- 1Institute of Hydrobiology, Jinan University, Guangzhou, China
- 2CAS Key Laboratory of Renewable Energy, Guangdong Provincial Key Laboratory of New and Renewable Energy Research and Development, Guangzhou Institute of Energy Conversion, Chinese Academy of Sciences, Guangzhou, China
- 3CAS Key Laboratory of Tropical Marine Bio-resources and Ecology, Guangdong Key Laboratory of Marine Materia Medica, South China Sea Institute of Oceanology, Chinese Academy of Sciences, Guangzhou, China
Porphyridium exopolysaccharide (EPS) is a kind of high-value biopolymer with various biological activities secreted by microalgae belonging to Porphyridium genus. Salinity is one of the important environmental factors affecting the growth of microalgae. In order to study the effect of salinity, the yield, biochemical characteristics and antioxidant activity of EPS in Porphyridium purpureum FACHB 806 cultured at four salinity levels (5‰, 20‰, 35‰ and 50‰) were investigated in this study. The results demonstrated that P. purpureum FACHB 806 could grow at all salinity levels. The highest cell density and EPS yield per cell were 6.6 × 107 cells·mL-1 and 29.1 pg·cell-1, which were obtained in the 20‰ and 50‰ salinity, respectively. With the increase of salinity, the percentage of xylose and galactose decreased while the percentage of glucose increased. Among all salinity levels, the 5‰ salinity group achieved the maximum contents of total carbohydrate and uronic acid of EPS, and the 50‰ salinity obtained the highest protein content of EPS. Compared to the 20‰ and 35‰ salinity groups, EPS of the 5‰ and 50‰ salinity showed stronger hydroxyl radical scavenging ability. These results indicated that salinity could influence the yield, biochemical composition and antioxidant activity of EPS, which will provide a new strategy to improve the yield and antioxidant activity of EPS.
1 Introduction
Microalgae exopolysaccharide (EPS) is a kind of high-molecular-weight biopolymer secreted by microalgae into the surrounding environment (Liu et al., 2016). EPS mainly perform a variety of physiological functions, including desiccation tolerance, osmotic stress and toxic substances removal (Xiao and Zheng, 2016). Microalgal EPS exhibit complex chemical structures, with most being heteropolysaccharides consisting of glucose and galactose linked by glycosidic bonds (Babiak and Krzemińska, 2021). Currently, a vast majority of known microalgal EPS are sulfated polysaccharides with a variety of biological activities such as hypocholesterolemic, antioxidant and antitumor activities (Raposo et al., 2014). Microalgal EPS has great potential application in nutraceutical and pharmaceutical products.
Porphyridium sp. can secrete a large amount of sulfated EPS, the average molecular weight of which is 2,000 – 7,000 kDa (Li et al., 2019a). The monosaccharide composition includes xylose, galactose, glucose, trace amounts of rhamnose, arabinose and mannose (Geresh et al., 1992). Porphyridium EPS also had high sulfate content (~4.5%), which was related to their high antioxidant activity (Tannin-Spitz et al., 2005). Among the P. cruentum EPS, the 6.55 kDa fragment had stronger antioxidant activity than the 60.66 kDa and 256 kDa fragments (Sun et al., 2009). Additionally, among EPS fractions including IPSI-A, IPSI-B and IPS-II purified by an anion exchange column and gel chromatography from Isochrysis galbana, IPS-II showed the strongest antioxidant activity due to its higher uronic acid and sulfate contents than that of IPSI-A and IPSI-B (Sun et al., 2014). Interestingly, there are potential relationship between monosaccharide composition and antioxidant activity. For example, the EPS-1, EPS-2 and EPS-3 of Weissella cibaria SJ14 were isolated by an ion-exchange chromatography of DEAE Sepharose with 0.1 mol·L-1, 0.5 mol·L-1 and 1.0 mol·L-1 NaCl gradient elution, respectively, the antioxidant activity of EPS-1 was remarkably stronger than EPS-3, EPS-1 was rich in mannose while EPS-3 was rich in galactose (Zhu et al., 2018). In summary, these results indicated that the structural characteristics of EPS, including molecular weight, monosaccharide composition and the content of sulfate and uronic acid, could greatly affect their antioxidant activity.
Salinity is one of the important environmental factors affecting the growth of marine microalgae (Bazzani et al., 2021). Salinity stress leads to a series of physiological and biochemical responses such as photosynthesis inhibition, secondary metabolites synthesis and osmoregulation (Lu et al., 2006). Previous studies indicated that change in salinity could affect the accumulation of microalgal intracellular polysaccharides. Polysaccharides not only participate in osmoregulation but also act as homeostatic regulatory protectors during salinity stress (Shetty et al., 2019). In Chlamydomonas reinhardtii, the expression level of genes participating in the metabolism of starch increased in response to salinity stress (Wang et al., 2018). In addition, salinity influences the synthesis pathways and structural characteristics of EPS. For example, the secretion of EPS in Synechocystis sp. was stimulated by high salinity, and salinity also caused a change in the monosaccharide composition and ratio of EPS (Ozturk and Aslim, 2010). Salinity also influenced the biological activity of microalgal EPS. Both antioxidant and anticoagulant activities of Spirulina platensis EPS were influenced by salinity (Emad et al., 2010).
Porphyridium has strong salinity tolerance and the 35‰ – 46‰ salinity had no significant effect on its growth (Li et al., 2019a). Salinity could influence the synthesis of EPS, total lipids and phycoerythrin in Porphyridium (Lu et al., 2020). This study aimed to investigate the effect of salinity on the yield, biochemical characteristics and antioxidant activity of P. purpureum EPS. Moreover, the relationship between structural characteristics of P. purpureum EPS and antioxidant activity was explored.
2 Materials and methods
2.1 Microalgal strain and culture conditions
P. purpureum FACHB 806, originally isolated by the Ocean University of China, was purchased from the Freshwater Algae Culture Collection of the Institute of Hydrobiology (http://algae.ihb.ac.cn/) in China. P. purpureum FACHB 806 were cultured in the modified ASW medium (Li et al., 2019b). The temperature was maintained at 25 ± 1°C. Illumination was provided by the single-sided T8 fluorescent lamp (Philips, Shanghai, China), with the photoperiod at 24 h: 0 h (light: dark). Carbon source and agitation were supplied by bubbling CO2-enriched compressed air (1% CO2, v: v).
2.2 Experimental design
P. purpureum FACHB 806 was cultured for 7 days in ~300 mL column photobioreactors (Φ 3 cm × 60 cm). By centrifugation at 3,500 rpm for 5 min, FACHB 806 in logarithmic growth phase was collected and then re-inoculated into the fresh modified ASW medium with different salinities, including 5‰, 20‰, 35‰ and 50‰. The initial optical density at the wavelength of 750 nm (OD750) was 0.40 ± 0.02. Each treatment had two biological replicates (n = 2), and each measurement had three technical replicates (n = 3). Microalgal cells and EPS concentration were measured on days 0, 3, 6, 9, 12 and 15. On day 15, EPS were collected and purified for their determination of chemical composition and antioxidant activity.
2.3 Morphological observations and cell count measurements
Cell morphology of P. purpureum FACHB 806 was observed on day 15 by a BX53 light microscope (Olympus, Tokyo, Japan). The microalgae cells were counted every three days using a XB-K-25 hematocytometer (Shanghai Qiujing Biochemical Reagent Instrument, Shanghai, China).
2.4 Determination of EPS concentration
The EPS concentration was measured using the phenol-sulfuric acid method (Dubois et al., 1956). The standard curve of polysaccharides was drew using the glucose (AR, Guangzhou Chemical Reagent Factory, Guangzhou, China) as the standard substance. Regression equation of standard curve is “y = 0.0115x + 0.0036, R2 = 0.999”. The culture (5.0 mL) was centrifuged at 8,000 rpm for 10 min. The supernatant was collected for the determination of EPS concentration. The supernatant (1.0 mL) was taken in glass tube, and then 0.5 mL of 6% phenol solution (AR, Guangzhou Chemical Reagent Factory, Guangzhou, China) and 2.5 mL sulfuric acid (AR, Guangzhou Chemical Reagent Factory, Guangzhou, China) were added. The absorbance was measured at 490 nm using a TU-1810 UV-Visible spectrophotometer (Persee General Instrument Co. Ltd., Beijing, China).
