- 1Laboratorio de Percepción Remota y SIG, Centro de Investigación y de Estudios Avanzados del Instituto Politécnico Nacional, Departamento de Recursos del Mar, Mérida, Mexico
- 2Departamento de Recursos del Mar, CONACYT - Centro de Investigación y de Estudios Avanzados del Instituto Politécnico Nacional, Mérida, Mexico
- 3Recursos del Mar, CONACYT – Centro de Investigación y de Estudios Avanzados del Instituto Politécnico Nacional, Mérida, Mexico
- 4Harte Research Institute, Texas A&M University & Kalanbio, A.C., Mérida, Mexico
- 5Posgrado en Ciencias en Restauración Ecológica, Universidad Autónoma del Carmen, Cd. del Carmen, Mexico
- 6Programa de Tortugas Marinas, Centro de Investigaciones Pesqueras, La Habana, Cuba
- 7Aquarium del Puerto de Veracruz, Veracruz, Mexico
- 8Programa de Conservación de Tortugas Marinas, Pronatura Península de Yucatán, A. C. Mérida, Mexico
- 9Área de Protección de Flora y Fauna Laguna de Términos-Comisión Nacional de Áreas Naturales Protegidas, Cd. del Carmen, Mexico
- 10Santuario Playa Rancho Nuevo, Comisión Nacional de Áreas Naturales Protegidas, Rancho Nuevo, Mexico
Marine turtles are of the highest ecological concern in terms of conservation and restoration programs and marine territorial ordering. Marine resource-extracting industries have severe negative impacts on their populations and their habitats. Thus, private industries and decision makers require the best and most robust spatially explicit scientific knowledge for a sustainable and responsible operation and government administration. Therefore, the identification of marine turtle hotspots is a strategic milestone for ecosystem management and an integral ecological knowledge about any region. We identified and delimited marine turtle hotspots in the Gulf of Mexico and Mesoamerican Reef. We analyzed satellite-tracked data of 178 individuals belonging to four marine turtle species. We used Brownian bridge movement models to estimate space use surfaces and map algebra to weight and merge layers of multiple species, life stages, and movement phases. The Yucatan Peninsula and central Veracruz in Mexico, along with the Florida Keys and Louisiana coast in the US, harbor the primary hotspots where marine turtles aggregate. We defined four primary hotspots used for migration in the western Gulf of Mexico, around the Yucatan Peninsula, a northern route following the Loop Current, and a southern one towards Gorda Bank, Central America. This study is the largest assessment of marine turtle hotspots in Mexico and Cuba and supports strategies for reinforcing regional management actions for their conservation, as well as a stronger response and preparedness for tackling anthropic threats to these species. This milestone contributes to extending the knowledge frontiers about these species to a new level.
Introduction
Marine turtles are a relevant ecological group owing to their vulnerability to several natural and anthropogenic threats. They occupy different marine and coastal ecosystems in their lifetimes configuring conditions that bolster their ecological role as a sentinel, umbrella, and key species for maintaining ecological integrity in marine ecosystems (Caro and O’Doherty, 1999; Wabnitz et al., 2010; Gradzens et al., 2014; Hunter et al., 2016; Kalinkat et al., 2017; Hazen et al., 2019; Liceaga-Correa et al., 2022).
Marine turtles have strong philopatry for their critical habitats, which include areas for nesting, mating, migration, feeding, development, and residency (FitzSimmons et al., 1997; Shaver et al., 2016; Gaos et al., 2017). Therefore, the identification and mapping of their habitats is crucial for conserving and recovering their populations, as national and multinational policymaking agencies require robust spatially explicit information for adapting and improving their actions.
Key marine ecological habitats have been identified from different approaches. Areas that have a high biodiversity value and are frequently associated with threatened species or particular ecological processes have been mostly reported to date (Worm et al., 2003; Bass et al., 2011; Edgar and Brooks, 2011; Hazen et al., 2013; Bax et al., 2015). Several efforts have been taken to define marine mega fauna hotspots using distinct approaches, most of which consider the spatial distribution, density, and conservation status of focal species (Lascelles et al., 2016). Using mostly satellite-tracking data of marine turtles, hotspots have been reported for loggerhead populations in the northwestern Atlantic (Ceriani et al., 2017) and the Mediterranean (Schofield et al., 2009, Schofield et al., 2013), green turtles in the Mediterranean (Stokes et al., 2015), hawksbills in the Arabian region (Pilcher et al., 2014), leatherbacks in southern Africa (Robinson et al., 2016), and Kemp’s ridleys in the Gulf of Mexico (GoM) (Gradzens and Shaver, 2020).
Several studies have reported aggregation and migratory corridors of marine turtles in the Gulf of Mexico (Shaver et al., 2013; Shaver et al., 2016; Gradzens and Shaver, 2020; Iverson et al., 2020; Hart et al., 2020; Uribe-Martínez et al., 2021). However, doing a multispecies and multi-life stage study is challenging because of the geographic scope of their life-stages and the difficult access to their spatial distribution data to delineate their populations’ hotspots. Lamont et al. (2015) combined satellite records and distribution models for different loggerhead life-stages in the Gulf of Mexico and delimited their overlapping. But considerable knowledge gaps remain, and the present study aims to address some of them.
Marine turtles contribute to essential ecosystem functions in the ocean linked to environmental services that benefit human societies, such as the modulation influence on blue carbon storage by seagrasses when marine turtles forage on them, and the community balance at healthy coral reefs, that support tourism and fishing industries when they feed on algae and sponges (Bjorndal and Jackson, 2003; Goatley et al., 2012; Hammerschlag et al., 2019; Johnson et al., 2020). These species interact with distinct anthropic activities that may disrupt their long-term viability and jeopardize their biological processes (Cuevas et al., 2018; Hart et al., 2018a; Cuevas et al., 2019). Therefore, spatially explicit information about their hotspots is a much-needed milestone for environmental risk assessments and policies that support effective management, conservation, mitigation, and restoration strategies using the sentinel and umbrella roles of these charismatic species.
This socioenvironmental context demands humanistic, technological, and scientific information of our oceans to implement public policies that prevent, reduce, and control the negative impacts of anthropogenic activities on marine ecosystems. The aim is to achieve a balance between responsible exploitation of natural goods and services, and conservation of the ecosystems that provide them. Thus, the identification of marine turtle distribution and biodiversity hotspots in the Gulf of Mexico is a milestone that can expand the frontier of knowledge on this intensively exploited ecosystem.
The objective of the present study was to identify multispecies and multi-life stage distribution and diversity hotspots of marine turtles in the Gulf of Mexico and Mesoamerican Reef. The results are expected to expand the ecological knowledge on endangered species in this region and strengthen and improve policy-making processes, which in turn can ameliorate the compliance of national preparedness and response capabilities with multinational agreements and commitments for the conservation of endangered marine species and ecosystems.
Materials and methods
Study Area
Our study area was delineated by the movement behavior of satellite-tracked marine turtles from 54 nesting beaches located in Tamaulipas, Veracruz, Campeche, Yucatan, and Quintana Roo, Mexico, the southern Gulf of Mexico and northwestern Caribbean Sea, and the southeastern Cuban littoral (Table S1). These nesting beaches were selected based on their relevance in terms of the number of nests for each species and taking into account the number of previously satellite-tracked individuals from each of the nesting beaches (Moncada-Gavilán, 2005; Moncada-Gavilán et al, 2012; Uribe-Martínez et al., 2021; Liceaga-Correa et al., 2022).
Satellite telemetry
A total of 178 individuals from four species—Caretta caretta (loggerheads), Chelonia mydas (greens), Eretmochelys imbricata (hawksbills), and Lepidochelys kempii (Kemp’s ridleys)—were satellite tracked from 1996 to 2019. These individuals included 5 adult male hawksbills, 167 breeding females (14 loggerheads, 64 greens, 65 hawksbills, and 24 Kemp’s ridleys), and 6 immature individuals (3 loggerheads and 2 greens). The males were captured in-water, and a few were tagged after rehabilitation (Cuevas et al., 2020). The females were mostly randomly selected and captured from their nesting beaches after laying eggs (Uribe-Martínez et al., 2021); only 2 were captured in-water (using a free-weight net). The selection of nesting beaches for tagging females aimed to have all rookeries from the study area represented (Cuevas et al., 2019; Uribe-Martínez et al., 2021). To contend with bias effects by unbalanced sampled individuals per life-stage, we weighted them, as described in further paragraphs, and independently analyzed the distribution data of each of them, then rescaled the outputs, standardize, and mixed them with the others. On the other hand, the immature individuals were bycatch in artisanal fishery (mostly by four-inch monofilament gillnets used by artisanal fleets operating nearshore) and tagged before release.
