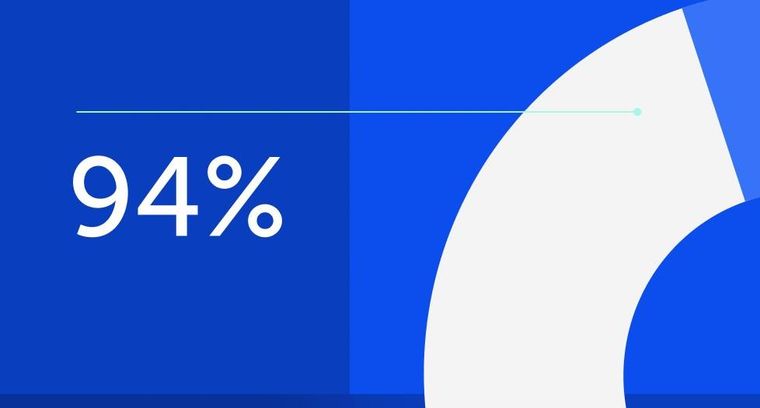
94% of researchers rate our articles as excellent or good
Learn more about the work of our research integrity team to safeguard the quality of each article we publish.
Find out more
ORIGINAL RESEARCH article
Front. Mar. Sci., 28 December 2022
Sec. Marine Biotechnology and Bioproducts
Volume 9 - 2022 | https://doi.org/10.3389/fmars.2022.1097594
This article is part of the Research TopicBioactive Terpenoids from Marine Organisms and Their Pharmacological ActivitiesView all 5 articles
Two new prenylated indole diketopiperazine alkaloids (PIDAs) penicamides A and B (1 and 2) and three new polyketides penicinones A−C (6−8), along with four known compounds deoxybrevianamide E (3), brevianamide V (4), 12,13-dehydroprolyl-2-(1,1-dimethylallyltryptophyl)diketopiperazine (5), and 4-hydroxyphenethyl 2-(4-hydroxyphenyl)acetate (9), were isolated and identified from the culture extract of the mangrove-derived fungus Penicillium sp. Their structures were fully elucidated by analyzing spectroscopic data. The absolute configurations of these compounds were determined by the comparison of experimental and calculated electronic circular dichroism (ECD) data and Mo2(OAc)4-induced and Rh2(OCOCF3)4-induced ECD experiments. Structurally, compound 1 is the first example of PIDAs featuring a 6/5/8/6/5 pentacyclic ring system with an α-hydroxy group at C-11, while compound 2 is a new analogue of PIDAs possessing the unique 3-methyleneindolin-2-ol moiety. In addition, compound 6 is a new lactone with the furo[3,4-b]pyran-5-one moiety. Compound 6 displayed potent cytotoxicity against murine melanoma (B16) cells, human breast adenocarcinoma (MCF-7) cells, and human hepatocellular carcinoma (HepG2) cells at 50.0 µM with inhibitory ratios of 82.7%, 75.1% and 95.9%, respectively. In addition, compound 6 exhibited significantly cytotoxic activity against the HepG2 cells, with an IC50 value of 3.87 ± 0.74 µM.
Fungi have been proven to produce a variety of secondary metabolites possessing significantly biological activities (Guo et al., 2020; Newman and Cragg, 2020; Carroll et al., 2022). Since the discovery of penicillin, cyclosporine, and statins, fungal natural products have made irreplaceable contributions to the development of medicine and human health (Bills and Gloer, 2016). In recent years, fungi from a mangrove environment, as plant mutualists, parasitizing the tropical and subtropical intertidal forest wetlands, are considered to be a new source of distinctive secondary metabolites with interesting biological activities (Xu, 2015; Carroll et al., 2022; Chen et al., 2022; Zhu et al., 2022). The fungal genus Penicillium is one of the most filamentous fungi widely distributed in terrestrial and marine habitats and contains more than 483 known species (Perrone and Susca, 2017; Houbraken et al., 2020). Among them, the mangrove-derived fungi of the genus Penicillium are prolific producers of diverse secondary metabolites with a wide range of pharmacological activities (Zhang et al., 2012; Bai et al., 2019; El-Bondkly et al., 2021; Ren et al., 2021), including antitumor agents sumalarin A (Meng et al., 2013) and brocazines A and B (Meng et al., 2014), α-glycosidase inhibitory agents peniisocoumarin C (Cai et al., 2018) and (R)-2-chloro-3-(8-hydroxy-6-methoxy-1-oxo-1H-isochromen-3-yl)propyl acetate (Qiu et al., 2020), antiviral agent simpterpenoid A (Li et al., 2018), antibacterial agent brevianamide S (Song et al., 2018), and protein tyrosine phosphatase 1B (PTP1B) inhibitory agent penerpene E (Zhou et al., 2019). Genome analyses of the biosynthetic gene clusters have also demonstrated that most of Penicillium fungi were biosynthetically talented in producing alkaloids and polyketides (Kozlovskii et al., 2012; Kozlovskii et al., 2015; El Hajj Assaf et al., 2020).
Prenylated indole diketopiperazine alkaloids (PIDAs), featured by tryptophan-containing cyclodipeptides and one or more isoprene units, are an important family of fungal secondary metabolites mainly produced by the genera Penicillium and Aspergillus (Li, 2010; Peng et al., 2014; Ma et al., 2016). Biogenetically, the skeleton of PIDAs could be derived from the condensation of L-tryptophan with another amino acid catalyzed by a non-ribosomal peptide synthetase, followed by subsequent modifications by tailoring enzymes to form a structural variability of this class of natural products (Ma et al., 2016; Wohlgemuth et al., 2017). PIDAs have also attracted considerable interest from organic chemists due to their high structural diversity with significantly biological activities (Baran and Corey, 2002; Ma et al., 2016; Li et al., 2019; Yang et al., 2021).
