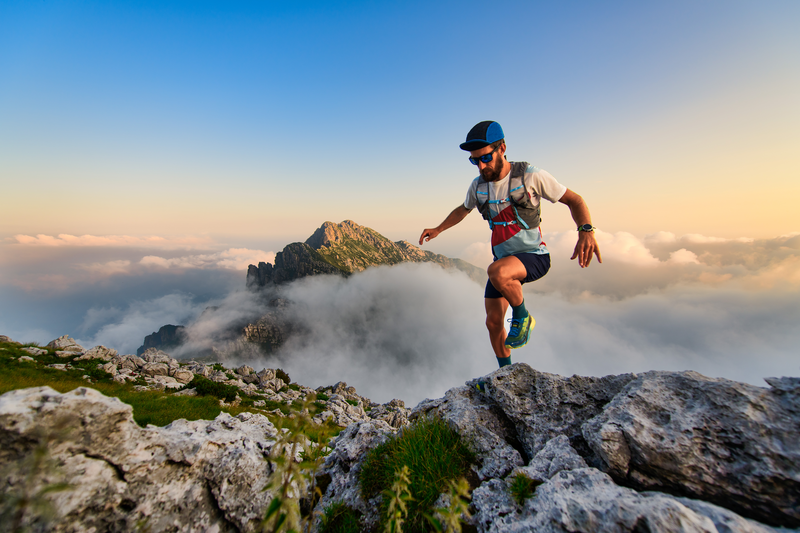
95% of researchers rate our articles as excellent or good
Learn more about the work of our research integrity team to safeguard the quality of each article we publish.
Find out more
ORIGINAL RESEARCH article
Front. Mar. Sci. , 23 December 2022
Sec. Marine Molecular Biology and Ecology
Volume 9 - 2022 | https://doi.org/10.3389/fmars.2022.1093176
Bacterial infection triggers apoptosis and immunity by regulating the expression of caspases. In this article, we report two caspase-8 paralogs, termed ToCas8 and ToCas8-like, from an economically important teleost golden pompano (Trachinotus ovatus). Caspase-8 is a classic cysteine protease that is essential for the initiation and execution of apoptosis and is a critical regulator in initiating death receptor-induced programmed cell death. The two proteins encoded by ToCas8 and ToCas8-like were characterized by multiple sequence alignment and protein structure analysis showing similarity to their counterparts in vertebrates and exhibiting typical features of the Caspase-8 protein family. Gene synteny analysis further confirmed that two isoforms of caspase-8 genes exactly existed in golden pompano. Phylogenetic analysis showed that the Caspase-8 proteins of the selected species in the study were clustered together, and Cas8 and Cas8-like were clustered into two different subgroups. In addition, ToCas8 and ToCas8-like were widely distributed and both were expressed at high levels in gill, kidney and intestinal tissues. Transcript levels of ToCas8 and ToCas8-like were significantly upregulated in response to LPS and Poly(I:C) challenges, suggesting that caspase-8 genes are involved in the immune response to pathogens. In summary, we have identified two isoforms of caspase-8 in golden pompano that are involved in the immune response. These findings will expand our understanding of the evolutionary history and functional characteristics of caspase-8 genes in vertebrates.
Apoptosis is one kind of programmed cell death that is regulated by Caspases and is essential for the development of the organism (D’Arcy, 2019). Caspases are an evolutionarily conserved family of cysteine-dependent endonucleases that hydrolyze their substrates after specific aspartic acid residues and play a key role in programmed cell death and inflammation (Spead et al., 2018). Caspases are expressed in both immune and non-immune cells, and the majority of Caspases are constitutively expressed during homeostasis (Kesavardhana et al., 2020). The Caspase enzyme is centrally involved in cell death and inflammation, and thus its related signaling pathway has been considered a tempting target for therapeutic intervention. Depending on their functional characteristics, the Caspase family can be divided into inflammatory Caspases (1, 4, 5, and 13) and apoptotic Caspases (2, 3, and 6-10) (Spead et al., 2018). Apoptotic caspases are divided into initiating caspases (2 and 8-10) and executive caspases (3, 6 and 7) according to the order in which they enter the apoptotic pathway (Bi et al., 2018). The N-terminal regions of initiating Caspases contain Caspase recruitment domains (CARD) or death effector domains (DED), which are required for the recruitment and activation of substrate proteins. Executing Caspases lacks an extended N-terminal pro-domain and requires cleavage by initiating Caspases for activation (Ramirez and Salvesen, 2018).
