- 1College of Transport and Communications, Shanghai Maritime University, Shanghai, China
- 2School of Management, Fudan university, Shanghai, China
- 3School of Economics, Jiangsu University of Technology, Jiangsu, China
With the construction of China’s ecological civilization and the proposal of carbon peaking and carbon neutrality goals, shore power has been vigorously developed as an important technology for the future green development of ports. However, China’s electricity is still mostly coal-fired, which produces many carbon emissions. Coupled with regional differences, shore power is by no means certain to lower carbon emissions compared with fuel throughout China. Considering the power energy structure in different regions, this paper establishes a carbon emission correlation model between fuel and shore power during ship berthing, calculates the feasibility and actual emission reduction effect of shore power in coastal ports, and studies the restriction condition of starting time for the use of shore power for ships attached to ports according to the national policy of mandatory use of shore power. The results show that only a small part of coastal provinces and cities are suitable for using shore power, and it is limited by the berthing time of the ship. However, this condition is not related to the size of ships but related to the proportion of power generation. Therefore, the government should develop shore power according to local conditions, and vigorously increase the proportion of clean energy, so that the shore power truly achieve zero carbon emissions.
1 Introduction
With the continuous rise of global temperature, greenhouse gases, especially carbon emissions, have attracted much attention from all over the world. In 2020, China pledged to achieve peak carbon emissions before 2030 and to strive to achieve carbon neutrality before 2060 (Sun et al., 2022). The transportation sector has an important role to play in achieving carbon peaking and carbon neutrality goals. As one of the industries difficult to decarbonize (Sharmina et al., 2021), the shipping industry has been attempting to take various measures to reduce the carbon emissions of ships, such as a carbon tax (Ding et al., 2020), ship speed optimization (Cariou et al., 2019), low-carbon fuel and alternative power (Xing et al., 2020), etc. These measures are mainly to reduce the carbon emissions of ships during navigation, but ships also consume fuel and produce CO2 in the process of berthing operations. Therefore, shore power, as one of the ways to reduce carbon emissions in port operations, has been widely studied and promoted in recent years (Lathwal et al., 2021).
Shore power refers to the power supply that connects to the power supply on the dock and turns off the ship’s generator when the ship docks so that the power load on the ship can be transferred to the shore seamlessly. It consists of three parts: the shore power system and infrastructure, the cable management system and the ship-side power system (Chen et al., 2019; Peng et al., 2020). It can not only effectively reduce ship emissions by approximately 48-70% during the ship’s stay in port (Zis et al., 2014) but also eliminate the noise and vibration caused by the operation of the ship’s generator. It is especially suitable for vessels with relatively fixed routes, large power consumption and high air pollutant emission levels when docking, such as container ships, ferries, cruise ships and medium and large bulk carriers (Dai et al., 2020).
With the attention of environmental protection in China, shore power as an effective way of saving energy and reducing emissions, has been vigorously promoted. By the end of 2021, port power facilities had covered approximately 7,500 berths across the country, with 75 percent coverage of five specialized berths, including container, passenger rolling, cruise, and passenger transport of over 3,000 tons and dry bulk cargo of over 50,000 tons (Zhang, 2022). However, due to the time-consuming connection of shore power and the lack of standardization in various countries (Yin et al., 2020) its utilization rate is less than 20% (Wang, 2022), In this regard, the Ministry of Transport proposed mandatory measures (MOT of the PRC, 2021): “If a ship with power-receiving facilities berths in a coastal port with shore power facilities for more than 3 hours and berths in an inland river for more than 2 hours, it shall use shore power. Otherwise, it will be punished based on the total power of the ship’s generator set, combined with the power of the ship’s generator set and the length of berthing.” However, from the perspective of shore power sources, the power source will also produce carbon emissions in the process of power generation; therefore, whether it is truly low carbon compared with fuel is controversial. At present, thermal power generation accounts for 71.13% of the total in China; hydropower and other new energy sources account for 28.87% (NBS of the PRC, 2021). It can be seen that thermal power generation is still the main source of carbon emissions. In addition, there are certain differences in the power consumption of different areas: hydropower has dominated the central part, the coastal areas have good nuclear power generation, and northwestern and northern China have good resources for wind power and solar power generation, but there is almost no clean energy power generation in the northeastern inland areas. Different provinces with ports also have different proportions of electricity and energy; therefore, mandatory measures may not be applicable to all areas, and the mandatory berthing time also needs to be verified.
Therefore, to further study the emission reduction effect of shore power compared with fuel, we verify the feasibility of shore power in different provinces and cities from the perspective of power sources, obtain specific conditions for using shore power, such as berthing time, and propose suggestions on the response strategies of each province and city according to the current shore power policy. This paper establishes a carbon emission correlation model between fuel and shore power during ship berthing and compares the carbon emissions generated using fuel and shore power to provide a reference for the promotion of shore power in different areas. The rest of this paper is organized as follows: Section 2 presents a literature review of shore power sources, emission reduction effects, and promotion optimization. Section 3 describes the methodology, including problem definition, symbolic terminology and mathematical models. Section 4 conducts a case study on the feasibility and conditions of using shore power in coastal port provinces and cities. Finally, Section 5 summarizes the conclusions and policy implications.
2 Literature review
Shore power is one of the important measures to reduce carbon emissions in ports. Current research has mainly focused on the emission reduction effect of shore power and how to promote and optimize shore power in terms of environmental issues.
