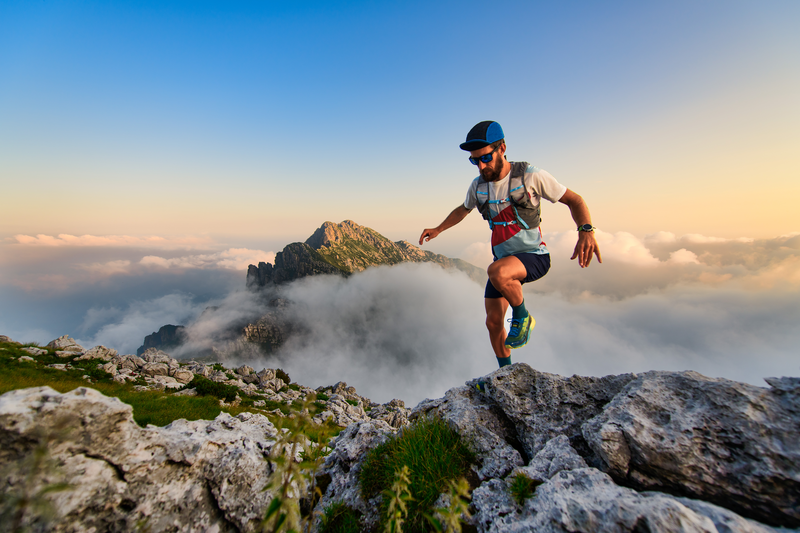
94% of researchers rate our articles as excellent or good
Learn more about the work of our research integrity team to safeguard the quality of each article we publish.
Find out more
ORIGINAL RESEARCH article
Front. Mar. Sci. , 22 November 2022
Sec. Coastal Ocean Processes
Volume 9 - 2022 | https://doi.org/10.3389/fmars.2022.1070737
This article is part of the Research Topic Macroecology of Coastal Zone under Global Changes View all 15 articles
The feeding selectivity of bivalves can play an important role in shaping the structure of phytoplankton communities of natural waters. This could be particularly true in waters with intensive bivalves farming, like Sungo Bay, Northern China. Understanding the role of bivalve feeding behavior is important for assessing how the dense cultivation of bivalves may affect phytoplankton community composition and food web structure in farm areas. In this study, we investigated the feeding selectivity of blue mussel Mytilus coruscus on natural phytoplankton assemblages in Sungo Bay using both optical microscopy and HPLC-pigment analysis. Results showed that cryptophytes dominated the phytoplankton community and made up 66.1% of the total phytoplankton abundance. A comparison of phytoplankton composition between natural and filtered seawater showed that M. coruscus preferred cryptophytes and dinoflagellates than Chaetoceros spp. and Skeletonema spp. Cryptophytes were not detected in gut contents by microscopic observation, while their marker pigment alloxanthin was present, suggesting they were also consumed by M. coruscus and can be readily digested. This highlights the shortcomings of microscopic methods and the significance of HPLC-pigment analysis in obtaining a comprehensive understanding of feeding selectivity of bivalves. The proportions of Chaetoceros spp. and Skeletonema spp. in gut contents were significantly lower than their proportions in the seawater, and contrastingly, the proportions of Cocconeis spp. and Pinnularia spp. showed opposite patterns. The marker pigments prasinoxanthin and zeaxanthin were detected in the gut of M. coruscus indicating that picophytoplankton (e.g., prasinophytes and Synechococcus) are also food sources for this bivalve. This information furthers our understanding of bivalve aquaculture and environment interactions.
Bivalves like mussels, oysters, and scallops are key species for mariculture in coastal waters worldwide (Ward and Shumway, 2004; Filgueira et al., 2016). It is well known that filter-feeding bivalves play an important role in shaping the structure of phytoplankton community (Dame and Prins, 1998; Nakamura and Kerciku, 2000; Filgueira et al., 2012). However, there remain unknowns about the relationship between bivalve filter-feeding and phytoplankton assemblages, particularly for dense populations of bivalves in farming areas (Cédric et al., 2003).
Bivalves are exposed to diverse particles (living and nonliving; large and small) in the marine environment. Generally, suspension-feeding bivalves capture particles of increasing size with increasing efficiency, until some size threshold is met, beyond which all particles cannot be effectively captured (reviewed by Rosa et al., 2018); for example, the threshold for M. edulis is ~4 µm (Riisgård, 1988; Riisgård & Larsen, 2010). It has been found that some particles are more likely to be captured by bivalves than others, despite being similar in size (Shumway et al., 1985; Naddafi et al., 2007). This suggests that qualitative aspects apart from size can influence selective feeding (Grizzle et al., 2001; Ward & Shumway, 2004; Rosa et al., 2021). Previous studies have found that some bivalves may have flexible feeding behaviors in response to changes in particle composition and concentrations (e.g. Bayne & Worrall, 1980; Hawkins et al., 1996; Beninger et al., 2008; Strohmeier et al., 2012).
In some previous studies, the issue of feeding selectivity in bivalves has been addressed using various combinations of laboratory cultured algae (Shumway et al., 1985; Bougrier et al., 1997; Pales Espinosa et al., 2016; Rosa et al., 2017). These studies have found that bivalves can preferentially select different algal species based on the size, morphology, particle shape, and motility (Ward & Shumway, 2004); toxicity, nutritional contents (Ren et al., 2006); and membrane composition of the prey (Rosa et al., 2018). Feeding experiments conducted in a laboratory with a cultured diet may not appropriately reflect in situ conditions. Short-term variations of phytoplankton biomass and species composition may continually occur in response to the changing marine environments (Filgueira et al., 2016). Previous studies have explored particle selection by suspension feeders using natural algal assemblages (Cognie et al., 2001; Yahel et al., 2006; Naddafi et al., 2007; Yahel et al., 2009; Safi and Hayden, 2010; Frau et al., 2016). In those studies, the microscopic method has generally been used to study the feeding of phytoplankton by bivalves. The comparison of phytoplankton assemblages between gut contents and the surrounding seawater has also been performed to deal with their differential utilization by bivalves (Kamermans, 1994; Rouillon et al., 2005). However, the microscopic method can easily omit fragile or small phytoplankton cells in seawater, especially in gut contents of bivalves (Trottet et al., 2008). This would introduce a bias into evaluating the effects of selective feeding of bivalves on phytoplankton biomass and community, as well as bivalve diet in the natural environment. In recent decades, high-performance liquid chromatography (HPLC) based pigments analysis has been developed, which provides a chemotaxonomic qualitative and quantitative analysis for phytoplankton as certain key pigments are sensitive indicator of different algal groups (Zapata et al., 2000). The HPLC-pigment method is appropriate for the identification of fragile cells and small cells, but it cannot provide accurate taxonomical information (to species or genus) of phytoplankton (Pan et al., 2020). Nevertheless, this method has provided much important information in the studies of both feeding and digestion of molluscs (Loret et al., 2000; Lavaud et al., 2018; Jiang et al., 2019). Consequently, a combination of these two methods might provide a further understanding of the effects of bivalve selective feeding on the profile of phytoplankton communities in aquaculture sites (Trottet et al., 2008).