In our ongoing studies to search for new bioactive compounds from the mangrove-derived fungi (Liu et al., 2021; Hou et al., 2021; Zhang et al., 2022), the strain Penicillium sp. LA032, isolated from the rhizospheric soil of a mangrove ecosystem, was subjected to fermentation on rice. The EtOAc extract showed cytotoxic activity, and its high performance liquid chromatography (HPLC) profiles indicated the presence of abundant secondary metabolites. Then, the large-scale fermentation on rice was performed. The fractionation of the EtOAc extract of this fungus led to the isolation of two new PIDAs penicamides A and B (1 and 2), and three new polyketides penicinones A−C (6−8), along with four known compounds, deoxybrevianamide E (3) (Song et al., 2012), brevianamide V (4) (Song et al., 2012), 12,13-dehydroprolyl-2-(1,1-dimethylallyltryptophyl)diketopiperazine (5) (Steyn, 1973), and 4-hydroxyphenethyl 2-(4-hydroxyphenyl)acetate (9) (Wang et al., 2009) (Figure 1). The details of the isolation, structure elucidation, and biological evaluation of these compounds are reported herein. In addition, the proposed biosynthetic pathways of compounds 1−5 are reported.
The details of the instruments and equipments can be found in the Supplementary Material.
The strain Penicillium sp. LA032 was isolated from the rhizospheric soil of a mangrove ecosystem collected from Beilun Estuary, Fangchenggang, Guangxi, People’s Republic of China. The fungus LA032 was further identified as Penicillium sp. (GenBank Accession No. OP804236), based on a basic local alignment search tool (BLAST) search. The strain was deposited in the laboratory of the Institute of Microbiology, Chinese Academy of Sciences, Beijing.
The strain was firstly cultured on a potato dextrose agar (PDA) medium at 28°C for 7 days. Then, some pieces of agar plugs were cultured on media (1% malt extract, 0.4% yeast extract, and 0.4% glucose) in 500 ml Fernbach flasks at 28°C on a rotary shaker (200 rpm) for 4 days to obtain the seed culture. Finally, the spore inoculums were incubated on 50 × 500 ml Fernbach flasks that contained 100 g of rice and 100 ml of distilled water at room temperature for 21 days.
The fermented products were extracted repeatedly with EtOAc (4 × 6.0 L), which were concentrated to afford 48.0 g of crude residue. The extract was subjected to silica gel column chromatography (CC) eluting with petroleum ether/EtOAc gradient elution to afford eight subfractions Fr.1−Fr.8. Fr.4 (2.1 g) eluted with 30% EtOAc was subjected to silica gel CC with CH2Cl2/MeOH to afford Fr.4.1−Fr.4.8. Fr.4.1 (165.3 mg) was further purified by octadecylsilanized (ODS) CC (20%−100% MeOH/H2O) to yield six subfractions Fr.4.1.1−Fr.4.1.6. Fr.4.1.5 (70.3 mg) eluted with 70% MeOH/H2O was further purified by reversed-phase (RP) HPLC (57% CH3CN/H2O for 60 min; 2.0 ml/min) to afford compound 6 (1.8 mg, tR 54.0 min). Fr.5 (560.0 mg) eluted with 50% EtOAc was separated by ODS CC using MeOH/H2O gradient elution to obtain five subfractions Fr.5.1−Fr.5.5. Fr.5.2 (19.0 mg) eluted with 40% MeOH was further purified by RP-HPLC (51% MeOH/H2O for 50 min; 2.0 ml/min) to get compounds 9 (3.6 mg, tR 28.5 min) and 8 (1.8 mg, tR 43.0 min). Fr.7 (630.0 mg) eluted with 80% EtOAc was separated by ODS CC (30%−100% MeOH/H2O) to afford five subfractions Fr.7.1−Fr.7.5. Fr.7.2 (45.0 mg) was further purified by RP-HPLC (57% MeOH/H2O for 40 min; 2.0 ml/min) to get compounds 3 (4.8 mg, tR 26.8 min), 4 (3.8 mg, tR 28.0 min), and 5 (3.4 mg, tR 30.2 min). Fr. 7.3 (1.01 g) was subjected to chromatography using a Sephadex LH-20 column (CH2Cl2/MeOH, 1:1) to yield six subfractions Fr.7.3.1−Fr.7.3.6. Fr.7.3.3 (30.4 mg) was further purified by RP-HPLC (47% CH3CN/H2O for 30 min; 2.0 ml/min) to get compounds 2 (3.1 mg, tR 21.6 min) and 1 (2.0 mg, tR 25.0 min). Fr.8 (860.0 mg) eluted with 90% EtOAc was purified by ODS CC eluting with MeOH/H2O gradient elution to afford seven subfractions Fr.8.1–Fr.8.7. Fr.8.6 (20.6 mg) eluted with 65% MeOH was further purified by RP-HPLC (70% MeOH/H2O for 30 min; 2.0 ml/min) to get compound 7 (3.1 mg, tR 28.0 min).
Penicilamide A (1): white amorphous powder; +53.3 (c 0.06, MeOH); UV (MeOH) λmax (log ϵ) 230 (2.12), 273 (1.56) nm; ECD (c 0.82 × 10−3 M, MeOH) λmax (Δϵ) 226 (+6.15), 256 (+1.66), 282 (−0.41) nm; IR (neat) νmax 3,343, 2,964, 1,735, 1,636, 1,429, 1,362, and 1,123 cm−1; 1H and 13C nuclear magnetic resonance (NMR) data, see Table 1; high-resolution electrospray ionisation mass spectrometry (HRESIMS) m/z 362.1508 [M + H]+ (calcd for C21H20N3O3, 362.1505).
Penicilamide B (2): white amorphous powder; −140.9 (c 0.10, MeOH); UV (MeOH) λmax (log ϵ) 246 (2.60), 333 (1.93) nm; ECD (c 0.81 × 10−3 M, MeOH) λmax (Δϵ) 234 (+52.3), 266 (−23.7), 341 (−11.3) nm; IR (neat) νmax 3,358, 2,973, 1,667, 1,420, and 1,243 cm−1; 1H and 13C NMR data, see Table 1; HRESIMS m/z 368.1970 [M + H]+ (calcd for C21H26N3O3, 368.1969).