The transmitter deployment slightly differed among projects, as different techniques and materials were available during the 23 years of this study. Briefly, the individuals were held in a safe place, and after securing them for tagging, the scutes in which the transmitter was to be deployed were cleaned using sandpaper, fresh water, and alcohol. Most of the tags were attached using fast cure epoxy glue and clay (e.g., Devco 5 min© and Loctite Magic Steel©), but a few were attached using fiber glass and polyester epoxy (Moncada-Gavilán, 2005; Cuevas et al., 2008; Moncada-Gavilán et al, 2012; Uribe-Martínez et al., 2021). Finally, marine antifouling paint was applied after the glue and clay cured, and the individuals were released at the same or nearest point where they were captured.
Tracks from this study dataset were acquired by several multi-institution research efforts in Mexico and Cuba, diverse satellite transmitters were used for over two decades (Table S1). However, all satellite transmitters used the ARGOS system, making them comparable in terms of the acquisition system and general data characteristics. All ARGOS location classes (LC) (e.g., 3, 2, 1, 0, A, and B) were used for these analyses, as the latter LCs (A and B) can also be accurate (Lopez et al., 2013; Boyd and Brightsmith, 2013; Uribe-Martínez et al, 2021). The location records were filtered to remove errors using the algorithm described by McConnell et al. (1992) implemented in the vmask function in the R argosfilter package (Freitas et al., 2012). Finally, we performed supervised data validation to eliminate records that the filters did not detect, as they were considered unrealistic given their distance, time, or location regarding the rest of its track. For further details, see Uribe-Martínez et al. (2021).
Finally, to consider the phase that we recorded for each location of the tracked individuals, all the location records were classified as migration, internesting, or foraging and residency (Cuevas et al., 2019; Hart et al., 2020).
Space use surface processing
We used Brownian bridges models (Horne et al., 2007) with the BBMM package in R (Nielson et al., 2014) to build the space use surface for each tracked individual. This numerical method uses the difference between the acquisition times of each pair of consecutive location records in any track to estimate the probability of an individual using any portion of the space between these locations for transit. It has been widely applied for studying the spatial and movement ecology of marine and terrestrial animals. The advantage of the Brownian bridge analysis is that it minimizes individual variations and movement errors, performs well even under poor data availability. Thus, it has been used to delimit home ranges, migration corridors, and animal movements (Papastamatiou et al., 2013; Liu et al., 2015; Silva et al., 2020). Like Uribe-Martínez et al. (2021) we used a fixed error location of 300 m and a maximum time lag of 5000 min (3.47 days). Finally, we delineated the individual moving ranges by defining a 70% isoline of the created space use probability surface, which was considered the most probable used area by the tracked turtle.
Hotspot identification
As important geographic areas are defined by aggregations of multiple individuals of the same or multiple species at a given site (Uribe-Martínez et al., 2021), we adopted a methodological approach that considered the number of individuals in the same geographic area and weighed each movement phase, life-stage, and species (Bolten et al., 2011; Cuevas et al., 2018; Cuevas et al., 2019). For each of these categories we merged the individual movement ranges (70%) to build a layer containing the number of individuals occurring at any site of a space use surface. This layer was rescaled to values from 0 to 1 to make all layers comparable, which were then multiplied by their corresponding weighting values.
Those values of the movement phases were adopted from (Cuevas et al., 2018; Cuevas et al., 2019). and defined as follows: internesting (0.2857), migrating (0.1429), and feeding/residency (0.5714). For life-stage, we adapted the values proposed by Bolten et al. (2011): hatchlings (0.25), immatures (0.33), and adults (0.45). Although we did not analyze hatchlings, for mathematical purposes, we estimated their weight, so that the sum of the three values was 1.
The weights for each species were defined using the analytic hierarchy process (Saaty, 2008) approach. We interviewed 48 marine turtle specialists in Mexico, considering the four criteria established by the Mexican Extinction Risk Assessment Method for Wildlife (Sánchez-Salas et al., 2013), which is the official method to define the species enlisted for protection under the Mexican law (NOM-059-SEMARNAT-2010). The criteria to evaluate a species are (1) its distribution amplitude, (2) the natural condition of its habitat with respect to its life history, (3) its intrinsic vulnerability, and (4) the impact of anthropogenic activities on the species. We obtained the following values: C. mydas (0.1026), C. caretta (0.2564), L. kempii (0.3077), and E. imbricata (0.333).
The merged layers grouping the individual ranges were then multiplied by their corresponding values of movement phase, life-stage, and species, and finally rescaled from 0 to 1, where 1 was the highest intensity of the hotspot value. For smoothing and visual improvement purposes, the final expression of the layer values was performed using a hexagonal lattice (10 km width/hexagon) and statistically categorized, maximizing the variability among categories and minimizing it in the interior (North, 2009), as movement/aggregation range, secondary hotspots, and primary hotspots.
Results
We defined multispecies and multi-life stage hotspots for four marine turtle species in the GoM and Western Caribbean (WC). This is the first study in the GoM and WC including the Mesoamerican Reef region (MR) that integrates an articulated regional panorama of how marine turtles use their marine space at different stages of their life. The only zones that were not occupied were the deep basins (>1,000 m) of these regions, although some of them were frequently crossed by the turtles during their migrations (Figure 1).
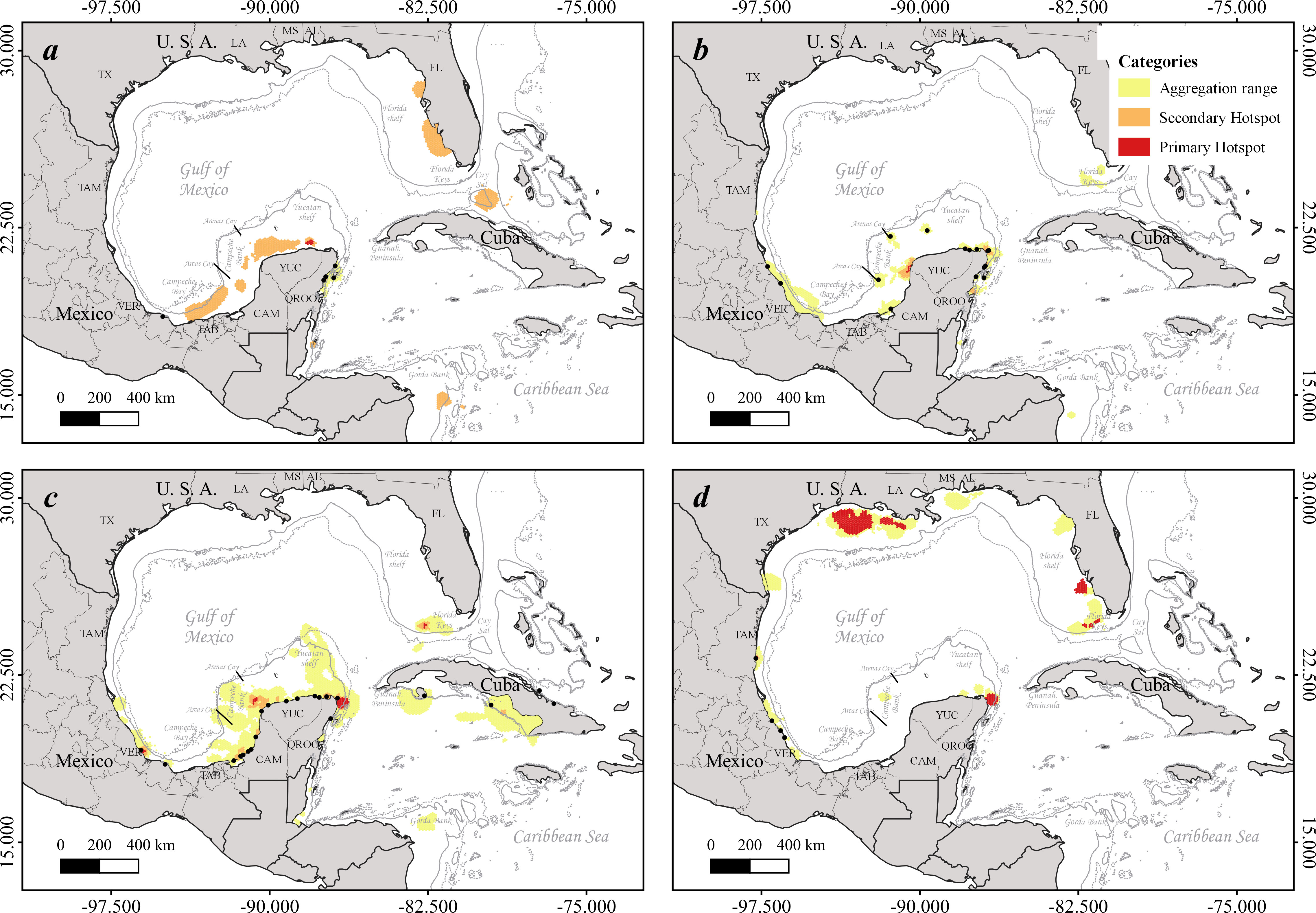
Figure 1 Aggregation hotspots for breeding female marine turtles, integrated with the internesting and feeding/residency movement phases. We present three hotspot categories for C. caretta (A, n=10), C. mydas (B, n=65), E. imbricata (C, n=79), and L. kempii (D, n=24). Continuous thin gray line depicts the 200 m isobath, and the dashed thin line depicts the 500 m isobath (https://www.gebco.net/). Black dots represent the releasing sites of the satellite-tracked individuals.
