- 1Stock Assessment Division, National Research Institute of Fisheries Science, Yokohama, Japan
- 2Fisheries Technology Institute, Japan Fisheries Research and Education Agency, Hakatajima Filed Station, Imabari, Japan
- 3Laboratory of Marine Biology, Department of Bioresource Sciences, Faculty of Agriculture, Kyushu University, Fukuoka, Japan
- 4Marine Fisheries Research and Development Center, Japan Fisheries Research and Education Agency, Yokohama, Japan
- 5Fisheries Technology Institute, Japan Fisheries Research and Education Agency, Nagasaki, Japan
- 6Fisheries Resources Institute, Japan Fisheries Research and Education Agency, Hatsukaichi, Japan
- 7Fisheries Resources Institute, Japan Fisheries Research and Education Agency, Shiogama, Japan
- 8Settlement Resource Division, Ibaraki Prefectural Fisheries Research Institute, Hitachinaka, Japan
- 9Fisheries Resources Institute, Japan Fisheries Research and Education Agency, Yokohama, Japan
Maternal effects on offspring viability significantly contribute to the productivity and resilience of exploited marine fish populations. The temporal trend of biomass of chub mackerel (Scomber japonicus) in the western North Pacific showed cyclic fluctuation patterns, but it has been rapidly increasing since 2011. Although there are several assumptions regarding the mechanisms of the population dynamics of this species in these waters, there is a contradiction in the effects of thermal environmental conditions on recruitment success. We explored the effects of maternal age and/or previous spawning experience on egg and offspring traits of captive chub mackerel in response to thermal conditions. Moreover, from the relationship between the growth rate and temperature experienced by captive fish, the experienced temperatures of wild specimens were assessed. The sizes of eggs spawned from females aged 1–3 years were negatively correlated with temperature, but the effects of maternal age on egg size and nutrition were significant with larger and nutrient-rich eggs spawned from 2- or 3-year-old females (repeat spawners) than those from 1-year-old females (first-time spawners) at a given temperature. Such temperature- and maternal-age-dependent egg traits reflected the standard length of first-feeding larvae. The effects of maternal age on the starvation tolerance of larvae after hatching and body sizes of first-feeding larvae were also evident in larvae derived from 3-year-old females showing longer starvation tolerance and larger body heights than those from 1-year-old females. Further, larvae derived from 3-year-old females grew significantly faster than those from 1-year-old females at the tested temperatures. The process of ontogenetic development and growth rate of captive specimens until age 25 days were positively correlated with temperature. Meanwhile, the estimated temperatures experienced by wild specimens until age 25 days ranged from 18.0 to 20.3°C. These findings suggested that there were opposing thermal responses between maternal and offspring traits of chub mackerel, but the temperatures experienced by the main recruit fish may be restricted within the range of temperatures available for spawning. We discuss the mechanisms of reproductive success of this species in accordance with the maternal spawning experience and thermal effects on life history traits.
1. Introduction
Understanding the population dynamics of exploited marine fish is a key objective for improving sustainable management schemes. There is a growing awareness that maternal effects on offspring viability represent a significant component of the productivity and resilience of populations (Marshall et al., 2008; Green, 2008; Hixon et al., 2014; Ohlberger et al., 2022). Body size, age, and/or spawning experience of individual females could affect the survival of offspring in the early life stages through variations in the quantity and quality of eggs produced and the onset timing and duration of spawning. Large, old repeat spawners (fish with previous spawning experience) generally allocate more energy for egg production than small, young first-time spawners (fish during their first year of spawning) by increasing relative fecundity (total number of eggs produced per body weight) and egg size (Barneche et al., 2018a). Egg size is an important surrogate measure of maternal investment indicating quality and linked to several fundamental and adaptive traits of the offspring (Chambers, 1997). Larvae hatched from larger eggs generally are larger and grow faster than those hatched from smaller eggs. Such traits could confer survival advantages under paradigms of recruitment variability such as “bigger is better” and “growth-selective mortality” (Houde, 2008). Meanwhile, old, large repeat spawners generally spawn earlier and longer than young, small first-time spawners (Wright and Trippel, 2009; Hixon et al., 2014). This bet-hedging strategy could be advantageous for the survival of their larvae because the timing of occurrence of prey and larvae may be unpredictable in each spawning season in terms of the match/mismatch hypothesis (Cushing, 1990; Houde, 2008).
Maternal environment can also represent an integral source of maternal effects as the environment can act directly on reproductive investment, which then acts to influence offspring traits (Green, 2008). Temperature is a major environmental factor influencing the life-history traits of individual fish. In multiple-spawning fish, egg size exhibits temperature-related variation, with larger eggs produced in cooler water. This phenomenon may be because of a direct physiological response in which the temperature experienced by a female during oogenesis influences the size of the eggs produced (Chambers, 1997). Moreover, higher temperatures and ad libitum food availability facilitate faster growth of larval and juvenile fish under favorable thermal conditions in each life stage (Pörtner and Peck, 2010). Thus, the thermal environment can have profound effects on the life history traits of adult and juvenile fish (McBride et al., 2015).
Chub mackerel (Scomber japonicus) in the western North Pacific migrates widely from subtropical to subarctic regions, and the biomass of this species shows cyclic fluctuations under climate variability similar to those of Japanese sardines and Japanese anchovies (Takasuka et al., 2008a; Yatsu, 2019). The biomass of the Pacific stock of chub mackerel was high during the 1970s (Figure 1), but thereafter it rapidly declined and remained low until 2011, probably because of the influence of intense fishing pressures and occurrence of successive lower levels of recruit fish biomass (Kawai et al., 2002; Yatsu et al., 2005; Yukami et al., 2021). After 2011, the total biomass of chub mackerel rapidly increased along with frequent occurrences of higher levels of recruit fish biomass. During this period, the biomass of older spawning fish (age ≥ 4 years) and their proportion to the total spawning stock biomass substantially increased because of the marked decrease in fishing efforts in the waters off eastern Japan, which was also influenced by the Great East Japan Earthquake (Yatsu, 2019; Yukami et al., 2021). Thus, the total biomass of the Pacific stock of chub mackerel in recent years was historically the highest, but the mechanisms involved in the substantial increase in the levels of recruit fish biomass remain unclear.
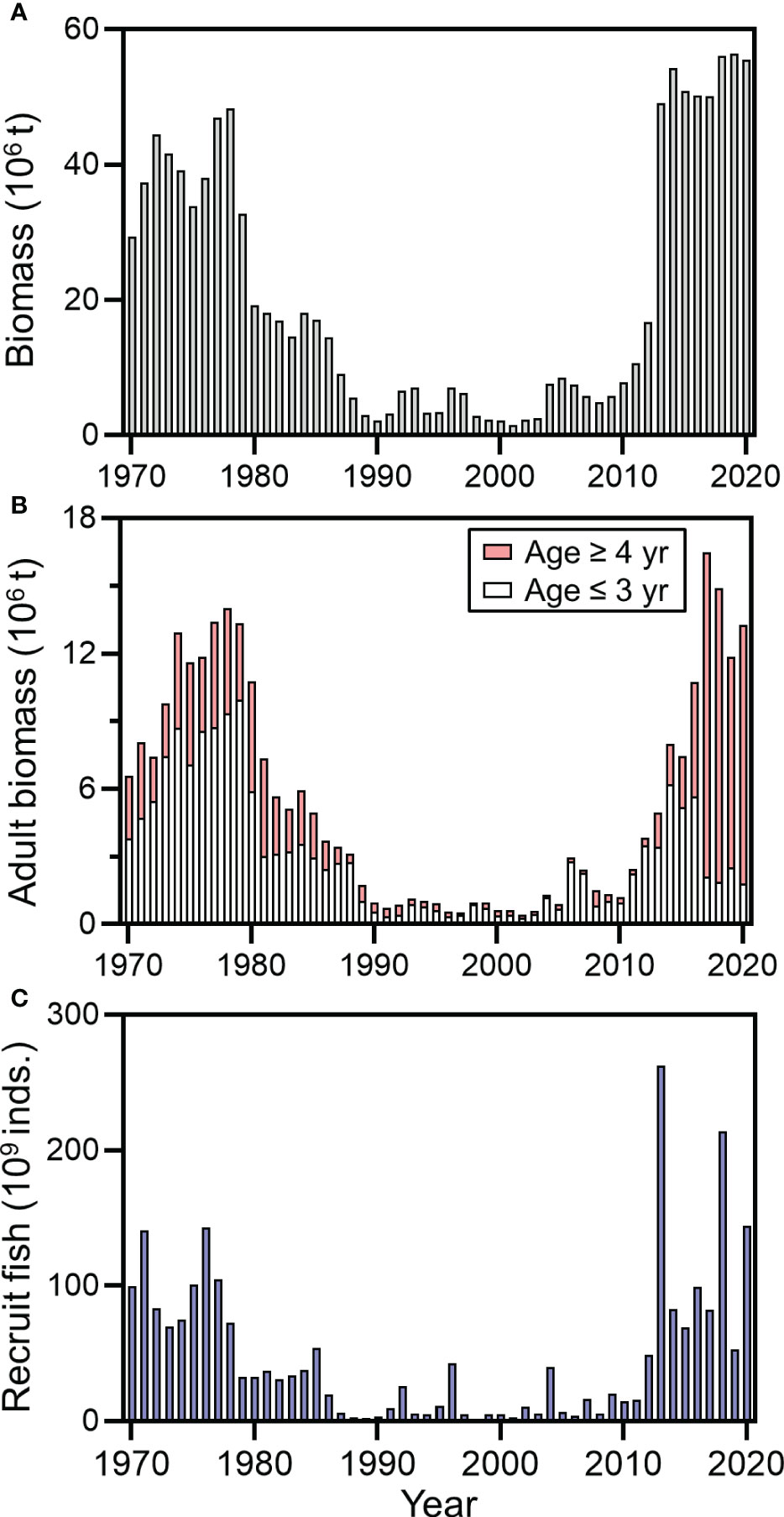
Figure 1 Total biomass (A), adult biomass (B), and number of recruit fish (C) of the Pacific stock of chub mackerel during 1970–2020 (after Yukami et al. (2021)). Adult biomass at age ≤3 and ≥4 years are also shown (B). Most females of age ≥4 years were considered repeat spawners that had spawned at least once in their lifetime.
There are some assumptions regarding the mechanisms of recruitment dynamics of the Pacific stock of chub mackerel, but they show contradictions in the effects of thermal environmental conditions on recruitment success. Field surveys have indicated a positive correlation between larval growth and temperature in nursery grounds (Figure 2), suggesting that higher temperatures facilitate faster growth and subsequently better survival in the early life stages (Kamimura et al., 2015; Taga et al., 2019). Conversely, the fork length of age-0 chub mackerel was negatively correlated with sea surface temperature in the nursery and feeding grounds (Kuroshio–Oyashio transition area) during April–June, suggesting that lower temperatures influenced by Oyashio (cold and nutrient-rich current) extension in spring may lead to better prey availability for larvae and juvenile chub mackerel (Watanabe and Yatsu, 2004). The recruitment-stock production model of this stock indicated that recruitment per spawning stock biomass was negatively correlated with winter sea surface temperature in the main spawning ground (Izu Islands area, eastern Japan) (Yatsu et al., 2005). The relationships between recruitment and oceanographic features suggest that there were opposite effects of temperature on spawners (negative correlation) and larvae (positive correlation). For spawners, lower temperatures before spawning may lead to more favorable feeding conditions for adult fish, resulting in accelerated maturation and possibly enhanced egg quality (Kaneko et al., 2019). Otolith stable oxygen isotope ratios and their growth analyses suggest that larvae hatched in the early spawning season (cooler water) grow faster than those hatched in the later season (Higuchi et al., 2019). Inter- and intra-annual changes in the gonad index of adult fish and abundance of egg production suggest that larger and older fish appear to start spawning earlier than smaller and younger ones, particularly first-time spawners, as the onset of maturation is delayed in the first-time spawners (Watanabe, 2010; Kanamori et al., 2019). These reports indicate the necessity to clarify the interactions between maternal effects and thermal responses on offspring viability to elucidate the reproductive success of the Pacific chub mackerel stock.
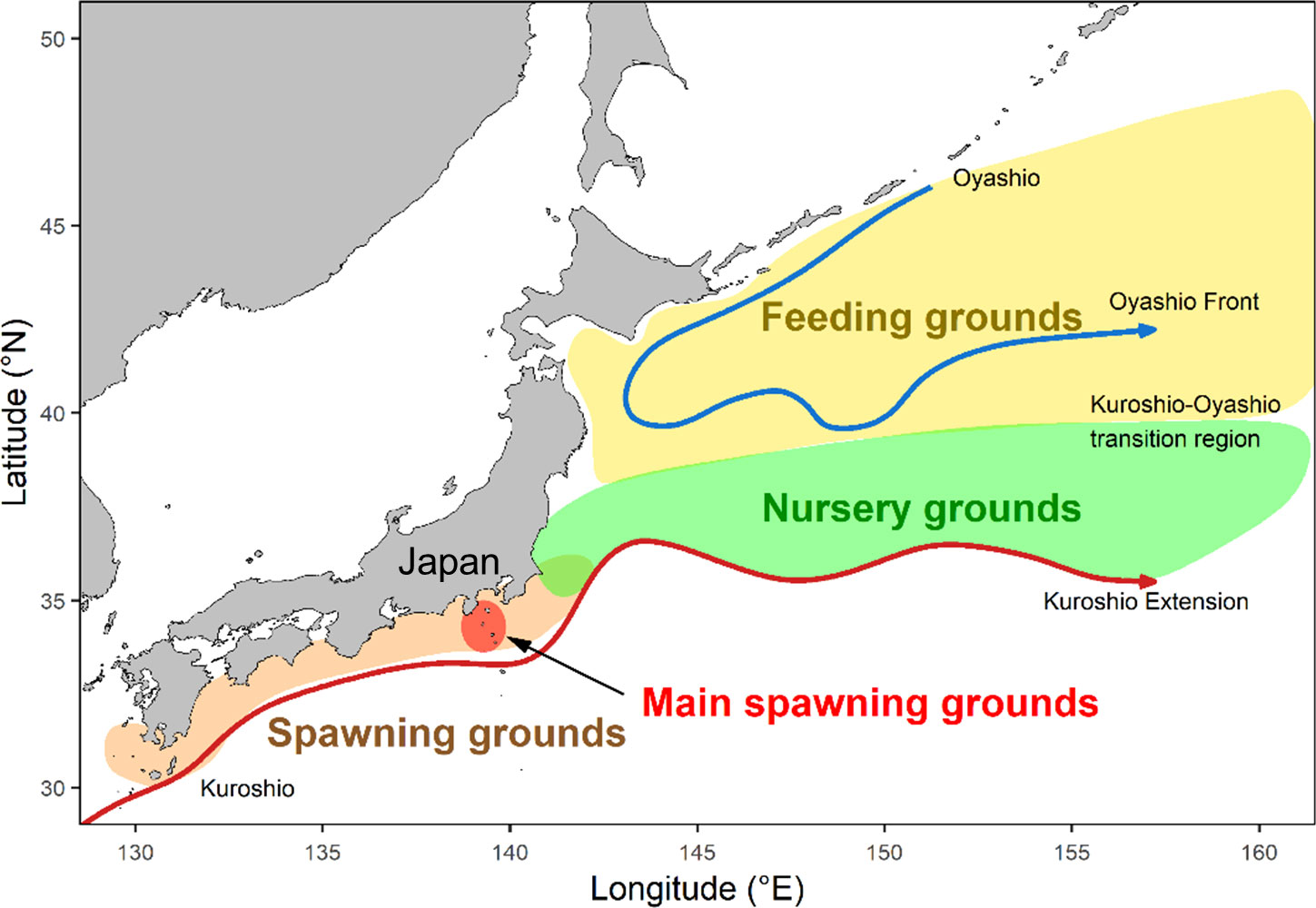
Figure 2 Map presenting the life-history stage-specific habitats of the Pacific stock of chub mackerel and major oceanographic features in the western North Pacific (after Yatsu (2019)). The main spawning grounds are located in the waters around Izu Islands, western Japan. The Kuroshio current contains warm water while the Oyashio current contains subarctic and highly productive water. The nursery and feeding grounds are in the Kuroshio-Oyashio transition region, consisting of a mixture of water from these two currents.