Caspase-8, a classic cysteine protease originally identified as a central player in programmed cell death triggered by death receptor stimulation, is involved in the signaling of cell death by TNF/nerve growth factor family receptors (Orning and Lien, 2021). Effective Caspases are dimeric enzymes that can be activated by cleaving their large and small subunits. Caspase-8, belonging to the initiating apoptotic type, possesses the capacity to cleave zymogens and thereby activate them (Gitlin et al., 2020). Caspase-8 can be self-cleaved and then converted to the active form, which can be released to the cell membrane to cleave the downstream effective Caspases (Kominami et al., 2012). Caspase-8 activity is regulated by FLICE-like inhibitory protein (FLIP) and Src-dependent phosphorylation at the site of Tyr380 (Fianco et al., 2018). Previous studies revealed that sustained hepatocyte apoptosis is a common determinant of hepatocellular carcinoma development in mammalian modes (Cao et al., 2016). Further studies have shown that Caspase-8 induces apoptosis and DNA damage in hepatocytes, and plays important role in protecting against hepatocarcinogenesis in mammals (Boege et al., 2017).
Golden pompano (Trachinotus ovatus) is widely aquacultured for its rapid growth speed, high disease resistance, and superior nutritional value, and has become one of the essential marine economic fish (Zhu et al., 2019; Da et al., 2022). In this study, we identified two paralogs of caspase-8 in golden pompano using the same method as in previous studies (Da et al., 2022). Subsequently, we confirmed these paralogs exactly existed in golden pompano with bioinformatic analysis and experimental proofs. Meanwhile, the transcription patterns of the two caspase-8 isoforms were detected. Finally, we also examined the changes in mRNA expression levels of ToCas8-like and ToCas8 after LPS and Poly(I:C) stimulation.
Golden pompano (body weight: 400 ± 50 g; n= 120) were purchased from Zhanjiang commercial fish farm. Healthy fish were maintained at 28°C in tanks with the recirculating seawater for two weeks before the experiment. The fish were fed twice daily with a commercial diet. The dissolved oxygen was monitored during the experiment. Three healthy individuals were anesthetized by MS222 (Sigma, USA) and dissected. Different tissues including the kidney, liver, stomach, spleen, intestine, brain, skin, gill, and muscle were collected and stored in liquid nitrogen for RNA extraction.
Polyinosinic:polycytidylic acid (Poly(I:C)) is a double-stranded RNA that mimics the action of viral RNA and is a common immunostimulant used in experiments. Lipopolysaccharide (LPS) is a basic component of the cell wall of Gram-negative bacteria. Poly(I:C) and LPS are model molecular models for simulating viral and bacterial infections, respectively. This study contained three groups: the control group, the poly(I:C), and the LPS infection groups respectively. The control group experiment was performed by injecting 100 μL of phosphate-buffered saline (PBS). The poly(I:C) stimulation group was performed by injecting 100 μL of poly(I:C) (Sigma, USA) that dissolved in PBS (poly(I:C) concentration of 1 mg/mL). Similarly, the LPS stimulation group experiment was performed by injecting 100 μL of LPS (Sigma, USA) that dissolved in PBS (LPS concentration of 0.2 mg/mL). To analyze changes in ToCas8 and ToCas8-like expression after pathogen stimulation, three fish were randomly selected at different time points after intraperitoneal injection, anesthetized with MS222 (Sigma, USA), and tissues (gill, kidney, and intestine) were surgically sampled and stored in liquid nitrogen.
Total RNA was isolated from the sampled tissues using Trizol reagent (TansGen Biotech, China). Total RNA concentrations were measured and the quality was assessed by 1.5% agarose gel electrophoresis, and then 1 µg of the total RNA was reversely transcribed to cDNA by using EasyScript All-in-One First-Strand cDNA Synthesis SuperMix for qPCR reagent Kit (TansGen Biotech, China).
To clone the full-length cDNA of ToCas8 and ToCas8-like, the primers were designed according to the predicted caspase-8 sequences based on genomic data of T. ovatus (NCBI ID: GCA_900607315.1). Amplification was performed by initial denaturation at 98° C for 2 min, followed by denaturation at 98° C for 30 s, annealing at 55° C for 30 s, extension at 72° C for 1 min, and finally a final extension at 72° C for 10 min. PCR products were then sequenced. All primers used for amplification are listed in Supplementary Table 1.
After sequencing to verify the correctness of the ToCas8 and ToCas8-like sequences. The deduced protein structures of the two ToCas8s were predicted using the Conserved Domain Architecture Retrieval Tool (https://www.ncbi.nlm.nih.gov/Structure/cdd/wrpsb.cgi). Meanwhile, the ClustalW multiple alignment program (http://www.ebi.ac.uk/clustalw/) was used for multiple alignments. Finally, the two paralogs were submitted to the NCBI database. Genetic synteny of caspase-8 genes was conducted to validate these caspase-8 paralogs are exactly existed in the golden pompano. Phylogenetic analysis was performed using MEGA 7.0 program based on the amino acid sequence alignment, with bootstrap test of 1000 replicates. Caspase protein sequences of representative species were downloaded from the NCBI (https://www.ncbi.nlm.nih.gov/) or Ensembl (http://asia.ensembl.org/index.html) databases (Supplementary Table 2).