The four studied species extensively used the GoM during different life stages (immature and reproductive) and for distinct life history movements. Post-nesting loggerheads established their primary and secondary aggregation hotspots on the Campeche Bay continental slope and the Yucatan shelf, as well as on the Florida shelf, Cay Sal in the Lucayan Archipelago (Bahamas), and the northern Gorda Bank on the border between Honduras and Nicaragua (Figure 1A). The Mexican Caribbean was used during internesting movements, and a few individuals established in the Mexican waters of central MR (Figure S1A). Loggerheads also used the Mexican Caribbean to migrate north and southwards from the WC basin to the southern MR and finally Nicaragua (Figure S2A). Others moved northward, crossed the Loop Current, and established in southwestern Florida (Figure 2). A primary aggregation hotspot was detected for immature loggerheads in central-southern Veracruz (Figure S3).
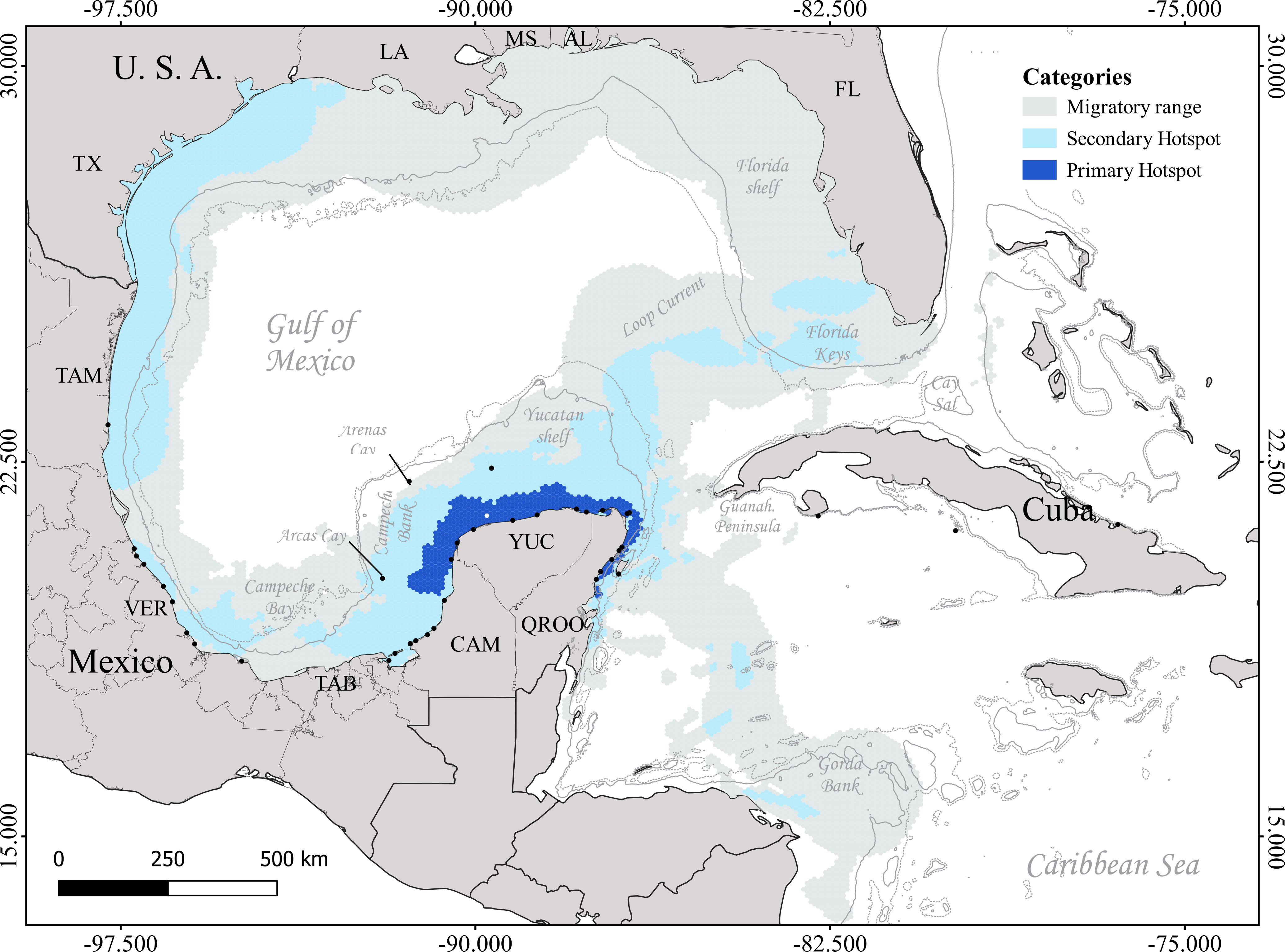
Figure 2 Migratory corridor hotspots used by the breeding females of the four marine turtle species satellite tracked from Mexico and Cuba. Continuous thin gray line depicts the 200 m isobath, and the dashed thin line represents the 500 m isobath (https://www.gebco.net/). Black dots represent the releasing sites of the satellite-tracked individuals.
With more spatially restricted aggregation habitats, green breeding females established their primary and secondary aggregation hotspots in coastal zones, mainly in the northwestern and northeastern Yucatan Peninsula (YP) in Mexico and the Florida Keys (Figure 1B). Interestingly, all tracked individuals breeding at isolated cays in the Campeche Bank ultimately established at continental residency hotspots around the YP (Figure 1A). This species had the smallest internesting and feeding/residency hotspots among the four species (Figures 1B, S1B). These hotspots received individuals from all Mexican GoM-MR states, as well as Cuba. These populations used a migratory corridor in the Campeche Bay in Mexico to reach their aggregation hotspots (Figure S2). The four species also used the major primary migratory hotspot in southern GoM in the northern YP, Mexico (Figure 2). This corridor is used by turtles to move from western GoM to the hotspots in Campeche Bank, northern MR, WC (Gorda Bank), and Florida Keys. A single dispersion route was demonstrated by an immature individual moving from central Mexican Caribbean to Cuba (Guanahacabibes Peninsula), but the other tracked individual stayed locally and established at Laguna de Terminos, Campeche (Figure S3A).
