal cells and developmental neurotoxicity, respectively. Our results highlight the neurotoxic risk of PS-NPs and BM-BDM and the complex interactive neurotoxic effects of the two. We anticipate that our study may lay the foundation for ecological risk assessment of OUVFs and PS-NPs and exploration of complex interactions between PS-NPs and hydrophobic organic pollutants.
Global climate change has led to a rapid increase in the amount of UV radiation reaching the earth (Zhou et al., 2022a). United States Environmental Protection Agency show that 90% of all skin cancers are caused by UV radiation (EPA, 2015). In response to this situation, organic ultraviolet (UV) filters (OUVFs, a collective term for aromatic organic compounds with carbonyl conjugates or heterocycles) with UV-A (315-400 nm) and UV-B (280-315 nm) absorption properties are widely produced and added to personal care products such as sunscreens and skin care products to protect human skin health (Huang et al., 2021). The concentration of OUVFs in these daily sunscreen products is as high as 10%, thus the potential risk of long-term input of OUVFs into the environment cannot be ignored (Xie et al., 2022).
With the washing of skin from human water recreation activities (e.g., swimming and beach bathing), the tailwater discharge from centrally treated domestic wastewater, and the direct release of domestic wastewater without centralized treatment from rural residents, OUVFs can eventually be discharged into water bodies and persist, especially in coastal areas with a developed maritime recreation industry, with overall levels at the ng/L-µg/L level and a few even at the mg/L level (Zhou et al., 2022a). Butyl methoxydibenzoyl methane (BM-DBM) is one of the main occurrences of OUVFs in the environment, whose levels in surface water (Rodriguez et al., 2015) and sediment (Tsui et al., 2015) can reach up to 1770.3 ng/L and 64.5 ng/g dry weight (dw), respectively, with an overall high level of contamination. In addition, OUVFs usually have high octanol-water partition coefficients (logKow = 4-8), which are prone to accumulate in the body and produce various toxic effects after ingestion by aquatic organisms (Zhou et al., 2022b). Ma et al. (2017) found a significant decrease in acetylcholinesterase (AChE) and catalase activities and a significant increase in superoxide dismutase and glutathione reductase activities in crucian carp (Carassius auratus) after 28 days of exposure to BM-DBM, indicating an impairment of the nervous system and antioxidant defense system. Liu et al. (2021a) also found that exposure of zebrafish embryos to BM-DBM induced a significant increase in heart rate and AChE activity, a significant decrease in locomotor activity, and a significant downregulation of DNA methyltransferase 1 and DNA methyltransferase 3b expression in larvae, which exhibited neurotoxicity and genotoxicity.
Microplastics (MPs), also a new pollutant, refer to organic polymeric plastic particles with particle sizes between 100 nm and 5 mm, of which polystyrene (PS) is the most commonly used one (Li et al., 2018; Jain et al., 2021; Zhou et al., 2022b). MPs below 100 nm, also known as nanoplastics (NPs), are usually derived from the fragmentation of large-size plastic waste. MPs are very closely related to OUVFs. On the one hand, OUVFs can be used in plastic products (e.g., textiles and coatings) to prevent the yellowing or aging of the material due to UV light (Ma et al., 2017). On the other hand, plastic particles can be widely added to personal care products such as scrubs and foundations as an alternative to natural abrasives to increase cleaning ability or fill pores for aesthetic effects (Kalikova et al., 2017; Lei et al., 2017). Therefore, OUVFs and MPs can be discharged into the environment simultaneously and coexist extensively. Existing studies have shown that MPs can adsorb and concentrate hydrophobic organic pollutants and influence the bioavailability and toxic effects of pollutants through carrier effects due to their small specific surface area and strong hydrophobicity (Santos et al., 2021; Sun et al., 2021). Liu et al. (2021a) had performed adsorption experiments of BM-DBM with 100 nm PS-NPs and found that PS-NPs adsorbed BM-DBM and caused a decrease in its concentration to 46% of the original level. They also found that the combined exposure of BM-DBM and PS-NPs attenuated the oxidative stress level in zebrafish (Danio rerio) and attributed it to the decrease in dissolved BM-DBM. However, Zhou et al. (2021) found that PS-NPs significantly elevated the tissue accumulation levels of the co-occurring contaminant ethylhexyl salicylate (EHS) in zebrafish (Danio rerio), as well as EHS-induced oxidative stress and thyrotoxic effects. Therefore, the carrier effects of PS-NPs on contaminants are very complex and need further exploration.
In this study, typical OUVFs BM-DBMs and 100 nm PS-NPs were selected as target contaminants, and non-transgenic (AB strain) and transgenic (Tg: HuC-GFP, Tg: Hb9-GFP) zebrafish embryos were selected as the subject organisms for indoor exposure simulation experiments to verify the following three hypotheses: 1) Both BM-DBMs and PS-NPs can cause abnormal growth and development and behavioral abnormalities in zebrafish embryos/larvae; 2) Both BM-DBM and PS-NPs can induce abnormal neuronal/motor neuron development, neurotransmitter system disorders and neuronal apoptosis in transgenic larvae; 3) BM-DBM and PS-NPs may produce interactive neurotoxicity on zebrafish.
BM-DBM (purity > 98%, CAS-no: 70356-09-1, Ref-code: CDAA-1130003) and fluorescent PS-NPs (100 nm, 10 mg/mL, Ref-code: SEQ-G-001) were supplied by AccuStandard Inc (NewHaven, USA) and Tianjin Saierqun Technology Co., Ltd (Tianjin, China), respectively. BM-DBM and PS-NPs stock solutions and dilutions of different concentrations were prepared by dimethyl sulfoxide (DMSO; purity > 99.9%; Amresco, Solon, USA). Additional information on procuring other products can be found in the Supplementary Materials (SM).
According to the manufacturer, the MPs used in this experiment were pristine particles without adding any adjuvants and chemicals. Dynamic light scattering and transmission electron microscopy were used to determine or observe the particle size distribution and morphology of PS-NPs. Results showed that the PS-NPs used in the experiments were spherical particles with an average particle size of 108.8 ± 25.8 nm (Figure 1), which was in general agreement with that described by the manufacturer.
Figure 1 Scanning electron microscopy (A) and Hydrodynamic diameter distribution (B) Exposure of non-transgenic zebrafish embryos.
The non-transgenic zebrafish embryos (Danio rerio) selected for this experiment were all healthy embryos from the same batch, with normal fertilization and reaching the blastocyst stage (2 h after fertilization) (EzeRinka Biotechnology Co., Ltd, Nanjing, China). 500 embryos were divided into 5 groups (100 embryos per group), including a blank control group, a solvent control group (0.01% (v/v) DMSO), a 2 μg/L BM-DBM (corresponding to 0.74 × 10-8 mol/L) alone exposure group, a 2 mg/L PS-NPs (corresponding to 4.4 × 1011 particles/L) alone exposure group, and a combined 2 μg/L BM-DBM and 2 mg/L PS-NPs exposure group, and exposed in 12-well cell culture plates for 5 d. Bioassays were replicated three times. All solutions were prepared with dechlorinated carbon-filtered water, where the mixture of BM-DBM and PS-NPs was left for 6 h in advance to reach adsorption equilibrium and the exposure solution was replaced once a day. The concentrations of BM-DBM (1770.3 ng/L) and PS-NPs (0.55-8.6 mg/L) were set based on reported environmentally relevant concentrations (Rodriguez et al., 2015; Torres-Ruiz et al., 2021).
