- 1School of Ocean Sciences, Bangor University, Menai Bridge, United Kingdom
- 2Biosciences, College of Life and Environmental Sciences, University of Exeter, Exeter, United Kingdom
- 3Centre National de la Recherche Scientifique (CNRS), Université de Perpignan, Perpignan, France
- 4Ecocean SAS, Montpellier, France
- 5School of Biological Sciences, University of Bristol, Bristol, United Kingdom
Demand for marine fisheries is rising despite global impacts on the productive capacity of wild fish stocks due to overfishing, habitat loss, and global warming. Fisheries enhancement programs—aimed at augmenting stocks by releasing juveniles into the wild—are expected to play an increasingly important auxiliary role in addressing capture-based fishery limitations into the future. However, concerns exist over the impacts and efficacy of aquaculture-based enhancement (ABE), releasing captive-bred fish into wild populations. An alternative but understudied approach for fisheries enhancement is wild post-larvae capture, culture, and release (PCCR). Here, we provide an overview of the PCCR process, from initial planning to measuring success, providing an overview of its implementation in a viable finfish fishery, the white seabream Diplodus sargus in the Mediterranean. We discuss management application of PCCR-based enhancement and its limitations, highlighting existing knowledge gaps and future research required to realize the full potential of this alternative approach. Notwithstanding some limitations–including limited uptake for full evaluation, some species restrictions, density-dependent mortality, and the remaining open challenge for stock enhancement generally of tracking released fish through to reproduction–PCCR offers potential as a credible auxiliary management tool for fisheries restoration.
1 Introduction
Many wild fish and invertebrate stocks have been critically reduced by environmental degradation and chronic overfishing, limiting the productive capacity of local and global fisheries (Neubauer et al., 2013; Pauly and Zeller, 2016). Complex interacting impacts of habitat loss, declining water quality, increasing ocean temperatures and changing chemistry, species invasions, and overfishing-induced changes in natural food webs and population dynamics combine to threaten the sustainability of marine fisheries (Perry et al., 2005; Neubauer et al., 2013; Iacarella et al., 2019). Climate warming alone is predicted to generate future declines in global fisheries yield by as much as 60% in some regions (Moore et al., 2018). Nonetheless, efforts to meet increasing human demands on fisheries continue. Latest published estimates of global fishery production stand at 178 million tons (Mt) per year, with 90 Mt from capture fisheries and the remaining 88 Mt from aquaculture production (FAO, 2022), although removal through wild capture fisheries likely far exceeds these estimates due to artisanal and unreported catches, and discarded bycatch (Pauly and Zeller, 2016).
Global evidence shows that effective fisheries management is instrumental in improving fish stock status to the point where stocks exceed target levels and can rebuild (Hilborn et al., 2020). There is increasing consensus that to rehabilitate depleted wild stocks and ensure future fisheries production, we must reduce fishing, restore critical habitats and stabilize the climate (Neubauer et al., 2013; Moore et al., 2018). However, maintaining fisheries often requires complementary solutions beyond simply managing production and yield, to account for complex social-ecological contexts (Lorenzen et al., 2010). One such solution is fisheries enhancement, a long-standing, widespread practice concerned with overcoming recruitment limitation, a common life-history bottleneck in many marine species (Lorenzen, 2014). Distinct from restocking (release of juvenile organisms to restore spawning biomass of depleted fish stocks), fisheries enhancement involves the regular release of juveniles to improve self-sustaining populations by augmenting their abundance where natural recruitment is limited (Bell et al., 2006; Taylor et al., 2017). Young, small-bodied marine animals are easily predated, resulting in high juvenile mortality rates (Planes and Lecaillon, 2001; Goatley and Bellwood, 2016). For example, studies of 24 taxonomically diverse species indicate that, on average, 55.7% of post-larval tropical reef fishes are consumed by predators within 1–2 days of settlement to the reef (Almany and Webster, 2006). Therefore, to augment stock abundance beyond natural levels, enhancement programs release reared animals that have grown large enough to avoid the jaws of common predators and are more likely to survive to maturity (Bell et al., 2006; Bartley and Bell, 2008). The practice is supported by well-established, rapidly progressing science that is theoretically and empirically consolidated (Taylor et al., 2017). As a result, scales of releases for enhancement can be vast and some operations report substantial positive cost–benefit ratios (Masuda and Tsukamoto, 1998; Dong et al., 2009).
Typically, fisheries enhancement programs breed and release farmed “seed” as juveniles, but this approach of releasing animals produced from captive broodstock (known as aquaculture-based enhancement; ABE) remains controversial (Bell et al., 2006; Taylor et al., 2017). Despite significant advances in aquaculture techniques, social and economic assessments, ecological modelling and genetic testing, concerns remain about the potential impacts on wild populations through genetic pollution, homogenization, and the spread of disease (Hilborn, 2004; Bell et al., 2006; Lorenzen et al., 2010). An alternative yet understudied approach for enhancement is the harvesting and captive-rearing of wild pelagic post-larvae for re-release as juveniles (known as post-larval capture, culture, and release; PCCR). By catching and keeping local wild post-larvae until the influence of size- and density-dependent processes on survival have subsided, PCCR-based enhancement aims to lessen the risk of altering the gene frequency of wild fish populations associated with homogenized gene profiles and inbreeding via the release of cultured hatchery-seed in ABE. Further, it is generally assumed that PCCR offers a potentially lower cost, theoretically more genetically diverse source of juveniles that are better adapted to natural conditions, and goes some way to reducing disease transmission through reduced time in captivity and fewer relocations during the culture period (Blaxter, 1976; Munro and Bell, 1997; Hair et al., 2002; Bartley and Bell, 2008). The process of PCCR does not, however, likely entirely mitigate concerns over risks to biosecurity and other wider ecological impacts. For example, while captured and cultured local wild post-larvae will have comparable gene frequencies to the wild stock (Munro and Bell, 1997; Hair et al., 2002), some risks remain due to minimized selective processes otherwise experienced during development in the wild, potentially allowing over-representation of certain genotypes compared to natural populations, over-domestication effects which may lead to reduced fitness in the wild, or conversely through selection of certain traits resilient to the trapping, transport and rearing processes associated with PCCR (Lorenzen et al., 2012). Wider ecological impacts include trophic impacts of removal of post-larvae as a valuable component of the food web, and issues of bycatch during post-larvae capture (Hair et al., 2002; Bell et al., 2009). Nonetheless, the capture and culture of wild-caught post-larvae to enhance fisheries has proven successful in several cases, and where done responsibly represents an exciting opportunity to enhance fishery production where local recruitment limitation may be driven by factors such as high mortality and advection of larvae, creating a disconnect between spawning grounds and suitable nursery habitat (Loneragan et al., 2013). Whilst most successful examples of PCCR are for marine invertebrate species (Sadovy de Mitcheson and Liu, 2008; Bell et al., 2009), including scallops in Japan (Uki, 2006), rock lobsters in Australia (Gardner et al., 2006) and spiny lobsters in Vietnam (Tuan et al., 2000), there is increasing interest in the expansion of PCCR techniques to finfish species, with recent examples for mullet in Egypt (Saleh, 2008), trials with reef-fishes in the Philippines (Lecaillon and Lourié, 2007), and for white seabream in the northwest Mediterranean (examined in detail in Tables 1–3). However, few studies have quantified the influence of PCCR on fishery yield, measured its economic benefits over other management approaches, or studied impacts on naturally recruited individuals in the enhanced stock (Lorenzen et al., 2010). This is likely partly due to the scale of operations trialed, the stock enhancement challenge of tracking released fish through to reproduction, and, until recently, a greater focus on the advancement of rearing techniques than for systematic planning, monitoring and quantitative assessment in the context of management goals (Lorenzen, 2014).