For the critically endangered hawksbills, the studied breeding female populations had well-defined neritic aggregation primary and secondary hotspots (Figure 1C). The western and northeastern coasts of the YP, along with central-southern Veracruz and the southern littoral of Cuba, harbored the main internesting hotspots (Figure S1A). The western and northeastern coasts of the YP are key feeding and residency hotspots for this species (Figure S1B), along with the Florida Keys and northern Gorda Bank, where some individuals migrated to establish (Figure 1C). Breeding males had aggregation hotspots adjacent or overlaying those of females both in Mexico and Cuba. Two individuals showed migratory movements from northern MR to Belize and Guatemala, and one male moved from southwestern Cuba towards Jamaica and the Lesser Antilles (Figure S3). These populations primarily moved through four migratory hotspots, including the main primary migratory corridor around the YP, which connected the western and eastern breeding populations with their feeding and residency hotspots at the Yucatan shelf and the Mexican Caribbean (Figure S2C). This major corridor was also used by individuals to move from Mexican beaches towards MR and WC (Figure 2), some traveling northward and crossing the Loop Current to reach the Florida Keys and Cuba, and some others moving from GoM to southern MR (Belize) and WC (Gorda Bank). Individuals from Cuba connected to the southward route to reach the MR region (northern Honduras) and Gorda Bank (Figure S2C), and one of them reaching Colombia, out of our study area. There was no record of any hawksbill individuals moving from southern to northern GoM.
Adult Kemp’s ridley breeding females stayed inside the GoM (Figure 1D), moving from their primary nesting beaches, Rancho Nuevo and central Veracruz, Mexico, to northern (Louisiana), northeastern (southern Florida shelf), and southeastern (Caribbean corner of the Yucatan Peninsula) GoM, where they foraged and established to reside after their reproductive season (Figure S1B). They used the western GoM as a primary migratory corridor to move towards their residency hotspots in north and south gulf (Figure S2D). Moreover, we did not obtain evidence of any individual moving out of this basin. They migrated remarkably close to the shore along the Mexican and US littorals, also using the main migratory hotspot around the YP to reach their foraging and residency hotspots in the Yucatan shelf and northern MR (Figure 2).
The final weighted integration of all species and life stages revealed four multispecies hotspots. From north to south, the coast of Louisiana is an important marine turtle hotspot, mainly because of the presence of L. kempii (Figure 3). The waters in southwestern Florida harbor primary and secondary hotspots for C. mydas, E. imbricata, and L. kempii. The Veracruz Reef System marine protected area and its adjacent waters in southwestern GoM are primary hotspots for C. caretta and E. imbricata, including resident immature individuals of C. caretta. The YP coast harbors important feeding primary hotspots and is also the corridor for the individuals of the four species coming from western, southern, and eastern GoM and Cuba (Figure 3). These waters were defined as a primary hotspot for marine turtles in the GoM and WC, as they harbor major residency and migratory hotspots, representing the main corridor in the south GoM for at least four species of endangered marine turtles.
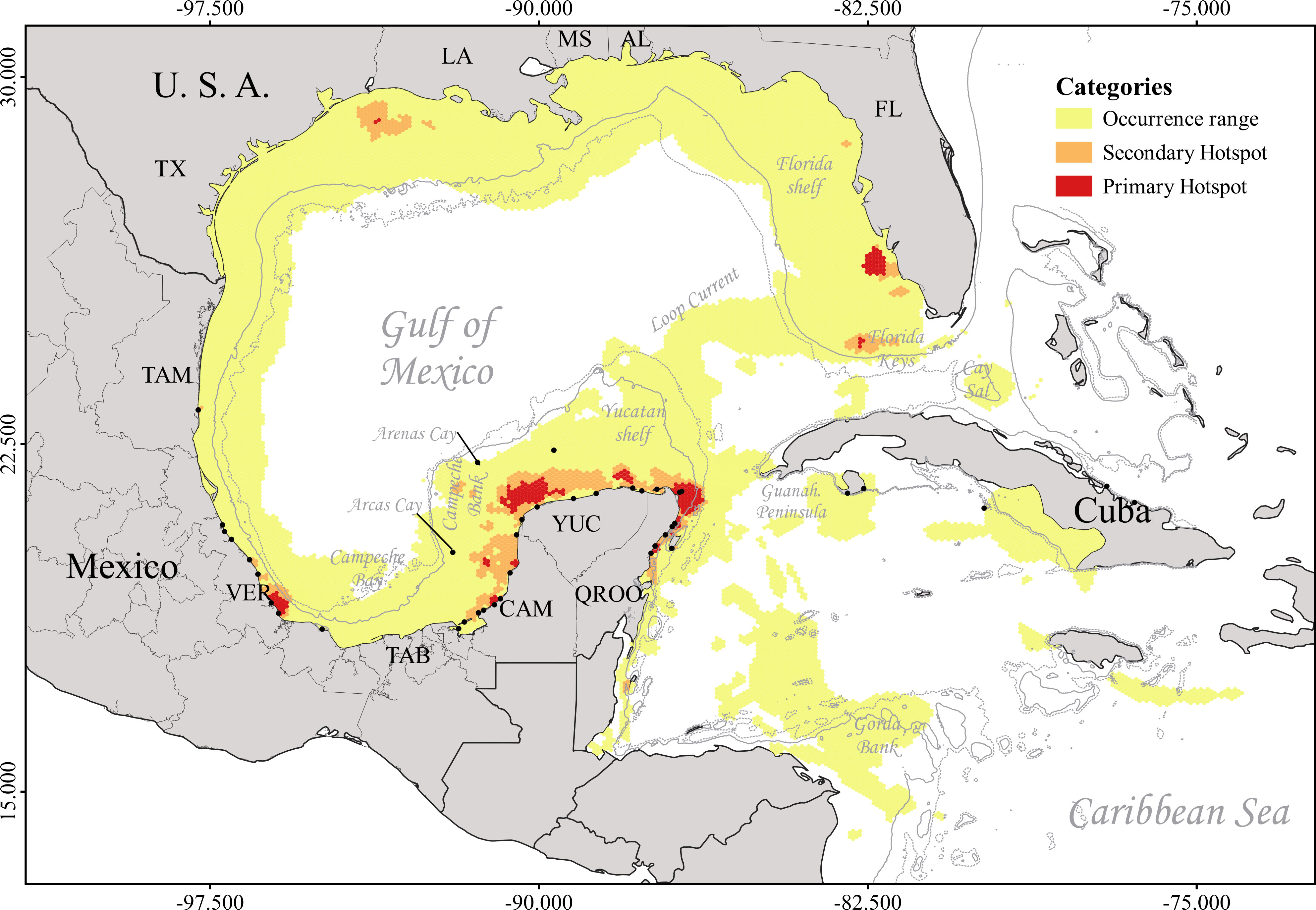
Figure 3 Multispecies and multi-life stage hotspots in the Gulf of Mexico and Mesoamerican Reef. Continuous thin gray line depicts the 200 m isobath, and the dashed thin line depicts the 500 m isobath (https://www.gebco.net/). Black dots represent the releasing sites of the satellite-tracked individuals.
Discussion
While numerous studies have satellite tracked marine turtle populations in major Mexican and Cuban rookeries, this is the first integral study that combined dispersed spatial distribution knowledge of four marine turtle species into advanced outputs that delimited the hotspots of these species. This study represents the actual frontier of knowledge for the GoM and WC. Important next steps for this work will be integrating analyses between northern and southern GoM marine turtle satellite tracking studies.
For greens and hawksbills given the number of tracked individuals, these outputs allow us to infer ecological knowledge at a population level (Schofield et al., 2013; Sequeira et al., 2019), highlighting the contribution to scientific knowledge about this imperiled species. Our results also validated some previously reported migratory corridors (e.g., for Kemp’s ridleys, Shaver et al., 2016; Gradzens and Shaver, 2020) and complement aggregation regions for the four marine turtle species (Hart et al., 2012; Hart et al., 2020; Iverson et al., 2020; Uribe-Martínez et al., 2021). The results also complement known migratory trajectories such as the loggerheads moving from north to south GoM (Girard et al., 2009; Hart et al., 2012; Tucker et al., 2014; Hart et al., 2018b). The use of deep oceanic zones in the GoM and WC by adult leatherbacks (Dermochelys coriacea) and younger life-stages of the other species during their oceanic dispersal, complements the intense and extended use of the study area (Putman and Mansfield, 2015; Aleksa et al., 2018; Putman et al., 2020; Evans et al., 2021; Sasso et al., 2021).
However, we also detected new multispecies and multi-life stage hotspots that expand our understanding of the spatial ecology of marine turtles in the GoM and WC. This new contribution highlights the need for prioritizing the identification of information gaps for specific rookeries and life stages, such as males and immature individuals during their ‘lost years’ (Cuevas et al., 2020; Mansfield et al., 2021), as well as the study of local scale movement ecology associated with the habitats they occupy.