In this study, we explored the effects of maternal age and spawning experience (first-time spawners versus repeat spawners) on the egg and larval traits of captive chub mackerel in response to thermal conditions. To evaluate the maternal effects on larval viability, we focused on egg size and nutrition as indicators of the egg quality of chub mackerel. Under captive conditions (ad libitum feeding availability), chub mackerel can attain sexual maturation at the age of one year (fork length: ~25 cm and body weight: 300 g) and were considered first-time spawners. Further, fish aged 2 and 3 years, which have spawned earlier in their lives, were considered repeat spawners. First, the effects of maternal age and temperature on the sizes of eggs and larvae of adult females of different age groups were examined. Second, the effects of maternal age (spawning experience) on the starvation tolerance and growth rate of larval fish were examined at different temperatures. Third, the effects of temperature on ontogenetic development and growth rate on the early life stages were examined under different temperature regimes. Based on the relationship between growth rate and temperature, the temperatures experienced by specimens collected from the Kuroshio–Oyashio transition areas (Kamimura et al., 2015) were also estimated. Finally, the mechanisms of reproductive success of the Pacific stock of chub mackerel are discussed in accordance with maternal and thermal effects on spawning and early life history traits.
2. Materials and methods
2.1. Fish rearing and induction of spawning
Experiments were conducted at the Fishery Research Laboratory of Kyushu University (KU) during 2009–2011 and Hakatajima Field Station of the National Research Institute of Fisheries and Environment of the Inland Sea (NRIFEIS) during 2016–2019 (Supplementary Table S1). Chub mackerel were caught from the wild using purse seine or fixed net in coastal waters off western Japan and reared for 0.5–2.5 years in sea pens at a fish farm in Oita Prefecture, western Japan. A total of 216 specimens were transferred to KU or NRIFEIS and moved into 3–50 kL concrete tanks circulated with seawater. Fish were assimilated and reared under natural photoperiod (10–14 h) and temperature (11–17°C) for 1–6 months before the onset of experiment. For experiments conducted at KU, all specimens (n = 83) were from fish farms, whereas for those at NRIFEIS, in addition to specimens from fish farms (n = 133), a total of 211 artificial seedlings at age 1–3 years were used to examine the effects of maternal age on egg and larval traits. The water temperature in the tank was monitored every hour using a data logger (Tidbit V2; Onset, MA, USA). Fish were daily fed with 1–1.5% of their body weight of commercial dry pellets (Marubeni Nisshin Feed Co., Ltd., Tokyo, Japan; Otohime EP 6: protein 52%, oil 11%, ash 18%, fiber 3%).
Spawning was induced based on a sustained-release GnRH delivery system protocol adopted from Shiraishi et al. (2008) and Nyuji et al. (2011; 2012). A total of 427 specimens were anesthetized with 2-phenoxyethanol (300 ppm). Of these, females with late vitellogenic oocytes (600–650 μm diameter), which were determined using measurement of oocyte diameter and clearing solution method (Yoneda et al., 2013), were selected after ovarian biopsy using a plastic catheter tube (Internal diameter: 2 mm). Males that oozed milt under gentle abdominal pressure were selected. After selecting the required number of females and males, females were intramuscularly injected with 400 μg/kg body weight GnRH agonist (D-Ala6, des-Gly10)-LHRH ethylamide (Sigma-Aldrich, St. Louis, USA) combined with coconut oil. The first spawning was observed 34–36 h post-injection, and subsequent daily spawning occurred between 22.00 and 24.00 h. In the following experimental system, daily spawning of chub mackerel was observed for 12–28 d after the injection. A total of 83 specimens (age 1 year, n = 20; age 2 years, n = 33; age 3 years, n = 30) were used for examination of the egg size–temperature relationship under ambient temperature while a total of 344 specimens (age 1 year, n = 139; age 2 years, n = 75; age 3 years, n = 130) were used for examination of the egg size–number of spawning events relationship at a constant temperature (19°C).
Adult specimens randomly selected (n = 312) were measured to within 0.1 mm of fork length (FL) and within 0.1 g of body weight (BW) before and after the experiments. Of these, a total of 209 specimens were measured to within 0.1g gonadal weight (GW) after the experiments. Fulton’s condition factor (K) is the morphometric condition factor of fish species representing the mass of an individual relative to its body length (Ricker, 1975). K was calculated using the following equation:
where SW (somatic weight, g) was calculated as BW – GW. From the R package of lme4 (Bates et al., 2015), linear mixed effects models (LMEM) with FL, BW, and condition factor as fixed effects and experimental trials as random effect indicated that FL and BW of 1-year-old specimens were significantly smaller than those of 2- and 3-year-old specimens (Supplementary Figure S1, P< 0.01), but no significant difference in CF among the three age groups was found (P = 0.08).
2.2. Effects of maternal age and temperature on egg traits
To examine the effect of temperature and spawning events on egg size, 1-, 2-, and 3-year-old adult females were reared at ambient water temperature of 19°C (range: 18.5–19.5°C) after the GnRHa treatment. During the experiments, fish were daily fed with 1–1.5% of their body weight of commercial dry pellets. Egg diameter was measured every spawning day using Image-Pro Plus ver. 7.01 (Media Cybernetics, Inc., Bethesda, MD, USA) after fixing in 10% neutral sea water formaldehyde. Some eggs were frozen for analysis of the energy content. Egg size was determined using the following equation:
where r is egg radius (mm). To ensure the effects on egg size, the experiments were conducted twice or thrice in different years of spawning, including specimens from the first to third spawning seasons. A total of 2790 eggs (age 1 year, n = 600, age 2 years, n = 900, age 3 years, n = 1290) were used for analysis of the egg size-temperature relationship while a total of 5040 eggs (age 1 year, n = 2010, age 2 years, n = 1110, age 3 years, n = 1920) were used for analysis of the egg size-number of spawning events relationships.
To determine the energy content of the eggs to be spawned, the concentrations of C and N per egg were determined in the eggs spawned between May and July 2019. Eggs were thawed at room temperature (18–22°C) and rinsed with distilled water; thereafter, a total of 200 eggs were pooled for each egg sample to ensure adequate numbers for the analysis and dried at 60°C in a micro-tube for 3–4 days. A total of 138 egg samples (age 1 year, n = 66; age 3 years, n = 72) were used for this analysis. The dried samples were ground to a fine powder and placed in tin capsules. Lipids were retained to assess the total energy in the eggs. The total C and N were analyzed using an elemental analyzer (Flash EA1112, Thermo Fisher Scientific Co., Ltd. MA, USA). The concentration of C or N in an egg was estimated by dividing the total amount of C or N by the total number of eggs used.
2.3. Effects of temperature and maternal age on larval traits
To examine the effect of maternal age and temperature on larval size, approximately 100 eggs to be spawned from 1- and 3-year-old adult females at 18, 20, and 22°C were placed in 30 L tank at identical spawning temperatures in duplicates. The timing of larval hatch of this species varied at different temperature regimes (Hunter and Kimbrell, 1980). Larvae were collected on the day of first feeding (age: 3 days at 20 and 22°C and 4 days at 18°C) and stored in 10% neutral sea water formaldehyde. The timing of first feeding was determined as that after >50% of larvae started feeding. Standard length (SL), eye diameter, body depth at anus, head height, and pectoral body depth (Supplementary Figure S2) were measured using Image-Pro Plus.To determine the effect of maternal age and temperature on starvation tolerance in early life stage, approximately 800 eggs spawned from 1- and 3-year-old females at constant temperature (19°C) were placed in 30 L tank at 20°C until hatching. A total of 30–80 hatchlings were transferred into 1 L glass beakers at three temperature treatments (18, 20, and 22°C) in duplicates. The number of dead larvae in each glass beaker was counted at intervals of 7–14 h, and the experiment was terminated when all the larvae were dead. To determine the effect on starvation tolerance, this experiment was performed twice in different years of spawning (Supplementary Table S1).
To examine the effects of maternal age and temperature on early growth rates, approximately 5000 eggs spawned from 1- and 3-year-old adult females at constant temperature (19°C) were transferred to 1 kL tanks with three temperature treatments in duplicates (target temperature: 17, 20, and 23°C). A 14/10-h light/dark cycle was maintained. The water temperature in the tanks was monitored hourly using a data logger. After the eyes of the larvae were pigmented, S-type rotifer (Brachionus plicatilis) complexes cultured with highly unsaturated fatty acid-enriched Chlorella vulgaris (Super Chlorella V12; Chlorella Industry Co., Ltd., Fukuoka, Japan) were provided at 20 rotifers per mL in 1 kL tanks from age 2 or 3 days. Rotifer density was monitored daily and maintained by adding new rotifers when necessary. Kaneko et al. (2019) suggested that the thermal environment experienced during the first 10 days after spawning may significantly influence the recruitment success of chub mackerel in the western North Pacific. Thus, the experiment was terminated at 10 d of age. After anesthetizing at first feeding with 2-phenoxyethanol (300 mg/l), the larvae were preserved in 10% neutral sea water formaldehyde. Daily growth rate (G1) was calculated as follows:
The mean SL at first feeding was calculated from the specimens at 3 or 4 days of age in each tank.
2.4. Effects of temperature on development and growth in early life stages
Approximately 2500 and 5000 eggs spawned from 2- and 3-year-old females were transferred into 0.5 and 1 kL tanks, respectively, at different temperature regimes ranging from 17 to 23°C, in which a total of 11 trials were conducted. The experiment was terminated when the larvae were 25-day-old because it was the approximate duration associated with recruitment success of chub mackerel. Approximately 20-day-old larvae of 20 mm FL appear to start migrating to the Kuroshio–Oyahshio transition area (Kamimura et al., 2015); individuals with high initial growth until 27 days of age appear to proactively enter the cooler nutrient-rich area for further rapid growth (Higuchi et al., 2019). The methods of rearing fish in the early stages are mentioned above. From age 14–25 days Artemia sp. nauplii enriched with highly unsaturated fatty acids (Super Capsule Powder, Chlorella Industry Co., Ltd.) were provided at 0.2–1.5 Artemia mL−1 day−1. Specimens were collected at the first feeding (age 3 or 4 days) and at the end of the experiment (age 25 days) to estimate the daily growth rate of individuals and determine developmental stages. Daily growth rate (G2) was calculated as follows:
The mean SL at the first feeding was calculated from the specimens in each tank. Specimens at 25 days of age were classified into three stages: larva (SL< 12 mm), juvenile (SL: 12–30 mm), and post-juvenile (SL ≤ 30 mm), according to Watanabe (1970) and Taga et al. (2019); in the post-juvenile stage, individuals usually start swimming in schools. Data on the daily growth rates of chub mackerel collected from the Kuroshio Extension to the Kuroshio–Oyashio transition areas during 2002–2011 (Kamimura et al., 2015) were used to estimate the experienced temperature until the age of 25 days. These specimens were considered the main recruit fish in each sampling year, as their growth rates in the April cohort, most of their specimens, explained significant variations in the abundance of recruitment and recruitment per spawning stock biomass.
2.5. Statistical analysis
The effects of temperature, number of eggs spawned, and maternal age on egg size were analyzed using the LMEM with restricted maximum likelihood estimation. During the spawning cycle, vitellogenesis is usually completed on the previous day of spawning, and subsequently, oocyte maturation and ovulation proceed on the day of spawning (Nyuji et al., 2011; Nyuji et al., 2012). Thus, to examine the effect of temperature on egg size, the average temperatures on the day before and on the day of spawning were used. The models included temperature or the number of eggs spawned and maternal age as fixed effects and experimental trials as random effect.
Maternal age was initially a 3-level factor representing year of age (age 1, 2 or 3 years). The significance of the fixed effects was tested using the R package of lmerTest, Satterthwaite approximation (Kuznetsova et al., 2017). The model of each factor with and without natural logarithmic transformation was selected based on the minimum conditional Akaike information criterion using the R package of cAIC (Vaida and Blanchard, 2005) in addition to the selection of grouping of maternal age classes. To determine whether egg size was more strongly influenced by temperature or the number of spawning events, chi-square test was performed to analyze deviance. The amounts of C and N per egg were compared between eggs spawned from 1- and 3-year-old females using LMEM, including sampling days as random effect.
Maternal age was a 2-level factor representing year of age (age 1 or 3 years). The effects of maternal age and temperature on the SL of first-feeding larvae were examined using LMEM with maternal age (2-level factor as abovementioned) and temperature treatment (3-level factor; 18, 20 or 22°C treatment) as fixed effects and tank as a random effect.