During exposure, mortality and hatching rate were recorded daily (n=3). The number of caudal contractions per minute and heart rate was recorded at 24 hours post fertilization (hpf) and 48 hpf (12 individuals, n=3), respectively. At 96 hpf, the malformation rate was recorded, and larvae (3 individuals, n=3) were stained with acridine orange (AO) to assess neuronal apoptosis (n=3). At 120 hpf, larval body lengths (12, n=3) were recorded, and larval movements were analyzed using a zebrafish behavioral analyzer. Finally, 40 larvae were taken and rapidly frozen in liquid nitrogen and placed in a -80°C refrigerator for subsequent determination of neurodevelopment-related gene expression. The remaining larvae were homogenized in phosphate buffer solution (100 mM, pH = 7.2) at a ratio of 1:9 (w:v), centrifuged at 6000 g for 10 min at 4°C, and the supernatant was stored at -80°C for determination of neurotransmitter content and regulatory enzyme activity. All embryos were incubated in an overhead LED light incubator with a light/dark cycle of 14 h/10 h at 28 ± 1°C, pH between 7.0 and 7.5, and dissolved oxygen >6.5 mg/L.
In order to clearly observe apoptotic cells in neural tissue by AO staining, non-transgenic zebrafish embryos were treated with PTU to inhibit melanogenesis. 30 non-transgenic zebrafish embryos with normal fertilization and reaching the blastocyst stage were selected and divided into five groups (6 embryos per group). Then the five groups of embryos were exposed for 96 h to five solutions containing PTU, including a blank solution, a 0.01% (v/v) DMSO solution, a 2 μg/L BM-DBM solution, a 2 mg/L PS-NPs solution, and a mixture of 2 μg/L BM-DBM and 2 mg/L PS-NPs solution. At 96 hpf, 3 larvae were selected for in situ AO staining to visualize apoptotic cells. The rest of the experimental design or conditions were the same as the non-transgenic zebrafish embryo exposure experiment.
The transgenic zebrafish embryos (Tg: HuC-GFP, Tg: Hb9-GFP) selected for this experiment were also healthy embryos with normal fertilization and reaching the blastocyst stage. 50 embryos of each of the two transgenic zebrafish species were selected and divided into five groups (10 embryos in each group). Then the five groups of embryos were transferred to five solutions (blank solution, 0.01% (v/v) DMSO solution, 2 μg/L BM-DBM solution, 2 mg/L PS-NPs solution, and a mixture of 2 μg/L BM-DBM and 2 mg/L PS-NPs) containing PTU until 72 hpf. At 72 hpf, the development of larval neurons and motor neurons was observed by fluorescence microscopy, respectively. The rest of the experimental design or conditions were the same as the non-transgenic zebrafish embryo exposure experiment. All animal experiments were performed in accordance with relevant guidelines and regulations and were approved by the Nanjing Tech University Institutional Animal Care and Use Committee (IACUC).
At 96 hpf, the embryos were stained with AO (5 mg/L) to detect apoptotic cells in zebrafish embryos. 6 embryos in each group were selected, washed 3 times with water, and incubated in AO solution at 5 mg/L for 30 min at 28°C under protection from light. Then, embryos were rinsed with water 3 times, and apoptotic cells were observed and photographed with a fluorescence microscope (Nikon, DS-Qi2, Japan).
9 larvae of 5 dpf were selected from each group for behavioral analysis. All larvae’s locomotor behavior was examined without shock or noise interference. After 10 min of acclimation, zebrafish were subjected to 30 min of alternating light-dark stimulation (5 min light-5 min dark alternation) using a laboratory zebrafish video tracking system (ViewPoint Life Sciences Inc, Lyon, France) to record the locomotor behavior of larvae in a 24-well plate (1 larva/well). Trajectory maps of the larvae were collected every 150 s, and the larvae’s movement distance, average velocity, and acceleration under light and dark conditions were analyzed.
Dopamine (DA), acetylcholine (ACh), gamma-aminobutyric acid (GABA) and serotonin (5-HT) contents and AChE, tyrosine hydroxylase (TH), tryptophan hydroxylase (TPH) and glutamate decarboxylase 1 (GAD1) activities were measured using diagnostic enzyme-linked immunosorbent assay kits according to the manufacturer’s instructions. Protein concentrations were measured using the method described by Bradford (1976). Detailed measurement procedures are described in the SM.
The qRT-PCR data of neurogenin 1 (Neurog1), growth associated protein 43 (Gap43), glial fibrillary acidic protein (Gfap), synapsin IIa (Syn2a), myelin basic protein a (Mbpa), elav like neuron-specific RNA binding protein 3 (Elavl3), alpha 1b-tubulin (α1b-Tubulin), Finkel-Biskis-Jinkins osteosarcoma oncogene (C-fos), brain-derived neurotrophic factor (Bdnf), sonic hedgehog signaling molecule a (Shha), Bcl2 associated x (Baxa), apoptosis regulator a (Bcl2a), and apoptosis-related cysteine peptidase a (Caspase-3a) were all expressed as threshold cycle (Ct) values, and the mRNA expression levels were calculated using the 2−ΔΔCt method (Livak and Schmittgen, 2001). The gene-specific primers of all the genes were designed based on zebrafish sequences available at NCBI (http://www.ncbi.nlm.nih.gov/), and the β-actin gene was selected as the internal standard. Detailed procedures and primer information are provided in the Supplementary Table 1.
SPSS 20.0 (SPSS, RRID: SCR_002865) was used for statistical analysis. All data were presented as mean ± standard deviation, and Levene’s and Shapiro-Wilk tests were used to initially verify the homogeneity and normality of the variance. One-way analysis of variance and Tukey’s test was used to assess differences between control and differentially exposed groups, and Dunnett’s test was used to determine whether differences were statistically significant. p < 0.05 was considered a statistically.
The growth and development of zebrafish embryos after exposure to PS-NPs, BM-DBM, and mixtures of the two are shown in Figure 2. The hatching rate of embryos at 48 hpf and 72 hpf was significantly lower in all experimental groups compared with the control group, while the tail retraction frequency at 24 hpf, the mortality rate at 24 hpf and 48 hpf, malformation rate at 96 hpf and body length at 120 hpf were not significantly different from the control group. The heart rate of 72 hpf larvae in the BM-DBM alone exposure group was significantly decreased compared to the control group. In contrast, the heart rate of 72 hpf larvae in both the PS-NPs alone and combined exposure groups did not change significantly.
Figure 2 Response of growth and development indicators. SC represents the solvent control group. Groups that did not contain the same letter were significantly different (P < 0.05).
In zebrafish, the spontaneous movements of 17-29 hpf larvae originate from the innervation of spinal motor neurons (Vliet et al., 2017). The spontaneous motor abilities of embryos in all exposed groups in this study were not significantly different from those of controls, suggesting that neither BM-DBM nor PS-NPs negatively affects spinal motor neurons in early embryos. Heart rate is an essential indicator of cardiac developmental toxicity. Heart rate disorders may lead to abnormalities in the vascular system and cardiac hemodynamics, resulting in multisystem toxic effects on larvae (Liu et al., 2021a). In this study, BM-DBM significantly reduced the heart rate of 72 hpf larval fish, suggesting that BM-DBM is developmentally cardiotoxic to zebrafish. However, the heart rate of 72 hpf larvae was not affected in both the PS-NPs alone exposure group and the combined PS-NPs and BM-DBM exposure group, i.e., the presence of PS-NPs may have an antagonistic effect with the cardiotoxicity of BM-DBM. Liu et al. (2021a) had found that 100 nm PS-NPs could adsorb BM-DBM and cause a decrease in the concentration of dissolved BM-DBM to 46% of the original, which may further lead to a decrease in BM-DBM bioavailability, and this could be the reason for the antagonistic effect of PS-NPs with BM-DBM in this study.
