- 1Laboratorio de Necton y Ecología de Arrecifes, Centro de Investigaciones Biológicas del Noroeste (CIBNOR), La Paz, Baja California Sur, México
- 2Red Sea Research Center, Division of Biological and Environmental Science and Engineering (BESE), King Abdullah University of Science and Technology (KAUST), Thuwal, Saudi Arabia
- 3Laboratorio de Esclerocronología de Corales Arrecifales, Unidad Académica de Sistemas Arrecifales, Instituto de Ciencias del Mar y Limnología, Universidad Nacional Autónoma de México, Puerto Morelos, Q. Roo., México
- 4Tecnológico Nacional de México / IT Bahía de Banderas, Bahía de Banderas, Nayarit, México
- 5Laboratorio de Ecología Marina, Centro de Investigaciones Costeras, Centro Universitario de la Costa, Universidad de Guadalajara, Puerto Vallarta, Jalisco, México
- 6Department of Biological Sciences, Louisiana State University, Baton Rouge, LA, United States
- 7Instituto de Investigaciones Oceanológicas, Universidad Autónoma de Baja California, Ensenada, Baja California, México
- 8Departamento de Ecología Marina, Centro de Investigación Científica y de Educación Superior de Ensenada, Ensenada, México
- 9CONACyT-Centro de Investigaciones Biológicas del Noroeste (CIBNOR), La Paz, Baja California Sur, México
Differences in selective pressures and the energetic cost of gametes in gonochoristic corals should vary with the sex of the colony, which may lead to sexual dimorphism. Coral colonies are composed of subunits (corallites) that create a complex morphological architecture. If corallite features are distinct between sexes, then the degree of coordinated change among these subunits may also vary (phenotypic modularity). This study tested for sexual dimorphism in the corallites of the reef-building coral Porites lobata, a gonochoric broadcast spawner, and compared this with previously demonstrated sexual dimorphism in its congener P. panamensis, a gonochoric brooder. Corallite area in P. lobata was 17% larger for males than for females (p < 0.05). Phenotypic modularity analysis showed that the integration of skeletal traits differs between sexes in both P. lobata and in P. panamensis. In P. lobata, females showed a higher trait integration than males, while the opposite pattern was observed in P. panamensis. Our results demonstrate corallite traits differentiate between sexes and suggest that between-sex differences in the degree of corallite integration may vary with reproductive mode.
1 Introduction
Sexual dimorphism is widespread among animals but has been considered rare in colonial marine taxa (Levitan, 2010). Recent studies on scleractinian corals, however, have found sexual dimorphism in size (Kramarsky-Winter and Loya, 1998; Loya and Sakai, 2008), calcification rates (Cabral-Tena et al., 2013; Tortolero-Langarica et al., 2016; Tortolero-Langarica et al., 2017; Mozqueda-Torres et al., 2018; Cruz-Ortega et al., 2020), skeletal isotopic signals (Cabral-Tena et al., 2013), and corallite morphologies (González-Espinosa et al., 2018). From these studies (Table 1), corallite differences have been found for only one species (González-Espinosa et al., 2018), leading us to ask whether the dimorphism previously observed (primarily in growth) could be seen in corallite’s traits.
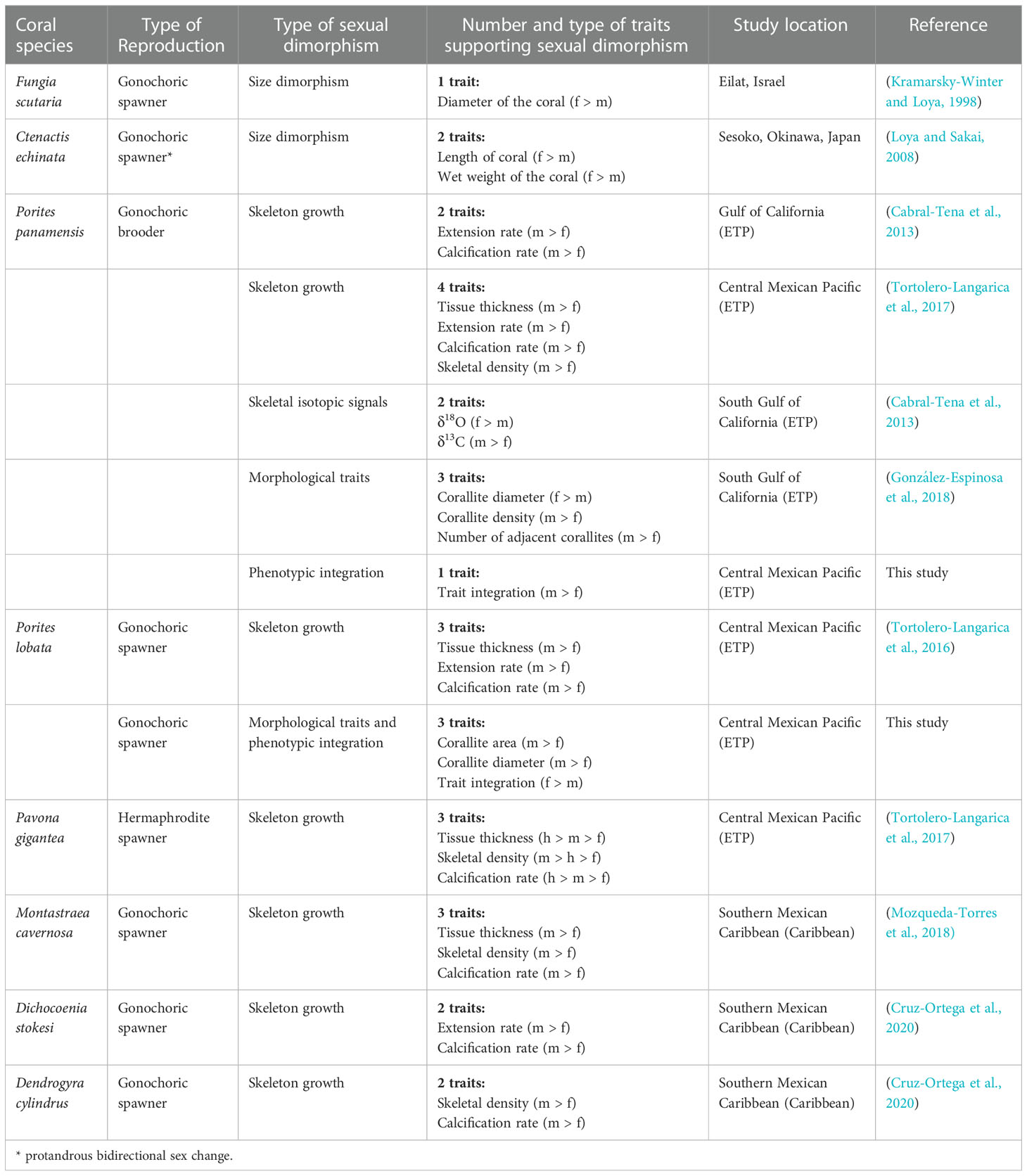
Table 1 Evidence of sexual dimorphism in coral reef-building species. Males (m), hermaphrodites (h), females (f), Eastern Tropical Pacific (ETP).
While inter-sexual differences may be more subtle in marine invertebrates (both clonal and unitary) than the conspicuous sexual dimorphism seen in many terrestrial animals (Berns, 2013), differences in mating strategies and the energetic cost of gametes (Parker, 1979) remain and should select for different trait optima between sexes (Parker, 1979; Levitan, 2008). In brooding gonochoristic corals, females both capture sperm for internal fertilization and, critically, brood larvae within their polyps, while males merely release sperm (Baird et al., 2009; Harrison, 2011). These differences may explain the divergent morphologies of their corallites (González-Espinosa et al., 2018). Meanwhile, in gonochoristic broadcast spawning corals, both sexes release their gametes and fertilization occurs externally (Baird et al., 2009; Harrison, 2011), with females freed of any larval care beyond provisioning eggs. This dissimilarity between reproductive mode could lead to different levels of sexual dimorphism in the corallites of reef-building species.