The novel contributions of this study include the first report of a primary aggregation hotspot for immature loggerheads in southern Veracruz, where they reside and develop, despite the fact that major nesting beaches are far away. Further research on the origin and ontogeny of these individuals is needed. Moreover, the delineation of migratory hotspots, which may be considered as soft mesoscale structures, strengthens the research on biogeographical processes in terms of the genetic diversity and plasticity of these species (Harrison et al., 2018). These hotspots facilitate the interchange of rookeries between inner GoM and WC, with a key role of ocean currents, which has been suggested to be an evolutionary strategy to sustain population diversity and provide viability for restoring their populations regionally (Roberts et al., 2004; Stiebens et al., 2013; González-Garza et al., 2015; Carreras et al., 2018; Clusa et al., 2018; Labastida-Estrada et al., 2018; van der Zee et al., 2021).
The extended use of the GoM, MR, and WC by marine turtles exposes them to several negative interactions with natural and anthropogenic threats (Hart et al., 2018a; Cuevas et al., 2019). In particular, the fishing and energy (hydrocarbons and wind) industries are two of the highest threats of concern because of their severity of impacts on marine turtle populations and their habitats, as well as for its extensive occurrence in the GoM and WC (Wallace et al., 2010; Wallace et al., 2017; Scales et al., 2018; Wallace et al., 2020). Outlining marine turtle hotspots is a milestone for a strong national preparedness system, including multiple governmental and private sectors that use such spatially explicit knowledge, as they are required to provide information for decision support on extractive and non-extractive anthropic activities that threaten marine turtle integrity.
In particular, financing assessment processes for the oil industry operation, and soon for the leasing fields for wind energy projected for northern1 and southern GoM2, acknowledge the hotspots of endangered species and their habitats to evaluate and specify mitigation and compensation actions that must be accomplished according to international standards (International Finance Corporation, 2019). Such risk assessments always demand the most updated scientific information and expertise knowledge to accomplish robust and responsible biodiversity management and investment plans. Thus, our results provide the knowledge for making these decisions.
In addition, harmful industrial and fishing practices (e.g., not using turtle excluder devices, TEDs) affect marine turtle species and have serious socioeconomic and political consequences. In 2010 and 2021, the U.S. government withdrew Mexican shrimp importation certification because Mexico’s TED program did not meet the standards established under Section 609 of United States Public Law 101-162 (Platt, 2010; U. S. Department of State, 2021). Although these embargos were temporary, they prevented an income of approximately 90-300 million dollars per year and affected approximately 8,600 jobs. The strategic spatially explicit information presented in this study is key for potential interaction assessments between marine turtles and fishing, and it contributes sustaining more robust plans fostering responsible fishing.
This contextual knowledge configures the foundation scaffold to balance the exploitation and conservation of marine territories while respecting the essential biological and ecological attributes that sustain the resilience capabilities of marine ecosystems. Thus, expeditious and effective management actions must be implemented to secure ocean health. Our results support local and regional decision making, as well as national and international politics, as they extend the frontiers of knowledge on spatial ecology of marine turtles in the GoM and WC, keeping at front the premise of fostering interdisciplinary, intersectoral, and multinational work to properly cope with the scale of the biological process that sustains the long-term viability of these species and their key ecosystem functions. Finally, this paper supports several research lines on marine turtles at multiple temporal and spatial scales and emphasizes the need to continue the path for translating this scientific knowledge into public politics in the form of decrees, policies, management regionalization, and conservation plans for the ecosystems in the large Gulf of Mexico and Mesoamerican Reef System.
Data availability statement
The raw data presented in the figures supporting the conclusions of this article will be made available by the authors, without undue reservation.
Ethics statement
The animal study was reviewed and approved by the Direccón General de Vida Silvestre, SEMARNAT, permit: SGPA/DGVS/01756/07, SGPA/DGVS/02085/11, SGPA/DGVS/04530/11, SGPA/DGVS/04795/13, SGPA/DGVS/09583/15, SATI/PC/016/16, SGPA/DGVS/05560/16, SGPA/DGVS/06438/17, SGPA/DGVS/03989/17, and SGPA/DGVS/6159/19.
Author contributions
Conceptualization, EC, AU-M and MAL-C; methodology, EC, AU-M, ML-C and SG-F; software, AU-M. Validation, FM-G, RG-D-M, VG-H and ML-C. Formal analysis, AU-M, EC and SG-F. Investigation, EC, AU-M, SG-F, MAL-C, VG-H and FM-G. Resources, MAL-C, EC, AU-M and SG-F. Data curation, AU-M, SG-F, EC, RG-D-M and FM-G; writing—original draft preparation, EC, AU-M and MAL-C. Writing—review and editing, SG-F, ML-C, VG-H, FM-G, RG-D-M and MAL-C. Visualization, EC and AU-M; supervision, EC, AU-M and ML-C. Project administration, EC, ML-C and AU-M. Funding acquisition, EC, ML-C, AU-M, FM-G, RG-D-M and MAL-C. All authors contributed to manuscript revision, read, and approved the submitted version.
Funding
Data from Marine turtle critical habitats project was funded by the Mexican National Council for Science and Technology – Mexican Ministry of Energy – Hydrocarbon Fund, project 201441. This is a contribution of the Gulf of Mexico Research Consortium (CIGoM). We acknowledge PEMEX’s specific request to the Hydrocarbon Fund to address the environmental effects of oil spills in the Gulf of Mexico, and the funding source did not participate in the design of this assessment. Pronatura Península de Yucatán received funding from the Mexican National Council for Science and Technology - Mexican Ministry of Environment and Natural Resources/FOMIX State of Campeche (projects #107770/# CAMP‐2005‐C01‐046), the National Fish and Wildlife Foundation (#2006-0091-005), Alliance WWF- Carlos Slim Fund, Chelonia, Inc. Cuban data were acquired thanks to the funs provided by Japan Bekko Association, with additional support from Wildlife Management International. The Rancho Nuevo sea turtle tracking project was funded by Petrofac. Special thanks to Wildlife Computers Inc. for providing satellite tags to Laboratorio de Ecología Espacial y del Movimiento.
Acknowledgments
Pronatura Península de Yucatán thanks Satellite Tracking and Analysis Tool (STAT), to all Staff, students and volunteers in its Sea Turtle Conservation Program who collaborated in field work for attaching the tags. To the Directors of the Natural Protected Areas - CONANP where the individuals were tagged. CINVESTAV-CIGoM thanks the project team who contributed to marine turtle tagging: P.A. García-Alvarado, G. Mexicano-Cíntora, A.G. Ortíz-Méndez, D. Espinoza-Puch, E.B. Palafox-Juárez, H. Hernández-Núñez, E.R. Castro-Pineda. We would like to thank the National Commission of Natural Protected Areas that facilitated the field work through the directors of their regional offices (Planicie Costera y Golfo de Mexico, Península de Yucatán), as well as by the directors and staff to the following natural protected areas: APFF Laguna Madre y Delta del Río Bravo, Santuario Playa de Rancho Nuevo, PN Sistema Arrecifal Veracruzano, APFF Sistema Arrecifal Lobos-Tuxpan, RB Los Tuxtlas, APFF Laguna de Términos, RB Los Petenes, RB Ría Celestún, PN Arrecife Alacranes, RB Ría Lagartos, Santuario Playa Río Lagartos, PN Isla Contoy, APFF Yum Balam, RB Caribe Mexicano, PN Arrecifes de Puerto Morelos, PN Tulum, PN Arrecifes de Cozumel, APFF Isla de Cozumel. Also the public and private institutions in charge of biological monitoring of sea turtle nesting who contributed and facilitated the turtle tagging, some staff of the former institutions also collaborated in turtle tagging: Vida Milenaria, Comisión Federal de Electricidad, Universidad Veracruzana, Desarrollo sustentable, Grupo Tortuguero de la Laguna, Universidad Autónoma del Carmen, Universidad Autónoma de Campeche, Quelonios, Estación de Investigación Oceanográfica del Carmen, 7a y 9a Zonas Navales de la Secretaría de Marina Armada de Mexico, SEMABICC-Gobierno de Campeche, Fileteras del Petén, Gobierno de Yucatán, Fundación Palace, Dorado Royale, H. Municipio de Solidaridad, Hotel Paradisus, Fundación de Parques y Musesos de Cozumel Quintana Roo, Karisma, Centro Ecológico Akumal, Fundación Bahía Príncipe, H. Ayuntamiento de Tulum, Flora, Fauna y Cultura de Mexico, Parque Xcaret. We acknowledge the Reviewers for the suggestions to improve the manuscript. We thank the Headship of the Departamento de Recursos del Mar del CINVESTAV for its financial help to publish this article.