Penicinone A (6): white amorphous powder; −20.0 (c 0.10, MeOH); UV (MeOH) λmax (log ϵ) 245 (3.50) nm; ECD (c 1.01 × 10−3 M, MeOH) λmax (Δϵ) 246 (+31.0), 285 (−17.9) nm; IR (neat) νmax 3,399, 2,955, 2,927, 1,765, 1,644, 1,456, and 1,034 cm−1; 1H and 13C NMR data, see Table 2; HRESIMS m/z 295.1546 [M − H]− (calcd for C16H23O5, 295.1545).
Penicinone B (7): yellow oil; UV (MeOH) λmax (log ϵ) 224 (1.56), 274 (2.05) nm; IR (neat) νmax 3,436, 2,949, 1,735, 1,610, 1,438, and 1,219 cm−1; 1H and 13C NMR data, see Table 2; HRESIMS m/z 297.1332 [M + H]+ (calcd for C15H21O6, 297.1333).
Penicinone C (8): colorless oil; −10.0 (c 0.10, CHCl3); UV (MeOH) λmax (log ϵ) 217 (2.69), 276 (0.56) nm; ECD (c 1.00 × 10−3 M, MeOH) λmax (Δϵ) 220 (+1.88) nm; IR (neat) νmax 3,370, 2,958, 1,731, 1,614, 1,516, and 1,225 cm−1; 1H and 13C NMR data, see Table 2; HRESIMS m/z 309.1104 [M + Na]+ (calcd for C17H18O4Na, 309.1103).
The ligand-compound 6 (0.2 mg) was added into a dry solution of Mo2(OAc)4 (0.7 mg) in dimethyl sulfoxide (DMSO) (400 μl) and was subjected to CD measurements. Firstly, the ECD spectrum was recorded immediately after mixing. Subsequently, its evolution was monitored every 10 min until stationary. Finally, the inherent ECD was subtracted. The diagnostic band observed at ca. 312 nm in the induced ECD spectrum was correlated to the absolute configuration of the 7,8-diol moiety on the basis of Snatzke’s regulations (Frelek et al., 1997).
Compound 8 (0.3 mg) was dissolved in a dry solution of the CH2Cl2 (500 μl). Then, 0.6 mg of [Rh2(OCOCF3)4] was added. Subsequently, the first induced ECD spectrum was recorded at once. The following spectrum was measured every 5 min for four times. The correlation between the absolute configuration of C-8 secondary alcohol in 8 and the sign of the E band at 350 nm was inferred using the bulkiness rule (Gerards and Snatzke, 1990; Frelek and Szczepek, 1999).
The ECD calculations were performed as described previously (Zhang et al., 2022). A conformational search was performed using Maestro 10.2 (Ji and Xu, 2021). The conformers were then optimized using the density functional theory method at the B3LYP/6-311G(2d,p) level by the software package Gaussian 09 (Frisch et al., 2013). The 60 lowest energy conformations were calculated by the ECD calculations using the time-dependent density functional theory (TDDFT) methodology at the CAM-B3LYP/6-311G(d,p) level.
Cytotoxic assays were conducted as previously described (Liu et al., 2015). The detailed procedure is described in the Supplementary Material.
The fungus Penicillium sp. LA032 was fermented on rice solid culture and extracted three times with EtOAc. The EtOAc extract (48.0 g) was fractionated by silica gel vacuum liquid chromatography (VLC), Sephadex LH-20 CC, and semipreparative HPLC to afford five new compounds (1, 2 and 6−8) and four known compounds.
Penicilamide A (1) was obtained as white amorphous powder. The molecular formula of 1 was determined as C21H19N3O3 by the HRESIMS molecular ion peak at m/z 362.1508 [M + H]+ (calcd for C21H20N3O3, 362.1505), indicating 14 degrees of unsaturation. The IR spectrum showed absorption bands at 3,343, 2,964, 1,735, 1,636, and 1,429 cm−1, indicating the presence of amino, hydroxy, carbonyl, and aromatic functional groups. The 1H NMR spectrum (Table 1 and Figure S1) exhibited one exchangeable proton (δH 10.03), nine olefinic or aromatic protons (δH 7.53, 7.30, 7.14, 6.93, 6.92, 6.75, 6.31, 6.14, and 5.97), one methylene (δH 3.99 and 3.54), and two singlet methyls (δH 1.57 and 1.48). The 13C NMR spectrum (Figure S2) and heteronuclear single quantum coherence (HSQC) spectrum (Figure S3) showed the presence of 21 carbon resonances, including two methyls, one methylene, nine olefinic or aromatic methines, and nine quaternary carbons (two sp3 quaternary carbons at δC 38.4 and 73.8; five sp2 carbons at δC 103.3, 126.3, 128.9, 136.0, and 142.8; and two amide carbonyls at δC 165.1 and 156.9). Taking the above NMR data and molecular formula into consideration, an aromatic system, four double bonds and two amide carbonyl functionalities accounted for 10 indices of hydrogen deficiency, suggesting that 1 was a pentacyclic compound. The disubstituted indole moiety (rings A and B) in 1 was assigned by the heteronuclear multiple bond correlations (HMBC) correlations (Figures 2 and S5) from H-4 to C-3 (δC 103.3), C-6 (δC 121.7), C-8 (δC 136.0), and C-9 (δC 128.9) and from H-7 to C-5 (δC 119.7), C-8, and C-9, together with the 1H-1H COSY cross-peaks (Figures 2 and S4) of H-4/H-5/H-6/H-7. The characteristic NMR data of two amide carbonyls (C-12 and C-18: δC 165.1 and 156.9, respectively) and the HMBC cross-peaks from H-16 to C-17 (δC 126.3) and C-18 and from H-14 to C-17, along with the 1H-1H COSY cross-peaks of H-14/H-15/H-16, permitted the completion of the diketopiperazine ring and the unsaturated proline moiety (rings D and E). The other HMBC correlations from H2-10 to C-2 (δC 142.8), C-3, C-9, C-11 (δC 89.0) and C-12, from H-20 to C-18 and C-22 (δC 38.4), from H-21 to C-2, and from H3-23/H3-24 to C-2, C-21 (δC 141.9), and C-22, combined with the 1H-1H COSY cross-peak of H-20/H-21, safely expounded that rings B and D were cyclized at C-20 and a nitrogen atom to form an azocane subunit (ring C), completing the unusual 6/5/8/6/5 pentacyclic ring system. The chemical shift value for C-11 (δC 89.0) indicated that the carbon C-11 was substituted by the hydroxyl group, which was also supported by the molecular formula of 1. Then, the structure of 1 was finally determined.