In modular plants, both morphology and integration of traits can differ between sexes (Barrett and Hough, 2013). As corals are modular organisms, sexual dimorphism could appear not only in the most fundamental unit of colony morphology (a single tentacled polyp and its underlying skeleton, the corallite) but also in the degree of integration among these units (Lasker et al., 2003; Shaish et al., 2007). The environment can induce morphological changes in colony architecture (phenotypic plasticity) (Todd, 2008) and in the integration between these traits (e.g., branch density and length, polyp aperture and distance between them), as seen in octocorals (Sánchez and Lasker, 2003; Sánchez et al., 2007) and scleractinian corals (Paz-García et al., 2015). Such integration of traits (modularity) has not been compared between sexes in corals.
Different reproductive modes carry different energetic costs among reef corals (Ward, 1995; Hall and Hughes, 1996), which in turn may lead to different levels of sexual dimorphism among reef-building species. In this study, we tested for sexual dimorphism in the corallite features and phenotypic modularity of two congeneric corals with separate sexes but different modes of reproduction: Porites lobata and P. panamensis. P. lobata is a broadcast spawner that shows sexual dimorphism in skeletal growth rates (Tortolero-Langarica et al., 2016; Table 1) but whose corallite morphology has not been previously tested for sexual dimorphism. Meanwhile, P. panamensis broods its larvae (Carpizo-Ituarte et al., 2011). Its corallites show sexual dimorphism in their diameter, density, and number of neighboring corallites (González-Espinosa et al., 2018) but the integration of these traits has not been analyzed before.
2 Materials and methods
2.1 Sample collection and sex identification
Porites lobata is one of the most abundant massive coral species in the eastern tropical Pacific (Glynn et al., 2017). We collected samples at Isla Isabel National Park in the central Mexican Pacific (Figure 1) in October 2013 (permission number: DGOPA.04552.040711.1798). All colonies (~70 cm in diameter size) were collected using a hammer and chisel from the same habitat at depths 3–5 m to minimize phenotypic differences caused by different light or flow microenvironments. We collected 18 colonies total: 12 males and 6 females. Species identification was based on visual taxonomical characteristics (Veron, 2000). P. lobata is a gonochoric broadcast spawner (Glynn et al., 1994; Neves, 2000) whose gametes mature throughout the year along the eastern Pacific (Glynn et al., 1994; Glynn et al., 2011). A small percentage of hermaphroditic colonies have been reported in Costa Rica (Glynn et al., 1994), although reproductive season and hermaphroditism have not been documented in Mexico.
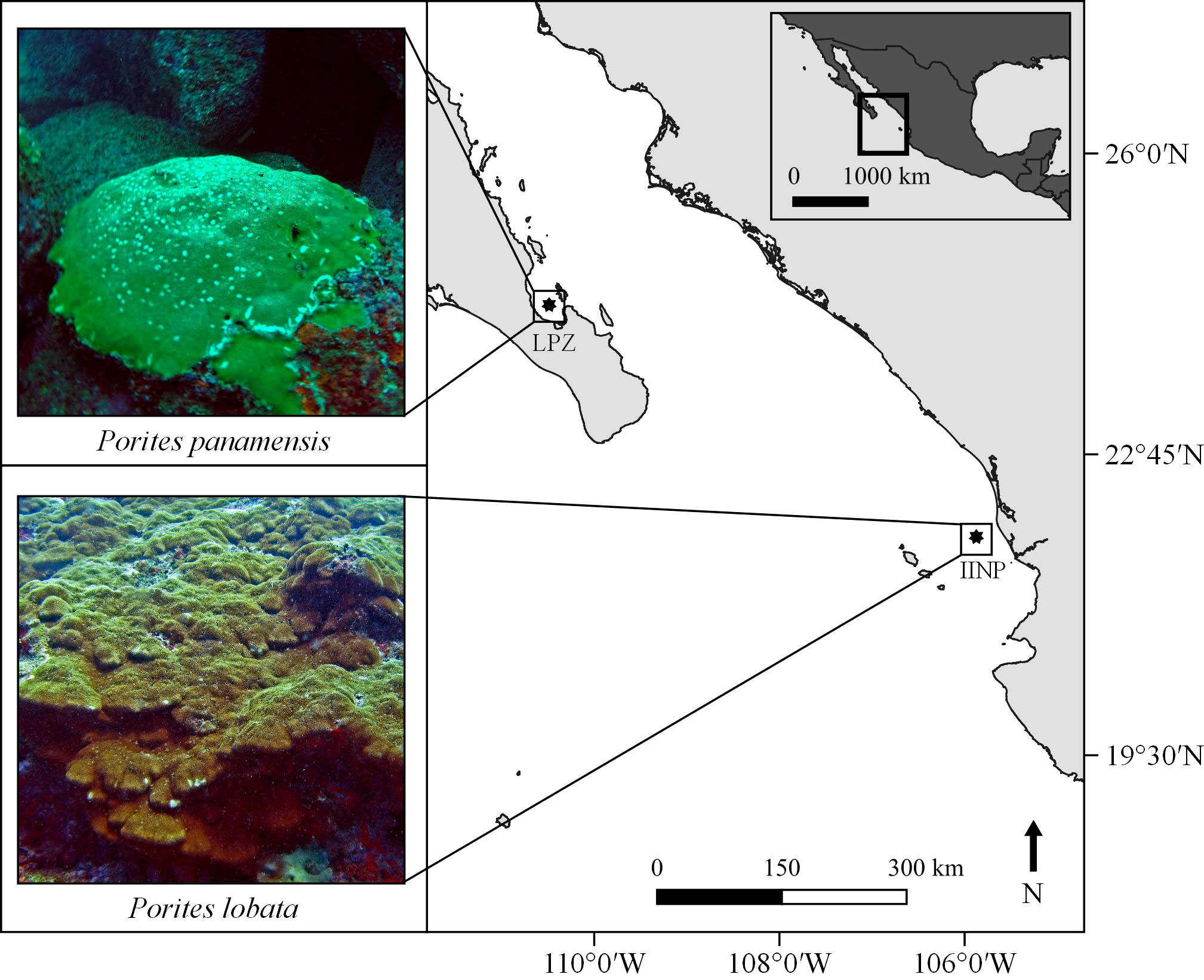
Figure 1 Study sites in the central Mexican Pacific. Black star denotes collection sites. Collection sites were Bahía de La Paz (LPZ) for P. panamensis and Isla Isabel National Park (IINP) for P. lobata. Colonies from P. panamensis were collected and described in previous study done by González-Espinosa et al (2018).
Colony sex was determined using the histological procedure of Cabral-Tena et al., 2013 and Tortolero-Langarica et al (2016). Briefly, two subsamples were used from each colony (previously collected in Tortolero-Langarica et al., 2016). One (~ 5 x 5 cm) was decalcified to obtain tissue, dehydrated, and stained using Masson’s trichrome protocol. Each colony was sexed by inspecting for the presence of oocytes or spermaries using a Zeiss AxioVision R1 microscope. The second fragment (~ 10 x 10 cm) was used for morphological analysis of the skeleton and its constituent corallites. Tissue was removed with 5% sodium hypochlorite to characterize their morphological traits.