In the present study, we investigated the selective feeding of phytoplankton assemblages by M. coruscus in farming waters of Sungo Bay. We focused on two aspects of feeding selectivity: clearance (also referred to as ‘retention’) efficiency in the seawater, and a comparison between seawater and gut contents. The species compositions of phytoplankton in seawater and mussel gut contents were identified using microscopic counting and HPLC-pigment analysis. This study furthers our understanding of feeding selectivity and diet composition of M. coruscus, and more broadly about the potential effects of bivalve aquaculture on phytoplankton communities.
Sungo Bay is located in northern China and characterized by a temperate climate. It has been used for farming bivalves (e.g., oyster Crassostrea gigas and Chinese scallop Chlamys farreri) and seaweed (Saccharina japonica) for several decades. The feeding experiments were conducted on a floating platform in a semi-enclosed dock of Sungo Bay (37°02′07′′ N, 122°32′58′′ E), China. The seawater used for the experiments was pumped from 50 m off the shore at a depth of 3m (Fig 1), which is about 3 km away from an aquaculture area. The water temperature and salinity were around 24°C and 31, respectively. Previous study showed that the diatoms are the most common species and dominate the phytoplankton community in this area (Wang, 2017; Hou et al., 2021). During the past several decades, the species number and Shannon’s diversity index of phytoplankton in Sungo Bay have declined, which has been ascribed to the high biomass of cultured shellfish (Hou et al., 2021).
Eight groups of feeding experiments with M. coruscus were performed. The experimental groups NO. 1-6 and NO. 7-8 were performed on Sept. 16 and 20, 2018, respectively (Table 1). Before the experiments, all mussels were placed in a large plastic chamber (20 l) over 24 h with continuous seawater influx from a branch pipe of the headwater, which was the same source as the feeding experiments (Figure 1). In each experimental group, three mussels were placed in independent 1.0 l PVC chambers (as described in Cranford et al., 2016) supplied with seawater. The chambers were mounted on magnetic stirrers to ensure that the water was well mixed. The chambers were maintained in flow-through until all mussels were observed to be open. At that point, the flow of water was interrupted, and the chambers became static. The size distribution of the particles in each chamber was monitored using a PAMAS S40 GO instrument (PAMAS, Rutesheim, Germany) (Figure 1). Particle counts were measured once every 30 seconds throughout the static incubations. Particles were both counted across different size bins. Static incubations were run either until ~50% of particles larger than 8.0 µm were depleted, or for a maximum of one hour, which resulted in different incubations times across experimental groups (Table 1). The length of M. coruscus ranged from 45 to 56 mm. The experimental groups NO. 1-5 and 7 were used for studying the clearance of phytoplankton cells by M. coruscus using the microscopic method and the experimental groups NO. 6 and 8 were for the clearance of phytopigments by the HPLC pigments determination.
Table 1 Experimental design and total phytoplankton biomass in each experimental group (n=3 mussels for each group).
Figure 1 Feeding experiments in the Sungo Bay. (P, PAMAS; M, Magnetic stirrer; SA, Maintenance of M. coruscus samples).
For the experimental groups NO. 1-5 and 7, triplicate aliquots of 1.0 L seawater from the headwater were collected for microscopic analyses. After completion of each experiment, the filtered seawater in the chamber was also collected, and its volume was measured. Seawater samples were fixed by Lugol’s solution and concentrated to 5–15 mL overnight settling, with 0.1 mL placed in a counting chamber and counted under light microscopy (Olympus BH-2, Olympus, Tokyo, Japan). Each sample was counted in triplicate and at least 350 cells were identified per counting.
For the experimental groups NO. 6 and 8, 1.0 L seawater (n=3) from the headwater was filtered through GF/F filters (0.7 μm pore size, 47 mm diameter, Whatman). After feeding experiments, the volume of remaining seawater in each chamber was measured, and then filtered as described above. The filters were immediately put into liquid nitrogen and stored until HPLC analysis. Pigment extraction and determination were performed according to Zapata et al. (2000). The 21 pigment standards were purchased from DHI (Denmark) (Table S1). All HPLC procedures were described by Pan et al. (2020).
After completion of the feeding experiments, mussel gut contents were immediately collected into a plastic tube by making an incision through to the gut cavity and rinsing of contents using filtered seawater (GF/F filter (< 0.7 μm)). Gut contents of the 15 individuals in experimental Groups NO. 1-5 were fixed in Lugol’s solution for phytoplankton analysis by microscope described as in Section Microscopic observations for phytoplankton. Due to heavy disturbance by detritus, only 7 out of 15 mussel gut samples could achieve counts of > 200 algal cells (note that cryptophyte species were not observed due to digestion, see Section 3.4), which was the adopted threshold to guarantee the accuracy of the data (Venrick, 1978). Consequently, the other 8 samples were excluded in this study. The gut content of the 3 individuals of Group NO. 6 and 8 were filtered onto independent GF/F filters for pigments analysis described as in Section HPLC-pigment analysis for sea water.
In each experimental group, clearance efficiency (CE) of phytoplankton for each M. coruscus was calculated as follows (Vahl, 1972):
where, PBbf and PBaf are the phytoplankton biomass (cell density (cell l-1) by microscopic counting and pigments concentration (ng l-1) by HPLC analysis) in the natural seawater (headwater) and experimental water before and after the experiment, respectively.
For the whole experiment, the ratios of clearance efficiency (RCE) for certain phytoplankton genera or groups against total phytoplankton were calculated to describe the degree of selective clearance by M. coruscus, as shown in formula (2):
where CEphyto is CE of certain phytoplankton genera or group; CEtotal is CE of total phytoplankton. For certain phytoplankton genera or group, RCE > 1 means it is selectively filtered by M. coruscus, while RCE < 1 means it is selectively rejected.
Given the different static incubation times and individual variability in terms of pumping activity, a refiltration index was calculated for comparative purposes across different mussels. This index describes the total amount of water that was pumped by each mussel in the individual chamber during the measurements. This refiltration index was calculated as a ratio between the volume of water pumped by the mussel and the total volume of the chamber. To calculate the volume of water pumped by the mussel, pumping rate (PR) was calculated as:
Where λaverage is the slope of ln (particle concentration) over time of particles which are captured with 100% efficiency by the mussel (see Cranford et al., 2016 for a detailed description of this equation). In this study, particles ranging between 8.25 and 10.75 µm were assumed to be 100% captured and used to calculate λaverage. To avoid error generated from non-constant pumping, or very low particle counts, only slopes with an associated r2 ≥ 0.90 were used (Cranford et al., 2016). V is the chamber volume, and PR is pumping rate in units of l h-1. The refiltration index (RI) was calculated dividing the total volume pumped by the mussel by the volume of the chamber. A RI greater than 1 indicates that the mussel pumped more water than the volume of the chamber.