The absolute configuration for compound 1 was determined unequivocally by the comparison of the experimental and calculated ECD curves. The ECD spectra of 11R-(1a) and 11S-(1b) were performed using the time-dependent density functional theory (TD-DFT) at the CAM-B3LYP/6-311G(d,p) level. The calculated ECD spectrum of 11R-(1a) matched well with the experimental one of 1 (Figure 3), which allowed the assignment of the absolute configuration of 1 as 11R.
Penicilamide B (2) had a molecular formula of C21H25N3O3 as deduced from the HRESIMS and 13C NMR data, with 11 degrees of unsaturation, which is 16 mass units higher than that of deoxybrevianamide E (3) (Song et al., 2012). The 1H, 13C, and HSQC spectroscopic data (Table 1 and Figures S7–S9) suggested the presence of two methyls, four methylenes (one sp2 methylene), two sp3 methines, six olefinic/aromatic methines, and seven quaternary carbons including two amide carbonyls (δC 164.3 and 165.7). The 1H NMR and 13C NMR data (Table 1) of 2 were similar to those of the coisolated compound 3, with slight differences in the chemical shift values of C-2, C-3, C-9, C-10, C-11, and C-20, suggesting their structural similarity. A further analysis of their NMR data indicated that the olefin C-2/C-3 in 3 was replaced by the olefin C-3/C-10 in 2 and an extra hydroxy group was located at C-2 in 2, as supported by the HMBC correlations (Figures 2 and S11) from 1-NH (δH 6.26), H-21, H3-23, and H3-24 to the sp3 quaternary carbon C-2 (δC 98.4) and C-20, from H-4 (δH 7.31) to the olefinic quaternary carbon C-3 (δC 149.0), from H-10 (δH 6.38) to C-2, C-3, C-9, C-11, and C-12 and from H-11 (δH 5.26) to C-3 and the olefinic methine carbon C-10 (δC 121.1), together with the 1H-1H COSY cross-peak (Figures 2 and S10) of H-10/H-11. In the nuclear overhauser effect spectroscopy (NOESY) spectrum (Figures 4 and S12), the NOESY correlation of H-4/H-10 suggested the olefin C-3/C-10 as Z geometry. In addition, the cross-peaks of H-11 with H-17 and H3-24 suggested that these protons were cofacial, implying the existence of two possible configurations 2R,11S,17S-(2a) and 2S,11R,17R-(2b). Lastly, the absolute configuration of 2 was also established by ECD calculations. The experimental ECD curve was consistent with the calculated one of 2R,11S,17S-(2a), indicating the 2R,11S,17S configuration of 2. Finally, compound 2 was established as a PIDA featuring a rare 3-methyleneindolin-2-ol moiety.
Penicinone A (6), obtained as white amorphous powder, had a molecular formula of C16H24O5 as deduced from the negative HRESIMS peak at m/z 295.1546 [M − H]− (calcd for C16H23O5, 295.1545) and the NMR data (Table 2), implying five indices of hydrogen deficiency. The analysis of the 1H, 13C, and HSQC spectra (Table 2 and Figures S13–S15) showed the presence of two methyls, six sp3 methylenes, one sp2 methylene (δC/H 91.7/4.02, 5.04), two oxygenated methines (δC/H 89.0/4.31 and 66.9/4.20), one sp3 quaternary carbon (δC 72.2), three sp2 quaternary carbons, and one carbonyl (δC 167.5). The NMR data of 6 were almost similar to those of isoaigialone B (Silva et al., 2017) except that one oxygenated methine and one methyl group were absent, while the exocyclic double bond (δC/H 91.7/4.99, 5.04; δC 149.5) signals were observed in 6. The HMBC correlations (Figures 2 and S17) from H2-10 (δH 4.99, 5.04) to C-3 (δC 149.5) and C-4 (δC 163.7) further confirmed the existence of an exocyclic double bond at C-3 and C-10 positions of 6. A comprehensive analysis of the 1H-1H COSY (Figures 2 and S16) and HMBC correlations corroborated the planar structure of compound 6 with the furo[3,4-b]pyran-5-one ring system as shown. The NOESY correlations (Figures 4 and S18) of H3-11 with H-6 and H-8 suggested that these protons were on the same side of the pyran ring. Furthermore, Snatzke’s method was employed to address the absolute configuration of the 7,8-diol unit. After mixing 6 and dimolybdenum tetraacetate [Mo2(OAc)4] in DMSO, ECD data were measured between 250 and 450 nm. The inherent ECD was subtracted. The positive Cotton effect (CE) at 312 nm (Figure 5) in the induced spectrum of 6 indicated a 7R,8R configuration of the 7,8-diol (Frelek et al., 1997; Di Bari et al., 2001). Thus, the absolute configuration of 6 was established as 6R,7R,8R.