2.5 Preparation of crude EPS
After 15 days cultivation, the culture was centrifuged at 8,000 rpm for 10 min, the supernatant was collected and concentrated by a RE-2000A rotary evaporator (Yarong Co. Ltd., Shanghai, China) at 50°C. The 95% ethanol (Puboxin Biotechnology Co., Ltd., Beijing, China) was added to the supernatant and the flocs were collected for freeze-drying (95% ethanol: supernatant = 4: 1, v: v). The flocs was redissolved in distilled water and the solution was dialyzed to remove small molecules (500 Da cutoff). After dialyzing, the 95% ethanol was added to the solution and the floating flocs were collected for freeze-drying (95% ethanol: supernatant = 4: 1, v: v) (Chen et al., 2010). The freeze-dried flocs was the crude EPS.
2.6 Fourier transform infrared spectroscopy (FT-IR) analysis of EPS
The EPS (~2 mg) mixed with KBr (~200 mg) were prepared for FT-IR (Fourier-transform infrared absorption) analysis. The infrared spectrum of EPS (500 – 4,000 cm-1) was measured in an IR affinity-1 Fourier transform infrared spectrometer (Shimadzu Corporation, Kyoto, Japan) with 32 scans and a resolution of 4.0 cm-1.
2.7 Determination of monosaccharide composition of EPS
The derivatization of EPS was performed using the Luo’s method (Luo et al., 2010). The EPS (5.0 mg) was dissolved in 2.0 mL of trifluoroacetic acid solution (2.0 mol·L-1) (AR, Macklin Biochemical Technology Co., Ltd., Shanghai, China) and the mixture was hydrolyzed at 110°C for 2 h, then repeatedly co-distilled with methanol (AR, Tianjin Damao Chemical Reagent Co., Ltd., Tianjin, China) to dryness. Hydroxylamine (AR, Macklin Biochemical Technology Co., Ltd., Shanghai, China), inositol hydrochloride (99%, Macklin Biochemical Technology Co., Ltd., Shanghai, China) and pyridine (99%, Macklin Biochemical Technology Co., Ltd., Shanghai, China) were added to the EPS hydrolysate and shaken at 90°C for 30 min, then acetic anhydride (AR, Guangzhou Chemical Reagent Factory, China) was added and shaken at 90°C for 30 min. After cooling, the aldononitrile acetate derivative was obtained and used to analyze the monosaccharide composition of EPS. The monosaccharide composition was determined by GC-2014 gas chromatograph (Shimadzu Corporation, Kyoto, Japan) equipped with a flame ionization detector and an SH-Rtx-5 capillary column (30 m × 0.25 mm × 0.25 µm, Shimadzu Corporation, Kyoto, Japan). Argon was used as the carrier gas at a flow rate of 1 mL·min-1. The column temperature was programmed from 120°C to 180°C (with a hold of 4 min). The temperatures of injection port and detector were maintained at 250°C and 280°C, respectively.
2.8 Determination of total soluble protein of EPS
Total protein content was determined using the Lowry method (Lowry et al., 1951). The standard curve of protein was drew using the protein standard substance in the kit (Beijing Solarbio Science & Technology Co., Ltd, Beijing, China). Regression equation of standard curve is “y = 1.1911x + 0.0097, R2 = 0.999”. A total of 2.0 mL of Folin-phenol A was added to 200 μL of EPS solution and left at room temperature for 10 min. Then 20 μL of Folin-phenol B was added and bathed at 37°C for 30 min. Finally, the absorbance was measured at 650 nm using a UV-160 U spectrophotometer (Shimadzu Scientific Instruments, Inc., Columbia, USA).
2.9 Determination of the content of uronic acid and sulfate
The content of uronic acid was determined using the meta-hydroxyphenyl method (Blumenkrantz and Asboe-Hansen, 1973). The standard curve of uronic acid was drew using the glucuronic acid (≥ 98%, Puboxin Biotechnology Co., Ltd., Beijing, China) as the standard substance. Regression equation of standard curve is “y = 0.0585x + 0.0085, R2 = 0.999”. Approximately, 1.5 mL of sulfuric acid-tetraborate (anhydrous, ≥ 99%, Macklin Biochemical Technology Co., Ltd., Shanghai, China) was added to 0.25 mL of EPS solution and bathed at 100°C for 5 min. After cooling in a water-ice bath, 25 μL of 0.15% meta-hydroxyphenyl (HPLC, ≥ 97%, Puboxin Biotechnology Co., Ltd., Beijing, China) was added. The absorbance value was measured at 520 nm a Shimadzu UV-160 U spectrophotometer and the value was recorded as A. Due to the production of pinkish color in the presence of sulfuric acid-tetraborate at 100°C, 0.15% meta-hydroxyphenyl was replaced by 25 μL of 0.5% NaOH (AR, Guangzhou Chemical Reagent Factory, Guangzhou, China) to eliminate the interference. The absorbance value at 520 nm was recorded as A0. The difference between A0 and A was obtained and used to calculate the content of uronic acid.
The content of sulfate was determined by ICS-2500 ion chromatography (DIONEX, Sunnyvale, USA) (Reim, 1991). EPS with 2.0 mL of 1.0 mmol·L-1 hydrochloric acid (Shenzhen Bolinda Technology Co., Ltd., Shenzhen, China) were hydrolyzed at 100°C for 6 h and filtered using a 0.45 µm microporous membrane. The mixture volume was adjusted to 5.0 mL using the distilled water and used to determine the content of sulfate.
2.10 Determination of hydroxyl radical scavenging ability
A modified Li’s method was employed to determine the hydroxyl radical (·OH) scavenging activity (Li et al., 2008). The EPS solution with different concentrations (0.2, 0.4, 0.6, 0.8 and 1.0 mg·L-1), 1,10-phenanthroline (0.75 mmol·L-1) (99%, Kuer Chemical Technology Co., Ltd., Beijing, China) and FeSO4 (0.75 mmol·L-1) (AR, Guangzhou Chemical Reagent Factory, Guangzhou, China) were dissolved in phosphate buffer (0.15 mol·L-1, pH 7.4) (AR, Guangzhou Chemical Reagent Factory, Guangzhou, China), then 0.01% H2O2 (AR, Guangzhou Chemical Reagent Factory, Guangzhou, China) was added. The absorbance was measured at 536 nm after bathing at 37°C for 30 min. The hydroxyl radical scavenging activity was estimated using the following equation:
A0 is the absorbance of the mixture containing 1,10-phenanthroline, FeSO4, H2O2 and H2O; A1 is the absorbance of the mixture containing 1,10-phenanthroline, FeSO4, H2O2 and EPS solution; A2 is the absorbance of the mixture containing 1,10-phenanthroline, FeSO4, H2O and EPS solution.
2.11 Statistical analysis
The means and standard deviations shown in all figures and tables were obtained from two biological replicates and three technical replicates. One-way ANOVA was performed using SPSS 18.0 statistical software (SPSS Inc., Chicago, IL, USA). Differences between sample means were analyzed using the least significant difference method (significance level, 0.05).
3 Results
3.1 The growth characteristics of P. purpureum FACHB 806
As shown in Figure 1A, a large red pigment region and a lot of transparent particles was observed in the cells of FACHB 806. The cell size increased with the decrease of salinity from 50‰ to 5‰. The pigment region of FACHB 806 under 50‰ salinity showed more red color than that of other salinity groups, which indicated that the change of salinity might influence the content of pigment.