The β-actin gene was selected as an internal reference gene for tissue expression and temporal expression profiles in golden pompano. Real-time PCR analysis was performed using TB Green® Premix Ex Taq™ II (Takara, China). The reaction system was 10 μL of TB GreenPremix Ex TaqII, 1 μL of cDNA template, 0.5 μL of forward and reverse primers, and 8 μL of ddH2O, and the amplification procedure was performed on a Light Cycler Real-Time system (Roche, Switzerland). The cycling protocol for qRT-PCR was an initial start stage at 95°C for 30 s, followed by 40 cycles at 95°C for 5 s, 55°C for 30 s, and an elongation stage at 72°C for 30 s. The melt curve analysis of PCR products was verified for the target genes. Each experiment was repeated three times. The relative mRNA expression level was calculated by the 2−ΔΔCT method (Da et al., 2022).
The data of qRT-PCR results are presented as mean ± standard error (SEM). One-way analysis of variance (ANOVA) was performed by using SPSS 21.0 software (Chicago, IL, USA). GraphPad Prism 5.0 software (San Diego, CA, USA) was applied to drawing the figures. P<0.05 was considered significant.
The full-length ToCas8 and ToCas8-like cDNAs (GenBank accession numbers: OP407846, OP407847) are 1,638 and 1,149 bp in length and encode proteins of 545 and 382 amino acids, respectively. Online analysis using NCBI Conserved Domain Search revealed that DED and DD domains were observed at the N-terminal of the mammalian Caspase-8 proteins. Consistent with the Cas8 proteins in tilapia and grouper, two DED domains were observed at the N-terminal of golden pompano Cas8 and both Cas8 and Cas8-like proteins had a CASc structural domain at the C-terminal end (Figure 1B). Differently, Caspase-8-like proteins of pompano and grouper possessed a CARD domain in comparison with other isoforms (Figure 1A). The results demonstrated that the conserved structural domains of the two caspase-8 paralogs of golden pompano are consistent with the initiating Caspases and exhibit typical features of the Caspase-8 protein family.
Figure 1 Sequence alignments and domain organization of golden pompano caspase proteins. (A) Multiple alignment of the amino acid sequences of golden pompano Caspase-8s proteins. (B) Schematic protein domain architecture of the corresponding Caspase-8 proteins in vertebrates. CARD, caspase recruitment domain; DED, death effector domain; DD, Death Domain, CASc, caspase, interleukin-1 beta converting enzyme (ICE) homologs.
The genetic loci of two potential caspase-8 paralogs in the golden pompano genome were first identified, and the genetic neighbors of caspase-8 were tested to determine whether they were conserved. The results showed that the surrounding genes of caspase-8 were highly conserved and that cas8 and cas8-like genes were located on the same chromosome, which was also observed in most teleosts. Interestingly, two cas8-like genes were found in the genomes of zebrafish, Nile tilapia, and spotted gar (Figure 2).
Figure 2 Genetic analysis. Syntenic comparisons of Caspase-8 genes in teleosts. Boxes represent genes, and different genes are identified by different colored boxes; lines represent intergenic regions.
To determine the phylogenetic relationships of caspase gene families. A total of 58 caspase full-length amino acid sequences were used to reconstruct the phylogenetic tree. The results demonstrated that the phylogenetic tree was classified into inflammatory caspase subgroup (Caspase1), initiating caspase subgroup (Cas2, Cas8-10) and executive caspases (Cas3, Cas6 and Cas7) (Figure 3). Meanwhile, ToCas8 clustered with Caspase-8 in fish species, and the Caspase-8 family was further divided into Cas8 and Cas8-like subfamilies. The amino acid sequence identity between two ToCas8s was 28.7%. ToCas8 was closely related to the homologous genes of swordfish (Xiphias gladius), grouper (Epinephelus lanceolatus), Chinese perch (Siniperca chuats), with similarities of 74.1%, 68.5%, and 69.3%, respectively. ToCas8-like was closely related to ToCas8-like of amberjack (Seriola dumerili), swordfish, and Japanese rice fish (Oryzias latipes), with similarities of 80.5%, 68%, and 66.4%, respectively (Figure 3).
Figure 3 Phylogenetic analysis of Cas8 and other Caspase family members based on the alignment of amino acid sequences using MEGA 6. The tree was deduced by the neighbor-joining method, based on 1000 bootstrap replications for bootstrap analysis.
