- 1Key Laboratory of Tropical Marine Bio-resources and Ecology, South China Sea Institute of Oceanology, Chinese Academy of Sciences, Guangzhou, China
- 2Sanya Institute of Ocean Eco-Environmental Engineering, Sanya, China
- 3Innovation Academy of South China Sea Ecology and Environmental Engineering, Chinese Academy of Sciences, Guangzhou, China
- 4Guangdong Provincial Key Laboratory of Applied Marine Biology, South China Sea Institute of Oceanology, Chinese Academy of Sciences, Guangzhou, China
- 5University of Chinese Academy of Sciences, Beijing, China
Bacterial pathogen removal function in seagrass meadows is gaining attention worldwide, with enhancing particle sedimentation as the main potential mechanism. Unfortunately, seagrass meadows are declining to patchiness and fragmentation due to anthropogenic activities and global climate change. However, the effects of seagrass decline on bacterial pathogen removal potential are poorly understood, limiting our ability to understand coastal-living humans and marine organisms that suffered pressure from diseases and other health-related effects in response to seagrass decline. Here we investigated abundance of putative bacterial pathogens (including Vibrio spp., Salmonella spp., Staphylococcus spp., and Enterococcus spp.) in trapped particles under different canopy structures of Enhalus acoroides patches in South China Sea. The abundance of Vibrio spp., Salmonella spp., and Staphylococcus spp. trapped particles, respectively, was observed much greater in seagrass patches with high density and height than other patches, and significantly positive correlations with seagrass density and height were observed. This was mainly ascribed to seagrass patches of high density and height being able to trap more particles. Surprisingly, however, the Enterococcus spp. abundance showed negative correlations with density and area. Enterococcus spp. is generally inhibited in well-oxygenated environments, of which the microenvironment of dissolved oxygen might be mediated by seagrass density and area. Overall, this research suggests that the potential of removal of putative bacterial pathogen was inhibited in fragmented seagrass meadow; therefore, coastal-living humans and marine organisms might suffer from an increasing risk of diseases due to the decline of seagrass meadows.
Introduction
About 40% of the world’s population inhabit a narrow coastal band that takes up 7% of the Earth’s land area (McGranahan et al., 2007). At a global scale, more than 80% of domestic wastewater is discharged into rivers or ocean without any treatment (Programme, U.N.W.W.A 2017), thus introducing bacterial pathogens of human and marine organisms to coastal waters (Stewart et al., 2008; Perkins et al., 2014). Coastal-living humans can suffer diseases and other health-related effects when exposed to bacterial pathogens in the ocean (Graciaa et al., 2018; Klohmann and Padilla-Gamiño, 2022). Seagrass meadows, mainly distributed along the coastal areas, are recently highlighted for reducing seawater bacterial pathogens capable of causing diseases in humans and marine organisms (Lamb et al., 2017). Although there is little information about the underlying mechanisms of seagrass meadows for removing putative bacterial pathogens, it is likely due to the mechanically trapped particles (Guan et al., 2019; Deng et al., 2021; Klohmann and Padilla-Gamiño, 2022). Therefore, seagrass meadows can help decrease bacterial pathogen pressure and improve human and marine organism health.
Unfortunately, human activities and global climate change have led to seagrass meadows’ worldwide decline at 7% of the annual rate (Waycott et al., 2009). Seagrass decline can result in continuously changing seagrass meadows to patchiness and fragmentation (Montefalcone et al., 2010; Evans et al., 2018). In different patches, there were always observed variations of seagrass canopy structure, like meadow area, seagrass shoot density, and height (Ramage and Schiel, 1999; McCloskey and Unsworth, 2015)—for example, the shoot density and shoot height of a disturbed and patchy intertidal Zostera marina seagrass meadow in Porthdinllaen, North Wales ranged from 278.4 to 2,321.6 shoots m-2 and 17.3 to 35.4 cm, respectively (McCloskey and Unsworth, 2015). Changes of seagrass canopy structure can significantly influence the potential of trapped seawater particles (Gacia et al., 2003). It has been reported that longer and wider leaves of Enhalus acoroides can enhance more particle deposition in comparison to Thalassia hemprichii (Komatsu et al., 2004; Deng et al., 2021). Similarly, Barcelona et al. (2021) found that particles trapped by the blades of seagrass in the whole canopy increased with canopy density. Therefore, changes of seagrass canopy structure associated with trapped particle potential may influence the removal of seawater bacterial pathogens. However, empirical evidence of seagrass canopy structure influencing bacterial pathogen removal potential is otherwise rare.
In this study, we investigated the putative bacterial pathogen abundances (including Vibrio spp., Salmonella spp., Staphylococcus spp., and Enterococcus spp.) in the trapped particles of different canopy structures of E. acoroides patches in Li’an Bay of South China Sea, which are influenced by aquaculture and human wastewater discharge along the bay’s shoreline (Cui et al., 2021). The results of this study will help reveal the effects of seagrass decline on the removal potential of putative bacterial pathogen as well as provide a scientific basis for improving the management of seagrass meadow protection.