To examine the effect of maternal age on the starvation tolerance of hatched larval fish, a generalized LMEM incorporating binominal response distribution with a logit link function was performed using the R package of glmmML (Broström and Holmberg, 2011). The full models combined maternal age (2-level factor as abovementioned), time after hatching and temperature treatment (3-level factor as abovementioned) as fixed effects and the tank as random effect.
The effects of maternal age on the relationship between body parts and SL of first-feeding larvae were examined using LMEM with SL and maternal age (2-level factor as abovementioned) as fixed effects and tank and temperature treatment as random effects.
The effects of maternal age and temperature on SL-days after hatching relationship andG1were examined using LMEM, with maternal age (2-level factor as abovementioned) and temperature treatment (3-level factor; low, middle or high temperature treatment) as fixed effects and tank as a random effect.
The model of each factor, with and without natural logarithmic transformation, was selected based on the minimum conditional Akaike information criterion. All model fittings were conducted using backward elimination.
The effects of temperature and ontogenetic developmental stage on G2 were examined using LMEM. The full model combined temperature and developmental stage (3-level factor; larval, juvenile or post juvenile stage) as fixed effects and trial as random effect.
Model selection was performed as described above. Statistical analyses were performed using R version 4.0.3 (R Core Team, 2021) and GraphPad Prism 8 (GraphPad Software, LLC, CA, USA).
3. Results
3.1. Effect of maternal age on egg size with respect to temperature
A negative correlation was observed between temperature and size of eggs of adult females of all age groups reared in ambient water temperature (Table 1 and Figure 3A). However, there was a significant difference in the regressions of the egg size–temperature relationships between eggs of 1-year-old and 2- and 3-year-old females. Size of eggs spawned from 1-year-old females was approximately 79% of that from females aged 2–3 years at the tested temperatures. At ambient temperature, significant effects of the number of spawning events and maternal age on egg size was observed; however, egg size was more influenced by temperature (slope = -0.026) than the number of spawning events (slope = -0.004; χ2 = 31.28, P< 0.001). At constant water temperature (19°C), there was a negative correlation between egg size and the number of spawning events (Figure 3B), and the egg sizes spawned by 1-year-old females were significantly smaller than those of 2–3-year-old. During the spawning events observed, the estimated slope (-0.002) of the regression model at a constant temperature was approximately 8.2% of the relevant model (-0.026) in water at ambient temperature.
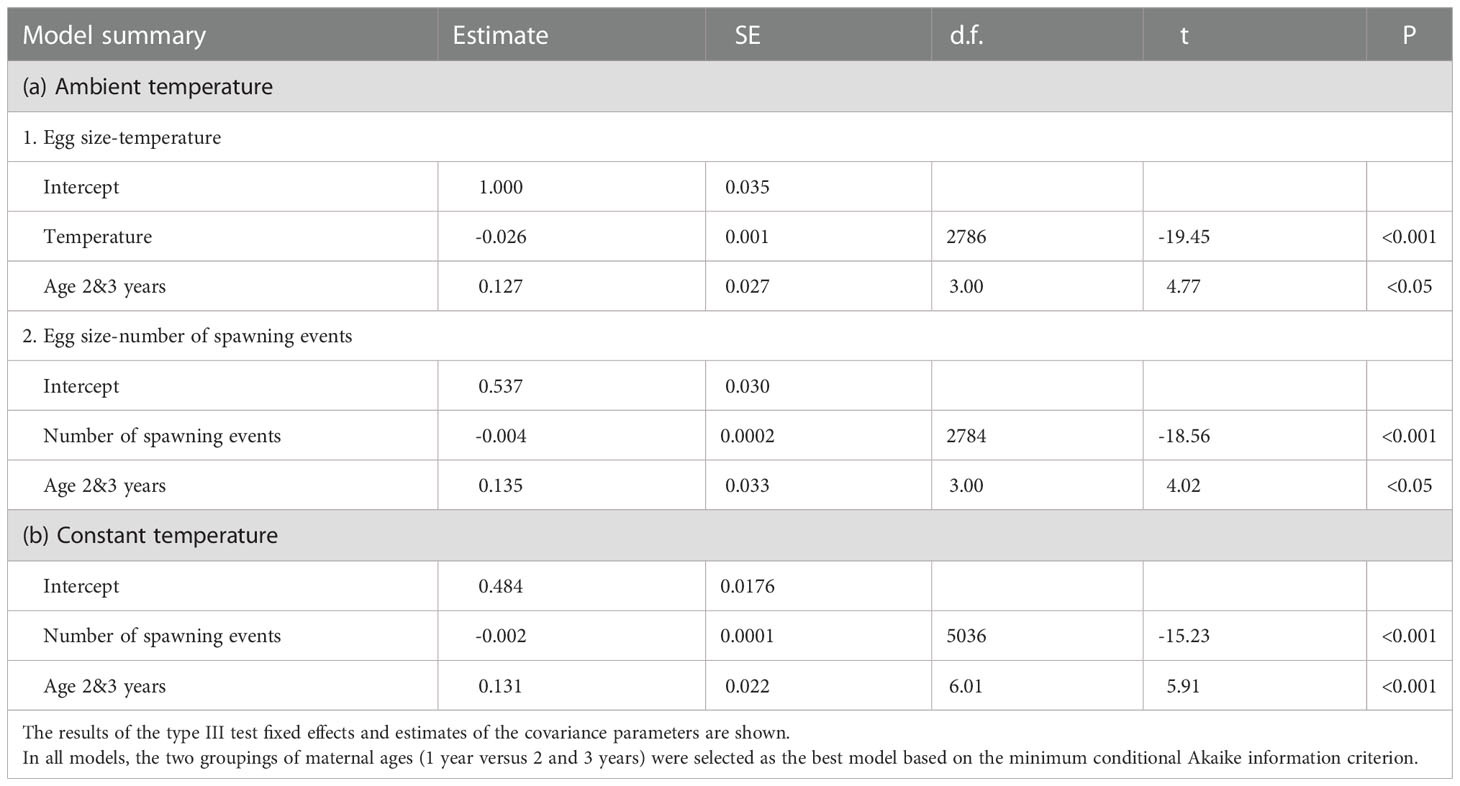
Table 1 Summary table for linear mixed-effects model estimates fitted using restricted maximum likelihood for the effect of temperature, number of spawning events, and maternal age (ages: 1 year and 2 and 3 years) on egg size (mm3).
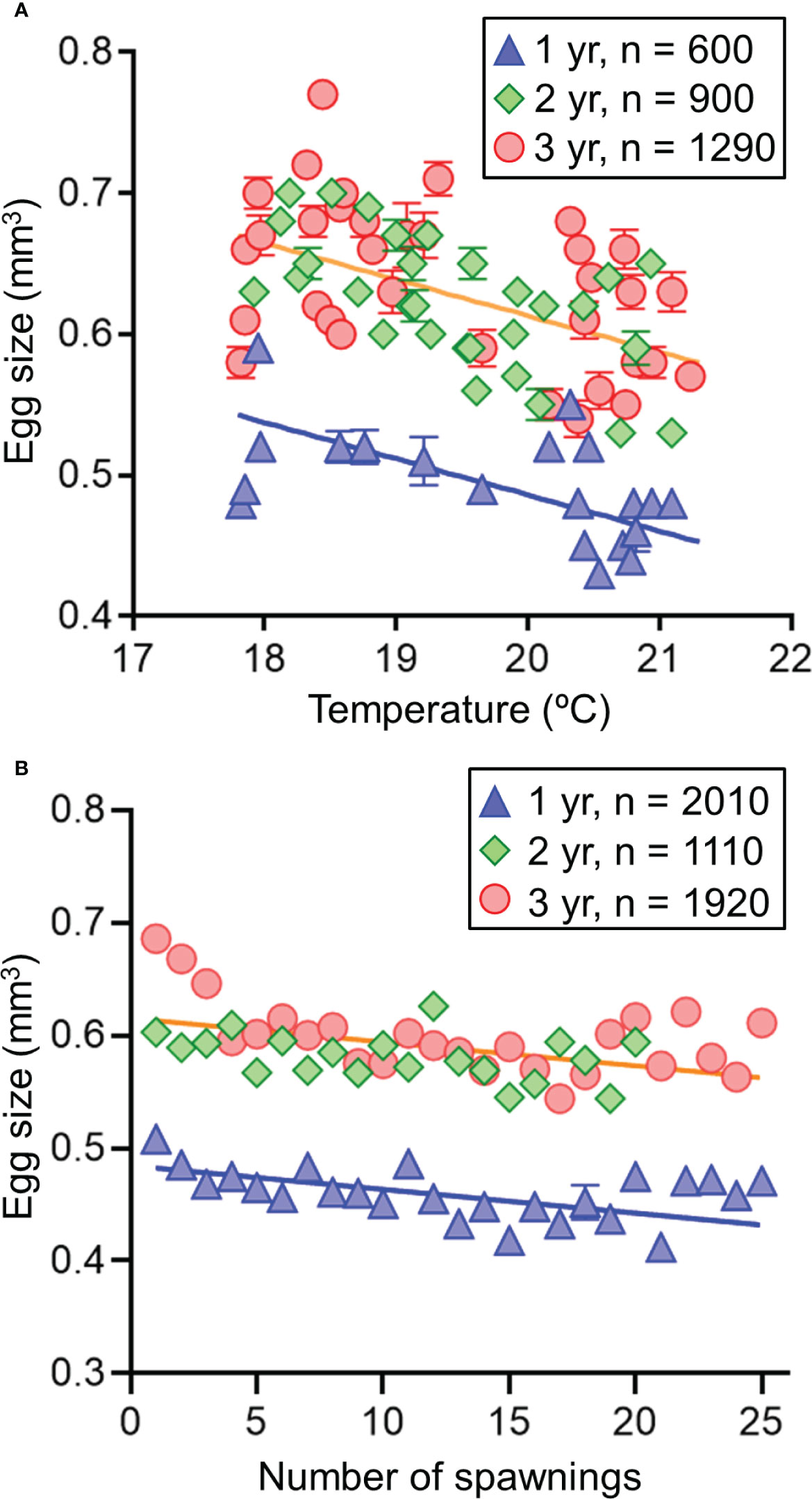
Figure 3 Relationships of egg volume (A) with ambient temperature (B) and the number of spawning events at constant temperature (19°C) of 1-, 2-, and 3-year-old chub mackerels. Predicted lines using the linear mixed effect models fitted to observed data are shown (blue line: age 1 year; orange line: age 2 and 3 years). Data are shown as mean ± SE.
There were significant differences in the amounts of C and N per egg between 1-year-old female eggs and 3-year-old female eggs under constant temperature conditions with respect to differences in egg size, but no significant difference in the C/N ratios per egg was observed between the two age classes (Table 2 and Figure 4). LMEM showed that the estimated egg size, amount of C in eggs, and amount of N in eggs of 1-year-old females (0.44 mm3, 15.34 µg, and 3.12 µg) was approximately 78, 76, and 75% of those of 3-year-old females (0.57mm3, 20.27 µg, and 4.16 µg), respectively. LMEM showed that the estimated C/N ratio per egg in the two female age classes was 4.89.
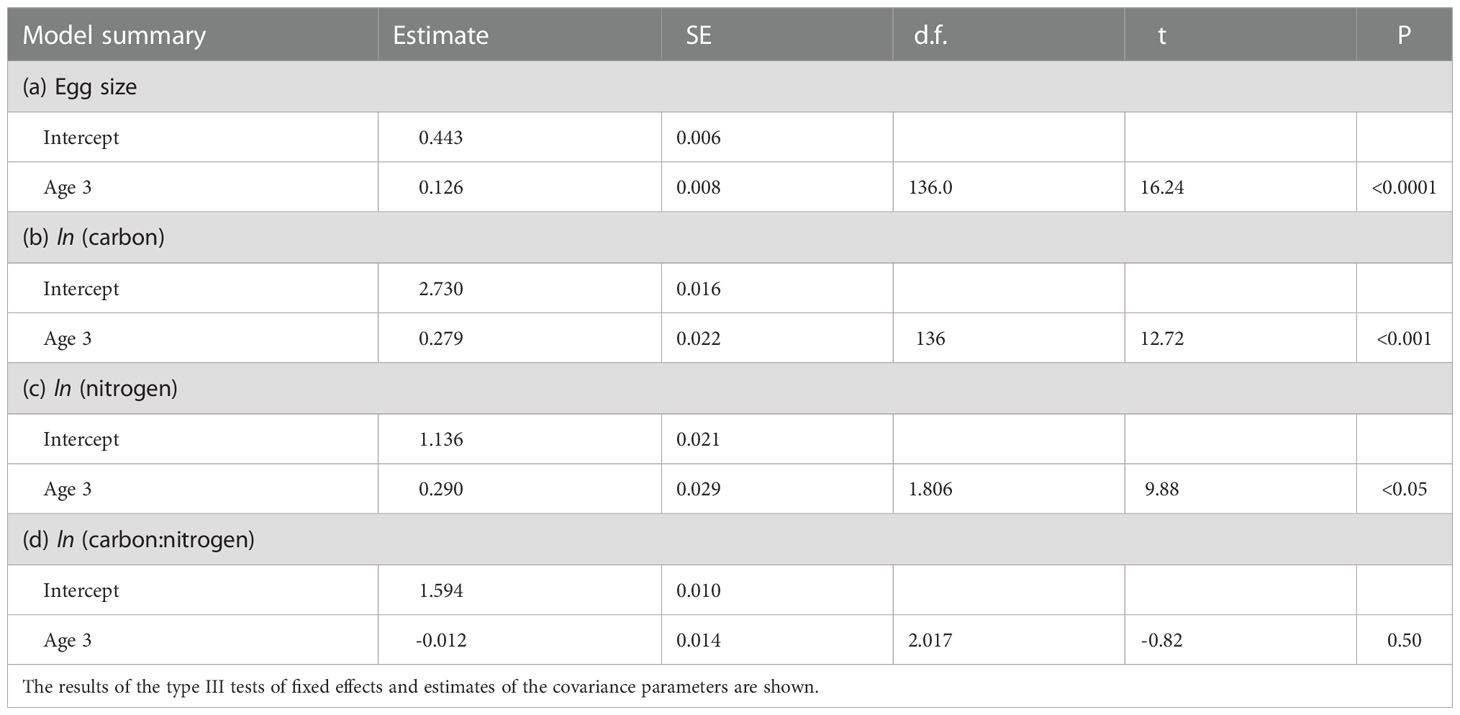
Table 2 Summary table for linear mixed effects model estimates fitted using restricted maximum likelihood for the size (mm3), amounts of carbon and nitrogen, and carbon/nitrogen ratio of eggs of 1-year-old and 3-year-old females.