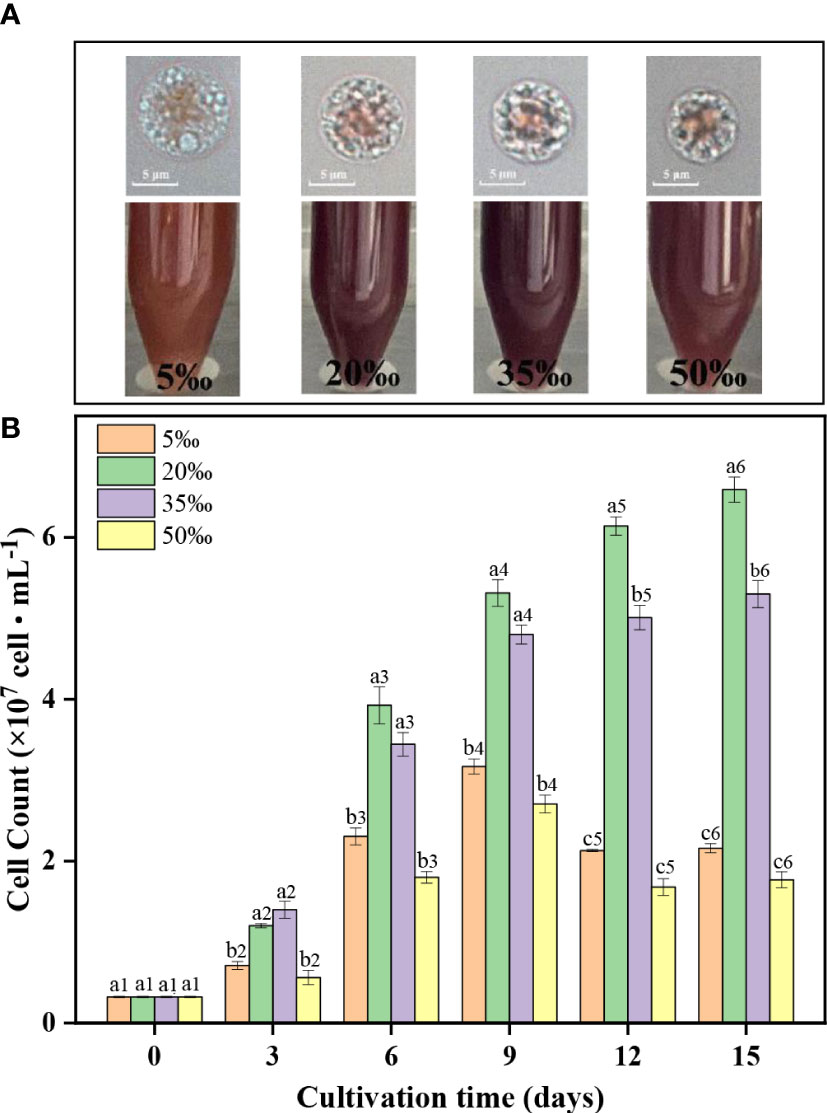
Figure 1 The cell morphology (A) and cell count (B) of Porphyridium purpureum FACHB 806 under different salinities. The values shown are the averages of two biological replicates and three technical replicates ± standard deviation. Different letters indicate significant differences (a1 – b1, day 0; a2 – b2, day 3; a3-b3, day 6; a4-b4, day 9; a5-c5, day 12; a6-c6, day 15).
As shown in Figure 1B, the cell counts of 20‰ and 35‰ salinity groups gradually increased with the culture time, but the cell counts of 5‰ and 50‰ salinity groups reached the maximum on day 9 and then began to decrease. In addition, the cell counts of the 20‰ and 35‰ salinity groups were significantly higher than that of 5‰ and 50‰ salinity groups at the same culture period. At the end of the culture time, the 20‰ salinity group obtained the highest cell density (6.6 × 107 cells·mL-1), which was 205%, 24% and 273% higher than that of the 5‰, 35‰ and 50‰ salinity groups, respectively. The above results indicated low (5‰) and high (50‰) salinity were unfavorable to the growth of FACHB 806. Among the four salinities levels, the optimal salinity condition for the growth of FACHB 806 was 20‰.
3.2 Production of EPS in P. purpureum FACHB 806
The EPS yield at different salinities were measured in Figure 2A. The EPS yield at four salinity levels increased with the prolong of culture time. At the end of the culture time, the 20‰ salinity group obtained the highest EPS yield (691.4 mg·L-1), which was 26.6%, 5.7% and 25.5% higher than that of the 5‰, 35‰ and 50‰ salinity groups, respectively. The above results indicated low (5‰) and high (50‰) salinity were unfavourable to the EPS yield of FACHB 806, and the 20‰ salinity was most beneficial to improve the production of EPS.
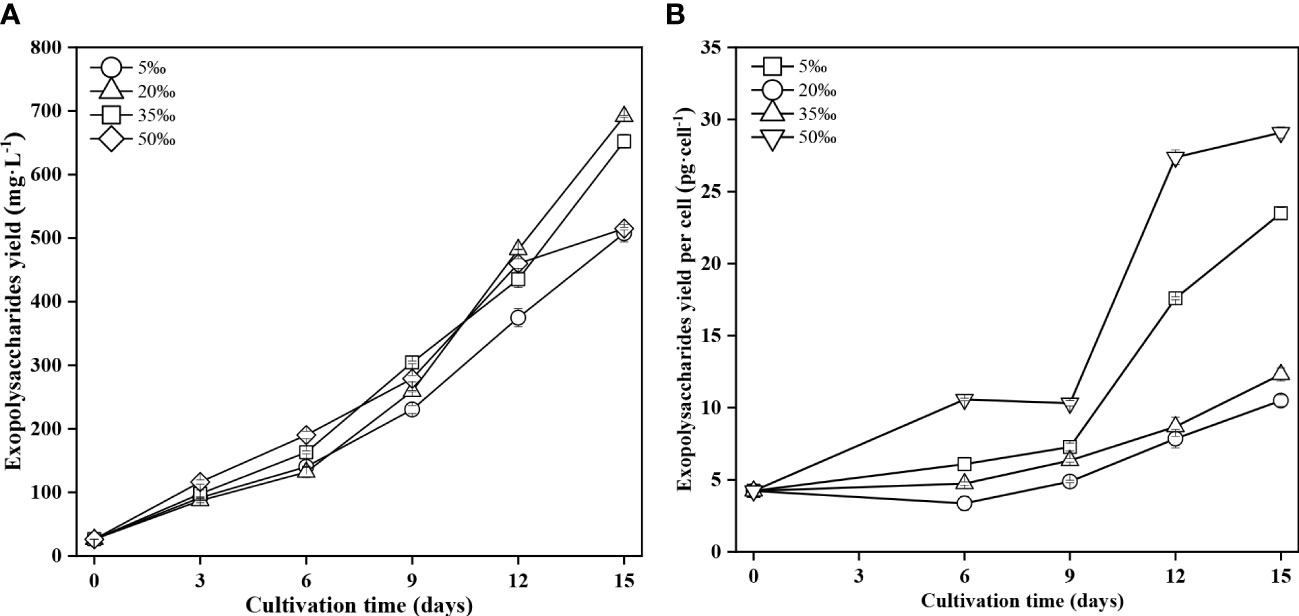
Figure 2 Exopolysaccharide yield (A) and exopolysaccharide yield per cell (B) of Porphyridium purpureum FACHB 806 under different salinities. The values shown are the averages of two biological replicates and three technical replicates ± standard deviation.
The EPS yield per cell showed an increasing trend with the culture time at all salinity levels (Figure 2B). The 5‰ and 50‰ salinity groups showed the higher EPS yield per cell than that of 20‰ and 35‰ salinity groups. At the end of the culture time, the EPS yield per cell in the 50‰ salinity reached 29.1 pg·cell-1, which was 177% and 137% higher than that of the 20‰ and 35‰ salinity groups, respectively. And the EPS yield per cell in the 5‰ salinity reached 23.5 pg·cell-1, which was 124% and 91% higher than that of the 20‰ and 35‰ salinity groups, respectively. These results indicated that low (5‰) and high (50‰) salinity could promote the synthesis of EPS in FACHB 806, and the 50‰ salinity was the most favorable for the synthesis of EPS.
3.3 Biochemical characteristics of EPS in P. purpureum FACHB 806
The FT-IR spectra of EPS at different salinities within the range of 4,000 – 400 cm-1 were measured in Figure 3. The absorption peaks at 3,324 – 3,311 cm-1 represented the stretching vibration of hydroxyl (-OH). And the weak peaks at 2,925 – 2,931 cm-1 were the stretching vibration of C-H. The absorption peak at 1,410 – 1,400 cm-1 indicated the stretching vibration of the C-O bond in C-OH. And the absorption peaks at 1,258 – 1,240 cm-1 were attributed to the asymmetrical stretching vibration of the S=O bond in the sulfate group (Wang et al., 2013). The absorption peaks at 1,096 – 1,031 cm-1 might have appeared due to the C-O stretching vibration in C-O-C (Kacurakova et al., 2000). The absorption peaks at 895 – 881 cm-1 resulted from the vibration of the β-configuration pyran ring (Choi et al., 2021). However, no C-O-S absorption peaks were observed in the 5‰ salinity. The above results implied that low salinity (5‰) could influence the chemical structure of EPS.