ToCas8s gene spatial expression pattern was determined using qRT-PCR, with β-actin serving as an internal control. The results showed that the ToCas8 genes were widely expressed in all tissues examined, but at inconsistent levels, and that the expression patterns of ToCas8 and ToCas8-like were similar (Figure 4). Meanwhile, high transcription levels of the ToCas8 and ToCas8-like were detected in the gill, kidney, and intestine, and the lowest levels were detected in the muscle and brain, respectively. The expression level of ToCas8 in the gill was 6.16-fold that in the muscle. The expression level of ToCas8-like in the gill, kidney and intestine was significantly higher than that in the brain (P < 0.01), which was 6.85-, 7.39-, and 3.29-fold that in the brain, respectively.
Figure 4 Relative expression levels of cas8 [(A), Tocas8; (B), Tocas8-like] mRNA in different tissues of golden pompano. These tissues included the liver, kidney, gill, brain, intestine, muscle, skin, spleen, and stomach. β-actin was selected as an internal reference gene to calibration cDNA template. These values are presented as mean ± standard deviation (n = 3).
To understand the link between the ToCas8 genes and the immune response in T. ovatus, changes in mRNA expression of ToCas8s after LPS or Poly(I:C) stimulation were measured by quantitative PCR.
In gill, ToCas8 expression decreased rapidly from 6 h to 12 h after LPS injection and increased obviously at 24 h (Figure 5A). After Poly(I:C) injection, ToCas8 expression increased markedly at 3 h, then declined at 12 h and again increased from 24 h to 48 h (Figure 5A). Differently, ToCas8-like expression showed a noticeable increase after intraperitoneal injection of LPS and Poly(I:C) and reached the maximum expression level after 3 hours (Figure 5B). In kidney, ToCas8 expression declined considerably from 3 h to 12 h after LPS and Poly(l:C) administration and then increased from 24 h to 48 h (Figure 6A). ToCas8-like expression peaked at 24 h after LPS and Poly(I:C) injection and then decreased at 48 h (Figure 6B). In intestine, ToCas8 expression declined significantly from 3 to 12 h and then increased from 24 to 48 h after LPS and poly(I:C) administration (Figure 7A). After LPS injection, ToCas8-like expression decreased considerably within 3 hours and then increased between 6 and 48 hours, whereas after poly(I:C) injection, ToCas8-like expression decreased within 3 hours, then increased within 6 hours and decreased again between 12 and 48 hours of LPS and poly(I:C) injection (Figure 7B).
Figure 5 Real-time quantitative PCR was performed to detect cas8 [(A), Tocas8; (B), Tocas8-like] mRNA expression levels in gill after LPS and Poly(I:C) stimulation. β-actin was selected as an internal reference gene to calibrate cDNA template.These values are presented as mean ± standard deviation (n = 3).
Figure 6 Real-time quantitative PCR was performed to detect cas8 [(A), Tocas8; (B), Tocas8-like] mRNA expression levels in kidney after LPS and Poly(I:C) stimulation. β- actin was selected as an internal reference gene to calibrate cDNA template.These values are presented as mean ± standard deviation (n = 3).
Figure 7 Real-time quantitative PCR was performed to detect cas8 [(A), Tocas8; (B), Tocas8-like) mRNA expression levels in intestine after LPS and Poly(I:C) stimulation. β-actin was selected as an internal reference gene to calibrate cDNA template. These values are presented as mean ± standard deviation (n = 3).
Caspases are widely distributed in organisms ranging from yeast to vertebrates (Madeo et al., 2004). In the present study, we identified two paralogs of cas8 in golden pompano. Multiprotein sequence alignment showed that although Caspase-8 proteins are unconserved across species, for example, mouse Caspase-8 showed 32% and 21.5% identity with ToCas-8-like and ToCas-8, respectively. However, the functional domains of these proteins are highly similar between species. Consistent with earlier research concerning caspase-8-like genes in Crassostrea hongkongensis (Xiang et al., 2013) and Crassostrea gigas (Li et al., 2015), suggesting the functional roles of Caspase-8 may be variable in vertebrates. Meanwhile, golden pompano Cas8 only shared 28.7% identity with its paralogs Caspase-8-like, implying the two different isoforms of Caspase-8 may play different roles in golden pompano. The mouse Caspase-8 contains DD and DED motifs at the N-terminus and a CASc domain at the C-terminus, which is consistent with humans (Varfolomeev et al., 1998). Protein secondary structure analysis revealed that ToCas8-like possesses a CARD domain at amino acid positions between 7-81. Some studies have found a caspase8-like gene closely related to the caspase8 gene in the genomes of zebrafish, puffer fish, and medaka (Sakata et al., 2007; Wei et al., 2019; Fu et al., 2020). The predicted protein has an active CASc and caspase recruitment domain but lacks an N-terminal DED motif, which is consistent with the ToCas8-like structure (Sakamaki et al., 2007). In contrast, ToCas8 contains two highly conserved DEDs at amino acid positions between 3-78 and 90-172, respectively. Both ToCas8 and ToCas8-like contained a CASc structural domain at the C-terminus.
