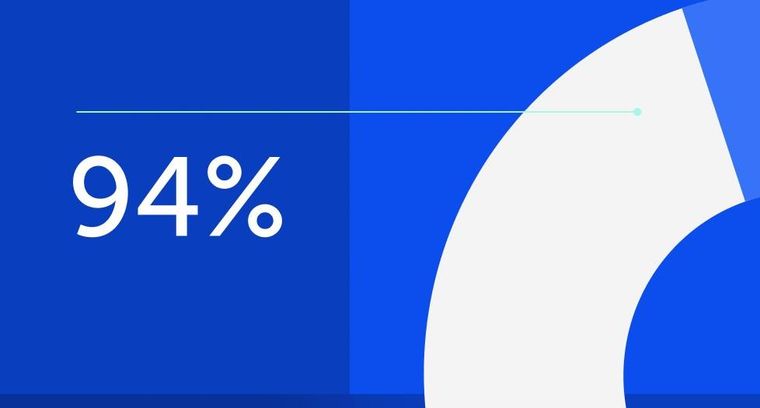
94% of researchers rate our articles as excellent or good
Learn more about the work of our research integrity team to safeguard the quality of each article we publish.
Find out more
ORIGINAL RESEARCH article
Front. Mar. Sci., 02 December 2022
Sec. Aquatic Physiology
Volume 9 - 2022 | https://doi.org/10.3389/fmars.2022.1068997
This article is part of the Research TopicMicrobiome dynamics as biomarkers of welfare status in aquatic speciesView all 6 articles
The individual Probiotic application has become increasingly widespread in aquaculture and has been extensively studied. However, investigating probiotics as water additives in the grouper culture is still lacking. This study evaluated the functional efficacy of the Bacillus subtilis CICC 10071 (3 × 1011 CFU / g) and Lactobacillus sp. (8 × 1011 CFU / g) combination in a 1:1 ratio on the rearing water quality, water microbial community structure, and growth performance of hybrid grouper. Depending on the additive concentrations of probiotics, we designed four groups, each in triplicate: control (WT, 0g/m3), low concentration (WL, 0.038g/m3), middle concentration (WM, 0.075g/m3), and high concentration (WH, 0.113g/m3). The result shows that throughout the 22-day feeding period, the water supplementation of probiotics significantly decreased Ammonia (NH3) and nitrite (NO2-) in culture water. Final weight (FW), Specific growth rate (SGR), and Weight gain rate (WGR) in treated groups were higher than that in the control group (P<0.05). Analysis of water microbiota revealed that the dominant phylum Bacteroidetes, Proteobacteria, Cyanobacteria, and Actinobacteria enriched in the culture water. Furthermore, we found that the Probiotics combination could significantly reduce the abundance of Cetobacterium (phyla Fusobacteria) related to ammonia and nitrite. The Phylogenetic Investigation of Communities by Reconstruction of Unobserved States 2 (PICRUSt2) also shows that the 'metabolism of other amino acids and ‘Fatty acid biosynthesis’ functions of water microbiota were reinforced by the addition of the probiotic combination. Thus, the probiotic combination exhibited a range of advantages in the grouper culture environment, and further in-depth studies are needed.
Aquaculture is a rapidly expanding sector that presently supplies more than half of the world’s human fish consumption (Theuerkauf et al., 2019; Gephart et al., 2020). Currently, the grouper (Epinephelus spp.) is one of the most important coastal aquaculture species in many Asian countries (Pierre et al., 2008). In 2022, the production of grouper in China (including Taiwan) reached 204,119 tons (Fisheries, M.o.A.a.R.A.o.t.P.s.R.o.C.N.F.T.E.C.o.C.C.S.o, 2022). The culture of grouper has the characteristics of high input costs and risks as it requires a long breeding cycle (8–16 months), attentive management, and steady water quality. In the coastal areas of China, ponds and small-scale cages comprise the main methods to culture grouper (Li et al., 2011). In pond water, heterotrophic bacteria can degrade fish feces and feed residues into nitrogen- and phosphorous-containing inorganic matter for use by microorganisms and algae (Ni et al., 2018). However, overfeeding and a high stocking density will excessively increase inorganic matter (Person-Le Ruyet et al., 2008; Zhang et al., 2020). Huge amounts of nitrogenous accumulation (ammonia and nitrite) induce a deterioration in the water quality, disease outbreaks, and the stress response of the fish body, causing the fish to finally die (Eddy, 2005). In order to address farmers’ needs, it is essential to develop an effective, reproducible, and environment-friendly pond culture technology (Rimmer and Glamuzina, 2019).
Probiotics are beneficial bacterial products for the maintenance of fish health and the culture environment, which could be safe alternatives to synthetic antibiotics (Dawood et al., 2019; Amenyogbe et al., 2020; Amenyogbe et al., 2022b). In recent years, the application of probiotics for bioremediation has been a research hotspot regarding sustainable aquaculture (Amenyogbe et al., 2020). It has been extensively used in the culture practice of different aquatic animals (Amenyogbe et al., 2020; Amenyogbe et al., 2022a; Amenyogbe et al., 2022b; Amenyogbe et al., 2022c). The addition of different probiotic strains has several benefits, including the decomposition of organic matter, removal of nutrients, and promotion of fish growth performance (El-Haroun et al., 2006; Yun et al., 2021). Currently, the most notable candidate strains for probiotic application are Bacillus and lactic acid bacteria (Soltani et al., 2019; Chizhayeva et al., 2022). Bacillus species are Gram-positive, endospore-forming aerobic or facultative anaerobes, all rod-shaped bacteria (Soltani et al., 2019), which have been widely used in aquaculture, producing digestion- and immune-related enzymes that benefit aquatic animals (Ray et al., 2010; Askarian et al., 2012). The Bacillus subtilis strain 7K, isolated from the hybrid grouper gastrointestinal tract, could inhibit iridovirus infection and stimulate growth performance (Zhou et al., 2019). Insightful molecular research revealed that the indigenous Bacillus influenced Toll-like receptor/myeloid differentiation factor 88 (TLR/MyD88) signaling in the grouper intestine (Yang et al., 2019). Similarly, Lactobacillus sakei BK19 could be used as feed in addition to strengthening the immunity status of kelp grouper (Epinephelus bruneus) (Harikrishnan et al., 2010).
Many scholars have already focused on the beneficial functions of Bacillus and Lactobacillus as feed additives for grouper (Reyes-Becerril et al., 2014; Shiu et al., 2015; He et al., 2017). However, the practical application of probiotic combinations, such as water additives, in aquatic environments is less well studied. In terms of higher adhesion and more antimicrobial compounds, the use of multispecies probiotics is perhaps more effective than that of monospecies (Nayak, 2010; Amenyogbe et al., 2022a). We expect a probiotic cocktail to target the improvement of the growth performance of grouper and the prevention of the quick collapse of aquatic environments. In this study, a control group and three experimental groups with different amounts of added probiotics (from low to high) were designed. The beneficial impacts of probiotic addition on the grouper growth performance and the culture water quality were evaluated. Furthermore, we investigated the compositional and functional changes of the microbial community within the rearing water after probiotic addition.