2.2 Morphological traits and analysis of modularity
2.2.1 Morphological traits and comparison between sexes
Five morphological traits were selected for analysis (Table 2) based on their potential to delimit between Porites species (Brakel, 1977; Weil, 1992; Budd et al., 1994) and reveal sexual dimorphism in P. panamensis (González-Espinosa et al., 2018). A Nikon D5300 digital camera was used to capture images for corallite density (Figure 2A). Due to the small size of corallites of P. lobata (Figure 3C), high-resolution images were also obtained using a Hitachi S-3000N Scanning Electron Microscope (SEM). Those SEM images were used to measure morphological traits in 10 random corallites per colony (Figure 2B; Table 2). Previous studies done on extinct and extant Porites species have found an average from 5-10 corallites best represent the colony by tempering the variability among single corallites (Foster, 1986; Weil, 1992; López-Pérez, 2013; González-Espinosa et al., 2018; Tisthammer and Richmond, 2018). We selected only mature corallites and avoided the smallest corallites as they could be new corallites recently formed by extratentacular budding (Pichon, 2011) or broken corallites that would give imprecise measurements. Morphological traits were analyzed using ImageJ ver. 1.60 software (https://imagej.nih.gov/ij/ ) (Schindelin et al., 2015). Images were calibrated with a grid with standard dimensions. Statistical analyses were performed using colony means. After testing the data for normality (Kolmogorov–Smirnov test) and homogeneity of variances (Levene test), the Student’s t-test was used to determine trait differences between sexes in P. lobata.
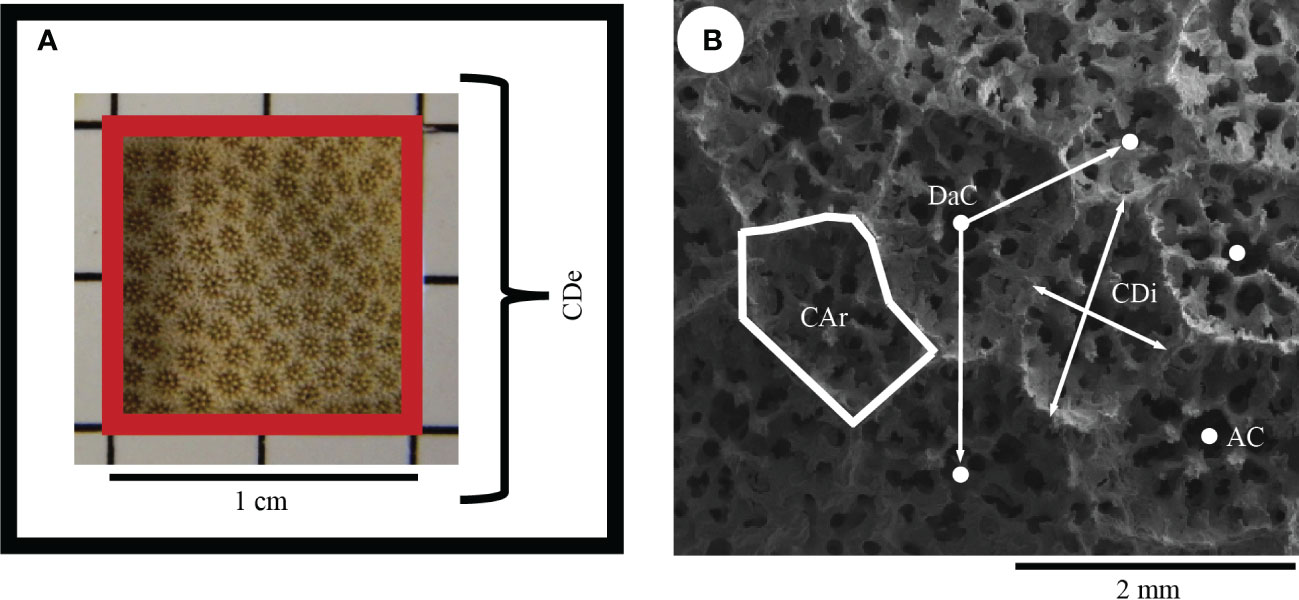
Figure 2 Morphological traits used to detect sexual dimorphism. (A) corallite density (CDe) (B) corallite diameter (CDi), number of adjacent corallites (AC), distance of adjacent corallites (DaC), and corallite area (CAr). Descriptions of each morphological trait are summarized in Table 2.
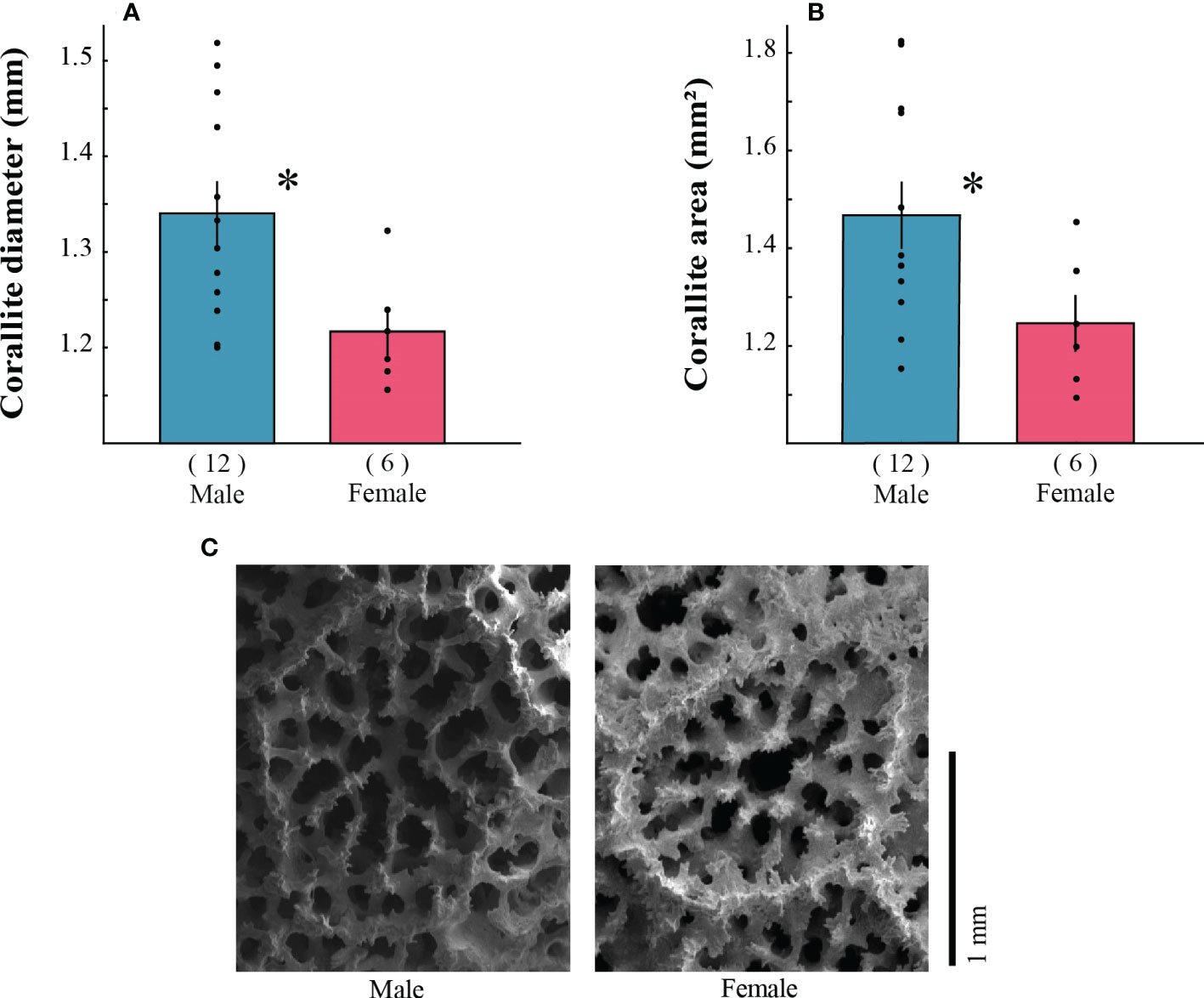
Figure 3 Sexual dimorphism in morphological and corallite traits of Porites lobata corals (A, B). Mean values (± standard error) of male (blue) and female (red). Black dots represent the data points per colony. Numbers in parenthesis indicate the number of colonies. Significance level: t-test, * p < 0.05. Visual representation of corallites of P. lobata (C).
