- 1Zhejiang Province Key Laboratory of Mariculture and Enhancement, Zhejiang Marine Fisheries Research Institute, Zhoushan, China
- 2Marine and Fisheries Institute, Zhejiang Ocean University, Zhoushan, China
Portunus trituberculatus (swimming crab) is an important breeding crab in China. The current breeding mode of swimming crab is still the traditional “spring seedling and winter harvest” breeding mode. In recent years, researchers have begun to explore a new off-season breeding mode through autumn seedling. In this study, the rate of embryonic development, embryo antioxidant ability and hatching rate of swimming crab in different water temperatures in an off-season breeding mode (breeding in early autumn) and digestive enzyme activity of newly hatched larvae were compared. The results showed that the duration of each development stage of swimming crab embryos was gradually reduced with increasing water temperature. The total development time was 9.43 d at 27°C and only 6.88 d at 33°C. These effects were accompanied by an increase in the development rate from 0.11 d-1 to 0.15 d-1. The total effective accumulated temperature under the 4 temperature conditions was basically maintained at about 150°C·d (147.08 ∼ 153.62°C·d), and there was no significant difference between different groups. The development of embryos at 27°C and 29°C was very synchronous, and no abnormal embryos were observed. Conversely, at 31°C, the later stage of development exhibited asynchrony, and diapause and death were noted in some embryos. At 33°C, more embryos died. The embryo hatching rate was approximately 70% at 27°C and 29°C, and the rate was significantly reduced at 31°C and 33°C. Specifically, the rate was only 13.89% at 33°C. As the water temperature increased, the activities of superoxide dismutase (SOD) and glutathione peroxidase (GSH-PX) as well as the total antioxidant capacity (T-AOC) and malondialdehyde (MDA) levels of embryos increased first and then decreased, reaching the highest value at 31°C. Catalase (CAT) activity exhibited the opposite trend which was the lowest at 29 °C with a value of only 0.17 U/mg prot and the highest at 33°C with a value of up to 0.51 U/mg prot. At temperatures of 27°C, 29°C and 31°C, the differences in various digestive enzymes of newly hatched larvae primarily manifested as the high activities of pepsin (PEP) and α-amylase (AMS) at 31°C, and other differences were not obvious. At 33°C during embryonic development, the activities of various digestive enzymes were relatively low, especially the activities of trypsin (TPS) and cellulase (CL), which were significantly lower than those of the other three temperature groups. Therefore, judging from the antioxidant index of embryos and the digestive enzyme activity of newly hatched larvae, the embryonic development was still good when the water temperature was 31°C, accompanied by water temperatures above 31°C that seriously affected enzyme activity. Overall, a temperature below 31°C represents the appropriate temperature for embryonic development in autumn in swimming crabs.
Introduction
Protunus trituberculatus (swimming crab), an economic marine crab with the advantages of delicious meat and rich nutrition, is popular among consumers in China and represents a valuable exported aquatic product (He et al., 2017). Due to the implementation of a forbidden fishing period in the natural sea area, the proliferation and release of swimming crabs and the protection of the water ecological environment in the past decade, the wild population of swimming crabs along the coast of China has increased yearly with the annual catch amount of the whole country stabilizing at approximately 500,000 tons from 2015 to 2020 (China Fisheries Statistical Yearbook, 2015-2021). However, the establishment of the forbidden fishing system has also resulted in a lack of crabs available on the market during the forbidden fishing period. Especially in 2017, the Ministry of Agriculture of the People’s Republic of China clearly stipulated that the annual forbidden fishing period for swimming crabs is from May 1 to August 1, leading to a three-month gap in the market for swimming crabs.
Since the 1990s, China has conducted more systematic research on swimming crabs in seedling breeding (Fan et al., 2008), breeding of fine varieties (Gao et al., 2015), efficient pond culture (He et al., 2017), prevention and control of common diseases (Wan et al., 2011), and development of compound feed (Han et al., 2018). The results have promoted the rapid development of artificial cultivation of swimming crabs. In 2020, the cultivation area of swimming crabs in China was approximately 20,671 ha, and the production also reached 100,895 tons (data from China Fisheries Statistical Yearbook, 2021). However, the current cultivation mode remains the traditional “spring seedling and winter harvest”. Under this model, the seedlings are raised in spring (April to May), cultured until winter, harvested, and marketed in winter. Thus, the current cultivation mode cannot meet the market demands of cultured swimming crabs from May to August.
Off-season marketing through off-season breeding is an effective method to accelerate the transformation and upgrading of the fisheries industry, ensure a balanced supply of aquatic products and achieve sustained and rapid growth of fishermen’s income. To fill the market gap of swimming crabs during the forbidden fishing period, researchers began to explore a new off-season breeding and cultivation model to meet the demands of the next year’s off-season market by breeding in autumn (Yu et al., 2021). The weather in early autumn in South China is still relatively hot, and the water temperature of the culture pond is approximately 30°C with temperatures occasionally reaching up to 33°C. As an important element in the study of aquatic animal reproductive biology, embryonic development is the basis of larval development. Numerous studies have demonstrated that aquatic animals respond more rapidly to changes in the water environment during embryonic development, and unsuitable environments will seriously affect embryonic development. Specifically, high temperature often leads to embryonic deformity or diapause and cause severe embryonic death (Zhong, 2014; Güralp et al., 2017; Ashaf-Ud-Doulah et al., 2021). Therefore, it is of great significance to determine the appropriate temperature and temperature limits of embryonic development for the breeding and cultivation of swimming crabs in autumn.
The morphology and rate of embryonic development as well as the final hatching rate are important indicators for evaluating the quality of embryonic development. In addition, antioxidant enzyme activity can reflect the physiological condition of embryos to a certain extent (Fan et al., 2019). In addition, digestive enzyme activity can reflect the feeding and digestive capacity of the newly hatched larvae of swimming crabs (Pan and Wang, 1997). Thus, this study compares the rate of embryonic development, embryo antioxidant ability and hatching rate of swimming crab in different water temperatures in an off-season breeding mode (breeding in early autumn) and digestive enzyme activity of newly hatched larvae. The study goal is to identify the temperature limits of swimming crab embryonic development, providing a crucial theoretical basis and practical reference for the selection of off-season breeding time and regulation of water temperature.
Materials and methods
Source of experimental crabs and temporary rearing conditions
In mid-August 2021, 80 wild female swimming crabs with well-developed gonads, sound limbs, an average body weight of 155.48 ± 12.63 g and a carapace length of 68.13 ± 3.27 mm were purchased from a fishing vessel in Daishan County, Zhoushan City, Zhejiang Province. The selection of female crabs with well- developed ovaries was carried out by adopting a non-intrusivemethod of shining a bright light through the underside of the crab carapace (Wu et al., 2010). Crabs were transported to the test site of Zhejiang Marine Fisheries Research Institute and temporarily reared in an indoor culture pond (2.0 × 6.0 × 1.0 m) with 10-cm thick mud and sand at the bottom. The following pool conditions were maintained: 0.4 m water depth, 29.1 ± 0.1°C water temperature, 26.5 ± 0.5 salinity, and DO ≥ 5 mg/ml. During the temporary rearing period, oxygen was continuously increased for 24 hours. Fresh Sinonovacula constricta were fed at 5:00 pm daily at approximately 10% of the crab’s body weight, and the feeding amount was flexibly adjusted depending on the feeding condition. In addition, isothermal seawater (seawater filtered by screen and dark precipitated) was changed at 7:00 am daily with a renewed seawater volume of 1/2.
Establishment of different water temperatures
Experiments were conducted in 12 culture buckets with four temperature gradients (27°C, 29°C, 31°C and 33°C). Here, 27°C was controlled by a refrigerating machine, and temperatures of 29°C, 31°C and 33°C were controlled by heating rods. Three replicates were performed for each temperature group. Forty-eight crabs with good vitality were selected from the culture pond, numbered individually with a white paint pen on their backs, and randomly divided equally into 12 culture buckets. Thus, there were 12 crabs in 3 buckets of each temperature group. The spawning status of each crab was observed every 8 hours, and the time when the crab began to spawn was recorded.