The study was carried out on the breeding farm of Guangdong Evergreen Feed Industry Co., Ltd., Donghai Island, MaZhang District, Zhanjiang City, Guangdong Province, China (20°97′34.8" ′N, 110°52′59.6" E). An open plastic film pond under a natural environment was constructed. A total of 12 fiberglass tanks (50 cm × 40 cm × 110 cm) were fixed on the surface of the pond water, each tank containing 200 L of seawater. The seawater was pumped up from the coastal area and settled in the dark sedimentation tank for 24 h. Thereafter, the seawater in the experimental tanks was continuously aerated for 24 h. Prior to the experiment, the aquaculture water had high transparency and no apparent water color.
The probiotic product is a powder formulation that was stored at 4°C to avoid microbial contamination. The viability and the concentration of the probiotic species were studied following the methods described by (Amenyogbe et al., 2022a; Amenyogbe et al., 2022b; Amenyogbe et al., 2022c), with slight modifications. In summary, 1 g of the probiotic powder was homogenized in 9.0 ml of sterile phosphate-buffered saline (PBS) and serially diluted. A volume of 0.1 ml was then spread in triplicate on MRS agar medium (Lactobacillus; ThermoFisher Scientific, Shanghai, China) and NGA agar medium (Bacillus): 10.0 g of beef extract, 10.0 g of peptone, 10.0 g of glucose, 5.0 g of NaCl, 15.0 g of agar, and 1,000 ml distilled water (pH 7.0). The colonies were incubated at 30°C for 72 h under microaerophilic (Lactobacillus) and aerophilic (Bacillus) conditions and counted weekly. The highest level of viability was found in the first week of an experimental study on the survival of probiotics before this one. We maintained the probiotic levels in the culture water by supplementing the probiotic product at 3-day intervals.
For the experiment, 300 healthy hybrid groupers (3 months old; Epinephelus fuscoguttatus ♀× Epinephelus polyphekadion ♂) were purchased from a grouper aquafarm on Donghai Island. After acclimatizing for 14 days, 240 healthy hybrid groupers with similar body weights (average initial weight = 35.94 ± 0.26 g) were randomized to the experimental tanks (N = 20 fish per tank). Each tank contained two air stones for the maintenance of adequate dissolved oxygen content.
The commercial probiotic Nitriclear® was produced by Bairui Biotech Company (Foshan, China; http://www.brshengwu.cn/news.aspx?nid=251). The probiotic powder contained Lactobacillus sp. (8 × 1011 CFU/g) and B. subtilis CICC 10071 (3 × 1011 CFU/g), which were purchased from the Chinese Center of Industrial Culture Collection, mixed in a 1:1 ratio. The added dose was divided into four grades according to the company recommendations: WT (control group; 0 g/m3, i.e., 0 g/ha), WL (low-concentration group; 0.038 g/m3, i.e., 375 g/ha), WM (moderate-concentration group; 0.075 g/m3, i.e., 750 g/ha), and WH (high-concentration group; 0.113 g/m3, i.e., 1,125 g/ha). Each treatment had three replicate tanks. The probiotic product was mixed with clear seawater and added to the culture water at 11 a.m. every 3 days (days 1, 4, 7, 10, 13, 16, and 19). The fish were fed with a commercial feed (crude protein, ≥40%; crude fibre, ≤5.0%; crude ash, ≤16%; crude lipid, ≥6%; moisture, ≤12%; total phosphorus, 0.90–1.60; and lysine, ≥2.10) (Guangdong Yuequn Marine Biology Research and Development Co., Ltd., Jieyang, China) twice daily (at 9 a.m. and 5 p.m.) at a rate of 3% of the fish body weight. No leftover feeds were observed throughout the experimental period. The water was cleansed of fish feces through siphoning 4 h after the first feeding every day throughout the trial period. The experiment was performed in August 2021 and lasted 22 days. No water was exchanged during the experiments.
In order to monitor the changes in the water quality parameters during the trial period, before the application of probiotics, water sampling was carried out at 10 a.m. every 3 days (days 1, 4, 7, 10, 13, 16, 19, and 22). The culture water sample in each tank was sampled using 500 ml organic glass hydrophore and kept in 12 plastic bottles. Subsequently, the sampling bottles were kept on ice and immediately delivered to the laboratory for further analysis. The total ammonia (NH3), nitrite (), nitrate (), and phosphate () of the culture water were analyzed with the SmartChem® 200 Wet Chemistry Analyzer (KPM Analytics, Westborough, MA, USA). The salinity of the culture water was monitored in a suit daily using the salinity detector AZ8371 (AZ Instrument, Taichung City, Taiwan). The pH was detected with a PHSJ-3F pH meter (INESA Scientific Instrument Co., Ltd, Shanghai, China).
All grouper were taken from the experimental tanks after 22 days to measure the fish body and liver weight. The body length of the grouper was also measured. The final body weight (FW), weight gain rate (WGR), hepatosomatic index (HSI), condition factor (CF), and specific growth rate (SGR) were calculated according to the following equations:
where W0 is the initial weight and Wt the final weight of the grouper in grams.
where Wc is the weight of the liver in grams.
where L is the body length of the grouper in centimeters.
where d represents the breeding days.
where Si is the initial number of fish and SF the final number of surviving fish.
Microbiota samples of the culture water were collected using a GM-0.33A diaphragm vacuum pump (Jinten, Tianjin, China), with three biological replicates for each group. Microporous filters (0.45 μm) with microbiota were placed into a 15-ml sterile tube and stored at −80°C in an ultra-low freezer until subsequent experiments.
The genome DNA of water bacteria was extracted using HiPure Soil DNA Kit (model D3142; Meiji Biotechnology Co., Ltd., Guangzhou, China) according to the manufacturer’s instructions. In the PCR process, the targeted V3–V4 regions of DNA were explicitly amplified using the following primers: 341F (5′-CCTACGGGNGGCWGCAG-3′) and 806R (5′-GGACTACHVGGGTATCTAAT-3′). The PCR products were quantified in a NanoDrop 2000 spectrophotometer (Nanodrop, Wilmington, DE, USA), and the integrity was checked with the agarose gel electrophoresis method. The library was sequenced in Illumina Novaseq 6000 (Illumina, San Diego, CA, USA). Gene Denovo Biotechnology Co., Ltd (Guangzhou, China) performed all the aforementioned steps. Illumina reads were deposited in the National Center for Biotechnology Information (NCBI) database under NCBI Bio-project PRJNA893097.
All data were statistically analyzed using SPSS software version 24 (SPSS Inc., Chicago, Il, USA) and calculated using one-way analysis of variance (ANOVA). Tukey’s and the Games–Howell post-hoc tests were used depending on the results of the homogeneity of variances. Bioinformatic analysis of the water microbiota was performed on Omicsmart (http://www.omicsmart.com).
As shown in Table 1 and Figure 1, the final pH values ranged between 7.77 and 7.35, with the values of the probiotic-treated culture water groups being significantly lower than that of the WT group (p< 0.05). As the experiment progressed, the pH value decreased, showing a fluctuation in all groups. In terms of the nitrogen-related physicochemical parameters, the concentrations of ammonia nitrogen and nitrite were significantly (p< 0.05) reduced with the addition of probiotics in the culture water. The ammonia removal rates were 71.61%, 84.11%, and 85.94% compared to the WT group (ammonia removal rate = final concentration in the treatment group/final concentration in the WT). The phosphate concentration also tended to decrease, but was not significant. In contrast, the nitrate concentration increased with treatment, and a significant difference was shown between the WT and WH groups (p< 0.05).
Figure 1 Combined line graph of the water quality parameters within 22 days of the experiment detected at 3-day intervals.
