- 1Guangzhou Marine Geological Survey, China Geology Survey, Guangzhou, China
- 2Southern Marine Science and Engineering Guangdong Laboratory (Guangzhou), Guangzhou, China
- 3South China Sea Institute of Oceanology, Chinese Academy of Sciences, Guangzhou, China
Macrobenthos play an important role in global biogeochemical cycles and carbon energy exchange. Macrobenthic communities on continental shelves are alimented through the sedimentation of organic material. Sedimentary organic carbon is the key step for the carbon cycling within the continental shelf benthic trophic web. However, the quantitative relationship between the macrobenthos and sediments based on total organic carbon (TOC), and the trophic pathways of macrobenthos applied by stable carbon and nitrogen isotope data (δ13C and δ15N) on the continental shelf are still limited. Therefore, the continental shelf of the northern South China Sea (NSCS), with abundant macrobenthos, was chosen as the research area. In this paper, TOC, total nitrogen (TN), δ13C and δ15N of the macrobenthos and sediments, the eco-exergy (Ex), structural eco-exergy (Exst) of macrobenthos communities of the NSCS were analysed. The TOC and TN in macrobenthos varied widely between 1.05% and 71.08% and between 0.29% and 11.36%, respectively, which were much higher than those in sediments. Trophic pathways (food sources and trophic levels) were established by applying δ13C and δ15N values of the main macrobenthic species. The similar stable isotope ratios and the trophic levels of most macrobenthos (2.0–3.4) might indicate similar food compositions and living habits. Eco-exergy grades were positively correlated with the average trophic level value of macrobenthos (correlation coefficient R=0.79). The relationship between the Ex value and the weight of required sediments estimated by the average organic carbon showed a typical positive correlation (R=0.98). This establishment of quantitative relationship provides a useful method for evaluating the structural functions of the ecosystem and researching the carbon sequestration potential of the macrobenthos community.
Introduction
Benthic communities on continental shelves are alimented through the sedimentation of organic material by pelagic primary and secondary production in the euphotic zone (Loc'H et al., 2008). In particular, in the coastal upwelling area of a continental shelf, the benthic ecosystem is influenced by the sedimentation of rich organic material. At present, studies on the structure, function and energy flow of benthic ecosystems on continental shelves have mainly focused on the spatial variability in production regimes and biodiversity structures (Xu et al., 2019; Feng et al., 2021). However, the relationship between macrobenthic carbon and organic carbon in sediments, and the trophic pathways of macrobenthos applied by organic carbon isotope on the continental shelf are still limited.
Macrobenthos usually refers to an ecological group consisting of all or most of the organisms living on the surface of the seafloor or sediments that cannot pass through a 0.5 mm pore screen. Macrobenthos are widely distributed in various habitats ranging from intertidal zones to the deep sea, including wetlands, seagrass beds, estuaries, deep-sea hydrothermal vents and cold springs. The diversity of habitats contributes to the existence of various types of benthic organisms, which is important to the global marine biogeochemical cycle (Lewis, 1996; Castro and Huber, 2011). On the one hand, macrobenthos play a significant role in aquatic-benthic coupling and biogeochemical cycling of elemental C, N, P and S through biological sedimentation and disturbance. The existence of a macrobenthos community accelerates the decomposition of submarine debris and regulates material exchange at the mud-water interface (Liu et al., 2015). On the other hand, the community structure, diversity pattern and biomass changes in benthic organisms determine the development of larvae of large invertebrates, which has been considered as a crucial link in energy research on the food web (Pilditch et al., 2015). Furthermore, macrobenthos vitalize the food chain and boost the energy flow and material cycle in marine ecosystems. The organic debris deposited in the water layer can be fully utilized by benthic organisms, and the decomposition of nutrients is promoted at the same time. The trophic pathways (food sources and trophic levels) here can be established by determining the δ13C and δ15N of the main benthic species.
In recent decades, the ocean carbon cycle has increasingly attracted attention in the research field of the global biogeochemical cycle. Studies have shown that approximately half of anthropogenic carbon dioxide emissions are absorbed by the ocean every year. Carbon dioxide is transformed into different compounds of carbon through complex biogeochemical processes. Marine organisms are important carriers in the movement and storage of carbon in the ocean (Feely et al., 2004). As the key component in biogeochemical processes, marine organisms not only represent a component of organic carbon debris but are also important producers. Upper-layer organisms, represented by plankton, produce organic carbon debris by feeding-metabolism process sinks. Some of this debris is utilized by macrobenthic organisms and converted into macrobenthic carbon. If the relationship between the macrobenthos and sediments based on TOC can be established through a relatively simple and operable ecosystem energy balance model, it can provide a scientific research basis and practical technical approach for the study of the ocean carbon cycle.
Eco-exergy estimation and classification based on the thermodynamic equilibrium conditions of ecosystems can be used in such an energy balance model of ecosystems (Jørgensen and Nielsen, 2007). Eco-exergy theory derives from thermodynamic concepts (Jørgensen, 2002). It is assumed that the coupled air-water-sediment system consists of part of the water inside the system and the surrounding environment under thermodynamic equilibrium (Figure 1). When a certain amount of organic matter enters the system from the external environment, the system will be separated from the surrounding environment with the previous equilibrium. Eco-exergy is considered the total amount of work done by an ecosystem from the initial assumed state to the state of thermodynamic equilibrium with its surrounding medium. This amount is derived from biochemical energy and information contained in the organism, which can be used to measure the process of energy conversion, utilization and transmission. The energy required to maintain the thermodynamic equilibrium of the macrobenthic ecosystem in the study area (kJ/m2) can be estimated by eco-exergy indicators (Tang et al., 2018). The weighting factor (β) is a key factor for estimating the values of Ex and Exst. It accounts for the information that the organisms carry in the macrobenthos. However, there are various ways to determine the β value, such as the trophic groups, coding gene content for broad taxonomic/ecological groups, and genome size. In this study, the β value was estimated by the number of nucleotides and the number of duplicated genes. Similarly, the carbon energy needed to support the macrobenthic ecosystem can be estimated by the conversion of the biocarbon content, benthic secondary productivity and ecological efficiency. Most of this carbon energy comes from carbon in the food chain, i.e., carbon obtained from organic debris in sediments and the upper water column. The relationship among these components can be established from the perspective of energy flow and balance. Therefore, we introduce the thermodynamic equilibrium hypothesis and eco-exergy theory to establish the relationship between macrobenthos and sediments based on organic carbon and then estimate the weight of organic carbon debris needed to support the energy balance of benthic ecosystems (Figure 1).