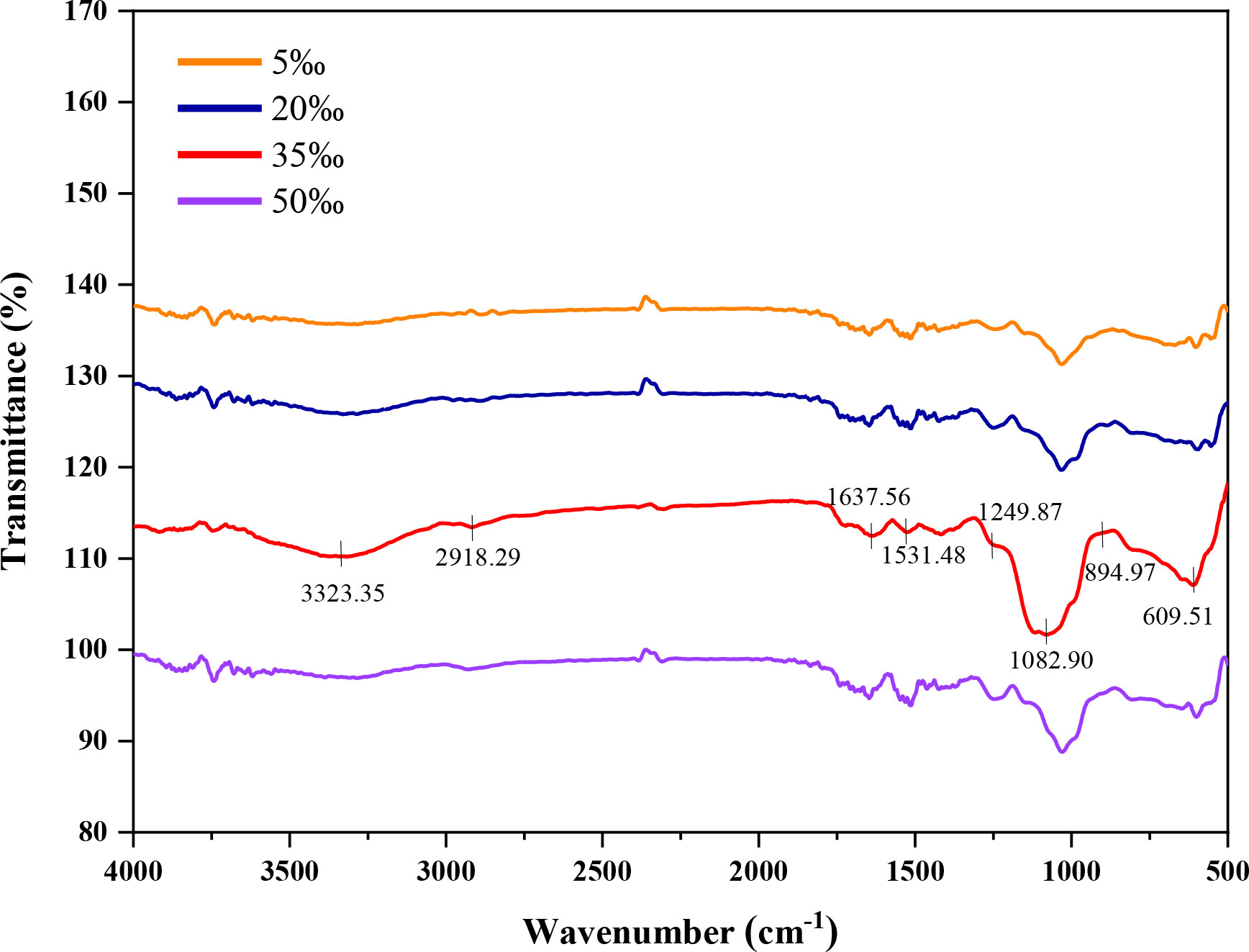
Figure 3 Fourier-transform infrared absorption spectra of Porphyridium purpureum FACHB 806 exopolysaccharide under different salinities.
The FACHB 806 EPS of four salinity levels were mainly composed of xylose, glucose and galactose, but the monosaccharide percentages were different (Table 1). The xylose percentage decreased with the increase of salinity, and the highest percentage (31.8%) was obtained in the 5‰ salinity group. The glucose percentage of EPS increased with the increase of salinity, and the 50‰ salinity group achieved the highest percentage (40.8%). However, the galactose percentage showed a decreasing trend with the increase of salinity, the maximum percentage reached 40.4% in the 5‰ salinity group. These results suggested that low salinity (5‰) could increase the percentage of galactose and xylose, but decrease the percentage of glucose.
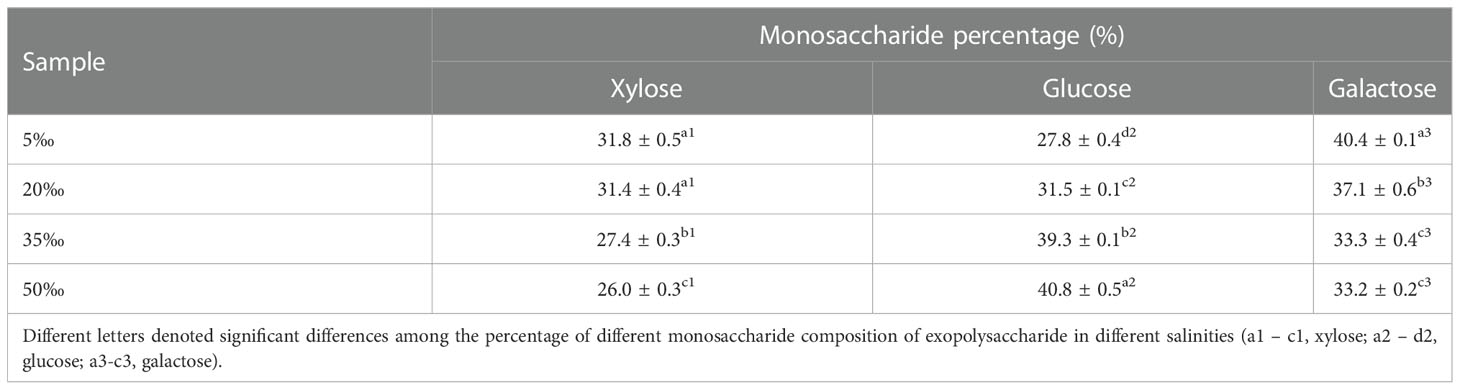
Table 1 Monosaccharide percentage of Porphyridium purpureum FACHB 806 exopolysaccharide under different salinities.
The carbohydrate, uronic acid, protein and sulfate contents of EPS were shown in Table 2. The carbohydrate and uronic acid contents decreased with the increase of salinity and the maximum contents reached 62.5% and 14.4% in the 5‰ salinity, respectively. The highest content of protein reached 13.7% in the 50‰ salinity group, and the 35‰ salinity group achieved the maximum sulfate content (14.7%). The above results indicated that the chemical compositions of EPS were influenced by salinity, the low salinity (5‰) was favorable to elevate the contents of carbohydrate and uronic acid, but high salinity (50‰) could elevate the content of protein.