One-way ANOVA (Fisher LSD Test) was performed to evaluate the differences in the composition of phytoplankton assemblages in seawater and gut contents of M. coruscus, which was performed using SPSS 19.0. The agreement between RCE of phytoplankton assemblages and CE of total phytoplankton was evaluated using linear regression analysis. The relationships between CE of phytoplankton assemblages (and pigments) and RI were determined using logarithmic fit analysis. The above correlation analysis was performed by Origin 2017 software.
A total of 57 phytoplankton species were identified by microscope, including 48 diatoms, 6 dinoflagellates and 1 cryptophyte sp. (Figure 2). Cryptophytes, around 5-8 μm in diameter, were found as the dominant group, with average abundance of 19.69×104 cell l-1, and it made up 66.1% of the total phytoplankton abundance. Diatoms Chaetoceros spp. (1.58×104 cell l-1) and Cocconeis scutellum (1.08×104 cell l-1) followed in abundance. Dinoflagellates (including Gymnodinium spp., Gonyaulax spinifera, Gyrodinium spp. and Prorocentrum spp.) only comprised 3.5% of total phytoplankton abundance.
Figure 2 Some dominant phytoplankton species in this study. (A) Cryptophytes sp.; (B) Chaetoceros sp.; (C) Skeletonema costatum; (D) Pseudo-nitzschia sp.; (E) Nitzschia sp.; (F) Pinnularia sp.; (G) Cocconeis scutellum; (H) Amphora sp.; (I) Gonyaulax spinifera; (J) Gymnodinium sp.; (K) Gyrodinium spirale (A cryptophytes; B, C: centric diatoms; D–H: Pennatae diatoms; I–K: dinoflagellates).
Phytoplankton biomass (cell abundance and Chl a concentration) before feeding experiments varied among different experimental groups due to the fluctuation of natural seawater (Table 1). Due to different incubation times and pumping rate across individuals, large differences of RI among the triplicate parallel experiments existed within each group (e.g., NO. 3, 5) (Table 1). Consequently, different experimental groups showed some variations of clearance efficiency of different phytoplankton genus/groups (Figure S1).
Given that the RI by M. coruscus varied between individual mussels in the experiments (Table 1), the clearance efficiency of phytoplankton cells was explored in relation to refiltration index (RI) (Figure 3) rather than comparing the proportions of phytoplankton before vs. after feeding experiments (Figure S1). Significant statistical relationships were found between RI and total phytoplankton cells (F=247; P< 0.01), as well as different groups (P< 0.01) (Figure 3). Moreover, these relationships revealed a differential clearance efficiency for the different algal groups. For a RI of 1.0, cryptophytes had the highest clearance efficiency (83%) while centric diatoms had the lowest (63%) (Figures 3B, F, respectively).
Figure 3 Logarithmic relationships between clearance efficiency of phytoplankton cells and refiltration index (RI) by M. coruscus.
There was a large variation in the ratio of clearance efficiency (RCE) of different phytoplankton assemblages to total phytoplankton at RI = 1 by M. coruscus (Figure 4). The RCE of cryptophytes against total phytoplankton was the highest (1.06) while Chaetoceros spp. Showed the lowest (0.80), representing preferential filtration and rejection, respectively. The other centric diatom Skeletonema spp. Also showed a relatively low RCE. By comparison, the pennate diatoms and dinoflagellates showed similar RCR within a range of 0.91-0.99.
Figure 4 The ratio of clearance efficiency (RCE) of dominant phytoplankton assemblages against total phytoplankton at Refiltration Index = 1. The following abbreviations were used for the different communities: Chae, Chaetoceros spp.; Ske, Skeletonema spp.; Cocc, Cocconeis spp.; Pseu, Pseudo-nitzschia spp.; Nitz, Nitzschia spp.; Amph, Amphora spp.; Tra, Trachyneis spp.; Pinn, Pinnularia spp.; Gymn, Gymnodinium spp.; Gony, Gonyaulax spp.; Gyro, Gyrodinium spp.; Cry, cryptophytes.
The relationship between RCE and CE of total phytoplankton by M. coruscus differed across phytoplankton groups (Figure 5). With an increasing CE and decreasing total phytoplankton density in the remaining seawater after feeding experiments, the RCE of the dinoflagellates decreased (P< 0.01) while the centric diatoms showed an increasing trend (P< 0.01). It was noteworthy that the RCE of centric diatoms never exceeded 1.0. By comparison, the RCE of cryptophytes was relatively stable and always above 1.0. The average RCE of cryptophytes was significantly higher than centric diatoms (P< 0.01). No significant difference of RCE was observed among the other phytoplankton assemblages (P > 0.05) (Figure 5).
Figure 5 The relationship between the ratio of clearance efficiency of different phytoplankton groups against total phytoplankton (RCE) and CE of total phytoplankton by M. coruscus.
Significant relationships were found between the CE of different pigments and RI (F=8.98; P< 0.05) except for zeaxanthin (F=2.37; P = 0.19) (Figure 6). These relationships differed across pigments. When the RI was 1.0, alloxanthin and peridinin (marker pigment of cryptophytes and dinoflagellates, respectively) had the highest CE (77%-78%), and fucoxanthin (marker pigment of diatoms) ranked the third (Figures 6A–C). By comparison, prasinoxanthin and zeaxanthin (marker pigment of prasinophytes and Synechococcus, respectively) had the lowest clearance efficiency (27%-31% at RI = 1.0) (Figures 6D, E, respectively). Chl a, as an estimation of the total phytoplankton biomass, showed a moderate clearance efficiency (56% at RI = 1.0) (Figure 6G).
Figure 6 Logarithmic relationships between clearance efficiency of phytopigments and refiltration index by M. coruscus.
The 7 samples having counts > 200 cells were distributed over five experimental groups, and the total amount of phytoplankton cells in gut contents ranged from 1.7×103 to 15.3×103 cells ind-1 (Table S2). Therefore, the pooled data of 15 water samples was compared to 7 mussels (Table 2 and Table S2) to explore differences in the composition of phytoplankton between them. A total of 31 phytoplankton species (or genera) were observed in the gut contents (Table S2). It is noteworthy that cryptophyte cells were not observed in the gut contents. The proportion of Chaetoceros spp. and Skeletonema spp. was significantly lower in the gut contents than in the seawater (P< 0.05). By contrast, Cocconeis spp. and Pinnularia spp. showed significantly higher proportion in the former than the latter (P< 0.05) (Table 2).
Table 2 Average density and composition of phytoplankton assemblages in sea water and gut contents (in accordance with gut contents, phytoplankton composition in seawater was only calculated for total diatoms and dinoflagellates but cryptophytes).
Similarly, a comparison of the seawater and gut contents showed different pigment structure (Table 3; Figure S2). In the natural seawater, fucoxanthin and prasinoxanthin contributed the highest proporation of total marker pigments (Table 3). By comparison, the proportion of prasinoxanthin significantly decreased in the gut contents of M. coruscus (P< 0.01). Zeaxanthin and lutein showed similar changes. Meanwhile, proportion of alloxanthin showed a significant increasing trend, from 6.65 ± 0.65% to 31.46 ± 4.41% (P< 0.01) (Table 3).