2.1 Traceability of shore power
The carbon emissions of shore power are closely related to the method of electricity generation; that is, the actual energy mix of the grid, such as coal, nuclear energy, photovoltaic energy, etc., should be considered (Peng et al., 2019). For a cleaner and greener environment, the development of renewable energy is very important to reduce air pollution by using shore power (Kotrikla et al., 2017). Studies have shown that the source of electricity has gradually shifted from thermal combustion to wind energy, tidal energy, and solar energy (Kalikatzarakis et al., 2018). Among them, wind energy, as a clean, natural and abundant renewable energy source, has high global potential. However, according to Kumar et al. (2016), while offshore wind resources are more promising than onshore resources and can compete with fossil-fuel power plants in terms of efficiency and output, there is no advanced technology to fully utilize them. There are also scholars studying wave-power generation for sustainable coastal development, but there are challenges such as high costs (Xu and Huang, 2018). Solar energy is not conducive to the stable operation of the power grid because of its large randomness, intermittency and volatility (Gao et al., 2022). Therefore, renewable energy is still unable to meet the power demand of ships in the short term.
According to the CEC data of the PRC (2020), China’s electricity energy is still dominated by fossil-fuel thermal power generation. Although the development of hydropower resources in clean energy has been very high, tidal power generation has long been established as a demonstration project, but it has not been promoted. In the future, China’s power generation method will gradually change to new energy sources such as wind energy and solar energy as the core sources and hydropower as the auxiliary source, thereby gradually reducing the proportion of thermal power in the overall power system (Zhang et al., 2015). Therefore, from the perspective of shore power traceability, the widespread use of shore power is an inevitable trend, but it is currently affected by the cleanliness of the power generation energy. The emission reduction effect of shore power needs to be improved.
2.2 Emission reduction effect of shore power
Shipping is increasingly recognized as an important source of air pollutants such as NOx, SO2, CO, particulate matter (PM) and the main greenhouse gas, CO2. Most CO2 emissions from shipping occur during the time ships spend in port (Styhre et al., 2017). Some scholars taking Guangzhou Port as an example compared the environmental benefits of two emission reduction measures using low-sulfur oil and shore power technology. The results showed that shore power has significant advantages in reducing NOx emissions (He et al., 2020). In addition, quantitative calculations for Kaohsiung Port have shown the long-term potential of shore power to improve environmental and socioeconomic conditions by reducing port emissions (Tseng and Pilcher, 2015). To reduce air pollution, the Italian government has considered using shore power to reduce air pollution caused by ships and has conducted an economic analysis (Adamo et al., 2014).
However, some scholars are skeptical about the emission reduction effect of shore power. The European Commission has been hoping for a shift to an energy-efficient model for shipping, but research has shown that the implementation of shore power can be challenging. For most EU member states, shore power will help reduce CO2 emissions, but the proportion of power generation energy varies from country to country; therefore, not all maritime countries can use shore power to reduce CO2 emissions (William, 2010). In countries with a high carbon content in the electricity supply, the use of shore power from the national grid can lead to increased carbon emissions compared to the use of standard diesel generators (Winkel et al., 2016). In addition, according to the requirement of “connecting shore power first, then loading and unloading operations”, it takes time for ships to connect to shore power, which may cause delays. If the carbon reduction effect of shore power is considered from the perspective of the entire voyage, the increase in carbon emissions due to delays caused by shore power can almost be offset by the reduction in shore power (Dai et al., 2020). Therefore, the actual emission reduction effect of shore power needs to be considered and studied in various aspects according to the characteristics of different areas.
2.3 Promotion and optimization of shore power
The research of Chen et al. (2019) shows that the promotion of shore power is subject to 12 constraints in four aspects: technological application, economic cost, operation management, and policy system. The most critical among them are the policy support system, the construction standards for shore power, and the laws and regulations. From the perspective of technical application, Tang et al. (2018) proposed a hybrid energy system including shore power, offshore photovoltaics, batteries, and diesel, considering different shore power prices and emission policies and optimizing them. There have also been discussions on the challenges of shore power technology to the voltage, frequency, power and other technical requirements of ships and onshore ships. By comparing various shore power technologies, it has been recommended to adopt new shore power technologies compatible with TN and IT standards (Kumar et al., 2019; Wu et al., 2013). In response to the high initial investment and operating costs of shore power and the lack of attractiveness, Dai et al. (2019) proposed an environmental and technical–economic analysis framework combined with an emissions trading scheme (ETS) to evaluate the economic feasibility of shore power, because shore power investment in the port is only economically feasible if the port can profit from the sale of electricity. Tan et al. (2021) established a ship selection behavior model for the economics of shore power to assist shipowners in making decisions about using shore power or fuel. The inland river shore power service evaluation system was established to realize an evaluation of the environmental benefits of a shore power system in a designated area.
In addition, the optimization of shore power can mainly be considered from the perspectives of shipowners, ports and governments (Qi et al., 2020). From these three perspectives, economic problems and management defects are the main obstacles to the wider application of shore power. In addition, government subsidies play an important role in the deployment of shore power (Wu and Wang, 2020). Therefore, it is necessary to strengthen the cooperation among shipowners, ports and governments.