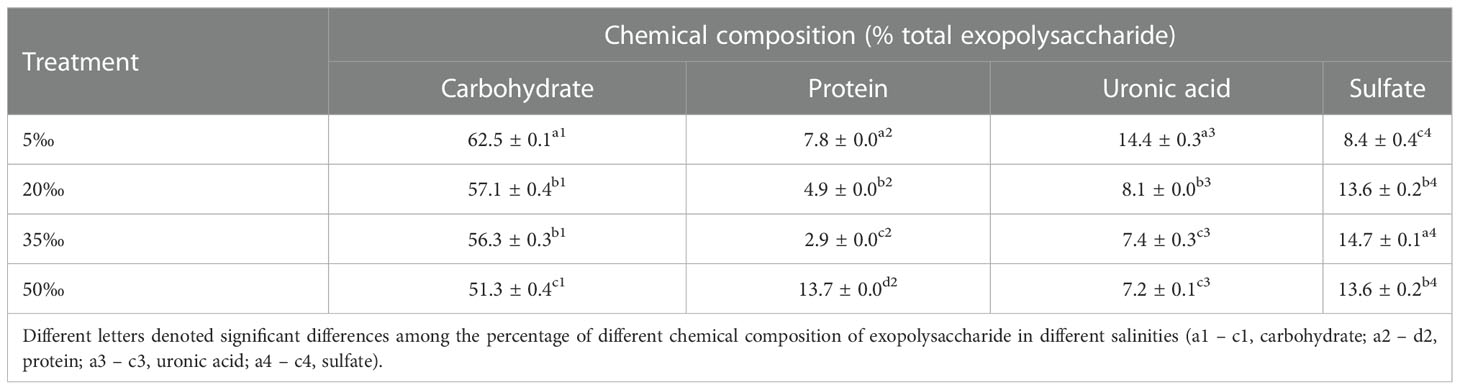
Table 2 Chemical composition of Porphyridium purpureum FACHB 806 exopolysaccharide under different salinities.
3.4 Hydroxyl radical scavenging activity of P. purpureum FACHB 806 EPS
As shown in Figure 4, the hydroxyl radical scavenging activity of FACHB 806 EPS increased in a dose-dependent manner. When the concentration was more than 0.6 mg·mL-1, the scavenging activity of the 5‰ and 50‰ salinity groups significantly higher than that of the 20‰ and 35‰ salinity groups. When the FACHB 806 EPS concentration was 1.0 mg·L-1, the 50‰ salinity obtained the maximum scavenging activity (78.4%), which was 8.4%, 30.4% and 21.4% higher than that of the 5‰, 20‰ and 35‰ salinity groups, respectively. But the scavenging activities of four salinity levels were significantly lower than those of ascorbic acid. These results suggested that low (5‰) and high salinity (50‰) could improve the hydroxyl radical scavenging activity of EPS in FACHB 806.
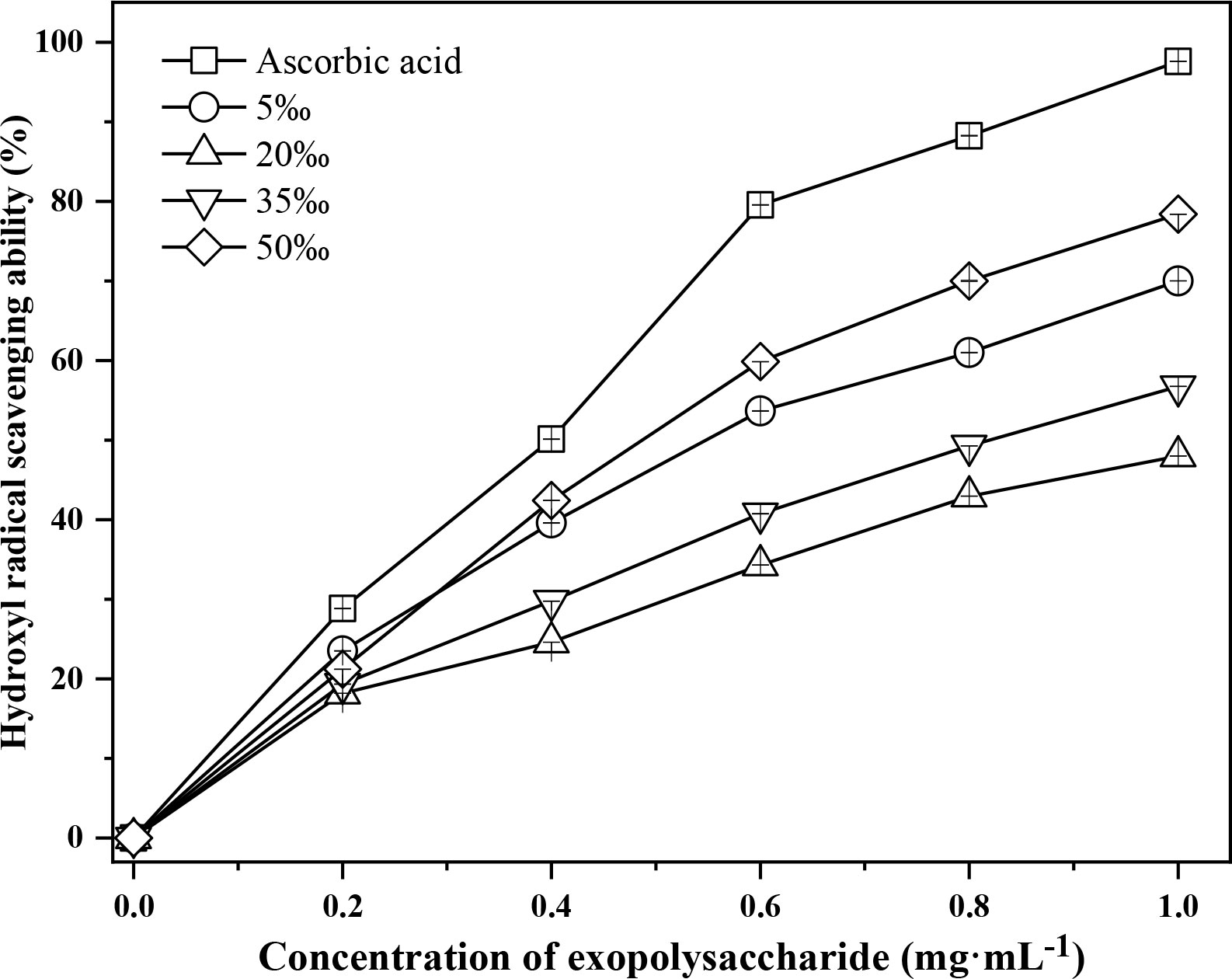
Figure 4 Hydroxyl radical scavenging activity of Porphyridium purpureum FACHB 806 exopolysaccharide under different salinities. The values shown are the averages of two biological replicates and three technical replicates ± standard deviation.
4 Discussion
The salinity stress could influence the cell morphology of microalgae, especially for the unicellular microalgae lacking cell walls (Shetty et al., 2019). Our study showed that the cell size of FACHB 806 decreased with the increase of salinity. Previous study indicated that the hyperosmotic dehydration could lead to the reduction in cell size of microalgae (e.g. Chrysotila carterae, Chaetoceros muelleri, Nannochloropsis sp., Phaeodactylum tricornutum, Tisochrysis lutea, Tetraselmis suecica, Amphora sp., Navicula sp., Dunaliella salina) at high salinity (Ishika et al., 2018). D. salina were able to change rapidly the cell size in response to salinity changes, but it could subsequently recover the original cell size by adjusting the concentration of intracellular glycerol and ions (Chen et al., 2011). The present study showed that FACHB 806 could grow normally in salinities ranging from 5‰ to 50‰ (Figure 1), which indicated that FACHB 806 had a wide range of salinity tolerance. The tolerance mechanism of salinity stress varies among microalgae. Some microalgae could change the concentration of the intracellular metabolite to improve salinity tolerance (Bazzani et al., 2021). Microalgal EPS, which was usually wrapped around the algal cells, could provide a stable microenvironment and a natural barrier to protect the cells from the unfavorable environment (Rossi and De Philippis, 2015). Our study showed that the secretion of EPS was enhanced under the low (5‰) and high salinity (50‰) conditions. Similar results have been reported in previous studies. The EPS concentration of D. salina was 16.9-fold higher at high salinity (5.0 mol L-1 NaCl) compared to low salinity (0.5 mol L-1 NaCl) (Mishra and Jha, 2009).