In mammals, birds, and amphibians, Caspase8 is structurally similar to Caspase10 and Cflar, all three possessing DED and CASC domains (Sakamaki and Satou, 2009). Studies have shown that caspase-10 plays a critical role in the regulation of apoptosis, caspase-8 and caspase-10 have different functions in human T cell subsets (Salmena and Hakem, 2005; Li et al., 2008). cFlar was identified as a molecule that regulates Caspase-8 activation (Lees et al., 2020). The caspase-18 gene is located between the caspase-8 and caspase-10 motifs in the chicken genome and also has DED and CASC structural domains (Sakamaki et al., 2015). The chicken caspase 18 gene has a very high structural similarity to chicken caspase 8 and exhibits a similar mRNA expression pattern (Reno et al., 2022). It has been proposed that these four genes emerged early in vertebrate evolution as a result of replication events acting on the ancestral casp8 gene (Sakamaki et al., 2015). During the evolution to bony fishes, these genes were recombined and a new fish-specific casp8-like gene (card-casp8), replacing the DED motif with a CARD motif, appeared next to the casp8 locus (Sakata et al., 2007).
Caspases are classified into two groups based on their function properties, including inflammatory Caspases and apoptotic Caspases (Yuan et al., 2018). Caspase-1, -4, -5, -11, and -12 belong to the inflammatory Caspase family and regulate inflammatory responses and cell death in the host to defend against microbes (Kesavardhana and Kanneganti, 2017). The functions of apoptotic Caspases are primarily involved in initiating and executing apoptosis, which can be further divided into initiating (-2, -8, -9, and -10) and executive Caspases (-3, -6 and -7). Consistently, phylogenetic results in the present study further confirmed the conclusion, and the two ToCas8 paralogs were clustered into cas8 and cas8-like subfamily respectively, suggesting multiple cas8 genes widely existed in teleosts.
Quantitative PCR was conducted to investigate the pattern of tissue distribution of ToCas8 and ToCas8-like. The results showed that high expression levels of ToCas8 and ToCas8-like transcripts were detected in the gill, kidney, and intestine, and the lower levels were examined in the muscle and brain. In terrestrial animals, pathogens infect their hosts through their gastrointestinal route, while pathogens in water generally enter their hosts through the gills. The gill is a semi-permeable barrier between the bony fish and the external environment. Consequently, the gill is a primary organ involved in the immune response (Koppang et al., 2015). The intestinal mucosa of fish faces similar challenges as the gill. Meanwhile, the kidney is one of the largest lymphoid and immunoreactive organs in bony fishes (Xu et al., 2018). The high expression of ToCas8s in gill, kidney and intestine indicates that ToCas8s are actively involved in apoptosis and immune response in immune organs. Differently, in studies in mice, the expression levels of Cas8 mRNA were higher in the spleen, thymus, lung, liver, and kidney than in the heart, brain, and testis, while almost undetectable in skeletal muscle in adult mouse (Sakamaki et al., 1998). The expression pattern of the casp8 gene in humans is consistent with that in mice (Muzio et al., 1996). Meanwhile, caspase8 gene was found to be highly expressed in blood but expressed at lower levels in the spleen and trunk kidney in Japanese flounder (Li et al., 2019). In Hong Kong oyster, caspase-8 mRNA was widely detected in a variety of tissues and highly expressed in the labial palate, gonads, and gills (Xiang et al., 2013). This expression pattern is also found in sea bass (Reis et al., 2010). These studies indicate that the functions of caspase-8 may be variable due to the tissue-specific distributed pattern in different animals.