At present, the research on shore power has mainly focused on the development and overall promotion and optimization of shore power. Few scholars have considered the inconsistency of the power energy structure in different areas of China from the perspective of shore power sources and the actual emission reduction effect of shore power relative to fuel. There is a lack of research on shore power construction strategies according to local conditions. Therefore, this paper traces the source of shore power, mainly considering thermal power, hydropower, nuclear power, wind power, solar power and other power generation methods. Combined with the power energy structure in different areas, the carbon emission correlation model between fuel and shore power during ship berthing is established, and the calculation of the actual emission reduction effect is completed. Finally, the necessary conditions for the berthing time for ships to use shore power to achieve carbon emission reduction are determined, and pertinent suggestions for realizing the construction of shore power in Chinese ports according to local conditions are proposed.
3 Methodologies
3.1 Problem definition
The purpose of this paper is to study the real emission reduction effect by using shore power instead of auxiliary engine fuel for ships calling at Chinese ports, considering the power traceability of the shore power system. The main issues can be divided into the following two parts:
(1) Under the current power energy structure of coastal provinces and cities in China, how can we determine whether the ports of each province or city are suitable for using the shore power system?
(2) What is the specific emission reduction effect of different ships using shore power? What are the basic conditions for determining that a ship is suitable for using shore power?
This paper solves the above problems by establishing a mathematical model. The main parameters involved in the model are given below:
where Yi is the amount of carbon emissions reduced o from ship i using shore power instead of fuel during the berthing operation, g;
Δ is the marginal carbon emission reduction benefit from a ship using shore power, g/kWh;
CEFi is the carbon emissions from fuel used by ship i at berth, g;
CEEi is the carbon emissions from the whole process of shore power usage from ship i at berth, g;
CEEFi is the carbon emissions from preparing the shore power for ship i, g;
CEEEi is the carbon emissions from using the shore power by ship i, g;
FCiis the fuel consumption of ship i at berth, g;
ECi is the total electricity consumption using the shore power by ship i at berth, kW;
ECGi is the electricity required by ship i when using shore power, kW;
ECTi is the power loss when ship i uses shore power, kW;
FCEi is the fuel consumption for preparing the shore power for ship i, g;
Pi is the auxiliary rated power of ship i, kW;
LF is the auxiliary engine load factor of the ships at berth;
SFC is the amount of fuel required per unit of electricity, g/kWh;
Ti is the dwell time during loading and discharging of ship i at berth, h;
t is the time for preparing shore power, h;
T*is the basic berthing time of a ship for carbon emission reduction by using shore power, h;
GTi is the gross tonnage of ship i, GT or TEU;
DTi is the deadweight of ship i, t or TEU;
v is the handling efficiency of the port of call, t/h or TEU/h;
YF is the carbon emission factor of the fuel used by marine auxiliary engines;
YEJ is the average carbon emission factor of energy j;
ηj is the proportion of power generation of energy j in an area; and
µ is the average power loss factor.
3.2 Mathematical model
In this section, a shore power–fuel carbon emission correlation model is established. It calculates the actual emission reduction effect of ships using shore power instead of fuel. Then, we determine whether the port is suitable for using shore power systems. Finally, we determine the basic conditions for carbon emission reduction by using shore power. The model is described as follows:
The correlation model can be divided into two parts: the calculation of the carbon emissions of ships using shore power and auxiliary engine fuel.
3.2.1 Fuel-carbon emission model
When ships are berthing, only auxiliary engines are used to power the ship; therefore, the ship fuel-carbon emission model can be established based on the ship’s auxiliary fuel consumption formula (Moreno-Gutierrez et al., 2018).
3.2.2 Shore power–carbon emission model
When a ship uses shore power, its carbon emission sources can be divided into two parts:
(1) The carbon emissions from auxiliary fuel consumption when the ship is waiting to connect to and disconnect from the shore power;
(2) The carbon emissions from the traceability process of power consumption.
Its calculation formula is as follows:
Among them, the carbon emission calculation formula of the fuel part is basically the same as that of the fuel-carbon emission model, but the berthing time becomes the waiting time for the ship to connect and disconnect the shore power.
The carbon emissions of the shore power part need to consider the loss of electric energy in the transmission process. The calculation formula is as follows:
According to the China Energy Statistical Yearbook (Department of Energy Statistics. National Bureau of Statistics, 2013), the average CO2 emission coefficient γEj of each power source is shown in Table 1.
The total power consumed by ships at berth using shore power includes the power required for the ships’ operation and the power loss in the power grid. Therefore, the calculation is divided into two parts: the power consumption process and the power transmission and transformation process.
3.2.2.1 Power consumption
The rated power of the ship’s auxiliary engine is a key parameter to calculate the ship’s electricity consumption. The relationship function between the auxiliary engine rated power of different types of ships and the gross tonnage of the ship obtained by the study has a good fitting effect (Gutierrez et al., 2019). It can be useful for the calculation of Pi , as shown in Table 2.