Observation of embryonic development and calculation of effective accumulated temperature
Once a berried female was found, embryonic development was continuously observed as described by Yu et al. (2021). Observations were made every 1 hour before the blastocyst stage and every 6 hours after the blastocyst stage.), and fixed with Bouin’s solution. The embryonic development of each crab was observed using a biological microscope (model: BELONA-XSZ 07), and the development time of each stage of embryonic development was recorded. Regarding the determination of development stage, the criterion was that 60% of the embryos showed developmental characteristics of a certain period. The effective accumulated temperature during embryonic development was calculated based on the following formula (Zhang et al., 2011; Liu et al., 2021):
Where C is the biological zero of embryonic development of swimming crab; T is the habitat water temperature (°C); V is developmental rate (1/N); n is the temperature gradient number. K is the effective accumulated temperature (°C·d) for embryonic development of swimming crab; N is the time required for the development of embryos (d).
Determination of embryo hatching rate
The embryo hatching rate was measured in beakers containing 500 ml seawater. The water temperature in the beakers was controlled by a thermostat water bath with four temperature gradients of 27°C, 29°C, 31°C and 33°C, and three beakers were used for each temperature group. When the embryos in each temperature group developed to the prehatching stage and the heartbeat reached greater than 200 beats/min, 30 embryos were removed from the abdomen of broodstock crabs with a pipette (4 crabs were selected from each temperature group only to measure embryo hatching rate) and then divided equally into 3 corresponding beakers for hatching. The next day, the hatching of embryos in beakers was observed. The hatching rate was calculated as follows:
Hatching rate = n2/n1 × 100%
where n2 indicates the number of embryos hatched in the beaker, and n1 indicates the total number of selected embryos in the beaker.
Determination of the embryo antioxidant index and digestive enzyme activity of newly hatched larvae
4 crabs were selected from each temperature group only to determine the embryo antioxidant index and digestive enzyme activity of newly hatched larvae. When the embryos developed to the prehatching stage (heartbeat was approximately 200 beats/min), approximately 5.0 g embryos were obtained from the abdomen of broodstock crabs. After these embryos were quickly dried by absorbent paper, they were placed in centrifuge tubes and stored at -80°C. These embryos were used for subsequent determination of antioxidant enzyme activity. Embryo samples were rinsed with preprepared physiological saline (4°C, 0.9%), and then 10% homogenate was made by adding prechilled physiological saline in the mass-volume ratio (1 g:10 ml) and mechanical homogenization under ice bath conditions. Subsequently, the supernatant was centrifuged at 4°C for 10 min at 2,500 r/min and stored in a -80°C refrigerator for the determination of antioxidant enzyme activity. Notably, for the determination of the total antioxidant ability, the mass-volume ratio was 1 g:4 ml, and the centrifugation conditions were 12,000 r/min to sufficiently break the cells to release the antioxidants. The enzyme solutions were prepared in different concentrations in accordance with the specific needs. Finally, the index was determined.
When the embryo developed to the prehatching stage, broodstock crabs of all temperature groups were moved to 24 hatching buckets of the same water temperature (30°C), and 500 L volume was prepared in advance for hatching (1 crab was put in each hatching bucket). After hatching, all larvae were removed immediately with a 200-mesh sieve. Similarly, excess water was absorbed with absorbent paper, packed in centrifuge tubes and store it in a -80°C refrigerator. The supernatant was obtained from the newly hatched larvae samples in the same manner as described above and used for digestion enzyme determination (cellulase should be determined by centrifugation at 4000 r/min).
The activities of superoxide dismutase (SOD), catalase (CAT), glutathione peroxidase (GSH-Px), total antioxidant capacity (T-AOC) and the levels of malondialdehyde (MDA)of the embryos were measured using kits produced by Nanjing Jiancheng Bioengineering Institute. Similarly, the activities of pepsin (PEP), trypsin (TPS), lipase (LPS), α-amylase (AMS) and cellulase (CL) of the newly hatched larvae were determined using commercially available kits (produced by Nanjing Jiancheng Bioengineering Institute).
Analysis of data
Excel 2019 was used to collect the experimental data, and all results are expressed as the mean ± standard deviation (mean ± SD). The data were subjected to one-way analysis of variance (one-way ANOVA) using SPSS 22.0 software. Multiple comparisons were performed by LDS, and significant differences were indicated when P< 0.05.
Results
Embryonic development characteristics of swimming crabs
During the experiment, there was no natural death of the broodstock crabs under the four water temperatures.The cleavage mode during the embryonic development of swimming crabs is typical superficial cleavage. The embryonic development of swimming crabs was divided into seven stages of embryonic development, namely, zygote, cleavage, blastocyst, gastrula, nauplius, protozoea, and prehatching stages, based on the morphological characteristics of each stage. When round or oval zygotes were closely distributed on the outside of the setae of the pleopods, the zygotes were dark in color, and the colorless transparent egg membrane was tightly adhered to the surface of the zygotes (Figure 1A). The surface of the embryo at cleavage was covered by a transparent egg membrane, and clear cleavage grooves were visible (Figure 1B). Multiplicative growth was noted throughout this development period with the zygotes eventually entering the 256-cell stage after eight cleavages. At the blastocyst stage, the cleavage grooves disappeared, and the surface of the embryo exhibited a uniform and dense state without any massive structure. In addition, a mass of blastomeres were arranged on the surface of the embryo and wrapped by a thin blastoderm (Figure 1C). In addition, the yolk granules filled all the blastocoel under the blastoderm, forming the yolk sac. At the gastrula, one end of the yolk inside the embryo invaginated from the outside to the inside, and a small crescent-shaped transparent area appeared, forming the archenteron and protostoma (Figure 1D). During this period, the cells achieved the recombination of blastocyst cells by movement. Given constant cell proliferation and differentiation, cells and tissues gradually underwent morphological self-organization. The transparent area at the nauplius gradually increased while the yolk color faded step by step, and the yolk mass was also vaguely visible (Figure 1E). In addition, with the continuous cell division and differentiation, the optic lobe rudiment, antenna rudiment and mandible rudiment were gradually formed, and the number of appendages also increased until seven pairs formed. At the protozoea stage, the transparent area further expanded. In addition, the yolk area decreased, and stripes of jacinth eyespots began to appear on both sides of the transparent area (Figure 1F). Subsequently, the compound eye pigment area expanded and became darker. The cardiac rudiment appeared on the back of the yolk sac, and a heartbeat was soon identified. However, the heart rate was low and irregular. Until the prehatching stage, the yolk was gradually absorbed, and only a small amount remained beside the compound eye. The color deepened while the area of compound eye pigment became larger, and the telson appeared at this stage with obvious abdominal somites (Figure 1G). Moreover, the heartbeat was rhythmic with a speed of up to 200 beats/min. This stage was followed by the larvae exiting the membrane (Figure 1H).