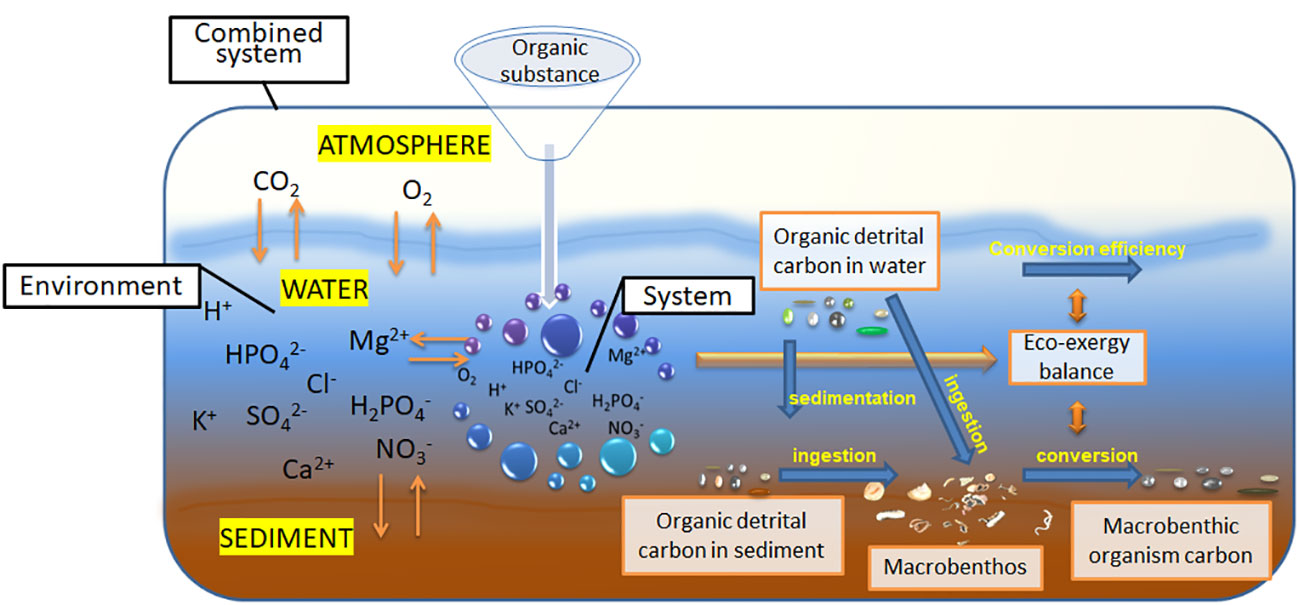
Figure 1 The relationship between macrobenthic carbon and organic carbon in sediments under the hypothetical system of thermodynamic equilibrium.
The aims of this study include the following: i. to discuss the difference between the organic carbon contents in the macrobenthos and sediments; ii. to establish trophic pathways (food sources and trophic levels) by determining the δ13C and δ15N of the main macrobenthic species in the NSCS; and iii. to find the quantitative relationship between macrobenthic carbon and organic carbon in sediments. The findings of this work will provide new ideas and useful supplements for the further study of the carbon energy flow and balance between systems.
Materials and methods
Study area
The South China Sea (SCS) is located in southeastern Asia and has an average depth of approximately 1350 m. It is the largest marginal sea in the world, stretching 3.5×106 km2 from 23°57’N in the north to 3°00’N in the south and from 99°10’E in the west to 122°10’E in the east (Chen et al., 2006). The SCS also carries the most frequent human activity in the ocean. The study area, the NSCS, is affected by both the natural environment and year-round human activities. There are many estuaries (such as the Pearl River estuary) that introduce fresh water into the NSCS (Jiang and Wang, 2018).
In the NSCS, two typical continental shelf upwelling areas are located east of Hainan Island, the east of the Leizhou Peninsula and the southeast of Zhanjiang Bay (noted as Qiongdong), and the inshore areas are located from the Shantou Coast to the Nanri Islands of the Fujian Coast (noted as Yuedong) (Jing et al., 2009). Upwelling areas are characterized by low temperature, high salinity and high potential density. It has a significant effect on fisheries and coastal environmental protection in the NSCS (Jing et al., 2009). The summer coastal upwelling serves as a nutrient pump, which is most nourished to marine lives in the middle of July (Jiang and Wang, 2018). The broad continental shelf in the northwestern and southern areas of the SCS not only receives a large amount of clastic sediments from land and islands but also supports abundant marine lives, resulting in a high carbonate content in the surface sediments.
Sample collection and analysis
This study was carried out during the first cruise by Sun Yat-sen University in 2016 in the NSCS, from the Pearl River estuary down to the edge of the NSCS (Figure 2). The 11 sampling sites in the NSCS were at different depths (13 m–76 m). In this area, two typical upwelling areas (Qiongdong and Yuedong) and shelf areas near the Pearl River Delta have abundant macrobenthos. Sediment was sampled using a stainless-steel box corer, and only the upper 5 cm of each sample was packed in polyethylene bags and frozen at -20°C until experimental analysis was performed.
Macrobenthos samples were collected using a stainless-steel box corer in 2016. The sediment samples were chosen with a 10 cm *10 cm *15 cm steel box and filtered through a 65-mesh sieve. Later, macrobenthos samples were sorted, measured, weighed and then stored frozen (-20°C) until further onboard processing. The macrobenthos samples included many kinds of macroinvertebrates, such as crabs, shrimps, echinoderms and polychaetes. The macrobenthos samples were washed and freeze-dried for later study. Part of the macrobenthos samples was ground into fine and homogeneous powder and tested to determine TN content and nitrogen isotopes. Some crabs, shrimps, echinoderms and polychaetes were acidified with 1 mol/L hydrochloric acid to remove the carbonate, and the macrobenthos samples were then rinsed with deionized water and freeze-dried. Finally, approximately 0.5 mg of powdered macrobenthos samples was tested to determine the TOC content and δ13C.
Experimental methods
The sediment samples for the elemental and stable isotopic analyses were also freeze-dried, homogenized and ground into powder. The sediment samples were divided into two subsamples. One subsample for the TOC and δ13C analyses was treated with 4 mol/L hydrochloric acid to remove carbonate, subsequently rinsed with deionized water (6–8 times), and then freeze-dried. The other subsample was used for TN and δ15N analyses, and the bulk samples were not acidified before analysis.
The TOC and TN contents of the samples, including the macrobenthos samples and surface sediment samples, were determined by a Delta Plus Advantage isotope ratio mass spectrometer. δ13C and δ15N were measured by a Delta Plus Advantage isotope ratio mass spectrometer after the removal of the carbonate and drying at the laboratory in the South China Sea Institute of Oceanology, China Academy of Sciences. The results are reported in parts per mil (‰):
where δ (‰) is δ13C (‰) or δ15N (‰); Rsample and Rreference are the isotopic ratios of the benthos and surface sediment samples and reference. For carbon, the reference was the Pee Dee belemnite (PDB), and for nitrogen, it was atmospheric nitrogen gas (δ15N = 0‰). The analytical precision was 0.1‰ for δ13C and 0.15‰ for δ15N based on replicate measurements of a reference standard.
As δ15N values provide an indication of the trophic position of a consumer in the food chain, the trophic level was estimated with the following formula:
where δ15Nbase is the nitrogen stable isotope ratio of the primary consumers. In this study, δ15Nbase was the lowest value of δ15N (6.87‰) of the primary consumers (Polychaetes). δ15Nconsumer is the nitrogen stable isotope ratio of the consumers, and δ15N is the enrichment value in the process of trophic grade transfer (the average value is approximately 3.4‰) (Post, 2002).
Key formulas for calculation
Eco-exergy indicators include the eco-exergy (Ex) and structural eco-exergy (Exst) indicators:
where Ex (kJm-3) is the total eco-exergy of a community; K is the total number of components selected; βk is a conversion factor for component k (Park et al., 2001; Jørgensen, 2002; Ludovisi and Poletti, 2003); Ck is the concentration of component k, which is calculated with the biomass per unit of volume or area; Exst (kJg-1) is the total structural eco-exergy of a community, and Ct is the total concentration or biomass of organic components in the system.