Our results demonstrated that the cell division of FACHB 806 was inhibited at low (5‰) and high (50‰) salinity. This result is in agreement with those of many previous studies. For instance, the growth rate of P. cruentum was 3-fold higher at optimal salinity (0.8 mol·L-1 NaCl) compared to 1.5 mol·L-1 NaCl (Lee et al., 1989). P. purpureum grew slowly under excessively low (0 ‰) or high salinity (68 ‰) conditions (Lu et al., 2020). The growth inhibition of microalgae when exposed to salinity stress might be attributed to the loss of water, ionic imbalance, reduction of photosynthesis rate and overproduction of reactive oxygen species (Martínez-Roldán et al., 2014). Furthermore, microalgae was obliged to consume a lot of energy to maintain cell turgor pressure and resist the osmotic stress under salinity stress, so their growth might be inhibited (Kirst, 1990). Salinity is one of the most important factors that can affect the EPS production of microalgae, and the synthesis of EPS was attributed to maintain the osmotic balance between the intracellular and extracellular environments (Morais et al., 2022). Previous studies have indicated that salinity stress could cause the intracellular ions imbalance and the key enzymes inactivation, thus affecting the cellular metabolism, such as carbon fixation, photosynthesis and respiration (Martínez-Roldán et al., 2014). The above physiological response ultimately affected the synthesis of compounds inside and outside the cell. The production process of EPS mainly includes the synthesis of nucleoside sugar precursors, the assembly of oligosaccharide units and secretion of polysaccharide (Pereira et al., 2009). Moreover, the enzymes involved in the cell wall polysaccharide and lipopolysaccharide formation might be related to the EPS synthesis (Mozzi et al., 2003). For example, the down-regulated level of sulfate-adenylyl transferase gene decreased the synthesis of Haloferax mediterranei EPS under salinity stress, which indicated that the gene expression level of sulfate-adenylyl transferase might be connected with the synthesis of EPS (Pacholak et al., 2021). The result demonstrated that salinity stress could regulate the synthesis of EPS by affecting the expression level of related enzyme genes in the assembly pathway of oligosaccharide units. Moreover, low salinity could induce the over-expression level of the sulfate-adenylyl transferase in Kappaphycus alvarezii (Liu et al., 2011). The present study showed that the highest yield of FACHB 806 EPS was obtained in 20‰ salinity, which inferred that the salinity stress might affect the synthesis of EPS by changing the expression level of gene, such as the sulfate-adenylyl transferase. However, the confirmation of speculation still need to further study in the future. Microalgae EPS have specific structural, physicochemical characteristics and different biological activities, which indicated that it had the potential application in various field such as food, cosmetic and biomaterials (Moreira, 2022). This study demonstrated that the 20‰ salinity not only increased the growth of FACHB 806, but also promoted the yield of EPS. Thus, the 20‰ salinity might be an appropriate salinity in the large-scale culture of P. purpureum FACHB 806.
The monosaccharide composition analysis of EPS is the most important step for the further discovery of its physicochemical properties, structure and structure-bioactivity relationship (Xie et al., 2013). Previous studies have revealed that the monosaccharide composition could affect the physicochemical properties of EPS (Xiao and Zheng, 2016). For example, the presence of rhamnose and fucose in the EPS of diatom could change the adhesion and flocculation of cells (Willis et al., 2013). Moreover, the culture conditions also markedly affected the monosaccharide composition of microalgal EPS (Xiao and Zheng, 2016). Four monosaccharides of EPS from D. salina were dramatically increased at 3.0 mol·L-1 NaCl (Mishra and Jha, 2009). Furthermore, when three stains of Synechocystis were exposed to 0.2 mol·L-1 NaCl, the percentage of glucose in EPS was increased in Synechocystis sp. BASO507 and BASO511, and the percentage of xylose was increased in Synechocystis sp. BASO444 (Ozturk and Aslim, 2010). Our study showed that salinity also affected the percentage of monosaccharides of EPS in FACHB 806, with low salinity (5‰) causing the highest percentage of galactose (41.1%) and high salinity (50‰) leading to highest percentage of glucose (40.8%). Thus, the change in the percentage of monosaccharides might be an adaptive behavior of FACHB 806 to salinity stress.
The antioxidant activity of EPS depends on its structure, including molecular weight, functional groups and monosaccharide composition (Zhong et al., 2019). The antioxidant activity of FACHB 806 EPS was mainly reflected in the scavenging capacity of hydroxyl radicals (Wang et al., 2021). Our study showed that the scavenging capacity of hydroxyl radicals of the 5‰ and 50‰ salinity groups higher than other groups (Table 3). Hydroxyl radicals are the most harmful reactive oxygen species, which are mainly responsible for the oxidative damage of biomolecules (Erel, 2004). Uronic acid and sulfate in EPS could inhibit the generation of hydroxyl radicals by chelating Fe2+ and Cu2+ (Qi et al., 2006). Exopolysaccharides THPS-2 from Tetragenococcus halophilus with high sulfate and uronic acid contents had stronger hydroxyl radical scavenging ability than THSP-1 (Yang et al., 2022). Therefore, EPS with high sulfate and uronic acid contents often exhibit stronger hydroxyl radical scavenging ability (Zhong et al., 2019). In our study, the 5‰ salinity group had the high uronic acid and sulfate contents of EPS, which might chelate more metal ions and improve its hydroxyl radical scavenging activity. As a result, it was inferred that the uronic and sulfate might play an important role in the hydroxyl radical scavenging activity of FACHB 806 EPS. The presence of protein could also affect the antioxidant activity of EPS. Some specific amino acids have a quenching effect on free radicals and could also reduce the accumulation of free radicals by providing electrons (Andrew and Jayaraman, 2020). The EPS of Inonotus obliquus with the protein content of 23.5% had higher hydroxyl radicals scavenging activity than its EPS with the protein content of 13.3% (Xiang et al., 2012). Our study indicated the 50‰ salinity group had highest content of protein and scavenging activity compared to other groups (Table 3). The antioxidant activity of EPS were also affected by the composition of monosaccharides (Andrew and Jayaraman, 2020). In our study, the 50‰ salinity group had highest percentage of glucose and hydroxyl radical scavenging ability. The antioxidant activity of Hirsutella sp. EPS showed significant correlation with mannose and glucose content, but the galactose content was not correlated with antioxidant activity (Meng et al., 2015). It was speculated that the chemical structure derived from glucose might play an important role in the hydroxyl radical scavenging activity of FACHB 806 EPS. Furthermore, the change of monosaccharides composition indicated the variation of the primary structures of EPS, which determined the spatial structure of EPS. In fact, the change of the monosaccharide percentage could cause the alteration of spatial arrangement of polysaccharide, which led to the exposure of polysaccharide hydroxyl groups and ultimately improved the chelate Fe2+ and Cu2+ activity of polysaccharide (Gulcin, 2006; Wang et al., 2016). The increase of chelate metal activity of polysaccharides represented the improvement of antioxidant activity (Shen et al., 2018). In the case of changes in the spatial structure of EPS, some the active groups encapsulated within EPS might be re-exposed to the surface of EPS molecules, or some the active groups outside EPS might be re-encapsulated inside the EPS macromolecule. This might be a possible reason for the influence of monosaccharide composition on the antioxidant activity of EPS.

Table 3 IC50 based on hydroxyl radical scavenging activity of Porphyridium purpureum exopolysaccharide under different salinities.
5 Conclusion
The low (5‰) and high salinity (50‰) were unfavorable to the growth of P. purpureum FACHB 806, but could promote the synthesis of EPS. The EPS of FACHB 806 had pyranose rings with a glycosidic bond type of β-configuration, and the monosaccharide composition of EPS included xylose, glucose and galactose. Not only the change of salinity could alter the contents of uronic acid, sulfate and protein of EPS, but also could influence the percentage of monosaccharides. Moreover, low (5‰) and high salinity (50‰) could improve to the hydroxyl radical scavenging activity of EPS. The hydroxyl radical scavenging activity of EPS might be relevant to the contents of protein, uronic acid, sulfate and the percentage of monosaccharides.
Data availability statement
The raw data supporting the conclusions of this article will be made available by the authors, without undue reservation.
Author contributions
QL: Conceptualization, Data curation, Formal analysis, Investigation, Methodology, Writing – original draft. YC and XL: Data curation, Formal analysis, Investigation. YL: Conceptualization, Data curation, Formal analysis, Investigation, Methodology. JX: Conceptualization, Funding acquisition, Supervision, Resources, Writing – review and editing. TL and WX: Data curation, Formal analysis, Writing – review and editing. AL: Conceptualization, Funding acquisition, Project administration, Resources, Supervision, Writing – review and editing. All authors contributed to the article and approved the submitted version.
Funding
This work was supported by Key Laboratory of Tropical Marine Bio-resources and Ecology, Chinese Academy of Sciences (LMB20211006) and Engineering Research Center for Tropical and Subtropical Aquatic Ecological Engineering, Ministry of Education, Jinan University, P. R. China (No. 2021B0402).