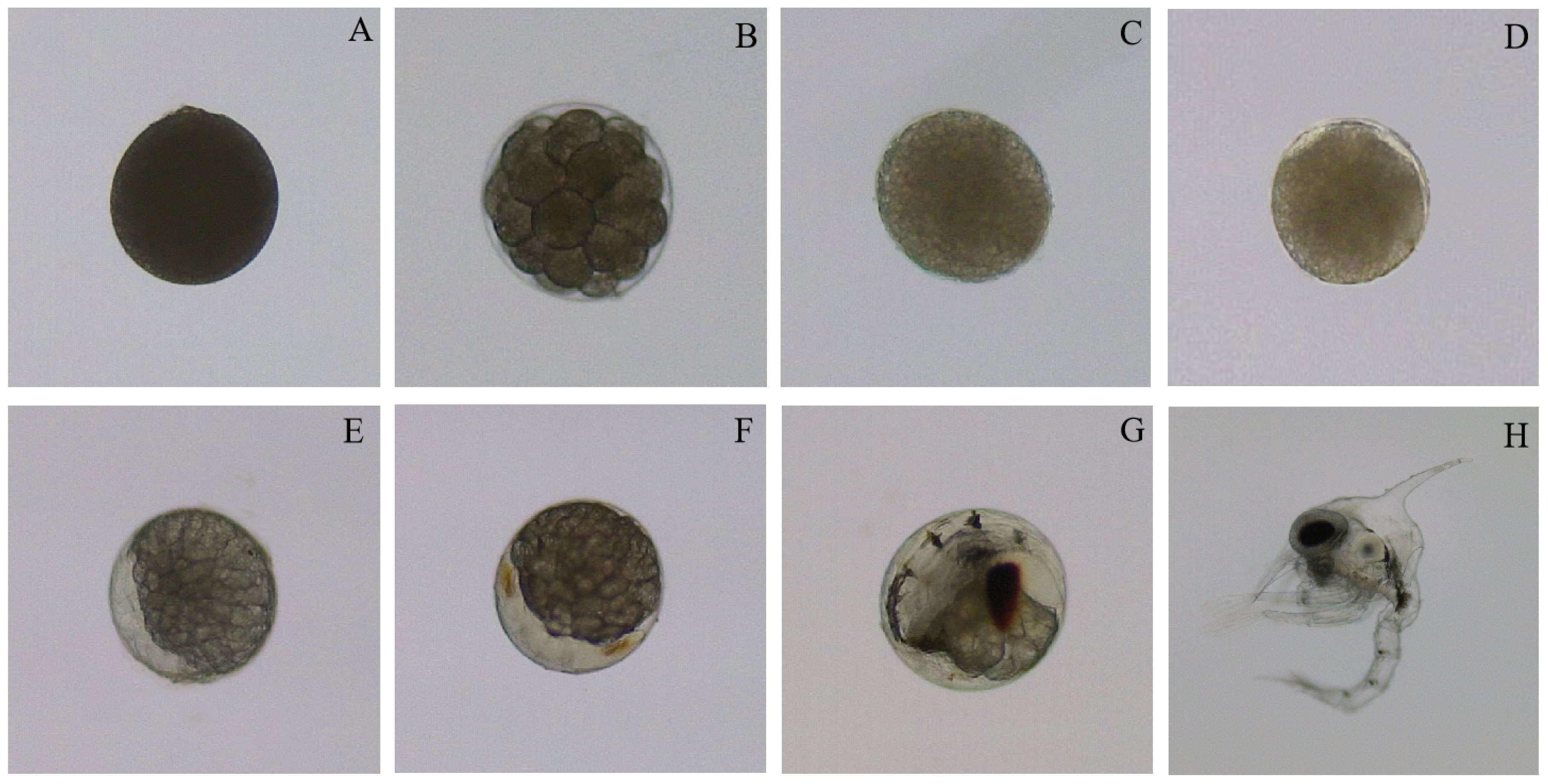
Figure 1 Embryonic development characteristics of swimming crab (A): Zygote; (B): Cleavage; (C): Blastocyst; (D) Gastrula; (E): Nauplius; (F): Protozoea; (G): Prehatching stage; (H): Newly hatched larvae.
Effect of water temperature on embryonic development rate and total effective accumulated temperature of swimming crabs
As shown in Table 1, the duration of each development stage gradually shortened with increasing water temperature, especially the total development time. The total development time was 9.43 d at 27°C and only 6.88 d at 33°C, representing an approximately one-quarter reduction. Correspondingly, the development rate increased from 0.11 h-1 to 0.15 h-1. Regression analysis of total development time and development rate with water temperature was used to obtain the following regression equation of total embryonic development time and water temperature: N=-0.4174T+20.448 (R2 = 0.9414, Figure 2). In addition, the regression equation of embryonic development rate and water temperature was V=0.0065T-0.0667 (R2 = 0.9766, Figure 3). A highly significant linear correlation was observed. Additionally, the highest total effective accumulated temperature was 153.62°C·d at 27°C, and compared with the other three groups, these values were not significantly different (P>0.05).
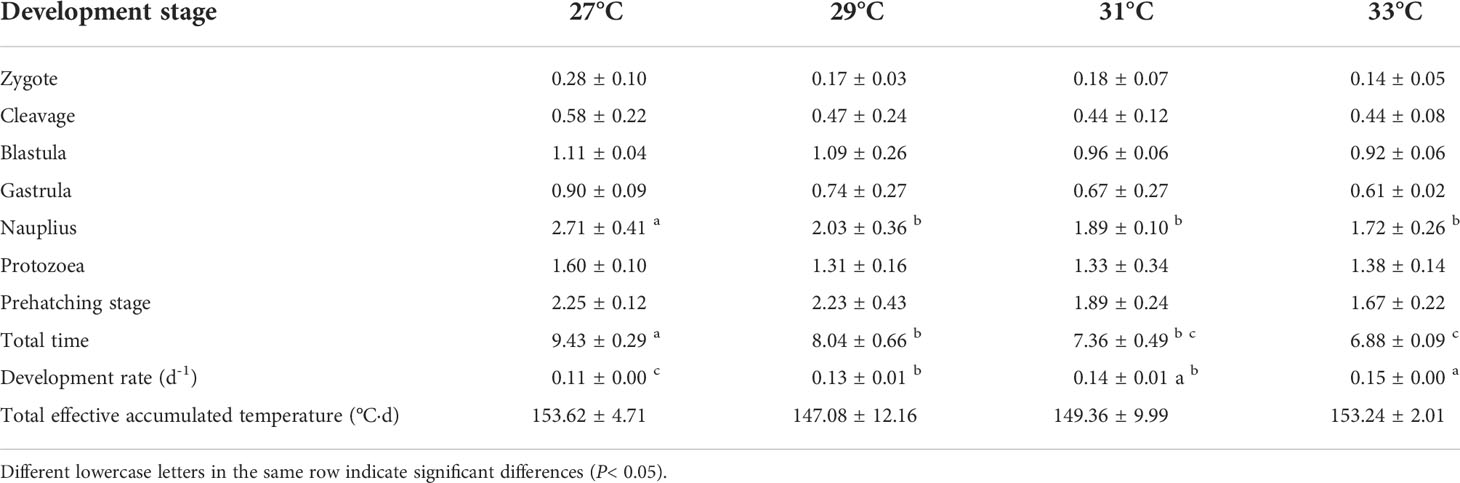
Table 1 The embryonic development time and effective accumulated temperature of swimming crab at four water temperatures.
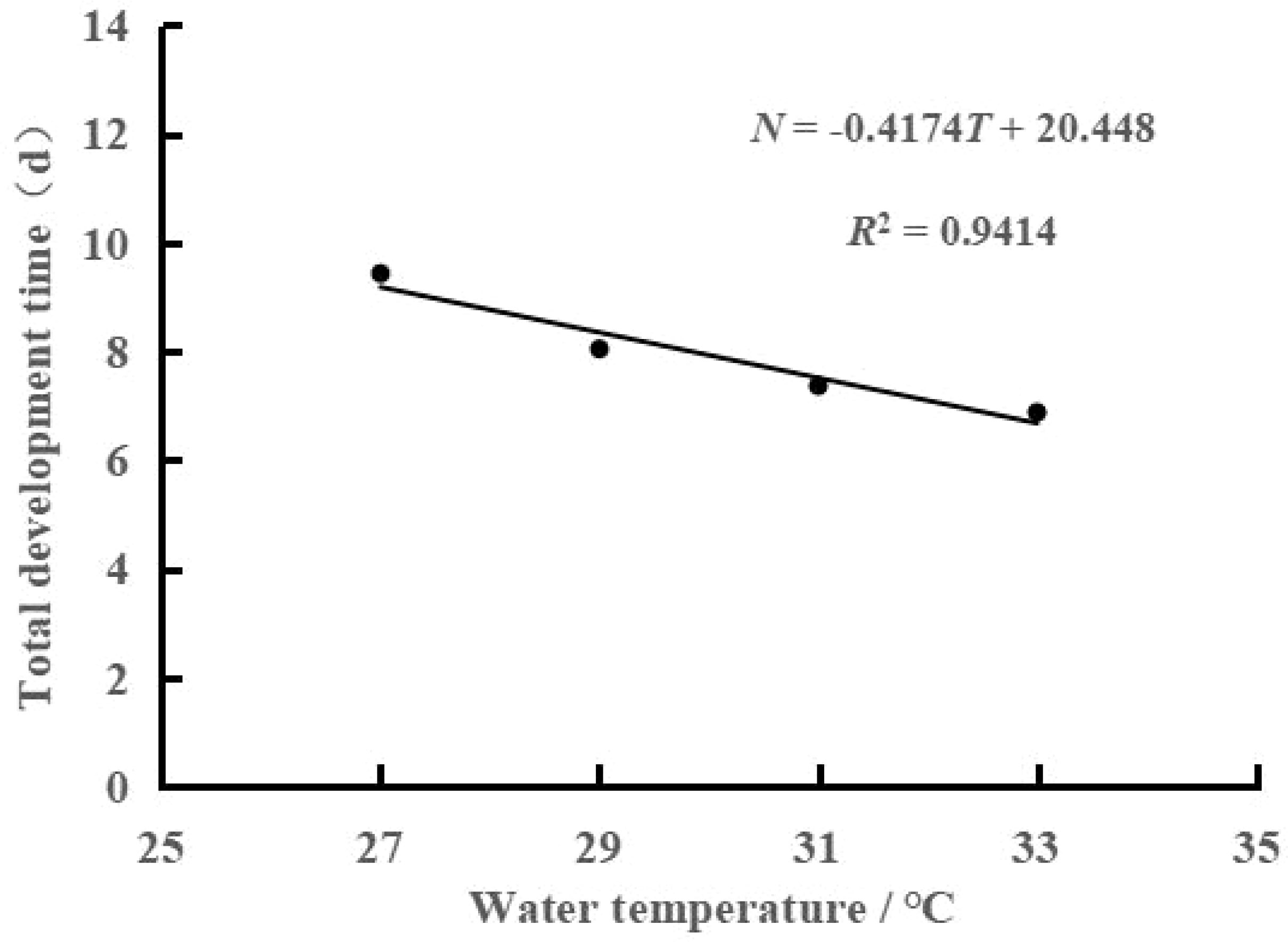
Figure 2 Relationship between time (N) and water temperature (T) for embryonic development of swimming crab.