Data on the growth performance of grouper with and without probiotic treatment are shown in Table 2. There was no significant difference in the SR data; only one fish in the WM group died during the experiment. The FW, WGR, and SGR increased with increasing concentrations of the added probiotics and reached maximum values in the WH group (p< 0.05). These results suggest that groupers had good growth performance under the probiotic-treated conditions. There was also an increasing trend in the HSI, indicating that the grouper which received probiotic treatment had better capacity for fatty metabolism.
Table 2 Growth performance parameters of hybrid grouper cultured in seawater with added probiotics and in the control seawater.
The bacterial community was assessed with high-throughput sequencing to determine the influence of probiotics on the water microbiota. After data pre-processing and removing low-quality reads, 1,278, and 266 of the total effective tags were obtained. A total of 13,469 operational taxonomic units (OTUs) were also observed, and the sample values ranged from 884 to 1,391 OTUs (n = 12).
The Shannon and Simpson indexes were used to estimate the bacterial species richness and evenness in the water column. All of the treatment groups showed higher values compared to the control group, but were not significant (p > 0.05). The values Hof Chao1 and ACE showed no significant differences among groups. The Good’s coverage index for all samples was 99.7%, meaning that nearly all bacterial species were obtained and suitable for further microbiota analysis (Table 3).
Table 3 Comparisons of the alpha diversity indexes between the control and the three probiotic-treated groups.
The species profiling histogram showed the taxonomic species richness of the bacterial species in the culture water in both the control and probiotic-treated groups. At the phylum level, the dominant phyla in the WT, WL, WM, and WH groups were Bacteroidetes (35.54%, 21.78%, 36.32%, and 35.70%), Proteobacteria (18.20%, 35.81%, 34.19%, and 30.08%), Cyanobacteria (8.58%, 16.15%, 4.15%, and 10.07%), Actinobacteria (13.73%, 9.16%, 4.18%, and 6.45%), Firmicutes (3.66%, 7.06%, 9.90%, and 4.69%), Verrucomicrobia (10.70%, 2.62%, 5.38%, and 4.28%), and Fusobacteria (5.10%, 0.28%, 0.37%, and 0.09%) (Figure 2A). Notably, the Alphaproteobacteria affiliated with Proteobacteria had the highest proportion of abundance, accounting for 10.69%, 20.23%, 25.06%, and 21.34% in the WT, WL, WM, and WH groups, respectively. In addition, the abundance of Planctomycetes (0.84%, 2.81%, 1.80%, and 5.83%) was significantly higher (p< 0.05) in the treatment groups compared with that in the control group.
Figure 2 (A) Stacked bar graph representing the relative abundance of each bacterial taxon (top 10 taxa) within each sample at the phylum level. (B) Stacked bar graph representing the relative abundance of each bacterial taxon (top 20 taxa) within each sample at the family level.
At the family level (for the WT, WL, WM, and WH groups respectively), Flavobacteriaceae (22.06%, 11.68%, 23.58%, and 19.63%), Rhodobacteraceae (7.79%, 9.60%, 17.83%, and 10.71%), Microbacteriaceae (11.52%, 5.75%, 2.38%, and 5.01%), Rubritaleaceae (10.62%, 2.07%, 5.24%, and 3.97%), Cryomorphaceae (3.54%, 3.35%, 7.64%, and 4.19%), and Peptostreptococcaceae (2.47%, 3.30%, 7.45%, and 2.92%) were dominant (Figure 2B). Members from the family Rhodobacteraceae were more abundant within the water columns of the probiotic-treated groups than that of the control group. Intriguingly, in the control group, the Fusobacteriaceae (phylum Fusobacteria) showed significantly increased abundance compared to the probiotic-treated groups (5.10%, 0.28%, 0.37%, and 0.09% for the WT, WL, WM, and WH groups, respectively; p< 0.05). In contrast, the reverse was found for Family_XII (phylum Firmicutes) (0.05%, 2.00%, 0.74%, and 0.45% for the WT, WL, WM, and WH groups, respectively; p< 0.05) (Table 4).
Table 4 Differences in the relative abundance of rearing water microbiota among four different groups.
To further compare the differences in the community structure of the culture water microbiota between groups, principal coordinate analysis (PCoA) based on weighted UniFrac (unique fraction metric) distance was performed. As shown in Figure 3A, the x- and y-axis of the PCoA plot represented 47.25% of the contribution. Analysis of similarities (ANOSIM) with weighted UniFrac distance revealed differences in the control and treatment groups (R = 0.361, p = 0.004). It can be identified that the bacterial communities of the control group had a higher inter-group distance than those of the probiotic-treated groups. As the probiotic-treated groups only showed differences in the concentration of the additive, it was reasonable to assume that the three sample clusters were very close to each other. According to the above results, probiotics as water additives could change the bacterial community structure of the grouper culture water to a certain extent.
Figure 3 (A) Principal coordinate analysis (PCoA) plot based on weighted UniFrac distances. (B) Heatmap of the predicted microbial function at level 2 using PICRUSt2. (C) Box plot of the water microbiota predicted to function at level 2 (one-way ANOVA followed by Turkey’s honestly significant difference (HSD) post-hoc analysis). Boxes with different superscript letters are significantly different (p< 0.05). (D) Box plot of the water microbiota predicted to function at level 3 (as above).
The function of the water microbiota in the grouper culture has not been thoroughly investigated. The function prediction was first applied to evaluate the functional change in the grouper culture water with the addition of probiotics. As shown in Supplementary Table S1, in Kyoto Encyclopedia of Genes and Genomes (KEGG) level 1, metabolism and genetic information processing are the most active pathways of the water microbiota. In the metabolism category (level 2), “amino acid metabolism,” “carbohydrate metabolism,” “metabolism of terpenoids and polyketides,” and “metabolism of cofactors and vitamins” were predicted to be the most enriched pathways (Figure 3B). The results of the one-way ANOVA with Tukey’s honestly significant difference (HSD) test showed that “metabolism of other amino acids” and “fatty acid biosynthesis” were significantly increased in the treated groups (p< 0.05) (Figures 3C, D).
Environmental factors showed a close association with the community structure of the water microbiota. The dimensionality of the relationship between the community structures and environmental factors was reduced and fitted using the redundancy analysis (RDA) and Envir test. The clear separation of the control group and the probiotic-treated groups was shown in the RDA biplot (Figure 4A). Samples in the WT group were positioned on the top left of the origin of the coordinate axis, while those of the WL, WM, and WH group were all nearly on the bottom right of the coordinate axis. The concentrations of ammonia and nitrite were significant environmental factors that were negatively correlated with the x-axis and positively correlated with the y-axis. Additionally, the envfit analysis verified that the ammonia concentration explained 65.4% of the variation (r2 = 0.6547, p = 0.011), while the nitrite concentration explained 57.2% of the variation (r2 = 0.5729, p = 0.03). Furthermore, the indicator analysis results (Figure 4B) showed that Cetobacterium had extremely significant correlations (p< 0.001) with the values of pH, NH3, and NO2.
Figure 4 (A) Redundancy analysis (RDA) was used to produce a two-dimensional sorting map relating the culture water microbiota to the water quality indicators. (B) Pearson’s correlation heatmap analysis at the genus level. *p< 0.05; **p< 0.01; ***p< 0.001.