Table 3 Composition of the 7 detectable marker pigments in sea water and gut contents (n=3) in Experimental Group NO.6.
The results indicated that cryptophytes dominated the phytoplankton community in Sungo Bay, which was not observed in previous studies (Wang, 2017; Hou et al., 2021). In natural seawater, cryptophytes were rarely reported to be a dominant phytoplankton group, largely due to the omission by microscopic methods (easily deformed or broken in fixed seawater samples) (Gieskes & Kraay, 1983).
HPLC-pigments analysis demonstrated that cryptophytes contribute a high proportion to algal biomass along the coastal areas of China (Wang et al., 2018; Pan et al., 2020). Our results showed that M. coruscus preferentially filtered the cryptophytes and dinoflagellates (Figures 3, 5). The preferential feeding on flagellates has been previously observed in bivalves (Dupuy et al., 2000b; Safi and Hayden, 2010). The mussel Perna canaliculus preferentially ingested flagellated cells, including dinoflagellates and other small flagellates, in the natural seawater from Pelorus Sound (New Zealand) (Safi and Hayden, 2010). Moreover, Naddafi et al. (2007) revealed that a freshwater bivalve species (Dreissena polymorpha) could selectively clear cryptophytes, chrysophyceaens and dinoflagellates. Ren et al. (2006) found the assimilation efficiency of flagellates by mussels was significantly higher than diatoms. Shellfish may have ability to select more nutritious dinoflagellates over diatoms (Menden-Deuer and Lessard, 2000).
Similarly, the laboratory study of Pales Espinosa et al. (2016) reported that cryptophyte Rhodomonas lens and R. salina tended to be more easily filtered by Crassostrea virginica and M. edulis and less rejected through pseudofeces than others.
Retention efficiency of bivalves in the natural seawater is generally dependent on the diameter of particles, which increases non-linearly with particle size (Strohmeier et al., 2012; Rosa et al., 2015). However, the retention efficiency of different particle sizes “varies spatially and seasonally and possibly in direct response to changes in the seston” (Strohmeier et al., 2012). Bivalves may capture smaller particles more efficiently than larger particles due to differences of cell shape, flexibility and swimming ability (Ward and Shumway, 2004). In this study, cryptophytes dominated the phytoplankton community with the size ranging 5-8 μm in diameter, which was much smaller than the other dominant chained diatom Chaetoceros spp. (composing 3-6 cells, 3-4 μm for width and 15-30 μm for length of chains), while the latter was poorly cleared during these experiments. Similar to Chaetoceros spp., also Skeletonema spp. had the chained shapes (composing 4-9 cells, 8-10 μm for width and 50-100 μm for length of chains) (Figure 2). It has previously been suggested bivalves would retain the phytoplankton with narrow widths (e.g.,<3 μm) and length to width ratio >3 at a low efficiency (Rosa et al., 2015), which may be one reason for low capture efficiency of both the large sized diatom species in this study. By comparison, most diatom species (i.e., Pennatae diatoms), which were unicellular and small, and some of which are elliptical (e.g., Pinnularia sp. and Cocconeis scutellum), showed relatively high clearance efficiency (Figures 2, 4). Diatoms may still be the most important food resources for mussels due to their high density and diversity along the Chinese coasts (Wang, 2017; Wang et al., 2018; Pan et al., 2020).
Preferential feeding selection in bivalves occurs in three stages: the first pre-ingestive processing (selective particle retention), the second pre-ingestive processing (particle transport) and post-ingestive processing (Cognie et al., 2001; Ward and Shumway, 2004; Frau et al., 2016). Particle retention is the first step in the feeding process during which captured particles are retained by the ctenidia of bivalves (Rosa et al., 2018). In this study, the preferential filtration of cryptophytes and several diatom genus did not indicate that those contributed significantly to the food source of bivalves because the second pre-ingestive selection (i.e., pseudofeces) was not investigated. A comparison of phytoplankton assemblages between seawater and gut contents could help understand preferential feeding selection (especially for cryptophytes) in bivalves as discussed in Section Comparison of phytoplankton assemblages between seawater and gut contents.
Our results suggested that CE of phytopigments was an effective proxy of evaluating the degree of selective clearance of different phytoplankton groups by M. coruscus. Size-fractionated pigment analysis showed that alloxanthin, peridinin and fucoxanthin distributed in micro- and nano-fractions while prasinoxanthin and zeaxanthin were mainly concentrated in pico-fraction (< 2.7 μm) in the seawater of Sungo Bay (Jiang, unpublished data). Like the results of the microscopic method (Figure 3), the HPLC-pigment analysis indicated that cryptophytes (i.e., alloxanthin) and dinoflagellates (i.e., peridinin) were preferentially cleared by M. coruscus (Figures 6A, B). In agreement with the previous studies (Dupuy et al., 2000b; Strohmeier et al., 2012), our results further demonstrated that M. coruscus filters pico-plankton prasinophytes and Synechococcus (i.e. prasinoxanthin and zeaxanthin, respectively) at lower efficiency than larger planktonic species (Figures 6D, E).
Comparisons of phytoplankton composition between natural seawater and gut contents of bivalves have been conducted with a single sampling, similarly to previous studies (Loret et al., 2000; Tan and Ransangan, 2017; Jiang et al., 2019). The comparisons revealed differences in phytoplankton composition between gut contents and surrounding seawater supporting previous findings (Loret et al., 2000; Tan and Ransangan, 2017; Jiang et al., 2019; Asaduzzaman et al., 2020). Our study has shown that cryptophyte cells dominated in seawater but were not observed in gut contents. By comparison, alloxanthin, the marker pigment of cryptophytes, was selectively cleared from seawater and showed a relatively high proportion in gut content compared to most of the other pigments (Figure 6; Table 3). This demonstrated fast digestion of cryptophytes by M. coruscus. Our results are similar to other studies in which alloxanthin is preferentially accumulated in the gut contents, suggesting the preferential feeding selectivity on cryptophytes by bivalves (Loret et al., 2000; Jiang et al., 2019). This contribution could also be affected by the presence of detrital matter (e.g., aggregates of dead cells) in the water column, which has not been assessed in this study. Similarly, Cucci et al. (1985) revealed that the cryptomonad Chroomonas salina was not found in the feces of M. edulis whereas diatom and dinoflagellate cells could be detected without significant digestion. Moreover, C. salina cells were completely digested and absorbed during passage through the gut by several other bivalve species (Shumway et al., 1985). The non-existence of cryptophyte cells in gut was presumably due to its fragile cell membrane which was broken during the digestion process. Results from this study highlight the importance of HPLC-pigment method in studying food composition in the gut contents of bivalves. Although some fragile flagellates such as cryptophytes may not be easily detected in gut contents by the microscopic method, they can be an important food supply for bivalves in the natural environment (Cucci et al., 1985; Shumway et al., 1985; Loret et al., 2000; Jiang et al., 2019).