Species abundance is a widely used index in the measurement of biodiversity because it is intuitive and easy to calculate. However, the Shannon index, Simpson index and Margalef index are more commonly used in the biodiversity index system, and these indices are often smaller than the species abundance. The commonly used biodiversity indices calculated in this study are as follows (Table 1):
The macrobenthos could be classified according to β-values (Jørgensen et al., 2005) and could also reflect the trophic level of the organism. The β-values of polychaetes, brittle stars, sea urchins, crabs, shrimps, molluscs and demersal fishes in this study were 133, 91, 91, 232, 232, 310 and 499, respectively. To simplify the calculation process, we hypothesize that all the macrobenthos feed on sedimentary organic matter, thus the average organic carbon content of the macrobenthos with the same β-values could be measured. The average organic carbon contents of the macrobenthos were used in formulas (4) and (5), while the required organic carbon content could be obtained by conversion. The required sediment weight in the sampling sites was calculated with the organic carbon content of the sediment:
where Fs is the required sediments to maintain ecological balance (g); Xs is the TOC content in the sediment (%); Xb is the average value of the TOC contents in the macrobenthos (%) according to the β-values; Fb is the dry weight of each macrobenthic organism (g), without bones or shells; and R is the average conversion efficiency, generally approximately 10% (Christensen and Pauly, 1992; Ren et al., 2020).
Linear regression analysis and test based on the least square method (OLS)
OLS was used to investigate the relationships among TOC, TN and the mean grain size in the surface sediments, as shown in Figure 3; the relationship between TOC and TN in the macrobenthos and TOC and TN in the surface sediments, as shown in Figure 4; and the relationship between Ex and the weight of required sediments using the average TOC content of macrobenthos, as shown in Figure 5. We used Origin 9.0 software to draw Figures 3–5 in the OLS regression method and then used Adobe Photoshop 10.1 for postprocessing.
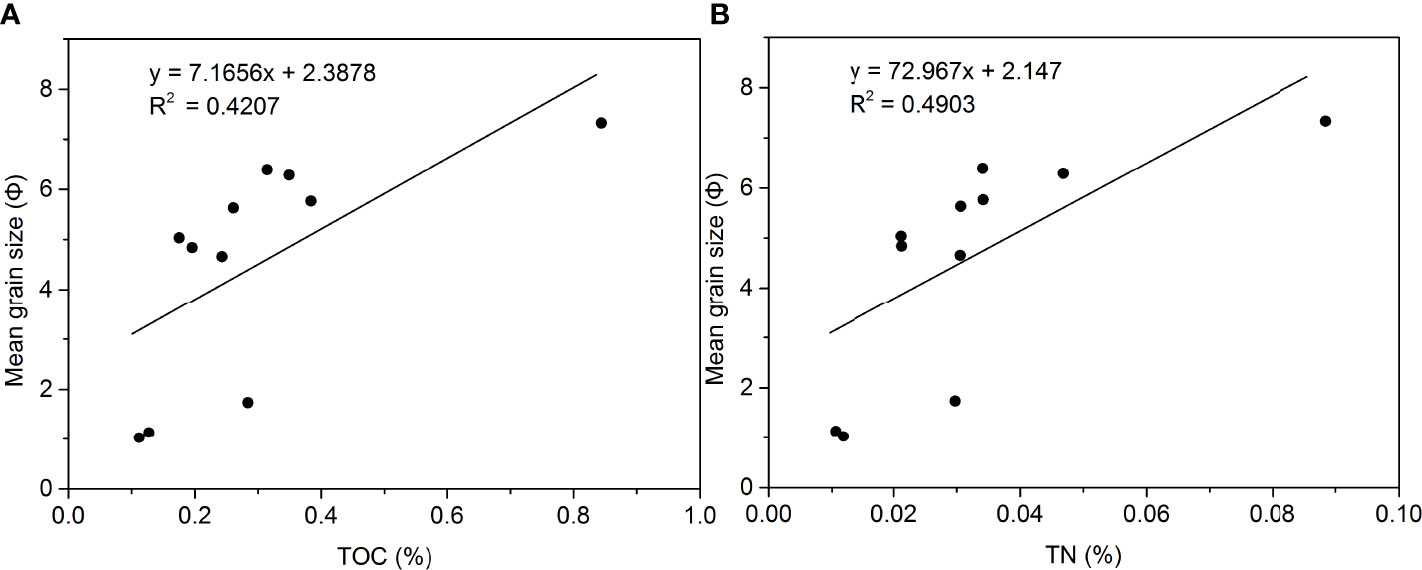
Figure 3 (A) Regression between TOC and mean grain size in sediments from the NSCS; (B) regression between TN and mean grain size in sediments from the NSCS.
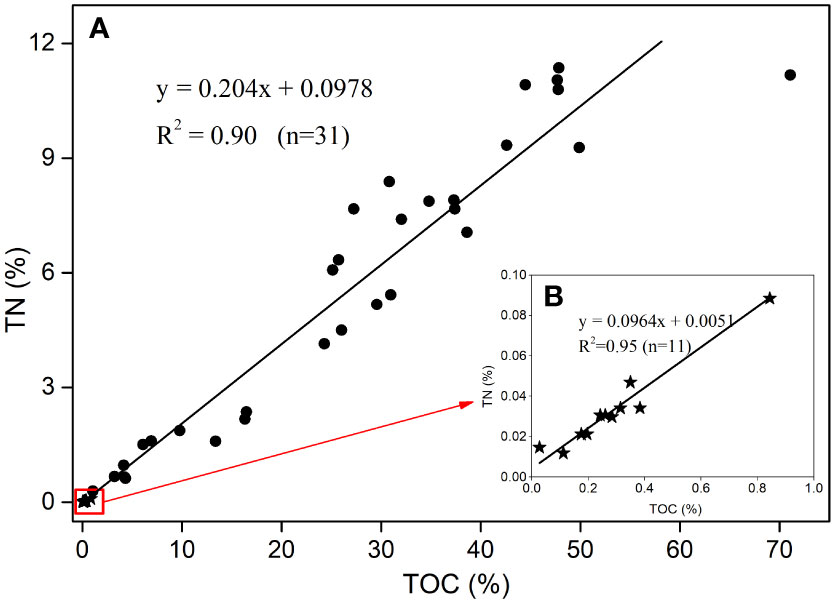
Figure 4 The differences between TOC and TN in the macrobenthos (black dots) and TOC and TN in sediments (black stars) from the NSCS. (A) Regression between TOC and TN in the macrobenthos; (B) regression between TOC and TN in sediments (after enlarging the red square).