Caspases play key roles in inflammatory and apoptotic cell death. Molecular pathways controlled by Caspase-dependent substrate cleavage are evolutionarily conserved in eukaryotes and regulate essential cellular processes by inducing apoptosis or triggering inflammatory cell death (Kesavardhana et al., 2020). Caspase-8 is converted to the active form through the interaction between the DED structural domains and then bound to FADD, forming the Fas-FADD-Casp8 complex, known as the death-inducing signaling complex (DISC). Several RNA viruses have been shown to readily activate the Caspase8-related apoptotic pathway (Günther et al., 2015). A previous study showed that Caspase-9 activity in mouse oligodendrocytes was 12-fold higher in virus-infected cells than in mock-infected cells at 24 h after viral infection, and Caspase-8 activity was also found to be dramatically increased in virus-infected cells (Liu et al., 2006). In Japanese flounder, the gene expression of the initiating Cas8 was upregulated by LPS and poly(I:C) treatment (Li et al., 2019). Similarly in Hong Kong oyster, Cas8 was found to be significantly up-regulated in hemocytes followed by Vibrio aestuarianus, or Salmo cerevisiae challenge (Xiang et al., 2013). Consistently, increased expression of ToCas8s was detected in the gill, kidney, and intestine after stimulation with LPS and Poly(I:C) in golden pompano. ToCas8 expression increased in the gills after Poly(I:C) stimulation for 3 hours, and increased expression was detected in the kidney and intestine after 24 hours. After 24 hours of LPS stimulation, the expression of ToCas8 in the tested tissues increased. After stimulation with LPS and Poly(I:C) for 3 hours, the expression of ToCas8-like increased in the gills, 6 hours later in the intestine, and 24 hours later in the kidney. These results demonstrate that ToCas8s were more sensitive to Poly(I:C) stimulation, and ToCas8-like responded more rapidly to LPS and Poly(I:C). The results indicate that both ToCas8 and ToCas8-like are involved in the defense against pathogen infection.
In summary, we identified two paralogs of Caspase-8 in golden pompano. The qRT-PCR results showed that these two caspase-8 paralogs were expressed mainly in the gill, kidney and intestine. Challenge experiments showed that ToCas8 and ToCas8-like are involved in resistance to pathogen infection, suggesting that they play an essential role in the immune system.
The datasets presented in this study can be found in online repositories. The names of the repository/repositories and accession number(s) can be found in the article/Supplementary Material.
The animal study was reviewed and approved by Guangdong Provincial Key Laboratory of Pathogenic Biology and Epidemiology for Aquatic Economic Animals Ethics Committee.
FD performed the experiments and wrote the manuscript. SC and JJ designed the experiments and revised the manuscript of FD. XW and GL assisted with fish rearing and injection experiments. All authors contributed to the article and approved the submitted version.
This work was financially supported by National Natural Science Foundation of China (No. U20A2065) and Guangdong Natural Science Foundation (No. 2021A1515010532).
The authors of the present study thank the Guangdong Provincial Key Laboratory of Aquatic Animal Disease Control and Healthy Culture for providing the experimental facilities.
The authors declare that the research was conducted in the absence of any commercial or financial relationships that could be construed as a potential conflict of interest.
All claims expressed in this article are solely those of the authors and do not necessarily represent those of their affiliated organizations, or those of the publisher, the editors and the reviewers. Any product that may be evaluated in this article, or claim that may be made by its manufacturer, is not guaranteed or endorsed by the publisher.
The Supplementary Material for this article can be found online at: https://www.frontiersin.org/articles/10.3389/fmars.2022.1093176/full#supplementary-material
Bi X., Liu X., Bi D., Sun Y. (2018). Identification of caspase-6 and caspase-7 from miiuy croaker and evolution analysis in fish. Fish Shellfish Immunol. 83, 406–409. doi: 10.1016/j.fsi.2018.09.050
Boege Y., Malehmir M., Healy M. E., Bettermann K., Lorentzen A., Vucur M., et al. (2017). A dual role of caspase-8 in triggering and sensing proliferation-associated DNA damage, a key determinant of liver cancer development. Cancer Cell 32, 342–359. doi: 10.1016/j.ccell.2017.08.010