Materials and methods
Study area and sampling site setting
This study was conducted in Li’an Bay (18°25′30″ N, 109°2′30″ E) of Hainan Island, South China Sea, which is a nearly enclosed bay with E. acoroides as the dominant seagrass distributed in shallow coastal waters (Huang et al., 2006). Dense populations and shrimp pond cultures are distributed around the bay’s shoreline, and wastewater is discharged to coastal waters (Cui et al., 2021). The continuous seagrass meadows declined to patches or fragments under human pressure, presenting obvious heterogeneity in seagrass canopy structure. During August 2019, 20 sampling sites (S1–S20) of different E. acoroides patches were set in the northwest area of this bay. At each meadow patch, we measured the meadow area, average seagrass shoot density, and height, which represent the seagrass canopy structure heterogeneity.
Sample collection and analysis
One to three water column particle deposition traps (PVC centrifuge tubes 10 cm in height and 20 cm in diameter) were set under each patch within E. acoroides seagrass meadow (Deng et al., 2021). Small patches were set with one trap, while big patches were set with no more than three traps. The traps were retrieved after a deployment of 2 days. Once collected, all the particles were stored at 4°C for subsequent putative bacterial pathogen abundance analysis within 2 to 3 h.
The selective medium Slanetz–Bartley (Hopebio, China; Moaddab and Töreci, 2000), thiosulphate–citrate–bile salt sucrose agar (BD, USA; Uchiyama, 2000), CHROMagar Salmonella (CHROMagar, France; Webb et al., 2009), and CHROMagar Staphylococcus (CHROMagar, France; Cao et al., 2015) were respectively used to determine the abundance of Enterococcus spp., Vibrio spp., Salmonella spp., and Staphylococcus spp., in trapped particles. Briefly, 5 g of trapped particles was added to 50 ml sterilized 0.85% NaCl. The mix was vortexed for 2 min and then ice-bathed for 2 min, repeating the procedure three times and then resting for 5 min. Then, the original supernatant was directly screened on the four selective agars with 100 µl/plate. At the same time, 10 ml of the original supernatant was filtered onto a 0.45-µm polyethersulfone membrane filter (Supor-200, Pall), and the filter was then placed on the four selective agars with one filter/plate. For the selection of Enterococcus spp., the plates were successively incubated at 37.0°C for around 4 h and then at 44°C for around 44 h. For the selection of Vibrio spp., Salmonella spp., and Staphylococcus spp., the plates were incubated at 37.0°C for 24 h. After incubation, the clones were counted. The abundance of the bacteria in sediment (cfu g-1 DW) was calculated based on the dilution folds and the wet weight/dry weight conversion.
Data analysis
Data analysis was carried out using PRIMER v6 with PERMANOVA+ add-on and Python 3.8.7. First, seagrass canopy metrics data was normalized (zero mean normalization), and then triangular resemblance matrix was created by analyzing the Bray–Curtis similarity between the sampling sites. Principal component analysis (PCA) plots were used to provide explanation of seagrass canopy structure linked to the driving factors, with the sampling sites divided into different groups. Second, the relationships between seagrass canopy metrics (area, seagrass shoot density, and height) and the putative bacterial pathogen abundance data were evaluated by distance-based redundancy analyses (db-RDA). A one-way multifactorial permutational analysis of variance was subsequently performed to determine the statistically significant differences among the groups divided by PCA. Furthermore, Pearson correlation analysis was conducted between seagrass canopy metrics and putative bacterial pathogens. All main and interaction effects with p <0.05 were considered statistically significant.
Results
Details on the patch variables from the 20 sites are presented in Table 1. A PCA showed first two principal components (PC) that explained 83% variation of all seagrass patch canopy structures (Figure 1). PC1 showed a strong correlation with density (r = 0.692) and height (r = 0.686), while PC2 was observed to have a positive correlation with area (r = 0.974) (Figure 1). All the sampling seagrass patches can be divided into four groups by the two PCs (Figure 1). S1, S2, and S3 patches had a small area, respectively, and those with low density and height were marked as the SA+LDH group, while S5, S6, and S8 had a large area, respectively, but with low density and height and thus marked as the LA+LDH group (Figure 1 and Table 1). Furthermore, S7, S16, and S19 had a large area and high density and height, thus named as the LA+HDH group, and the other sampling sites were of relatively high density and height but with a small area, thus named as the SA+HDH group (Figure 1 and Table 1).