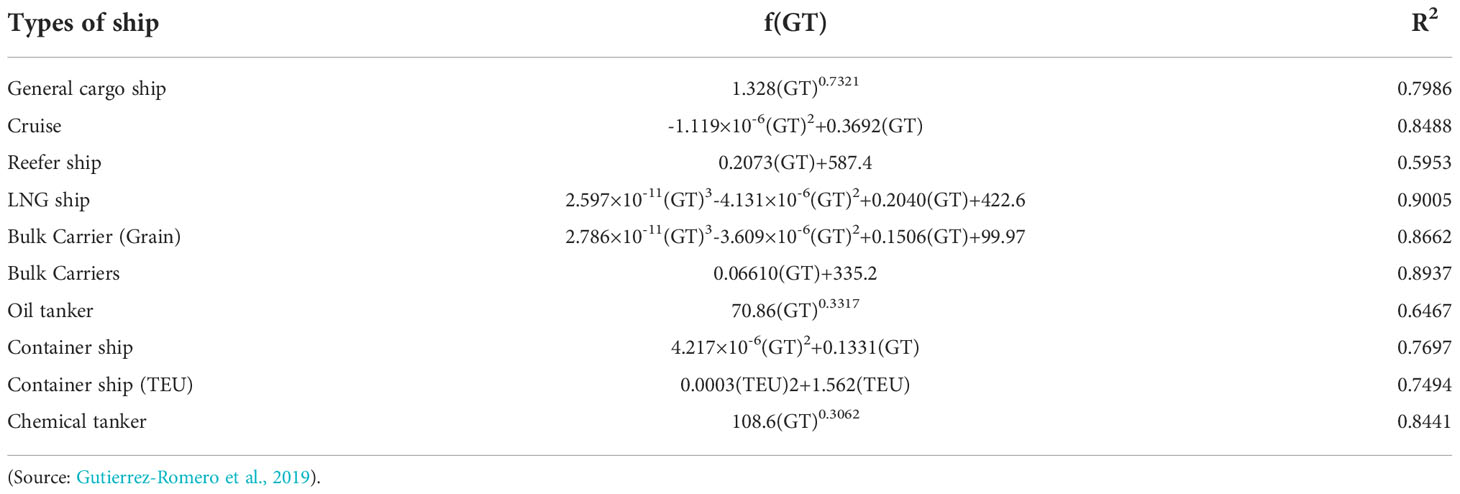
Table 2 Relation function of auxiliary engine-rated power and gross tonnage of various types of marine auxiliary engines.
3.2.2.2 Power loss
In the process of power supply, the power system will inevitably have some power loss, including the energy loss caused by transmission lines and the power loss caused by transformers and other power(Sundaram, 2022). In addition, there is also the loss of transmission from the grid to the onshore power supply. The power consumption formula in the power consumption process is as follows:
3.3 Judgment rules based on the correlation model
By analyzing the constructed correlation model, the criteria for determining whether the ship calling at a port is suitable for using shore power can be obtained. When Yi <0, the ship is suitable for using shore power at this port; otherwise, it cannot achieve carbon emission reduction by using shore power. In addition, the correlation model can determine the cleanliness of shore power from two perspectives: port and ship.
By deforming the judgment rule (Yi <0), the marginal carbon emission reduction benefit of port shore power can be obtained, and the cleanliness of shore power can be determined from the perspective of the port.
According to the above judgment rules, when the proportion of thermal power generation in the area is low, Δ>0, then the marginal carbon emission reduction benefit of using shore power at the port is positive, and the shore power system can achieve carbon emission reduction; and when the proportion of thermal power generation in the area is high, Δ≤0, then the port is not suitable for using shore power.
Determining from the perspective of the ship, when Δ>0, Yi =0; then, the solution equation for the basic berthing time is as follows:
For a port with positive marginal carbon emission reduction benefits of shore power, the port is suitable for using shore power systems; when the berthing time of the ship exceeds the basic berthing time, the ship is suitable for using shore power at this port.
4 Case analysis
4.1 The power energy structure of coastal ports
According to the shore power–fuel carbon emission correlation model, the power energy structure has a significant impact on whether the ports are suitable for using shore power. This section first collects data from various provinces and cities in China in 2021 (see Appendix A for detailed data). Among them, the provinces and cities where Chinese coastal ports are located are currently dominated by thermal power generation, but there are certain differences in the power energy structure in different provinces and cities.
In addition, the power grid is usually used between the provinces in China for power transmission. At present, the six regional power grids belonging to the State Grid in China have basically achieved interconnection and interoperability. Therefore, the Chinese power distribution and transmission network can be divided into two parts: the State Grid and Southern Power Grid (Abhyankar et al., 2020). According to an assumption (Chen et al., 2022), the power generation of each province or city first meets its own electricity demand. If there is surplus electricity, it will be transmitted to other provinces and cities through the grid market, as shown in Table 3.