We performed a canonical discriminant analysis (CDA) comparing sexual dimorphism observed in P. lobata (this study) with data for P. panamensis (https://doi.org/10.5061/dryad.5d6sp) from González-Espinosa et al (2018). We performed a random partition of 50% of the total colonies of P. panamensis from the dataset to perform analysis with a similar sample size in both Porites species. We used four out of five morphological traits (corallite density, corallite diameter, distance of adjacent corallites, and number of adjacent corallites; Table 2) to perform the CDA. Corallite area was not used because it was not measured by González-Espinosa et al. (2018) All statistical analyses were conducted with STATISTICA 7 software (www.statsoft.com).
2.2.2 Analysis of modularity
The four morphological traits used in the CDA (Table 2: traits 1-4) were also used for the modularity analysis. Integration between morphological traits was analyzed using conditional independence tests and independence graphs (Magwene, 2001). This method reveals the interaction between two traits after removing the effect of other traits and provides insight into whether traits are evolving in a coordinated manner or independently. Due to the number of traits measured in each species, second-order partial correlation coefficients (PCC) for both Porites species were computed from log-transformed data. The PCC was estimated for each set of two variables, controlling for the remaining variables (two). The PCC analyses were performed using STATISTICA 7.
Weak trait interactions were removed from the model by statistically comparing the PCCs for edge exclusion deviance (Magwene, 2001). The strength of the interactions was calculated by the edge strength (Magwene, 2001; Paz-García et al., 2015). For this model, we used four categories to classify the strength of the interactions based on the edge strengths results: weak integration (0-0.2), regular integration (0.21-0.4), strong integration (0.41-0.6), and very strong (>0.6) integration between traits. A graphic model was constructed for each species and sex (2 species × 2 sex), resulting in 4 different models in total. The resulting graphical model represents morphological traits in nodes and integration among traits by lines. The edge strength was represented in the graphical model as followed: weak integration (thin dotted line), regular integration (thick dotted line), strong integration (thin continuous line), and very strong integration (thick continuous line). The modularity level in each graphical model will depend on the strength (line thickness) and the number of trait connections (lines) for each model.
3 Results
3.1 Corallite traits
We analyzed 49 colonies for the CDA and modularity analysis: 18 from Porites lobata (12 males, 6 females) and 31 from Porites panamensis (12 males, 19 females). In P. lobata, two morphological traits were significantly larger in male colonies than female colonies (Figure 3; Supplementary Table S1). Male colonies had larger corallite areas (by 17%; p = 0.047) and diameters (by 10%; p = 0.024) than females (Figure 3; Supplementary Table S1). For P. panamensis, González-Espinosa et al. (2018) found the opposite: females had wider corallite diameters than males (p = 0.020), while males had higher corallite density (p = 0.016) and number of adjacent corallites (p = 0.005).
Sexual dimorphism in corallite size was statistically supported for both species in the CDA (Wilk’s λ= 0.11596, F(12,111) = 11.676, p 0.0001, Figure 4, Supplementary Table S2). For the CDA, the first axis explained 86.1% of the variance and the second axis explained an additional 10.4% between the species and sexes (Figure 4). The CDA resulted in a correct classification rate above 66%, with an average of 77% for males and females from both species (Supplementary Table S3).
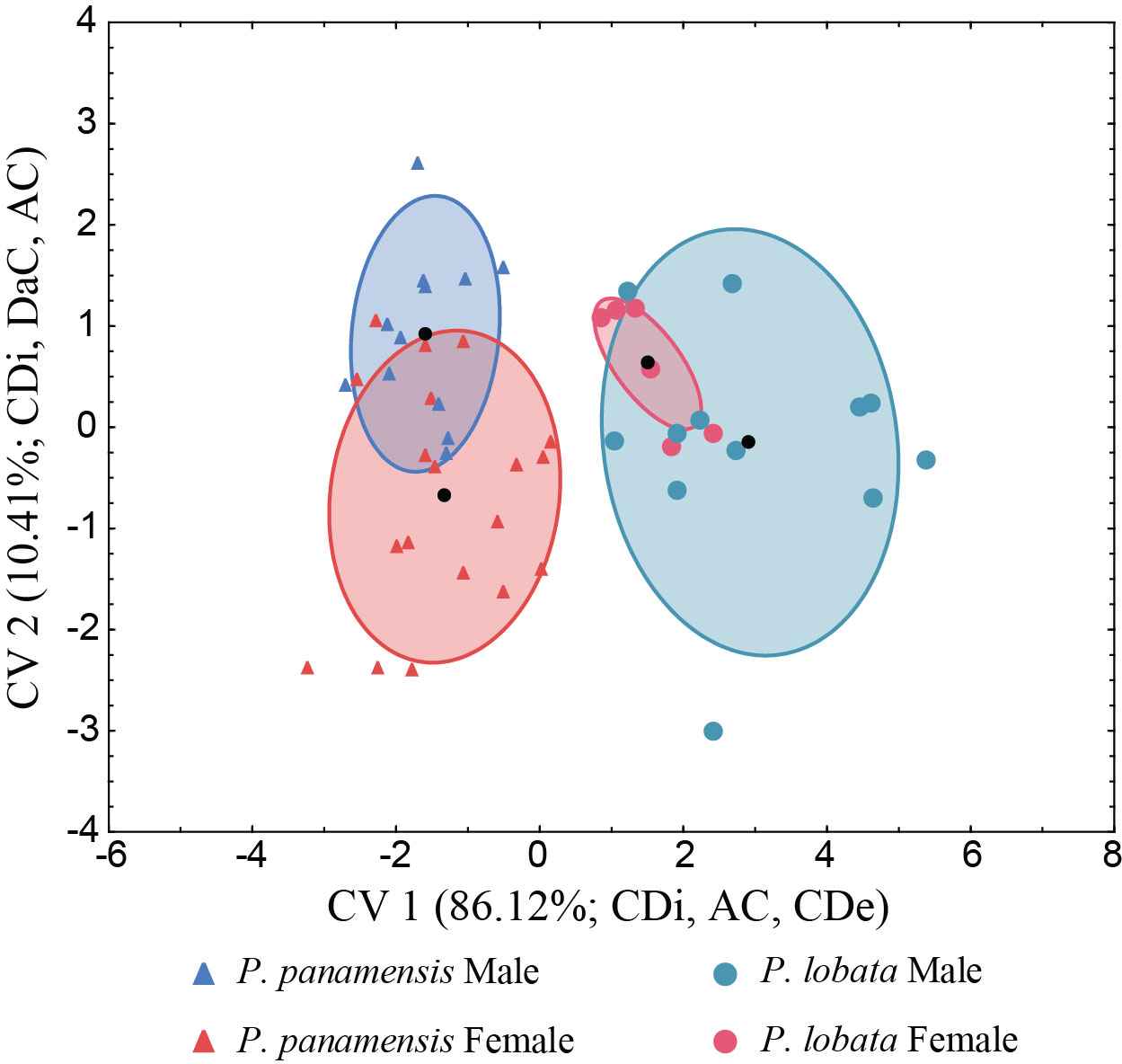
Figure 4 Canonical discriminant analysis between Porites lobata and Porites panamensis morphological traits. Each symbol represents one colony. P. panamensis: Male (N= 12), Female (N= 19), P. lobata: Male (N= 12), Female (N= 6). CDe, corallite density; CDi, corallite diameter; AC, adjacent corallites; and DaC, distance of adjacent corallites.