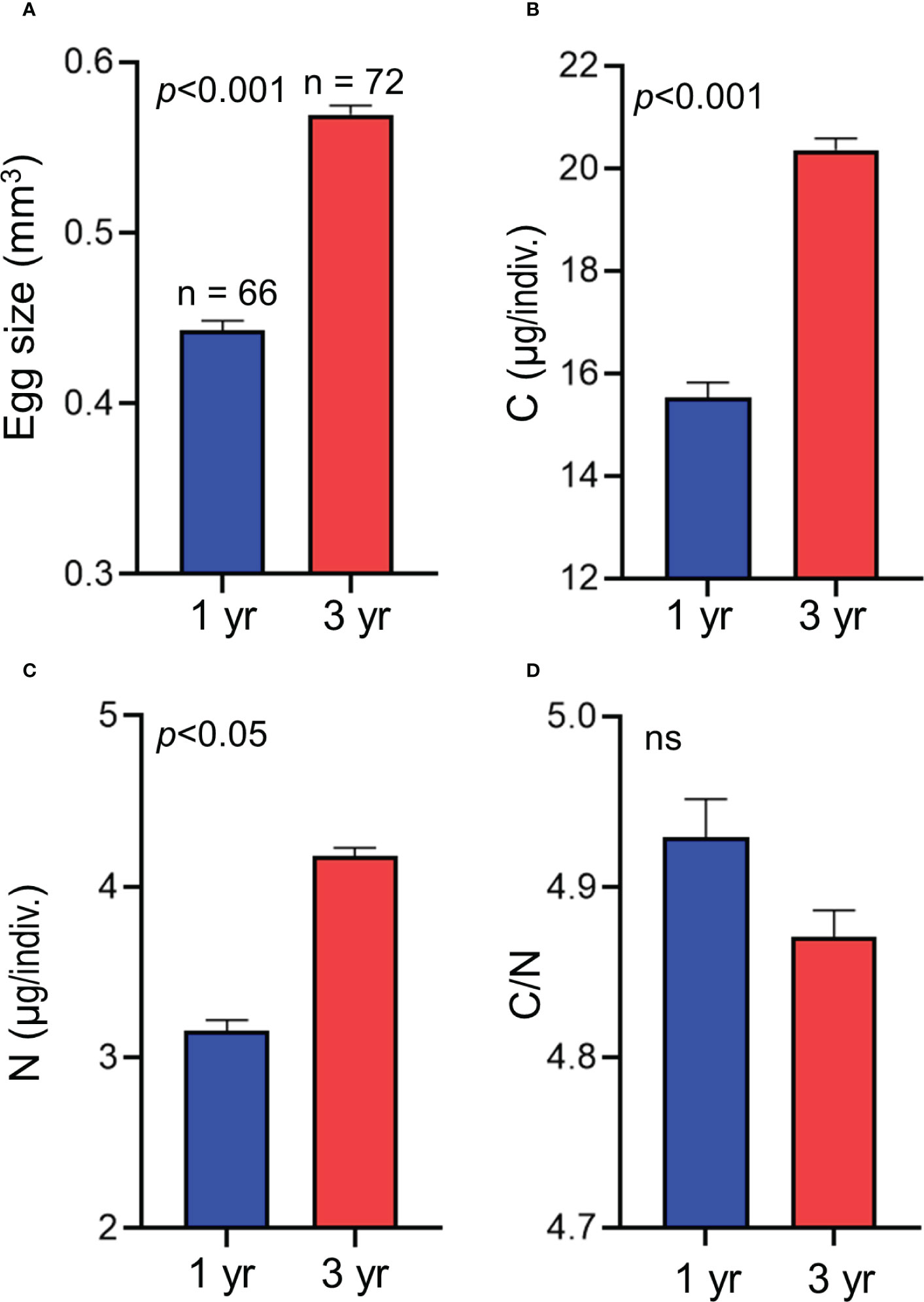
Figure 4 Mean size (A), amounts of carbon (B) and nitrogen (C), and carbon/nitrogen ratio (D) of each egg laid by 1- and 3-year-old chub mackerels at constant temperature (19°C). Result of comparison of value between the two age classes using linear mixed effect model is also shown. Numbers indicate the sample size. Data are shown as mean ± SE.
3.2. Effect of maternal age on larval traits with respect to temperature
There were clear effects of maternal age and spawning temperature on the SL of the larvae at the onset of feeding (Table 3). Temperature negatively affected the SL of first-feeding larvae, but the SL of larvae derived from 3-year-old females was larger at the tested temperatures than that of 1-year-old females. The mouth width, head height, eye diameter, and body depth at anus of the first-feeding larvae were positively correlated with SL, but there was no significant correlation between pectoral body depth and SL (Table 4 and Figure 5). The effects of maternal age on the relationship of SL with body depth at anus and pectoral body depth were significant, indicating that the body sizes of first-feeding larvae derived from 3-year-old females were larger than those from 1-year-old females.
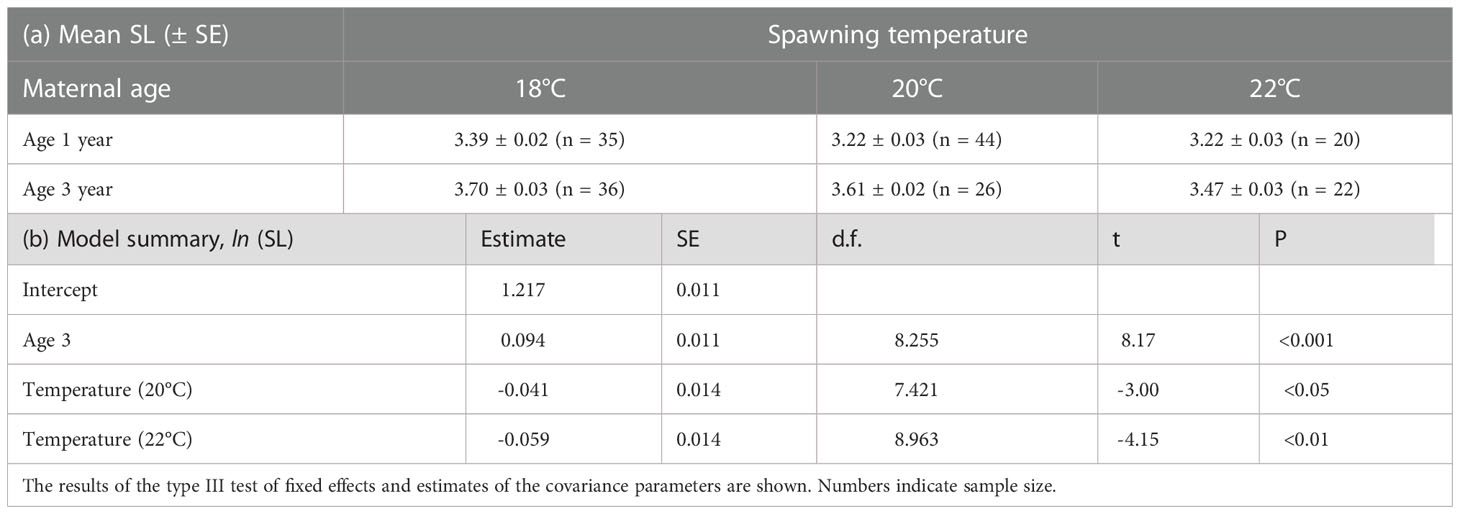
Table 3 Mean standard length (± SE) of first-feeding larvae spawned by 1-year-old and 3-year-old females at different temperatures and summary table for linear mixed effects model fitted using restricted maximum likelihood for the effect of maternal age on SL of first-feeding larvae of chub mackerel.
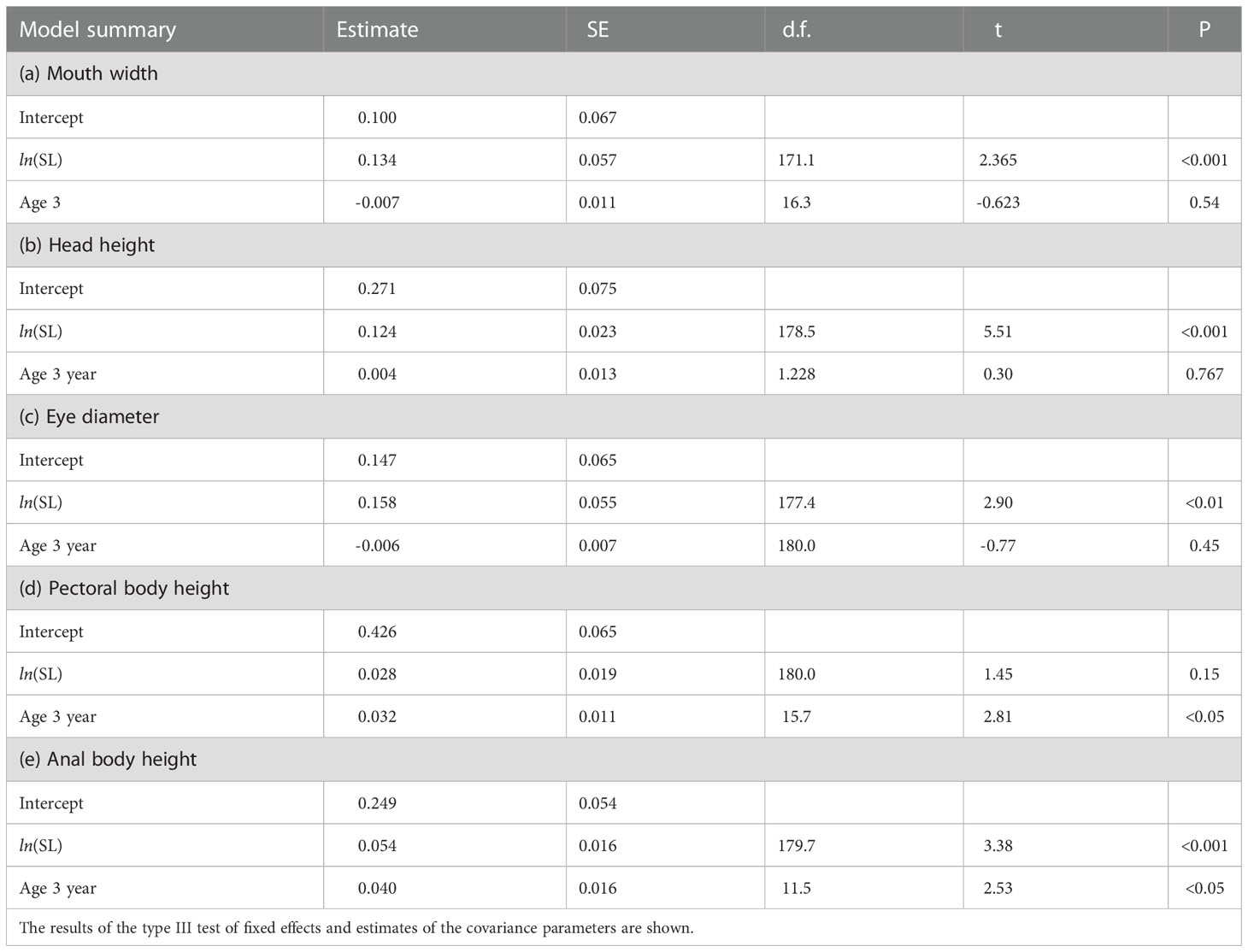
Table 4 Summary table for linear mixed effects model estimates fitted using restricted maximum likelihood for the effect of maternal age (1 and 3 years) on the relative growth of body parts in relation to the SL of first-feeding larvae of chub mackerel.
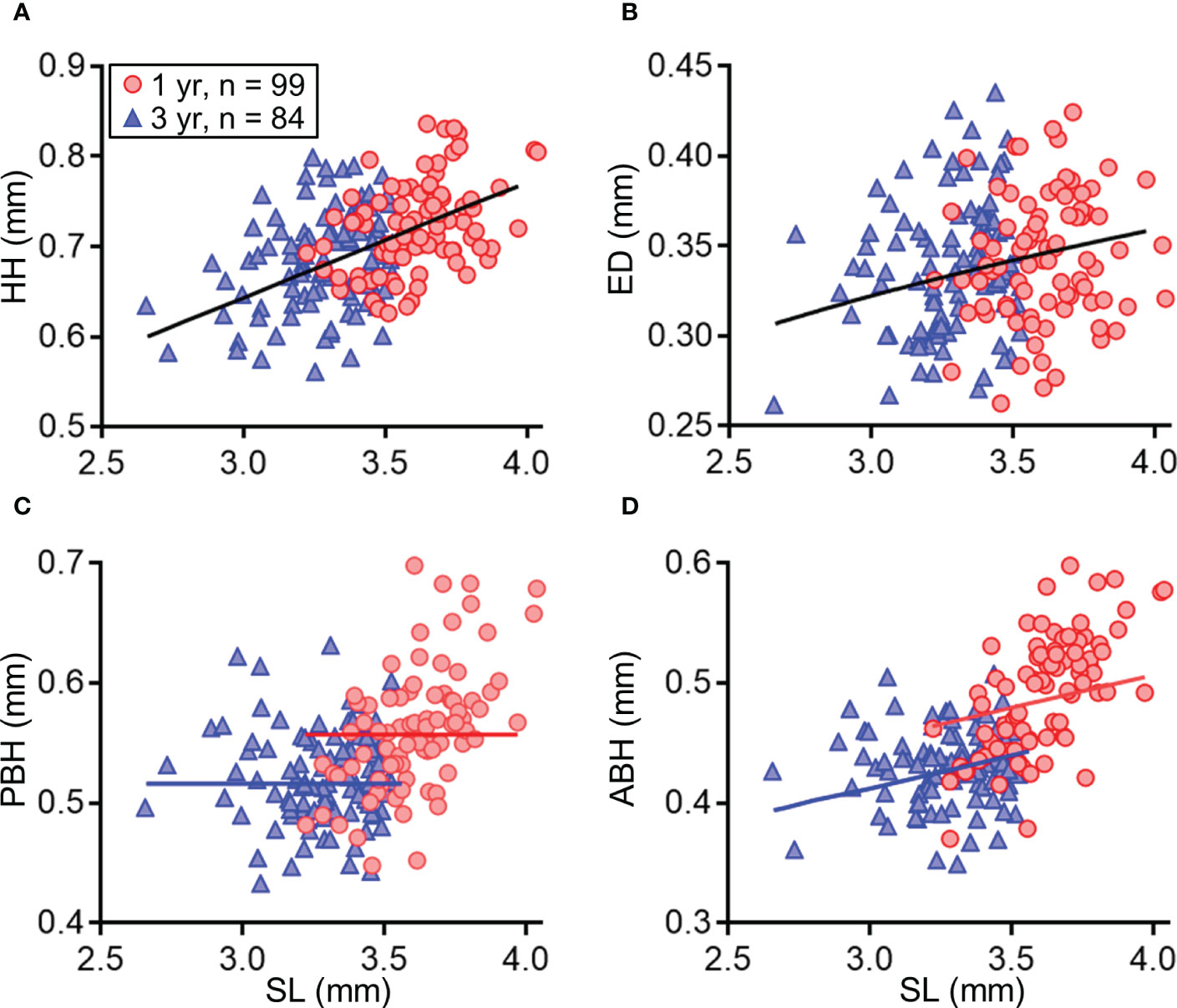
Figure 5 Morphological measurements of first-feeding larvae of chub mackerel (A) and the effects of maternal age (1 or 3 years) on the relationships between body parts and standard length (SL; A–D). Predicted lines using the linear mixed effect model fitted to the observed data on head height (HH, A), eye diameter (ED, B), pectoral body depth (PBH, C) and body depth at anus (ABH, D) are shown in each maternal age class (black line: age 1 and 2 years; blue line: age 1 year; red line: age 3 years). Numbers indicate sample size.