Here, we synthesize the existing literature to examine the use of PCCR and its application to marine fisheries enhancement. We broadly follow the widely accepted principles for responsible marine stock enhancement from initial appraisal and goal-setting through to operational implementation and requirement for adaptive management (Blankenship and Leber, 1995; Lorenzen et al., 2010). Specifically, we highlight ‘best practice’ for three key phases: 1) identifying the context-specific need for PCCR intervention; 2) PCCR process of post-larval capture, culture, and release; and 3) measuring ‘success’. For each of these three phases we examine a PCCR program in the northwest Mediterranean for the white seabream Diplodus sargus (Tables 1–3; Figures 1–3), drawing on additional data from ongoing projects currently trialing the methods across sixty species in the region (Table S1). Finally, we summarize management implications, identify current and future limitations, and propose areas for further research.
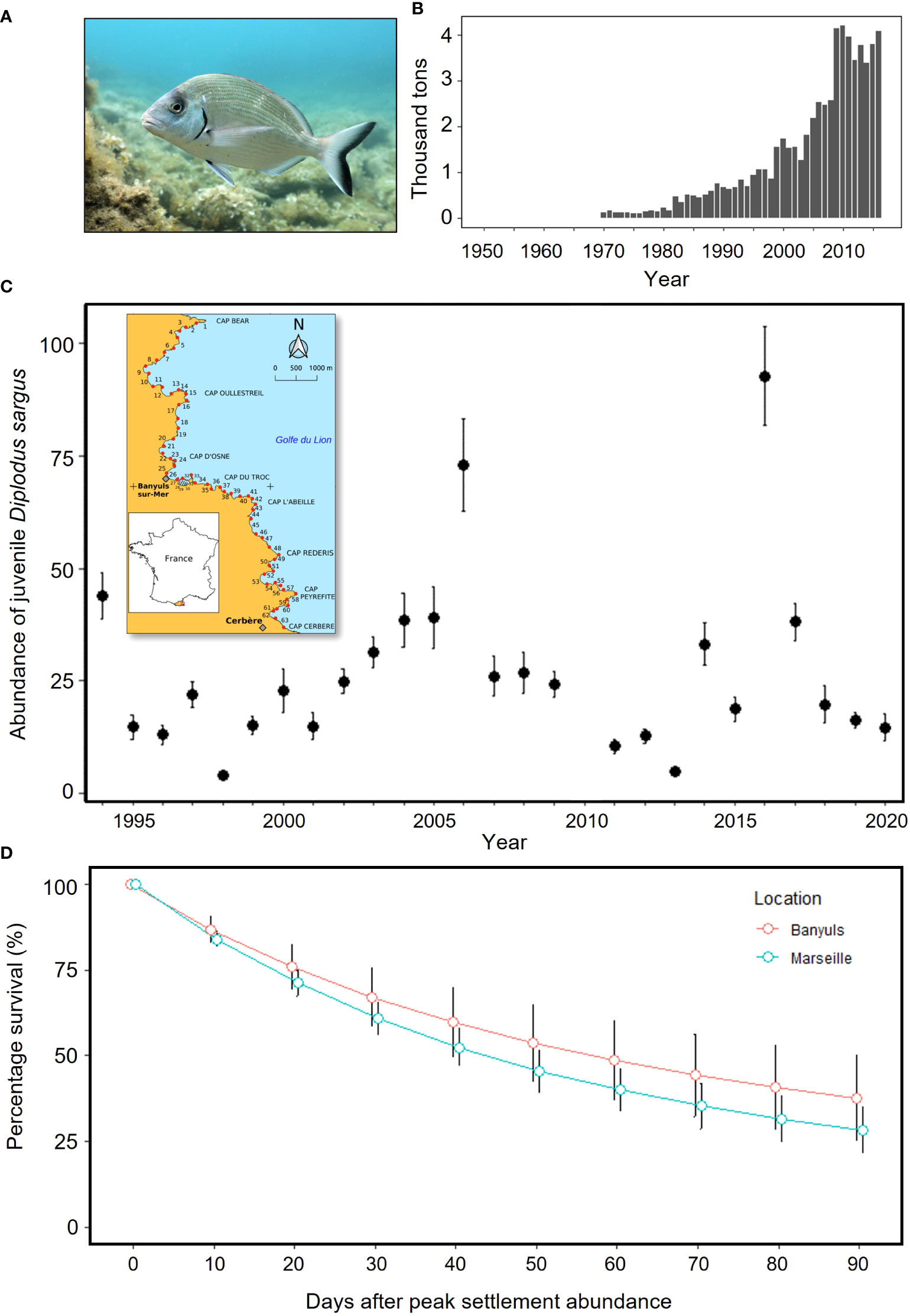
Figure 1 (A) Adult white seabream Diplodus sargus; photo: © Virginie Hartmann; (B) FAO global capture production estimates of D sargus (FAO, 2021); (C) local mean abundance ± SE of juvenile D sargus per section surveyed within nursery habitat in French Mediterranean coastline (see inset map with numbered sections); (D) local survival rates (mean ± SE) of D sargus in Banyuls and Marseille, NW Mediterranean, after peak settlement abundance (data replotted from Macpherson et al., 1997).