Penicinone B (7) was obtained as yellow oil. Its molecular formula was deduced as C15H20O6 (six degrees of unsaturation) by the analysis of its HRESIMS and 1D NMR data (Table 2). The interpretation of its IR spectrum indicated the presences of hydroxy (3436 cm−1) and α,β-unsaturated lactone carbonyl (1735 cm−1) functional groups. The analysis of the 1H, 13C and HSQC NMR data (Table 2 and Figures S19–S21) of 7 revealed the presence of five methylenes (one oxygenated), two methoxy groups (δC/H 60.4/4.02 and 51.6/3.61), six olefinic carbons (three protonated), and two ester carbonyl carbons (δC 167.5 and 174.1), accounting for five degrees of unsaturation. Therefore, the remaining one unsaturation indicated the presence of a monocyclic ring system in 7. The characteristic signals of the α,β-unsaturated lactone subunit corresponding to a lactone carbonyl carbon (δC 167.5), a quaternary carbon (δC 141.0) and two olefinic carbons (δC 172.3 and 91.9, respectively), as well as the HMBC correlations (Figures 2 and S23) from H-2 to C-1, C-3 and C-4 and from H3-15 to C-3, permitted the completion of the 2(5H)-furanone moiety with the methoxy carbon C-15 attached at C-3. The 1H-1H COSY cross-peaks (Figures 2 and S22) of H-6/H-7/H2-8/H2-9/H2-10/H2-11, combined with the HMBC cross-peaks from H2-10, H2-11 and H3-13 to C-12 (δC 174.1), established the C-6–C-13 subunit. Furthermore, the HMBC correlations from H-6 to C-4 and C-14, from H-7 to C-5, and from H2-14 to C-4, C-5 and C-6 demonstrated that both C-6 and C-14 were directly attached to the C-4/C-5 double bond at C-5. The remaining hydroxyl group was substituted at C-14, which are deduced by the chemical shift of C-14 (δC 55.6) and the molecular formula of 7. The large coupling constant of the olefinic protons of H-6 and H-7 (15.6 Hz) suggested the olefin C-6/C-7 as E geometry. The NOESY correlation H3-15 to H-2 and the lack of NOESY cross-peak between H3-15 and H2-14 indicated the Z-configuration of the C-4/C-5 double bond based on the previous literature (Phainuphong et al., 2017). Therefore, the structure of 7 was established as depicted.
Penicinone C (8) was isolated as colorless oil. The molecular formula of 8 was established as C17H18O4 from the positive HRESIMS data (m/z 309.1104 [M + Na]+, calcd 309.1103). The 1H and 13C NMR data (Table 2) of 8 were close to those of 4′-hydroxyphenylethyl 4,8(R)-dihydroxyphenylpropionate (Sun et al., 2021), except that the hydroxy group at C-4 disappeared in 8. This observation was supported by the HMBC correlations (Figures 2 and S29) from H2-7 (δH 2.87, 3.01) to C-1 (δC 138.6), C-2 (δC 130.5), C-6 (δC 130.5) and C-9 (δC 174.5) and from H-2 (δH 7.18) and H-6 (δH 7.18) to C-4 (δC 127.3), as well as the 1H-1H COSY cross-peaks (Figures 2 and S28) of H-3/H-4/H-5. The absolute configuration of C-8 in 8 was determined by the circular dichroism data of the in situ formed [Rh2(OCOCF3)4] complex, with the inherent contribution subtracted (Gerards and Snatzke, 1990; Frelek and Szczepek, 1999). The Rh2(OCOCF3)4-induced ECD spectrum of 8 showed positive CE at 350 nm (Figure 6), suggesting the 8S configuration (Gerards and Snatzke, 1990; Frelek and Szczepek, 1999). Therefore, the absolute configuration of compound 8 was established as 8S.
Figure 6 ECD spectrum of the [Rh2(OCOCF3)4] complex of compound 8 with the inherent ECD spectrum subtracted.
The structures of four known compounds, deoxybrevianamide E (3) (Song et al., 2012), brevianamide V (4) (Song et al., 2012), 12,13-dehydroprolyl-2-(1,1-dimethylallyltryptophyl)diketopiperazine (5) (Steyn, 1973) and 4-hydroxyphenethyl 2-(4-hydroxyphenyl)acetate (9) (Wang et al., 2009) (Figure 1), were assigned by the interpretation of their spectroscopic data with those reported in the literature.
To the best of our knowledge, the PIDAs featuring the 6/5/8/6/5 pentacyclic moiety are rare in nature, with only 10 compounds being reported (Steyn, 1973; Quang et al., 2013; Zhuravleva et al., 2021). Moreover, the hydroxy group is usually located at the C-3, C-16, or C-17 position in these metabolites (Quang et al., 2013; Zhuravleva et al., 2021). Penicilamide A (1) represents the first example of PIDAs featuring the unique 6/5/8/6/5 pentacyclic skeleton with an α-hydroxy group at the C-11 position. Meanwhile, penicilamide B (2) is a new PIDA with a 3-methyleneindolin-2-ol moiety. Biogenetically, PIDAs 1−5 could be derived from the condensation of L-tryptophan with L-proline. The biosynthetic intermediate I was firstly produced, followed by reverse C2 prenylation to afford the intermediate 3. Starting from compound 3, compounds 1, 2, 4, and 5 could be generated via oxidation, dehydrogenation, and cyclization reactions. The plausible biosynthetic pathways were proposed as shown in Scheme 1. In addition, compound 6 was established as a rare furo[3,4-b]pyran-5-one skeleton with an n-heptyl moiety. Meanwhile, compounds 7 and 8 are γ-butenolide and phenolic acid derivatives, respectively.
Compounds 1−7 were tested for their cytotoxic activity against B16 (murine melanoma cells), MCF-7 (human breast adenocarcinoma cells), and HepG2 (human hepatocellular carcinoma cells) at 50.0 μM. Compound 6 displayed potent cytotoxicity against B16, MCF-7, and HepG2 cell lines at 50.0 µM with inhibitory ratios of 82.7%, 75.1%, and 95.9%, respectively (Figure 7). Furthermore, compound 6 showed more potent cytotoxicity against HepG2 cells (IC50 value of 3.09 ± 0.03 µM) than that of the positive-control cisplatin (IC50 value of 8.71 ± 0.28 μM). Compound 6 also showed cytotoxicity against MCF-7 and B16 cells, with IC50 values of 30.01 ± 0.52 and 27.91 ± 0.17 μM, respectively, whereas the positive-control cisplatin showed IC50 values of 4.22 ± 0.46 and 20.39 ± 3.25 μM, respectively. Other compounds did not show obviously inhibitory effects on the cell lines tested at 50.0 μM (Table 3).