Selective filtration of different diatom species by M. coruscus was also observed, with significantly lower proportions of Chaetoceros spp. and Skeletonema spp. in the gut contents than the seawater (Table 2). Rejection of diatoms by bivalves is understood to be species-dependent (also might be size-dependent) (Cucci et al., 1985; Defossez and Hawkins, 1997; Naddafi et al., 2007). Tan & Ransangan (2017) reported P. viridis showed a tendency to reject Chaetoceros spp. Similarly, Bougrier et al. (1997) observed that the ratio of rejection to filtration for some diatoms species (e.g., Chaetoceros and Nitzschia) was greater than that of the flagellates by the oyster Crassostrea gigas. The shape of diatom Chaetoceros spp. and Skeletonema spp. were characterized by spicules and long-chains in the natural seawater as described in Section Preferential feeding selection of Mytilus coruscus. Moreover, the intercalary and terminal setae along two sides of the Chaetoceros chain will greatly span to nearly 30 μm. Defossez and Hawkins (1997) suggested that particles larger than 22.5 μm were preferentially rejected as pseudofeces by M. coruscus. Although the size of single Chaetoceros and Skeletonema cells was around 5-12 μm (Figure 2), the chained cells greatly exceeded this threshold (22.5 μm). In comparison, a significant feeding selectivity of Cocconeis spp. and Pinnularia spp. by M. coruscus was observed. As shown in Figure 2, both diatom species are oval or with rounded ends, without spiny surface and sharp edges. Similarly, Asaduzzaman et al. (2020) showed that P. viridis preferentially ingested the Coscinodiscophyceae, Bacillariophyceae, Fragillariophyceae, as well as Dinophyceae, in the south-east coast of the Bay of Bengal. Therefore, we suggest that the size and shape of diatom species (even flagellates) might be an important factor influencing the feeding selectivity of M. coruscus in this study, which is in agreement with the results of Defossez and Hawkins (1997). Nevertheless, it should be acknowledged that feces and pseudofeces were not collected in this study, and hence the current data are not sufficient for estimating the assimilation efficiency of different diatom assemblages, as well other algal groups. Future efforts should quantitatively analyze the phytoplankton biomass in water, feces, pseudofeces and gut contents, which would further our understanding of filtration and egestion of shellfish on different algal assemblages in natural waters.
According to Sonier et al. (2016), picophytoplankton can contribute to mussel growth in intensive culture environments where picophytoplankton biomass is high. Retention efficiencies of picophytoplankton by Mytilus edulis was estimated 20 ± 2.0% in eastern Prince Edward Island (Sonier et al., 2016). In agreement with Sonier et al. (2016), our results suggested that picoplankton could be an important fraction of the diet. In this study, prasinoxanthin and zeaxanthin had the clearance efficiency of 27%-31% at RI = 1.0 (Figures 6D, E), and it was further evidenced by their presence in gut contents (Figure S2). Aggregation of picoplankton might be an important way for the filtration of these small particles by of bivalves (Ward and Shumway, 2004). Moreover, compared with other filter-feeding bivalves (e.g., oysters), mussels more efficiently filter and retain picophytoplankton (Richard et al., 2022).
Feeding experiments were carried out in short time (0.26-1 h) with high biomass of M. coruscus (1 mussel l-1) in a static chamber. Under these conditions, our results suggested M. coruscus can change the composition of phytoplankton community by selective clearance. Flagellated phytoplankton cells, especially cryptophytes, may be subject to greater filtration pressure than some diatom species under the feeding behavior of M. coruscus. Similarly, field investigation revealed that flagellates were selectively cleared by intensive oyster aquaculture (Jiang et al., 2016). This study also showed that mussels removed picoplankton at lower efficiency than the larger planktonic species (Figure 6), which was consistent with previous reports (Dupuy et al., 2000b; Strohmeier et al., 2012). This process may skew the phytoplankton community toward the relatively fast-growing pico-sized species. It was reported that picoplankton contributed a high proportion to total phytoplankton biomass in some shellfish aquaculture areas (Vaquer et al., 1996; Safi and Gibbs, 2003; Cranford et al., 2008; Jiang et al., 2016). Weissberger and Glibert (2021) reported that the oyster Crassostrea virginica preferentially filtered large phytoplankton cells (e.g., diatoms and flagellates) and rejected small cells (cyanobacteria), which would be another mechanism by which bivalve filter-feeding may impact phytoplankton communities. In addition to small cell size, low nutritional value may be a reason why filter-feeding bivalves do not feed extensively on picoplankton (Weissberger and Glibert, 2021). The selective feeding process of bivalves may be the dominant factor that determines the size distribution of the phytoplankton community in semi-enclosed areas with high cultured bivalve biomass (Vaquer et al., 1996; Jiang et al., 2016).
Several diatom species, such as Chaetoceros spp. and Skeletonema spp. showed lower clearance efficiency than other diatoms from seawater by M. coruscus, and also less aggregation in gut contents (Table 2). Other studies have found that Chaetoceros spp. and Pseudo-nitzschia spp. are easily rejected in pseudofeces in some bivalves (Mafra et al., 2009; Safi and Hayden, 2010; Tan & Ransangan, 2017). Furthermore, some algal species can survive in the pseudofeces and feces and return to the water column (Santelices and Correa, 1985; Barillé & Cognie, 2000). These diatoms are the most common species and frequently dominate the phytoplankton community in the near shore waters along the coasts of China, especially in aquaculture areas (Wang et al., 2006; Wang, 2017). Lower filtration and/or higher rejection for these species than other diatoms might increase the potential tendency toward their domination in seawater.
Intensive bivalve cultivation could cause a significant reduction of the micro and nano-sized diatoms and dominance of picophytoplankton in the farm area (Jiang et al., 2016). Apart from bivalve filtration activity, dynamics and size distribution of phytoplankton are influenced by combined environmental factors, such as water residence time, light intensity and nutrients regime (Naddafi et al., 2007; Filgueira et al., 2012; Filgueira et al., 2016). Consequently, the environmental impact of bivalve aquaculture is site-specific, which highlights the importance of site selection for shellfish aquaculture (Silva et al., 2011). Researchers rarely considered the size structure and taxa composition of phytoplankton when they investigated the environmental impact and carrying capacity of shellfish aquaculture by numerical models, such as FARM model (Ferreira et al., 2007; Silva et al., 2011). This study further suggests that species-dependent (also size-dependent) disturbance of phytoplankton community should be considered for evaluating the environmental impact of bivalve cultivation in future studies.