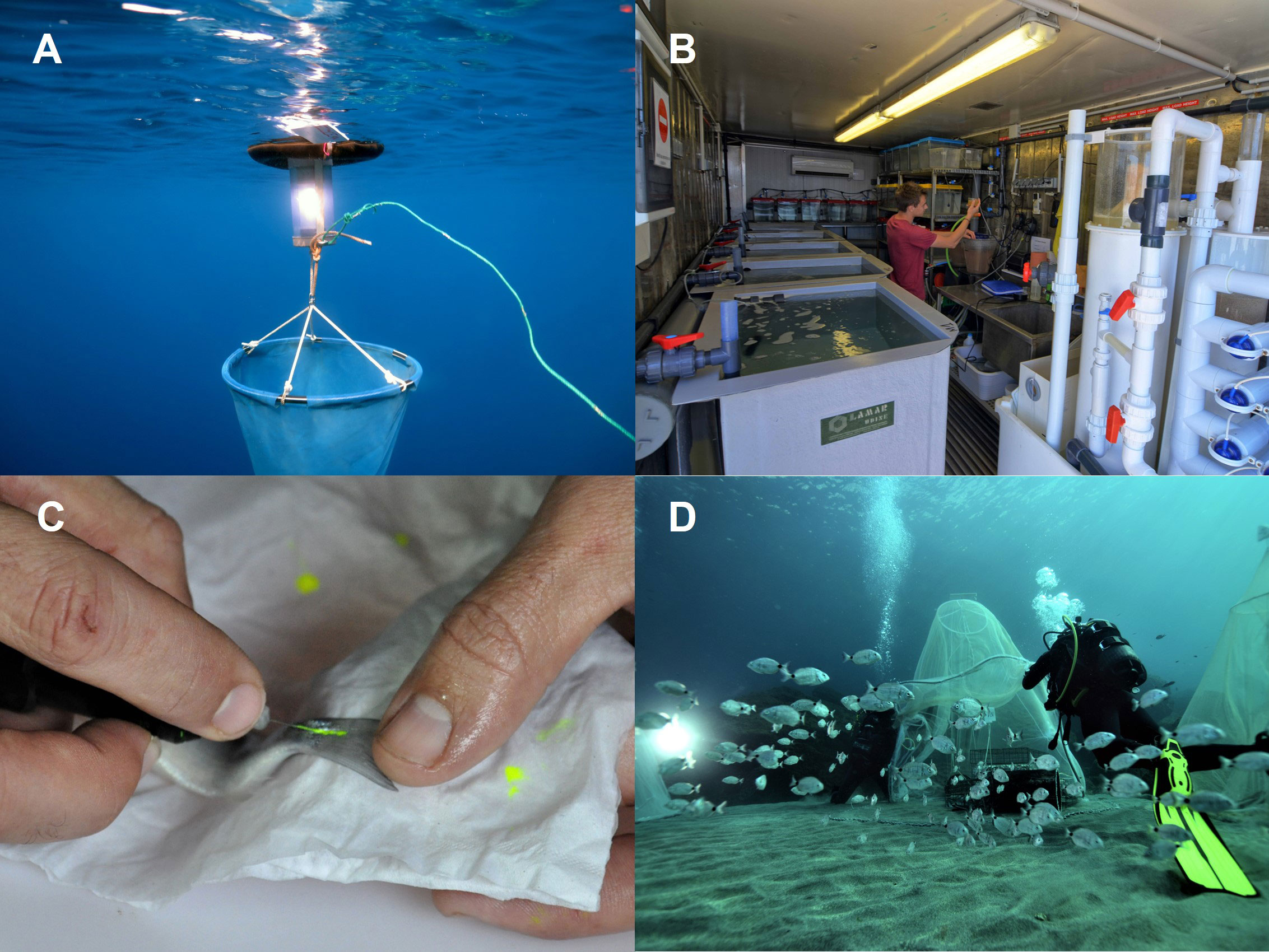
Figure 2 (A) Capture of wild post-larvae with light traps; (B) culture of wild-caught post-larvae in aquaria; (C) pre-release tagging with elastomer implant at the base of the tail fin; (D) staggered release of juveniles using tents for initial acclimation to enhance survival rates. Photos: © Rémy Dubas.
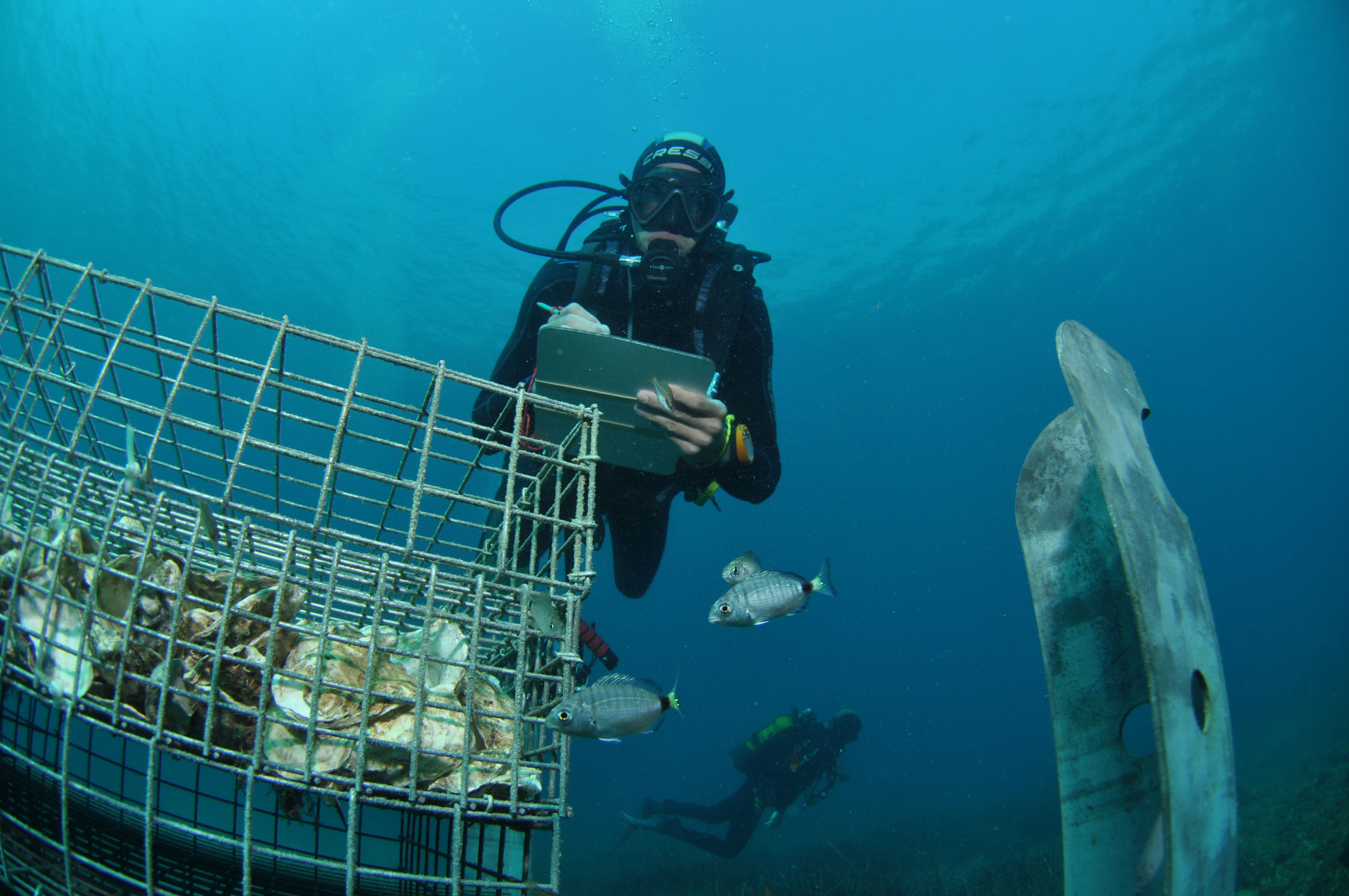
Figure 3 Monitoring survival of tagged and released Diplodus sargus with underwater visual surveys. Photo: © Rémy Dubas.