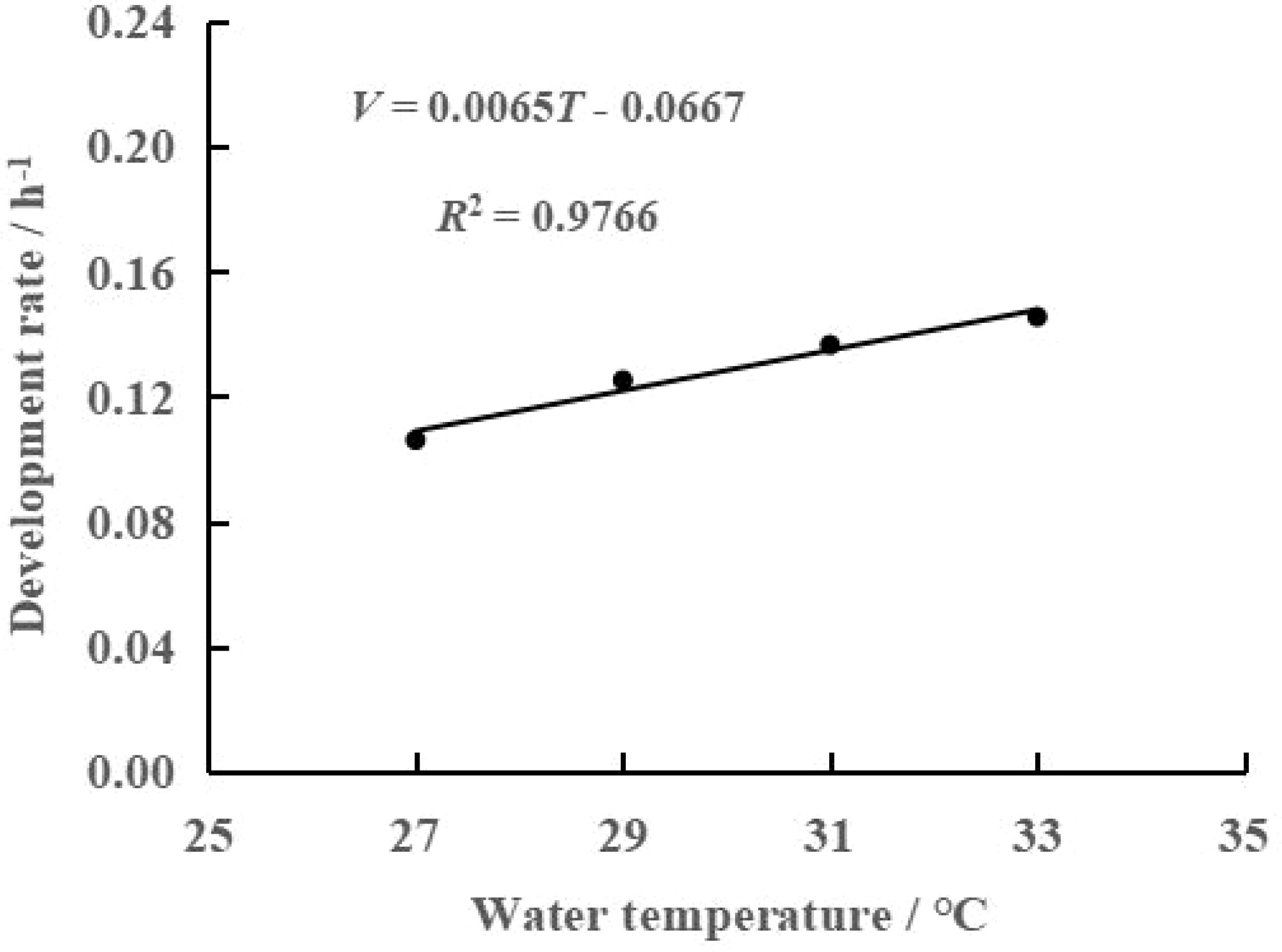
Figure 3 Relationship between rate (V) and water temperature (T) for embryonic development of swimming crab.
Effect of water temperature on the embryonic development synchronization and hatching rate of swimming crabs
For the same broodstock crab, embryonic development was very synchronous at 27°C and 29°C, and no abnormal embryos were observed (Figure 4A, B). However, at 31°C, the embryos were not synchronous at the later stage of development. For example, when most of the embryos entered protozoea, some were still in the nauplius, and some embryos exhibited diapause and death (Figure 4C). The situation was more serious at 33°C; in particular, many embryos with darker color and their internal structures were not visible (Figure 4D). Notably, there were no dead eggs remaining in the abdomen of the broodstock crabs after the embryos hatched at 27°C and 29°C, and no dead embryos that sank to the bottom of the water were visible in the hatching bucket. However, at 31°C and 33°C, the abdomen of the broodstock crabs was not so clean, especially at 33°C, when more dead eggs were left in the abdomen, and many dead embryos and dead newly hatched larvae could be observed at the bottom of the hatching bucket. To obtain a clearer understanding of the final hatching rate of embryos at the four water temperatures, the hatching rate of embryos was observed individually in beakers. The results revealed that the higher hatching rates of approximately 70% at 27°C and 29°C, and the rate significantly decreased to only 13.89% at 33°C (Figure 5).
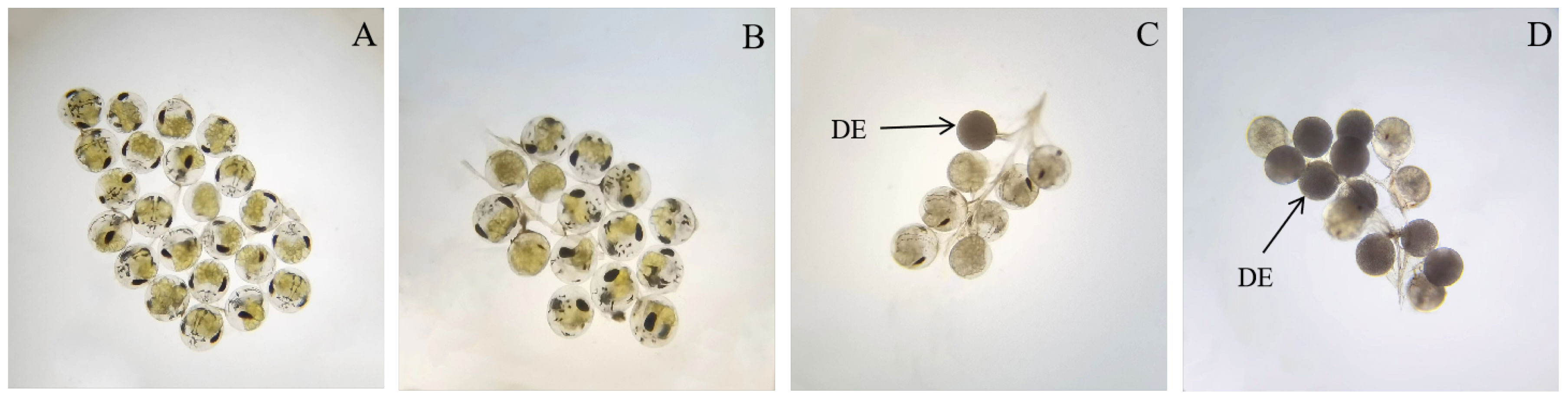
Figure 4 Embryonic development synchronization of swimming crab at four water temperatures (A: 27°C; B: 29°C; C: 31°C; D: 33°C; DE: Dead embryos).