In summary, two new PIDAs penicamides A and B (1 and 2) and three new polyketides penicinones A−C (6−8), together with four known compounds, were identified from the mangrove-derived fungus Penicillium sp. Compound 1 possesses an unusual 6/5/8/6/5 pentacyclic ring system with an α-hydroxy group at C-11, which was rarely encountered in the PIDAs. Compound 2 is the first reported natural product of PIDAs with a 3-methyleneindolin-2-ol moiety. Compound 6 is a rare furo[3,4-b]pyran-5-one skeleton with an n-heptyl moiety. Compound 6 exhibited significant cytotoxicity against HepG2 cells, which might be worthy for further pharmacological study and structural modification toward more potent drug leads. These findings not only enriched the chemical space of the PIDA and polyketide families but also provided important hints for the discovery of new drug leads.
The original contributions presented in the study are included in the article/Supplementary Material. Further inquiries can be directed to the corresponding author/s.
Conceptualization, LL. Methodology, RH. Resources, RH, JZ, and SN. Data curation, RH and JZ. Writing–original draft preparation, RH. Writing–review and editing, RH and LL. Project administration and funding acquisition, LL. All authors contributed to the article and approved the submitted version.
This research was funded by grants from National Key Research and Development Program of China (2021YFC2100600) and the National Natural Science Foundation of China (32022002 and 21977113).
The authors declare that the research was conducted in the absence of any commercial or financial relationships that could be construed as a potential conflict of interest.
All claims expressed in this article are solely those of the authors and do not necessarily represent those of their affiliated organizations, or those of the publisher, the editors and the reviewers. Any product that may be evaluated in this article, or claim that may be made by its manufacturer, is not guaranteed or endorsed by the publisher.
The Supplementary Material for this article can be found online at: https://www.frontiersin.org/articles/10.3389/fmars.2022.1097594/full#supplementary-material
Bai M., Zheng C. J., Huang G. L., Mei R. Q., Wang B., Luo Y. P., et al. (2019). Bioactive meroterpenoids and isocoumarins from the mangrove-derived fungus Penicillium sp. TGM112. J. Nat. Prod. 82, 1155–1164. doi: 10.1021/acs.jnatprod.8b00866
Baran P. S., Corey E. J. (2002). A short synthetic route to (+)-austamide, (+)-deoxyisoaustamide, and (+)-hydratoaustamide from a common precursor by a novel palladium-mediated indole → dihydroindoloazocine cyclization. J. Am. Chem. Soc 124, 7904–7905. doi: 10.1021/ja026663t
Bills G. F., Gloer J. B. (2016). Biologically active secondary metabolites from the fungi. Microbiol. Spectr. 4, 1–32. doi: 10.1128/microbiolspec.FUNK-0009-2016
Cai R. L., Wu Y. N., Chen S. H., Cui H., Liu Z. M., Li C. Y., et al. (2018). Peniisocoumarins A-J: Isocoumarins from Penicillium commune QQF-3, an endophytic fungus of the mangrove plant Kandelia candel. J. Nat. Prod. 81, 1376–1383. doi: 10.1021/acs.jnatprod.7b01018
Carroll A. R., Copp B. R., Davis R. A., Keyzers R. A., Prinsep M. R. (2022). Marine natural products. Nat. Prod. Rep. 39, 1122–1171. doi: 10.1039/D1NP00076D
Chen S. H., Cai R. L., Liu Z. M., Cui H., She Z. G. (2022). Secondary metabolites from mangrove-associated fungi: source, chemistry and bioactivities. Nat. Prod. Rep. 39, 560–595. doi: 10.1039/D1NP00041A
Di Bari L., Pescitelli G., Pratelli C., Pini D., Salvadori P. (2001). Determination of absolute configuration of acyclic 1,2-diols with Mo2(OAc)4. 1. snatzke’s method revisited. J. Org. Chem. 66, 4819–4825. doi: 10.1021/jo010136v
El-Bondkly E. A. M., El-Bondkly A. A. M., El-Bondkly A. A. M. (2021). Marine endophytic fungal metabolites: A whole new world of pharmaceutical therapy exploration. Heliyon 7, e06362. doi: 10.1016/j.heliyon.2021.e06362
El Hajj Assaf C., Zetina-Serrano C., Tahtah N., Khoury A. E., Atoui A., Oswald I. P., et al. (2020). Regulation of secondary metabolism in the Penicillium genus. Int. J. Mol. Sci. 21, 9462. doi: 10.3390/ijms21249462
Frelek J., Ikekawa N., Takatsuto S., Snatzke G. (1997). Application of [Mo2(OAc)4] for determination of absolute configuration of brassinosteroid vic-diols by circular dichroism. Chirality 9, 578–582. doi: 10.1002/(SICI)1520-636X(1997)9:5/6<578::AID-CHIR27>3.0.CO;2-K
Frelek J., Szczepek W. J. (1999). [Rh2(OCOCF3)4] as an auxiliary chromophore in chiroptical studies on steroidal alcohols. Tetrahedron: Asymmetry 10, 1507–1520. doi: 10.1016/S0957-4166(99)00115-9
Frisch M. J., Trucks G. W., Schlegel H. B. M., Scuseria G. E., Robb M. A., Cheeseman J. R., et al. (2013). Gaussian 09, revision e. 01 (Wallingford, CT: Gaussian, Inc).