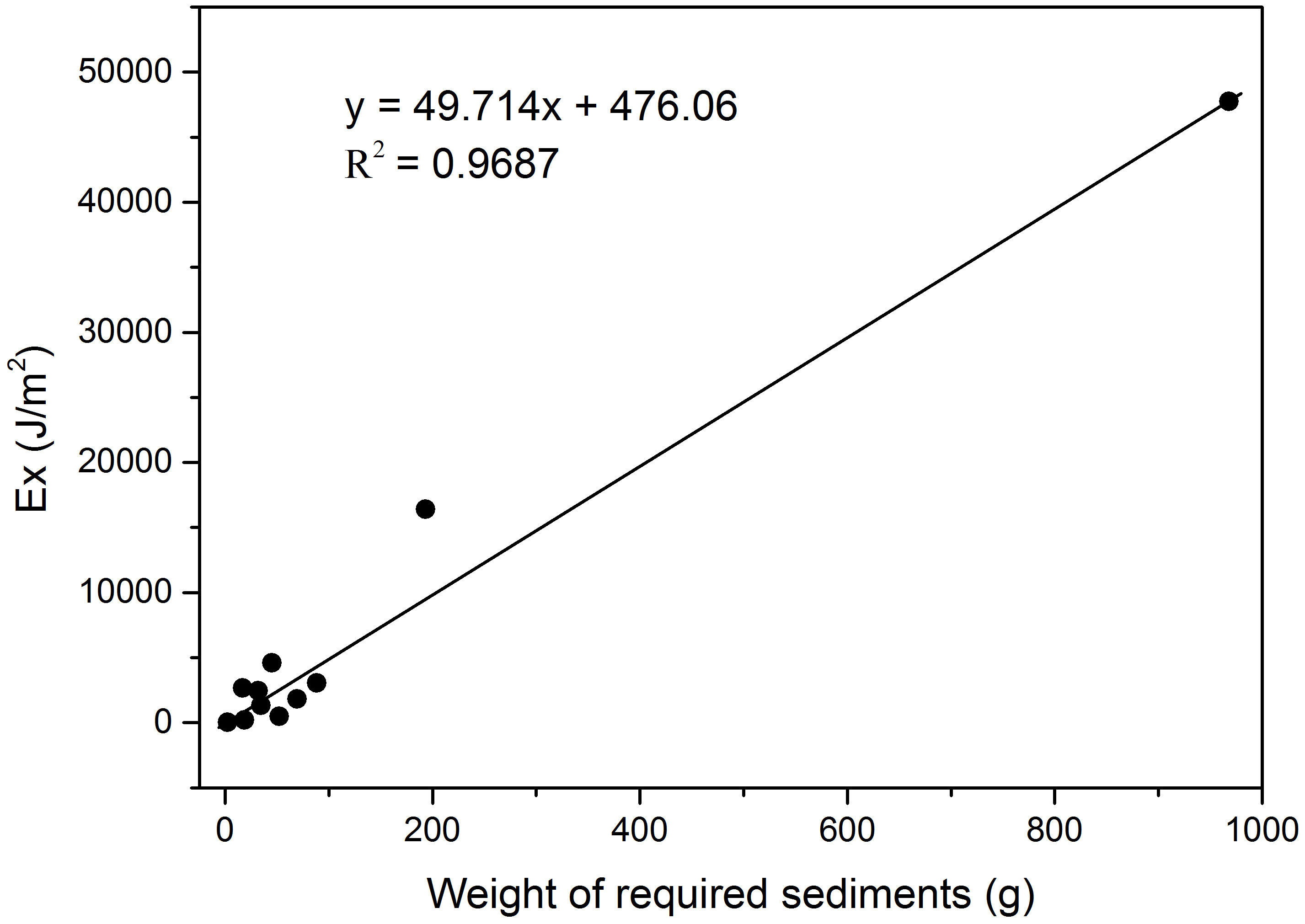
Figure 5 The relationship between Ex and the weight of required sediments using the average TOC content of macrobenthos at the 11 sampling sites.
Results
Characteristics of the carbon parameters of macrobenthic organisms
Five carbon/nitrogen parameters were also detected in 4 species of the 31 biological individuals: the TOC, TN, δ13C, δ15N and TOC/TN ratios. The contents of TOC and TN in the macrobenthic organisms ranged from 1.05% to 71.08% and from 0.29% to 11.36%, with average values of 27.01% and 5.61%, respectively. The δ13C values ranged from -16.68 ‰ to -21.85‰, and the δ15N values ranged from 6.97‰ to 14.53‰, with mean values of -19.37‰ and 9.59‰, respectively. The TOC/TN ratios were between 3.55 and 6.97, with a mean value of 5.10.
Characteristics of the carbon parameters in the surface sedimentary environment
Five carbon/nitrogen parameters were detected in more than 6 sections of the surface sediment samples: the TOC, TN, δ13C, δ15N and TOC/TN ratios. The grain size parameters of the surface sediments were also detected at the corresponding stations.
The TOC and TN contents in the sediments were between 0.11% and 0.84% and between 0.011% and 0.088%, with average values of 0.30% and 0.033%, respectively. Similarly, the contents of δ13C and δ15N ranged from -25.6‰ to -21.4‰ and from 4.58‰ to 7.48‰, with average values of -22.61‰ and 6.07‰, respectively. TOC/TN ranged from 7.46 to 11.98, with an average value of 9.31.
The results of grain size analysis showed that the surface sediment grains in the NSCS could be divided into those mainly consisting of sand, silt, and clay, with relatively low gravel content. The mean grain size (Mz) in the sediments of the NSCS was between 1.03Φ and 7.33Φ, with an average value of 4.53Φ.
Trophic levels of macrobenthos
The trophic levels of most macrobenthos on the northern shelf of the SCS ranged from 2.0 to 4.4, and the trophic levels of most macrobenthos were between 2.0 and 3.4. The average trophic levels in sampling sites B1, E3, F2, G4, H2, H4, I2 and I2-A were 2.81, 2.57, 2.75, 2.75, 2.87, 4.22, 2.74 and 2.55, respectively. The trophic levels of fish (2.8), crabs (2.7), shrimp (2.4–3.0), molluscs (2.4–3.0), most polychaetes (2.4–2.9) and some brittle stars (2.8) were similar. Different macrobenthos at different sites had similar trophic levels as a result of food sources similar to those found by other researchers (Loc'H et al., 2008; Kiyashko et al., 2014). The trophic levels of most of the brittle stars (2.0–2.1) and one polychaete (2.0) were low. However, the trophic levels of a few polychaetes had high values of 4.4 and 4.2, which might be related to the consumption of bacteria with relatively high δ15N values. The trophic level of sea urchins was high at 3.9 as a result of foods including insoluble sedimentary organic matter with relatively high δ15N values.
Community structure and function
As shown in Figure 6, the eco-exergy indicators and diversity indices of all the stations were estimated and reflected the structure and function of benthos from the perspective of thermodynamics and community structure, respectively (Tang et al., 2015). The results showed that the regional variations in Ex and Exst had different trends from site B1 to site I2-A and from north to south. Figure 6A shows that the Ex values of the macrobenthic communities in H4 and I2 were higher than those in the other sites, which means that Ex is higher in the southern part of the study area (in the area northeast of Hainan Island) than in the northern part (the area near the Pearl River Estuary, Guangdong Province). However, the spatial distribution of Exst (Figure 6A) is different from that of Ex, and the Exst values of sites B1 and F2 are higher than the average value, i.e., the values of Ex and Exst are generally inconsistent in the study area. In addition, the values of the biodiversity indicators basically showed an upward trend from sections B to I, which is the same as the trend of Ex (Figure 6B).