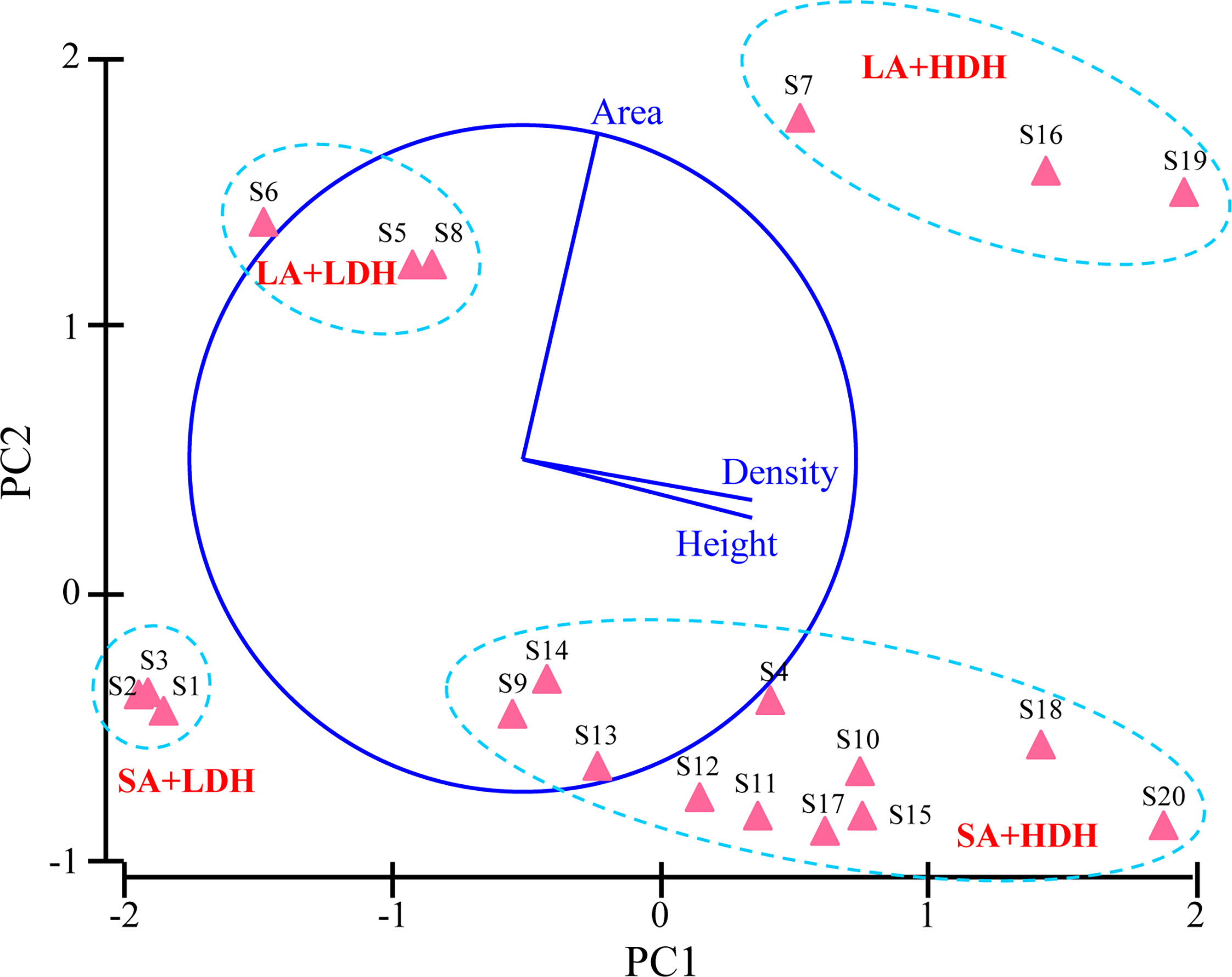
Figure 1 Principal component analysis (PCA) depicting the extent of differences in seagrass canopy structure among all sampling patches. PC1 = 50.5% variation and PC2 = 32.4% variation.
The average abundance of Vibrio spp., Salmonella spp., Staphylococcus spp., and Enterococcus spp. in the trapped particles beneath the E. acoroides ranged from 5,987.67 to 36,976.27, 120.44 to 657.18, 377.11 to 673.69, and 267.41 to 1,540.89 cfu g-1 DW, respectively, with the average abundance of all sampling patches being 18,272.25, 333.57, 529.12, and 550.33 cfu g-1 DW, respectively. There was a significant effect of seagrass canopy structure on the putative bacterial pathogen communities in the trapped particles (pseudo-F = 7.2746, P-perm = 0.001). The abundance of Vibrio spp., Salmonella spp., and Staphylococcus spp. was observed to be generally higher in groups of LA+HDH and SA+HDH than in other groups, while that of Enterococcus spp. showed an opposite trend. The db-RDA analyses of the four putative bacterial pathogens’ data explained 64.9% of the variation in the first two axes (Figure 2). The db-RDA analyses confirmed the clear separation of putative bacterial pathogen communities under the four groups’ patches according to seagrass canopy metrics. Db-RDA1 generally separated the putative bacterial pathogen communities of high-density-and-height-canopy sites from that of low-density-and-height-canopy sites, while db-RDA2 separated the large-area sites from small-area sites (Figure 2). There were strong positive correlations between area and db-RDA2 (r = 0.940) and strong negative correlations between density (r = -0.781), height (r = -0.551), and db-RDA1, respectively.