Hatchability, malformation, mortality, and body length are important toxicological endpoints of growth and developmental toxicity, with hatchability being a key indicator for estimating the developmental status of zebrafish embryos (Younes et al., 2018). The hatching of embryos is usually delayed when neurotransmitter levels are affected (Schoots et al., 1983). Although no significant changes were found in mortality, malformation rate, and body length of zebrafish embryos at different periods in all experimental groups in this experiment, the hatching rate of embryos at 48 hpf and 72 hpf were significantly decreased compared with the control group, indicating that both PS-NPs and BM-DBM can be toxic to embryos for growth and development. It also suggested that PS-NPs and BM-DBM may interfere with the neurotransmitter system and thus be neurotoxic, which needs further exploration. Furthermore, Duan et al. (2020) found that PS particle enrichment occurs on the surface of the chorionic membrane of embryos exposed to PS-NPs, which can lead to internal hypoxia and thus delay embryo hatching, and this may also be one of the reasons for the reduced hatching rate in the PS-NPs alone exposed group and its combined exposure group with BM-DBM in this experiment.
The behavioral responses of 120 hpf larvae under different exposure conditions are shown in Figure 3. The locomotor velocity and total locomotor distance of larvae in the BM-BDM and PS-NPs exposure groups alone or combination were significantly decreased compared to the control group in either light or dark conditions. In comparison, the acceleration of larvae in all experimental groups was not significantly different from the control group.
Figure 3 Behavioral response of 120 hpf zebrafish larvae exposed to BM-BDM and PS-NPs alone or in combination. SC represents the solvent control group. *indicates significant differences between the test group and the solvent control group.
Locomotor behavior following light-dark cycle stimulation has been widely used as a basic and comprehensive indicator for assessing the neurotoxicity of low-level neuro disruptors in fish (Yu et al., 2021). Behavioral responses are 10-100 times more sensitive to understanding the plausible ecotoxicological effects of environmental contaminants compared to mortality (Gyimah et al., 2021). Using light-dark-light transition tests, this study found that both single and combined exposure to BM-BDM and PS-NPs at the environmental level significantly reduced the locomotor ability of larvae under both light and dark conditions. The observations of this study are also consistent with those of Liu et al. (2021b), although the concentrations of both BM-DBM and PS-NPs used by us (2 mg/L PS-NPs, 2 μg/L BM-BDM) and Liu et al. (10 μg/L PS-NPs, 10 μg/L BM-BDM) were different. Such effects may result from a variety of causes, such as defects in neuronal development or abnormalities in biochemical parameters due to alterations in the expression of genes related to the development of the central nervous system (CNS) and changes in neurotransmitters (Fu et al., 2020). Based on this hypothesis, the effects of BM-DBM and PS-NPs on neuronal as well as motor neuron development were assessed using imaged transgenic Tg-HuC-GFP and Tg-Hb9-GFP zebrafish embryos. The expression of genes related to nervous system development or apoptosis, neurotransmitter content, and neurotransmitter-regulated enzyme activities were also examined to comprehensively assess the neurotoxic mechanisms of BM-DBM and PS-NPs. Furthermore, the combined exposure did not further inhibit the locomotor activity of the larvae on the basis of the exposure alone, i.e., the behavioral inhibition between PS-NPs and BM-BDM did not show either synergistic or antagonistic effects, and the interaction mechanism between them needs to be further investigated.
The development of neurons and motor neurons in 72 hpf larvae under different exposure conditions is shown in Figures 4A–C. Compared with the control group, all BM-BDM and PS-NPs alone and combined exposure groups of Tg-Hb9-GFP transgenic larvae showed axonal deletion and loss of axonal continuity, with the combined exposure group having significantly shorter axon length compared to the control group. Green fluorescent protein (GFP) fluorescence was significantly reduced in the brain and spinal cord of Tg-HuC-GFP transgenic larvae in all alone and combined exposure groups compared with the control group.
Figure 4 Development of (A) motor neurons and (B, C) neurons in 72 hpf larvae under BM-BDM and PS-NPs exposure conditions alone or in combination. SC represents the solvent control group. Different letters represent significant differences (P < 0.05).
Compared to immunohistochemical staining, which requires fixed samples, transgenic fish have the advantage of visualizing in vivo to assess neurological development in real-time (Fitzgerald et al., 2021). In Hb9-GFP transgenic zebrafish, the GFP specifically binds in motor neurons regulated by the Hb9 gene, so Hb9-GFP transgenic zebrafish can be used to assess the effects of contaminants on zebrafish motor neurons during early developmental stages (Cheesman et al., 2004). Changes in motor neurons are considered important for many contaminants causing changes in motor behavior in early zebrafish (Brustein et al., 2003). In this study, we found that both 2 μg/L BM-BDM and 2 mg/L PS-NPs significantly inhibited axonal development in 72 hpf Tg-Hb9-GFP transgenic larval fish motor neurons, specifically manifesting axonal deletion and loss of axonal continuity (Figure 4A). The above results are consistent with behavioral responses in 120 hpf larvae, suggesting a potential causal relationship between behavioral abnormalities induced by PS-NPs and BM-BDM and secondary motor neuron growth impairment. In addition, the axon length of 72 hpf Tg-Hb9-GFP transgenic larvae in the combined exposure group was also significantly shortened, suggesting the effects of PS-NPs and BM-BDM on motor neuron axons may have synergistic effects.
In addition to the effects on motor neuron axon growth, the effects of PS-NPs and BM-BDM alone or in combination on CNS development in transgenic zebrafish (HuC-GFP) were also evaluated. In HuC-GFP transgenic zebrafish, GFP is expressed in neurons and integrated into the promoter sequence of the Elavl3 gene, which encodes the neuron-specific RNA-binding protein HuC (Wang et al., 2022). Thus transgenic zebrafish (HuC-GFP) can be used to assess the effects of contaminants on the neurodevelopmental system of zebrafish at early developmental stages (Wang et al., 2022). In this study, we found that both BM-BDM and PS-NPs alone or in combination significantly reduced GFP fluorescence in HuC-GFP transgenic zebrafish brain and spinal cord, implying that neuronal development was affected and resulted in reduced numbers, which is also consistent with defective motor neuron axon development and behavioral abnormalities in the present study. To investigate the reason why BM-BDM and PS-NPs inhibit the development of larval neurons or motor neurons, we further determined the expression of neurodevelopmental or apoptosis-related genes and assessed apoptosis in the CNS of larval fish by AO staining.
The expression of genes related to the development of the nervous system in larvae of different exposure groups is shown in Figure 5. For the exposure group alone, BM-BDM induced a significant decrease in the expression levels of Neurog1, C-fos, Gap43, Syn2a, and a significant increase in the expression levels of Gfap and Shha compared to the control group, while Elavl3, Bdnf, Mbpa, and α1b-Tubulin expression did not change significantly. PS-NPs induced significant down-regulation of Elavl3, Neurog1, C-fos, Gap43, Syn2a, α1b-Tubulin expression and significant up-regulation of Gfap expression, and no significant changes in Bdnf, Mbpa, and Shha expression. For larvae in the combined exposure group, Neurog1, Gfap, and Shha expression levels were not significantly different compared to the control group, while Elavl3, C-fos, Gap43, Syn2a, and α1b-Tubulin expression levels were significantly decreased. Compared with the BM-BDM exposure group alone, no significant changes in Gap43, Syn2a, and C-fos expression levels occurred in the combined exposure group, and Elavl3 and α1b-Tubulin expression levels were significantly decreased.
Figure 5 Expression of genes related to CNS development in larvae exposed to BM-BDM and PS-NPs alone or in combination. SC represents the solvent control group. Groups that did not contain the same letter were significantly different (P < 0.05).