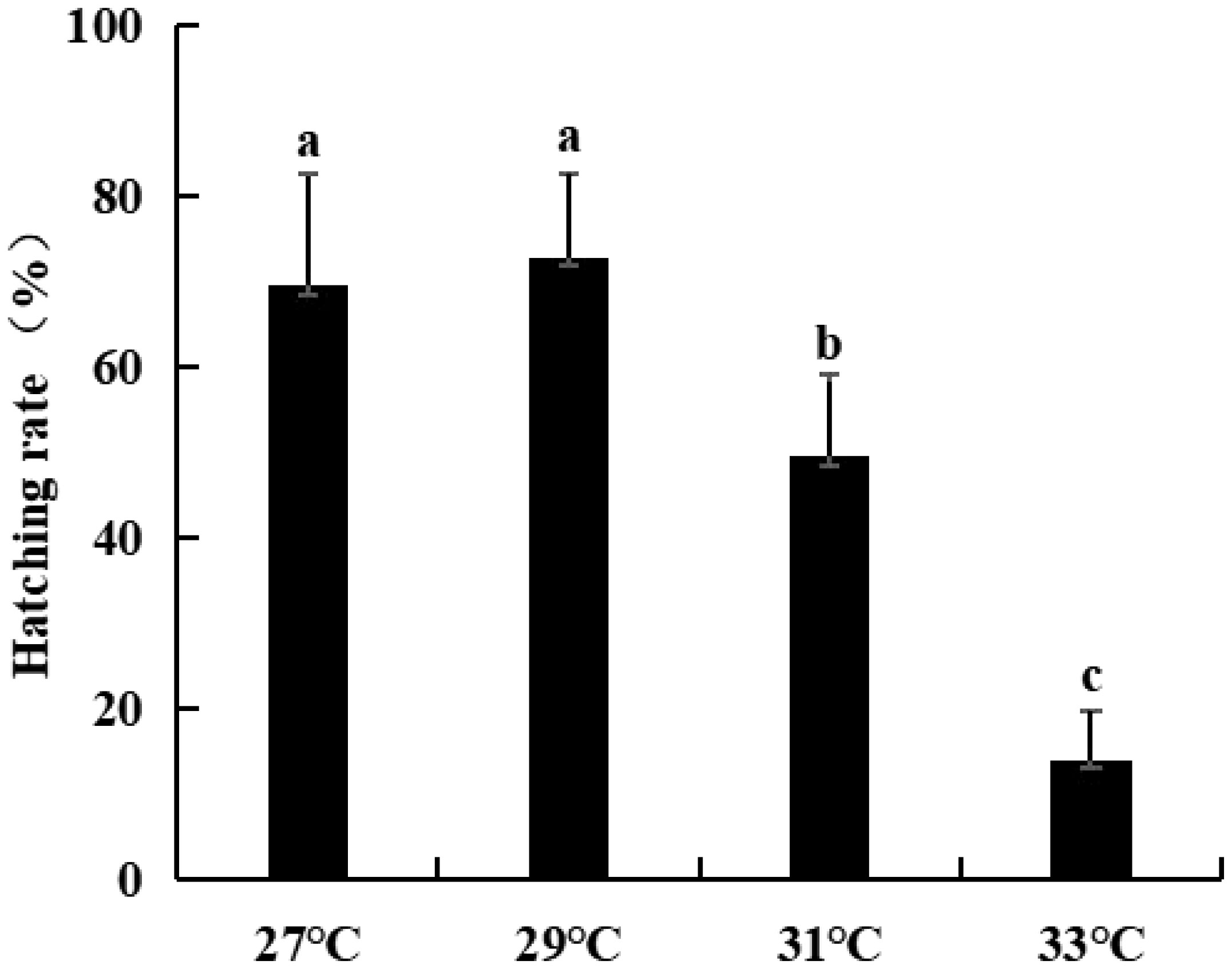
Figure 5 Embryo hatching rate of swimming crab at four water temperature. Different lowercase letters above the figure column indicate that there are significant differences between different temperature groups (P< 0.05).
Effect of water temperature on the embryo antioxidant index of swimming crabs
With increasing water temperature, the activities of SOD, GSH-PX and T-AOC as well as the MDA content showed a trend of first increasing and then decreasing and was the highest at 31°C (Figure 6). Among them, the SOD activity at 31°C reached 188.42 U/mg prot, which was significantly higher than that noted in the other groups (P<0.05), whereas GSH-PX activity and MDA content were not significantly different compared with values obtained from the other groups. The trend of CAT activity was opposite to the above four indicators. Specifically, with increasing water temperature, the enzyme activity first decreased and then increased. CAT activity was the lowest at 29°C (0.17 U/mg prot), and the highest at 33°C (0.51 U/mg prot). In addition, significantly greater CAT activity was noted at 31°C compared with that at 27°C and 29°C (P<0.05). In general, the water temperatures tested in this study had minimal effects on the embryo antioxidant index of swimming crabs. Relatively high values were obtained at 31°C.
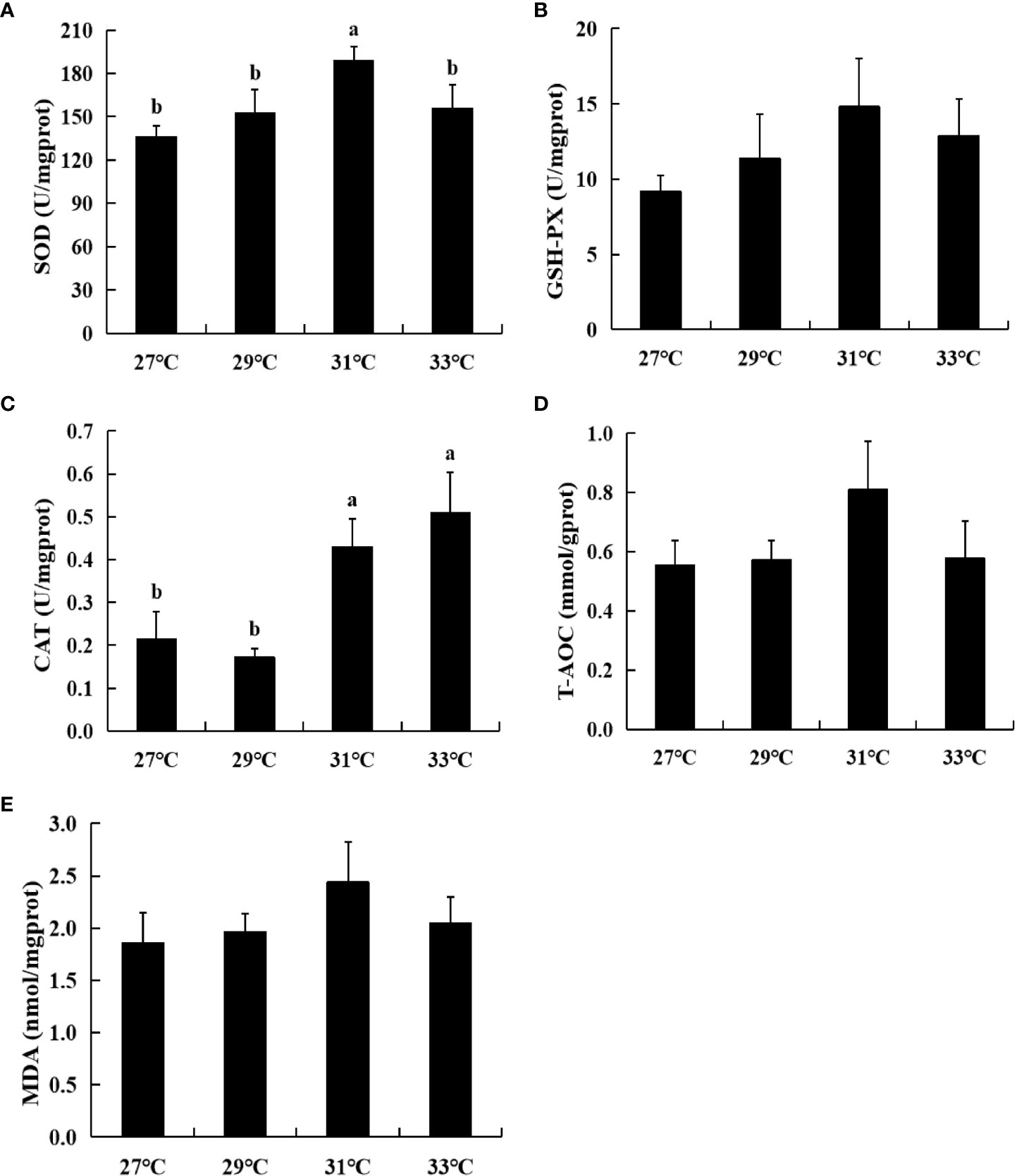
Figure 6 Embryo antioxidant index of swimming crab at four water temperatures. (A) Superoxide dismutase (SOD); (B) Glutathione peroxidase (GSH-Px); (C) Catalase (CAT); (D) Total antioxidant capacity (T-AOC); (E) Malondialdehyde (MDA). Different lowercase letters above the figure column indicate that there are significant differences between different temperature groups (P< 0.05).