Gerards M., Snatzke G. (1990). Circular dichroism. XCIII determination of the absolute configuration of alcohols, olefins, epoxides, and ethers rom the CD of their “in situ” complexes with [Rh2(O2CCF3)4]. Tetrahedron: Asymmetry 1, 221–236. doi: 10.1016/S0957-4166(00)86328-4
Guo L. F., Liu G. R., Liu L. (2020). Caryophyllene-type sesquiterpenoids and α-furanones from the plant endophytic fungus Pestalotiopsis theae. Chin. J. Nat. Med. 18, 261–267. doi: 10.1016/S1875-5364(20)30032-7
Houbraken J., Kocsubé S., Visagie C. M., Yilmaz N., Wang X. C., Meijer M., et al. (2020). Classification of aspergillus, Penicillium, Talaromyces and related genera (Eurotiales): An overview of families, genera, subgenera, sections, series and species. Stud. Mycol. 95, 5–169. doi: 10.1016/j.simyco.2020.05.002
Hou B. L., Liu S. S., Huo R. Y., Li Y. Q., Ren J. W., Wang W. Z., et al. (2021). New diterpenoids and isocoumarin derivatives from the mangrove-derived fungus Hypoxylon sp. Mar. Drugs 19, 362. doi: 10.3390/md19070362
Ji C. F., Xu X. Z. (2021). Recent advancements of macrolide hybrids against Staphylococcus aureus. Curr. Top. Med. Chem. 21, 2455–2473. doi: 10.2174/1568026620999201203213733
Kozlovskii A. G., Antipova T. V., Zhelifonova V. P. (2015). Biosynthesis of biologically active low-molecular weight compounds by fungi of the genus Penicillium (review). Appl. Biochem. Microbiol. 51, 236–242. doi: 10.1134/s0003683815020118
Kozlovskii A. G., Zhelifonova V. P., Antipova T. V., Baskunov B. P., Kochkina G. A., Ozerskaya S. M. (2012). Secondary metabolite profiles of the Penicillium fungi isolated from the arctic and antarctic permafrost as elements of polyphase taxonomy. Microbiology 81, 306–311. doi: 10.1134/S0026261712030071
Li S. M. (2010). Prenylated indole derivatives from fungi: structure diversity, biological activities, biosynthesis and chemoenzymatic synthesis. Nat. Prod. Rep. 27, 57–78. doi: 10.1039/b909987p
Liu L., Chen X. X., Li D., Zhang Y., Li L., Guo L. D., et al. (2015). Bisabolane sesquiterpenoids from the plant endophytic fungus Paraconiothyrium brasiliense. J. Nat. Prod. 78, 746–753. doi: 10.1021/np5009569
Liu G. R., Niu S. B., Liu L. (2021). Alterchromanone a, one new chromanone derivative from the mangrove endophytic fungus Alternaria longipes. J. Antibiot. (Tokyo) 74, 152–155. doi: 10.1038/s41429-020-00364-4
Li H. L., Xu R., Li X. M., Yang S. Q., Meng L. H., Wang B. G. (2018). Simpterpenoid a, a meroterpenoid with a highly functionalized cyclohexadiene moiety featuring gem-propane-1,2-dione and methylformate groups, from the mangrove-derived Penicillium simplicissimum MA-332. Org. Lett. 20, 1465–1468. doi: 10.1021/acs.orglett.8b00327
Li H. Q., Xu D., Sun W. G., Yang B. Y., Li F. L., Liu M. T., et al. (2019). HPLC-DAD-directed isolation of linearly fused prenylated indole alkaloids from a soil-derived Aspergillus versicolor. J. Nat. Prod. 82, 2181–2188. doi: 10.1021/acs.jnatprod.9b00183
Ma Y. M., Liang X. A., Kong Y., Jia B. (2016). Structural diversity and biological activities of indole diketopiperazine alkaloids from fungi. J. Agric. Food Chem. 64, 6659–6671. doi: 10.1021/acs.jafc.6b01772
Meng L. H., Li X. M., Lv C. T., Huang C. G., Wang B. G. (2014). Brocazines a-f, cytotoxic bisthiodiketopiperazine derivatives from Penicillium brocae MA-231, an endophytic fungus derived from the marine mangrove plant Avicennia marina. J. Nat. Prod. 77, 1921–1927. doi: 10.1021/np500382k
Meng L. H., Li X. M., Lv C. T., Li C. S., Xu G. M., Huang C. G., et al. (2013). Sulfur-containing cytotoxic curvularin macrolides from Penicillium sumatrense MA-92, a fungus obtained from the rhizosphere of the mangrove Lumnitzera racemosa. J. Nat. Prod. 76, 2145–2149. doi: 10.1021/np400614f
Newman D. J., Cragg G. M. (2020). Natural products as sources of new drugs over the nearly four decades from 01/1981 to 09/2019. J. Nat. Prod. 83, 770–803. doi: 10.1021/acs.jnatprod.9b01285
Peng J. X., Gao H. Q., Li J., Ai J., Geng M. Y., Zhang G. J., et al. (2014). Prenylated indole diketopiperazines from the marine-derived fungus Aspergillus versicolor. J. Org. Chem. 79, 7895–7904. doi: 10.1021/jo5010179
Perrone G., Susca A. (2017). Penicillium species and their associated mycotoxins. Methods Mol. Biol. 1542, 107–119. doi: 10.1007/978-1-4939-6707-0_5
Phainuphong P., Rukachaisirikul V., Tadpetch K., Sukpondma Y., Saithong S., Phongpaichit S., et al. (2017). γ-Butenolide and furanone derivatives from the soil-derived fungus Aspergillus sclerotiorum PSU-RSPG178. Phytochemistry 137, 165–173. doi: 10.1016/j.phytochem.2017.02.008
Qiu P., Cai R. L., Lin L., She Z. G. (2020). Three new isocoumarin derivatives from the mangrove endophytic fungus Penicillium sp. YYSJ-3. Chin. J. Nat. Med. 18, 256–260. doi: 10.1016/S1875-5364(20)30031-5