In the previous section, we stated that both BM-BDM, PS-NPs, and mixtures induced abnormal neuronal and motor neuron development. Therefore, this section evaluated the expression of 10 vital neurodevelopment-related genes to explore the molecular mechanisms by which BM-BDM and PS-NPs induce developmental neurotoxicity in larvae. Elavl3 (HuC) is one of the first marker genes expressed in neurons and is considered an early neuronal differentiation marker for developing zebrafish (Kim et al., 1996). In the present study, we found that PS-NP exposure alone and in combination induced a significant decrease in Elavl3 expression, which is consistent with our neuronal imaging data in larval brains, i.e., significant neuronal loss. BM-DBM did not induce significant changes in Elavl3, suggesting that Elavl3 is not a major cause of BM-DBM-induced neuronal damage. Neurog1 is a key regulator of dorsal root ganglion neuronal growth and development (Quiñones et al., 2010). Neurog1 inhibition can disrupt dorsal root ganglion neuronal differentiation and lead to glial cell expression (Park et al., 2021). Gfap is predominantly expressed in CNS astrocytes and plays an essential role in astrocyte structure and function, including supporting and isolating neurons, forming and repairing myelin sheaths, and forming the blood-brain barrier (Song et al., 2022). Upregulation of Gfap expression indicates neural tissue damage and induces the body to repair neurons and turn on the defense of the blood-brain barrier (Park et al., 2012). C-fos is a marker gene for neuronal activity (Joo et al., 2016), and its downregulation in expression may be an indication of contaminant-induced neural damage. In this study, we found that both PS-NPs and BM-DBM induced significant down-regulation of Neurog1 and C-fos and significant up-regulation of Gfap in larvae, suggesting that both can disrupt dorsal root ganglion neuronal development and induce neural tissue damage during embryogenesis.
In addition, Neurog1 and Gfap expressions in the combined exposure group were restored to control levels, i.e., a possible antagonistic effect between PS-NPs and the dorsal root ganglion neuronal inhibitory effect of BM-DBM. This may be due to the decreased dissolved BM-DBM and BM-DBM bioavailability due to the adsorption of BM-DBM by PS-NPs. There is also a reason that the adsorption of BM-DBM by PS-NPs occupies the adsorption sites of PS-NPs and reduces the adhesion of PS-NPs on the surface of embryonic chorionic villi, thus decreasing the bioavailability of PS-NPs (Liu et al., 2021a). Bdnf is a neurotrophic factor that promotes neuronal survival, growth and differentiation, and synaptic plasticity, and its reduced expression is a signal of impaired neuronal development (Song et al., 2022). None of the experimental groups in this study showed abnormal Bdnf expression, which may not be a target gene for PS-NPs and BM-DBM-induced neurotoxicity production.
Gap43 is a crucial gene for synaptogenesis, axonogenesis, and regeneration after injury (Wang et al., 2015a). Motor neuron formation, regeneration, and plasticity may be disrupted once Gap43 expression is downregulated (Gu et al., 2019). Syn2a is a marker of synapse formation, and Mbp is involved in the myelination of axons (Wang et al., 2015b; Song et al., 2022). Downregulation of Syn2a expression may negatively affect synaptic origin and differentiation, and reduction of Mbpa expression may affect axonal myelination (Wang et al., 2015b; Chu et al., 2021). In the present study, we found that both PS-NPs and BM-DBM exposure alone or in combination induced a significant decrease in Gap43 and Syn2a expression, suggesting that synapse or axon formation and regeneration may be negatively affected, which is consistent with the imaging data of motor neurons in Tg-Hb9-GFP transgenic larvae. A1b-Tubulin affects axonal and dendritic development in the nervous system by encoding intermediate filament proteins that form part of the microtubule cytoskeleton (Wang et al., 2015b). Its downregulation is thought to have essential effects on cytoskeletal alternations (Wang et al., 2015b). Shha is a morphogen in many systems and is thought to affect axonal guidance cues in axons (Ingham and McMahon, 2001). In this study, we observed a down-regulation of α1b-Tubulin expression in the PS-NPs alone group, and a significant up-regulation of Shha expression in the BM-DBM alone exposed group, implicating differences in the target genes for axonal abnormalities induced by PS-NPs and BM-DBM. In addition, Shha expression was significantly down-regulated to control levels in the combined exposure group compared to BM-DBM alone, i.e., PS-NPs may have inhibited their motor neuron toxicity by adsorption of BM-DBM.
In summary, both BM-DBM and PS-NPs can affect neuronal differentiation, synaptogenesis, axonal growth, and cytoskeleton formation by inducing Neurog1, Gfap, C-fos, Gap43, and Syn2a abnormalities, which can have damaging effects on the structure and function of the CNS and lead to developmental neurotoxicity. Shha is a specific gene for BM-DBM-induced developmental neurotoxicity, while PS-NPs are specific for Elavl3 and α1b-Tubulin. Furthermore, combined exposure of PS-NPs and BM-DBM may induce Neurog1, Gfap, and Shha expression to return to control levels by reducing PS-NPs or BM-DBM bioavailability, thereby attenuating both-induced developmental neurotoxicity.
Figure 6A shows the imaging results of AO staining in the brain of 72 hpf larvae after BM-BDM and PS-NPs exposure alone or in combination. Results showed that many green fluorescent particles appeared in the brain of larvae of all BM-BDM and PS-NPs alone or combined exposure groups, i.e., both BM-BDM and PS-NPs induced different degrees of apoptosis in the CNS of larvae. The results of apoptosis-related gene expression showed that both BM-BDM and PS-NPs alone or combined exposure induced a significant increase in the expression levels of anti-apoptotic gene Bcl2a and pro-apoptotic genes Baxa and Caspase-3a compared with the control group, while only BM-BDM alone and combined exposure groups induced a significant increase in Baxa/Bcl2a, a key indicator of apoptosis (Figure 6B).
Figure 6 Apoptosis (A) and expression of apoptosis-related genes (B) in the CNS of 72 hpf larvae under BM-BDM and PS-NPs exposure alone or in combination. Where green dots represent apoptosis. SC represents the solvent control group. Groups that did not contain the same letter were significantly different (P < 0.05).
Organisms exposed to internal or/and external environmental noxious stimuli can activate apoptotic signaling cascade pathways and induce apoptosis (Cui et al., 2020). Apoptosis is an important regulator of growth and development, and apoptosis in neuronal cells ultimately leads to developmental brain defects and developmental neurotoxicity (Salminen and Paul, 2014). AO staining is commonly used to detect apoptosis in larval zebrafish brains (Gu et al., 2019). According to the results of AO staining, both BM-BDM and PS-NPs alone or in combination induced apoptosis and were located mainly in the brain. These results are consistent with the diminished GFP fluorescence and behavioral response in the brain of Tg-HuC-GFP transgenic larvae in this experiment, implying that apoptosis may be an important cause of developmental neurotoxicity induced by BM-BDM and PS-NPs alone or in combination with exposure. In addition, several studies have previously detected the presence of contaminants, such as persistent organic pollutants and nanoparticles, in the brains of zebrafish larvae exposed to exogenous contaminants, suggesting that all of the above substances can enter the CNS through the blood-brain barrier. Contaminants entering the brain can induce neurotoxicity at the cellular and molecular levels through oxidative stress or inflammatory responses (Lu et al., 2016; Zhou et al., 2022b), which could be the reason for the apoptosis induced by BM-BDM and 100 nm PS-NPs in this experiment.