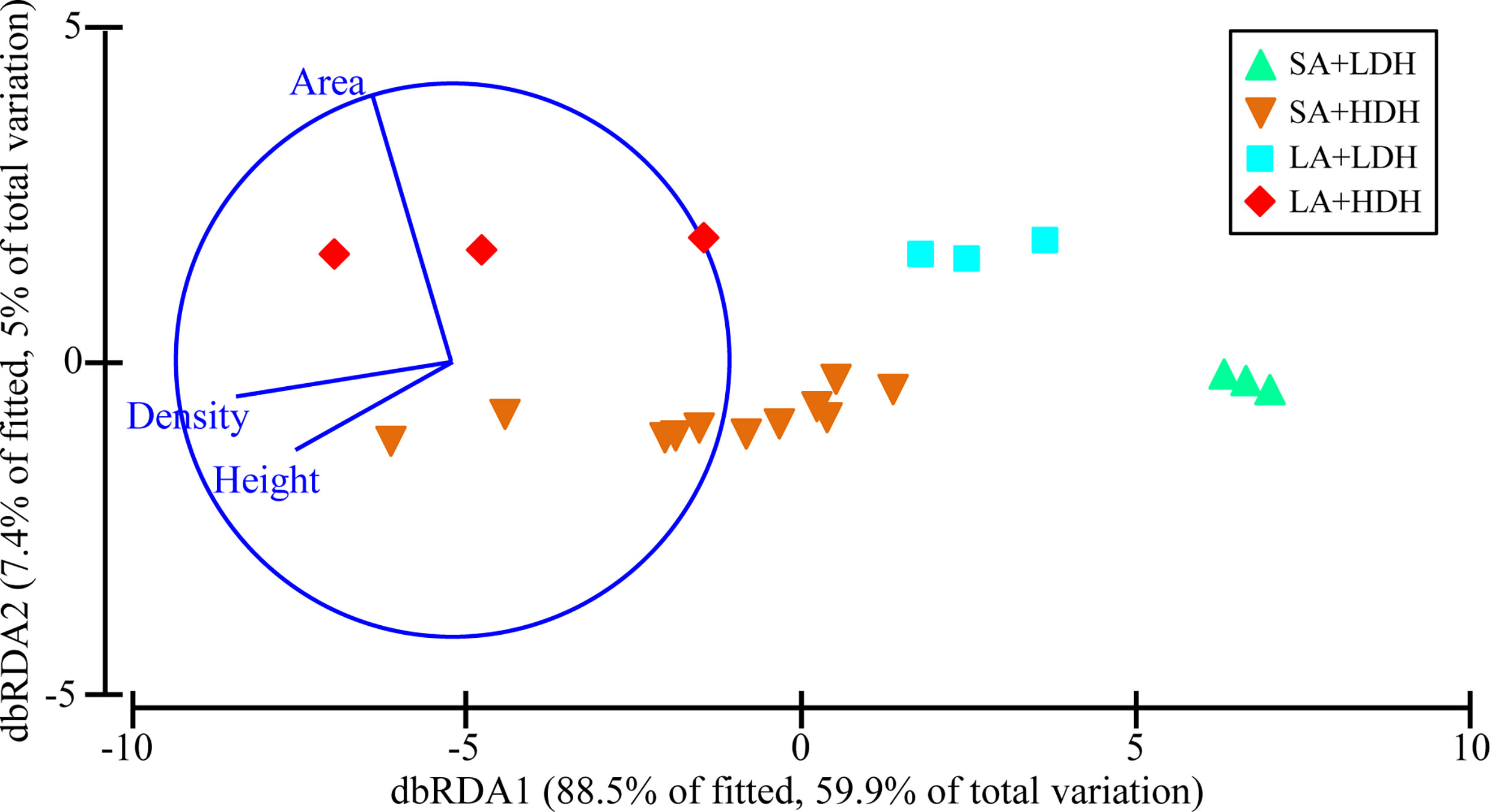
Figure 2 Distance-based redundancy analysis (db-RDA) ordination of putative bacterial pathogen data fitted to seagrass canopy structure variables. The plot represents a db-RDA ordination based upon the Bray–Curtis distance of all the sampling sites.
Pearson correlation analysis was further conducted for the correlation between each putative bacterial pathogen with each seagrass canopy metric. The abundance of Vibrio spp. and Staphylococcus spp., respectively, was observed to have significantly positive correlations with density and height, while the abundance of Salmonella spp. was only shown to have a significantly positive correlation with density (Figure 3). In contrast, Enterococcus spp. abundance was significantly negatively correlated with density and area (Figure 3).