Conflict of interest
The authors declare that the research was conducted in the absence of any commercial or financial relationships that could be construed as a potential conflict of interest.
Publisher’s note
All claims expressed in this article are solely those of the authors and do not necessarily represent those of their affiliated organizations, or those of the publisher, the editors and the reviewers. Any product that may be evaluated in this article, or claim that may be made by its manufacturer, is not guaranteed or endorsed by the publisher.
References
Andrew M., Jayaraman G. (2020). Structural features of microbial exopolysaccharides in relation to their antioxidant activity. Carbohyr. Res. 487, 107881. doi: 10.1016/j.carres.2019.107881
Babiak W., Krzemińska I. (2021). Extracellular polymeric substances (EPS) as microalgal bioproducts: A review of factors affecting EPS synthesis and application in flocculation processes. Energies 14 (13), 4007. doi: 10.3390/en14134007
Bazzani E., Lauritano C., Mangoni O., Bolinesi F., Saggiomo M. (2021). Chlamydomonas responses to salinity stress and possible biotechnological exploitation. J. Mar. Sci. 9 (11), 1242. doi: 10.3390/jmse9111242
Blumenkrantz N., Asboe-Hansen G. (1973). New method for quantitative determination of uronic acids. Anal. Biochem. 54, 484–489. doi: 10.1016/0003-2697(73)90377-1
Chen H., Lao Y. M., Jiang J. G. (2011). Effects of salinities on the gene expression of a (NAD+)-dependent glycerol-3-phosphate dehydrogenase in Dunaliella salina. Sci. Total Environ. 409 (7), 1291–1297. doi: 10.1016/j.scitotenv.2010.12.038
Chen B. L., You W. L., Huang J., Yu Y., Chen W. P. (2010). Isolation and antioxidant property of the extracellular polysaccharide from Rhodella reticulata. World J. Microbi. Biot. 26 (5), 833–840. doi: 10.1007/s11274-009-0240-y
Choi I. S., Ko S. H., Lee M. E., Kim H. M., Yang J. E., Jeong S. G., et al. (2021). Production, characterization, and antioxidant activities of an exopolysaccharide extracted from spent media wastewater after Leuconostoc mesenteroides WiKim32 fermentation. ACS Omega 6 (12), 8171–8178. doi: 10.1021/acsomega.0c06095
Dubois M., Gilles K. A., Hamilton J. K., Rebers P. T., Smith F. (1956). Colorimetric method for determination of sugars and related substances. Anal. Chem. 28 (3), 350–356. doi: 10.1021/ac60111a017
Emad A. S., Sanaa M. M. S., Vikramjit S. (2010). Salt stress enhancement of antioxidant and antiviral efficiency of Spirulina platensis. J. Med. Plants Res. 4 (24), 2622–2632. doi: 10.5897/jmpr09.300
Erel O. (2004). A novel automated method to measure total antioxidant response against potent free radical reactions. Clin. Biochem. 37 (2), 112–119. doi: 10.1016/j.clinbiochem.2003.10.014
Geresh S., Lupescu N., Arad S. M. (1992). Fractionation and partial characterization of the sulphated polysaccharide of Porphyridium. Phytochemistry 32 (12), 4181–4186. doi: 10.1016/0031-9422(92)80439-L
Gulcin I. (2006). Antioxidant and antiradical activities of l-carnitine. Life Sci. 78 (8), 803–811. doi: 10.1016/j.lfs.2005.05.103
Ishika T., Bahri P. A., Laird D. W., Moheimani N. R. (2018). The effect of gradual increase in salinity on the biomass productivity and biochemical composition of several marine, halotolerant, and halophilic microalgae. J. Appl. Phycol. 30 (3), 1453–1464. doi: 10.1007/s10811-017-1377-y
Kacurakova M., Capek P., Sasinkova V., Wellne N., Ebringerova A. (2000). FT-IR study of plant cell wall model compounds: Pectic polysaccharides and hemicelluloses. Carbohyd. Polym. 43 (2), 195–203. doi: 10.1016/S0144-8617(00)00151-X
Kirst G. (1990). Salinity tolerance of eukaryotic marine algae. Annu. Rev. Plant Biolo. 41, 21–53. doi: 10.1146/annurev.pp.41.060190.000321
Lee Y. K., Tan H. M., Low C. S. (1989). Effect of salinity of medium on cellular fatty acid composition of marine alga Porphyridium cruentum (Rhodophyceae). J. Appl. Phycol. 1, 19–23. doi: 10.1007/BF00003531.
Li Y., Jiang B., Zhang T., Mu W., Liu J. (2008). Antioxidant and free radical-scavenging activities of chickpea protein hydrolysate (CPH). Food Chem. 106 (2), 444–450. doi: 10.1016/j.foodchem.2007.04.067
Li S. H., Ji L., Shi Q. W., Wu H. Z., Fan J. H. (2019a). Advances in the production of bioactive substances from marine unicellular microalgae Porphyridium spp. Bioresour. Technol. 292, 122048. doi: 10.1016/j.biortech.2019.122048
Li T., Xu J., Wu H. B., Jiang P. L., Chen Z. S., Xiang W. Z., et al. (2019b). Growth and biochemical composition of Porphyridium purpureum SCS-02 under different nitrogen concentrations. Mar. Drugs 17 (2), 124. doi: 10.3390/md17020124
Liu L., Pohnert G., Wei D. (2016). Extracellular metabolites from industrial microalgae and their biotechnological potential. Mar. Drugs 14 (10), 191. doi: 10.3390/md14100191
Liu C. L., Wang X. L., Huang X. H., Liu J. G. (2011). Identification of hypo-osmotically induced genes in Kappaphycus alvarezii (Solieriaceae, rhodophyta) through expressed sequence tag analysis. Bot. Mar. 54 (6), 557–562. doi: 10.1515/bot.2011.067
Lowry O., Rosebrough N., Farr A. L., Randall R. (1951). Protein measurement with the folin phenol reagent. J. Bio. Chem. 193 (1), 265–275. doi: 10.1016/s0021-9258(19)52451-6
Lu X. D., Nan F. R., Feng J., Lv J. P., Liu Q., Liu X. D., et al. (2020). Effects of different environmental factors on the growth and bioactive substance accumulation of Porphyridium purpureum. Int. J. Environ. Res. Public Health 17 (7), 2221. doi: 10.3390/ijerph17072221
Lu I. F., Sung M. S., Lee T. M. (2006). Salinity stress and hydrogen peroxide regulation of antioxidant defense system in Ulva fasciata. Mar. Biol. 150 (1), 1–15. doi: 10.1007/s00227-006-0323-3
Luo J. G., Liu J., Sun Y., Ye H., Zhou C. H., Zeng X. X. (2010). Medium optimization, preliminary characterization and antioxidant activity in vivo of mycelial polysaccharide from Phellinus baumii pilát. Carbohyd. Polym. 81 (3), 533–540. doi: 10.1007/s00227-006-0323-3
Martínez-Roldán A. J., Perales-Vela H. V., Cañizares-Villanueva R. O., Torzillo G. (2014). Physiological response of Nannochloropsis sp. to saline stress in laboratory batch cultures. J. Appl. Phycol. 26 (1), 115–121. doi: 10.1007/s10811-013-0060-1
Meng L., Sun S. S., Li R., Shen Z. P., Wang P., Jiang X. L. (2015). Antioxidant activity of polysaccharides produced by Hirsutella sp. and relation with their chemical characteristics. Carbohyd. Polym. 117, 452–457. doi: 10.1016/j.carbpol.2014.09.076
Mishra A., Jha B. (2009). Isolation and characterization of extracellular polymeric substances from micro-algae Dunaliella salina under salt stress. Bioresour. Technol. 100 (13), 3382–3386. doi: 10.1016/j.biortech.2009.02.006
Morais M. G., Santos T. D., Moraes L., Vaz B. S., Morais E. G., Costa J. A. V. (2022). Exopolysaccharides from microalgae: Production in a biorefinery framework and potential applications. Bioresour. Technol. Rep. 18, 101006. doi: 10.1016/j.biteb.2022.101006
Moreira J. B., Kuntzler S. G., Bezerra P.Q. M., Cassuriaga A. P. A., Zaparoli M., da Silva J. L. V., et al (2022). Recent advances of microalgae exopolysaccharides for application as bioflocculants. Polysaccharides 3 (1), 264–276. doi: 10.3390/polysaccharides3010015.