There were clear effects of maternal age and temperature on the survival rate of larvae under starvation from hatching (Table 5 and Figure 6). Temperature negatively affected the time to survival, but the time to survival of 50% larvae derived from 3-year-old females was significantly longer at the tested temperatures than those from 1-year-old females.
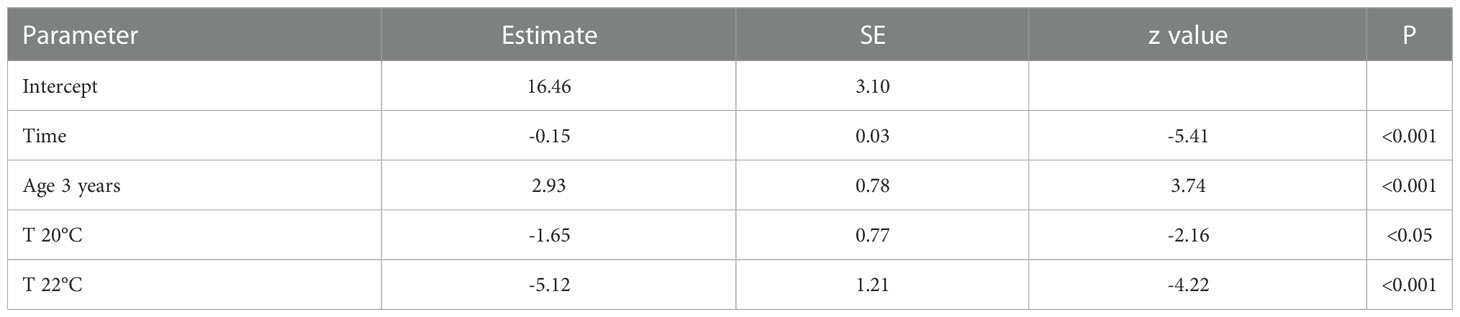
Table 5 Summary table for generalized linear mixed effects model of the effect of duration after hatch (time), maternal age (1 and 3 years), and temperature (18, 20, and 22°C) on survival rate during starvation of chub mackerel larvae.
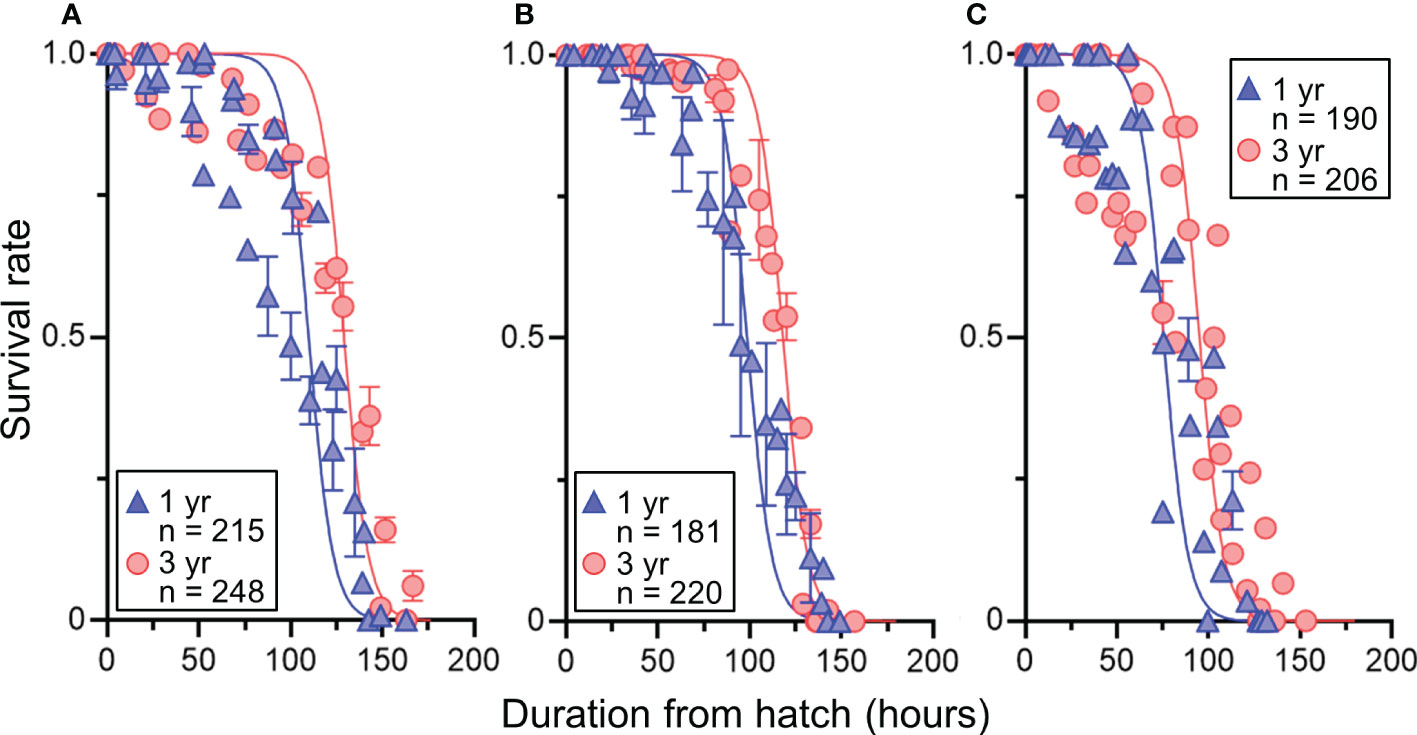
Figure 6 Effects of maternal age (1 or 3 years) on the relationship between survival rate and duration from hatching of chub mackerel larvae at different temperature treatments (A = 18°C, B = 20°C, C = 22°C). Predicted lines using the generalized mixed effect models fitted to the observed data are shown. Data are shown as mean ± SE. Numbers indicate sample sizes.
3.3. Effect of maternal age and temperature on early growth
During the period of 0–10 days after hatching, the SL of larvae derived from 3-year-old females was significantly higher than those from 1-year-old females at three different temperature treatments (Table 6 and Figure S3). The estimated SL of larvae at age 10 days derived from 1-year-old females were approximately 85% of those from 3-year-old females in low, middle, and high temperature treatments. The effects of maternal age and temperature on growth rate were obvious (Table 6 and Figure 7). LMEM indicated that the estimated growth rates of larvae derived from 3-year-old females in low, middle, and high temperature treatments were 55%, 31%, and 20% higher than those from 1-year-old females, respectively.
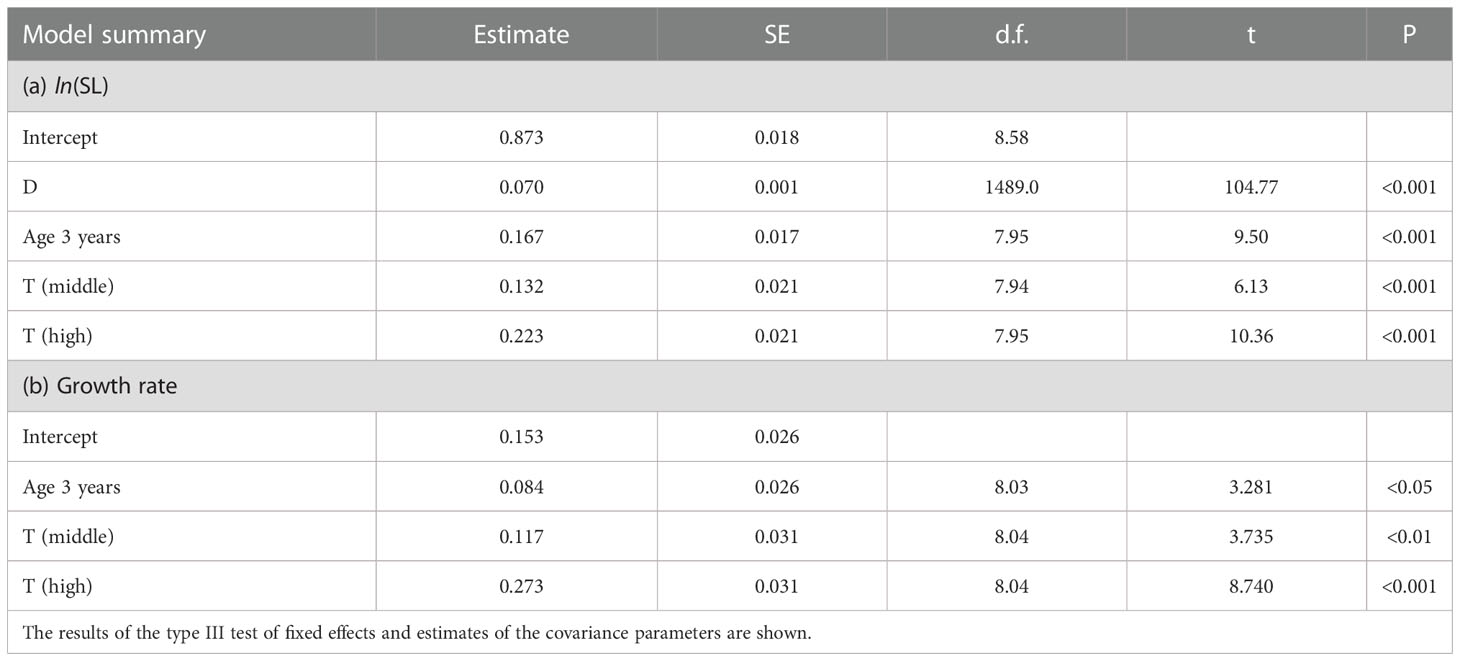
Table 6 Summary table for linear mixed effects model estimates fitted using restricted maximum likelihood for the effect of maternal age (1 versus 3 years) and temperature (low, middle, and high temperature treatments) on the SL–days after hatching (D) relationship and growth rate of chub mackerel larvae by 10 days post-hatching.
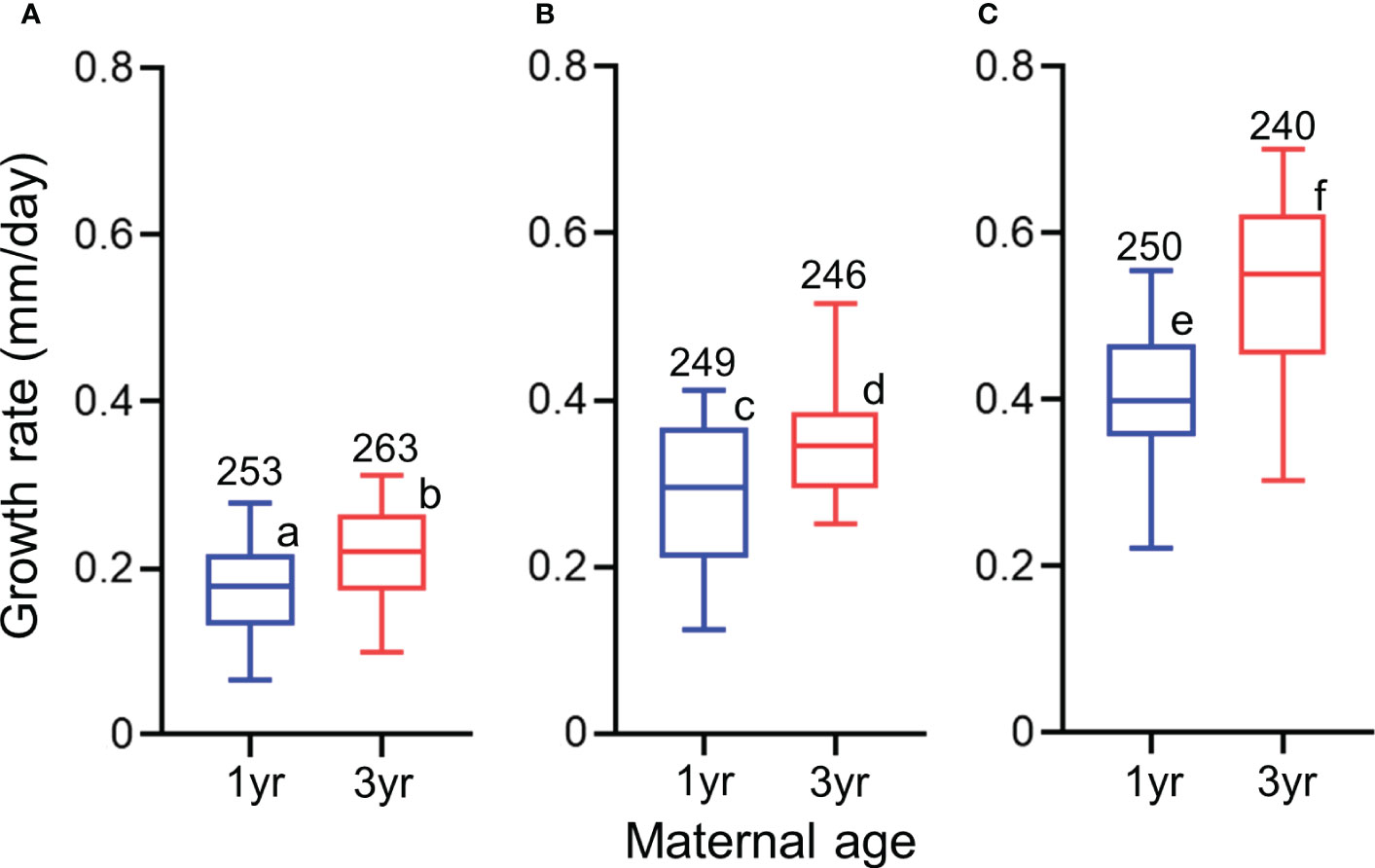
Figure 7 Effect of maternal age (1 or 3 years) on the growth rate of 10-day-old chub mackerel in different temperature treatments: (A) = low temperature (16.6°C), (B) = medium temperature (20.6°C), and (C) = high temperature (22.3°C). The whiskers represent 5th and 95th percentiles of the data. Numbers indicate sample size Different letters indicate different age groups in the linear mixed effect model (a< b< c< d< e< f; P< 0.05).