This study highlights the usefulness of combining HPLC-pigment analysis with microscopic methods in providing a better understanding of the effect of feeding behavior of bivalves on phytoplankton communities. Of the available phytoplankton diet, M. coruscus preferentially filtered larger sized flagellates e.g., cryptophytes, compared to smaller species. However, cryptophytes were not observed in gut contents by microscopy while the marker pigment (i.e., alloxanthin) showed a relatively high proportion by HPLC-pigment analysis. This result suggests fast digestion of cryptophytes by M. coruscus and implied that microscopic observation of gut contents could underestimate their contribution to the diet of mussels. Selective feeding of different diatom species by M. coruscus was also observed, with significantly lower proportions of Chaetoceros spp. and Skeletonema spp. in gut contents than the seawater. The size and shape of diatom species might be an important factor influencing the feeding selectivity of mussels. Picoplankton cannot be neglected as potential food in the natural environment, as evidenced by the presence of their characteristic pigments in the gut. This selective feeding suggests that intensive mariculture of M. coruscus may skew the phytoplankton community toward the relatively fast-growing pico-sized species.
The raw data supporting the conclusions of this article will be made available by the authors, without undue reservation.
Ethical review and approval was not required for the animal study because the bivalve Mytilus coruscus used in this experiment were provided by Rongcheng Chudao Aquatic Products Co. Ltd. Ethical approval was not required for this study because no endangered animals were involved. Specimen collection and maintenance were performed in strict accordance with the recommendations of Animal Care Quality Assurance in China.
TJ, HP and LS wrote the original manuscript. ZJ, RF, ØS, TS, PC, and ZC attended the experiments and took part in the data analysis. All authors contributed to the article and approved the submitted version.
This study was funded by the National Key Research and Development Program of China (2019YFD0901401), the National Natural Science Foundation of China (42176206), Natural Science Foundation of Shandong Province (ZR2021MD071), the project Environment and Aquaculture Governance (CHN17/0033, Ministry of Foreign Affairs, Norway), Major Scientific and Technological Innovation Project of Shandong Provincial Key Research and Development Program (2019JZZY020706).
We would like to thank Dr. X. Xing and Mr. J. Cen for their help in identifying the species of phytoplankton. We are also grateful to Miss M. Bai and Dr. W. Jiang who aided in field investigation and sample analysis.
The authors declare that the research was conducted in the absence of any commercial or financial relationships that could be construed as a potential conflict of interest.
All claims expressed in this article are solely those of the authors and do not necessarily represent those of their affiliated organizations, or those of the publisher, the editors and the reviewers. Any product that may be evaluated in this article, or claim that may be made by its manufacturer, is not guaranteed or endorsed by the publisher.
The Supplementary Material for this article can be found online at: https://www.frontiersin.org/articles/10.3389/fmars.2022.1070737/full#supplementary-material
Asaduzzaman M., Akter S., Hoque N. F., Shakil A., Noor A. R., Akter M. N., et al. (2020). Multifaceted linkages among eco-physiological factors, seasonal plankton dynamics and selective feeding behavior of the green mussel (Perna viridis) in the south-east coast of the bay of Bengal. J. Sea Res. 164, 101933. doi: 10.1016/j.seares.2020.101933
Barillé L., Cognie B. (2000). Revival capacity of diatoms in bivalve pseudofaeces and faeces. Diatom Res. 15 (1), 11–17. doi: 10.1080/0269249x.2000.9705483
Bayne B., Worrall C. (1980). Growth and production of mussels Mytilus edulis from two populations. Mar. Ecol. Prog. Ser. 3 (4), 317–328. doi: 10.3354/meps003317
Beninger P. G., Valdizan A., Decottignies P., Cognie B. (2008). Impact of seston characteristics on qualitative particle selection sites and efficiencies in the pseudolamellibranch bivalve Crassostrea gigas. J. Exp. Mar. Biol. Ecol. 360, 9–14. doi: 10.1016/j.jembe.2008.03.003
Bougrier S., Hawkins A. J. S., Héral M. (1997). Preingestive selection of different microalgal mixtures in crassostrea gigas and mytilus edulis, analysed by flow cytometry. Aquaculture 150 (1-2), 123–134. doi: 10.1016/s0044-8486(96)01457-3
Cédric B., Jon G., Anthony H., Fang J., Zhu M., Mélanie B. (2003). Modelling the effect of food depletion on scallop growth in sungo bay (China). Aquat. Living Resour. 16 (1), 10–24. doi: 10.1016/s0990-7440(03)00003-2
Cognie B., Barillé L., Rincé Y. (2001). Selective feeding of the Oystercrassostrea gigas fed on a natural microphytobenthos assemblage. Estuaries 24 (1), 126–134. doi: 10.2307/1352819
Cranford P. J., Li W., Strand Ø., Strohmeier T. (2008). Phytoplankton depletion by mussel aquaculture, high resolution mapping, ecosystem modeling, and potential indicators of ecological carrying capacity. International Council for the Exploration of the Seas (ICES).
Cranford P. J., Strohmeier T., Filgueira R., Strand Ø. (2016). Potential methodological influences on the determination of particle retention efficiency by suspension feeders: Mytilus edulis and Ciona intestinalis. Aquat. Biol. 25, 61–73. doi: 10.3354/ab00660
Cucci T. L., Shumway S. E., Newell R. C., Selvin R., Guillard R. L., Yentsch ,. C. M. (1985). Flow cytometry: a new method for characterization of differential ingestion, digestion and egestion suspension feeders. Mar. Ecol. Prog. Ser. 24, 201–204. doi: 10.3354/meps024201
Dame R. F., Prins ,. T. C. (1998). Bivalve carrying capacity in coastal ecosystems. Aquat. Ecol. 31, 409–421. doi: 10.1023/A:1009997011583
Defossez J. M., Hawkins ,. A. J. S. (1997). Selective feeding in shellfish: size-dependent rejection of large particles within pseudofaeces from Mytilus edulis, Ruditapes philippinarum and Tapes decussatus. Mar. Biol. 129 (1), 139–147. doi: 10.1007/s002270050154
Dupuy C., Vaquer A., Lam-Hoai T., Rougier C., Mazouni N., Lautier J., et al. (2000b). Feeding rate of the oyster crassostrea gigas in a natural planktonic community of the Mediterranean thau lagoon. Mar. Ecol. Prog. Ser. 205 (1), 171–184. doi: 10.3354/meps205171