The pond water provides survival conditions for aquatic animals and the decomposition of organic matter. However, an artificial aquatic environment has always displayed weak self-purification ability. In aquaculture practice, the water quality parameters reflect the health status of aquatic animals and provide a reference for subsequent practice. High concentrations of unionized ammonia and nitrite have been considered as the leading cause of sudden death of aquatic fishes (Eddy, 2005). The capacity of probiotics as water additives to improve the quality of aquaculture water has been demonstrated, such as in the culture process of Nile tilapia (Kord et al., 2022), Colossoma macropomum (Costa et al., 2021), and Cyprinus carpio L. (Abiri et al., 2022).
Regarding the bioremediation capacity of probiotics in the water quality of grouper culture, our study found that the concentrations of ammonia and nitrite in the probiotic-treated groups significantly decreased compared to those in the control group (p< 0.05). This result is consistent with the finding of Zink et al. (2011), who reported that the commercial probiotic product EcoAqua contained a variety of Bacillus, which reduced the concentration of unionized ammonia. In addition, the concentration of nitrate, a final ammonia oxidation product, increased significantly in the WH group compared with the WT group (p< 0.05). It is believed that the changes in the series of nitrogenous compounds were caused by the addition of Bacillus sp. and the uptake of toxic nitrogenous compounds through the rearing water (Zokaeifar et al., 2014; Gao et al., 2018). Several studies have suggested that Lactobacillus lacks the ability to take up nitrogenous substances from the water environment (Talpur et al., 2013; Dash et al., 2016; Flores-Valenzuela et al., 2021). However, the pH decline phenomenon was detected in this study (p< 0.05), as in the majority of Lactobacillus water quality regulation studies (Ma et al., 2009; Valdes et al., 2013; Dash et al., 2016). The organic acid generated by Lactobacillus is responsible for the considerable reduction in pH across all probiotic-treated groups as the amount of probiotics added in each treatment group increases. It is known that the pH and the temperature of rearing water are closely associated with ammonia toxicity (Emerson et al., 1975). Hence, the low pH resulting from Lactobacillus also has an undisputed benefit in reducing the ammonia stress on culture aquatic species.
In the present study, significant changes in the growth performance of grouper were observed in the probiotic-treated groups (p< 0.05). A consensus has been reached among many prior researchers that feeds supplemented with Bacillus and Lactobacillus could promote the growth performance of various fish species (Wang, 2011; Doan et al., 2018; Silva et al., 2021). Yan et al. (2016) reported that the dietary application of Bacillus pumilus SE5 also significantly improved the FW, WG, and SGR at day 60. Similarly, an increasing trend of these growth indexes was also observed in the present study. There are numerous avenues for probiotics to influence host growth and health beneficially, such as the secretion of digestive enzymes, modulation of the immune system positively, and the contribution of nutrients. However, in this study, the same beneficial functions may result from the swallowing of probiotics down to the grouper’s gastrointestinal tract with feeding and osmolality-regulated behavior. We believe that this case is probably related to the contribution of water quality improvement. The acute toxicity and sublethal effects of high concentrations of ammonia and nitrite could end the fish-feeding behavior (Rodrigues et al., 2007). This study observed the same phenomenon in the WT group: only a few of the grouper swam to the top layer of water, and the food-snatching behavior was reduced at the late stages of the experiment.
A review of the literature discovered a lack of studies focused on the relationship between the community structure of the rearing water microbiota of carnivorous fish and probiotic addition. The present study showed no significant differences in the Chao1 and ACE indexes after probiotic treatment (p > 0.05). The Chao1 and ACE values in the WM and WH groups decreased compared to those in the WT group. On the contrary, the Chao1 and ACE indexes in the WL group increased. We presumed that the fluctuation of the bacterial richness might be related to the decrease in pH. Zhang et al. (2022) reported that the Chao1 index of ruminal microbiota was significantly higher at pH 6.6 than that at pH 6.0. A number of studies have also found that the richness of the soil bacterial community is positively correlated with the soil pH (Huang et al., 2016a; Yan et al., 2021). However, there is a lack of relevant literature on the relationship between pH and the bacterial richness of pond culture water, which needs further research. Although the HSD test results were not significant (p > 0.05), the Shannon and Simpson indexes were slightly increased in the treatment groups, indicating that the addition of probiotics increased the evenness of the rearing water microbiota.
The stacked bar graph of the microbial community in this study displayed the dominant species in the grouper rearing seawater: Bacteroidetes, Proteobacteria, Cyanobacteria, Actinobacteria, and Firmicutes. This result is in accordance with other studies indicating that these bacterial phyla occupy the dominant ecological niches within seawater ecosystems (Sun et al., 2015; Zhang et al., 2021). This study showed significant differences in the phyla Planctomycetes and Fusobacteria. After the probiotic addition, all 16S water samples also showed that the abundance of Proteobacteria in the treated groups was higher than that in the control group, although not significant.
Bacteria in the phylum Planctomycetes are considered of the anaerobic ammonium oxidation (ANAMMOX) type, attracting great concern from researchers (Lodha et al., 2021). These bacteria are detectable in the nitrification biofilter of marine recirculating aquaculture systems (Wang et al., 2013; Lage and Bondoso, 2014; van Teeseling et al., 2015; Huang et al., 2016b), biofloc shrimp ponds (Addo et al., 2021), and rearing water of Lateolabrax maculatus culture (Duan et al., 2021). The current study showed that the relative abundance of clades within Planctomycetes was increased in the water samples of biofloc shrimp ponds with the addition of probiotics, which also contained Bacillus sp. and Lactobacillus sp. (Huerta-Rabago et al., 2019). Our study combined the water quality data with the abundance of Planctomycetes (including the genera Gimesia, Planctomicrobium, Rhodopirellula, Blastopirellula, and SM1A02) in the probiotic treatment. We boldly speculate that Planctomicrobium could absorb the organic acid produced by Lactobacillus for quick multiplication. Moreover, ammonia and nitrite were subsequently utilized as substrates for the ANAMMOX process (Smits et al., 2009; Li et al., 2014; Chen et al., 2020). However, the high dissolved oxygen level became the limiting factor in avoiding uncontrolled bacterial production. The functions of Lactobacillus and Bacillus in the grouper culture practice remain not thoroughly studied and fully understood.
It is worth noting that Cetobacterium (phylum Fusobacteria) was identified as indicator species in the indicator analysis, which was only significantly enriched in the control group. Cetobacterium spp. are Gram-negative anaerobes recognized to benefit the intestinal health of aquatic fishes (Tsuchiya et al., 2008). Numerous studies have shown that Cetobacterium primarily colonized the digestive system of carnivorous fish species such as Lepomis macrochirus, Micropterus salmoides, Culter alburnus, and Siniperca chuatsi (Larsen et al., 2014; Liu et al., 2016). Finegold et al. (2003) and Meng et al. (2021) reported that Cetobacterium could contribute to protein digestion and vitamin B12 production. The reduction of Cetobacterium with the probiotic addition might be related to the concentrations of ammonia and nitrite. Another school of thought suggests that lower relative abundances of Cetobacterium correlate with a higher growth performance of fish, e.g., in grouper, suggesting that Cetobacterium can have a detrimental effect in some circumstances (Standen et al., 2015; Li et al., 2019; Liu et al., 2021). Cetobacterium also colonized the outer layer of the recirculating water system biofilm at 20 and 59 days. Ammonia and nitrite showed an increasing trend, and the pH decreased to 6.1 at 59 days (Itoi et al., 2007). These agree with the results of our indicator analysis, in which Cetobacterium showed an extremely significant positive correlation with ammonia, nitrite, and pH (p< 0.001). We speculate that the Cetobacterium excreted in feces can occupy certain niches in the rearing water environment. However, with the emergence of the low nitrogen source (NH3 and ) environment, it will always fail in the competition for resources. Studies on the microbial function of Cetobacterium concerning the water environment are lacking; future studies are needed to confirm this result and elucidate the underlying mechanism.