The effect of temperature on ontogenetic development at 25 days of age was obvious (Figure 8A). In water at 17.0°C, all 25-day-old specimens were in the larval stage, while juvenile fish were observed in water at 17.2°C. Specimens in post-juvenile stage appeared in water at 18.0°C, and all stages were found in water above 22°C. The developmental stage and temperature significantly affected the daily growth rate in the 25-day-old specimens (Table 7 and Figure 8B). The range of estimated daily growth rate at larval, juvenile, and post-juvenile stages were 0.18–0.24 mm/day (17.0–17.2°C), 0.47–1.29 mm/day (17.2–20.3°C), and 1.08–2.47 mm/day (18.0–23.2°C), respectively. In 25-day-old wild fish, the estimated developmental stage was juvenile (0.63) or post-juvenile (0.37), and none of the fish were at the larval stage. The 5th and 95th percentiles of growth rate of 25-day-old wild fish (n = 520) were 0.79 and 1.71 mm/day, respectively. The estimated range of temperature at the juvenile stage, covering the range of growth rates of wild fish, was from 18.4 to 20.3°C, while that at post-juvenile stage was from 18.0 to 20.3°C.
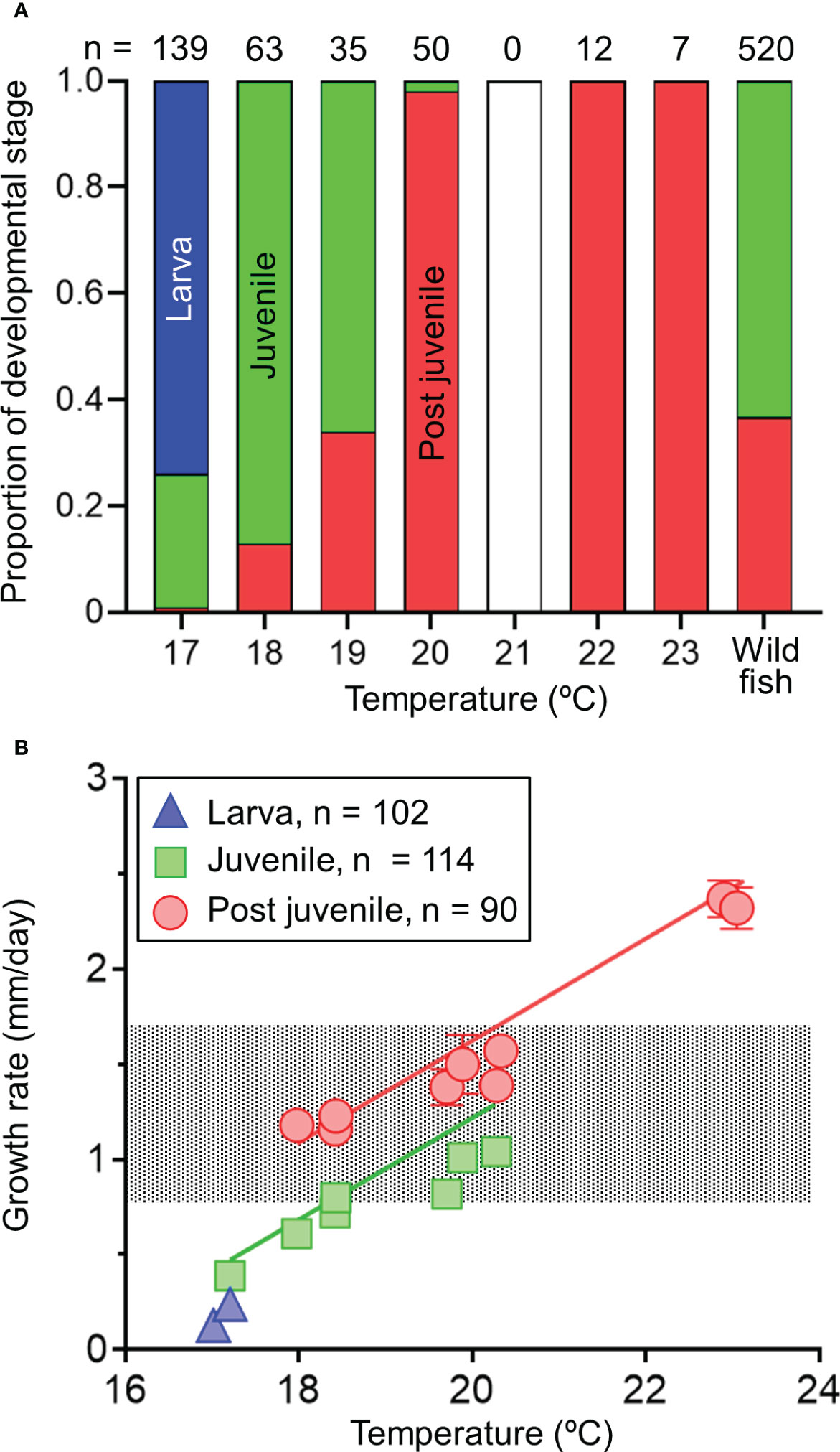
Figure 8 Relationships between the proportion of developmental stage and temperature (A) of 25-day-old chub mackerels and growth rate (mm/day) and temperature (B). Specimens were classified into larval (blue), juvenile (green), and post juvenile (red) stages. Predicted lines using the linear mixed effect models fitted to the observed data in different stages (straight lines) and the range of 5–95 percentiles of growth rates of wild fish collected in 2002–2011 (dotted area) are shown (B). Data are shown as mean ± SE (B). The proportion of developmental stage in the wild fish estimated from the growth rate until age 25 days is also shown (A). Numbers indicate sample sizes.
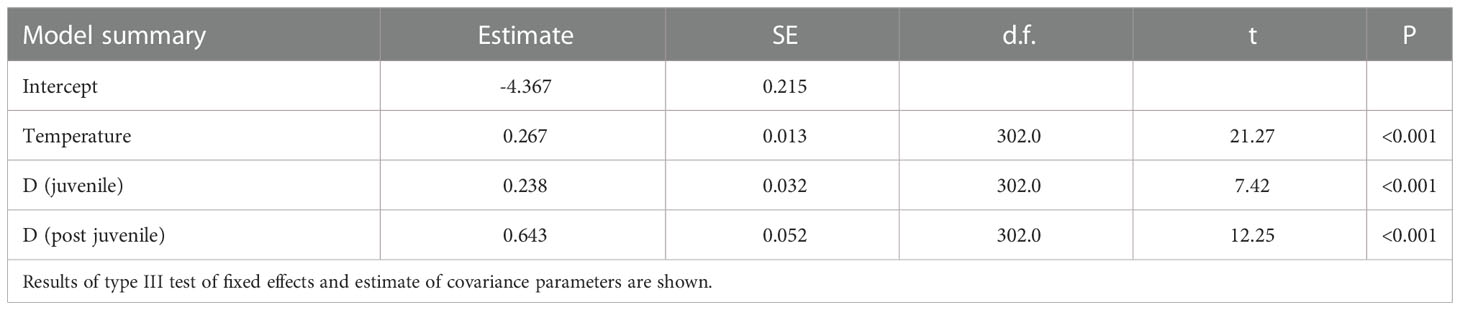
Table 7 Summary table for linear mixed effects model estimates fitted using restricted maximum likelihood for the effect of temperature and developmental stage (D, larva, juvenile, post-juvenile) on growth rate of chub mackerel by 25 days post-hatch.
4. Discussion
Our findings showed that the maternal effects on egg and offspring traits of chub mackerel were evident in 3-year-old females (repeat spawners), which produced larger eggs and larvae with higher starvation tolerance and faster growth at the tested temperatures than those in 1-year-old females (first-time spawners). Moreover, opposing thermal responses were observed between the maternal and offspring traits of this species. Lower temperatures led to larger eggs, first-feeding larvae, and longer starvation tolerance, but this resulted in slower development and growth of offspring. This partly explains why there were contradicting assumptions regarding the effects of thermal environmental conditions on the recruitment success of chub mackerel in the western North Pacific.
4.1. Maternal and thermal effects on egg and larval traits
This study showed that the egg size of chub mackerel was influenced by both maternal age and temperature. Temperature showed commonalities in 1–3-year-old females, with a decrease in egg size in water with increasing temperature. However, there was also a negative correlation between egg size and the number of eggs spawned by females maintained at a constant temperature. A decline in egg sizes of individual females as spawning progressed has been observed in some multiple-batch-spawning capital breeders, referring to the situation in which reproduction is supported using energy stored in advance of the spawning season (McEvoy and McEvoy, 1991; Kjesbu et al., 1996; Trippel, 1998; Rideout et al., 2005), probably because of the adjustment of depleted energy by the end of the spawning season (McBride et al., 2015). Chub mackerel appears to be a capital breeder, as the lipid content in its muscle, with peaks in autumn and winter, decreases with gonadal development in spring (Yoshimitsu et al., 2018). However, the regression slope of egg size–temperature relationship was significantly higher than that of the egg size–number of spawning events at ambient temperature and between ambient and constant temperatures, indicating that temperature could be more influential than the number of eggs spawned. The negative correlation between egg size and temperature may be because of a direct physiological response in which the temperature experienced by a female during vitellogenesis influences the size of egg she produces, as has been found in many fish species (Laptikhovsky, 2006; Yoneda et al., 2014; Barneche et al., 2018b). Meanwhile, the effects of maternal age on the size and nutritional contents of eggs were evident as eggs derived from 2- and 3-year-old females (repeat spawners) were significantly larger and more nutritious than those from 1-year-old females (first-time spawners). This partly agrees with the assumption that larger and older females reproduce disproportionately more than smaller and younger females (Hixon et al., 2014; Barneche et al., 2018a), as there were significant differences in the FL and BW of specimens between the two age classes. In captive Atlantic cod (Gadus morhua) controlled for parental history, the spawning experience of individuals influences egg characteristics more than body size because the egg sizes spawned from second- and/or third-time (repeat) spawners are significantly larger than those from first-time spawners (Kjesbu et al., 1996; Trippel, 1998). In this study, the relationship between egg size and the number of spawnings under constant temperature conditions were monitored from the first to the third spawning season. Although body size might be a co-influential factor for variation in egg size, maternal spawning experience, rather than maternal age, could have significant effects on the size and nutritional content of chub mackerel eggs.
The effects of maternal spawning experience on larval starvation tolerance after hatching and the body size of the first-feeding larvae were obvious. Larvae derived from repeat spawners exhibited higher starvation tolerance, SL, and body heights within a given SL than those derived from first-time spawners. Large body sizes of larvae may be beneficial for swimming ability and prey selection, as SL showed positive correlations with swimming speed and mouth width in chub mackerel larvae (Hunter and Kimbrell, 1980). Moreover, even under ad libitum feeding conditions at a given temperature, larvae derived from repeat spawners grew significantly faster until 10 days of age than those from first-time spawners. These results suggest that the large and nutrient-rich eggs from repeat spawners lead to the enhancement of starvation tolerance and growth potential in the early life stages. Our preliminary observations showed that even within the same SL range, 10-day-old larvae derived from repeat spawners swam significantly faster than those from first-time spawners (Nakamura, personal communication). These results appear to be inconsistent with the observations on haddock (Rideout et al., 2005). Differences in larval morphology induced by differences in egg size spawned over the season persisted for about 5 days after hatching, but the effect of egg size on larval growth was only found at low prey densities and not high prey abundance. In Sebastes spp., which are livebearers with internal fertilization, although there was no effect of maternal age or size on the hatchling size, the larvae derived from older females had larger oil globules than those from younger ones; the larvae from older females survived longer under starvation and grew faster in later life than those from younger ones (Berkeley et al., 2004; Sogard et al., 2008).
4.2. Thermal effects on offspring traits
The effects of temperature on the ontogenetic development and growth rate of chub mackerel at 25 days of age were obvious, as found in previous studies involving wild and captive chub mackerels (Hunter and Kimbrell, 1980; Kamimura et al., 2015; Taga et al., 2019). Higher temperatures under ad libitum food availability led to more advanced development and faster growth than lower temperatures, probably accompanied by an increase in daily food rations (Hunter and Kimbrell, 1980). Our findings also showed that the transitional periods in developmental stages appeared to occur in a limited range of temperatures; larvae were only found at<18°C, whereas juveniles were observed in waters at 17–20°C. As observed by Hunter and Kimbrell (1980), cannibalism toward slower growing fish was commonly observed after specimens reached the juvenile stage. Further, cannibalism continued in the post-juvenile stage as they began to swim in schools in this study. In a given water temperature, faster growing individuals probably acquired more energy than other individuals, including from cannibalism, in a tank. Thus, the evaluation of the relationship between growth rate and temperature at the larval and juvenile stages presented here may be limited. However, all specimens at age 25 days in water at 17.0°C were at larval stage, suggesting that temperatures below 17°C experienced until 25 days after hatching may be a limiting factor for the transition from larval to juvenile stages (Pörtner et al., 2006; Pörtner and Peck, 2010).