Effect of water temperature during embryonic development on digestive enzyme activity of newly hatched larvae of swimming crab
The digestive enzyme activities differed among the newly hatched larvae of swimming crabs subject to different water temperatures during embryonic development, even under the same rearing conditions. PEP, TPS, LPS, AMS and CL activities all increased and then decreased with increasing water temperature during embryonic development (Figure 7). At 29°C, TPS, LPS and CL exhibited the highest activities, reaching 6529.77 U/mg prot, 2.68 U/mg prot and 76.10 U/mg prot, respectively. Similar values were obtained at 27°C and 31°C. However, once the temperature reached 33°C, TPS, LPS and CL activities declined rapidly with values of only 1767.19 U/mg prot, 1.47 U/mg prot and 37.88 U/mg prot, respectively. In addition, TPS and CL activities at 33°C were significantly lower than those obtained at 27°C, 29°C and 31°C. In addition, the activities of PEP and AMS at 31°C were the highest at 4.33 U/mg prot and 4.95 U/mg prot, respectively. These values decreased significantly at 33°C to 2.30 U/mg prot and 3.01 U/mg prot, respectively.
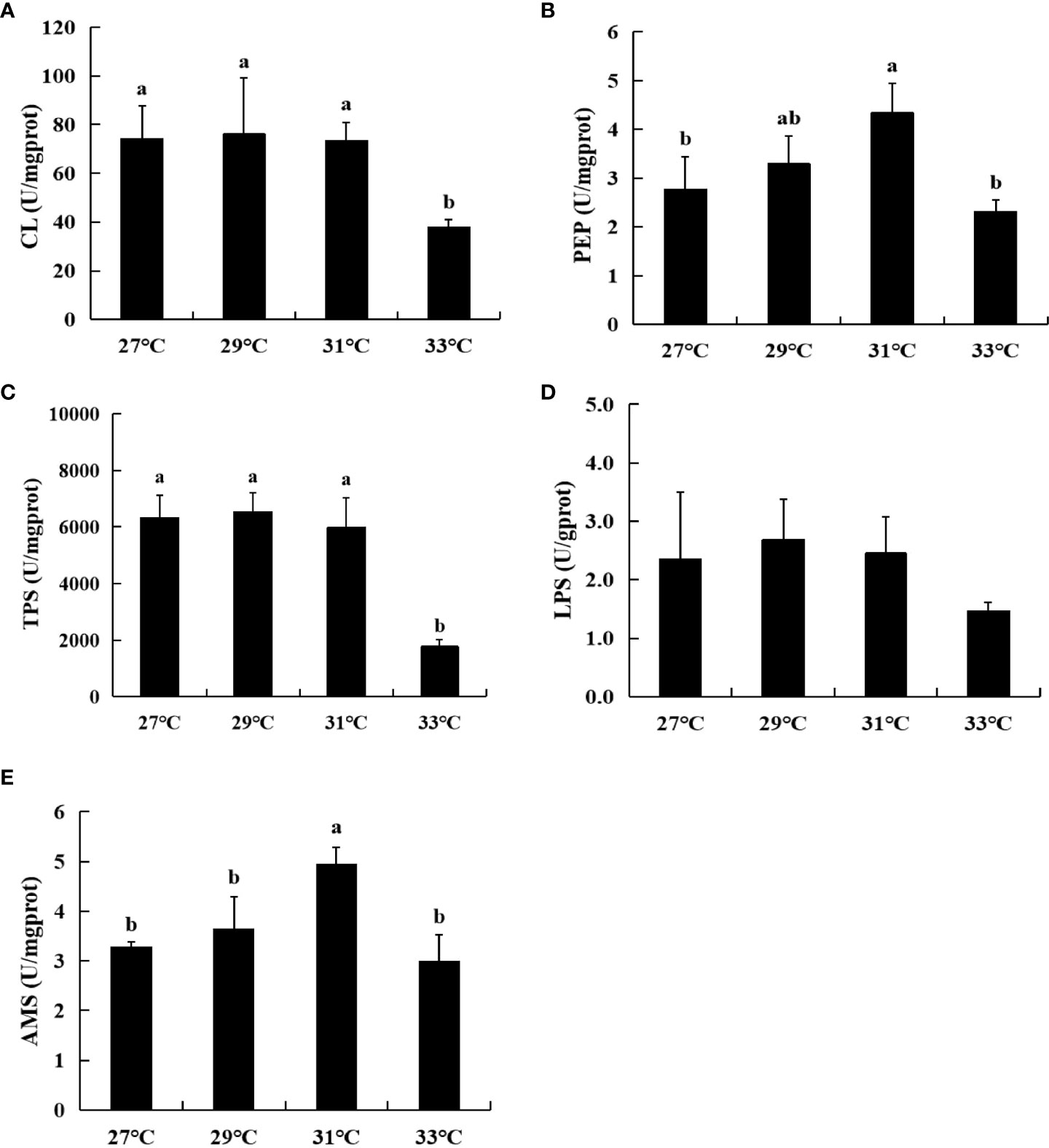
Figure 7 Digestive enzyme activity of newly hatched larvae of swimming crab at four water temperatures during embryonic development. (A) Cellulase (CL); (B) Pepsin (PEP); (C) Trypsin (TPS); (D) Lipase (LPS); (E) α-amylase (AMS). Different lowercase letters above the figure column indicate that there are significant differences between different temperature groups (P< 0.05).
Discussion
The embryonic development of aquatic animals is crucial in the study of evolutionary biology, and the stage of embryonic development in crustaceans, shrimps and crabs is typically classified based on different external morphological features, such as the proportion of yolk during embryonic development, the presence of rudiment, changes in the number of appendages, the pigment degree of compound eyes, and the appearance of heartbeat (Nagao et al., 1999). In our study, based on the method of Yu et al. (2021) in combination with the changes in important external characteristics during the embryonic development of swimming crabs, embryonic development was divided into seven stages, zygote, cleavage, blastocyst, gastrula, nauplius, protozoea, and prehatching stages. The duration of each developmental stage was shorter in autumn, and the whole embryonic development process lasted less than 10 d. In contrast, Xue et al. (2001) reported that the whole embryonic development process in spring took approximately 28 d when the water temperature was 12~19.8°C. It was evident that the high temperature in autumn significantly shortened embryonic development time. Moreover, this study demonstrated that the duration of each development stage was gradually reduced as the water temperature increased from 27 to 33°C. The total development time was 9.43 d at 27°C and only 6.88 d at 33°C.
It has been confirmed in other shrimp and crab species that the protein within the yolk is the main energy source and structural material for embryonic development. As the temperature increased under suitable conditions, the activity of protein degrading enzymes in the embryo increases, which contributes to enhancing the relevant metabolic activities and formation of structural material in the egg. These findings imply that the accelerated rate of yolk consumption promotes the rate of embryonic development (Babu, 1987; Huang et al., 2011; Liu et al., 2021). The concept of effective accumulated temperature represents the total amount of heat accumulated necessary for embryonic development; this constant is referred to as the thermal constant (Ito et al., 1980). In Cynoglossus semilaevis (Du et al., 2004), Pelteobagrus vachelli (Yuan et al., 2005) and Pomoxis nigromaculatus (Zhong, 2014), different embryonic development stages were found to have different sensitivities to the hatching temperature, resulting in deviations in the effective accumulated temperature of embryonic development at different water temperatures. Swimming crabs in this study had very similar effective accumulated temperatures of embryonic development at the four temperatures, which were almost unaffected by water temperature.