Ferreira J. G., Hawkins A. J. S., Bricker S. B. (2007). Management of productivity, environmental effects and profitability of shellfish aquaculture — the farm aquaculture resource management (FARM) model. Aquaculture 264, 160–174. doi: 10.1016/j.aquaculture.2006.12.017
Filgueira R., Grant J., Bacher C., Carreau M. (2012). A physical-biogeochemical coupling scheme for modeling marine coastal ecosystems. Ecol. Inform. 7, 71–80. doi: 10.1016/j.ecoinf.2011.11.007
Filgueira R., Guyondet T., Comeau L. A., Tremblay R. (2016). Bivalve aquaculture-environment interactions in the context of climate change. Global Change Biol. 22, 3901–3913. doi: 10.1111/gcb.13346
Frau D., Molina F. R., Mayora G. (2016). Feeding selectivity of the invasive mussel Limnoperna fortune (Dunker 1857) on a natural phytoplankton assemblage: what really matters? Limnology 17 (1), 47–57. doi: 10.1007/s10201-015-0459-2
Gieskes W. W. C., Kraay G. W. (1983). Dominance of cryptophyceae during the phytoplankton spring bloom in the central north Sea detected by HPLC analysis of pigments. Mar. Biol. 75 (2-3), 179–185. doi: 10.1007/bf00406000
Grizzle R. E., Bricelj V. M., Shumway S. E. (2001). Chapter 8 physiological ecology of mercenaria mercenaria. Dev. Aquacult. Fish. Sci. 31 (1), 305–382. doi: 10.1016/s0167-9309(01)80036-3
Hawkins A., Smith R., Bayne B. L., Héral M. (1996). Novel observations underlying the fast growth of suspension-feeding shellfish in turbid environments: Mytilus edulis. Mar. Ecol. Prog. Ser. 131 (1-3), 179–190. doi: 10.3354/meps131179
Hou X., Gao Y., Du M., Jiang W., Li F., Dong S., et al. (2021). Temporal and spatial variation in phytoplankton community structure and their relationship with environmental factors in sanggou bay. Prog. Fish Sci. 42 (2), 18–27. doi: 10.19663/j.issn2095-9869.20200130001
Jiang T., Chen F., Yu Z., Lu L., Wang Z. (2016). Size-dependent depletion and community disturbance of phytoplankton under intensive oyster mariculture based on HPLC pigment analysis in daya bay, south China Sea. Environ. Pollut. 219, 804–814. doi: 10.1016/j.envpol.2016.07.058
Jiang T., Wang L., Zhang F., Fang X., Lu L., Zhang J., et al. (2019). Selective feeding of bay scallop Argopecten irradians on phytoplankton community revealed by HPLC analysis of phytopigments in bohai Sea, China. J. Oceanol. Limnol. 37, 1746–1755. doi: 10.1007/s00343-019-8280-0
Kamermans P. (1994). Similarity in food source and timing of feeding in deposit- and suspension-feeding bivalves. Mar. Ecol. Prog. Ser. 104 (1), 63–75. doi: 10.3354/meps104063
Lavaud R., Artigaud S., Le Grand F., Donval A., Soudant P., Flye-Sainte-Marie J. F., et al. (2018). New insights into the seasonal feeding ecology of Pecten maximus using pigments, fatty acids and sterols analyses. Mar. Ecol. Prog. Ser. 590, 109–129. doi: 10.3354/meps12476
Loret P., Pastoureaud A., Bacher C., Delesalle B. (2000). Phytoplankton composition and selective feeding of the pearl oyster Pinctada margaritifera in the takapoto lagoon (Tuamotu archipelago, French polynesia): in situ study using optical microscopy and HPLC pigment analysis. Mar. Ecol. Prog. Ser. 199, 55–67. doi: 10.3354/meps199055
Mafra L. L., Bricelj V. M., Ward J. E. (2009). Mechanisms contributing to low domoic acid uptake by oysters feeding on Pseudo-nitzschia cells. II. selective rejection. Aquat. Biol. 6 (1-3), 213–226. doi: 10.3354/ab00122
Menden-Deuer S., Lessard ,. E. J. (2000). Carbon to volume relationships for dinoflagellates, diatoms, and other protist plankton. Limnol. Oceanogr. 45, 569–579. doi: 10.4319/lo.2000.45.3.0569
Naddafi R., Pettersson K., Eklöv P. (2007). The effect of seasonal variation in selective feeding by zebra mussels (Dreissena polymorpha) on phytoplankton community composition. Freshw. Biol. 52, 823–842. doi: 10.1111/j.1365-2427.2007.01732.x
Nakamura Y., Kerciku F. (2000). Effects of filter-feeding bivalves on the distribution of water quality and nutrient cycling in a eutrophic coastal lagoon. J. Mar. Syst. 26, 209–221. doi: 10.1016/s0924-7963(00)00055-5
Pales Espinosa E. P., Cerrato R. M., Wikfors G. H., Allam B. (2016). Modeling food choice in the two suspension-feeding bivalves, Crassostrea virginica and Mytilus edulis. Mar. Biol. 163 (2), 40. doi: 10.1007/s00227-016-2815-0
Pan H., Li A., Cui Z., Ding D., Qu K., Zheng Y., et al. (2020). A comparative study of phytoplankton community structure and biomass determined by HPLC-CHEMTAX and microscopic methods during summer and autumn in the central bohai Sea, China. Mar. pollut. Bull. 155, 111172. doi: 10.1016/j.marpolbul.2020.111172
Ren J. S., Ross A. H., Hayden B. J. (2006). Comparison of assimilation efficiency on diets of nine phytoplankton species of the greenshell mussel Perna canaliculus. J. Shellfish Res. 25, 887–892. doi: 10.2983/0730-8000(2006)25[887:coaeod]2.0.co;2
Richard M., Bec B., Bergeon L., Hébert M., Mablouké C., Lagarde F. (2022). Are mussels and oysters capable of reducing the abundances of picochlorum sp., responsible for a massive green algae bloom in thau lagoon, France? J. Exp. Mar. Biol. Ecol. 556, 151797. doi: 10.1016/j.jembe.2022.151797
Riisgård H. (1988). Efficiency of particle retention and filtration rate in 6 species of northeast American bivalves. Mar. Ecol. Prog. Ser. 45, 217–223. doi: 10.3354/meps045217
Riisgård H., Larsen P. (2010). Particle capture mechanisms in suspension-feeding invertebrates. Mar. Ecol. Prog. Ser. 418, 255–293. doi: 10.3354/meps08755
Rosa M., Ward J. E., Holohan B. A., Shumway S. E., Wikfors G. H. (2017). Physicochemical surface properties of microalgae and their combined effects on particle selection by suspension-feeding bivalve molluscs. J. Exp. Mar. Biol. Ecol. 486, 59–68. doi: 10.1016/j.jembe.2016.09.007
Rosa M., Ward J. E., Ouvrard M., Holohan B. A., Espinosa E. P., Shumway S. E., et al. (2015). Examining the physiological plasticity of particle capture by the blue mussel, Mytilus edulis, (L.): Confounding factors and potential artifacts with studies utilizing natural seston. J. Exp. Mar. Biol. Ecol. 473, 207–217. doi: 10.1016/j.jembe.2015.09.005
Rosa M., Ward J. E., Shumway S. E. (2018). Selective capture and ingestion of particles by suspension-feeding bivalve molluscs: A review. J. Shellfish Res. 37 (4), 727–746. doi: 10.2983/035.037.0405