Specimens collected at 25 days of age in the Kuroshio–Oyashio transition area were considered either at the juvenile or post-juvenile stage, and no fish were found at the larval stage. Moreover, the growth rates of 25-day-old wild fish ranging from 0.79 to 1.71 mm/day corresponded to estimated temperature of 18.0–20.3°C from the growth rate–temperature relationships at juvenile and post-juvenile stages. Our estimated temperature of the wild fish almost agrees with those estimated from bioenergetic individual-based model (18.5–20.1°C) applied to chub mackerel in the Northwest Pacific (Guo et al., 2022). However, Guo et al. (2022) suggested that there was no significant effect of temperature on early life growth and prey conditions were the dominant environmental factors controlling growth. It is noted that specimens collected in the Kuroshio–Oyashio transition area had already survived the larval stage and migrated from the coastal waters off eastern Japan (around main spawning grounds, Figure 2). In chub mackerel, swimming and feeding abilities rapidly develop around the transition from larval to juvenile stages in association with the precocious development of the digestive system (Kohno et al., 1984; Park et al., 2015). Such ontogenetic shifts could assist growth acceleration after the juvenile stage, at a given temperature, as mentioned above. In wild chub mackerel larvae, a higher growth rate could be accompanied by either higher temperature or both higher temperature and better prey availability (Sassa and Tsukamoto, 2010; Kamimura et al., 2015), but the importance of the prey environment increases as individuals grow in the early stages of life (Taga et al., 2019).
4.3. Potential mechanism of reproductive success of chub mackerel
Previous studies on the relationship between early growth rate and recruitment indexes of chub mackerel in the western North Pacific support the assumption of growth-selective mortality (Kamimura et al., 2015; Kaneko et al., 2019; Taga et al., 2019; Takasuka et al., 2020; Guo et al., 2022) as found in Atlantic mackerel Scomber scombrus (Robert et al., 2007); faster growth in the early life stages leads to better recruitment. This is likely to be accompanied by the finding that even within the same range of SL, fast-growing individuals of juvenile chub mackerel showed higher swimming ability (burst speed) than the slow-growing conspecifics, probably owing to differences in morphological traits (Nakamura et al., 2022). Offspring have to migrate from coastal waters off Japan, spawning grounds, to Kuroshio–Oyashio transition areas, nurseries, and feeding grounds for recruitment (Figure 2, Yukami et al., 2021). Faster growing juveniles often experience a cooler water temperature, where they can access a highly nutritious diet, resulting in further rapid growth (Higuchi et al., 2019). Thus, thermal and/or prey environments surrounding larvae and juveniles, which are largely influenced by the directions of the Kuroshio and Oyashio currents (Kaneko et al., 2019; Guo et al., 2022), could be key determinants of variation in the growth rate of individuals and year classes. Our findings on the maternal and thermal contributions to offspring traits provide additional insights into the mechanism of reproductive success of chub mackerel in these waters (Figure 9).
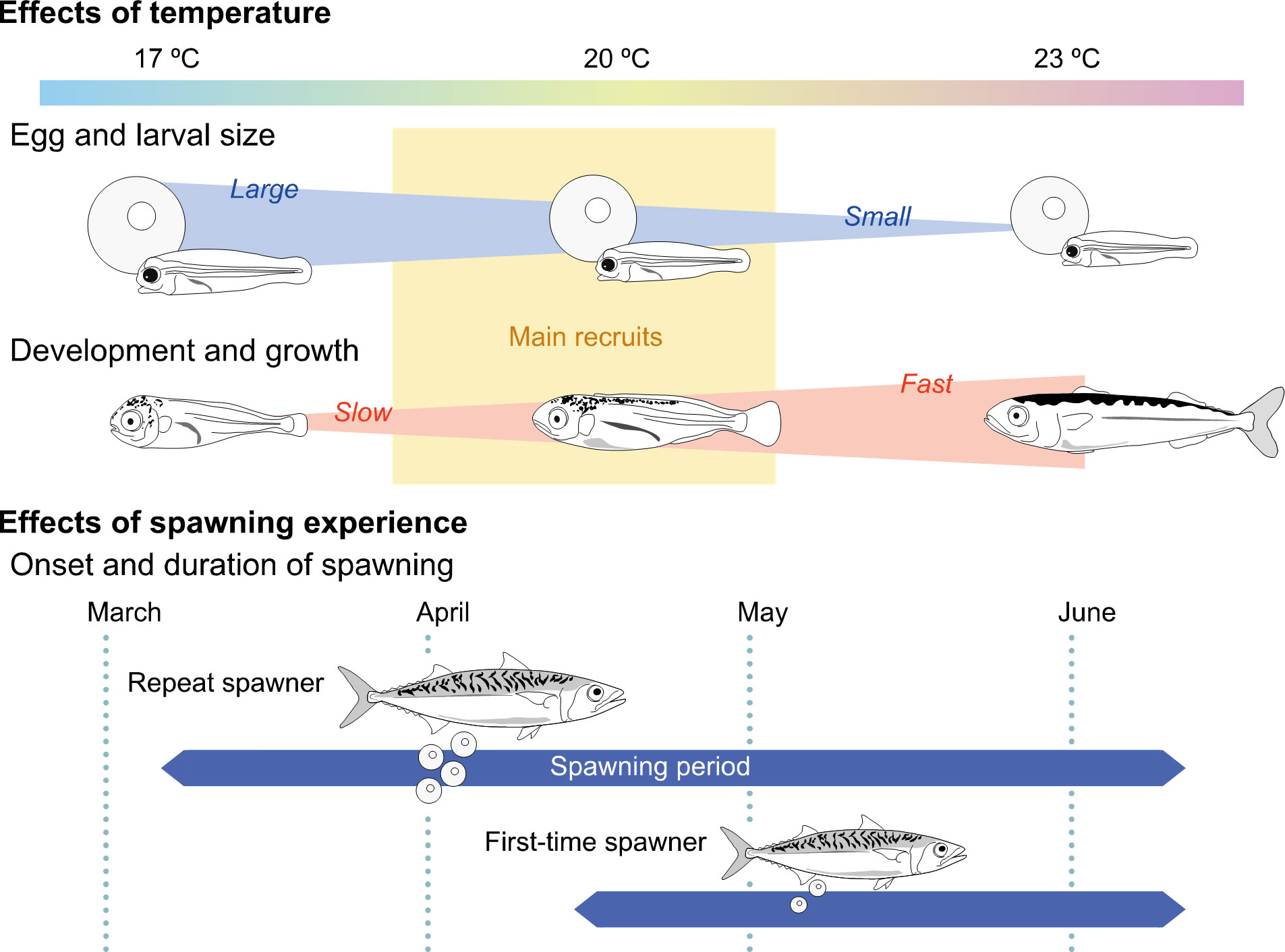
Figure 9 Schematic representation of the effects of temperature and spawning experience on life history traits of chub mackerel. The size of egg and larva at first-feeding and ontogenetic development and growth were differently affected by temperature; the larval and egg size decrease with increasing temperature, while development and growth are advanced and faster in warmer waters. The estimated temperature of wild fish (main recruits) experienced until age 25 days ranged from 18.0 to 20.3°C. The onset timing and duration of spawning appeared to differ between repeat spawners and first-time spawners (after Watanabe and Yatsu, 2006; Watanabe, 2010); the repeat spawners start spawning earlier and longer than the first-time spawners. Larvae derived from repeat spawners were larger on the day of first-feeding and grew faster than those from first-time spawners at identical temperatures.
The sizes of eggs and larvae at the first feeding, end of endogenous feeding, and ontogenetic development and growth are differently affected by temperature. Eggs and first-feeding larvae become smaller with increasing temperature, whereas ontogenetic development and growth occur earlier and faster in warmer waters. Meanwhile, the estimated temperature experienced by wild fish (main recruits) until the age of 25 days ranged from 18.0 to 20.3°C, which is equivalent to the temperatures around the spawning grounds during April (Kaneko et al., 2019). This appears to be restricted to a range of spawning temperatures (16–22°C) found in the waters of Pacific coast of Japan (Takasuka et al., 2008b). This implies that the restricted temperature range may be the thermal window that ensures both the egg quality of maternal fish and the growth rate (ontogenetic development) of offspring, which is necessary for the recruitment of chub mackerel. On the other hand, the onset timing and duration of spawning appear to differ between repeat spawners and first-time spawners (Watanabe and Yatsu, 2006; Watanabe, 2010); the repeat spawners are likely to start spawning earlier and longer than the first-time spawners. Interestingly, during the 1970s and 1980s, when relatively higher recruitment was observed (Figure 1), peak spawning was concentrated in April, whereas in the 1990s and 2000s, when lower levels of recruitment were observed, peak spawning shifted to May (Watanabe and Yatsu, 2006; Watanabe, 2010; Kanamori et al., 2019). Our findings showed that larvae derived from repeat spawners were larger on the day of first feeding and grew and swam faster than those from first-time spawners even under identical thermal conditions. Such traits found in repeat spawners and their offspring may act as a buffer against environmental variations (Anderson et al., 2008; Hixon et al., 2014), enhancing the probability of the early survival of chub mackerel in the western North Pacific. This suggests that repeat spawners of chub mackerel could contribute more to reproductive success than first-time spawners, which partly explains the higher levels of recruit fish biomass in these waters in the recent years.
In conclusion, our findings highlight the importance of maternal contribution to the viability of chub mackerel offspring. Maternal spawning experience, rather than maternal age, could have profound effects on the size and nutritional content of eggs of chub mackerel. Large and nutrient-rich eggs laid by repeat spawners developed into large first-feeding larvae, accompanied by higher growth and swimming potentials than those from first-time spawners. Such an advantage in the early life stages can promote rapid growth within a certain limit of the thermal environment and may allow for easier migration from spawning grounds to nursery grounds. This is inconsistent with the traditional assumption that females of different sizes or ages produce the same number and quality of offspring per unit of body weight (Hixon et al., 2014). Because changes in the age structure of spawning stock biomass can affect the variability, productivity, stability, and yield of fish populations, considering maternal effects on population dynamics has become more important for sustainable fishery management of exploited marine fish (Shelton et al., 2012; Shelton et al., 2015; Ohlberger et al., 2022). Our findings could assist in incorporating the effect of maternal spawning experience on offspring viability into more accurate population dynamics projections of chub mackerel in the western North Pacific region.
Data availability statement
The datasets for this study can be found in online repositories. The name of repository and accession number can be found: https://doi.org/10.6084/m9.figshare.21285600.
Ethics statement
The animal study was reviewed and approved by Faculty of Agriculture and Graduate Course at Kyushu University and according to the laws (No. 105) and notifications (No. 6) of the Japanese government Animal welfare of Fisheries Research and Education Agency, Japan (50322001) and were approved by the committee of animal welfare of National Research Institute of Fisheries and Environment of Inland Sea, Fisheries Research and Education Agency (no. 2016-8).
Author contributions
MY, AK, MM and AS designed the study. MY, HK, MNy, MNa, MT and TT conducted experiments. MY and HT analyzed the experimental data. MT and YK provided the data on wild fish. MY wrote the original manuscript. HK, MNy, MNa, MT, AK, MM, AS, TT, HT and YK gave beneficial advice and edited the manuscript. All authors contributed to the article and approved the submitted version.
Funding
This research was funded by a subproject on studies on the prediction and application of fish species alternation (SUPRFISH, JPJ000555), financed by the Agriculture, Forestry and Fisheries Research Council of Japan, and was supported by the Promotion Programs for the Marine Fisheries Stock Assessment and Evaluation for Japanese Waters from Fisheries Agency of Japan and by Grant-in-Aid for Challenging Research (Pioneering) (KAKENHI No. 19H05540) and Scientific Research (B) (KAKENHI No. 22H02421) from the Japan Society for the Promotion of Science.
Acknowledgments
We thank the staff and students of the Laboratory of Marine Biology at Kyushu University and the staff for National Research Institute of Fisheries and Environment of Inland Sea (NRIFEIS), Hakatajima Field Station for their assistance with rearing experiments. We would like to thank Editage (www.editage.com) for English language editing.
Conflict of interest
The authors declare that the research was conducted in the absence of any commercial or financial relationships that could be construed as a potential conflict of interest.
Publisher’s note
All claims expressed in this article are solely those of the authors and do not necessarily represent those of their affiliated organizations, or those of the publisher, the editors and the reviewers. Any product that may be evaluated in this article, or claim that may be made by its manufacturer, is not guaranteed or endorsed by the publisher.
Supplementary material
The Supplementary Material for this article can be found online at: https://www.frontiersin.org/articles/10.3389/fmars.2022.1063468/full#supplementary-material
References
Anderson C. N. K., Hsieh C., Sandin S. A., Hewitt R., Hollowed A., Beddington J., et al. (2008). Why fishing magnifies fluctuations in fish abundance. Nature 452, 835–839. doi: 10.1038/nature06851
Barneche D. R., Burgess S. C., Marshall D. J. (2018b). Global environmental drivers of marine fish egg size. Glob. Ecol. Biogeogr. 27, 890–898. doi: 10.1111/geb.12748
Barneche D. R., Robertson D. R., White C. R., Marshall D. J. (2018a). Fish reproductive-energy output increases disproportionately with body size. Science 645, 642–645. doi: 10.1126/science.aao6868
Bates D., Mächler M., Bolker B., Walker S. (2015). Fitting linear mixed-effects models using lme4. J. Stat. Softw. 67, 1–48. doi: 10.18637/jss.v067.i01
Berkeley S. A., Chapman C., Sogard S. M. (2004). Maternal age as a determinant of larval growth and survival in a marine fish, Sebastes melanops. Ecology 85, 1258–1264. doi: 10.1890/03-0706
Broström G., Holmberg H. (2011). Generalized linear models with clustered data: Fixed and random effects models. Comput. Stat. Data Anal. 55, 3123–3134. doi: 10.1016/j.csda.2011.06.011
Chambers R. C. (1997). “Environmental influences on egg and propagule sizes in marine fishes,” in Early life history and recruitment in fish populations. Eds. Chambers R. C., Trippel E. A. (London: Chapman & Hall), 63–102.
Core Team R. (2021). R: A language and environment for statistical computing (Vienna, Austria: R Foundation for Statistical Computing). Available at: https://www.R-project.org/.