2 Post-larval capture, culture, and release for fisheries enhancement: A three-phase process
Fisheries enhancement is likely best-suited to coastal species that start life as pelagic larvae before settling to shallow inshore habitats where they remain site-attached as juveniles and adults, creating self-replenishing populations (Bell et al., 2006; Lorenzen et al., 2010). The success of fisheries enhancement programs generally, and of PCCR specifically, in contributing to target stocks is thus likely influenced by a species’ life history traits (Garlock et al., 2017). PCCR may therefore not be effective for largely pelagic, migratory finfish taxa such as tunas and sharks in open populations, where juveniles mature late, may not contribute to population growth rates until they reach sub-adulthood, and disperse over long distances and thus may not contribute to the target fishery (Kindsvater et al., 2016). The specific approaches required for post-larval capture, culture, and release, and the costs involved, will be determined by the life-history characteristics of the candidate species, including larval duration, nursery habitat requirements, predation vulnerability and the status of local biota (Bell et al., 2006; Lorenzen et al., 2010). Using wild-caught animals compared to hatchery-farmed seed removes the need for aquaculture systems to house broodstock, avoids challenges in feeding newly-hatched larvae, likely reduces concerns for genetic pollution, and potentially increases success because wild-caught animals may adapt better to ocean conditions, although the latter two are likely situation-dependent (Blaxter, 1976).
2.1 Identifying the context-specific need for PCCR intervention
Context-specific appraisal of the need for enhancement and early goal-setting are critical for understanding how efforts can contribute to existing management (Lorenzen et al., 2010). Hatchery-based enhancement programs highlight the importance of identifying when and where intervention will add value to existing management, specifically where benefits can be generated beyond managing exploitation of wild stocks alone (Lorenzen, 2005; Bartley and Bell, 2008). An understanding of stock dynamics via assessment and modelling that examines critical factors such as source–sink population dynamics, strength of density-dependent mortality, and spatio-temporal recruitment variation will indicate the potential contribution of enhancement to broader fishery management goals (Gardner et al., 2006; Lorenzen et al., 2010). However, the local context-specific motivation for enhancement may be multifaceted, potentially including a need to bolster abundance of declining stock, precautionary enhancement of popular target fisheries, more general biodiversity enhancement and ecological restoration, and the provision of alternative livelihoods for small-scale fishers (Table 1; Hilborn, 2004; Lorenzen, 2005). Enhancement suitability will depend on the status of the target stock balanced against the extent of habitat and recruitment limitation, density-dependence, local environmental stressors, economic viability, existing fisheries management and stakeholder engagement (Taylor et al., 2017). involving stakeholders has proven critical for complementing controls on harvesting such as in the governance of marine protected areas, and in fisheries management initiatives that provide alternative livelihoods and reward active stewardship of marine resources (Lorenzen, 2005). Therefore, to optimize successful PCCR intervention, initial appraisals conducted with stakeholders should develop early accountable decision-making, aid in prioritising and selecting species for enhancement, and evaluate socio-economic and ecological costs and benefits (Table 1; Lorenzen et al., 2010).
In the French Mediterranean the white seabream Diplodus sargus is of both commercial and recreational importance (Figure 1B), and whilst spatial restrictions on its harvest exist throughout the region, and a minimum landing size is enforced, a combination of a lack of catch limits, recruitment bottlenecks and high mortality rates (60–99%) of newly settled D. sargus (Figure 1D) have elicited concerns over the sustainability of the fishery (Table 1). Given the historic importance of the species, its life-history is increasingly well understood. Post-larvae are easily harvested, withstand captive rearing and release, and are easily monitored and tracked due to their high site-fidelity on rocky reefs and surrounding areas. Thus, in the French Mediterranean, this management context together with the species’ suitability for PCCR led to the development of a program in partnership with local stakeholders aimed at enhancing regional production of the species (Table 1).
2.2 PCCR process of post-larval capture, culture, and release
2.2.1 Capture
Large-scale collection of early life-history stages of marine organisms for captive aquaculture demonstrates that wild post-larvae can be caught and maintained economically and in good condition for the purpose of PCCR enhancement (Bell et al., 2005; Lovatelli and Holthus, 2008). Innovative methods for optimizing catches of post-larvae exist for molluscs (e.g. oysters, mussels, scallops), crustaceans (e.g. shrimp, lobsters, crabs), echinoderms (sea cucumbers) and finfishes (e.g. milkfish, catfish, mullet, seabass, snapper) (Sadovy de Mitcheson and Liu, 2008). Identifying where, when, and how to catch abundant, healthy post-larvae is essential for viable PCCR operations, and relies on an understanding of species-specific behavior and ecology, and the local gene frequency of the wild fishery stock to ensure genetic complementarity on release (Bell et al., 2006; Sadovy de Mitcheson and Liu, 2008; Bell et al., 2009). The arrival of settlement-stage coral reef fishes to shallow coastal waters typically occurs at night (Dufour and Galzin, 1993; Holbrook and Schmitt, 1997), but the temporal and spatial abundance of post-larvae can be highly variable (Bell et al., 2009). At low latitudes, coral reef fish typically settle during each new moon phase (Bell et al., 2009), whereas settlement tends to be seasonal at higher latitudes (Faillettaz et al., 2020). Identifying areas of typically high settlement will maximise post-larvae capture as devices may be positioned accordingly (e.g. in shallow water close to nursery habitat, or in channels leading into closed lagoons; Bell et al., 2009). As with capture-based aquaculture, bycatch in harvesting of wild post-larvae can have significant environmental and biodiversity impacts which should be minimised through the use of selective gears and techniques (Sadovy de Mitcheson and Liu, 2008; Bell et al., 2009; Lenfant, 2015). Targeting multiple species for rearing and release has been proposed as a way to limit unwanted bycatch during harvesting (Bell et al., 2009). Techniques for harvesting post-larvae include crest nets for reef fish, purpose-built temporary shelters for some invertebrates (Hair et al., 2002), settlement structures (Herrnkind et al., 1999) and puerulus collectors (Phillips et al., 2001) for lobsters, and light-traps for species that are attracted to light and are competent swimmers (e.g. Figure 2A; Bell et al., 2009). Light-traps are commonly used for targeting post-larval finfish arriving from the plankton because they hold and maintain 100s–1000s of individuals (Lecaillon, 2012; Faillettaz et al., 2020). General guidelines for minimising mortality and optimising catches of wild post-larvae include: 1) ensuring water-flow within collection chambers of enclosed capture devices to supply sufficient oxygen until animal collection or release (Lecaillon and Lourié, 2007); 2) minimising duration of retention of catch by, for example, clearing traps deployed the previous evening at daybreak and sorting target species on site with bycatch released at or near the collection site at night to minimise predation (Bell et al., 2009); and 3) transporting catches promptly to aquaculture facilities in conditions that minimise stress (Portz et al., 2006). Whilst the selective nature of the capture methods used may influence the genetic composition of the post-larvae harvested from the wild (Munro and Bell, 1997; Faillettaz et al., 2020), harvesting post-larvae from areas of planned releases will ensure comparable gene frequency to the wild population (Munro and Bell, 1997).