3.2 Modularity
Patterns of modularity differed between sexes for both species (Figure 5; Supplementary Tables S4, S5). The distance between adjacent corallites (DaC) showed the highest level of integration between traits in all four models (Figure 5), while corallite density (CDe) also showed higher coordination between traits in both species (Figure 5). Female colonies showed higher trait integration (i.e., higher strength and number of trait connections) than male colonies in P. lobata, while P. panamensis showed the opposite pattern.
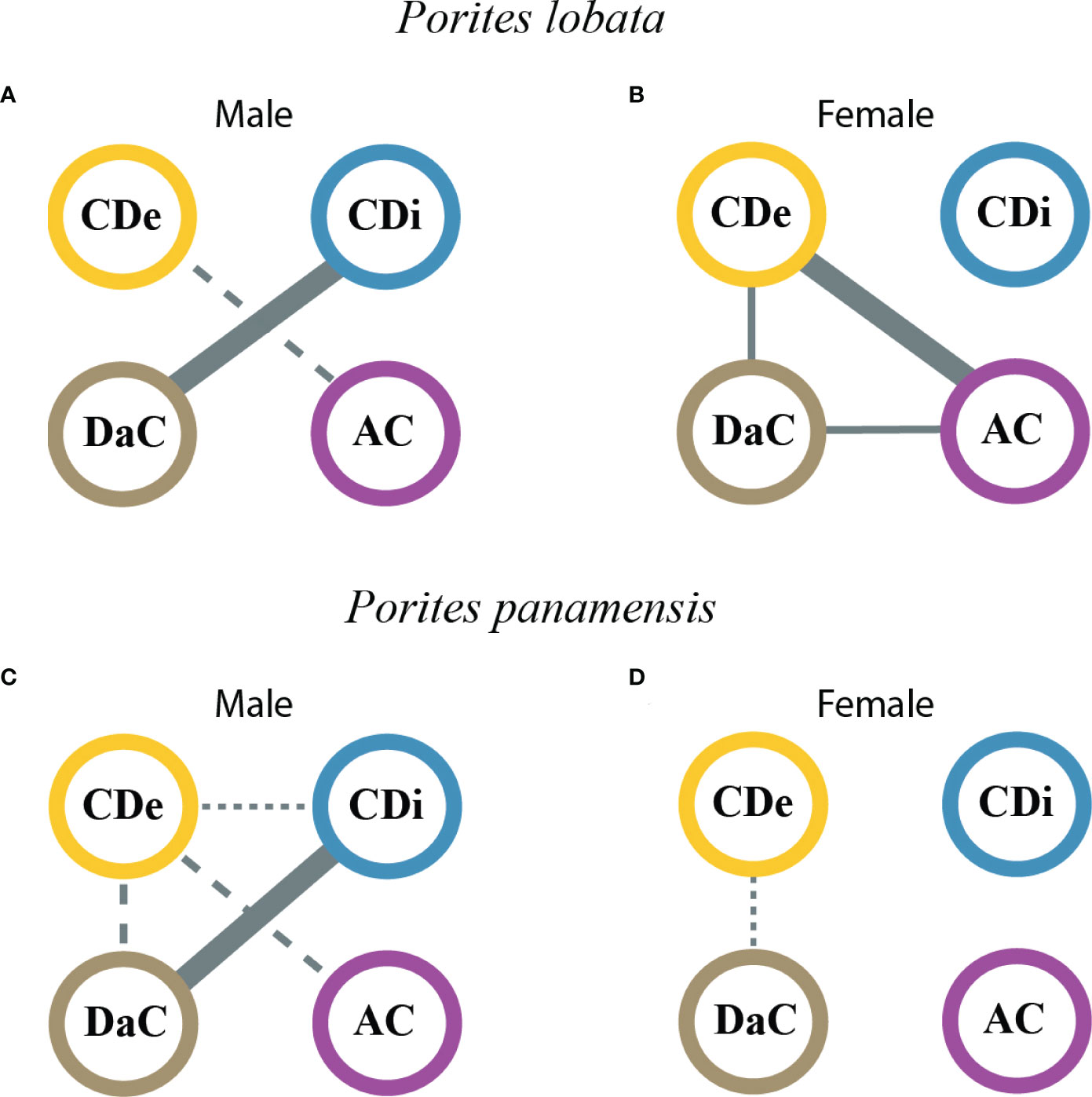
Figure 5 Modularity differences between sexes of Porites lobata (A, B) and of Porites panamensis (C, D) Graphical models of the level of modularity of male (A, C) and female (B, D) colonies, where modularity is indicated by the number of trait connections (lines) and their strength (thicker= stronger coordination).
For P. lobata, while the number of integrated traits was similar between sexes (two integrated traits for males, three for females), the connections between traits were different (Figures 5A, B; Supplementary Table S4). Corallite density was integrated with adjacent corallites (AC) in both sexes, but the other associations varied between the sexes. Corallite density, the distance of adjacent corallites, and the number of adjacent corallites were the most integrated traits in female colonies, showing two connections (Figure 5B). In contrast, male colonies showed a unique connection between corallite diameter (CDi) and the distance of adjacent corallites (Figure 5A).
While females of P. lobata showed slightly higher integration than males, the pattern was reversed in P. panamensis, where males showed a higher integration of traits (4 connections between traits; Figure 5C, Supplementary Table S5) than females (1 connection between traits; Figure 5D, Supplementary Table S5). Corallite density and distance between adjacent corallites were the most integrated traits in both sexes (Figures 5C, D).
4 Discussion
4.1 Sexual dimorphism in corals
The broadcast spawning coral Porites lobata is sexually dimorphic both in corallite morphology and at the level of correlated change among corallite traits (Figures 3–4B, Supplementary Tables S1, S4, S6). Sexual dimorphism in scleractinians has been reported for corallite size in solitary corals (Kramarsky-Winter and Loya, 1998; Loya and Sakai, 2008; see Table 1), for skeletal growth, and for calcification rates (see Table 1), as well as for corallite morphology (González-Espinosa et al., 2018). In two gonochoric broadcast spawners from the family Fungiidae (Kramarsky-Winter and Loya, 1998; Loya and Sakai, 2008), small individuals are males whereas large individuals were females. In three massive Caribbean species (Mozqueda-Torres et al., 2018; Cruz-Ortega et al., 2020), skeletal growth rates are higher for male colonies than female colonies. Likewise, in the eastern Pacific, P. lobata (Tortolero-Langarica et al., 2016), P. panamensis (Cabral-Tena et al., 2013; Tortolero-Langarica et al., 2017), and the hermaphrodite broadcast spawner Pavona gigantea (Tortolero-Langarica et al., 2017) show sexual dimorphism in annual skeletal growth, all with faster growth in male colonies. Carricart-Ganivet et al. (2013) found differences between sexes in skeletal growth and density banding in Siderastrea siderea, although a more recent study on this same species employing smaller sample sizes (and at a different location) failed to reveal any differences (Benson et al., 2019).