The function prediction of the culture water microbiota was performed, and Tukey’s HSD test was used to calculate the significance in each group. As observed, at the level 2 KEGG pathway, the metabolism of other amino acids in the probiotic-treated groups was enhanced. Furthermore, at level 3, the gene abundance related to fatty acid biosynthesis was enriched in the treated groups. Such an increase in the microorganism metabolism of macronutrients is reminiscent of our findings on the water quality and the community structures of the water microbiota. Proteobacteria was believed to be a crucial denitrifier widely distributed in various environments (Baek et al., 2003; Park et al., 2006; Shao et al., 2011). A possible explanation for this series of changes might be that the nitrate produced by the nitration reactions of Bacillus and native nitrifying bacteria provided an incentive for increasing Proteobacteria. At the same time, the organic matter (feed residues and fish feces) contained in eutrophicated water was taken advantage of by Proteobacteria to produce the fatty acid and amino acid for the generation of the bacterial membrane and functional elements. Additionally, the genus Hyphomonas was dominant over the other species in Alphaproteobacteria, which, as Cho et al. (2019) reported, may have the ability to diffuse volatile indoles to promote the algae biomass of Chlorella. Therefore, this application has reasonable prospect on the combination with microalgae to manipulate the ecosystem function of the aquaculture pond all-to-all.
Based on the results of this study, it can be concluded that water supplementation with a probiotic combination (Bacillus sp. and Lactobacillus sp.) significantly decreased the toxic ammonia and nitrite of the grouper culture water. Furthermore, the growth performances of the hybrid grouper, including FW, SGR, and WGR, were enhanced, with the addition of probiotics providing a favorable culture environment to survive and grow. In addition, the probiotic mixture positively changed the community structure of the culture water microbiota and elicited a significant reinforcement effect on the nutritional metabolism of the water microbiota.
The datasets presented in this study can be found in online repositories. The names of the repository/repositories and accession number(s) can be found below: BioProject, PRJNA893097.
The animal protocols for this study were reviewed and approved by The Guangdong Ocean University Research Council (approval no. GDOU-LAE-2021-021).
JH: Methodology, visualization, software, formal analysis, data curation, investigation, writing—original draft, and methodology, project administration. EA: Writing—review and editing, investigation, data curation, and formal analysis. GO: Sampling, data curation, and formal analysis. YL and XJ: Investigation, data curation, and formal analysis. ZW: Methodology, sampling, and visualization. GC: Conceptualization, methodology, software, validation, formal analysis, investigation, supervision, resources, funding acquisition, and writing—review and editing. All authors contributed to the article and approved the submitted version.
This work was supported by a grant from the National Key R&D Program of China (no. 2020YFD0900200).
We thank Professor Chen Gang and all the Fish Seed Engineering and Breeding Laboratory teachers he led for their guidance and valuable opinion. We are also grateful to Guangdong Evergreen Feed Industry Co., Ltd. for providing the experimental site and the Guangdong Ocean University and Shanghai Ocean University colleagues for their assistance in setting up the experimental equipment and sampling. Technical support from AMS Alliance (China) with the Smartchem 200 to alleviate the workload on water quality detection is gratefully acknowledged.
The authors declare that the research was conducted in the absence of any commercial or financial relationships that could be construed as a potential conflict of interest.
All claims expressed in this article are solely those of the authors and do not necessarily represent those of their affiliated organizations, or those of the publisher, the editors and the reviewers. Any product that may be evaluated in this article, or claim that may be made by its manufacturer, is not guaranteed or endorsed by the publisher.
The Supplementary Material for this article can be found online at: https://www.frontiersin.org/articles/10.3389/fmars.2022.1068997/full#supplementary-material
Abiri S. A., Chitsaz H., Najdegerami E. H., Akrami R., Jalali A. S. (2022). Influence of wheat and rice bran fermentation on water quality, growth performance, and health status of common carp (Cyprinus carpio l.) juveniles in a biofloc-based system. Aquaculture 555, 738168. doi: 10.1016/j.aquaculture.2022.738168
Addo F. G., Zhang S. H., Manirakiza B., Ohore O. E., Shudong Y. (2021). The impacts of straw substrate on biofloc formation, bacterial community and nutrient removal in shrimp ponds. Bioresour. Technol. 326, 124727. doi: 10.1016/j.biortech.2021.124727
Amenyogbe E., Chen G., Wang Z. L., Huang J. S., Huang B. S., Li H. J. (2020). The exploitation of probiotics, prebiotics and synbiotics in aquaculture: present study, limitations and future directions. : a review. Aquacult. Int. 28 (3), 1017–1041. doi: 10.1007/s10499-020-00509-0
Amenyogbe E., Luo J., Fu W.-J., Abarike E. D., Wang Z.-L., Huang J.-S., et al. (2022a). Effects of autochthonous strains mixture on gut microbiota and metabolic profile in cobia (Rachycentron canadum). Sci. Rep. 12 (1), 17410. doi: 10.1038/s41598-022-19663-x
Amenyogbe E., Yang E. J., Xie R. T., Huang J. S., Chen G. (2022b). Influences of indigenous isolates Pantoea agglomerans RCS2 on growth, proximate analysis, haematological parameters, digestive enzyme activities, serum biochemical parameters, antioxidants activities, intestinal morphology, disease resistance, and molecular immune response in juvenile's cobia fish (Rachycentron canadum). Aquaculture 551, 737942. doi: 10.1016/j.aquaculture.2022.737942
Amenyogbe E., Zhang J. D., Huang J. S., Chen G. (2022c). The efficiency of indigenous isolates Bacillus sp. RCS1 and Bacillus cereus RCS3 on growth performance, blood biochemical indices and resistance against Vibrio harveyi in cobia fish (Rachycentron canadum) juveniles. Aquacult. Rep. 25, 101241. doi: 10.1016/j.aqrep.2022.101241
Askarian F., Zhou Z. G., Olsen R. E., Sperstad S., Ringo E. (2012). Culturable autochthonous gut bacteria in Atlantic salmon (Salmo salar l.) fed diets with or without chitin. characterization by 16S rRNA gene sequencing, ability to produce enzymes and in vitro growth inhibition of four fish pathogens. Aquaculture 326, 1–8. doi: 10.1016/j.aquaculture.2011.10.016
Baek S. H., Kim K. H., Yin C. R., Jeon C. O., Im W. T., Kim K. K., et al. (2003). Isolation and characterization of bacteria capable of degrading phenol and reducing nitrate under low-oxygen conditions. Curr. Microbiol. 47 (6), 462–466. doi: 10.1007/s00284-002-4058-9