In the example of the white seabream in the French Mediterranean, wild post-larvae were captured during three years (2011, 2014, 2015) by local artisanal fishers using light traps (Table 1; Figure 2A). At this stage, post-larvae are epipelagic and positively phototactic and light traps are effective in harvesting before settlement. Traps were deployed at sunset and retrieved the following morning around the new moon (i.e., the darkest period of the lunar phase) between May and June, coinciding with the early stages of natural settlement. Traps were placed in typical shallow nursery habitat of the species (Table 2). Where trap deployment was not possible or catches were low, they were complemented by the use of hand-nets in shallow (<1 m) areas. Survival was monitored during transfer to aquaria for rearing and was 98% overall (Table 2).
2.2.2 Culture
To maximise likelihood of survival, information on ecological processes that determine natural mortality of post-larvae can inform decisions on duration of culture and optimal size at release. Survival of post-larvae is determined by both density-dependent and size-dependent processes, as recruits compete for space and food in order to grow to a size and become less vulnerable to predation. For example, density has a strong effect on the survival of juvenile white seabream D. sargus at sizes below 20 mm TL, whilst survival at sizes above 20 mm TL is independent of density (Cuadros et al., 2018). A meta-analysis on the subject indicates that density-dependence in mortality ceases completely above 20% of the asymptotic length at which growth is zero (L∞, Lorenzen and Camp, 2019). Species-specific research is therefore key in determining the optimal size for release, and rearing wild post-larvae in hatcheries until they reach a size refuge from predation can increase survival compared to the high natural mortality of settlement-stage juveniles in the wild that remain highly vulnerable to both density- and size-dependent mortality (Planes et al., 1998; Bell et al., 2009; Lorenzen, 2022). Predation risk is directly linked to body size and is highest for small-bodied juveniles that remain vulnerable to predators who are limited to maximum prey sizes by their oral gape size (Goatley and Bellwood, 2016; Mihalitsis and Bellwood, 2017). An understanding of the local system and the presence of common predators and their maximum prey size can therefore inform optimal body size for release and maximize post-release survival. For example, mortality rates of even the largest newly recruiting coral reef fishes can be as high as 60% per day, but these rates diminish rapidly as surviving recruits increase in body size and gain experience of predators (Goatley and Bellwood, 2016). Natural mortality of juvenile banana prawn Penaeus merguiensis is size-dependent, dropping from 89% per week at 2 mm carapace length to 2% per week at 15 mm carapace length (Wang and Haywood, 1999). Very low survival rates for wild spat of the spiny lobster Panulirus ornatus in Vietnam are transformed into survival rates of 90% during grow-out in protective aquaculture facilities (Tuan et al., 2000). Mean survival rates of 93% are reported for wild-caught and cultured spat of blacklip pearl oyster Pinctada margaritifera in the Solomon Islands if reared to a size-refuge from predation (Friedman and Bell, 2000). Similarly, the capture and culture of D. sargus in France transforms early settlement survival rates of 1–35% in the wild (Macpherson et al., 1997; Planes et al., 1998) to survival rates of 79% with PCCR intervention (Figure 2B; Table 2).
Substantial development in culturing technology has enabled cost-effective rearing of fish (Kitada, 2020), with technical guidelines freely available for developing appropriate facilities and practices (Lovatelli and Holthus, 2008). Key aspects include aquaria design, nutritional requirements and feeding regimes (which may include weaning onto artificial food for rearing and then back onto live food prior to release), duration of grow-out and release size, and conditioning animals for release, including acclimation to local ocean temperatures and behavioural training (Taylor et al., 2017; Näslund, 2021).
Drastic differences between captive and natural environments may elicit physiological, morphological, behavioural and ecological effects on captive-reared juveniles which can impede survival in the wild (Lorenzen et al., 2012; Näslund, 2021). Nonetheless, as rearing practices and technology have advanced, wild post-larvae captured during settlement are likely to be inherently better adapted for life in natural conditions than those reared from captive broodstock in hatcheries (Bartley and Bell, 2008). While some facets of behaviour may be innate, others are learned during juvenile development so preparation can enhance post-release growth and survival (Kieffer and Colgan, 1992; Näslund, 2021). Behavioural training may include aspects of foraging, predator recognition and avoidance (Kieffer and Colgan, 1992; Ferrari et al., 2015; Näslund, 2021), ensuring animals do not habituate to life in aquaria (Kieffer and Colgan, 1992; Näslund, 2021). These principles were followed in our example of rearing D. sargus in the laboratory (Table 2). In contrast, poor fish husbandry such as overcrowding, overfeeding, poor water-quality, and disease transmission can result in high mortality during culture and threaten post-release spread of disease from captive to wild organisms, fueling concerns over biosecurity (Booth and Cox, 2003; Gardner et al., 2006; Bell et al., 2009). Wild-caught post-larvae generally demonstrate lower prevalence of disease than hatchery-reared juveniles (Langdon, 1989; Hair et al., 2002), although such risks may be further mitigated through effective disease management, such as species-specific and modern aquaculture systems and rearing practices (e.g. UV filters, net sterilisation), the implementation of a health monitoring program for juveniles with screening prior to release (Lorenzen et al., 2010), the use of risk analysis frameworks (Bell et al., 2006; Bartley and Bell, 2008), and avoiding translocations between separate populations (Langdon, 1989; Humphrey, 1995; Munro and Bell, 1997). In addition to health screening prior to release, the genetic composition of the reared population may be assessed for comparison with the wild population.
In our example of the white seabream, aquaria conditions, including stocking density and feeding regime, were tailored specifically to the species based on its natural ecology and monitored throughout the rearing process. Following capture and transport, D. sargus were acclimatised to aquaria facilities (Figure 2B) before being fed on a variety of feeds, including live zooplankton, to promote natural feeding behaviours and minimise domestication (Table 2). Initial stocking densities were 2 fish L-1, which were reduced to 1 fish L-1 once fish increased to above 25mm in size, and again to 0.5 fish L-1 at sizes above 45mm until release. Along with size-sorting of fish during these stages, this was aimed at reducing competition and aggression between conspecifics. Artificial shelters were provided to promote the development of natural behaviors. Implementing the measures described above, over a rearing period of 4–6 months, in our case study juvenile D. sargus reached a minimum TL of 7–8 cm with markedly higher survival than that reported in the wild (Table 2).