The remaining electricity consumption in Guangdong, Guangxi, and Hainan is provided by the Southern Power Grid, and that in the other eight provinces and cities is provided by the State Grid (Abhyankar et al., 2020). According to each area’s own power generation and grid distribution (this study ignores the loss rate of cross-provincial distribution), the actual power energy structure of 11 coastal port provinces and cities is shown in Figure 1 (see Appendix A for detailed data):
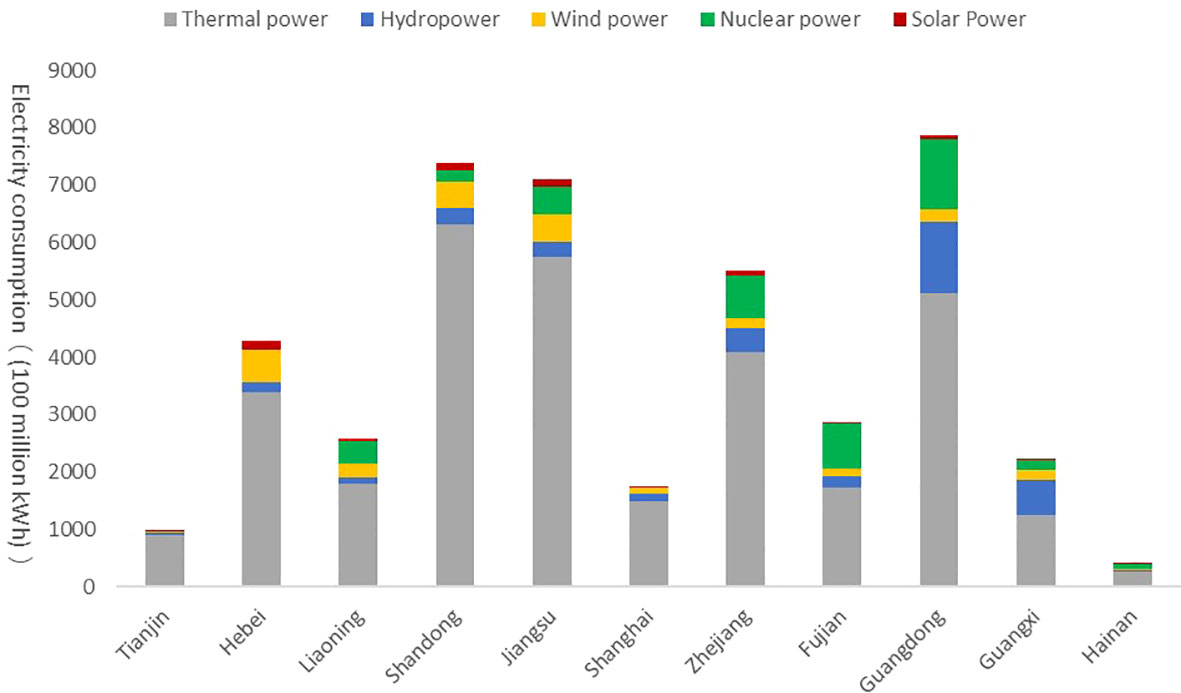
Figure 1 The actual power energy structure of 11 coastal port provinces and cities. (Source: NBS of the PRC, 2021).
4.2 Model Input
The important parameters of the model are set, as shown in Table 4.
In addition to the above parameters, the dock-loading efficiency is affected by the operation efficiency of the quay crane and the number of quay cranes, and the number of quay cranes is related to the length of the ship. Therefore, the dock-loading efficiency needs to consider the actual terminal equipment and ship conditions. In addition, to set the loading efficiency, the study determines the revised content of the container ship design ship size in the “Code for General Plane Design of Seaports” issued by the Ministry of Communications and the actual situation of Xiamen Port’s container terminal, as shown in Table 5.
4.3 Feasibility analysis of port shore power
Solving the relational model, if Δ>0, for some ships that meet certain conditions, the use of shore power can achieve carbon emission reduction, and the larger the value of Δ is, the better the carbon emission reduction effect of using shore power. Therefore, regarding Δ as an indicator for determining the cleanliness of port shore power, combined with the current situation of the actual power energy structure in China, a reasonable assumption is made for the case.
Chinese statistics in 2021 show that coal power accounts for nearly 90% of thermal power generation, oil power accounts for a very low proportion, and photovoltaic power generation accounts for more than 99% of solar power generation. Therefore, in this case study, assuming that coal power and gas power account for 90% and 10% of thermal power generation, respectively, and photovoltaic power generation is used for solar power generation, the correlation model solution results can be obtained (see Appendix A for detailed data). Through the analysis of the solution results, under the current power energy structure, the marginal carbon emission reduction benefits of Tianjin, Hebei, Liaoning, Shandong, Jiangsu, Shanghai and Zhejiang are all negative. Among the 11 coastal port provinces and cities in China, only the ports in Fujian, Guangdong, Guangxi and Hainan are suitable for using shore power.
Although China is still dominated by thermal power generation, with the development of clean energy, the proportion of coal power and even thermal power will gradually decrease in the future. Therefore, in the case of widespread use of clean energy in the future, it can be foreseen that the promotion of shore power is imperative. However, the current national policy of mandatory use of shore power is not applicable to provinces and cities whose current power energy structure is biased toward thermal power.
4.4 Carbon emission reduction effect and condition analysis of shore power
The feasibility analysis of shore power in provinces and cities with coastal ports shows that the power energy structure of Fujian, Guangdong, Guangxi and Hainan is suitable for the use of shore power. Therefore, this case study selects Haikou Port and Xiamen Port as cases, taking the container ship Taking as the object (assuming that the ship is fully loaded). Considering the relationship between the loading efficiency and ship size, the relationship between the specific carbon emission reduction effect of the shore power and the size of the ship is analyzed, and the results are shown in Figure 2.
In Figure 2, under the same loading efficiency, the reduction in carbon emissions of ships using shore power is positively correlated with the size of the ship. Overall, large ships are more suitable for using shore power than small ships. In addition, for ships of the same size, the cleaner the power energy structure at the port of call is, the better the carbon emission reduction effect of using shore power.
According to the analysis of the carbon emission reduction effect, only ships that meet certain conditions are suitable for using shore power. For the policy of mandatory use of shore power, this case study also examines the basic berthing time (when the actual berthing time of the ship exceeds this value, the carbon emission of the ship using shore power begins to be lower than that of using fuel).