Mozzi F., Savoy de Giori G., Font de Valdez G. (2003). UDP-Galactose 4-epimerase: a key enzyme in exopolysaccharide formation by Lactobacillus casei CRL 87 in controlled pH batch cultures. J. Appl. Microbiol. 94 (2), 175–183. doi: 10.1046/j.1365-2672.2003.01821.x
Ozturk S., Aslim B. (2010). Modification of exopolysaccharide composition and production by three cyanobacterial isolates under salt stress. Environ. Sci. pollut. Res. 17 (3), 595–602. doi: 10.1007/s11356-009-0233-2
Pacholak A., Gao Z. L., Gong X. Y., Kaczorek E., Cui Y. W. (2021). The metabolic pathways of polyhydroxyalkanoates and exopolysaccharides synthesized by Haloferax mediterranei in response to elevated salinity. J. Proteomics 232, 104065. doi: 10.1016/j.jprot.2020.104065
Pereira S., Zille A., Micheletti E., Moradas-Ferreira P., De Philippis R., Tamagnini P. (2009). Complexity of cyanobacterial exopolysaccharides: Composition, structures, inducing factors and putative genes involved in their biosynthesis and assembly. FEMS Microbiol. Rev. 33 (5), 917–941. doi: 10.1111/j.1574-6976.2009.00183.x
Qi H. M., Zhang Q. B., Zhao T. T., Hu R. H., Zhang K., Li Z. E. (2006). In vitro antioxidant activity of acetylated and benzoylated derivatives of polysaccharide extracted from Ulva pertusa (Chlorophyta). Bioorg. Med. Chem. Lett. 16 (9), 2441–2445. doi: 10.1016/j.bmcl.2006.01.076
Raposo M. F., de Morais A. M., de Morais R. M. (2014). Influence of sulphate on the composition and antibacterial and antiviral properties of the exopolysaccharide from Porphyridium cruentum. Life Sci. 101 (1-2), 56–63. doi: 10.1016/j.lfs.2014.02.013
Reim R. E. (1991). Total sulfite in cellulosics by ion exclusion chromatography with electrochemical detection. J. Food Sci. 56, 1079–1090. doi: 10.1111/j.1365-2621.1991.tb14646.x
Rossi F., De Philippis R. (2015). Role of cyanobacterial exopolysaccharides in phototrophic biofilms and in complex microbial mats. Life 5 (2), 1218–1238. doi: 10.3390/life5021218
Shen S. G., Jia S. R., Wu Y. K., Yan R. R., Lin Y. H., Zhao D. X., et al. (2018). Effect of culture conditions on the physicochemical properties and antioxidant activities of polysaccharides from Nostoc flagelliforme. Carb. Polym 198, 426–433. doi: 10.1016/j.carbpol.2018.06.111
Shetty P., Gitau M. M., Maroti G. (2019). Salinity stress responses and adaptation mechanisms in eukaryotic green microalgae. Cells 8 (12), 1657. doi: 10.3390/cells8121657
Sun Y. Y., Wang H., Guo G. L., Pu Y. F., Yan B. L. (2014). The isolation and antioxidant activity of polysaccharides from the marine microalgae Isochrysis galbana. Carbohyd. Polym. 113, 22–31. doi: 10.1016/j.carbpol.2014.06.058
Sun L. Q., Wang C. H., Shi Q. J., Ma C. H. (2009). Preparation of different molecular weight polysaccharides from Porphyridium cruentum and their antioxidant activities. Int. J. Biol. Macromol. 45 (1), 42–47. doi: 10.1016/j.ijbiomac.2009.03.013
Tannin-Spitz T., Bergman M., Van-Moppes D., Grossman S., Arad S. (2005). Antioxidant activity of the polysaccharide of the red microalga Porphyridium sp. J. Appl. Phycol. 17 (3), 215–222. doi: 10.1007/s10811-005-0679-7
Wang J., Hu S., Nie S., Yu Q., Xie M. (2016). Reviews on mechanisms of In vitro antioxidant activity of polysaccharides. Oxid. Med. Cell Longev 2016, 5692852. doi: 10.1155/2016/5692852
Wang W. N., Li Y., Zhang Y., Xiang W. Z., Li A. F., Li T. (2021). Comparison on characterization and antioxidant activity of exopolysaccharides from two Porphyridium strains. J. Appl. Phycol. 33 (5), 2983–2994. doi: 10.1007/s10811-021-02518-9
Wang N., Qian Z. X., Luo M. W., Fan S. J., Zhang X. J., Zhang L. Y. (2018). Identification of salt stress responding genes using transcriptome analysis in green alga Chlamydomonas reinhardtii. Int. J. Mol. Sci. 19 (11), 3359. doi: 10.3390/ijms19113359
Wang X., Zhang Z., Yao Q., Zhao M., Qi H. (2013). Phosphorylation of low-molecular-weight polysaccharide from enteromorpha linza with antioxidant activity. Carb. Polym 96 (2), 371–375. doi: 10.1016/j.carbpol.2013.04.029
Willis A., Chiovitti A., Dugdale T. M., Wetherbee R. (2013). Characterization of the extracellular matrix of Phaeodactylum tricornutum (Bacillariophyceae): structure, composition, and adhesive characteristics. J. Phycol. 49 (5), 937–949. doi: 10.1111/jpy.12103
Xiang Y. L., Xu X. Q., Li J. (2012). Chemical properties and antioxidant activity of exopolysaccharides fractions from mycelial culture of Inonotus obliquus in a ground corn stover medium. Food Chem. 134 (4), 1899–1905. doi: 10.1016/j.foodchem.2012.03.121
Xiao R., Zheng Y. (2016). Overview of microalgal extracellular polymeric substances (EPS) and their applications. Biotechnol. Adv. 34 (7), 1225–1244. doi: 10.1016/j.biotechadv.2016.08.004
Xie J. H., Shen M. Y., Nie S. P., Liu X., Zhang H., Xie M. Y. (2013). Analysis of monosaccharide composition of cyclocarya paliurus polysaccharide with anion exchange chromatography. Carb. Polym 98 (1), 976–981. doi: 10.1016/j.carbpol.2013.07.011
Yang X. M., Wu J. R., An F. Y., Xu J. W., Bat-Ochir M., Wei L. L., et al. (2022). Structure characterization, antioxidant and emulsifying capacities of exopolysaccharide derived from Tetragenococcus halophilus SNTH-8. Int. J. Biol. Macromol. 208, 288–298. doi: 10.1016/j.ijbiomac.2022.02.186
Zhong Q. W., Wei B., Wang S. J., Ke S. Z., Chen J. W., Zhang H. W., et al. (2019). The antioxidant activity of polysaccharides derived from marine organisms: An overview. Mar. Drugs 17 (12), 674. doi: 10.3390/md17120674
Keywords: Porphyridium, salinity, exopolysaccharide, biochemical composition, antioxidant activity
Citation: Li Q, Chen Y, Liu X, Li Y, Xu J, Li T, Xiang W and Li A (2023) Effect of salinity on the biochemical characteristics and antioxidant activity of exopolysaccharide of Porphyridium purpureum FACHB 806. Front. Mar. Sci. 9:1097200. doi: 10.3389/fmars.2022.1097200
Received: 13 November 2022; Accepted: 16 December 2022;
Published: 06 January 2023.
Edited by:
Zhongshan Zhang, Huzhou University, ChinaReviewed by:
Wenjun Li, Yantai Institute of Coastal Zone Research (CAS), ChinaMeilin He, Nanjing Agricultural University, China
Qunju Hu, Zhejiang Ocean University, China
Copyright © 2023 Li, Chen, Liu, Li, Xu, Li, Xiang and Li. This is an open-access article distributed under the terms of the Creative Commons Attribution License (CC BY). The use, distribution or reproduction in other forums is permitted, provided the original author(s) and the copyright owner(s) are credited and that the original publication in this journal is cited, in accordance with accepted academic practice. No use, distribution or reproduction is permitted which does not comply with these terms.
*Correspondence: Aifen Li, dGlnZXJAam51LmVkdS5jbg==; Jin Xu, eHVqaW5AbXMuZ2llYy5hYy5jbg==