2.2.3 Release
The development of suitable release strategies (e.g. optimum habitat, season, minimum body size, stocking density) can improve immediate post-release juvenile survival and reduce initial emigration, and has been the subject of extensive research in fisheries enhancement (Mills et al., 2006; Bartley and Bell, 2008; Lorenzen et al., 2013; Table 2). Strategies can include: i) identification of locations close to initial capture sites with suitable habitat (Gardner et al., 2006; Mills et al., 2006); ii) careful timing of releases (Dawley et al., 1986; Broadley et al., 2017); and iii) assisting acclimation on release by providing fixed or temporary artificial structures as protection from predation and environmental stressors (e.g. Gardner et al., 2006; Mills et al., 2006; Ferrari et al., 2015). All three of these strategies were implemented when releasing D. sargus in the French Mediterranean (Table 2). Release locations were in areas with available nursery habitat in close proximity to original capture sites, timing coincided with optimal sea states (low currents and no swell), and post-larvae were at a body size that minimised predation risk. Acclimation to ocean conditions was maximised by using fine mesh tents to retain released fish for the first 24 h, and artificial habitat was provided to further reduce predation risk and ensure available nursery habitat (Table 2, Figure 2D).
Survival of released organisms is constrained by the carrying capacity of the receiving system, and so excessive densities may negate the benefits of well-designed release strategies due to increased intraspecific interactions including competition for food and density-dependent effects on growth, with substantial juvenile mortality if additional releases of juveniles exceed the system capacity (Lorenzen and Enberg, 2002; Kitada, 2018; Kitada, 2020). Whilst rearing post-larvae to larger sizes prior to release may limit density-dependent effects towards growth rather than mortality (Lorenzen and Camp, 2019), quantitative assessment of system capacity including available habitat is critical for responsible and effective enhancement programs (Lorenzen et al., 2013; Taylor et al., 2017), and may highlight the need for providing artificial habitat. Decision support tools, such as EnhanceFish (Medley and Lorenzen, 2006), and predictive mathematical models of population dynamics are designed to estimate optimum release densities based on resource availability, species-specific consumption rates and habitat requirements, trophic dynamics and ecological impacts related to releases (Lorenzen, 2006; Lorenzen et al., 2010). Whilst no quantitative modelling was undertaken for D. sargus in the Mediterranean, a 25-year time series of survey data on natural densities of juvenile D. sargus along the target system indicated that an optimal release density of 500 – 1,000 individuals annually across 20 km of coastline was necessary to ensure recruitment to the population (P Lenfant, 2022 personal communication, 8th November). Release protocols may be tested with field-trials of tagged animals (e.g. Figures 2C and 3) to assess recapture rates, survival through time and local migration (Mills et al., 2006). In our case study, colour-coded tagging according to the release site was implemented when releasing D. sargus to facilitate ongoing monitoring (Table 2; Figures 2C and 3), although caution is advised in extrapolating the results of small pilot studies which may not reflect juvenile survival at larger scales (Hilborn, 2004; Bartley and Bell, 2008).
Enhancement efficacy and mitigation of potential ecological impacts may be further addressed at the release stage; for example, minimising selective processes to avoid potential bias towards slower-growing individuals by identifying a minimum release-size based on average growth in the wild (Lorenzen, 2006). As mentioned previously, screening of reared populations can provide an understanding of the genetic composition and overall health of the population prior to release, ensuring that disease free and genetically comparable individuals are released into the wild population.
2.3 Measuring ‘success’
Measuring the success of PCCR enhancement for meeting predetermined goals is critical but remains an open challenge (Bartley and Bell, 2008; Lorenzen et al., 2010). Programs must show that: i) they can catch and rear juveniles to a suitable size for release; ii) the released fish survive to be recaptured and augment abundance of the wild stock (i.e. an additive effect of stocking); and iii) the costs (ecological, social, financial) of the program do not exceed the benefits (Hilborn, 2004; Taylor et al., 2017). Widespread uptake of capture-based aquaculture provides clear evidence of an increasing capability to capture, culture, and release post-larval organisms, cost-effectively and often at large-scales (Lorenzen, 2005; Lovatelli and Holthus, 2008). How PCCR enhancement impacts the abundance and productive capacity of the target fishery will depend on the species and context, including: spatial and temporal variation in the supply of post-larvae (Bell et al., 2009); the magnitude of natural recruitment of the spawning stock, which may reduce the value of releasing juveniles (Kitada, 2018); the extent of wild stock depletion (Neubauer et al., 2013); the scale of operation and carrying capacity of the receiving system due to density-dependent mortality (Bell et al., 2006; Kitada, 2020); and the impact of existing fisheries management measures (Lorenzen, 2008). Many enhancement initiatives have failed to collect data to demonstrate an additive effect of stocking on the total abundance and catches of the target stock in addition to the naturally recruited component, or show positive effects on the local ecosystem. This is in large part due to the inherent challenge of tracking released fish through to recruitment to the adult population, in some cases hampered by a lack of scientific, institutional and fisheries management engagement in the planning and evaluation (Bell et al., 2006; Lorenzen et al., 2010). Effective measurement of PCCR impacts on a target fishery requires well-designed experiments for collecting quantitative information on post-release performance and interactions (Lorenzen, 2014), including non-enhanced control sites and replicate releases (Hilborn, 2004), effective tracking programs (Amoroso et al., 2017), quantitative modelling approaches to assess changes in the target stock following enhancement (Lorenzen et al., 2010), and molecular tools to assess genetic diversity of both the wild and reared population (Cushman et al., 2019). Whilst the former may be relatively easily achievable and appropriate for smaller scale projects, a lack of capacity and expertise may prevent quantitative assessments of success of a PCCR program in contributing to the wild stock and its gene frequency. Indeed, this was the case for D. sargus in the French Mediterranean, which relied on traditional field observations and monitoring (Table 3), demonstrating high site-fidelity in underwater visual surveys (Table 3), but with the higher survival during captive rearing compared to the wild only potentially indicative of longer-term increases in recruitment and stock enhancement.
Ultimately, the efficacy of operations will depend on the extent to which released organisms survive and augment populations rather than replace them (Bartley and Bell, 2008; Green et al., 2013; Kindsvater et al., 2016). Mark–recapture studies enable evaluation of initial survival and movement, and ensure that released fish can be identified (Table 3; Lorenzen et al., 2010). Further, recent and rapid advances in the use of telemetry techniques that allow monitoring of large numbers of juveniles and adults in their emigration and habitat-use offer great potential for assessing contribution to adult population enhancement (Abecasis et al., 2013; Taylor et al., 2013), and can indicate behavioural interactions with local resident stocks that might lead to displacement (Green et al., 2013). To measure success from a fisheries management perspective, moving beyond a more singular fisheries production perspective (i.e. yield; Lorenzen et al., 2010), evaluation can quantify social impacts and assess the extent that enhancement complements existing management efforts (Lorenzen, 2008; Lorenzen, 2014). The available tools should allow well-managed PCCR programs to ensure and demonstrate that the ecological risks of genetic pollution and disease in wild populations are minimized and mitigated against, and offer a potentially valuable alternative to traditional ABE. Once ecological and social impacts are known, economic viability may be quantified to ensure the program costs are appropriate to the benefits (Lorenzen et al., 2010). Insights from marine-ranching programs suggest that the economic efficacy of enhancement operations will also vary with the socio-economic importance of the target species, the technology required and the local property rights over the stock (Whitmarsh, 2001).