Different species of crustaceans exhibit differences in temperature tolerance during embryonic development. Embryos can develop normally at suitable water temperatures. Once the water temperature exceeds the tolerance range, development desynchrony, diapause or even death occur (Ashaf-Ud-Doulah et al., 2021). Related studies found that the embryos of Scylla serrata at cleavage were uneven and irregular at 35 °C water temperature. After 18 hours of cleavage to the 132-cell stage, the cleavage stopped, and the embryos gradually died (Zeng et al., 1991). In Eriocheir sinensis, approximately 50% of the embryo developed asynchrony when cleavage reached the 128-cell stage at a water temperature of 26°C (Zhao et al., 1993). The embryos of Macrobrachium nipponense could not hatch normally at water temperatures greater than 36°C (Xing and Liu, 1996). Procambarus clarkii is grown at water temperatures greater than 28°C, the embryo cannot develop normally, and deformities and even death occur (Liu et al., 2021). The present study had similar findings. At a water temperature of 31°C, the embryos of swimming crabs began to develop asynchronously accompanied by partial embryo mortality. When the water temperature reached 33°C, many embryos died. A related study by Aritaki and Seikai (2004) suggested that this phenomenon may be caused by the inhibition of yolk degrading enzyme activity under high temperature conditions, resulting in changes in the permeability of embryonic cell membranes, disruption in the process of embryonic organ differentiation and formation, and a significant increase in the embryo deformity and diapause rate. Furthermore, the hatching rate of in vitro embryos of swimming crabs was higher (approximately 70% at 27°C and 29°C) and decreased significantly with increasing water temperature (31°C and 33°C), especially the hatching rate, which was only 13.89% at 33°C. On the one hand, massive embryos exhibit diapause and death; on the other hand, excessive water temperature may reduce the activity of hatching enzymes or even cause irreversible inactivation, thereby reducing the embryonic hatching rate.
With the continuous deepening of research, it is recognized that the ability of aquatic organisms to adapt and resist adverse external factors in early development stages is importantly linked to their self-protection systems in metabolic processes. The most important of these systems is the antioxidant system, which maintains the dynamic balance of free radicals in the body (Livingstone, 2001). Numerous studies have revealed that when aquatic animals are exposed to external high- or low-temperature stress, they will produce excessive harmful reactive oxygen species, and the organism will eliminate these reactive oxygen species by producing more antioxidant enzymes to maintain the normal physiological activities of cells and the organism (Gieseg et al., 2000; Martínez-Álvarez et al., 2005). As important antioxidant enzymes, SOD, CAT and GSH-Px play pivotal roles in the self-protection system during embryonic development and represent the key enzymes involved in the intraembryonic antioxidant process (Huang et al., 2010). SOD and GSH-Px activities as well as the T-AOC level of swimming crab embryos were proportional to the water temperature in the range of 27°C to 31°C. It is hypothesized that the increase in water temperature can induce an increase in embryo activity and mobilize SOD, GSH-Px and other related enzymes to resist the stress response generated by high temperature. However, the regulatory capacity of the embryo is limited, and the enzyme activity cannot increase indefinitely. Thus, when the water temperature is too high, the enzymes and their synthesis in the embryo will be altered, and the enzyme activity will be significantly reduced. This phenomenon explains why the SOD and GSH-Px activities decrease significantly at 33°C. CAT activity is the highest at 33°C, which may be that CAT is not sensitive to temperature and has a higher tolerance, or it can activate the activity of CAT only at high temperature.As the final product of the oxidation of unsaturated fatty acids in the organism, the level of MDA indirectly reflects the severity of the condition of cells attacked by free radicals (Parihar et al., 1997). The ability of swimming crab embryos to eliminate harmful free radicals is weakened at high temperatures (31°C and 33°C), leading to increased peroxidation of lipids, such as MDA.
Given that a single index of antioxidant enzyme activity was not able to explain the developmental response of swimming crab embryos under high temperature conditions, this study was conducted to evaluate the quality of embryos by combining various digestive enzymes (CL, PEP, TPS, LPS and AMS) of the newly hatched larvae to better assess the quality of embryonic development at high temperatures. Numerous studies believe that the level of digestive enzyme activity of aquatic poikilothermic animals determines their ability to digest and absorb nutrients, thus determining their growth and development speed. The nutrients required by swimming crabs during embryonic development are directly supplied by endogenous nutrients, whereas the nutrients for the development of newly hatched larvae are provided by residual yolk and gradually change to exogenous nutrients at a later stage. In addition, the differences in the activity of different digestive enzymes can directly reflect the ability of larvae to absorb and utilize different nutrients (Pan and Wang, 1997). In our study, the differences in digestive enzymes of the newly hatched larvae at 27°C, 29°C and 31°C during embryonic development were mainly demonstrated by the increased activities of PEP and AMS at 31°C along with other insignificant differences. The activities of digestive enzymes of the newly hatched larvae at 33°C during embryonic development were relatively low; in particular, the activities of TPS and CL were significantly lower than those of the other three temperature groups, further indicating that the newly hatched larvae at 33°C during embryonic development were of poor quality. The influencing mechanism needs further study.
Conclusion
Overall, the embryonic development of swimming crabs occurs faster and in a shorter timeframe (generally less than 10 d)under higher water temperatures (27~33°C) in autumn. Here, a temperature of approximately 29°C represented a suitable water temperature for embryonic development of swimming crabs in autumn. At this temperature, the embryo antioxidant ability and digestive enzyme activity of newly hatched larvae are increased, and the embryo hatching rate and quality of larvae are excellent. Although the embryo hatching rate is more affected at 31°C, the surviving embryos and newly hatched larvae are still of better quality. The most serious limitation is that at 33°C, asynchronous embryonic development is noted. In addition, an extremely low hatching rate and poor quality of surviving embryos and newly hatched larvae were observed. Therefore, we suggest that under the off-season breeding mode of swimming crab, the water temperature for embryo development should be controlled within 31°C to ensure the quality of embryo development.
Data availability statement
The original contributions presented in the study are included in the article/supplementary material. Further inquiries can be directed to the corresponding author.
Author contributions
JH and LW: Investigation, Formal analysis, Writing -original draft. HY: Conceptualization, Data curation, Formal analysis. YP and DZ: Visualization, Writing - reviewing and editing. WX: Supervision, Funding acquisition, Writing - reviewing and editing. All authors contributed to the article and approved the submitted version.
Funding
This work was supported by the Basic Public Welfare Program of Zhejiang Province (Grant No. LGN22C190005); the National Science Foundation of China (Grant No.32172993); the Major Agricultural Technology Cooperation Plan of Zhejiang Province (Grant No. 2022XTTGSC04); the planned project of Zhejiang Marine Fisheries Research institute (HYSCZ-202105, HYS-CZ-202209).
Conflict of interest
The authors declare that the research was conducted in the absence of any commercial or financial relationships that could be construed as a potential conflict of interest.
Publisher’s note
All claims expressed in this article are solely those of the authors and do not necessarily represent those of their affiliated organizations, or those of the publisher, the editors and the reviewers. Any product that may be evaluated in this article, or claim that may be made by its manufacturer, is not guaranteed or endorsed by the publisher.