Cushing E. H. (1990). Plankton production and year-class strength in fish populations: an update of the match/mismatch hypothesis. Adv. Mar. Biol. 26, 249–293. doi: 10.1016/S0065-2881(08)60202-3
Green B. S. (2008). Maternal effects in fish populations. Adv. Mar. Biol. 54, 1–105. doi: 10.1016/S0065-2881(08)00001-1
Guo C., Ito S., Kamimura Y., Xiu P. (2022). Evaluating the influence of environmental factors on the early life history growth of chub mackerel (Scomber japonicus) using a growth and migration model. Prog. Oceanogr. 206, 102821. doi: 10.1016/j.pocean.2022.102821
Higuchi T., Ito S., Ishimura T., Kamimura Y., Shirai K., Shindo H., et al. (2019). Otoliths oxygen isotope analysis and temperature history in early life stages of the chub mackerel Scomber japonicus in the kuroshio-oyashio transition region. Deep Sea Res. II 169–170, 104660. doi: 10.1016/j.dsr2.2019.104660
Hixon M. A., Johnson D. W., Sogard S. M. (2014). BOFFFFs: on the importance of conserving old-growth age structure in fishery populations. ICES J. Mar. Sci. 71, 2171–2185. doi: 10.1093/icesjms/fst200
Houde E. D. (2008). Emerging from hjort’s shadow. J. Northwest Atl. Fish. Sci. 41, 53–70. doi: 10.2960/J.v41.m634
Hunter J. R., Kimbrell C. A. (1980). Early life history of pacific mackerel, Scomber japonicus. Fish Bull. 78, 89–101.
Kamimura Y., Takahashi M., Yamashita N., Watanabe C., Kawabata A. (2015). Larval and juvenile growth of chub mackerel Scomber japonicus in relation to recruitment in the western north pacific. Fish. Sci. 81, 505–513. doi: 10.1007/s12562-015-0869-4
Kanamori Y., Takasuka A., Nishijima S., Okamura H. (2019). Climate change shifts the spawning ground northward and extends the spawning period of chub mackerel in the western north pacific. Mar. Ecol. Prog. Ser. 624m, 155–166. doi: 10.3354/meps13037
Kaneko H., Okunishi T., Seto T., Kuroda H., Itoh S., Kouketsu S., et al. (2019). Dual effects of reversed winter-spring temperatures on year-to-year variation in the recruitment of chub mackerel (Somber japonicus). Fish. Oceanogr. 28, 212–227. doi: 10.1111/fog.12403
Kawai H., Yatsu A., Watanabe C., Mitani T., Katsukawa T., Matsuda H. (2002). Recovery policy for chub mackerel stock using recruitment-per-spawning. Fish. Sci. 68, 963–971. doi: 10.1046/j.1444-2906.2002.00520.x
Kjesbu O. S., Solemdal P., Bratland P., Fonn M. (1996). Variation in annual egg production in individual captive Atlantic cod (Gadus morhua). Can. J. Fish. Aquat. Sci. 53, 610–620. doi: 10.1139/f95-215
Kohno H., Shimizu M., Nose Y. (1984). Morphological aspects of the development of swimming and feeding functions in larval Scomber japonicus. Bull. Japan. Soc Sci. Fish. 50, 1125–1137. doi: 10.2331/suisan.50.1125
Kuznetsova A., Brockhoff P. B., Christensen R. H. B. (2017). “lmerTest package: Tests in linear mixed effects models. J. Stat. Softw 82, 1–26. doi: 10.18637/jss.v082.i13
Laptikhovsky V. (2006). Latitudinal and bathymetric trends in egg size variation: A new look at thorson’s and rass’s rules. Mar. Ecol. 27, 7–14. doi: 10.1111/j.1439-0485.2006.00077.x
Marshall D. J., Allen R. M., Crean A. J. (2008). The ecological and evolutionary importance of maternal effects in the sea. Oceanogr. Mar. Biol. 46, 203–250. doi: 10.1201/9781420065756.ch5
McBride R. S., Somarakis S., Fitzhugh G. R., Albert A., Yaragina N. A., Wuenschel M. J., et al. (2015). Energy acquisition and allocation to egg production in relation to fish reproductive strategies. Fish Fish. 16, 23–57. doi: 10.1111/faf.12043
McEvoy L. A., McEvoy J. (1991). Size fluctuations in the eggs an newly hatched larvae of captive turbot (Scophthalmus maximus). J. Mar. Biol. Assoc. UK. 71, 679–690. doi: 10.1017/S0025315400053236
Nakamura M., Yoneda M., Morioka T., Takasuka A., Nishiumi N. (2022). Positive effects of fast growth on locomotor performance in pelagic fish juveniles. Oecologia 199, 589–597. doi: 10.1007/s00442-022-05216-6
Nyuji M., Selvaraj S., Kitano H., Ohga H., Yoneda M., Shimizu A., et al. (2012). Changes in the expression of pituitary gonadotropin subunits during reproductive cycle of multiple spawning female chub mackerel Scomber japonicus. Fish Physiol. Biochem. 38, 883–897. doi: 10.1007/s10695-011-9576-y
Nyuji M., Shiraishi T., Selvaraj S., In V. V., Kitano H., Yamaguchi A., et al. (2011). Immunoreactive changes in pituitary FSH and LH cells during seasonal reproductive and spawning cycles of female chub mackerel Somber japonicus. Fish. Sci. 77, 731–739. doi: 10.1007/s12562-011-0380-5
Ohlberger J., Langangen Ø, Stige L. C. (2022). Age structure affects population productivity in an exploited fish species. Ecol. Appl. 32, e2614. doi: 10.1002/eap.2614
Park S. J., Lee S. G., Gwak W. S. (2015). Ontogenetic development of the digestive system in chub mackerel Scomber japonicus larvae and juveniles. Fish. Aquat. Sci. 18, 301–309. doi: 10.5657/FAS.2015.0301
Pörtner H. O., Bennett A. F., Bozinovic F., Clarke A., Lardies M. A., Lucassen M., et al. (2006). Trade-offs in thermal adaptation: The need for a molecular to ecological integration. Physiol. Biochem. Zool. 79, 295–313. doi: 10.1086/499986
Pörtner H. O., Peck M. A. (2010). Climate change effects on fishes and fisheries: towards a cause-and-effect understanding. J. Fish Biol. 77, 1745–1779. doi: 10.1111/j.1095-8649.2010.02783.x
Ricker W. E. (1975). Computation and interpretation of biological statistics of fish populations. Bull. Fish. Res. Board Can. 191, 1–382.
Rideout R. M., Trippel E. A., Litvak M. K. (2005). Effects on egg size, food supply and spawning time on early life history success of haddock Melanogrammus aeglefinus. Mar. Ecol. Prog. Ser. 285, 169–180. doi: 10.3354/meps285169
Robert D., Castonguay M., Fortier L. (2007). Early growth and recruitment in Atlantic mackerel Scomber scombrus: discriminating the effects of fast growth and selection for fast growth. Mar. Ecol. Prog. Ser. 337, 209–219. doi: 10.3354/meps337209
Sassa C., Tsukamoto Y. (2010). Distribution and growth of Scomber japonicus and S. australasicus larvae in the southern East China Sea in response to oceanographic conditions. Mar. Eco. Prog. Ser. 419, 185–199. doi: 10.3354/meps08832
Shelton A. O., Hutchings J. A., Waples R. S., Keith D. M., Akçakaya H. R., Dulvy N. K. (2015). Maternal age effects on Atlantic cod recruitment and implications for future potential trajectories. ICES J. Mar. Sci. 72, 1769–1778. doi: 10.1093/icesjms/fsv058
Shelton A. O., Munch S. B., Keith D., Mangel M. (2012). Maternal age, fecundity, egg quality, and recruitment: linking stock structure to recruitment using an age-structured ricker model. Can. J. Fish. Aquat. Sci. 69, 1631–1641. doi: 10.1139/F2012-082
Shiraishi T., Ketkar S. D., Kitano H., Nyuji M., Yamaguchi A., Matsuyama M. (2008). Time course of final oocyte maturation and ovulation in chub mackerel Somber japonicus induced by hCG and GnRHa. Fish. Sci. 74, 764–769. doi: 10.1111/j.1444-2906.2008.01587.x
Sogard S. M., Berkeley S. A., Fisher R. (2008). Maternal effects in rockfishes Sebastes spp.: a comparison among species. Mar. Ecol. Prog. Ser. 360, 227–236. doi: 10.3354/meps07468
Taga M., Kamimura Y., Yamashita Y. (2019). Effects of water temperature and prey density on recent growth of chub mackerel Scomber japonicus larvae and juveniles along the pacific coast of boso–kashimanada. Fish. Sci. 85, 931–942. doi: 10.1007/s12562-019-01354-8
Takasuka A., Oozeki Y., Kubota H. (2008b). Multi-species regime shifts reflected in spawning temperature optima of small pelagic fish in the western north pacific. Mar. Eco. Prog. Ser. 360, 211–217. doi: 10.3354/meps07407
Takasuka A., Oozeki Y., Kubota H., Lluch-Cota S. E. (2008a). Contrasting spawning temperature optima: Why are anchovy and sardine regime shifts synchronous across the north pacific? Prog. Oceanogr. 77, 225–232. doi: 10.1016/j.pocean.2008.03.008
Takasuka A., Yoneda M., Oozeki Y. (2020). Density-dependent egg production in chub mackerel in the kuroshio current system. Fish. Oceanogr. 30, 38–50. doi: 10.1111/fog.12501
Trippel E. A. (1998). Egg size and viability and seasonal offspring production of young Atlantic cod. Trans. Am. Fish. Soc 127, 339–359. doi: 10.1577/1548-8659(1998)127<0339:esavas>2.0.co;2
Vaida F., Blanchard S. (2005). Conditional akaike information for mixed-effects models. Biometrika 92, 351–370. doi: 10.1093/biomet/92.2.351
Watanabe T. (1970). Morphology and ecology of early stages of life in Japanese common mackerel, Scomber japonicus houttuyn, with special reference to fluctuation of population. Bull. Tokai Reg. Fish Res. Lab. 62, 1–283.
Watanabe C. (2010). Changes in the reproductive traits of the pacific stock of chub mackerel Scomber japonicus and their effects on the population dynamics. Bull. Jap. Soc Fish. Oceanogr. 74 (Spec.), 46–50.
Watanabe C., Yatsu A. (2004). Effects of density-dependence and sea surface temperature on interannual variation in length-at-age of chub mackerel (Scomber japonicus) in the kuroshio-oyashio area during 1970–1997. Fish. Bull. 102, 196–206.
Watanabe C., Yatsu A. (2006). Long-term changes in maturity at age of chub mackerel (Scomber japonicus) in relation to population declines in the waters off northeastern Japan. Fish. Res. 78, 323–332. doi: 10.1016/j.fishres.2006.01.001
Wright P. J., Trippel E. A. (2009). Fishery-induced demographic changes in the timing of spawning: consequences for reproductive success. Fish Fish. 10, 283–304. doi: 10.1111/j.1467-2979.2008.00322.x
Yatsu A. (2019). Review of population dynamics and management of small pelagic fishes around the Japanese archipelago. Fish. Sci. 85, 611–639. doi: 10.1007/s12562-019-01305-3
Yatsu A., Watanabe T., Ishida M., Sugisaki H., Jacobson L. D. (2005). Environmental effects on recruitment and productivity of Japanese sardine Sardinops melanostictus and chub mackerel Scomber japonicus with recommendations for management. Fish. Oceanogr. 14, 263–278. doi: 10.1111/j.1365-2419.2005.00335.x
Yoneda M., Kitano H., Selvaraj S., Matsuyama M., Shimizu A. (2013). Dynamics of gonadosomatic index of fish with indeterminate fecundity between subsequent egg batches: application to Japanese anchovy Engraulis japonicus under captive conditions. Mar. Biol. 160, 2733–2741. doi: 10.1007/s00227-013-2266-9
Yoneda M., Kitano H., Tanaka H., Kawamura K., Selvaraj S., Ohshimo S., et al. (2014). Temperature- and income resource availability-mediated variation in reproductive investment in a multiple-batch-spawning Japanese anchovy. Mar. Ecol. Prog. Ser. 516, 251–262. doi: 10.3354/meps10969
Yoshimitsu T., Kato M., Kobayashi S. (2018). Relationship between seasonal variations of lipid content and gonadal development in the chub mackerel Scomber japonicus landed at the port of choshi. Nippon Suisan Gakkaishi 84, 1017–1024. doi: 10.2331/suisan.18-00025
Yukami R., Nishijima S., Kamimura Y., Furuichi S., Watabe R. (2021). Stock assessment and evaluation for the pacific stock of scomber japonicus in 2021 (Tokyo: Fisheries Agency and Fisheries Research and Education Agency of Japan), 56 pp. Available at: http://abchan.fra.go.jp/.
Keywords: egg size, early growth rate, larval size, ontogenetic development, population dynamics, recruitment, spawning experience, starvation tolerance
Citation: Yoneda M, Kitano H, Nyuji M, Nakamura M, Takahashi M, Kawabata A, Matsuyama M, Shimizu A, Tsuzaki T, Togashi H and Kamimura Y (2022) Maternal spawning experience and thermal effects on offspring viability of chub mackerel and their influence on reproductive success. Front. Mar. Sci. 9:1063468. doi: 10.3389/fmars.2022.1063468
Received: 07 October 2022; Accepted: 09 December 2022;
Published: 23 December 2022.
Edited by:
SM Sharifuzzaman, University of Chittagong, BangladeshReviewed by:
Gustavo Javier Macchi, Consejo Nacional de Investigaciones Científicas y Técnicas (CONICET), ArgentinaCristina García, Institute of Marine Research (CSIC), Spain
Copyright © 2022 Yoneda, Kitano, Nyuji, Nakamura, Takahashi, Kawabata, Matsuyama, Shimizu, Tsuzaki, Togashi and Kamimura. This is an open-access article distributed under the terms of the Creative Commons Attribution License (CC BY). The use, distribution or reproduction in other forums is permitted, provided the original author(s) and the copyright owner(s) are credited and that the original publication in this journal is cited, in accordance with accepted academic practice. No use, distribution or reproduction is permitted which does not comply with these terms.
*Correspondence: Michio Yoneda, yoneda_michio55@fra.go.jp
†Present address: Fisheries Technology Institute, Japan Fisheries Research and Education Agency, Miyazu Field Station, Miyazu, Japan