Robust cost–benefit assessments of PCCR programs remain a challenge as they rely on accurate data on returns to the fishery from released fish, which can only be gained through ongoing monitoring. Project costs may be significant, yet benefits may be both social and economic and maximised through stakeholder engagement in the process. Whilst accurate assessments are likely only possible after a number of years, in the case of the D. sargus PCCR program in the Mediterranean there is evidence of short-term net benefits to the regional fishing industry (Table 3; CDC Biodiversité, 2019).
3 Discussion
To address historic effects of over-exploitation in coastal capture-fisheries, enhancement programs are expected to grow in importance for management (Lorenzen et al., 2013; Taylor et al., 2017) and to expand beyond shellfish, with an increased focus on finfish species. PCCR provides a promising supplementary or alternative tool to captive-rearing organisms through to harvest, at least where the natural supply of wild post-larvae can support such operations (Hair et al., 2002; Lorenzen, 2008). Evidence from capture-based aquaculture demonstrates that wild post-larvae can be successfully captured and cultured, and hatchery-based enhancement programs unequivocally show that organisms can be reared and released cost-effectively and at large-scales, supporting the likely viability of PCCR-based enhancement that combines such approaches (Hair et al., 2002; Bell et al., 2006). Where PCCR can provide incomes for local coastal communities, it may provide additional socio-economic benefits and promote active stewardship of local marine resources (Hair et al., 2002; Lorenzen, 2005; Bell et al., 2009). In this review we have brought together the existing information on the established general principles of PCCR which can inform its future implementation.
Despite its potential, PCCR shares some limitations and difficulties in its commercial-scale operation with aquaculture-based enhancement (ABE) more generally (Bell et al., 2006; Lorenzen, 2014). The capacity of enhancement initiatives to ensure net-positive ecological and socio-economic impacts depends on species- and context-specific planning and monitoring (Hilborn, 2004; Bell et al., 2006). PCCR will not be suitable for some fisheries (e.g. pelagic and highly migratory) due to life-history traits, population characteristics and biological requirements of species (Hair et al., 2002; Kindsvater et al., 2016). Similarly, ecological, environmental and economic constraints may restrict effective operations (Bell et al., 2009). Specifically, temporal variation in larval supply and density-dependent mortality will likely limit the capacity for some large-scale species-specific PCCR operations, and avoidance of post-larval overfishing must be ensured (Sadovy de Mitcheson and Liu, 2008). Where severe stock declines stem from chronic overfishing and environmental degradation, even the best-planned and executed enhancement program will have limited gains (Neubauer et al., 2013; Young et al., 2018). Therefore, viable PCCR enhancement will be contingent on complex aspects of the ecology, status and existing management of the target fishery, the supply of wild post-larvae, and the scale and careful planning of PCCR operations (e.g. the number of individuals that survive to join the wild fishery balanced with local carrying capacity; Bartley and Bell, 2008; Bell et al., 2009). Increasingly sophisticated and accessible modelling tools are available to address this complexity and aid responsible application of fisheries enhancement by estimating optimum quantities of organisms for release, their likely post-release survival and local ecological impacts (Lorenzen, 2005; Medley and Lorenzen, 2006). Further, PCCR operations will likely benefit from longer-term operations and an adaptive approach where risks of temporal variation in post-larval supply or overfishing may be mitigated by targeting multiple species according to larval supply and stock status (Hair et al., 2002; Bell et al., 2009; i.e. 60 species in the CasCioMar project, Table 1 and Table S1; Lecaillon pers. comm.).
The assumed benefits of PCCR are often discussed in relation to issues surrounding genetic pollution, homogenization and the spread of disease in natural populations that are commonly associated with ABE (Munro and Bell, 1997; Hair et al., 2002). As PCCR sources post-larvae from wild assemblages, natural levels of genetic diversity both within and between populations is more likely to be maintained. Whilst the chosen harvesting technique may select for certain genotypes from the population, PCCR avoids the introduction of new genotypes to the population as occurs via the use of hatchery broodstock. Additionally, available molecular tools can allow for genetic monitoring of reared populations prior to release, although a detailed understanding of the impacts of PCCR on genetic diversity in the wild would require long-term monitoring of one or more populations (Cushman et al., 2019), and the long-term effects on genetic fitness of PCCR relative to ABE remain unknown. Similarly, the release of hatchery-reared stock may transmit diseases to natural populations or lower disease resistance through genetic effects (Lorenzen et al., 2010). However, both of these can be lessened through PCCR by rearing wild-sourced post-larvae, maintaining good husbandry practices and disease screening prior to release. Whilst disease monitoring within natural and released individuals would be beneficial, accurate assessments of the potential impacts of PCCR on disease transmission remain difficult. Although PCCR may not eliminate the ecological risks to wild populations completely, by following such best practices it may offer a promising alternative to minimize such risks compared to traditional ABE. Future research should seek to better understand these potential benefits of PCCR by addressing the outstanding knowledge gaps in relation to the potential ecological risks around genetic impacts and disease relative to traditional enhancement techniques to inform future management decisions.
Integrating PCCR with current fisheries enhancement approaches presents a unique opportunity for future research and development, with potential to examine programs across a variety of contexts, at ecologically relevant scales and with operational-scale assessment (Hilborn, 2004; Lorenzen, 2005; Lorenzen et al., 2013). Much can be learned from the substantial technical developments made in aquaculture practices and release strategies of hatchery schemes (Bell et al., 2005; Taylor et al., 2017). However, aquaculture-based enhancement increasingly requires innovative ecological and socio-economic solutions (Taylor et al., 2017). PCCR may be one such solution. For now, rather than specific technical demands, the main challenge facing PCCR is its limited uptake which restricts broad evaluation from early planning to full assessment (Bell et al., 2006; Bell et al., 2009). Fisheries enhancement programs are often driven by managers and resource-users employing readily-available methods, often with little or no involvement of fisheries scientists (Lorenzen, 2014; Taylor et al., 2017), and sometimes without quantifiable assessment of the outcome in the wider fisheries management context (Lorenzen et al., 2010). Practical trials and field studies are essential for developing appropriate methodologies and approaches to PCCR that may be specific to both sites and species. These may range from small-scale studies to assess immediate post-release survival and dispersal, to large-scale studies that release high numbers of individuals, with tracking techniques to allow assessments of carrying capacity and density-dependent growth responses. Coupled with modelling of larval supply and adult population dynamics (e.g. density-dependency and survival to adulthood), within a variety of socio-ecological contexts, such research will provide evidence on what works, what does not, and why (Bell et al., 2006). This review compiles available information on current approaches and established principles, supporting realisation of PCCR enhancement as a credible and valuable management tool.
Ethics statement
Written informed consent was obtained from the individual(s) for the publication of any identifiable images or data included in this article.
Author contributions
LR led on manuscript preparation. PL, AF, AG, and GL worked on the seabream project explored in this review and contributed information on the case study to the manuscript. All authors, including LC, LV, AR and SS, contributed to manuscript preparation and revisions. All authors contributed to the article and approved the submitted version.