According to the calculation formula of T* , the basic berthing time is not related to the size of the ship. Under the same power energy structure, the basic berthing time is a fixed value. In addition, ports in each area have different basic berthing times for shore power use due to their different power energy structures. The cleaner the power energy structure of a port is, the lower the requirements for its basic berthing time. In the case study, in Haikou Port, ships with a berthing time of more than 42.99 hours are suitable for using shore power; in Xiamen Port, ships with a berthing time of more than 16.41 hours can use shore power.
5 Conclusion and policy implications
5.1 Conclusion
This paper focuses on the emission reduction effect of shore power from the perspective of power traceability. Although shore power is an effective means to reduce ship-berthing carbon emissions, it is not applicable to all areas. The study establishes a shore power–fuel carbon emission correlation model and studies the feasibility of using shore power in the ports of coastal provinces and cities under the current traceability of power sources. In addition, we analyze the carbon emission reduction effect from shore power of specific ports. Finally, according to the national policy of compulsory use of shore power, the conditions and restrictions for ships calling at ports to use shore power are examined. The paper draws the main conclusion as follows: At present, most provinces and cities in coastal cities mainly rely on thermal power generation, but the proportion of clean energy varies. Among them, Guangxi accounts for almost half of the clean energy; Guangdong has a certain proportion of hydropower and nuclear power generation; Fujian and Hainan account for approximately 1/5 of nuclear power generation; and other coastal provinces and cities still have more than 70% thermal power generation. The feasibility calculation through the carbon emission correlation model shows that the marginal carbon emission reduction benefits of shore power in most ports are negative. Only four ports, Fujian, Guangdong, Guangxi and Hainan, are suitable for using shore power because of their relatively clean power energy structure. However, it is also related to the berthing time of the ship; that is, only when the berthing time of the ship exceeds the basic berthing time of using shore power at the port of call is the use of shore power related to lower net carbon emissions. Therefore, the basic berthing time of shore power use can be used as a condition for determining whether a ship is suitable for using shore power at the port of call, which has nothing to do with the size of the ship. In addition, the cleaner the power energy structure of the port area is, the higher the marginal carbon emission reduction benefit of the shore power system, and the lower the berthing time requirements for ships suitable for using shore power.
5.2 Policy implications
At present, most policies for shore power in China are methods of encouragement and support. Mandatory measures on berthing time ignore the differences in the power energy structure of ports in various areas. Therefore, this paper proposes suggestions for the promotion of shore power according to local conditions from the perspective of power traceability:
Different laws and policies should be formulated and implemented in accordance with the power energy structure, economic development level, port resources, etc., of different areas. Compulsory measures should be implemented for coastal ports in Fujian, Guangdong, Guangxi and Hainan. When the size of the ship and the loading and unloading time exceed a certain value, the responsible subject and reward and punishment measures for using shore power are clarified. Relevant laws and regulations should be formulated and improved according to the actual situation. At the same time, financial subsidies and support should be increased, the idle rate of shore power berths should be reduced, and the development of shore power should be ensured. For areas where the current use of shore power is still not clean enough, the use of shore power should not be mandatory for the time being.
Finally, there are some limitations in this paper. For example, the model does not fully consider the influence of practical factors and only studies the single ship type of coastal area and container. And to achieve China’s carbon peaking and carbon neutrality goals, the promotion of shore power is the inevitable trend of port development in the future, the future can be from inland areas and other ship form further in-depth study, but also to realize the energy revolution is a long process, each region should fully consider its electricity clean degree to shore power promotion policy and planning, at the same time, our country should actively develop new energy technologies, gradually increase the proportion of clean energy, so as to fundamentally make the shore power to achieve zero emissions, create a green, safe, economical and new port environment.
Data availability statement
The original contributions presented in the study are included in the article/Supplementary Material. Further inquiries can be directed to the corresponding author.
Author contributions
LS:Conceptualization, Writing - Review & Editing. PD:Conceptualization, Investigation, Writing - Original Draft. YX:Methodology, Formal analysis, Investigation. WL:Supervision. ZH: Resources. All authors contributed to the article and approved the submitted version.
Funding
This research was funded by the National Key R&D Program of China, grant number 2022YFF0903403.
Conflict of interest
The authors declare that the research was conducted in the absence of any commercial or financial relationships that could be construed as a potential conflict of interest.
Publisher’s note
All claims expressed in this article are solely those of the authors and do not necessarily represent those of their affiliated organizations, or those of the publisher, the editors and the reviewers. Any product that may be evaluated in this article, or claim that may be made by its manufacturer, is not guaranteed or endorsed by the publisher.
Supplementary material
The Supplementary Material for this article can be found online at: https://www.frontiersin.org/articles/10.3389/fmars.2022.1077289/full#supplementary-material
References
Abhyankar N., Lin J., Liu X., Sifuentes F. (2020). Economic and environmental benefits of market-based power-system reform in China: A case study of the southern grid system, resources. Conserv. Recycling. 153, 104558. doi: 10.1016/j.resconrec.2019.104558
Adamo F., Andria G., Cavone G., Capua C. D., Lanzolla A. M. L., Morello R., et al. (2014). Estimation of ship emissions in the port of taranto. Measurement. 47, 982–988. doi: 10.1016/j.measurement.2013.09.012
Cariou P., Parola F., Notteboom T. (2019). Towards low carbon global supply chains: A multi-trade analysis of CO2 emission reductions in container shipping. Int. J. production economics. 208, 17–28. doi: 10.1016/j.ijpe.2018.11.016
CEC of the PRC (2020). China Power industry annual development report (Beijing: China Building Materials Industry Press).