To determine the molecular mechanisms underlying the increase in apoptosis, the expression of apoptosis-related genes (Bcl2a, Baxa, and Caspase-3a) was evaluated. The Bcl2 and Bax genes of the Bcl2 family are the most critical regulatory genes known to be associated with apoptosis (Del Poeta et al., 2003). Bcl2 is an anti-apoptotic gene, while Bax opposes apoptosis-inhibiting proteins such as Bcl2 to prevent the anti-apoptotic effect of Bcl-2 (Del Poeta et al., 2003). Therefore, Bcl2/Bax is usually used as a key determinant of cell survival [46]. Caspase-3 is the most critical apoptotic executor downstream of the Caspase-3 cascade reaction (Jin et al., 2019). Bcl2 and Bax are not only involved in the regulation of Caspase-3 expression as an upstream regulatory mechanism of Caspase-3, but also as a direct substrate of Caspase 3 downstream (Scorrano and Korsmeyer, 2003). In the present study, Bcl2a, Baxa, and Caspase-3a expression were significantly elevated in all experimental groups of larvae, which may be the underlying cause of apoptosis induction in neuronal cells by PS-NPs and BM-BDM, which further validates the results of AO staining. In addition, Bcl2a/Baxa was significantly elevated in the combined exposure group compared with BM-BDM alone, suggesting that PS-NPs and BM-BDM may have synergistic effects in inducing neuronal apoptosis.
In addition to the growth and development of the nervous system, signaling between the synapses of various neurons is an important marker for assessing the functional impairment of the nervous system (Guo et al., 2018). Neurotransmitters are endogenous chemicals that transmit signals between neuronal synapses (Wang X. et al., 2015), and changes in their levels are essential for assessing neurochemical and toxicological phenotypes as well as behavioral development (Guo et al., 2021). In this section, we analyze four neurotransmitter contents and their key regulatory enzyme activities, including the cholinergic system (ACh, AChE), the dopaminergic system (DA, TH), the GABAergic system (GABA, GAD1), and the serotonergic system (5-HT, TPH). From Figure 7, it was found that BM-DBM induced a significant decrease in GAD1, TPH activity, and GABA and 5-HT contents, as well as a significant increase in DA activity and ACh and TH contents compared to the control group. None of the above indices in the PS-NPs and their combined exposure group with BM-DBM significantly differed from the control group.
Figure 7 Response of different neurotransmitter systems of larvae under BM-BDM and PS-NPs exposure conditions alone or in combination. SC represents the solvent control group. Groups that did not contain the same letter were significantly different (P < 0.05).
ACh was the first neurotransmitter identified whose accumulation causes disruption of cholinergic synapses and neuromuscular connections and leads to neurotoxicity (Tao et al., 2022).AChE regulates ACh levels by hydrolyzing ACh to choline and acetate to eliminate the excitatory effects of ACh accumulation on the postsynaptic membrane (Cheng et al., 2020). The present study found that BM-DBM induced a significant elevation in ACh content, whereas no elevation in AChE activity occurred to eliminate ACh. Several studies have found that the inhibition of AChE activity and its reduced ability to hydrolyze ACh are thought to be directly responsible for defective swimming behavior (Lin et al., 2013), so the elevation of ACh in the present study may be one of the key reasons for the reduced behavioral activity induced by BM-DBM. DA is synthesized from tyrosine catalyzed by the rate-limiting enzyme TH and mainly regulates locomotion, cognition, motivation, and development (Wasel and Freeman, 2020; Wang et al., 2022). It has also been shown that DA is involved in regulating the metabolism of the skeleton, a vital component of the locomotor system in zebrafish, including osteoblasts and osteoclasts (Lee et al., 2015). Thus, DA plays a key role in swimming performance in zebrafish (Lee et al., 2015). In our results, DA levels in larvae were significantly increased during early BM-DBM exposure, which is consistent with the elevated activity of TH, the rate-limiting enzyme for DA synthesis in larvae in this experiment. Guo et al. (2021) have also found that perfluorohexanoic acid (PFHxA) induced elevated DA levels as a protective response against neurotoxicant-induced neurological or behavioral toxicity. Therefore, the elevated DA levels in this experiment may be an adaptive response to BM-DBM-induced behavioral abnormalities and neurotoxicity production.
GABA is the primary inhibitory neurotransmitter of the vertebrate CNS and is synthesized from glutamate catalyzed by glutamic acid decarboxylase (GAD) (Guo et al., 2021). Therefore, GAD is the main determinant of GABA levels (Guo et al., 2021). The GABAergic system plays an important role in maintaining the balance between excitation and inhibition of nerves, and its abnormalities can lead to impaired neurocognitive function and behavioral deficits such as autism (Lee et al., 2017) In the present study, BM-DBM induced a significant decrease in GABA content, consistent with a decrease in GAD1 activity and locomotor activity, suggesting that BM-DBM can also induce neurotoxicity through the GABAergic system. The serotonergic system is involved in many behavioral disorders, including anxiety, fear, and stress. The main neurotransmitter of this system, 5-HT, is biosynthesized by tryptophan catalyzed by the rate-limiting enzyme TPH to promote a more constant motor output and plays an important role in stabilizing swimming patterns (Brustein et al., 2003; Fan et al., 2022). Several studies have reported that decreased 5-HT levels can reduce locomotor activity in zebrafish (Airhart et al., 2007; Airhart et al., 2012). Maximino et al. (2013) found a positive correlation between 5-HT levels and anxiety levels in larvae by a new tank test in zebrafish. Therefore, the decrease in 5-HT levels and TPH activity due to BM-DBM observed in our study may be partly responsible for the decrease in the swimming activity of larvae. Similar phenomena have been observed with other environmental pollutants. For example, polybrominated diphenyl ethers (DE-71) (Wang et al., 2016) and microcystin-LR (Wu et al., 2017) reduced 5-HT levels and caused behavioral abnormalities in zebrafish.
In summary, BM-DBM can affect the signaling between neuronal synapses by interfering with multiple neurotransmitter systems, thereby inducing functional impairment and behavioral abnormalities in the nervous system. Furthermore, we previously mentioned in 3.2 that abnormal neurotransmitter levels usually lead to a delay in embryo hatching (Schoots et al., 1983). Therefore, BM-DBM-induced abnormal neurotransmitter levels may be an important reason for the reduced hatching rate of embryos in the BM-DBM alone exposed group.
For the PS-NPs alone exposure group, neither the neurotransmitter content nor its regulatory enzyme activity occurred abnormally, indicating that the neurotransmitter system is not a target of the neurotoxicity of PS-NPs. In addition, GABA, 5-HT content, and GAD1 and TPH activities were significantly higher in the combined exposure group compared to BM-DBM alone, while ACh, DA content, and TH activity were significantly lower and reached the control level compared to BM-DBM alone, i.e., the presence of PS-NPs may have weakened the disruptive effects of BM-DBM on all neurotransmitter systems. Combined with the results of adsorption experiments performed by Liu et al. (2021a), we suggested that the decrease in dissolved BM-DBM due to the adsorption of BM-DBM by PS-NPs would further reduce the uptake of free BM-DBM by embryos or larvae, thus leading to a decrease in BM-DBM bioavailability. The above may be the main reason why PS-NPs inhibit the neurotransmitter interference toxicity of BM-DBM and the normalization of the neurotransmitter system in the combined exposure group. Sleight et al. (2017) had found that the presence of MPs reduced the bioavailability of 17α-estradiol and phenanthrene by 48% and 33%, respectively. Zhang et al. (2018) also found that PS-MPs attenuated the inhibitory effect of ROX on AChE activity in tilapia (Oreochromis niloticus) and attributed this to the adsorption of PS-MPs on ROX.