Chen C. J., Wang Y. Q., Jiang Y., Guo M. L., Cui M. H., Zhang T. C. (2020). Effects of organic-Matter-Induced short-term stresses on performance and population dynamics of anammox systems. J. Environ. Eng. 146 (10), 04020120. doi: 10.1061/(Asce)Ee.1943-7870.0001789
Chizhayeva A., Amangeldi A., Oleinikova Y., Alybaeva A., Sadanov A. (2022). Lactic acid bacteria as probiotics in sustainable development of aquaculture. Aquat. Living. Resour. 35, 10. doi: 10.1051/alr/2022011
Cho K., Heo J., Cho D. H., Tran Q. G., Yun J. H., Lee S. M., et al. (2019). Enhancing algal biomass and lipid production by phycospheric bacterial volatiles and possible growth enhancing factor. Algal. Research-Biomass. Biofuels Bioproducts. 37, 186–194. doi: 10.1016/j.algal.2018.11.011
Costa L. F. A., Lima J. S., Siqueira A. M. A., da Silva E. C. C., Lima V. C. F., da Silva L. A., et al. (2021). Effect of multi-species probiotic administration in Colossoma macropomum juvenile rearing: supplementation and bioremediation. Aquacult. Nutr. 27 (5), 1721–1729. doi: 10.1111/anu.13309
Dash G., Raman R. P., Prasad K. P., Marappan M., Pradeep M. A., Sen S. (2016). Evaluation of Lactobacillus plantarum as a water additive on host associated microflora, growth, feed efficiency and immune response of giant freshwater prawn, Macrobrachium rosenbergii (de man 1879). Aquacult. Res. 47 (3), 804–818. doi: 10.1111/are.12539
Dawood M. A. O., Koshio S., Abdel-Daim M. M., Van Doan H. (2019). Probiotic application for sustainable aquaculture. Rev. Aquacult. 11 (3), 907–924. doi: 10.1111/raq.12272
Doan H. V., Hoseinifar S. H., Khanongnuch C., Kanpiengjai A., Unban K., Kim V. V., et al. (2018). Host-associated probiotics boosted mucosal and serum immunity, disease resistance and growth performance of Nile tilapia (Oreochromis niloticus). Aquaculture 491, 94–100. doi: 10.1016/j.aquaculture.2018.03.019
Duan Y. F., Xiong D. L., Li Y., Ding X., Dong H. B. A., Wang W. H., et al. (2021). Changes in the microbial communities of the rearing water, sediment and gastrointestinal tract of Lateolabrax maculatus at two growth stages. Aquacult. Rep. 20, 100742. doi: 10.1016/j.aqrep.2021.100742
Eddy F. B. (2005). Ammonia in estuaries and effects on fish. J. Fish. Biol. 67 (6), 1495–1513. doi: 10.1111/j.1095-8649.2005.00930.x
El-Haroun E. R., Goda A. M. A. S., Chowdhury M. A. K. (2006). Effect of dietary probiotic Biogen((R)) supplementation as a growth promoter on growth performance and feed utilization of Nile tilapia Oreochromis niloticus (L.). Aquacult. Res. 37 (14), 1473–1480. doi: 10.1111/j.1365-2109.2006.01584.x
Emerson K., Russo R. C., Lund R. E., Thurston R. V. (1975). Aqueous ammonia equilibrium calculations: Effect of pH and temperature. J. Fish. Res. Board. Canada. 32 (12), 2379–2383. doi: 10.1139/f75-274
Finegold S. M., Vaisanen M. L., Molitoris D. R., Tomzynski T. J., Song Y., Liu C., et al. (2003). Cetobacterium somerae sp nov from human feces and emended description of the genus Cetobacterium. Syst. Appl. Microbiol. 26 (2), 177–181. doi: 10.1078/072320203322346010
Fisheries, M.o.A.a.R.A.o.t.P.s.R.o.C.N.F.T.E.C.o.C.C.S.o (2022). China Fishery statistical yearbook : 2022, China Agriculture Press: Beijing, China.
Flores-Valenzuela E., Miranda-Baeza A., Rivas-Vega M. E., Miranda-Arizmendi V., Beltran-Ramirez O., Emerenciano M. G. C. (2021). Water quality and productive response of Litopenaeus vannamei reared in biofloc with addition of commercial strains of nitrifying bacteria and Lactobacillus rhamnosus. Aquaculture 542, 736869. doi: 10.1016/j.aquaculture.2021.736869
Gao J. Q., Gao D., Liu H., Cai J. J., Zhang J. Q., Qi Z. L. (2018). Biopotentiality of high efficient aerobic denitrifier Bacillus megaterium S379 for intensive aquaculture water quality management. J. Environ. Manage. 222, 104–111. doi: 10.1016/j.jenvman.2018.05.073
Gephart J. A., Golden C. D., Asche F., Belton B., Brugere C., Froehlich H. E., et al. (2020). Scenarios for global aquaculture and its role in human nutrition. Rev. Fish. Sci. Aquacult. 29 (1), 122–138. doi: 10.1080/23308249.2020.1782342
Harikrishnan R., Balasundaram C., Heo M. S. (2010). Lactobacillus sakei BK19 enriched diet enhances the immunity status and disease resistance to streptococcosis infection in kelp grouper, Epinephelus bruneus. Fish. Shellfish. Immunol. 29 (6), 1037–1043. doi: 10.1016/j.fsi.2010.08.017
He R. P., Feng J., Tian X. L., Dong S. L., Wen B. (2017). Effects of dietary supplementation of probiotics on the growth, activities of digestive and non-specific immune enzymes in hybrid grouper (Epinephelus lanceolatus male x Epinephelus fuscoguttatus female). Aquacult. Res. 48 (12), 5782–5790. doi: 10.1111/are.13401
Huang Z. T., Wan R., Song X. F., Liu Y., Hallerman E., Dong D. P., et al. (2016b). Metagenomic analysis shows diverse, distinct bacterial communities in biofilters among different marine recirculating aquaculture systems. Aquacult. Int. 24 (5), 1393–1408. doi: 10.1007/s10499-016-9997-9
Huang R., Zhao D. Y., Zeng J., Shen F., Cao X. Y., Jiang C. L., et al. (2016a). pH affects bacterial community composition in soils across the huashan watershed, China. Can. J. Microbiol. 62 (9), 726–734. doi: 10.1139/cjm-2015-0783
Huerta-Rabago J. A., Martinez-Porchas M., Miranda-Baeza A., Nieves-Soto M., Rivas-Vega M. E., Martinez-Cordova L. R. (2019). Addition of commercial probiotic in a biofloc shrimp farm of Litopenaeus vannamei during the nursery phase: Effect on bacterial diversity using massive sequencing 16S rRNA. Aquaculture 502, 391–399. doi: 10.1016/j.aquaculture.2018.12.055
Itoi S., Ebihara N., Washio S., Sugita H. (2007). Nitrite-oxidizing bacteria, Nitrospira, distribution in the outer layer of the biofilm from filter materials of a recirculating water system for the goldfish Carassius auratus. Aquaculture 264 (1-4), 297–308. doi: 10.1016/j.aquaculture.2007.01.007
Kord M. I., Maulu S., Srour T. M., Omar E. A., Farag A. A., Nour A. A. M., et al. (2022). Impacts of water additives on water quality, production efficiency, intestinal morphology, gut microbiota, and immunological responses of Nile tilapia fingerlings under a zero-water-exchange system. Aquaculture 547, 737503. doi: 10.1016/j.aquaculture.2021.737503
Lage O. M., Bondoso J. (2014). Planctomycetes and rnacroalgae, a striking association. Front. Microbiol. 5. doi: 10.3389/fmicb.2014.00267
Larsen A. M., Mohammed H. H., Arias C. R. (2014). Characterization of the gut microbiota of three commercially valuable warmwater fish species. J. Appl. Microbiol. 116 (6), 1396–1404. doi: 10.1111/jam.12475
Li D., Alidina M., Drewes J. E. (2014). Role of primary substrate composition on microbial community structure and function and trace organic chemical attenuation in managed aquifer recharge systems. Appl. Microbiol. Biotechnol. 98 (12), 5747–5756. doi: 10.1007/s00253-014-5677-8