Cao L., Quan X. B., Zeng W. J., Yang X. O., Wang M. J. (2016). Mechanism of hepatocyte apoptosis. J. Cell Death 9, 19–29. doi: 10.4137/JCD.S39824
D’Arcy M. S. (2019). Cell death: a review of the major forms of apoptosis, necrosis and autophagy. Cell Biol. Int. 43, 582–592. doi: 10.1002/cbin.11137
Da F., Tan H., Wan X., Lin G., Jian J., Wen Z., et al. (2022). Molecular characterization, expression and response to immune challenges of 3 members of the toll-like receptor superfamily 11 in the golden pompano (Trachinotus ovatus). Aquaculture Rep. 25, 101268. doi: 10.1016/j.aqrep.2022.101268
Fianco G., Contadini C., Ferri A., Cirotti C., Stagni V., Barilà D. (2018). Caspase-8: a novel target to overcome resistance to chemotherapy in glioblastoma. Int. J. Mol. Sci. 19, 3798. doi: 10.3390/ijms19123798
Fu S., Ding M., Wang J., Yin X., Zhou E., Kong L., et al. (2020). Identification and functional characterization of three caspases in Takifugu obscurus in response to bacterial infection. Fish Shellfish Immunol. 106, 252–262. doi: 10.1016/j.fsi.2020.07.047
Gitlin A. D., Heger K., Schubert A. F., Reja R., Yan D., Pham V. C., et al. (2020). Integration of innate immune signalling by caspase-8 cleavage of N4BP1. Nature 587, 275–280. doi: 10.1038/s41586-020-2796-5
Günther C., Buchen B., He G. W., Hornef M., Torow N., Neumann H., et al. (2015). Caspase-8 controls the gut response to microbial challenges by tnf-α-dependent and independent pathways. Gut 64, 601–610. doi: 10.1136/gutjnl-2014-307226
Kesavardhana S., Kanneganti T. D. (2017). Mechanisms governing inflammasome activation, assembly and pyroptosis induction. Int. Immunol. 29, 201–210. doi: 10.1093/intimm/dxx018
Kesavardhana S., Malireddi R. S., Kanneganti T. D. (2020). Caspases in cell death, inflammation, and pyroptosis. Annu. Rev. Immunol. 38, 567–595. doi: 10.1146/annurev-immunol-073119-095439
Kominami K., Nakabayashi J., Nagai T., Tsujimura Y., Chiba K., Kimura H., et al. (2012). The molecular mechanism of apoptosis upon caspase-8 activation: quantitative experimental validation of a mathematical model. Biochim. Biophys. Acta (BBA)-Molecular Cell Res. 1823, 1825–1840. doi: 10.1016/j.bbamcr.2012.07.003
Koppang E. O., Kvellestad A., Fischer U. (2015). Fish mucosal immunity: gill. Mucosal Health aquaculture, 93–133. doi: 10.1016/B978-0-12-417186-2.00005-4
Lees A., McIntyre A. J., Crawford N. T., Falcone F., McCann C., Holohan C., et al. (2020). The pseudo-caspase FLIP (L) regulates cell fate following p53 activation. Proc. Natl. Acad. Sci. 117, 17808–17819. doi: 10.1073/pnas.2001520117
Li S., Li J., Peng W., Hao G., Sun J. (2019). Characterization of the responses of the caspase 2, 3, 6 and 8 genes to immune challenges and extracellular ATP stimulation in the Japanese flounder (Paralichthys olivaceus). BMC veterinary Res. 15, 1–12. doi: 10.1186/s12917-018-1763-y
Li C., Qu T., Huang B., Ji P., Huang W., Que H., et al. (2015). Cloning and characterization of a novel caspase-8-like gene in Crassostrea gigas. Fish Shellfish Immunol. 46, 486–492. doi: 10.1016/j.fsi.2015.06.035
Liu Y., Pu Y., Zhang X. (2006). Role of the mitochondrial signaling pathway in murine coronavirus-induced oligodendrocyte apoptosis. J. Virol. 80, 395–403. doi: 10.1128/JVI.80.1.395-403.2006
Li C., Zhao H., Hu Z., Liu Z., Wang L. E., Gershenwald J. E., et al. (2008). Genetic variants and haplotypes of the caspase-8 and caspase-10 genes contribute to susceptibility to cutaneous melanoma. Hum. Mutat. 29, 1443–1451. doi: 10.1002/humu.20803
Madeo F., Herker E., Wissing S., Jungwirth H., Eisenberg T., Fröhlich K. U. (2004). Apoptosis in yeast. Curr. Opin. Microbiol. 7, 655–660. doi: 10.1016/j.mib.2004.10.012
Muzio M., Chinnaiyan A. M., Kischkel F. C., O'Rourke K., Shevchenko A., Ni J., et al. (1996). FLICE, a novel FADD-homologous ICE/CED-3-like protease, is recruited to the CD95 (Fas/APO-1) death-inducing signaling complex. Cell 85, 817–827. doi: 10.1016/S0092-8674(00)81266-0
Orning P., Lien E. (2021). Multiple roles of caspase-8 in cell death, inflammation, and innate immunity. J. leukocyte Biol. 109, 121–141. doi: 10.1002/JLB.3MR0420-305R
Ramirez M. L. G., Salvesen G. S. (2018). A primer on caspase mechanisms. Semin. Cell Dev. Biol. 82, 79–85. doi: 10.1016/j.semcdb.2018.01.002
Reis M. I., Costa Ramos C., do Vale A., dos Santos N. M. (2010). Molecular cloning of sea bass (Dicentrarchus labrax l.) caspase-8 gene and its involvement in Photobacterium damselae ssp. piscicida triggered apoptosis. Fish Shellfish Immunol. 29, 58–65. doi: 10.1016/j.fsi.2010.02.016