Chen Y., Wang H., Yan Z., Xu X., Zeng D., Ma B. (2022). A two-phase market clearing framework for inter-provincial electricity trading in Chinese power grids. Sustain. Cities Society. 85, 104057. doi: 10.1016/j.scs.2022.104057
Chen J., Zheng T., Garg A., Xu L., Li S., Fei Y. (2019). Alternative maritime power application as a green port strategy: barriers in china. J. Cleaner Prod. 213, 825–837. doi: 10.1016/j.jclepro.2018.12.177
CPNN (2022). Green transformer has excellent performance and good prospects. Available at: https://www.cpnn.com.cn/news/tdftzh/202201/t20220118_1476330.html.
Dai L., Hu H., Wang Z. (2020). Is shore side electricity greener? an environmental analysis and policy implications. Energy Policy. 137, 111144. doi: 10.1016/j.enpol.2019.111144
Dai L., Hu H., Wang Z., Shi Y., Ding W. (2019). An environmental and techno-economic analysis of shore side electricity. Transportation Res. Part D: Transport Environment. 75, 223–235. doi: 10.1016/j.trd.2019.09.002
Department of Energy Statistics (2013). “National bureau of statistics,” in China Energy statistical yearbook. (Beijing: China Statistics Press).
Ding W. Y., Wang Y. B., Dai L., Hu H. (2020). Does a carbon tax affect the feasibility of Arctic shipping? Transportation Res. Part D-Transport environment. 80, 102257. doi: 10.1016/j.trd.2020.102257
Gao D., Kwan T. H., Dabwan Y. N., Hu M., Hao Y., Zhang T., et al. (2022). Seasonal-regulatable energy systems design and optimization for solar energy year-round utilization☆. Appl. Energy. 322, 119500. doi: 10.1016/j.apenergy.2022.119500
Gutierrez-Romero J. E., Esteve-Perez J., Zamora B. (2019). Implementing onshore power supply from renewable energy sources for requirements of ships at berth. Appl. Energy. 255, 113883. doi: 10.1016/j.apenergy.2019.113883
He W., Chen R., Li T., Hu B., Tian Y., Meng W. (2020). Comparative study on environmental benefits of using low-sulphur oil and shore power technology for ship berthing. E3S Web Conferences. 145, 2010. doi: 10.1051/e3sconf/202014502010
Ji C. X., El-Halwagi M. M. (2021). A data-driven study of IMO compliant fuel emissions with consideration of black carbon aerosols. Ocean Engineering. 218, 108241. doi: 10.1016/j.oceaneng.2020.108241
Kalikatzarakis M., Geertsma R. D., Boonen E. J., Visser K., Negenborn R. R. (2018). Ship energy management for hybrid propulsion and power supply with shore charging. Control Eng. Practice. 76, 133–154. doi: 10.1016/j.conengprac.2018.04.009
Kotrikla A. M., Lilas T., Nikitakos N. (2017). Abatement of air pollution at an Aegean island port utilizing shore side electricity and renewable energy. Mar. Policy 75, 238–248. doi: 10.1016/j.marpol.2016.01.026
Kumar J., Kumpulainen L., Kauhaniemi K. (2019). Technical design aspects of harbour area grid for shore to ship power: State of the art and future solutions. Int. J. Electrical Power Energy Systems. 104, 840–852. doi: 10.1016/j.ijepes.2018.07.051
Kumar Y., Ringenberg J., Depuru S. S., Devabhaktuni V. K., Lee J. W., Nikolaidis E., et al. (2016). Wind energy: Trends and enabling technologies. Renewable Sustain. Energy Rev. 53, 209–224. doi: 10.1016/j.rser.2015.07.200
Lathwal P., Vaishnav P., Morgan M. G. (2021). Environmental and health consequences of shore power for vessels calling at major ports in India. Environ. Res. Letters. 16, 064042. doi: 10.1088/1748-9326/ABFD5B
Moreno-Gutierrez J., Pajaro-Velazquez E., Amado-Sanchez Y., Rodríguez-Moreno R., Calderay-Cayetano F., Durán-Grados V. (2018). Comparative analysis between different methods for calculating on-board ship's emissions and energy consumption based on operational data. Sci. Total Environment. 650, 575–584. doi: 10.1016/j.scitotenv.2018.09.045
MOT of the PRC (2021). Port and ship shore power management measures. (the Ministry of Transport). Available at: https://xxgk.mot.gov.cn/2020/jigou/fgs/202109/t20210922_3619619.html.