Previous studies have suggested that female colonies may have less energy to invest in growth due to the high energetic cost of producing eggs (Hall and Hughes, 1996; Cabral-Tena et al., 2013. Males, in contrast, may be able to invest more energy in growth due to the lower energetic cost of sperm production. These previous studies (Table 1), along with our new results for corallite morphology, suggest that sexual dimorphism in corals may be common, regardless of phylogenetic lineage (seven genera), geographical location (Red Sea, East China Sea, Caribbean, and eastern Pacific) or spawning mode (broadcast spawner and brooder, this study), and that intersexual differences in corallite integration may facilitate plasticity that allows species to acclimate to different environmental conditions.
4.2 Sexual dimorphism in corallite size
Sexual dimorphism tied to corallite size operates differently in the broadcast spawner P. lobata (this study) than in its brooding congener P. panamensis (González-Espinosa et al., 2018). In P. lobata, corallite area was larger in male colonies than in female colonies (Figure 3; Supplementary Table S1). In contrast, P. panamensis showed larger corallites in female colonies compared to male colonies (González-Espinosa et al., 2018). Gamete production in different coral species is influenced by the number and size of gamete-producing mesenteries in each polyp, which in turn are limited by the space available inside a polyp (Hall and Hughes, 1996). As a result, a larger polyp in male or female (correlated with a larger corallite area) could increase fecundity (Hall and Hughes, 1996; Shlesinger et al., 1998; Leuzinger et al., 2003), as in other broadcast spawning invertebrates (Evans and Sherman, 2013). The patterns observed between species may be due to different constraints imposed on females in the two spawning modes. Broadcast spawning females produce far smaller eggs and there is no higher energy investment in larvae development within their polyps as brooders (Shlesinger et al., 1998; Glynn et al., 2017). If broadcast spawning females are already near their energetic limits of gamete production, there may be little selective pressure for larger female corallites, while larger male colonies may still be favored to increase sperm production to avoid low fertilization success due to sperm dilution (Oliver and Babcock, 1992). These could explain the differences between sexes in corallite size of P. lobata (Figure 3).
Corallite area has also been linked to trophic strategy, with variation in polyp size resulting from a trade-off between optimizing for autotrophy (small size) or heterotrophy (large size) (Conti-Jerpe et al., 2020). Porites species employ both autotrophic and heterotrophic nutritional sources (Palardy et al., 2005; Grottoli et al., 2006). A larger corallite area in males could enhance the capture of particulate organic matter and planktonic prey. Such heterotrophic nutrition sources, and the greater energy reserves they confer, may lead to a higher tolerance to bleaching when the autotrophic benefits of symbiosis are disrupted (Conti-Jerpe et al., 2020; Liu et al., 2022). As stressful warming events occur more frequently (Sully et al., 2019), future studies should test whether corallite size dimorphism results in physiological responses such as calcification rates and bleaching threshold between sexes.
The production of gametes is the most energetically costly physiological activity for corals, especially for female colonies (Hall and Hughes, 1996), sometimes limiting other processes such as skeletal extension and calcification (Cabral-Tena et al., 2013; Tortolero-Langarica et al, 2016; Tortolero-Langarica et al, 2017). While reproductive output increases with polyp size, the ratio of reproductive output to somatic tissue decreases with a higher polyp diameter (Leuzinger et al., 2003). Larger polyps require thicker skeletal elements for support, thereby reducing the space available for reproductive tissue (Leuzinger et al., 2003). Based on this inverse relationship and the high energetic cost of gamete production, if there is no selective pressure for female corals to increase their corallite size (as the pressure of larger sizes in female brooders), a reduced size in corallite size should be favored. Interestingly, the opposite trend has been observed in two solitary broadcast spawning fungiids, where females have larger polyps than males (Kramarsky-Winter and Loya, 1998; Loya and Sakai, 2008). Sexual size dimorphism can vary depending on different selective pressures as seen in P. panamensis (González-Espinosa et al., 2018). Further studies should bring more insight into sexual dimorphism in scleractinian corals.
4.3 Sexual dimorphism in corallite modularity
The integration of corallite traits in P. lobata was slightly higher for females than for males (Figures 4A, B; Supplementary Table S4). Integration may constrain the elements of a module (the corallite here) to evolve in concert due to directional selection on just one or a few traits in the module (Magwene, 2006; Villmoare, 2013; Klingenberg, 2014). Integration between corallite traits in P. lobata was much lower overall than in P. panamensis (Figure 5, Supplementary Tables S4, S5). Less integration, and thus weaker constraints, could allow for greater morphological plasticity in this broadcasting species (Klingenberg, 2014). Indeed, high morphological plasticity is characteristic of the corallites and whole colonies of P. lobata (Forsman et al., 2009). Intraspecific corallite variation is also high, showing differences between inshore and offshore colonies (Tisthammer and Richmond, 2018).
In contrast, in the brooding P. panamensis, corallite trait integration was lower in females than in males (Figure 4, Table S4). Each polyp in a female colony of P. panamensis can brood a single larva that can reach a diameter of 800 µm (Carpizo-Ituarte et al., 2011), potentially placing selective pressure on females to increase the internal space within each corallite to harbor larger larvae (González-Espinosa et al., 2018). Strong selection for larger corallites in females could alter the degree of integration of traits between colonies of different sexes and allow female colonies independence of trait change and greater morphological plasticity. Such plasticity could free them to vary their corallite structure depending on the environment (Todd, 2008) to cope with energetic constraints. This potential plasticity could include (1) increase in the size to accommodate larger larvae, (2) increase their energy supply by having a higher density of dinoflagellate algal symbionts, and (3) enable the polyps to feed on bigger particles. These hypotheses could be tested by measuring larvae size, Symbiodiniaceae density, and total lipid content and fatty acid composition as proxies for food uptake. Liu et al. (2022) tested two factors of the three proposed and found that corallite area increases the food uptake (i.e., higher levels of total lipid content and unsaturated fatty acids) but is not correlated with Symbiodiniaceae density in massive corals.
Sexual dimorphism in P. lobata and P. panamensis was supported by morphological and modularity analyses. Our findings also indicate that corallite diameter and area may be used to identify the sex of P. lobata colonies with modest accuracy. Due to this, these traits can potentially serve as target characteristics in other corals to better understand their reproductive biology. The distinct patterns of sexual dimorphism observed between reproductive modes (larger females in a brooder vs. larger males in a spawner) may be related to the different selective pressures that each sex faces.
Data availability statement
The original contributions presented in the study are included in the article/Supplementary Material. Further inquiries can be directed to the corresponding author.
Ethics statement
Permission to collect samples of P. lobata was granted by The Secretariat of Agriculture, Livestock, Rural Development, Fisheries and Food of Mexico (SAGARPA) (permit No. DGOPA.04552.040711.1798). The permitted collections were made in October 2013 at Isla Isabel National Park. Field collections were made by JJAT-L, APR-T, ALC-M, and with the support of the authorities on both National Parks.
Author contributions
DAP-G, EFB and RMP-P conceived and designed this study. JJAT-L, APR-T, ON-L, RAC-T and ALC-M conducted field work and processing of samples. RMP-P conducted imaging capture and measurements. RMP-P, DAP-G, EFB and MEH conducted data analysis. RMP-P and DAP-G wrote the manuscript. MEH contributed to improvements in the text. All authors contributed to the article and approved the submitted version.
Funding
This work was funded by the project 10023 of PEP Program of CIBNOR to EFB, grant PROMEP-220265 to APR-T, P/PIFI-2010-14MSU0010Z-10 to ALC-M, NGS-55349R-19 to APR-T, PROCER/CCER/DROPC/09/2016 to ALC-M, Marine BioGenomics funding and National Geographic Society.