Quang T. H., Lee D. S., Sohn J. H., Kim Y. C., Oh H. (2013). A new deoxyisoaustamide derivative from the marine-derived fungus Penicillium sp. JF-72. Bull. Korean Chem. Soc 34, 3109–3112. doi: 10.5012/bkcs.2013.34.10.3109
Ren J. W., Huo R. Y., Liu G. R., Liu L. (2021). New andrastin-type meroterpenoids from the marine-derived fungus Penicillium sp. Mar. Drugs 19, 189–197. doi: 10.3390/md19040189
Silva G. H., Zeraik M. L., de Oliveira C. M., Teles H. L., Trevisan H. C., Pfenning L. H., et al. (2017). Lactone derivatives produced by a Phaeoacremonium sp., an endophytic fungus from Senna spectabilis. J. Nat. Prod. 80, 1674–1678. doi: 10.1021/acs.jnatprod.5b00828
Song T. F., Chen M. X., Ge Z. W., Chai W. Y., Li X. C., Zhang Z. Z., et al. (2018). Bioactive penicipyrrodiether a, an adduct of GKK1032 analogue and phenol a derivative, from a marine-sourced fungus Penicillium sp. ZZ380. J. Org. Chem. 83, 13395–13401. doi: 10.1021/acs.joc.8b02172
Song F. H., Liu X. R., Guo H., Ren B., Chen C. X., Piggott A. M., et al. (2012). Brevianamides with antitubercular potential from a marine-derived isolate of Aspergillus versicolor. Org. Lett. 14, 4770–4773. doi: 10.1021/ol302051x
Steyn P. S. (1973). The structures of five diketopiperazines from Aspergillus ustus. Tetrahedron 29, 107–120. doi: 10.1016/S0040-4020(01)99384-6
Sun Y. B., Xia G. Y., Xia H., Wu Y. Z., Wang L. Y., Lin S. (2021). A new phenolic acid ester from solid culture of Colletotrichum capsici, an endophytic fungus from Paeonia lactiflora. Chin. J. Chin. Mater. Med. 46, 2837–2842. doi: 10.19540/j.cnki.cjcmm.20210402.201
Wang Y. N., Tian L., Hua H. M., Lu X., Sun S., Wu H. H., et al. (2009). Two new compounds from the broth of the marine fungus Penicillium griseofulvum Y19-07. J. Asian Nat. Prod. Res. 11, 912–917. doi: 10.1080/10286020903219923
Wohlgemuth V., Kindinger F., Xie X. L., Wang B. G., Li S. M. (2017). Two prenyltransferases govern a consecutive prenylation cascade in the biosynthesis of echinulin and neoechinulin. Org. Lett. 19, 5928–5931. doi: 10.1021/acs.orglett.7b02926
Xu J. (2015). Bioactive natural products derived from mangrove associated microbes. RSC Adv. 5, 841–892. doi: 10.1039/C4RA11756E
Yang J., Gong L. Z., Guo M. M., Jiang Y., Ding Y., Wang Z. J., et al. (2021). Bioactive indole diketopiperazine alkaloids from the marine endophytic fungus Aspergillus sp. YJ191021. Mar. Drugs 19, 157. doi: 10.3390/md19030157
Zhang Y., Li X. M., Shang Z., Li C. S., Ji N. Y., Wang B. G. (2012). Meroterpenoid and diphenyl ether derivatives from Penicillium sp. MA-37, a fungus isolated from marine mangrove rhizospheric soil. J. Nat. Prod. 75, 1888–1895. doi: 10.1021/np300377b
Zhang R. H., Zhang J. X., Huo R. Y., Xue Y. X., Hong K., Liu L. (2022). Sulfur-containing benzofurans and α-pyrones from the mangrove-derived fungus Talaromyces sp. WHUF0341. Front. Mar. Sci. 9. doi: 10.3389/fmars.2022.1034945
Zhou L. M., Kong F. D., Fan P., Ma Q. Y., Xie Q. Y., Li J. H., et al. (2019). Indole-diterpenoids with protein tyrosine phosphatase inhibitory activities from the marine-derived fungus Penicillium sp. KFD28. J. Nat. Prod. 82, 2638–2644. doi: 10.1021/acs.jnatprod.9b00620
Zhu J. J., Huang Q. S., Liu S. Q., Ding W. J., Xiong Y. H., Li C. Y. (2022). Four new diphenyl ether derivatives from a mangrove endophytic fungus Epicoccum sorghinum. Chin. J. Nat. Med. 20, 537–540. doi: 10.1016/S1875-5364(22)60171-7
Keywords: Penicillium sp., mangrove rhizospheric soil, fungus, secondary metabolites, cytotoxic activity
Citation: Huo R, Zhang J, Niu S and Liu L (2022) New prenylated indole diketopiperazine alkaloids and polyketides from the mangrove-derived fungus Penicillium sp. Front. Mar. Sci. 9:1097594. doi: 10.3389/fmars.2022.1097594
Received: 14 November 2022; Accepted: 12 December 2022;
Published: 28 December 2022.
Edited by:
Bin Wu, Zhejiang University, ChinaReviewed by:
Hao Gao, Jinan University, ChinaCopyright © 2022 Huo, Zhang, Niu and Liu. This is an open-access article distributed under the terms of the Creative Commons Attribution License (CC BY). The use, distribution or reproduction in other forums is permitted, provided the original author(s) and the copyright owner(s) are credited and that the original publication in this journal is cited, in accordance with accepted academic practice. No use, distribution or reproduction is permitted which does not comply with these terms.
*Correspondence: Ling Liu, bGl1bEBpbS5hYy5jbg==
Disclaimer: All claims expressed in this article are solely those of the authors and do not necessarily represent those of their affiliated organizations, or those of the publisher, the editors and the reviewers. Any product that may be evaluated in this article or claim that may be made by its manufacturer is not guaranteed or endorsed by the publisher.
Research integrity at Frontiers
Learn more about the work of our research integrity team to safeguard the quality of each article we publish.