Li X. P., Li J. R., Wang Y. B., Fu L. L., Fu Y. Y., Li B. Q., et al. (2011). Aquaculture industry in China: Current state, challenges, and outlook. Rev. Fish. Sci. 19 (3), 187–200. doi: 10.1080/10641262.2011.573597
Liu H., Guo X. W., Gooneratne R., Lai R. F., Zeng C., Zhan F. B., et al. (2016). The gut microbiome and degradation enzyme activity of wild freshwater fishes influenced by their trophic levels. Sci. Rep. 6, 24340. doi: 10.1038/srep24340
Liu Z. Y., Yang H. L., Hu L. H., Yang W., Ai C. X., Sun Y. Z. (2021). Dose-dependent effects of histamine on growth, immunity and intestinal health in juvenile grouper (Epinephelus coioides). Front. Mar. Sci. 8. doi: 10.3389/fmars.2021.685720
Li H. Q., Zhou Y., Ling H. Y., Luo L., Qi D. S., Feng L. (2019). The effect of dietary supplementation with Clostridium butyricum on the growth performance, immunity, intestinal microbiota and disease resistance of tilapia (Oreochromis niloticus). PloS One 14 (12), e0223428. doi: 10.1371/journal.pone.0223428
Lodha T., Narvekar S., Karodi P. (2021). Classification of uncultivated anammox bacteria and Candidatus uabimicrobium into new classes and provisional nomenclature as Candidatus brocadiia classis nov. and Candidatus uabimicrobiia classis nov. of the phylum Planctomycetes and novel family Candidatus scalinduaceae fam. nov to accommodate the genus Candidatus scalindua. Syst. Appl. Microbiol. 44 (6), 126272. doi: 10.1016/j.syapm.2021.126272
Ma C. W., Cho Y. S., Oh K. H. (2009). Removal of pathogenic bacteria and nitrogens by Lactobacillus spp. JK-8 and JK-11. Aquaculture 287 (3-4), 266–270. doi: 10.1016/j.aquaculture.2008.10.061
Meng X. L., Wu S. K., Hu W. P., Zhu Z. X., Yang G. K., Zhang Y. M., et al. (2021). Clostridium butyricum improves immune responses and remodels the intestinal microbiota of common carp (Cyprinus carpio l.). Aquaculture 530, 735753. doi: 10.1016/j.aquaculture.2020.735753
Nayak S. K. (2010). Probiotics and immunity: A fish perspective. Fish. Shellfish. Immunol. 29 (1), 2–14. doi: 10.1016/j.fsi.2010.02.017
Ni J. J., Li X. J., Chen F., Wu H. H., Xu M. Y. (2018). Community structure and potential nitrogen metabolisms of subtropical aquaculture pond microbiota. Appl. Ecol. Environ. Res. 16 (6), 7687–7697. doi: 10.15666/aeer/1606_76877697
Park H. I., Kim J. S., Kim D. K., Choi Y. J., Pak D. (2006). Nitrate-reducing bacterial community in a biofilm-electrode reactor. Enzyme Microbial. Technol. 39 (3), 453–458. doi: 10.1016/j.enzmictec.2005.11.028
Person-Le Ruyet J., Labbe L., Le Bayon N., Severe A., Le Roux A., Le Delliou H., et al. (2008). Combined effects of water quality and stocking density on welfare and growth of rainbow trout (Oncorhynchus mykiss). Aquat. Living. Resour. 21 (2), 185–195. doi: 10.1051/alr:2008024
Pierre S., Gaillard S., Prevot-D'Alvise N., Aubert J., Rostaing-Capaillon O., Leung-Tack D., et al. (2008). Grouper aquaculture: Asian success and Mediterranean trials. Aquat. Conservation-Marine. Freshw. Ecosyst. 18 (3), 297–308. doi: 10.1002/aqc.840
Ray A. K., Roy T., Mondal S., Ringo E. (2010). Identification of gut-associated amylase, cellulase and protease-producing bacteria in three species of Indian major carps. Aquacult. Res. 41 (10), 1462–1469. doi: 10.1111/j.1365-2109.2009.02437.x
Reyes-Becerril M., Ascencio F., Gracia-Lopez V., Macias M. E., Roa M. C., Esteban M.Á. (2014). Single or combined effects of Lactobacillus sakei and inulin on growth, non-specific immunity and IgM expression in leopard grouper (Mycteroperca rosacea). Fish. Physiol. Biochem 40(4):1169–80. doi: 10.1007/s10695-014-9913-z
Rimmer M. A., Glamuzina B. (2019). A review of grouper (Family serranidae: Subfamily epinephelinae) aquaculture from a sustainability science perspective. Rev. Aquacult. 11 (1), 58–87. doi: 10.1111/raq.12226
Rodrigues R. V., Schwarz M. H., Delbos B. C., Sampaio L. S. A. (2007). Acute toxicity and sublethal effects of ammonia and nitrite for juvenile cobia Rachycentron canadum. Aquaculture 271 (1-4), 553–557. doi: 10.1016/j.aquaculture.2007.06.009
Shao M. F., Zhang T., Fang H. H. P., Li X. D. (2011). The effect of nitrate concentration on sulfide-driven autotrophic denitrification in marine sediment. Chemosphere 83 (1), 1–6. doi: 10.1016/j.chemosphere.2011.01.042
Shiu Y. L., Hsieh S. L., Guei W. C., Tsai Y. T., Chiu C. H., Liu C. H. (2015). Using Bacillus subtilis E20-fermented soybean meal as replacement for fish meal in the diet of orange-spotted grouper (Epinephelus coioides, Hamilton). Aquacult. Res. 46 (6), 1403–1416. doi: 10.1111/are.12294
Silva V. V., Salomao R. A. S., Mareco E. A., Dal Pai M., Santos V. (2021). Probiotic additive affects muscle growth of Nile tilapia (Oreochromis niloticus). Aquacult. Res. 52 (5), 2061–2069. doi: 10.1111/are.15057
Smits T. H. M., Huttmann A., Lerner D. N., Holliger C. (2009). Detection and quantification of bacteria involved in aerobic and anaerobic ammonium oxidation in an ammonium-contaminated aquifer. Bioremediation. J. 13 (1), 41–51. doi: 10.1080/10889860802690562
Soltani M., Ghosh K., Hoseinifar S. H., Kumar V., Lymbery A. J., Roye S., et al. (2019). Genus bacillus, promising probiotics in aquaculture: Aquatic animal origin, bio-active components, bioremediation and efficacy in fish and shellfish. Rev. Fish. Sci. Aquacult. 27 (3), 331–379. doi: 10.1080/23308249.2019.1597010
Standen B. T., Rodiles A., Peggs D. L., Davies S. J., Santos G. A., Merrifield D. L. (2015). Modulation of the intestinal microbiota and morphology of tilapia, oreochromis niloticus, following the application of a multi-species probiotic. Appl. Microbiol. Biotechnol. 99 (20), 8403–8417. doi: 10.1007/s00253-015-6702-2
Sun F. L., Wang Y. S., Wu M. L., Sun C. C., Cheng H. (2015). Spatial and vertical distribution of bacterial community in the northern south China Sea. Ecotoxicology 24 (7-8), 1478–1485. doi: 10.1007/s10646-015-1472-2
Talpur A. D., Ikhwanuddin M., Abdullah M. D. D., Bolong A. M. A. (2013). Indigenous Lactobacillus plantarum as probiotic for larviculture of blue swimming crab, Portunus pelagicus (Linnaeus 1758): Effects on survival, digestive enzyme activities and water quality. Aquaculture 416, 173–178. doi: 10.1016/j.aquaculture.2013.09.018
Theuerkauf S. J., Morris J. A., Waters T. J., Wickliffe L. C., Alleway H. K., Jones R. C. (2019). A global spatial analysis reveals where marine aquaculture can benefit nature and people. PloS One 14 (10), e0222282. doi: 10.1371/journal.pone.0222282
Tsuchiya C., Sakata T., Sugita H. (2008). Novel ecological niche of Cetobacterium somerae, an anaerobic bacterium in the intestinal tracts of freshwater fish. Lett. Appl. Microbiol. 46 (1), 43–48. doi: 10.1111/j.1472-765X.2007.02258.x
Valdes C. E. M., Macias E. B., Alvarez-Gonzalez C. A., Hernandez C. T., Sanchez A. J. (2013). Microorganisms effect with probiotic potential in water quality and growth of the shrimp Litopenaeus vannamei (Decapoda: Penaeidae) in intensive culture. Rev. Biol. Trop. 61 (3), 1215–1228.