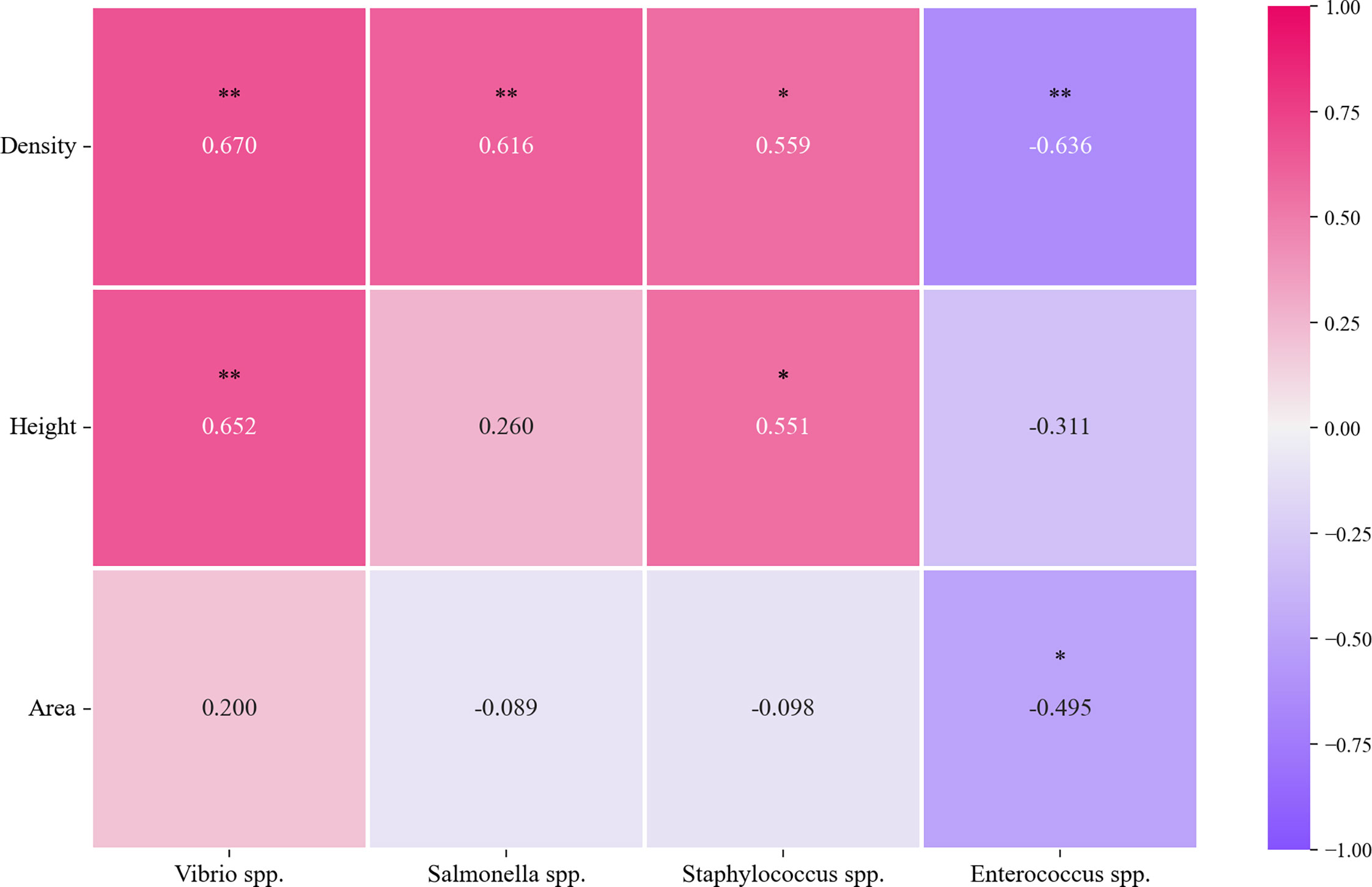
Figure 3 Relationship between four putative bacterial pathogens and three seagrass canopy metrics. Purple represents a negative correlation between variables, and red represents a positive correlation (*p<0.05, **p<0.01).
Discussion
The seagrass meadows of Li’an have shown obvious fragmentation and patchiness, of which the smallest patch was only 0.125 m2 and the lowest E. acoroides density was 64 shoots m-2. Human physical disturbance, including repeated clam digging and fishing, overload of aquaculture activities, and nutrient loading induced by wastewater discharge were the main reason for the seagrass decline and fragmentation of seagrass meadows in Li’an (Yu et al., 2019; Cui et al., 2021). Therefore, anthropogenic activities led to seagrass canopy structure heterogeneity within a fine-scale seagrass meadow. Anthropogenic activities induced the wastewater discharge with input of abundant particles associated with putative bacterial pathogens to seagrass meadows (Ghaderpour et al., 2014; Perkins et al., 2014). In this study, the reported Salmonella spp., Staphylococcus spp., and Enterococcus spp. abundance of trapped particles in coastal sediments was in the range of abundance values reported by previous studies (Craig et al., 2002; Bharathi and Nair, 2005; Perkins et al., 2014). However, the abundance of Vibrio spp. in this study was observed to be much higher than that previously reported in less human-impacted coastal areas (Comeau and Suttle, 2007; Perkins et al., 2014). The high abundance of Vibrio spp. in this study area should originate from coastal aquaculture wastewater discharge (Cui et al., 2021), suggesting that the seagrass meadows of this study were significantly influenced by coastal aquaculture as well.