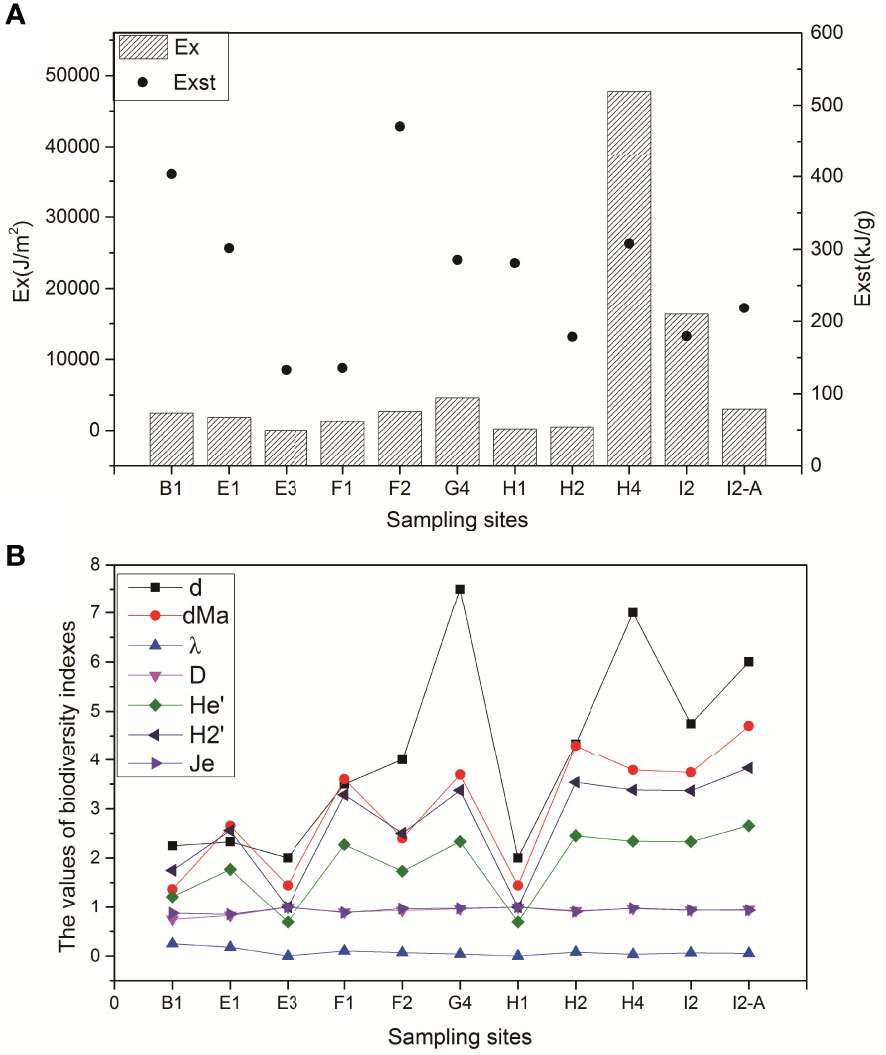
Figure 6 (A) The values of the Ex and Exst indicators at the sampling sites; (B) the values of the biodiversity indicators at the sampling sites in the NSCS.
The weight of eco-exergy balance required sediments
The average TOC contents were assumed to be 29.8%, 4.47%, 1.05%, 29.53%, 36.9%, and 27.23% in polychaetes (133), brittle stars (91), sea urchins (91), crabs (232) and shrimps (232), molluscs (310), and demersal fishes (499), respectively. The required sediment weights of the eleven sites calculated with TOC contents of the macrobenthos ranged widely from 2.2 g to 968.2 g used in formulas (4) and (5) (Figure 7). The required sediment weight of the Site H4 was the highest (968.2g), while the average value of all sites was only 138.2g (Figure 7).
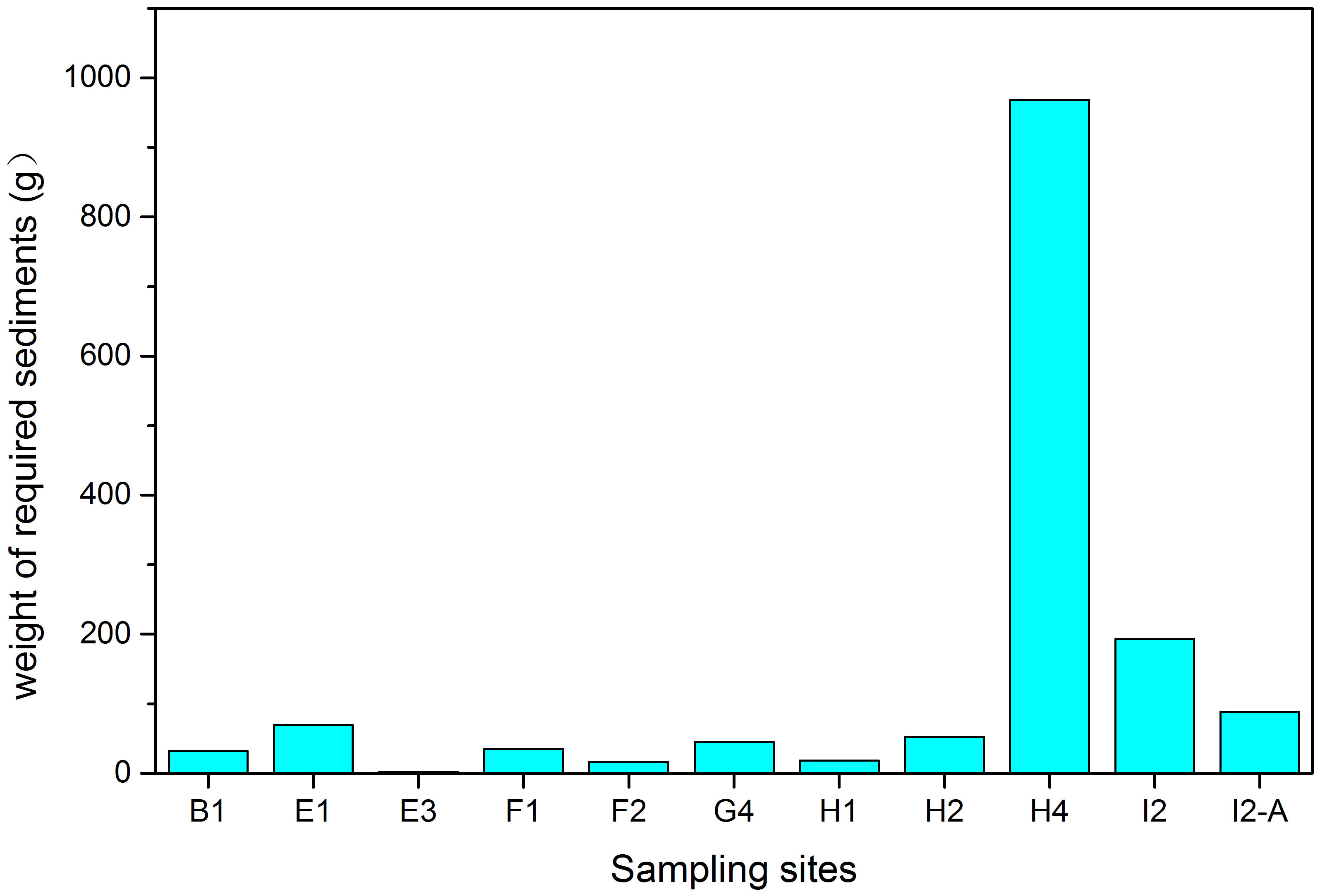
Figure 7 The grams of organic carbon sediments needed were calculated by using the average TOC content of macrobenthos at different sites and then added cumulatively.
Discussion
The differences between TOC and TN in the macrobenthos and TOC and TN in sediments
The organic carbon in the sediments is from dead organisms (Meyers, 1997). The TOC and TN contents in the sediments are positively correlated with the mean grain size (Figure 3), and the correlation coefficients R of the TOC and TN contents with the mean grain size in the surface sediments are 0.65 and 0.70, respectively, indicating that sediment particles can affect the preservation of TOC and TN. However, there are other factors affecting TOC and TN, such as the upper water column environment and biological perturbation. Macrobenthos play crucial roles in the functioning of marine ecosystems, such as the decomposition of organic matter and the transfer and recycling of nutrients within food webs (Xu et al., 2019).