Rosa M., Ward J. E., Shumway S. E. (2021). Examining the effects of microalgal metabolites on ciliary activity of the Eastern oyster crassostrea virginica. J. Shellfish Res. 40 (2), 231–237. doi: 10.2983/035.040.0204
Rouillon G., Guerra Rivas J., Ochoa N., Navarro E. (2005). Phytoplankton composition of the stomach contents of the mussel Mytilus edulis l. from two populations: comparison with its food supply. J. Shellfish Res. 24 (1), 5–14. doi: 10.2983/0730-8000(2005)24[5:PCOTSC]2.0.CO;2
Safi K. A., Gibbs M. M. (2003). Importance of different size classes of phytoplankton in beatrix bay, Marlborough sounds, new Zealand, and the potential implications for the aquaculture of the mussel, Perna canaliculus. N. Z. J. Mar. Freshw. Res. 37, 267–272. doi: 10.1080/00288330.2003.9517164
Safi K. A., Hayden B. (2010). Differential grazing on natural planktonic populations by the mussel Perna canaliculus. Aquat. Biol. 11, 113–125. doi: 10.3354/ab00297
Santelices B., Correa J. (1985). Differential survival of macroalgae to digestion by intertidal herbivore molluscs. J. Exp. Mar. Biol. Ecol. 88, 183–191. doi: 10.1016/0022-0981(85)90037-1
Shumway S. E., Cucci T. L., Newell R. C., Yentsch C. M. (1985). Particle selection, ingestion, and absorption in filter-feeding bivalves. J. Exp. Mar. Biol. Ecol. 91 (1-2), 77–92. doi: 10.1016/0022-0981(85)90222-9
Silva C., Ferreira J. G., Bricker S. B., DelValls T. A., Martín-Díaz M. L., Yáñez E. (2011) Site selection for shellfish aquaculture by means of GIS and farm-scale models, with an emphasis on data-poor environments. Aquaculture 318 (3–4), 444–457. doi: 10.1016/j.aquaculture.2011.05.033
Sonier R., Filgueira R., Guyondet T., Tremblay R., Olivier F., Meziane T., et al. (2016). Picophytoplankton contribution to Mytilus edulis, growth in an intensive culture environment. Mar. Biol. 163, 1–15. doi: 10.1007/s00227-016-2845-7
Strohmeier T., Strand Ø., Alunno-Bruscia M., Duinker A., Cranford ,. P. J. (2012). Variability in particle retention efficiency by the mussel Mytilus edulis. J. Exp. Mar. Biol. Ecol. 412 (3), 96–102. doi: 10.1016/j.jembe.2011.11.006
Tan K. S., Ransangan J. (2017). Feeding behaviour of green mussels, Perna viridis farmed in marudu bay, Malaysia. Aquacult. Res. 48, 1216–1231. doi: 10.1111/are.12963
Trottet A., Roy S., Tamigneaux E., Lovejoy C., Tremblay R. (2008). Impact of suspended mussels (Mytilus edulis l.) on plankton communities in a magdalen islands lagoon (Québec, canada): A mesocosm approach. J. Exp. Mar. Biol. Ecol. 365 (2), 103–115. doi: 10.1016/j.jembe.2008.08.001
Vahl O. (1972). Efficiency of particle retention in Mytilus edulis l. Ophelia 10, 17–25. doi: 10.1080/00785326.1972.10430098
Vaquer A., Troussellier M., Courties C., Bibent B. (1996). Standing stock and dynamics of picoplankton in the thau lagoon (northwest Mediterranean coast). Limnol. Oceanogr. 41 (8), 1821–1828. doi: 10.4319/lo.1996.41.8.1821
Venrick E. L. (1978). “How many cells to count?,” in Phytoplankton manual. Ed. Sournia A. (UNESCO), 167–180.
Wang X. (2017). Studies on phytoplankton community under integrated multi-trophic aquaculture modes in sanggou bay (Guangzhou: MSc dissertation, Jinan University).
Wang L., Ou L., Huang K., Chai C., Wang Z., Wang X., et al. (2018). Determination of the spatial and temporal variability of phytoplankton community structure in daya bay via HPLC-CHEMTAX pigment analysis. J. Oceanol. Limnol. 36 (3), 750–760. doi: 10.1007/s00343-018-7103-z
Wang Z., Qi Y., Chen J., Ning X., Yang Y. (2006). Phytoplankton abundance, community structure and nutrients in cultural areas of daya bay, south China Sea. J. Mar. Syst. 62 (1), 85–94. doi: 10.1016/j.jmarsys.2006.04.008
Ward J. E., Shumway ,. S. E. (2004). Separating the grain from the chaff: particle selection in suspension- and deposit-feeding bivalves. J. Exp. Mar. Biol. Ecol. 300 (1-2), 83–130. doi: 10.1016/j.jembe.2004.03.002
Weissberger E. J., Glibert P. M. (2021). Diet of the eastern oyster, crassostrea virginica, growing in a eutrophic tributary of Chesapeake bay, Maryland, USA. Aquacult Rep. 20, 100655. doi: 10.1016/j.aqrep.2021.100655
Yahel G., Eerkes-Medrano D. I., Leys S. P. (2006). Size dependent selective filtration of ultraplankton by hexactinellid glass sponges. Aquat. Microb. Ecol. 45 (2), 181–194. doi: 10.3354/ame045181
Yahel G., Marie D., Beninger P. G., Eckstein S., Genin A. (2009). In situ evidence for pre-capture qualitative selection in the tropical bivalve lithophaga simplex. Aquat. Biol. 6 (1-3), 235–246. doi: 10.3354/ab00131
Keywords: feeding selectivity, preferential feeding, phytoplankton, pigments, Mytilus coruscus, Sungo Bay
Citation: Jiang T, Pan H, Steeves L, Jiang Z, Filgueira R, Strand Ø, Strohmeier T, Cranford PJ and Cui Z (2022) Effect of Mytilus coruscus selective filtration on phytoplankton assemblages. Front. Mar. Sci. 9:1070737. doi: 10.3389/fmars.2022.1070737
Received: 15 October 2022; Accepted: 31 October 2022;
Published: 22 November 2022.
Edited by:
Meilin Wu, South China Sea Institute of Oceanology (CAS), ChinaReviewed by:
Zhanhui Qi, Key Laboratory of South China Sea Fishery Resources Exploitation and Utilization, South China Sea Fisheries Research Institute (CAFS), ChinaCopyright © 2022 Jiang, Pan, Steeves, Jiang, Filgueira, Strand, Strohmeier, Cranford and Cui. This is an open-access article distributed under the terms of the Creative Commons Attribution License (CC BY). The use, distribution or reproduction in other forums is permitted, provided the original author(s) and the copyright owner(s) are credited and that the original publication in this journal is cited, in accordance with accepted academic practice. No use, distribution or reproduction is permitted which does not comply with these terms.
*Correspondence: Tao Jiang, amlhbmd0YW9waHlAMTYzLmNvbQ==; Zhengguo Cui, Y3VpemdAeXNmcmkuYWMuY24=
Disclaimer: All claims expressed in this article are solely those of the authors and do not necessarily represent those of their affiliated organizations, or those of the publisher, the editors and the reviewers. Any product that may be evaluated in this article or claim that may be made by its manufacturer is not guaranteed or endorsed by the publisher.
Research integrity at Frontiers
Learn more about the work of our research integrity team to safeguard the quality of each article we publish.