In summary, both BM-DBM and PS-NPs can cause growth and developmental toxicity and neurotoxicity due to reduced embryo hatching rate and abnormal larval behavior. Imaging results of transgenic zebrafish showed that both BM-DBM and PS-NPs induced neuronal loss and motor neuron axon deletion in larvae. Further testing revealed that both BM-DBM and PS-NPs induced developmental neurotoxicity production by inducing abnormal expression of Neurog1, Gfap, C-fos, Gap43, and Syn2a. They also can induce apoptosis and exacerbate neuronal damage by inducing abnormalities in apoptotic genes Bcl2a, Baxa, and Caspase-3a. In addition, BM-DBM specifically interferes with multiple neurotransmitter systems and affects signaling between neuronal synapses, thereby contributing to functional impairment of the nervous system and behavioral abnormalities in larvae. Finally, PS-NPs may exert synergistic and antagonistic effects on BM-DBM-induced developmental neurotoxicity and apoptotic toxicity, respectively. In this paper, the neurotoxic mechanisms of BM-DBM and PS-NPs at environmental concentrations and the interactive neurotoxic effects of coexisting PS-NPs and BM-DBM were comprehensively explored at the tissue and molecular levels, which laid the foundation for the interactive ecological risk assessment of BM-DBM and PS-NPs.
The datasets presented in this study can be found in online repositories. The names of the repository/repositories and accession number(s) can be found in the article/Supplementary Material.
The animal study was reviewed and approved by Animal Care and Use committee of Nanjing Tech University.
RZ: Conceptualization, writing-original draft, supervision. HY: Methodology, formal analysis, writing-original draft. DZ: Data curation, formal analysis, investigation. SY: Validation, investigation. ZS: Investigation. QJ: Investigation. ZD: Conceptualization, funding acquisition, project administration. All authors contributed to the article and approved the submitted version.
This work was supported by the National Natural Science Foundation of China (42207317), Jiangsu Funding Program for Excellent Postdoctoral Talent (2022ZB387), Natural Science Research of the Jiangsu Higher Education Institutions of China (22KJB610015) and National Key Research and Development Program of China (2018YFC1800603).
The authors declare that the research was conducted in the absence of any commercial or financial relationships that could be construed as a potential conflict of interest.
All claims expressed in this article are solely those of the authors and do not necessarily represent those of their affiliated organizations, or those of the publisher, the editors and the reviewers. Any product that may be evaluated in this article, or claim that may be made by its manufacturer, is not guaranteed or endorsed by the publisher.
The Supplementary Material for this article can be found online at: https://www.frontiersin.org/articles/10.3389/fmars.2022.1099984/full#supplementary-material
Airhart M. J., Lee D. H., Wilson T. D., Miller B. E., Miller M. N., Skalko R. G. (2007). Movement disorders and neurochemical changes in zebrafish larvae after bath exposure to fluoxetine (PROZAC). Neurotoxicol. Teratol. 29 (6), 652–664. doi: 10.1016/j.ntt.2007.07.005
Airhart M. J., Lee D. H., Wilson T. D., Miller B. E., Miller M. N., Skalko R. G., et al. (2012). Adverse effects of serotonin depletion in developing zebrafish. Neurotoxicol. Teratol. 34 (1), 152–160. doi: 10.1016/j.ntt.2011.08.008
Bradford M. M. (1976). A rapid and sensitive method for the quantitation of microgram quantities of protein utilizing the principle of protein-dye binding. Anal. Biochem. 72 (1), 248–254. doi: 10.1016/0003-2697(76)90527-3
Brustein E., Saint-Amant L., Buss R. R., Chong M., McDearmid J. R., Drapeau P. (2003). Steps during the development of the zebrafish locomotor network. J. Physiol. Paris 97 (1), 77–86. doi: 10.1016/j.jphysparis.2003.10.009
Cheesman S. E., Layden M. J., Von Ohlen T., Doe C. Q., Eisen J. S. (2004). Zebrafish and fly Nkx6 proteins have similar CNS expression patterns and regulate motoneuron formation. Development 131 (21), 5221–5232. doi: 10.1242/dev.01397
Cheng B., Jiang F., Su M., Zhou L., Zhang H., Cao Z., et al. (2020). Effects of lincomycin hydrochloride on the neurotoxicity of zebrafish. Ecotox. Environ. Safe. 201, 110725. doi: 10.1016/j.ecoenv.2020.110725
Chu S., Kwon B. R., Lee Y. M., Zoh K. D., Choi K. (2021). Effects of 2-ethylhexyl-4-methoxycinnamate (EHMC) on thyroid hormones and genes associated with thyroid, neurotoxic, and nephrotoxic responses in adult and larval zebrafish (Danio rerio). Chemosphere 263, 128176. doi: 10.1016/j.chemosphere.2020.128176
Cui J., Ding H., Yao Y., Liu W. (2020). Inhibition mir-92a alleviates oxidative stress and apoptosis of alveolar epithelial cells induced by lipopolysaccharide exposure through TLR2/AP-1 pathway. BioMed. Res. Int. 2020, 9673284. doi: 10.1155/2020/9673284
Del Poeta G., Venditti A., Del Principe M. I., Maurillo L., Buccisano F., Tamburini A., et al. (2003). Amount of spontaneous apoptosis detected by Bax/Bcl-2 ratio predicts outcome in acute myeloid leukemia (AML). Blood 101 (6), 2125–2131. doi: 10.1182/blood-2002-06-1714
Duan Z., Duan X., Zhao S., Wang X., Wang L. (2020). Barrier function of zebrafish embryonic chorions against microplastics and nanoplastics and its impact on embryo development. J. Hazard. Mater. 395, 122621. doi: 10.1016/j.jhazmat.2020.122621
EPA. United States Environmental Protection Agency (2015) Health effects of UV radiation. Available at: https://www.epa.gov/sunsafety/health-effects-uv-radiation.