van Teeseling M. C. F., Mesman R. J., Kuru E., Espaillat A., Cava F., Brun Y. V., et al. (2015). Anammox planctomycetes have a peptidoglycan cell wall. Nat. Commun. 6, 7878. doi: 10.1038/ncomms7878
Wang Y. (2011). Use of probiotics Bacillus coagulans, Rhodopseudomonas palustris and Lactobacillus acidophilus as growth promoters in grass carp (Ctenopharyngodon idella) fingerlings. Aquacult. Nutr. 17 (2), e372–e378. doi: 10.1111/j.1365-2095.2010.00771.x
Wang C., Xie B., Han L., Xu X. F. (2013). Study of anaerobic ammonium oxidation bacterial community in the aged refuse bioreactor with 16S rRNA gene library technique. Bioresour. Technol. 145, 65–70. doi: 10.1016/j.biortech.2013.01.170
Yan K., Dong Y. F., Gong Y. B., Zhu Q. L., Wang Y. P. (2021). Climatic and edaphic factors affecting soil bacterial community biodiversity in different forests of China. Catena 207, 105675. doi: 10.1016/j.catena.2021.105675
Yang H. L., Sun Y. Z., Hu X., Ye J. D., Lu K. L., Hu L. H., et al. (2019). Bacillus pumilus SE5 originated PG and LTA tuned the intestinal TLRs/MyD88 signaling and microbiota in grouper (Epinephelus coioides). Fish. Shellfish. Immunol. 88, 266–271. doi: 10.1016/j.fsi.2019.03.005
Yan Y. Y., Xia H. Q., Yang H. L., Hoseinifar S. H., Sun Y. Z. (2016). Effects of dietary live or heat-inactivated autochthonous Bacillus pumilus SE5 on growth performance, immune responses and immune gene expression in grouper Epinephelus coioides. Aquacult. Nutr. 22 (3), 698–707. doi: 10.1111/anu.12297
Yun L., Wang W., Li Y. Y., Xie M., Chen T., Hu C. Q., et al. (2021). Potential application values of a marine red yeast, Rhodosporidiums sphaerocarpum YLY01, in aquaculture and tail water treatment assessed by the removal of ammonia nitrogen, the inhibition to vibrio spp., and nutrient composition. PloS One 16 (2), e0246841. doi: 10.1371/journal.pone.0246841
Zhang M. M., Liang G. G., Zhang X. L., Lu X. T., Li S. Y., Wang X., et al. (2022). The gas production, ruminal fermentation parameters, and microbiota in response to Clostridium butyricum supplementation on in vitro varying with media pH levels. Front. Microbiol. 13. doi: 10.3389/fmicb.2022.960623
Zhang R., Liu W. C., Liu Y., Zhang H. L., Zhao Z. H., Zou L. Y., et al. (2021). Impacts of anthropogenic disturbances on microbial community of coastal waters in shenzhen, south China. Ecotoxicology 30 (8), 1652–1661. doi: 10.1007/s10646-020-02297-y
Zhang X. Y., Zhang Y. Q., Zhang Q., Liu P. W., Guo R., Jin S. Y., et al. (2020). Evaluation and analysis of water quality of marine aquaculture area. Int. J. Environ. Res. Public Health 17 (4), 1446. doi: 10.3390/ijerph17041446
Zhou S., Song D. L., Zhou X. F., Mao X. L., Zhou X. F., Wang S. L., et al. (2019). Characterization of Bacillus subtilis from gastrointestinal tract of hybrid hulong grouper (Epinephelus fuscoguttatus x E-lanceolatus) and its effects as probiotic additives. Fish. Shellfish. Immunol. 84, 1115–1124. doi: 10.1016/j.fsi.2018.10.058
Zink I. C., Benetti D. D., Douillet P. A., Margulies D., Scholey V. P. (2011). Improvement of water chemistry with Bacillus probiotics inclusion during simulated transport of yellowfin tuna yolk sac larvae. North Am. J. Aquacult. 73 (1), 42–48. doi: 10.1080/15222055.2011.544622
Zokaeifar H., Babaei N., Saad C. R., Kamarudin M. S., Sijam K., Balcazar J. L. (2014). Administration of Bacillus subtilis strains in the rearing water enhances the water quality, growth performance, immune response, and resistance against Vibrio harveyi infection in juvenile white shrimp, Litopenaeus vannamei. Fish. Shellfish. Immunol. 36 (1), 68–74. doi: 10.1016/j.fsi.2013.10.007
Keywords: Bacillus, Lactobacillus, hybrid grouper, water quality, water microbiota, growth performance
Citation: Huang J, Amenyogbe E, Ou G, Li Y, Wen Z, Jiang X and Chen G (2022) Effects of Bacillus sp. and Lactobacillus sp. combination as a water additive on the culture pond water and growth performance of hybrid grouper (Epinephelus fuscoguttatus × Epinephelus polyphekadion). Front. Mar. Sci. 9:1068997. doi: 10.3389/fmars.2022.1068997
Received: 13 October 2022; Accepted: 07 November 2022;
Published: 02 December 2022.
Edited by:
Yafei Duan, South China Sea Fisheries Research Institute, ChinaReviewed by:
Ziyan Liu, Jimei University, ChinaCopyright © 2022 Huang, Amenyogbe, Ou, Li, Wen, Jiang and Chen. This is an open-access article distributed under the terms of the Creative Commons Attribution License (CC BY). The use, distribution or reproduction in other forums is permitted, provided the original author(s) and the copyright owner(s) are credited and that the original publication in this journal is cited, in accordance with accepted academic practice. No use, distribution or reproduction is permitted which does not comply with these terms.
*Correspondence: Gang Chen, Y2hlbmdAZ2RvdS5lZHUuY24=
Disclaimer: All claims expressed in this article are solely those of the authors and do not necessarily represent those of their affiliated organizations, or those of the publisher, the editors and the reviewers. Any product that may be evaluated in this article or claim that may be made by its manufacturer is not guaranteed or endorsed by the publisher.
Research integrity at Frontiers
Learn more about the work of our research integrity team to safeguard the quality of each article we publish.