Acknowledgments
We acknowledge Ariel Cruz Villacorta, Noemí Bocanegra Castillo, Arminda Mejía Rebollo, Claudia Pérez Estrada, and Enrique Morales Bojórquez of CIBNOR for assistance in laboratory work. We thank Erick Treviño Balandra for his assistance in the statistical analyses and the Marine BioGenomics group for support and suggestions in the early stages of preparing the manuscript.
Conflict of interest
The authors declare that the research was conducted in the absence of any commercial or financial relationships that could be construed as a potential conflict of interest.
Publisher’s note
All claims expressed in this article are solely those of the authors and do not necessarily represent those of their affiliated organizations, or those of the publisher, the editors and the reviewers. Any product that may be evaluated in this article, or claim that may be made by its manufacturer, is not guaranteed or endorsed by the publisher.
Supplementary material
The Supplementary Material for this article can be found online at: https://www.frontiersin.org/articles/10.3389/fmars.2022.1068391/full#supplementary-material
References
Baird A. H., Guest J. R., Willis B. L. (2009). Systematic and biogeographical patterns in the reproductive biology of scleractinian corals. Annu. Rev. Ecol. Evol. Syst. 40, 551–571. doi: 10.1146/annurev.ecolsys.110308.120220
Barrett S. C. H., Hough J. (2013). Sexual dimorphism in flowering plants. J. Exp. Bot. 64 (1), 67–82. doi: 10.1093/jxb/ers308
Benson B. E., Rippe J. P., Bove C. B., Castillo K. D. (2019). Apparent timing of density banding in the Caribbean coral Siderastrea siderea suggests complex role of key physiological variables. Coral Reefs 38, 165–176. doi: 10.1007/s00338-018-01753-w
Berns C. M. (2013). “The evolution of sexual dimorphism: Understanding mechanisms of sexual shape differences,” in Sexual dimorphism. Ed. Moriyama H. (London: IntechOpen), 1–16.
Brakel W. (1977). Corallite variation in Porites and the species problem in corals. Proc. 3rd Int. Coral Reef Symp. 1, 457–462.
Budd A. F., Johnson K. G., Potts D. C. (1994). Recognizing morphospecies in colonial reef corals: I. landmark-based methods. Paleobiology 20 (4), 484–505. doi: 10.5194/bg-13-2675-2016
Cabral-Tena R., Reyes-Bonilla H., Lluch-Cota S., Paz-García D., Calderón-Aguilera L., Norzagaray-López O., et al. (2013). Different calcification rates in males and females of the coral Porites panamensis in the gulf of California. Mar. Ecol. Prog. Ser. 476, 1–8. doi: 10.3354/meps10269
Carpizo-Ituarte E., Vizcaíno-Ochoa V., Chi-Barragán G., Tapia-Vázquez O., Cupul-Magaña A. L., Medina-Rosas P. (2011). Evidence of sexual reproduction in the hermatypic corals Pocillopora damicornis, Porites panamensis, and Pavona gigantea in banderas bay, Mexican pacific. Cienc. Mar. 37, 97–112. doi: 10.7773/cm.v37i1.1773
Carricart-Ganivet J. P., Vásquez-Bedoya L. F., Cabanillas-Terán N., Blanchon P. (2013). Gender-related differences in the apparent timing of skeletal density bands in the reef-building coral Siderastrea siderea. Coral Reefs 32, 769–777. doi: 10.1007/s00338-013-1028-y
Conti-Jerpe I. E., Thompson P. D., Wong C. W. M., Wong M., Oliveira N. L., Duprey N. N., et al. (2020). Trophic strategy and bleaching resistance in reef-building corals. Sci. Adv. 6, eaaz5443. doi: 10.1126/sciadv.aaz544
Cruz-Ortega I., Cabral-Tena R. A., Carpizo-Ituarte E., Grosso-Becerra V., Carricart-Ganivet J. P. (2020). Sensitivity of calcification to thermal history differs between sexes in the gonochoric reef-building corals Dichocoenia stokesi and Dendrogyra cylindrus. Mar. Biol. 167, 101. doi: 10.1007/s00227-020-03713-x
Evans J. P., Sherman C. D. H. (2013). Sexual selection and the evolution of egg-sperm interactions in broadcast-spawning invertebrates. Biol. Bull. 224, 166–183. doi: 10.1086/BBLv224n3p166
Forsman Z. H., Barshis D. J., Hunter C. L., Toonen R. J. (2009). Shape-shifting corals: molecular markers show morphology is evolutionarily plastic in Porites. BMC Evol. Biol. 9, 45. doi: 10.1186/1471-2148-9-45
Foster A. B. (1986). Neogene paleontology in the northern Dominican republic. 3. the family poritidae (Anthozoa: Scleractinia). Bull. Am. Paleontol 90, 47–123.
Glynn P. W., Colley S. B., Eakin C. M., Smith D. B., Cortés J., Gassman N. J., et al. (1994). Reef coral reproduction in the eastern pacific: Costa Rica, panamá, and Galapagos islands (Ecuador). II. poritidae. Mar. Biol. 118, 191–208. doi: 10.1007/BF00349785
Glynn P. W., Colley S. B., Guzmán H. M., Enochs I. C., Cortés J., Maté J. L., et al. (2011). Reef coral reproduction in the eastern pacific: Costa Rica, panamá and the galápagos islands (Ecuador). VI. agariciidae, Pavona clavus. Mar. Biol. 158, 1601–1617. doi: 10.1007/BF01313501
Glynn P. W., Manzello D. P., Enochs I. C. (2017). Coral reefs of the Eastern tropical pacific (Dordrecht: Springer Netherlands).
González-Espinosa P. C., Paz-García D. A., Reyes-Bonilla H., Cabral-Tena R. A., Balart E. F. (2018). Evidence of sexual dimorphism in skeletal morphology of a gonochoric reef coral. R. Soc Open Sci. 5, 171843. doi: 10.1098/rsos.171843
Grottoli A. G., Rodrigues L. J., Palardy J. E. (2006). Heterotrophic plasticity and resilience in bleached corals. Nature 440, 1186–1189. doi: 10.1038/nature04565
Hall V. R., Hughes T. P. (1996). Reproductive strategies of modular organisms: comparative studies of reef- building corals. Ecology 77, 950–963. doi: 10.2307/2265514
Harrison P. L. (2011). Sexual reproduction of scleractinian corals, in coral reefs: An ecosystem in transition. Eds. Dubinsky Z., Stambler N. (Dordrecht: Springer Netherlands), 59–85. doi: 10.1007/978-94-007-0114-4
Klingenberg C. P. (2014). Studying morphological integration and modularity at multiple levels: concepts and analysis. Philos. Trans. R. Soc B Biol. Sci. 369, 33–35. doi: 10.1098/rstb.2013.0249
Kramarsky-Winter E., Loya Y. (1998). Reproductive strategies of two fungiid corals from the northern red Sea : Environmental constraints? Mar. Ecol. Prog. Ser. 174, 175–182. doi: 10.3354/meps174175
Lasker H. R., Boller M. L., Castanaro J., Sánchez J. A. (2003). Determinate growth and modularity in a gorgonian octocoral. Biol. Bull. 205, 319–330. doi: 10.2307/1543295
Leuzinger S., Anthony K. R. N., Willis B. L. (2003). Reproductive energy investment in corals: scaling with module size. Oecologia 136, 524–531. doi: 10.1007/s00442-003-1305-5
Levitan D. R. (2008). Gamete traits influence the variance in reproductive success, the intensity of sexual selection, and the outcome of sexual conflict among congeneric sea urchins. Evolution 62, 1305–1316. doi: 10.1111/j.1558-5646.2008.00378.x
Levitan D. R. (2010). “Sexual selection in external fertilizers,”. Evolutionary Behav. Ecology eds Westneat D., Fox W. (New York), 1, 365–378.