Reno K. E., Cloft S. E., Wong E. A. (2022). Expression of genes associated with apoptosis in the residual yolk sac during the peri-hatch period of broiler chicks. Poultry Sci. 101, 101966. doi: 10.1016/j.psj.2022.101966
Sakamaki K., Imai K., Tomii K., Miller D. J. (2015). Evolutionary analyses of caspase-8 and its paralogs: deep origins of the apoptotic signaling pathways. Bioessays 37, 767–776. doi: 10.1002/bies.201500010
Sakamaki K., Nozaki M., Kominami K., Satou Y. (2007). The evolutionary conservation of the core components necessary for the extrinsic apoptotic signaling pathway, in medaka fish. BMC Genomics 8, 1–15. doi: 10.1186/1471-2164-8-141
Sakamaki K., Satou Y. (2009). Caspases: evolutionary aspects of their functions in vertebrates. J. fish Biol. 74, 727–753. doi: 10.1111/j.1095-8649.2009.02184.x
Sakamaki K., Tsukumo S., Yonehara S. (1998). Molecular cloning and characterization of mouse caspase-8. Eur. J. Biochem. 253, 399–405. doi: 10.1046/j.1432-1327.1998.2530399.x
Sakata S., Yan Y., Satou Y., Momoi A., Ngo Hazelett P., Nozaki M., et al. (2007). Conserved function of caspase-8 in apoptosis during bony fish evolution. Gene 396, 134–148. doi: 10.1016/j.gene.2007.03.010
Salmena L., Hakem R. (2005). Caspase-8 deficiency in T cells leads to a lethal lymphoinfiltrative immune disorder. J. Exp. Med. 202, 727–732. doi: 10.1084/jem.20050683
Spead O., Verreet T., Donelson C. J., Poulain F. E. (2018). Characterization of the caspase family in zebrafish. PloS One 13, e0197966. doi: 10.1371/journal.pone.0197966
Varfolomeev E. E., Schuchmann M., Luria V., Chiannilkulchai N., Beckmann J. S., Mett I. L., et al. (1998). Targeted disruption of the mouse caspase 8 gene ablates cell death induction by the TNF receptors, Fas/Apo1, and DR3 and is lethal prenatally. Immunity 9, 267–276. doi: 10.1016/S1074-7613(00)80609-3
Wei L., He L., Fu J., Liu Y., Ruan J., Liu L., et al. (2019). Molecular characterization of caspase-8-like and its expression induced by microcystin-LR in grass carp (Ctenopharygodon idella). Fish Shellfish Immunol. 89, 727–735. doi: 10.1016/j.fsi.2019.04.026
Xiang Z., Qu F., Qi L., Zhang Y., Tong Y., Yu Z. (2013). Cloning, characterization and expression analysis of a caspase-8 like gene from the Hong Kong oyster, Crassostrea hongkongensis. Fish Shellfish Immunol. 35, 1797–1803. doi: 10.1016/j.fsi.2013.08.026
Xu C., Li E., Suo Y., Su Y., Lu M., Zhao Q., et al. (2018). Histological and transcriptomic responses of two immune organs, the spleen and head kidney, in Nile tilapia (Oreochromis niloticus) to long-term hypersaline stress. Fish Shellfish Immunol. 76, 48–57. doi: 10.1016/j.fsi.2018.02.041
Yuan Y. Y., Xie K. X., Wang S. L., Yuan L. W. (2018). Inflammatory caspase-related pyroptosis: mechanism, regulation and therapeutic potential for inflammatory bowel disease. Gastroenterol. Rep. 6, 167–176. doi: 10.1093/gastro/goy011
Keywords: Trachinotus ovatus, caspase-8, phylogenetic analysis, tissue distribution, LPS challenge, Poly(I:C) stimulation, immune response
Citation: Da F, Wan X, Lin G, Jian J and Cai S (2022) Cloning, characterization and expression analysis of caspase-8 genes from the golden pompano (Trachinotus ovatus). Front. Mar. Sci. 9:1093176. doi: 10.3389/fmars.2022.1093176
Received: 08 November 2022; Accepted: 13 December 2022;
Published: 23 December 2022.
Edited by:
Andrew Stanley Mount, Clemson University, United StatesReviewed by:
Zhao Lv, Hunan Agricultural University, ChinaCopyright © 2022 Da, Wan, Lin, Jian and Cai. This is an open-access article distributed under the terms of the Creative Commons Attribution License (CC BY). The use, distribution or reproduction in other forums is permitted, provided the original author(s) and the copyright owner(s) are credited and that the original publication in this journal is cited, in accordance with accepted academic practice. No use, distribution or reproduction is permitted which does not comply with these terms.
*Correspondence: Shuanghu Cai, Y2Fpc2hAZ2RvdS5lZHUuY24=
Disclaimer: All claims expressed in this article are solely those of the authors and do not necessarily represent those of their affiliated organizations, or those of the publisher, the editors and the reviewers. Any product that may be evaluated in this article or claim that may be made by its manufacturer is not guaranteed or endorsed by the publisher.
Research integrity at Frontiers
Learn more about the work of our research integrity team to safeguard the quality of each article we publish.