The TOC and TN contents of macrobenthos (such as polychaetes, crabs and shrimps) and their corresponding sediments are shown in Figure 4A. The TOC and TN contents of the macrobenthos are widely different. The TOC contents of the macrobenthos ranged from 1.05% to 50.1%, and the highest TOC content was 71.08%. The TN contents of the macrobenthos ranged from 0.29% to 11.36% (Figure 4A). The TOC and TN contents in the macrobenthos in study area are similar to the TOC (4.1%–51.6%) and TN (0.7%–14.7%) contents in benthic invertebrates on a continental margin in the NW Atlantic (Parzanini et al., 2018). The TOC and TN contents in the macrobenthos are much higher than those in the sediments. The TOC and TN contents of the surface sediments are concentrated similarly in a small range (red square) (Figure 4). The TOC (0.11%–0.84%) and TN (0.01%–0.088%) contents in the surface sediments are close to the TOC (0.06%–1.02%) and TN (0.03%–0.19%) contents in the Pearl River Estuary and on the SCS shelf (Hu et al., 2006), and TOC (0.1%–1.0%) and TN (0.02%–0.15%) contents on the South Yellow Sea shelf (Liu et al., 2020). These values are lower than TOC (0.5%–1.9%) and TN (0.03%–0.2%) in the Mississippi Estuary and on the adjacent shelf (Bianchi et al., 2002), and TOC (1.13–1.97%) and TN (0.14%–0.2%) in the Mackenzie Estuary and on the adjacent shelf (Goni et al., 2000).
The correlation analysis shows that the correlation coefficient R of the TOC and TN contents in the macrobenthos is 0.95 as the confidence level is 0.01 (2-tailed) (Figure 4A). The correlation coefficient R of the TOC and TN contents in the surface sediments is 0.98 with the confidence level of 0.01 (2-tailed) (Figure 4B). There is a positive correlation between the TOC and TN contents in the sediments. This is similar to the results of other studies (Meyers, 1994; Hu et al., 2006; Jia et al., 2013; Liu et al., 2020).
Food sources and trophic levels of macrobenthic ecosystems
The material and energy flow of the food web in macrobenthic ecosystems is key to revealing the structure and function of benthic ecosystems. In the process of macrobenthic metabolism, organisms also tend to utilize light isotopes (12C and 14N) to participate in metabolism, such as respiration and excretion, resulting in the bioaccumulation of heavy isotopes (13C and 15N) (Deniro and Epstein, 1978). Therefore, trophic pathways (food sources and trophic levels) are established by 13C and δ15N of the main macrobenthic species in the aquatic ecosystem, which have been widely used in previous studies (Cai et al., 2002; Carabel et al., 2006; Sokolowski et al., 2012).
The δ13C values (-21‰ ~ -18‰) and δ15N values (7‰ ~ 11‰) of most polychaetes, crabs, shrimps, fishes, brittle stars and molluscs in the study area are shown in Figure 8, and species with similar stable isotope ratios may have similar food compositions and living habits. The δ13C values of macrobenthic organisms in the NSCS show marked similarities with those found in the continental shelf of the Bay of Biscay and in the Yap Trench in the Pacific Ocean. There are also points of similarities between the δ13C values of macrobenthic organisms in the NSCS and those in the Chongming tidal flat, China, and in the Sea of Japan. A significant discrepancy in the δ13C values of macrobenthic organisms indicates that the food composition and carbon flow of the NSCS and those in a continental margin in the Northwest Atlantic may be different (Table 2).
The minimum δ13C values of the macrobenthos in the Chongming tidal flat (-23.6‰) and in the Sea of Japan (-23.9‰) might be related to the input of terrigenous organic matter. The δ13C values of terrigenous organic matter are usually low, ranging from -26‰ to -28‰ (Meyers, 1997). The δ13C of consumers can reflect the δ13C value of food, and the enrichment degree of δ13C is 0–1‰ moving up the food chain (Fanelli et al., 2011). The δ13C value of the macrobenthos is higher than the δ13C value of food, as it accumulates along the food chain. On the other hand, the minimum δ13C value (-20.1‰) of the benthos in the continental margin and marine trench is less affected by terrigenous materials (Guo et al., 2018; Parzanini et al., 2018). The lowest value of the benthos (-21.85‰) in the SCS may be related to the δ13C value (-22.35‰) in the sediments. A similar relationship is also found for the lowest δ13C value in the benthos (-23.9‰) and that (-22.9‰) in sediments and particulate organic matter (POM) (-24.3‰) in the Sea of Japan (Kiyashko et al., 2014).
Significant similarities found in the values of δ15N in the macrobenthos from the NSCS, Chongming tidal flat, continental shelf of the Bay of Biscay, and continental margin in the Northwest Atlantic (Table 2) indicate analogous trophic pathways in these locations. In contrast, the δ15N values (11.9–17.9‰) in the Yap Trench are significantly higher than those in the other locations.
The highest δ15N value (14.53‰) of polychaetes in the NSCS (Figure 8) might be related to the bacteria and other microorganisms and insoluble sedimentary organic matter with relatively high δ15N values absorbed by the polychaetes (Hall and Meyer, 1998; Li et al., 2010). This occurred on the continental margin, similar to the high δ15N values of Harmothoe impar and Harmothoe derjugini (-13.7‰) in the Sea of Japan (Kiyashko et al., 2014) and the high δ15N values of Glycera rouxii (-14.26‰) on the continental shelf of the Bay of Biscay (Loc'H et al., 2008). The δ15N values of the benthos in the Yap Trench are also higher than those in this study as a result of more bacteria and other microorganisms and insoluble sedimentary organic matter with high δ15N existing in the Yap Trench (Guo et al., 2018).
Relationship between the organic carbon contents of macrobenthos and organic carbon contents insediments based on eco-exergy balance (β-value classification)
Ex and Exst, as integrated ecosystem health indicators, stand out as useful biomonitoring tools, potentially helpful as indicators to support environmental decisions with regard to protection and restoration measures (Linares et al., 2018). Ex value of macrobenthos community was affected by biodiversity in the northern continental shelf of the South China Sea. Tang et al. (2018) proposed a 4-division classification method (classified as IE, IIE, IIIE, IVE), where high/low values were divided by the average value (Ex or Exst) for all benthic communities within the defined area. In our study, the average value of Ex was 7345 KJ/m2 and the average value of Exst was 263 KJ/g. Using this method, the thermodynamic structures of the benthic communities in the study area were graded and analyzed. The grading results showed that H4 in the northern continental shelf of the South China Sea was graded as IE, I2 was IIE, five sampling sites (B1, E1, F2, G4 and H1) were IIIE, and four sites (E3, F1, H2 and I2-A) were IVE. Compared with the relatively healthy macrobenthic community in H4, the other sampling sites were in a subhealthly state, especially group IVE, which were relatively unstable and had low energy efficiency.
By quantifying the eco-exergy grades of each site, we performed a correlation analysis between the eco-exergy grades and the average trophic level of macrobenthos. The results showed that there were positively correlations (correlation coefficient R=0.79) by using the OLS regression method.
Investigating the relationships of macrobenthic invertebrates and sedimentation in aquatic environments is challenging because fine sediments act as both natural habitats and potential pollutants at excessive levels (Longing et al., 2010; Zhao et al., 2011). Preservation of organic matter in coastal marine sediments is an important process in the global cycle of carbon, as more than 90% of the carbon buried in the oceans occurrs in continental margin sediments (Ramaswamy et al., 2008). Marine macrobenthos are also an important part of marine carbon storage. Two thermodynamic indicators, Ex and Exst, were used in this integrated assessment. The results showed that there was a significant positive correlation (correlation coefficient R=0.98) between Ex value of macrobenthic ecosystem and the weight of required sediments to support the energy maintenance (Figure 5). Therefore, the average organic carbon content of macrobenthos could be calculated based on the same β value. In summary, the results would provide new ideas and useful supplements for further research on the carbon energy flow and balance of coastal ecosystems.