The putative bacterial pathogen abundance and communities of trapped particles were observed to be significantly different among the four types of seagrass patches, mainly driven by shoot density and height. It has been reported that physical collisions between the particles and the leaves retard water flow by the seagrass canopy, which is the main driving force for particle trapping within seagrass meadows (Komatsu et al., 2004; Hendriks et al., 2008). The high density and height of seagrass canopy can retard water flow more efficiently and elevate the possibility of physical collision, thereby trapping more particles (Gacia et al., 2003; Komatsu et al., 2004; Barcelona et al., 2021). Deng et al. (2021) reported that a higher daily particle deposition rate was observed under E. acoroides stands than under T. hemprichii stands within the fine-scale seagrass meadows, which is mainly attributed to the higher canopy of E. acoroides than that of T. hemprichii. Similarly, seawater particles, especially the fine particles, trapped by the seagrass leaves in the whole canopy increased with seagrass density (Barcelona et al., 2021). Furthermore, fine particles are associated with a higher abundance of putative bacterial pathogens in comparison with coarser particles (Maugeri et al., 2004; Lyons et al., 2007). Therefore, the abundance of trapped particles of Vibrio spp., Salmonella spp., and Staphylococcus spp., respectively, was generally observed to be much greater under high-density and tall-height seagrass patches in this study. Additionally, Vibrio spp., Salmonella spp., and Staphylococcus spp. were generally observed to have significantly positive correlations with seagrass density and height, which supported our assumption as well. In contrast to our expectation, area can only explain less than 5% variations of the putative bacterial pathogen abundance of different patches, and the abundance of Vibrio spp., Salmonella spp., and Staphylococcus spp., respectively, was shown to have no significant correlations with area. Navarrete-Fernández et al. (2022) reported that most trapped particles mainly accumulated along the outer and the inner edge of the meadow or just penetrate the few meters of the meadow. We assumed in this study that the trapped particles accumulated along the edge of seagrass meadows, which supported that the seagrass patch area size has less impact on particle trapping.
Interestingly, the abundance of Enterococcus spp. was observed to have negative correlations with density and area. Enterococcus spp. is consistently present and, being facultative anaerobic bacteria (Fisher and Phillips, 2009), it has been reported to be inhibited in well-oxygenated environments due to direct and indirect photodissociation effects (Curtis et al., 1992; Sassoubre et al., 2012). The concentration of dissolved oxygen in seawater in a large-area seagrass patch was 10–20% higher than in a small-area seagrass patch in the meadows of this study (unpublished data). Therefore, a high-density and large-area seagrass patch should release more oxygen into the seawater within a fine scale due to photosynthesis and consequently result in the abundance of Enterococcus spp. to decrease in this study. However, we can only speculate the potential effects of oxygen on the putative bacterial pathogen in this study. For a future study, we recommend the research effects of microenvironment variations within seagrass meadows on putative bacterial pathogen removal potential. Such research will help reveal the new mechanism for removing putative bacterial pathogen within seagrass meadows.
At a global scale, seagrass meadows are declining and become fragmentated. Given the decrease in the removal of putative bacterial pathogen potential in fragmented seagrass meadows, it could be argued that seagrass decline would lead to coastal-living humans and marine organisms suffering from high-risk diseases. Therefore, we suggest the recovery and protection of the declining seagrass meadows worldwide, which have important implications for human and marine organism health protection (Klohmann and Padilla-Gamiño, 2022).
Conclusion
We found that high-density and tall seagrass patches can trap a greater abundance of putative bacterial pathogens of Vibrio spp., Salmonella spp., and Staphylococcus spp. in water columns. These findings imply that seagrass decline can decrease the ecological function of putative bacterial pathogen removal potential. What is unknown, however, is that the abundance of trapped particles of Enterococcus spp. was observed to have negative correlations with density and area, which might be affected by dissolved oxygen variations. Further work is needed to conduct an evaluation of the putative bacterial pathogen changes in response to microenvironment variations within seagrass meadows.
Data availability statement
The raw data supporting the conclusions of this article will be made available by the authors, without undue reservation.
Author contributions
SL: conceptualization, methodology, and writing—original draft. YW: investigation, methodology, and data curation. HL: Investigation and validation. YR: formal analysis and software. ZJ: project administration and investigation. XZ: resources and data curation. YF: methodology and data curation. JL: formal analysis. XH: supervision, writing—review and editing, and funding acquisition. All authors contributed to the article and approved the submitted version.
Funding
This work was supported by the National Key Research and Development Program of China (2022YFF0802201), the Key Research and Development Project of Hainan (ZDYF2020180 and ZDYF2021SHFZ254), the National Natural Science Foundation of China (42176155, U1901221, and 41730529), and the Innovation Academy of South China Sea Ecology and Environmental Engineering, Chinese Academy of Sciences (ISEE2021PY06 and ISEE2021ZD03).
Conflict of interest
The authors declare that the research was conducted in the absence of any commercial or financial relationships that could be construed as a potential conflict of interest.
Publisher’s note
All claims expressed in this article are solely those of the authors and do not necessarily represent those of their affiliated organizations, or those of the publisher, the editors and the reviewers. Any product that may be evaluated in this article, or claim that may be made by its manufacturer, is not guaranteed or endorsed by the publisher.