Conflict of interest
The authors declare that the research was conducted in the absence of any commercial or financial relationships that could be construed as a potential conflict of interest.
Publisher’s note
All claims expressed in this article are solely those of the authors and do not necessarily represent those of their affiliated organizations, or those of the publisher, the editors and the reviewers. Any product that may be evaluated in this article, or claim that may be made by its manufacturer, is not guaranteed or endorsed by the publisher.
Supplementary material
The Supplementary Material for this article can be found online at: https://www.frontiersin.org/articles/10.3389/fmars.2022.1059678/full#supplementary-material
Footnotes
- ^ https://www.boem.gov/newsroom/press-releases/boem-initiates-environmental-assessment-offshore-wind-gulf-mexico
- ^ https://ibemar.net/estas-son-las-dos-areas-que-se-estan-considerando-para-los-parques-eolicos-del-golfo-de-mexico/
References
Aleksa K. T., Sasso C. R., Nero R. W., Evans D. R. (2018). Movements of leatherback turtles (Dermochelys coriacea) in the gulf of Mexico. Mar. Biol. 165, 158. doi: 10.1007/s00227-018-3417-9
Bass D., Anderson P., De Silva N. (2011). Applying thresholds to identify key biodiversity areas for marine turtles in Melanesia. Anim. Conserv. 14, 1–11. doi: 10.1111/j.1469-1795.2010.00385.x
Bax N. J., Cleary J., Donnelly B., Dunn D. C., Dunstan P. K., Fuller M., et al. (2015). Results of efforts by the convention on biological diversity to describe ecologically or biologically significant marine areas. Conserv. Biol. 30 (3), 571–581. doi: 10.1111/cobi.12649
Bjorndal K. A., Jackson J. B. C. (2003). “Roles of sea turtles in marine ecosystems: reconstructing the past” in The biology of Sea turtles, vol. Vol. II. (CRC Press Boca Raton, FL.).
Bolten A. B., Crowder L. B., Dodd M. G., MacPherson S. L., Musick J. A., Schroeder B. A., et al. (2011). Quantifying multiple threats to endangered species: an example from loggerhead sea turtles. Front. Ecol. Environment. 9 (5), 295–301. doi: 10.1890/090126
Boyd J. D., Brightsmith D. J. (2013). Error properties of Argos satellite telemetry locations using least squares and kalman filtering. PloS One 8, e63051. doi: 10.1371/journal.pone.0063051
Caro T. M., O’Doherty G. (1999). On the use of surrogate species in conservation biology. Conserv. Biol. 13, 805–814. doi: 10.1046/j.1523-1739.1999.98338.x
Carreras C., Pascual M., Tomás J., Marco A., Hochscheid S., Castillo J. J., et al. (2018). Sporadic nesting reveals long distance colonization in the philopatric loggerhead sea turtle (Caretta caretta). Sci. Rep. 8, 1435. doi: 10.1038/s41598-018-19887-w
Ceriani S. A., Weishampel J. F., Ehrhart L. M., Mansfield K. L., Wunder M. B. (2017). Foraging and recruitment hotspot dynamics for the largest Atlantic loggerhead turtle rookery. Sci. Rep. 7, 16894. doi: 10.1038/s41598-017-17206-3
Clusa M., Carreras C., Cardona L., Demetropoulos A., Margaritoulis D., Rees A. F., et al. (2018). Philopatry in loggerhead turtles caretta caretta beyond the gender paradigm. Mar. Ecol. Prog. Series. 588, 201–213. doi: 10.3354/meps12448
Cuevas E., Abreu-Grobois F., Guzmán-Hernández V., Liceaga-Correa M., van Dam R. (2008). Post-nesting migratory movements of hawksbill turtles eretmochelys imbricata in waters adjacent to the Yucatan peninsula, Mexico. Endangered Species Res. 10, 123–133. doi: 10.3354/esr00128
Cuevas E., Guzmán-Hernández V., Uribe-Martínez A., Raymundo-Sánchez A., Herrera-Pavón R. (2018). Identification of potential sea turtle bycatch hotspots using a spatially explicit approach in the Yucatan peninsula, Mexico. Chelonian Conserv. Biol. 17, 78–93. doi: 10.2744/CCB-1263.1
Cuevas E., Liceaga-Correa M., Uribe-Martínez A. (2019). Ecological vulnerability of two sea turtle species in the gulf of Mexico: An integrated spatial approach. Endangered Species Res. 40, 337–356. doi: 10.3354/esr00984
Cuevas E., Putman N. F., Uribe-Martínez A., López-Castro M. C., Guzmán-Hernández V., Gallegos-Fernández S. A., et al. (2020). First spatial distribution analysis of male sea turtles in the southern gulf of Mexico. Front. Mar. Science. 7. doi: 10.3389/fmars.2020.561846
Edgar G. J., Brooks T. M. (2011). Testing absolute and percentage thresholds in the identification of key biodiversity areas. Anim. Conserv. 14, 12–13. doi: 10.1111/j.1469-1795.2011.00438.x
Evans A., Valveree R. A., Ordoñez C., Carthy R. R. (2021). Identification of the gulf of Mexico as an important high-use habitat for leatherback turtles from central America. Ecosphere. 12 (8), e03722. doi: 10.1002/ecs2.3722
FitzSimmons N. N., Limpus C. J., Norman J. A., Goldizen A. R., Miller J. D., Moritz C. (1997). Philopatry of male marine turtles inferred from mitochondrial DNA markers. Proc. Natl. Acad. Sci. 94 (16), 8912–8917. doi: 10.1073/pnas.94.16.8912
Freitas C., Lydersen C., Ims R. A., Fedak M. A., Kovacs K. M. (2012). A simple new algorithm to filter marine mammal Argos locations. Mar. Mammal Science. 24, 315–325. doi: 10.1111/j.1748-7692.2007.00180.x
Gaos A. R., Lewison R. L., Jensen M. P., Liles M. J., Henriquez A., Chavarria S., et al. (2017). Natal foraging philopatry in eastern pacific hawksbill turtles. R. Soc. Open Science. 4 (8), 170153. doi: 10.1098/rsos.170153
Girard C., Tucker A. D., Calmettes B. (2009). Post-nesting migrations of loggerhead sea turtles in the gulf of Mexico: dispersal in highly dynamic conditions. Mar. Biol. 156, 1827–1839. doi: 10.1007/s00227-009-1216-z
Goatley C. H. R., Hoey A. S., Bellwood D. R. (2012). The role of turtles as coral reef macroherbivores. PloS One 7, e39979. doi: 10.1371/journal.pone.0039979
González-Garza B. I., Stow A., Sánchez-Téllez L. F., Zapata-Pérez O. (2015). Genetic variation, multiple paternity, and measures of reproductive success in the critically endangered hawksbill turtle (Eretmochelys imbricata). Ecol. Evolution. 5 (24), 5758–5769. doi: 10.1002/ece3.1844
Gradzens C., Marsh H., Fuentes M. M. P. B., Limpus C. J., Shimada T., Hamann M. (2014). Satellite tracking of sympatric marine megafauna can inform the biological basis for species co-management. PloS One 9(6), e98944. doi: 10.1371/journal.pone.0098944
Gradzens C., Shaver D. J. (2020). Satellite tracking can inform population-level dispersal to foraging grounds of post-nesting kemp’s ridley sea turtles. Front. Mar. Science. 7. doi: 10.3389/fmars.2020.00559
Hammerschlag N., Schmitz O. J., Flecker A. S., Lafferty K. D., Sih A., Atwood T. B., et al. (2019). Ecosystem function and services of aquatic predators in the anthropocene. Trends Ecol. Evol. 34, 369–383. doi: 10.1016/j.tree.2019.01.005
Harrison A. L., Costa P. D., Winship A. J., Benson S. R., Bograd S. J., Antolos M., et al. (2018). The political biogeography of migratory marine predators. Nat. Ecol. Evolution. 2, 1571–1578. doi: 10.1038/s41559-018-0646-8
Hart K. M., Iverson A. R., Fujisaki I., Lamont M. M., Bucklin D., Shaver D. J. (2018a). Marine threats overlap key foraging habitat for two imperiled Sea turtle species in the gulf of Mexico. Front. Mar. Science. 5. doi: 10.3389/fmars.2018.00336
Hart K. M., Iverson A. R., Fujisaki I., Lamont M. M., Bucklin D., Shaver D. J. (2018b). Sympatry or syntopy? investigating drivers of distribution and co-occurrence for two imperiled sea turtle species in gulf of Mexico neritic waters. Ecol. Evolution. 8, 12656–12669. doi: 10.1002/ece3.4691
Hart K. M., Lamont M. M., Fujisaki I., Tucker A. D., Carthy R. R. (2012). Common coastal foraging areas for loggerheads in the gulf of Mexico: Opportunities for marine conservation. Biol. Conserv. 145, 185–194. doi: 10.1016/j.biocon.2011.10.030
Hart K. M., Lamont M. M., Iverson A. R., Smith B. J. (2020). The importance of the northeastern gulf of Mexico to foraging loggerhead Sea turtles. Front. Mar. Science. 7. doi: 10.3389/fmars.2020.00330
Hazen E. L., Abrahms B., Brodie S., Carroll G., Jacox M. G., Savoca M. S., et al. (2019). Marine top predators as climate and ecosystem sentinels. Front. Ecol. Environment. 17, 565–574. doi: 10.1002/fee.2125
Hazen E. L., Suryan R. M., Santora J. A., Bograd S. J., Watanuki Y., Wilson R. P. (2013). Scales and mechanisms of marine hotspot information. Mar. Ecol. Prog. Series. 487, 177–183. doi: 10.1111/ddi.12411
Horne J. S., Garton E. O., Krone S. M., Lewis J. S. (2007). Analyzing animal movements using Brownian bridges. Ecology. 88 (9), 2354–2363. doi: 10.1890/06-0957.1
Hunter M., Westgate M., Barton P., Calhoun A., Pierson J., Tulloch A., et al. (2016). Two roles for ecological surrogacy: Indicator surrogates and management surrogates. Ecol. Indicators. 63, 121–125. doi: 10.1016/j.ecolind.2015.11.049
International Finance Corporation (2019) Guidance note 6: Biodiversity conservation and sustainable management of living natural resources. Available at: https://www.ifc.org/wps/wcm/connect/5e0f3c0c-0aa4-4290-a0f8-4490b61de245/GN6_English_June-27-2019.pdf?MOD=AJPERES&CVID=mRQjZva (Accessed September 24, 2022).