Conclusion
In this study, the TOC, TN, δ13C and δ15N of macrobenthos and sediments of the NSCS were measured. The Ex, Exst and biodiversity indicators of the macrobenthos communities were analysed. The quantitative relationship between macrobenthic carbon and organic carbon in sediments was obtained. The TOC and TN contents in the macrobenthic organisms varied widely between 1.05% and 71.08% and between 0.29% and 11.36%, with average values of 27.01% and 5.61%, respectively. The TOC and TN contents in the macrobenthos are much higher than those in sediments. The δ13C values range between -16.68‰ and -21.85‰, and the δ15N values range between 6.97‰ and 14.53‰, with average values of -19.37‰ and 9.59‰, respectively. Trophic pathways were established by providing δ13C and δ15N to the typical macrobenthic species, and the trophic levels of most macrobenthos (2.0–3.4) might indicate that they have similar food compositions and living habits. The average TOC in the macrobenthos among the polychaetes (133), brittle stars (91), sea urchins (91), crabs (232) and shrimps (232), molluscs (310), and demersal fishes (499), were assumed to be 29.8%, 4.47%, 1.05%, 29.53%, 36.9%, and 27.23%, respectively. These values were used to estimate the same weight of TOC in the required sediments in marine organic carbon cycle. The relationship between Ex value of macrobenthic ecosystem and the sediment weight required to support the energy maintenance showed a typical positive correlation. From the perspective of thermodynamic equilibrium, the minimum flux of the organic carbon necessary for maintaining the energy balance of macrobenthos community was quantified. The quantitative relationship between macrobenthic carbon and organic carbon in the sedimentary environment has also been established.
Data availability statement
The original contributions presented in the study are included in the article/supplementary material. Further inquiries can be directed to the corresponding authors.
Author contributions
DT and ZX designed the study. DT participated on expeditions and collected sediment and macrobenthic samples. XL, TP, XY, JH, and XH had laboratory work. XL and LJ worked with the graphs processing and polishing. DT, XL and ZX contributed to the evaluation of data and to writing the manuscript and revising the manuscript. All authors contributed to the article and approved the submitted version.
Funding
This work was supported by the Natural Science Foundation of China (NSFC) (No. 41806130), the Project of the China Geological Survey-Comprehensive Geological Survey (Nos. GC20220501; DD20211394), the Natural Science Foundation of Guangdong Province (Nos. 2018A0303130063; 2018A0303130125), Key Special Project for Introduced Talents Team of Southern Marine Science and Engineering Guangdong Laboratory (Guangzhou) (No. GML2019ZD0209).
Conflict of interest
The authors declare that the research was conducted in the absence of any commercial or financial relationships that could be construed as a potential conflict of interest.
Publisher’s note
All claims expressed in this article are solely those of the authors and do not necessarily represent those of their affiliated organizations, or those of the publisher, the editors and the reviewers. Any product that may be evaluated in this article, or claim that may be made by its manufacturer, is not guaranteed or endorsed by the publisher.
References
Bianchi T. S., Mitra S., McKee B. A. (2002). Sources of terrestrially-derived organic carbon in lower Mississippi river and Louisiana shelf sediments: implications for differential sedimentation and transport at the coastal margin. Mar. Chem. 77, 211–223. doi: 10.1016/S0304-4203(01)00088-3
Cai D., Zhang S., Zhang J. (2002). Applications of stable carbon and nitrogen isotope methods in ecological studies. J. Ocean Univ. Qingdao 32 (2), 287–295. doi: 10.16441/j.cnki.hdxb.2002.02.021
Carabel S., Godínez-Domínguez E., Verísimo P., Fernández L., Freire J. (2006). An assessment of sample processing methods for stable isotope analyses of marine food webs. J. Exp. Mar. Biol. Ecol. 336, 254–261. doi: 10.1016/j.jembe.2006.06.001
Chen C., Shiah F., Chung S., Liu K. (2006). Winter phytoplankton blooms in the shallow mixed layer of the South China Sea enhanced by upwelling. J. Marine Syst. 59 (1–2), 97–110. doi: 10.1016/j.jmarsys.2005.09.002
Christensen V., Pauly D. (1992). ECOPATH II - a software for balancing steady-state ecosystem models and calculating network characteristics. Ecol. Model. 61 (3-4), 169–185. doi: 10.1016/0304-3800(92)90016-8
Deniro M. J., Epstein S. (1978). Influence of diet on the distribution of carbon isotopes in animals. Geochim. Cosmochim. Ac 42 (5), 495–506. doi: 10.1016/0016-7037(78)90199-0
Fanelli E., Cartes J. E., Papiol V. (2011). Food web structure of deep-sea macrozooplankton and micronekton off the Catalan slope: Insight from stable isotopes. J. Marine Syst. 87, 79–89.
Feely R. A., Sabine C. L., Lee K., Berelson W., Kleypas J., Fabry V. J., et al. (2004). Impact of anthropogenic CO2 on the CaCO3 system in the oceans. Science 305 (5682), 362–366. doi: 10.1126/science.1097329
Feng Z., Ji R., Ashjian C., Zhang J., Campbell R., Grebmeier J. M. (2021). Benthic hotspots on the northern Bering and chukchi continental shelf: Spatial variability in production regimes and environmental drivers. Prog. Oceanoga 191, 102497. doi: 10.1016/j.pocean.2020.102497
Goni M. A., Yunker M. B., Macdonald R. W., Eglinton T. I. (2000). Distribution and sources of organic biomarkers in arctic sediments from the Mackenzie river and Beaufort shelf. Mar. Chem. 71, 23–51. doi: 10.1016/S0304-4203(00)00037-2
Guo C., Yang Z., Chen J., Zheng H., Zhang D., Ran L., et al. (2018). A preliminary study on the food resources and trophic levels of the benthic community in the yap trench based on stable carbon and nitrogen isotopes. HaiyangXuebao 40 (10), 51–60. doi: 10.3969/j.issn.0253-4193.2018.10.006
Hall R. O., Meyer J. L. (1998). The trophic significance of bacteria in a detritus- based stream food web. Ecology 79 (6), 1995–2012. doi: 10.1890/0012-9658(1998)079[1995:TTSOBI]2.0.CO;2
Hu J., Peng P., Jia G., Mai B., Zhang G. (2006). Distribution and sources of organic carbon, nitrogen and their isotopes in sediments of the subtropical pearl river estuary and adjacent shelf, southern China. Mar. Chem. 98, 274–285. doi: 10.1016/j.marchem.2005.03.008
Jørgensen S. E. (2002). Integration of ecosystem theories: A pattern (Dordrecht, The Netherlands: Springer, Kluwer Academic publishers).