References
Barcelona A., Oldham C., Colomer J., Garcia-Orellana J., Serra T. (2021). Particle capture by seagrass canopies under an oscillatory flow. Coast. Eng. 169, 103972. doi: 10.1016/j.coastaleng.2021.103972
Bharathi P. L., Nair S. (2005). Rise of the dormant: simulated disturbance improves culturable abundance, diversity, and functions of deep-sea bacteria of central Indian ocean basin. Mar. Georesources Geotechnology 23 (4), 419–428. doi: 10.1080/10641190500446805
Cao J. P., Han D., Wang J. (2015). A comparative study on quantitative detection of staphylococcus aureus in food. J. Anhui Agric. Sci 43 (8), 242-243+245. doi: 10.13989/j.cnki.0517-6611.2015.08.092
Comeau A. M., Suttle C. A. (2007). Distribution, genetic richness and phage sensitivity of vibrio spp. from coastal British Columbia. Environ. Microbiol. 9 (7), 1790–1800. doi: 10.1111/j.1462-2920.2007.01299.x
Craig D., Fallowfield H. J., Cromar N. (2002). Enumeration of faecal coliforms from recreational coastal sites: evaluation of techniques for the separation of bacteria from sediments. J. Appl. Microbiol. 93 (4), 557–565. doi: 10.1046/j.1365-2672.2002.01730.x
Cui L., Jiang Z., Huang X., Chen Q., Wu Y., Liu S., et al. (2021). Eutrophication reduces seagrass contribution to coastal food webs. Ecosphere 12 (6), e03626. doi:
Curtis T. P., Mara D. D., Silva S. A. (1992). Influence of pH, oxygen, and humic substances on ability of sunlight to damage fecal coliforms in waste stabilization pond water. Appl. Environ. Microbiol. 58 (4), 1335–1343. doi: 10.1128/aem.58.4.1335-1343.1992
Deng Y., Liu S., Feng J., Wu Y., Mao C. (2021). What drives putative bacterial pathogens removal within seagrass meadows? Mar. Pollut. Bull. 166, 112229. doi: 10.1016/j.marpolbul.2021.112229
Evans S. M., Griffin K. J., Blick R. A., Poore A. G., Vergés A. (2018). Seagrass on the brink: Decline of threatened seagrass posidonia australis continues following protection. PloS One 13 (4), e0190370. doi: 10.1371/journal.pone.0190370
Fisher K., Phillips C. (2009). The ecology, epidemiology and virulence of enterococcus. Microbiology 155 (6), 1749–1757. doi: 10.1099/mic.0.026385-0
Gacia E., Duarte C. M., Marbà N., Terrados J., Kennedy H., Fortes M. D., et al. (2003). Sediment deposition and production in SE-Asia seagrass meadows. Estuar. Coast. Shelf Sci. 56 (5), 909–919. doi: 10.1016/S0272-7714(02)00286-X
Ghaderpour A., Nasori K. N. M., Chew L. L., Chong V. C., Thong K. L., Chai L. C. (2014). Detection of multiple potentially pathogenic bacteria in matang mangrove estuaries, Malaysia. Mar. Pollut. Bull. 83 (1), 324–330. doi: 10.1016/j.marpolbul.2014.04.029
Graciaa D. S., Cope J. R., Roberts V. A., Cikesh B. L., Kahler A. M., Vigar M., et al. (2018). Outbreaks associated with untreated recreational water–united states 2000–2014. MMWR Morb Mortal Wkly Rep. doi: 10.15585/mmwr.mm6725a1
Guan C., Saha M., Weinberger F. (2019). Chemical defence of a seagrass against microfoulers and its seasonal dynamics. Appl. Sci. 9 (6), 1258. doi: 10.3390/app9061258
Hendriks I. E., Sintes T., Bouma T. J., Duarte C. M. (2008). Experimental assessment and modeling evaluation of the effects of the seagrass Posidonia oceanica on flow and particle trapping. Mar. Ecol. Prog. Ser. 356, 163–173. doi: 10.3354/meps07316
Huang X. P., Huang L. M., Li Y. H., Xu Z. Z., Fong C. W., Huang D. J., et al. (2006). Main seagrass beds and threats to their habitats in the coastal sea of south China. Chin. Sci. Bull. 51, 136–142. doi: 10.1007/s11434-006-9136-5
Klohmann C., Padilla-Gamiño J. L. (2022). Pathogen filtration: An untapped ecosystem service. Front. Mar. Sci. 1226. doi: 10.3389/fmars.2022.921451
Komatsu T., Umezawa Y., Nakakoka M., Supanwanid C., Kanamoto Z. (2004). Water flow and sediment in Enhalus acoroides and other seagrass beds in the Andaman Sea, off khao bae Na, Thailand. Coast. Mari. Sci. 29 (1), 63–68 doi: 10.15083/00040824
Lamb J. B., van de Water J. A., Bourne D. G., Altier C., Hein M. Y., Fiorenza E. A., et al. (2017). Seagrass ecosystems reduce exposure to bacterial pathogens of humans, fishes, and invertebrates. Science 355 (6326), 731–733. doi: 10.1126/science.aal1956
Lyons M. M., Lau Y.-T., Carden W. E., Ward J. E., Roberts S. B., Smolowitz R., et al. (2007). Characteristics of marine aggregates in shallow-water ecosystems: implications for disease ecology. EcoHealth 4 (4), 406–420. doi: 10.1007/s10393-007-0134-0
Maugeri T., Carbone M., Fera M., Irrera G., Gugliandolo C. (2004). Distribution of potentially pathogenic bacteria as free living and plankton associated in a marine coastal zone. J. Appl. Microbiol. 97 (2), 354–361. doi: 10.1111/j.1365-2672.2004.02303.x
McCloskey R. M., Unsworth R. K. (2015). Decreasing seagrass density negatively influences associated fauna. PeerJ 3, e1053. doi: 10.7717/peerj.1053
McGranahan G., Balk D., Anderson B. (2007). The rising tide: assessing the risks of climate change and human settlements in low elevation coastal zones. Environ. urbanization 19 (1), 17–37. doi: 10.1177/0956247807076960
Moaddab S. R., Töreci K. (2000). Species level identification and investigation of resistance to vancomycin and some other antibiotics in enterococcus strains. Türk Mikrobiyol Cem Derg 30, 77–84.