Liu C., Zhang Y., Huang L., Yu X., Luo Y., Jiang L., et al. (2022). Differences in fatty acids and lipids of massive and branching reef-building corals and response to environmental changes. Front. Mar. Sci. 9. doi: 10.3389/fmars.2022.882663
López-Pérez R. A. (2013). Species composition and morphologic variation of Porites in the Gulf of California. Coral Reefs 32, 867–878. doi: 10.1007/s00338-013-1031-3
Loya Y., Sakai K. (2008). Bidirectional sex change in mushroom stony corals. Proc. R. Soc B Biol. Sci. 275, 2335–2343. doi: 10.1098/rspb.2008.0675
Magwene P. M. (2001). New tools for studying integration and modularity. Evol. (N. Y). 55, 1734–1745. doi: 10.1111/j.0014-3820.2001.tb00823.x
Magwene P. M. (2006). Integration and modularity in biological systems: a review. Acta Zool. Sin. 52 (Supplement), 490–493.
Mozqueda-Torres M. C., Cruz-Ortega I., Calderon-Aguilera L. E., Reyes-Bonilla H., Carricart-Ganivet J. P. (2018). Sex-related differences in the sclerochronology of the reef-building coral Montastraea cavernosa: the effect of the growth strategy. Mar. Biol. 165, 32. doi: 10.1007/s00227-018-3288-0
Neves E. G. (2000). Histological analysis of reproductive trends of three Porites species from kane’ohe bay, hawai’i. Pac Sci. 54, 195–200.
Oliver J., Babcock R. (1992). Aspects of the fertilization ecology of broadcast spawning corals: sperm dilution effects and in situ measurements of fertilization. Biol. Bull. 183, 409–417. doi: 10.2307/1542017
Palardy J., Grottoli A., Matthews K. (2005). Effects of upwelling, depth, morphology and polyp size on feeding in three species of Panamanian corals. Mar. Ecol. Prog. Ser. 300, 79–89. doi: 10.3354/meps300079
Parker G. A. (1979). “Sexual selection and sexual conflict,” in Sexual selection and reproduction in insects. Eds. Blum M. S., Blum N. A. (New York: Academic Press), 123–166.
Paz-García D. A., Aldana-Moreno A., Cabral-Tena R. A., García-De-León F. J., Hellberg M. E., Balart E. F. (2015). Morphological variation and different branch modularity across contrasting flow conditions in dominant Pocillopora reef-building corals. Oecologia 178, 207–218. doi: 10.1007/s00442-014-3199-9
Pichon M. (2011). “Porites,” in Encyclopedia of modern coral reefs. Ed. Hopley D. (Dordrecht: Springer), 815–821.
Sánchez J. A., Aguilar C., Dorado D., Manrique N. (2007). Phenotypic plasticity and morphological integration in a marine modular invertebrate. BMC Evol. Biol. 7, 122. doi: 10.1186/1471-2148-7-122
Sánchez J. A., Lasker H. R. (2003). Patterns of morphological integration in marine modular organisms: supra-module organization in branching octocoral colonies. Proc. R. Soc London. Ser. B Biol. Sci. 270, 2039–2044. doi: 10.1098/rspb.2003.2471
Schindelin J., Rueden C. T., Hiner M. C., Eliceiri K. W. (2015). The ImageJ ecosystem: An open platform for biomedical image analysis. Mol. Reprod. Dev. 82, 518–529. doi: 10.1002/mrd.22489
Shaish L., Abelson A., Rinkevich B. (2007). How plastic can phenotypic plasticity be? the branching coral Stylophora pistillata as a model system. PloS One 2, e644. doi: 10.1371/journal.pone.0000644
Shlesinger Y., Goulet T. L., Loya Y. (1998). Reproductive patterns of scleractinian corals in the northern red Sea. Mar. Biol. 132, 691–701. doi: 10.1007/s002270050433
Sully S., Burkepile D. E., Donovan M. K., Hodgson G., van Woesik R. (2019). A global analysis of coral bleaching over the past two decades. Nat. Commun. 10 (1), 1264. doi: 10.1038/s41467-019-09238-2
Tisthammer K. H., Richmond R. H. (2018). Corallite skeletal morphological variation in Hawaiian Porites lobata. Coral Reefs 37, 445–456. doi: 10.1007/s00338-018-1670-5
Todd P. A. (2008). Morphological plasticity in scleractinian corals. Biol. Rev. 83, 315–337. doi: 10.1111/j.1469-185X.2008.00045.x
Tortolero-Langarica J. J. A., Carricart-Ganivet J. P., Cupul-Magaña A. L., Rodríguez-Troncoso A. P. (2017). Historical insights on growth rates of the reef-building corals Pavona gigantea and Porites panamensis from the northeastern tropical pacific. Mar. Environ. Res. 132, 23–32. doi: 10.1016/j.marenvres.2017.10.004
Tortolero-Langarica J., de J. A., Cupul-Magaña A. L., Carricart-Ganivet J. P., Mayfield A. B., Rodríguez-Troncoso A. P. (2016). Differences in growth and calcification rates in the reef-building coral Porites lobata: The implications of morphotype and gender on coral growth. Front. Mar. Sci. 3. doi: 10.3389/fmars.2016.00179
Veron J. E. N. (2000). Corals of the world Vol. 1–3 (Queensland: Australian Institute of Marine Science and CRR).
Villmoare B. (2013). Morphological integration, evolutionary constraints, and extinction: A computer simulation-based study. Evol. Biol. 40, 76–83. doi: 10.1007/s11692-012-9186-3
Ward S. (1995). Two patterns of energy allocation for growth, reproduction and lipid storage in the scleractinian coral Pocillopora damicornis. Coral Reefs 14, 87–90. doi: 10.1007/BF00303428
Keywords: reproduction, morphometrics, Porites, corallite, Eastern Tropical Pacific (ETP), phenotypic modularity
Citation: Pedraza-Pohlenz RM, Balart EF, Tortolero-Langarica JJA, Rodríguez-Troncoso AP, Hellberg ME, Norzagaray-López O, Cabral-Tena RA, Cupul-Magaña AL and Paz-García DA (2023) Sexual dimorphism in corallite size and modularity of a broadcast spawning coral, Porites lobata. Front. Mar. Sci. 9:1068391. doi: 10.3389/fmars.2022.1068391
Received: 12 October 2022; Accepted: 20 December 2022;
Published: 10 January 2023.
Edited by:
Yehuda Benayahu, Tel Aviv University, IsraelReviewed by:
Karen Lynn Neely, Nova Southeastern University, United StatesSarah Whitney Davies, University of North Carolina at Chapel Hill, United States
Copyright © 2023 Pedraza-Pohlenz, Balart, Tortolero-Langarica, Rodríguez-Troncoso, Hellberg, Norzagaray-López, Cabral-Tena, Cupul-Magaña and Paz-García. This is an open-access article distributed under the terms of the Creative Commons Attribution License (CC BY). The use, distribution or reproduction in other forums is permitted, provided the original author(s) and the copyright owner(s) are credited and that the original publication in this journal is cited, in accordance with accepted academic practice. No use, distribution or reproduction is permitted which does not comply with these terms.
*Correspondence: David A. Paz-García, dpaz@cibnor.mx