Iverson A. R., Benscoter A. M., Fujisaki I., Lamont M. M., Hart K. M. (2020). Migration corridors and threats in the gulf of Mexico and Florida straits for loggerhead Sea turtles. Front. Mar. Science. 7. doi: 10.3389/fmars.2020.00208
Johnson R. A., Gulick A. G., Constant N., Bolten A. B., Smulders F. O. H., Christianen M. J. A., et al. (2020). Seagrass ecosystem metabolic carbon capture in response to green turtle grazing across Caribbean meadows. J. Ecology. 108, 1101–1114. doi: 10.1111/1365-2745.13306
Kalinkat G., Cabral J. S., Darwall W., Ficetola G. F., Fisher J. L., Giling D. P., et al. (2017). Flagship umbrella species needed for the conservation of over-looked aquatic biodiversity: freshwater flagship umbrella species. Conserv. Biol. 31, 481–485. doi: 10.1111/cobi.12813
Labastida-Estrada E., Mackour.M’Rabet S., Díaz-Jaimes P., Cedeño-Vázquez J. R., Hénault Y. (2018). Genetic structure, origin, and connectivity between nesting and foraging areas of hawksbill turtles of the Yucatan peninsula: A study for conservation and management. Aquat. Conservation: Mar. Freshw. Ecosystems., 29(2), 1–12. doi: 10.1002/aqc.2999
Lamont M. M., Putman N. F., Fujisaki I., Hart K. (2015). Spatial requirements of different life-stages of the loggerhead turtle (Caretta caretta) from a distinct population segment in the northern gulf of Mexico. Herpetological Conserv. Biol. 10 (1), 26–43. Available at: http://www.herpconbio.org/Volume_10/Issue_1/Lamont_etal_2015.pdf.
Lascelles B. G., Taylor P. R., Miller M. G. R., Dias M. P., Oppel S., Torres L., et al. (2016). Applying global criteria to tracking data to define important areas for marine conservation. Diversity Distributions. 22, 422–431. doi: 10.1111/ddi.12411
Liceaga-Correa M. A., Uribe-Martínez A., Cuevas E. (2022). Ecological vulnerability of marine turtles as indicators of opportunities for regional socioecosystem management in the southern gulf of Mexico. Sustainability. 14, 184. doi: 10.3390/su14010184
Liu Y., Battaile B. C., Trites A. W., Zidek J. V. (2015). Bias correction uncertainty characterization of dead-reckoned paths of marine mammals. Anim. Biotelemetry. 3, 51. doi: 10.1186/s40317-015-0080-5
Lopez R., Malardé J. P. R., Gaspar P. (2013). Improving Argos doppler location using multiple-model kalman filtering. IEEE Trans. Geosci. Remote Sensing. 52, 4744–4755. doi: 10.1109/TGRS.2013.2284293
Mansfield K. L., Wyneken J., Luo J. (2021). First Atlantic satellite tracks of ‘lost years’ green turtles support the importance of the Sargasso Sea as a sea turtle nursery. Proc. R. Soc. B. 288 (1950), 20210057. doi: 10.1098/rspb.2021.0057
McConnell B. J., Chambers C., Fedak M. A. (1992). Foraging ecology of southern elephant seals in relation to the bathymetry and productivity of the southern ocean. Antarctic Science. 4, 393–398. doi: 10.1017/S0954102092000580
Moncada-Gavilán F. (2005). Migración de la tortuga verde (Chelonia mydas), la tortuga caguama (Caretta caretta) y la tortuga carey (Eretmochelys imbricata), en la plataforma cubana y aguas adyacentes (La Habana, Cuba: Centro de Investigaciones Biológicas del Noroeste, S. C).
Moncada-Gavilán F., Hawkes L. A., Fish M. R., Godley B. J., Manolis S. C., Medina Y., et al. (2012). Patterns of dispersal of hawksbill turtles from the Cuban shelf inform scale of conservation and management. Biol. Conserv. 148, 191–199. doi: 10.1016/j.biocon.2012.01.011
Nielson R. M., Sayer H., McDonald T. (2014) Brownian Bridge movement model (WEST, Inc.). Available at: https://cran.microsoft.com/2014-09-08/packages/BBMM/FBBMM.pdf (Accessed September 14, 2022).
North M. A. (2009). “A method for implementing a statistically significant number of data classes in the jenks algorithm,” in FSKD 2009: Sixth international conference on fuzzy systems and knowledge discovery. Eds. Chen Y., Deng H., Zhang D., Xzio Y. (Tianjin: IEEE Computer Society), 35–38. doi: 10.1109/FSKD.2009.319
Papastamatiou Y. P., Meyer C. G., Carvalho F., Dale J. J., Hutchinson M. R., Holland K. N. (2013). Telemetry and randmo-walk models reveal complex patterns of partial migration in a large marine predator. Ecology. 94 (11), 2595–2606. doi: 10.1890/12-2014.1
Pilcher N. J., Antonopoulou M., Perry L., Abdel-Moati M. A., Al Abdessalaam T. Z., Baldwin R., et al. (2014). Identification of important Sea turtle areas (ITAs) for hawksbill turtles in the Arabian region. J. Exp. Mar. Biol. Ecology. 460, 89–99. doi: 10.1016/j.jembe.2014.06.009
Platt J. (2010) Shell shock: U. s. state department bans shrimp imports from Mexico to protect endangered turtles (Scientific American). Available at: https://blogs.scientificamerican.com/extinction-countdown/shell-shock-u-s-state-department-bans-shrimp-imports-from-mexico-to-protect-endangered-turtles/ (Accessed September 24, 2022).