References
Aritaki M., Seikai T. (2004). Temperature effects on early development and occurrence of metamorphosis-related morphological abnormalities in hatchery-reared brown sole Pseudopleuronectes herzensteini. Aquac 240 (1-4), 517–530. doi: 10.1016/j.aquaculture.2004.06.033
Ashaf-Ud-Doulah M., Islam S. M. M., Zahangir M. M., Islam M. S., Brown C., Shahjahan M. (2021). Increased water temperature interrupts embryonic and larval development of Indian major carp rohu Labeo rohita. Aquacult Int. 29, 711–722. doi: 10.1007/s10499-021-00649-x
Babu D. E. (1987). Observation on the embryonic development and energy source in the crab Xantho bidentatus. Mar. Biol. 95 (1), 123–127. doi: 10.1007/BF00447493
Du W., Meng Z., Xue Z., Jiang Y., Zhuang Z., Wan R. (2004). Embryonic development of Cynoglossus semilaevis and its relationship with incubation temperature. J. Fisher Sci. China 11 (1), 48–53. doi: 10.3321/j.issn:1005-8737.2004.01.009
Fan Y., Shao P., Jia X., Gao J., Dou Y., Shi X., et al. (2019). Effects of temperature stress on partial antioxidant and non-specific immunity indices of Paramisgurnus dabryanus. Progr Fisher Sci. 40 (2), 58–64. doi: 10.19663/j.issn2095-9869.20180306001
Fan X., Wang C., Zhu D., Cui Z., Song W., Shao Y., et al. (2008). A research on feminization breeding of. Portunus trituberculatus. Mar. Fisher Res. 29 (5), 89–94. doi: 10.3969/j.issn.1000-7075.2008.05.014
Gao B., Liu P., Li J., Wang Q., Han Z. (2015). Effect of inbreeding on growth and genetic diversity of Portunus trituberculatus based on the full-sibling inbreeding families. Aquacult Int. 23 (6), 1401–1410. doi: 10.1007/s10499-015-9892-9
Gieseg S. P., Cuddihy S., Hill J. V., Davison W. (2000). A comparison of plasma vitamin c and e levels in two Antarctic and two temperate water fish species. Comp. Biochem. Phys. B. 125 (3), 371–378. doi: 10.1016/s0305-0491(99)00186-8
Güralp H., Pocherniaieva K., Blecha M., Policar T., Pšenička M., Saito T. (2017). Development, and effect of water temperature on development rate,of pikeperch Sander lucioperca embryos. Theriogenology 104, 94–104. doi: 10.1016/j.theriogenology.2017.07.050
Han T., Li X., Wang J., Wang C., Yang M., Zheng P. (2018). Effects of dietary astaxanthin (AX) supplementation on pigmentation, antioxidant capacity and nutritional value of swimming crab, Portunus trituberculatus. Aquac 490, 169–177. doi: 10.1016/j.aquaculture.2018.02.030
He J., Gao Y., Xu W., Yu F., Su Z., Xuan F. (2017). Effects of different shelters on the molting, growth and culture performance of Portunus trituberculatus. Aquac 481, 133–139. doi: 10.1016/j.aquaculture.2017.08.027
Huang X., Zhang L., Zhuang P., Jiang Q., Yao Z., Liu J., et al. (2010). Effect of cryopreservation on enzyme activity in embryo of macrobrachium rosenbergii. Mar. Fisher 32 (2), 166–171. doi: 10.13233/j.cnki.mar.fish.2010.02.009
Huang X., Zhuang P., Zhang L., Qu L., Yao Z., Liu. T., et al. (2011). Embryonic development and the variation of some metabolism enzyme activity during embryonic development of Chinese mitten crab ( Eriocheir sinensis). J. Fisher China 35 (2), 192–199. doi: 10.3724/SP.J.1231.2011.17130
Ito Y., Hokyo N., Fujisaki K. (1980). Animal population and community (Tokyo: Tokai University Press).
Liu Y., Zhang D., Tao Z., Fu F., Gu Z. (2021). Effects of temperature on embryonic and larval development of Procambarus clarkia. J. Huazhong Agricu Univer 40 (5), 146–153. doi: 10.13300/j.cnki.hnlkxb.2021.05.018
Livingstone D. R. (2001). Contaminant-stimulated reactive oxygen species production and oxidative damage in aquatic organisms. Mar. pollut. Bull. 42 (8), 656–666. doi: 10.1016/s0025-326x(01)00060-1
Martínez-Álvarez R. M., Morales A. E., Sanz A. (2005). Antioxidant defenses in fish: biotic and abiotic factors. Rev. Fish Biol. Fisher 15 (1-2), 75–88. doi: 10.1007/s11160-005-7846-4
Nagao J., Munehara H., Shimazaki K. (1999). Embryonic development of the hair crab Erimacrus isenbeckii. J. Crustacean Biol. 19 (1), 77–83. jstor.org/stable/1549549 doi: 10.2307/1549549
Pan L., Wang K. (1997). Studies on digestive enzyme activities and amino acid in the larvae of Portunus trituberculatus. J. Fisher China 21 (3), 246–251. cnki:sun:sckx.0.1997-03-003
Parihar M. S., Javeri T., Hemnani T., Dubey A. K., Prakash P. (1997). Response of superoxide dismutase, glutathione peroxidase and reduced glutathione antioxidant defenses in gills of the freshwater catfish (Heteropneustes fossilis) to short-term elevated temperature. J. Therm Biol. 22 (2), 151–156. doi: 10.1016/S0306-4565(97)00006-5
Wan X., Shen H., Wang L., Cheng Y. (2011). Isolation and characterization of Vibrio metschnikovii causing infection in farmed Portunus trituberculatus in China. Aquacult Int. 19 (2), 351–359. doi: 10.1007/s10499-011-9422-3
Wu X., Cheng Y., Zeng C., Wang C., Yang X. (2010). Reproductive performance and offspring quality of wild-caught and pond-reared swimming crab Portunus trituberculatus broodstock. Aquac 301, 78–84. doi: 10.1016/j.aquaculture.2010.01.016
Xing K., Liu M. (1996). The effect of temperature on embryonic development of Macrobrachium nipponense. J. Hebei Univer 16 (5), 22–26. cnki:sun:hbdd.0.1996-S1-006
Xue J., Du N., Lai W. (2001). Morphology of egg-larvae of swimming crab (Portunus trituberculatus) during embryonic development. Acta Zool. Sin. 47 (4), 447–452. doi: 10.3969/j.issn.1674-5507.2001.04.016
Yuan L., Xie X., Cao Z., Luo Y. (2005). Effects of temperature on embryonic development of the darkbarbel catfish Pelteobagrus vachelli. Act Zool. Sin. 51 (4), 753–757. doi: 10.3969/j.issn.1674-5507.2005.04.028
Yu H., Xu W., Zhang D., Cheng H., Wu K., He J. (2021). Development of embryos and larvae of Portunus trituberculatus (Decapoda, brachyura) in off-season breeding mode. Crustaceana 94 (6), 679–695. doi: 10.1163/15685403-bja10126
Zeng C., Wang G., Li S. (1991). Observation on embryonic development of Scylla serrata and study on the effect of temperature on embryonic development. Fujian Fisher 1, 45–50. doi: 10.14012/j.cnki.fjsc.1991.01.011
Zhang J., Chi C., Wu C. (2011). Biological zero temperature and effective accumulated temperature for embryonic development of Sepiella maindroni. South China Fisher Sci. 7 (3), 45–49. doi: 10.3969/j.issn.2095-0780·2011.03.008
Zhao Y., Du N., Lai W. (1993). Effects of different gradient temperature on embryonic development of the Chinese mitten-handed crab Eriocheir sinensis (Crustacea, decapoda). Zool Res. 14 (1), 49–53. doi: cnki:sun:sckx.0.1997-03-003
Keywords: Protunus trituberculatus, off-season breeding, water temperature, embryonic development, enzyme activity
Citation: He J, Wan L, Yu H, Peng Y, Zhang D and Xu W (2022) Effect of water temperature on embryonic development of Protunus trituberculatus in an off-season breeding mode. Front. Mar. Sci. 9:1066151. doi: 10.3389/fmars.2022.1066151
Received: 10 October 2022; Accepted: 01 November 2022;
Published: 16 November 2022.
Edited by:
Zhiguo Dong, Jiangsu Ocean University, ChinaReviewed by:
Rantao Zuo, Dalian Ocean University, ChinaIoannis A. Giantsis, University of Western Macedonia, Greece
Copyright © 2022 He, Wan, Yu, Peng, Zhang and Xu. This is an open-access article distributed under the terms of the Creative Commons Attribution License (CC BY). The use, distribution or reproduction in other forums is permitted, provided the original author(s) and the copyright owner(s) are credited and that the original publication in this journal is cited, in accordance with accepted academic practice. No use, distribution or reproduction is permitted which does not comply with these terms.
*Correspondence: Wenjun Xu, wjxu1971@hotmail.com
†These authors have contributed equally to this work