Fan B. Y., Dai L. L., Liu C. S., Sun Q., Yu L. Q. (2022). Nano-TiO2 aggravates bioaccumulation and developmental neurotoxicity of triphenyl phosphate in zebrafish larvae. Chemosphere 287, 132161. doi: 10.1016/j.chemosphere.2021.132161
Fitzgerald J. A., Konemann S., Krumpelmann L., Zupanic A., Vom Berg C. (2021). Approaches to test the neurotoxicity of environmental contaminants in the zebrafish model: from behavior to molecular mechanisms. Environ. Toxicol. Chem. 40 (4), 989–1006. doi: 10.1002/etc.4951
Fu J., Guo Y., Yang L., Han J., Zhou B. (2020). Nano-TiO2 enhanced bioaccumulation and developmental neurotoxicity of bisphenol a in zebrafish larvae. Environ. Res. 187, 109682. doi: 10.1016/j.envres.2020.109682
Guo X., Zhang S., Liu X., Lu S., Wu Q., Xie P. (2021). Evaluation of the acute toxicity and neurodevelopmental inhibition of perfluorohexanoic acid (PFHxA) in zebrafish embryos. Ecotox. Environ. Safe. 225, 112733. doi: 10.1016/j.ecoenv.2021.112733
Guo X., Zhang S., Lu S., Zheng B., Xie P., Chen J., et al. (2018). Perfluorododecanoic acid exposure induced developmental neurotoxicity in zebrafish embryos. Environ. pollut. 241, 1018–1026. doi: 10.1016/j.envpol.2018.06.013
Gu J., Zhang J., Chen Y., Wang H., Guo M., Wang L., et al. (2019). Neurobehavioral effects of bisphenol s exposure in early life stages of zebrafish larvae (Danio rerio). Chemosphere 217, 629–635. doi: 10.1016/j.chemosphere.2018.10.218
Gyimah E., Xu H., Dong X., Qiu X. C., Zhang Z., Bu Y. Q., et al. (2021). Developmental neurotoxicity of low concentrations of bisphenol a and s exposure in zebrafish. Chemosphere 262, 128045. doi: 10.1016/j.chemosphere.2020.128045
Huang Y., Law C. F., Lam T. K., Leung S. Y. (2021). Risks of organic UV filters: a review of environmental and human health concern studies. Sci. Total Environ. 755, 142486. doi: 10.1016/j.scitotenv.2020.142486
Ingham P. W., McMahon A. P. (2001). Hedgehog signaling in animal development: paradigms and principles. Gene. Dev. 15 (23), 3059–3087. doi: 10.1101/gad.938601
Jain S., Mishra D., Khare P. (2021). Microplastics as an emerging contaminant in environment: occurrence, distribution, and management strategy. Manage. Contaminants Emerg. Concern (CEC) Environ., 281–299. doi: 10.1016/B978-0-12-822263-8.00010-5
Jin M., Ji X., Zhang B., Sheng W., Wang R., Liu K. (2019). Synergistic effects of Pb and repeated heat pulse on developmental neurotoxicity in zebrafish. Ecotox. Environ. Safe. 172, 460–470. doi: 10.1016/j.ecoenv.2019.01.104
Joo J. Y., Schaukowitch K., Farbiak L., Kilaru G., Kim T. K. (2016). Stimulus-specific combinatorial functionality of neuronal c-fos enhancers. Nat. Neurosci. 19 (4), 642–642. doi: 10.1038/nn.4170
Kalikova G., Ali B., Skalar T., Bundschuh M., Gotvajn A. G. (2017). Wastewater treatment plant effluents as source of cosmetic polyethylene microbeads to freshwater. Chemosphere 188, 25–31. doi: 10.1016/j.chemosphere.2017.08.131
Kim C. H., Ueshima E., Muraoka O., Tanaka H., Yeo S. Y., Huh T. L., et al. (1996). Zebrafish elav/HuC homologue as a very early neuronal marker. Neurosci. Lett. 216 (2), 109–112. doi: 10.1016/0304-3940(96)13021-4
Lee E., Lee J., Kim E. (2017). Excitation/inhibition imbalance in animal models of autism spectrum disorders. Biol. Psychiat. 81 (10), 838–847. doi: 10.1016/j.biopsych.2016.05.011
Lee D. J., Tseng H. C., Wong S. W., Wang Z., Deng M., Ko C. C. (2015). Dopaminergic effects on in vitro osteogenesis. Bone Res. 3, 15020. doi: 10.1038/boneres.2015.20
Lei K., Qiao F., Liu Q., Wei Z., Qi H., Cui S., et al. (2017). Microplastics releasing from personal care and cosmetic products in China. Mar. pollut. Bull. 123 (1), 122–126. doi: 10.1016/j.marpolbul.2017.09.016
Li J., Liu H., Chen J. P. (2018). Microplastics in freshwater systems: A review on occurrence, environmental effects, and methods for microplastics detection. Water Res. 137, 362–374. doi: 10.1016/j.watres.2017.12.056
Lin Z., Dodd C. A., Filipov N. M. (2013). Short-term atrazine exposure causes behavioral deficits and disrupts monoaminergic systems in male C57BL/6 mice. Neurotoxicol. Teratol. 39, 26–35. doi: 10.1016/j.ntt.2013.06.002
Liu Y., Wang Y., Li N., Jiang S. (2021a). Avobenzone and nanoplastics affect the development of zebrafish nervous system and retinal system and inhibit their locomotor behavior. Sci. Total Environ. 806, 150681. doi: 10.1016/j.scitotenv.2021.150681
Liu Y., Wang Y., Ling X., Yan Z., Lu G. (2021b). Effects of nanoplastics and butyl methoxydibenzoylmethane on early zebrafish embryos identified by single-cell RNA sequencing. Environ. Sci. Technol. 55 (3), 1885–1896. doi: 10.1021/acs.est.0c06479
Livak K. J., Schmittgen T. D. (2001). Analysis of relative gene expression data using real-time quantitative PCR and the 2–ΔΔCT method. Methods 25 (4), 402–408. doi: 10.1006/meth.2001.1262
Lu Y., Zhang Y., Deng Y., Jiang W., Zhao Y. (2016). Uptake and accumulation of polystyrene microplastics in zebrafish (Danio rerio) and toxic effects in liver. Environ. Sci. Technol. 50 (7), 4054–4060. doi: 10.1021/acs.est.6b00183
Ma B., Lu G., Liu J., Yan Z., Yang H., Pan T. (2017). Bioconcentration and multi-biomarkers of organic UV filters (BM-DBM and OD-PABA) in crucian carp. Ecotox. Environ. Safe. 141, 178–187. doi: 10.1016/j.ecoenv.2017.03.034
Maximino C., Puty B., Benzecry R., Araujo J., Lima M. G., Batista E. D. O., et al. (2013). Role of serotonin in zebrafish (Danio rerio) anxiety: Relationship with serotonin levels and effect of buspirone, WAY 100635, SB 224289, fluoxetine and para-chlorophenylalanine (pCPA) in two behavioral models. Neuropharmacology 71, 83–97. doi: 10.1016/j.neuropharm.2013.03.006
Park D., Joo S. S., Lee H. J., Choi K. C., Kim S. U., Kim Y. B. (2012). Microtubule-associated protein 2, an early blood marker of ischemic brain injury. J. Neurosci. Res. 90 (2), 461–467. doi: 10.1002/jnr.22769
Park H., You H. H., Song G. (2021). Multiple toxicity of propineb in developing zebrafish embryos: Neurotoxicity, vascular toxicity, and notochord defects in normal vertebrate development. Comp. Biochem. Phys. C 243, 108993. doi: 10.1016/j.cbpc.2021.108993
Quiñones H. I., Savage T. K., Battiste J., Johnson J. E. (2010). Neurogenin 1 (Neurog1) expression in the ventral neural tube is mediated by a distinct enhancer and preferentially marks ventral interneuron lineages. Dev. Biol. 340 (2), 283–292. doi: 10.1016/j.ydbio.2010.02.012
Rodriguez A. S., Sanz M. R., Rodriguez J. R. B. (2015). Occurrence of eight UV filters in beaches of gran canaria (Canary islands). an approach to environmental risk assessment. Chemosphere 131, 85–90. doi: 10.1016/j.chemosphere.2015.02.054
Salminen L. E., Paul R. H. (2014). Oxidative stress and genetic markers of suboptimal antioxidant defense in the aging brain: a theoretical review. Rev. Neurosci. 25 (6), 805–819. doi: 10.1515/revneuro-2014-0046
Santos L., Rodríguez-Mozaz S., Barceló D. (2021). Microplastics as vectors of pharmaceuticals in aquatic organisms-an overview of their environmental implications. Case Stud. Chem. Environ. Eng. 3, 100079. doi: 10.1016/j.cscee.2021.100079