Nguyen P. N., Woo S. H., Kim H. (2022). Ship emissions in hotelling phase and loading/unloading in southeast Asia ports. Transportation Res. Part D-Transport Environment. 105, 103223. doi: 10.1016/j.trd.2022.103223
Peng Y., Dong M., Li X., Liu H., Wang W. (2020). Cooperative optimization of shore power allocation and berth allocation: A balance between cost and environmental benefit. J. Cleaner Production. 279, 123816. doi: 10.1016/j.jclepro.2020.123816
Peng Y., Li X., Wang W., Wei Z., Bing X., Song X. (2019). A method for determining the allocation strategy of on-shore power supply from a green container terminal perspective. Ocean Coast. Management. 167, 158–175. doi: 10.1016/j.ocecoaman.2018.10.007
Qi J., Wang S., Peng C. (2020). Shore power management for maritime transportation: Status and perspectives. Maritime Transport Res. 1, 100004. doi: 10.1016/j.martra.2020.100004
Sharmina M., Edelenbosch O.Y., Wilson C., Freeman R., Gernaat D., Gilbert P., et al. (2021). Decarbonising the critical sectors of aviation, shipping, road freight and industry to limit warming to 1.5–2°C. Climate Policy . 21:4, 455–474. doi: 10.1080/14693062.2020.1831430
Styhre L., Winnes H., Black J., Lee J. Y., Le-Griffin H. (2017). Greenhouse gas emissions from ships in ports – case studies in four continents. Transportation Res. Part D: Transport Environment. 54, 212–224. doi: 10.1016/j.trd.2017.04.033
Sun L., Cui H., Ge Q. (2022). Will China achieve its 2060 carbon neutral commitment from the provincial perspective? Adv. Climate Change Res. 13, 169–178. doi: 10.1016/j.accre.2022.02.002
Sundaram A. (2022). Multiobjective multi verse optimization algorithm to solve dynamic economic emission dispatch problem with transmission loss prediction by an artificial neural network. Appl. Soft Computing 124, 109021. doi: 10.1016/j.asoc.2022.109021
Tang R., Wu Z., Li X. (2018). Optimal operation of photovoltaic/battery/diesel/cold-ironing hybrid energy system for maritime application. Energy. 162, 697–714. doi: 10.1016/j.energy.2018.08.048
Tan Z.J., Liu Q.R., Song J.B., Wang H., Meng Q. (2021). Ship choice and shore-power service assessment for inland river container shipping networks. Transportation Res. Part D-Transport Environment. 94, 102805. doi: 10.1016/j.trd.2021.102805
Tseng P. H., Pilcher N. (2015). ). a study of the potential of shore power for the port of kaohsiung, Taiwan: To introduce or not to introduce? Res. Transportation Business Management. 17, 83–91. doi: 10.1016/j.rtbm.2015.09.001
Wang H. (2022). The average utilization rate of shore power is less than 20%. CPPCC DAILY 2022-04-21, 006. doi: 10.28660/n.cnki.nrmzx.2022.003017
William J. H. (2010). Assessment of CO2 and priority pollutant reduction by installation of shoreside power. Resources Conserv. Recycling 54 (7), 462–467. doi: 10.1016/j.resconrec.2009.10.002
Winkel R., Weddige U., Johnsen D., Hoen V., Papaefthimiou S. (2016). Shore side electricity in Europe: Potential and environmental benefits. Energy Policy. 88, 584–593. doi: 10.1016/j.enpol.2015.07.013
Wu L., Wang S. (2020). The shore power deployment problem for maritime transportation. Transportation Res. Part E: Logistics Transportation Rev. 135, 101883. doi: 10.1016/j.tre.2020.101883
Wu Z., Ye X., Xing M. (2013). Discussion on key technology of ship shore power. Electrotechnical Application 32, 22–26+60.
Xing H., Spence S., Chen H. (2020). A comprehensive review on countermeasures for CO2 emissions from ships. Renewable Sustain. Energy Rev. 134, 110222. doi: 10.1016/j.rser.2020.110222
Xu C., Huang Z. (2018). A dual-functional wave-power plant for wave-energy extraction and shore protection: A wave-flume study. Appl. Energy 229, 963–976. doi: 10.1016/j.apenergy.2018.08.005
Yin M., Wang Y., Zhang Q. (2020). Policy implementation barriers and economic analysis of shore power promotion in China. Transportation Res. Part D: Transport Environment. 87, 102506. doi: 10.1016/j.trd.2020.102506
Zhang Y. (2022). Strive to compose the chapter of water transport of transportation power. China Water Transport News 001, 2. doi: 10.28142/n.cnki.ncsyb.2022.000860
Zhang O., Yu S., Liu P. (2015). Development mode for renewable energy power in China: Electricity pool and distributed generation units. Renewable Sustain. Energy Rev. 44, 657–668. doi: 10.1016/j.rser.2015.01.020
Keywords: shore power, power source, carbon emissions, power energy structure, actual emission reduction effect
Citation: Sun L, Ding P, Xiong Y, Liu W and Hu Z (2022) Carbon emission reduction of shore power from power energy structure in China. Front. Mar. Sci. 9:1077289. doi: 10.3389/fmars.2022.1077289
Received: 22 October 2022; Accepted: 15 November 2022;
Published: 30 November 2022.
Edited by:
Jihong Chen, Shenzhen University, ChinaReviewed by:
Liupeng Jiang, Hohai University, ChinaMengli Xiao, East China University of Science and Technology, China
Copyright © 2022 Sun, Ding, Xiong, Liu and Hu. This is an open-access article distributed under the terms of the Creative Commons Attribution License (CC BY). The use, distribution or reproduction in other forums is permitted, provided the original author(s) and the copyright owner(s) are credited and that the original publication in this journal is cited, in accordance with accepted academic practice. No use, distribution or reproduction is permitted which does not comply with these terms.
*Correspondence: Pantong Ding, ZHB0OTkwMTA1QDE2My5jb20=