Jørgensen S. E., Ladegaard N., Debeljak M., Marques J. C. (2005). Calculations of exergy for organisms. Ecol. Model. 185 (2-4), 165–175. doi: 10.1016/j.ecolmodel.2004.11.020
Jørgensen S. E., Nielsen S. N. (2007). Application of exergy as the modynamic indicator in ecology. Energy 32, 673–685. doi: 10.1016/j.energy.2006.06.011
Jiang R., Wang Y. (2018). Modeling the ecosystem response to summer coastal upwelling in the northern south China Sea. Oceanologia 60, 32–51. doi: 10.1016/j.oceano.2017.05.004
Jia G., Xu S., Chen W., Lei F., Bai Y., Huh C. (2013). 100-year ecosystem history elucidated from inner shelf sediments off the pearl river estuary, China. Mar. Chem. 151, 47–55. doi: 10.1016/j.marchem.2013.02.005
Jing Z., Qi Y., Hua Z., Zhang H. (2009). Numerical study on the summer upwelling system in the northern continental shelf of the south China Sea. Cont. Shelf Res. 29, 467–478. doi: 10.1016/j.oceano.2017.05.004
Kiyashko S. I., Kharlamenko V. I., Sanamyan K., Alalykina I. L., Würzberg L. (2014). Trophic structure of the abyssal benthic community in the Sea of Japan inferred from stable isotope and fatty acid analyses. Mar. Ecol. Prog. Ser. 500, 121–137. doi: 10.3354/meps10663
Lewis J. (1996). Coastal benthos and global warming: Strategies and problems. Mar. pollut. Bull. 32 (10), 698–700. doi: 10.1016/0025-326X(06)00064-6
Linares M. S., Callisto M., Marques J. C. (2018). Compliance of secondary production and eco-exergy as indicators of benthic macroinvertebrates assemblages' response to canopy cover conditions in Neotropical headwater streams. Sci. Total Environ. 613–614, 1543–1550. doi: 10.1016/j.scitotenv.2017.08.282
Liu K., Lin H., Wang J., He X., Lin J., Huang Y., et al. (2015). Secondary productivity of macrozoobenthos in xiamen coastal waters. Chin. J. Ecol. 34 (12), 3409–3415. doi: 10.13292/j.1000-4890.2015.0315
Liu X., Tang D., Ge C. (2020). Distribution and sources of organic carbon, nitrogen and their isotopic composition in surface sediments from the southern yellow Sea, China. Mar. pollut. Bull. 150, 110716. doi: 10.1016/j.marpolbul.2019.110716
Li Z., Zuo T., Dai F., Jin X. (2010). Trophic level analysis of organisms from changjiang estuary and adjacent waters of southern yellow Sea in spring with stable isotope technology. J. Fishery Sci. China 17 (1), 103–109.
Loc'H F. L., Hily C., Grall J. (2008). Benthic community and food web structure on the continental shelf of the bay of biscay (north eastern Atlantic) revealed by stable isotopes analysis. J. Mar. Syst. 72 (1-4), 17–34. doi: 10.1016/j.jmarsys.2007.05.011
Longing S. D., Voshell J. R., Dolloff J. C. A., Roghair C. N. (2010). Relationships of sedimentation and benthic macroinvertebrate assemblages in headwater streams using systematic longitudinal sampling at the reach scale. Environ. Monit Assess. 161 (1-4), 517–530. doi: 10.1007/s10661-009-0765-4
Ludovisi A., Poletti A. (2003). Use of thermodynamic indices as ecological indicators of the development state of lake ecosystems: 2. Exergy specific exergy indices. Ecol. Model. 159, 223–238. doi: 10.1016/S0304-3800(02)00284-3
Meyers P. A. (1994). Preservation of elemental and isotopic source identification of sedimentary organic matter. Chem. Geol. 114, 289–302. doi: 10.1016/0009-2541(94)90059-0
Meyers P. A. (1997). Organic geochemical proxies of palaeooceanographic, palaeolimnological and palaeoclimatic processes. Org. Geochem. 27 (5–6), 213–250. doi: 10.1016/S0146-6380(97)00049-1
Park Y. S., Kwak I. S., Chon T. S., Kim J. K., Jørgensen S. E. (2001). Implementation of artificial neural networks in patterning and prediction of exergy in response to temporal dynamics of benthic macroinvertebrate communities in streams. Ecol. Model. 146, 143–157. doi: 10.1016/S0304-3800(01)00302-7
Parzanini C., Parrish C. C., Hamel J.-F., Mercier A. (2018). Trophic relationships of deep-sea benthic invertebrates on a continental margin in the NW Atlantic inferred by stable isotope, elemental, and fatty acid composition. Prog. Oceanoga. 168, 279–295. doi: 10.1016/j.pocean.2018.10.007
Pilditch C., Leduc D., Nodder S., Probert P., Bowden D. (2015). Spatial patterns and environmental drivers of benthic infaunal community structure and ecosystem function on the new Zealand continental margin. New Zeal. J. Mar. Fresh 49 (2), 224–246. doi: 10.1080/00288330.2014.995678
Post D. M. (2002). Using stable isotopes to estimate trophic position: models, methods, and assumptions. Ecology 83 (3), 703–718. doi: 10.1016/j.visres.2013.04.013
Ramaswamy V., Gaye B., Shirodkar P. V., Rao P. S., Chivas A. R., Wheeler D., et al. (2008). Distribution and sources of organic carbon, nitrogen and their isotopic signatures in sediments from the ayeyarwady (Irrawaddy) continental shelf, northern Andaman Sea. Mar. Chem. 111, 137–150. doi: 10.1016/j.marchem.2008.04.006
Ren X., Liu Y., Xu B., Zhang C., Ren Y., Chen Y., et al. (2020). Ecosystem structure in the haizhou bay and adjacent waters based on ecopath model. HaiyangXuebao 42 (6), 101–109. doi: 10.3969/j.issn.0253-4193.2020.06.012
Sokolowski A., Wolowicz M., Asmus H., Asmus R., Carlier A., Gasiunaité Z., et al. (2012). Is benthic food web structure related to diversity of marine macrobenthic communities? Estuar. Coast. Shelf S 108, 76–86. doi: 10.1016/j.ecss.2011.11.011
Tang D., Liu X., Zou X. (2018). An improved method for integrated ecosystem health assessments based on the structure and function of coastal ecosystems: a case study of the jiangsu coastal area, China. Ecol. Indic. 84, 82–95. doi: 10.1016/j.ecolind.2017.08.031
Tang D., Zou X., Liu X., Liu P., Zhamangulova N., Xu X., et al. (2015). Integrated ecosystem health assessment based on eco-exergy theory: a case study of the jiangsu coastal area. Ecol. Indic. 48, 107–119. doi: 10.1016/j.ecolind.2014.07.027
Xu Y., Sui J., Ma L., Dong D., Kou Q., Gan Z., et al. (2019). Spatial pattern of benthic macroinvertebrate communities and their relationship with environmental variables on the East China Sea shelf. Deep-Sea Res. Pt. II 169-170, 104633. doi: 10.1016/j.dsr2.2019.07.021
Yu J., Liu M., Hou L., Xu S., Ou D., Cheng S. (2008). Food Sources of Macrofaunal in East Chongming Salt Marsh as Traced by Stable IsotopesJournal of natural resources 23(2), 319 326. doi: 10.3321/j.issn:1000-3037.2008.02.016
Keywords: macrobenthos, sediments, eco-exergy indicators, trophic pathways, organic carbon
Citation: Tang D, Liu X, Peng T, Yang X, Jia L, He J, Huang X and Xia Z (2022) The quantitative relationship between macrobenthic carbon and organic carbon in sediments: A case study of the northern continental shelf of the South China Sea. Front. Mar. Sci. 9:1076795. doi: 10.3389/fmars.2022.1076795
Received: 22 October 2022; Accepted: 18 November 2022;
Published: 14 December 2022.
Edited by:
Zhen Wang, Shantou University, ChinaReviewed by:
Cui Hongpeng, China University of Geosciences, ChinaMao Longjiang, Nanjing University of Information Science and Technology, China
Copyright © 2022 Tang, Liu, Peng, Yang, Jia, He, Huang and Xia. This is an open-access article distributed under the terms of the Creative Commons Attribution License (CC BY). The use, distribution or reproduction in other forums is permitted, provided the original author(s) and the copyright owner(s) are credited and that the original publication in this journal is cited, in accordance with accepted academic practice. No use, distribution or reproduction is permitted which does not comply with these terms.
*Correspondence: Dehao Tang, tangdehao@mail.cgs.gov.cn; Zhen Xia, xia-zhen@163.com