Montefalcone M., Parravicini V., Vacchi M., Albertelli G., Ferrari M., Morri C., et al. (2010). Human influence on seagrass habitat fragmentation in NW Mediterranean Sea. Estuarine Coast. Shelf Sci. 86 (2), 292–298. doi: 10.1016/j.ecss.2009.11.018
Navarrete-Fernández T., Bermejo R., Hernández I., Deidun A., Andreu-Cazenave M., Cózar A. (2022). The role of seagrass meadows in the coastal trapping of litter. Mar. Pollut. Bull. 174, 113299. doi: 10.1016/j.marpolbul.2021.113299
Perkins T. L., Clements K., Baas J. H., Jago C. F., Jones D. L., Malham S. K., et al. (2014). Sediment composition influences spatial variation in the abundance of human pathogen indicator bacteria within an estuarine environment. PloS One 9 (11), e112951. doi: 10.1371/journal.pone.0112951
Programme, U.N.W.W.A (2017). "the untapped resource, the united nations world water development report" (Paris, France: UNESCO World Water Assessment Programme).
Ramage D. L., Schiel D. R. (1999). Patch dynamics and response to disturbance of the seagrass Zostera novazelandica on intertidal platforms in southern new Zealand. Mar. Ecol. Prog. Ser. 189, 275–288. doi: 10.3354/MEPS189275
Sassoubre L. M., Nelson K. L., Boehm A. B. (2012). Mechanisms for photoinactivation of enterococcus faecalis in seawater. Appl. Environ. Microbiol. 78 (21), 7776–7785. doi: 10.1128/AEM.00913-14
Stewart J. R., Gast R. J., Fujioka R. S., Solo-Gabriele H. M., Meschke J. S., Amaral-Zettler L. A., et al. (2008). The coastal environment and human health: microbial indicators, pathogens, sentinels and reservoirs. Environ. Health 7 (2), 1–14. doi: 10.1186/1476-069X-7-S2-S3
Uchiyama H. (2000). Distribution of vibrio species isolated from aquatic environments with TCBS agar. Environ. Health Prev. Med. 4 (4), 199–204. doi: 10.1007/BF02931258
Waycott M., Duarte C. M., Carruthers T. J., Orth R. J., Dennison W. C., Olyarnik S., et al. (2009). Accelerating loss of seagrasses across the globe threatens coastal ecosystems. Proc. Natl. Acad. Sci. 106 (30), 12377–12381. doi: 10.1073/pnas.0905620106
Webb K., Ritter V., Hammack T. (2009). CHROMagar salmonella detection test kit. J. AOAC Int. 92 (6), 1906–1909. doi: 10.1093/jaoac/92.6.1906
Keywords: seagrass meadows, fragmentation, canopy structure, pathogen, trapped particle
Citation: Liu S, Wu Y, Luo H, Ren Y, Jiang Z, Zhang X, Fang Y, Liang J and Huang X (2023) Seagrass canopy structure mediates putative bacterial pathogen removal potential. Front. Mar. Sci. 9:1076097. doi: 10.3389/fmars.2022.1076097
Received: 21 October 2022; Accepted: 07 December 2022;
Published: 04 January 2023.
Edited by:
Songzhe Fu, Dalian Ocean University, ChinaReviewed by:
Qiuying Han, Hainan Tropical Ocean University, ChinaLiu Guangxin, South China Sea Fisheries Research Institute (CAFS), China
Copyright © 2023 Liu, Wu, Luo, Ren, Jiang, Zhang, Fang, Liang and Huang. This is an open-access article distributed under the terms of the Creative Commons Attribution License (CC BY). The use, distribution or reproduction in other forums is permitted, provided the original author(s) and the copyright owner(s) are credited and that the original publication in this journal is cited, in accordance with accepted academic practice. No use, distribution or reproduction is permitted which does not comply with these terms.
*Correspondence: Xiaoping Huang, eHBodWFuZ0BzY3Npby5hYy5jbg==