Putman N. F., Mansfield K. L. (2015). Direct evidence of swimming demonstrates active dispersal in the sea turtle “Lost years”. Curr. Biol. 25, 1221–1227. doi: 10.1016/j.cub.2015.03.014
Putman N. F., Seney E. E., Verley P., Shaver D. J., López-Castro M. C., Cook M., et al. (2020). Predicted distributions and abundances of the sea turtle ‘lost years’ in the western north Atlantic ocean. Ecography. 43, 506–517. doi: 10.1111/ecog.04929
Roberts M. A., Schwartz T. S., Karl S. A. (2004). Global population genetic structure and male-mediated gene flow in the green sea turtle (Chelonia mydas): Analysis of microsatellite loci. Genetics. 166, 1857–1870. doi: 10.1093/genetics/166.4.1857
Robinson N. J., Morreale S. J., Nel R., Paladino F. V. (2016). Coastal leatherback turtles reveal conservation hotspot. Sci. Rep. 6, 37851. doi: 10.1038/srep37851
Saaty T. L. (2008). Decision making with the analytic hierarchy process. Int. J. Serv. Sci. 1, 83–98. doi: 10.1504/IJSSCI.2008.017590
Sánchez-Salas J., Muro G., Estrada-Castillón E., Alba-Ávila J. A. (2013). El MER: Un instrumento para evaluar el riesgo de extinción de especies en Mexico. Rev. Chapingo Serie Zonas Áridas. XII (1), 30–35. doi: 10.5154/r.rchsza.2012.06.037
Sasso C. R., Richards P. M., Benson S. R., Judge M., Putman N. F., Snodgrass D., et al. (2021). Leatherback turtles in the Eastern gulf of Mexico: Foraging and migration behavior during the autumn and winter. Front. Mar. Science. 8. doi: 10.3389/fmars.2021.660798
Scales K. L., Hazen E. L., Jacox M. G., Castruccio F., Maxwell S. M., Lewison R. L., et al. (2018). Fisheries bycatch risk to marine megafauna is intensified in Lagrangian coherent structures. PNAS. 115 (28), 7362–7367. doi: 10.1073/pnas.1801270115
Schofield G., Dimadi A., Fossette S., Katselidis K. A., Koutsoubas D., Lilley M. K. S., et al. (2013). Satellite tracking large numbers of individuals to infer population level dispersal and core areas for the protection of an endangered species. Diversity Distributions. 19, 834–844. doi: 10.1111/ddi.12077
Schofield G., Lilley M. K. S., Bishop C. M., Brown P., Katselidis K. A., Dimopoulos P., et al. (2009). Conservation hotspots: implications of intense spatial area use by breeding male and female loggerheads at the mediterranean’s largest rookery. Endangered Species Res. 10, 191–202. doi: 10.3354/esr00137
Sequeira A. M. M., Heupel M. R., Lea M. A., Eguíluz V. M., Duarte C. M., Meekan M. G., et al. (2019). The importance of sample size in marine megafauna tagging studies. Ecol. Appl. 29 (6), e01947. doi: 10.1002/eap.1947
Shaver D. J., Hart K. M., Fujisaki I., Rubio C., Sartain-Iverson A. R., Peña J., et al. (2016). Migratory corridors of adult female kemp’s ridley turtles in the gulf of Mexico. Biol. Conserv. 194, 158–167. doi: 10.1016/j.biocon.2015.12.014
Shaver D. J., Hart K. M., Fujisaki I., Rubio C., Sartain A. R., Pena J., et al. (2013). Foraging area fidelity for kemp's ridleys in the gulf of Mexico. Ecol. Evolution. 3 (7), 2002–2012. doi: 10.1002/ece3.594
Silva I., Crane M., Marshall B. M., Strine C. T. (2020). Reptiles on the wrong track? moving beyond traditional estimators with dynamic Brownian bridge movement models. Movement Ecology. 8, 43. doi: 10.1186/s40462-020-00229-3
Stiebens V. A., Merino S. E., Roder C., Chain F. J. J., Lee P. L. M., Eizaguirre C. (2013). Living on the edge: how philopatry maintains adaptive potential. Proc. R. Soc. B. 280, 20130305. doi: 10.1098/rspb.2013.0305
Stokes K. L., Broderick A. C., Canbolat A. F., Candan O., Fuller W. J., Glen F., et al. (2015). Migratory corridors and foraging hotspots: critical habitats identified for Mediterranean green turtles. Diversity Distributions. 21, 665–674. doi: 10.1111/ddi.12317
Tucker A. D., MacDonald B., Seminoff J. A. (2014). Foraging site fidelity and stable isotope values of loggerhead turtles tracked in the gulf of Mexico and northwest Caribbean. Mar. Ecol. Prog. Series. 502, 267–279. doi: 10.3354/meps10655
Uribe-Martínez A., Liceaga-Correa M., de los A., Cuevas E. (2021). Critical in-water habitats for post-nesting Sea turtles from the southern gulf of Mexico. J. Mar. Sci. Engineering. 9, 793. doi: 10.3390/jmse9080793
U. S. Department of State (2021) Notice of annual certification (Bureau of Oceans and International Environmental and Scientific Affairs). Available at: https://www.govinfo.gov/content/pkg/FR-2021-04-30/pdf/FR-2021-04-30.pdf (Accessed September 24, 2022). Annual Certification of Shrimp-Harvesting Nations. Public Notice: 11419.
van der Zee J. P., Christianen M. J. A., Bérubé M., Nava M., Schut K., Humber F., et al. (2021). The population genomic structure of green turtles (Chelonia mydas) suggests a warm-water corridor for tropical marine fauna between the Atlantic and Indian oceans during the last interglacial. Heredity. 127, 510–521. doi: 10.1038/s41437-021-00475-0
Wabnitz C., Balazs G., Beavers S., Bjorndal K., Bolten A., Christensen V., et al. (2010). Eco-system structure and processes at kaloko honokohau, focusing on the role of herbivores, including the green Sea turtle chelonia mydas, in reef resilience. Mar. Ecol. Prog. Series. 420, 27–44. doi: 10.3354/meps08846
Wallace B. P., Lewison R. L., McDonald S. L., McDonald R. K., Kot C. Y., Kelez S., et al. (2010). Global patterns of marine turtle bycatch. Conserv. Letters. 3, 131–142. doi: 10.1111/j.1755-263X.2010.00105.x
Wallace B. P., Stacy B. A., Cuevas E., Holyoake C., Lara P. H., Marcondes A. C., et al. (2020). Oil spills and sea turtles: documented effects and considerations - for response and assessment efforts. Endangered Species Res. 41, 17–37. doi: 10.3354/esr01009
Wallace B., Stacy B., Rissing M., Cacela D., Garrison L., Graettinger G., et al. (2017). Estimating Sea turtle exposures to deepwater horizon oil. Endangered Species Res. 33, 51–67. doi: 10.3354/esr00728
Keywords: Migratory corridors, Spatial ecology, Satellite tracking, Conservation management, Eretmochelys imbricata, Chelonia mydas, caretta caretta, lepidochelys kempii
Citation: Cuevas E, Liceaga-Correa MdlA, Uribe-Martínez A, Gallegos-Fernández SA, Moncada-Gavilán F, González-Díaz-Mirón RJ, López-Castro MC, Guzmán-Hernández V and López M (2022) Marine turtle hotspots in the Gulf of Mexico and Mesoamerican Reef: Strengthening management and preparedness. Front. Mar. Sci. 9:1059678. doi: 10.3389/fmars.2022.1059678
Received: 01 October 2022; Accepted: 04 November 2022;
Published: 21 November 2022.
Edited by:
Maria Josefina Olascoaga, University of Miami, United StatesReviewed by:
Nathan Freeman Putman, LGL (United States), United StatesMelissa Cook, Southeast Fisheries Science Center (NOAA), United States
Copyright © 2022 Cuevas, Liceaga-Correa, Uribe-Martínez, Gallegos-Fernández, Moncada-Gavilán, González-Díaz-Mirón, López-Castro, Guzmán-Hernández and López. This is an open-access article distributed under the terms of the Creative Commons Attribution License (CC BY). The use, distribution or reproduction in other forums is permitted, provided the original author(s) and the copyright owner(s) are credited and that the original publication in this journal is cited, in accordance with accepted academic practice. No use, distribution or reproduction is permitted which does not comply with these terms.
*Correspondence: María de los Angeles Liceaga-Correa, bWFyaWEubGljZWFnYUBjaW52ZXN0YXYubXg=; Abigail Uribe-Martínez, YWJpZ2FpbC51cmliZW1hcnRpbmV6QHRhbXVjYy5lZHU=