Schoots A. M., Meijer R. C., Denucé J. M. (1983). Dopaminergic regulation of hatching in fish embryos. Dev. Biol. 100 (1), 59–63. doi: 10.1016/0012-1606(83)90200-2
Scorrano L., Korsmeyer S. J. (2003). Mechanisms of cytochrome c release by proapoptotic BCL-2 family members. Biochem. Bioph. Res. Co. 304 (3), 437–444. doi: 10.1016/S0006-291X(03)00615-6
Sleight V. A., Bakir A., Thompson R. C., Henry T. B. (2017). Assessment of microplastic-sorbed contaminant bioavailability through analysis of biomarker gene expression in larval zebrafish. Mar. pollut. Bull. 116 (1), 291–297. doi: 10.1016/j.marpolbul.2016.12.055
Song Y., Liu S., Jiang X., Ren Q., Deng H., Paudel Y. N., et al. (2022). Benzoresorcinol induces developmental neurotoxicity and injures exploratory, learning and memorizing abilities in zebrafish. Sci. Total Environ. 834, 155268. doi: 10.1016/j.scitotenv.2022.155268
Sun S., Shi W., Tang Y., Han Y., Liu G. (2021). The toxic impacts of microplastics (MPs) and polycyclic aromatic hydrocarbons (PAHs) on haematic parameters in a marine bivalve species and their potential mechanisms of action. Sci. Total Environ. 783, 147003. doi: 10.1016/j.scitotenv.2021.147003
Tao Y., Li Z., Yang Y., Jiao Y., Qu J., Wang Y., et al. (2022). Effects of common environmental endocrine-disrupting chemicals on zebrafish behavior. Water Res. 208, 117826. doi: 10.1016/j.watres.2021.117826
Torres-Ruiz M., Vieja A. D. L., Gonzalez M. D. A., Lopez M. E., Portilla A. I. C. (2021). Toxicity of nanoplastics for zebrafish embryos, what we know and where to go next. Sci. Total Environ. 797, 149125. doi: 10.1016/j.scitotenv.2021.149125
Tsui M. M., Leung H., Kwan B. K., Ng K. Y., Yamashita N., Taniyasu S., et al. (2015). Occurrence, distribution and ecological risk assessment of multiple classes of UV filters in marine sediments in Hong Kong and Japan. J. Hazard. Mater. 292, 180–187. doi: 10.1016/j.jhazmat.2015.03.025
Vliet S. M., Ho T. C., Volz D. C. (2017). Behavioral screening of the LOPAC(1280) library in zebrafish embryos. Toxicol. Appl. Pharm. 329, 241–248. doi: 10.1016/j.taap.2017.06.011
Wang Q., Lai N. L. S., Wang X., Guo Y., Lam P. K. S., Lam J. C. W., et al. (2015a). Bioconcentration and transfer of the organophorous flame retardant 1, 3-dichloro-2-propyl phosphate causes thyroid endocrine disruption and developmental neurotoxicity in zebrafish larvae. Environ. Sci. Technol. 49 (8), 5123–5132. doi: 10.1021/acs.est.5b00558
Wang Q., Lam C. W., Man Y. C., Lai L. S., Kwok K. Y., Guo Y. Y., et al. (2015b). Bioconcentration, metabolism and neurotoxicity of the organophorous flame retardant 1,3-dichloro 2-propyl phosphate (TDCPP) to zebrafish. Aquat. Toxicol. 158, 108–115. doi: 10.1016/j.aquatox.2014.11.001
Wang X., Marthaler D., Rovira A., Rossow S., Murtaugh M. P. (2015). Emergence of a virulent porcine reproductive and respiratory syndrome virus in vaccinated herds in the united states. Virus Res. 210, 34–41. doi: 10.1016/j.virusres.2015.07.004
Wang X. F., Yang L. H., Wang Q. W., Guo Y. Y., Li N., Ma M., et al. (2016). The neurotoxicity of DE-71: effects on neural development and impairment of serotonergic signaling in zebrafish larvae. J. Appl. Toxicol. 36 (12), 1605–1613. doi: 10.1002/jat.3322
Wang Z., Zhao H. Y., Xu Y. Y., Zhao J. N., Song Z., Bi Y., et al. (2022). Early-life lead exposure induces long-term toxicity in the central nervous system: From zebrafish larvae to juveniles and adults. Sci. Total Environ. 804, 150185. doi: 10.1016/j.scitotenv.2021.150185
Wasel O., Freeman J. L. (2020). Chemical and genetic zebrafish models to define mechanisms of and treatments for dopaminergic neurodegeneration. Int. J. Mol. Sci. 21 (17), 5981. doi: 10.3390/ijms21175981
Wu Q., Yan W., Cheng H., Liu C., Hung T. C., Guo X., et al. (2017). Parental transfer of microcystin-LR induced transgenerational effects of developmental neurotoxicity in zebrafish offspring. Environ. pollut. 231, 471–478. doi: 10.1016/j.envpol.2017.08.038
Xie Z., Zhou R., Ding Z., Zhou D., Jin Q. (2022). Melanin interference toxicity or transgenerational toxicity of organic UV filter ethylhexyl salicylate on zebrafish. Sci. Total Environ. 845, 157365. doi: 10.1016/j.scitotenv.2022.157365
Younes N., Salem R., Al-Asmakh M., Altamash T., Pintus G., Khraisheh M., et al. (2018). Toxicity evaluation of selected ionic liquid compounds on embryonic development of zebrafish. Ecotox. Environ. Safe. 161, 17–24. doi: 10.1016/j.ecoenv.2018.05.064
Yu Y. J., Hou Y. B., Dang Y., Zhu X. H., Li Z. C., Chen H. B., et al. (2021). Exposure of adult zebrafish (Danio rerio) to tetrabromobisphenol a causes neurotoxicity in larval offspring, an adverse transgenerational effect. J. Hazard. Mater. 414, 125408. doi: 10.1016/j.jhazmat.2021.125408
Zhang S. S., Ding J. N., Razanajatovo R. M., Jiang H., Zou H., Zhu W. B. (2018). Interactive effects of polystyrene microplastics and roxithromycin on bioaccumulation and biochemical status in the freshwater fish red tilapia (Oreochromis niloticus). Sci. Total Environ. 648, 1431–1439. doi: 10.1016/j.scitotenv.2018.08.266
Zhou R., Lu G., Yan Z., Jiang R., Sun Y., Zhang P. (2021). Interactive transgenerational effects of polystyrene nanoplastics and ethylhexyl salicylate on zebrafish. Environ. Sci. Nano 8 (1), 146–159. doi: 10.1039/d0en00952k
Zhou R., Lu G., Yan Z., Jiang R., Sun Y., Zhang P. (2022a). Epigenetic mechanisms of DNA methylation in the transgenerational effect of ethylhexyl salicylate on zebrafish. Chemosphere 295, 133926. doi: 10.1016/j.chemosphere.2022.133926
Keywords: organic ultraviolet filters, nanoplastics, neurotoxicity, interaction toxicity, transgenic zebrafish
Citation: Zhou R, Yang H, Zhou D, Yang S, Shi Z, Jin Q and Ding Z (2022) Interactive neurotoxicity of environmentally relevant concentrations of polystyrene nanoplastics and butyl methoxydibenzoyl methane on early zebrafish embryos. Front. Mar. Sci. 9:1099984. doi: 10.3389/fmars.2022.1099984
Received: 16 November 2022; Accepted: 30 November 2022;
Published: 09 December 2022.
Edited by:
Jiannan Ding, Jiangnan University, ChinaCopyright © 2022 Zhou, Yang, Zhou, Yang, Shi, Jin and Ding. This is an open-access article distributed under the terms of the Creative Commons Attribution License (CC BY). The use, distribution or reproduction in other forums is permitted, provided the original author(s) and the copyright owner(s) are credited and that the original publication in this journal is cited, in accordance with accepted academic practice. No use, distribution or reproduction is permitted which does not comply with these terms.
*Correspondence: Zhuhong Ding, dzhuhong@njtech.edu.cn
Disclaimer: All claims expressed in this article are solely those of the authors and do not necessarily represent those of their affiliated organizations, or those of the publisher, the editors and the reviewers. Any product that may be evaluated in this article or claim that may be made by its manufacturer is not guaranteed or endorsed by the publisher.
Research integrity at Frontiers
Learn more about the work of our research integrity team to safeguard the quality of each article we publish.