Funding
The seabream project explored in this review was part funded by the European Commission (LIFE+ SUBLIMO project LIFE10NAT/FR/000200), facilitated by fishing permits from the French Administration (Direction Inter-Regional de la Méditerranée), and assistance in post-larvae collection by the professional fishermen institution (Prud'Homie Leucate, Prud'Homie Barcarès, Comité Régional des Pêches et des Elevages Marins d'Occitanie). The case study work was also carried-out in collaboration with the CasCioMar project, an initiative by the French Water Agency (Agence de l’Eau Rhône Méditerannée Corse), the CDC Biodiversité, the South Region and the artisanal fishermen association.
Acknowledgments
Thanks to Ecocean SAS staff for useful discussions and assistance in the preparation of this review. Thanks to reviewers who’s careful reading and insightful comments improved the final manuscript.
Conflict of interest
Authors AF, AG, and GL are employed by the company Ecocean Inc. Ecocean funded a joint meeting in the UK and supported LR’s time to write this review.
The remaining authors declare that the research was conducted in the absence of any commercial or financial relationships that could be construed as a potential conflict of interest.
Publisher’s note
All claims expressed in this article are solely those of the authors and do not necessarily represent those of their affiliated organizations, or those of the publisher, the editors and the reviewers. Any product that may be evaluated in this article, or claim that may be made by its manufacturer, is not guaranteed or endorsed by the publisher.
Supplementary material
The Supplementary Material for this article can be found online at: https://www.frontiersin.org/articles/10.3389/fmars.2022.1058497/full#supplementary-material
References
Abecasis D., Bentes L., Lino P. G., Santos M. N., Erzini K. (2013). Residency, movements and habitat use of adult white seabream (Diplodus sargus) between natural and artificial reefs. Estuar. Coast. Shelf Sci. 118, 80–85. doi: 10.1016/j.ecss.2012.12.014
Almany G. R., Webster M. S. (2006). The predation gauntlet: early post-settlement mortality in reef fishes. Coral reefs 25 (1), 19–22. doi: 10.1007/s00338-005-0044-y
Amoroso R. O., Tillotson M. D., Hilborn R. (2017). Measuring the net biological impact of fisheries enhancement: Pink salmon hatcheries can increase yield, but with apparent costs to wild populations. Can. J. Fisheries Aquat. Sci. 74 (8), 1233–1242.
Bartley D. M., Bell J. D. (2008). Restocking, stock enhancement, and sea ranching: Arenas of progress. Rev. Fisheries Sci. 16 (1-3), 357–365. doi: 10.1080/10641260701678058
Bell J. D., Bartley D. M., Lorenzen K., Loneragan N. R. (2006). Restocking and stock enhancement of coastal fisheries: Potential, problems and progress. Fish. Res. 80 (1), 1–8. doi: 10.1016/j.fishres.2006.03.008
Bell J. D., Clua E., Hair C. A., Galzin R., Doherty P. J. (2009). The capture and culture of post-larval fish and invertebrates for the marine ornamental trade. Rev. Fish. Sci. 17 (2), 223–240. doi: 10.1080/10641260802528541
Bell J. D., Rothlisberg P. C., Munro J. L., Loneragan N. R., Nash W. J., Ward R. D., et al. (2005). Restocking and stock enhancement of marine invertebrate fisheries (Elsevier).
Blankenship H. L., Leber K. M. (1995). A responsible approach to marine stock enhancement. Am. Fish. Soc. Symp. 15, 167–175.
Blaxter J. H. S. (1976). “Reared and wild fish: how do they compare?,” in Persoone G., Jaspers E. (Ed.) Proceedings of the 10th European Symposium on Marine Biology, Ostend, Belgium, Sept. 17-23, 1975: 1. Research in mariculture at laboratory- and pilot scale. IZWO: Wetteren. ISBN 90-6281-001-2. 620 pp
Broadley A. D., Tweedley J. R., Loneragan N. R. (2017). Estimating biological parameters for penaeid restocking in an Australian temperate estuary. Fish. Res. 186, 488–501. doi: 10.1016/j.fishres.2016.09.007
Booth J. D., Cox O. (2003). Marine fisheries enhancement in new Zealand: our perspective. N. Z. J. Mar. Freshwater Res. 37 (4), 673–690. doi: 10.1080/00288330.2003.9517198
CDC Biodiversité (2019). Évaluation socioéconomique des solutions fondées sur la nature (Paris, France: CDC Biodiversite). Available at: https://www.cdc-biodiversite.fr/.
Cuadros A., Basterretxea G., Cardona L., Cheminée A., Hidalgo M., Moranta J. (2018). Settlement and post-settlement survival rates of the white seabream (Diplodus sargus) in the western Mediterranean Sea. PloS One 13 (1), e0190278–e0190278. doi: 10.1371/journal.pone.0190278
Cushman E. L., Evans H. K., Moyer G. R., Raley M. E., Williams A. S., Darden T. L. (2019). Development of a standardized molecular tool and estimation of genetic measures for responsible aquaculture-based fisheries enhancement of American shad in north and south Carolina. Trans. Am. Fish. Soc. 148 (1), 148–162. doi: 10.1002/tafs.10127
Dawley E. M., Ledgerwood R. D., Blahm T. H., Sims C. W., Durkin J. T., Kirn R. A., et al. (1986). Migrational characteristics, biological observations, and relative survival of juvenile salmonids entering the Columbia river estuary 1966-1983 (Portland, Oregon: US Department of Energy, Bonneville Power Administration, Division of Fish and Wildlife).
Dong J., Jiang L., Tan K., Liu H., Purcell J. E., Li P., et al. (2009). Stock enhancement of the edible jellyfish (Rhopilema esculentum kishinouye) in liaodong bay, China: a review. Hydrobiologia 616 (1), 113–118. doi: 10.1007/s10750-008-9592-9
Dufour V., Galzin R. (1993). Colonization patterns of reef fish larvae to the lagoon at moorea island, French Polynesia. Mar. Ecol. Ser. 102, 143. doi: 10.3354/meps102143
Faillettaz R., Voué R., Crec’hriou R., Garsi L.-H., Lecaillon G., Agostini S., et al. (2020). Spatio-temporal patterns of larval fish settlement in the northwestern Mediterranean Sea. Mar. Ecol. Prog. Ser. 650, 153–173. doi: 10.3354/meps13191
FAO (2021) Species fact sheets: Diplodus sargus (Valenciennes 1830). FAO FishFinder - web site about FAO FishFinder FI institutional websites FAO fish div. Available at: http://www.fao.org/fishery/species/2370/en.
FAO (2022). The state of world fisheries and aquaculture 2022: Towards blue transformation (Rome, Italy: FAO).
Ferrari M. C. O., McCormick M. I., Meekan M. G., Chivers D. P. (2015). Background level of risk and the survival of predator-naive prey: can neophobia compensate for predator naivety in juvenile coral reef fishes? Proc. Biol. Sci. 282 (1799), 20142197. doi: 10.1098/rspb.2014.2197
FranceAgriMer (2020) Oceanic development, vertigo Lab, EUREKA MER. rapport final population A: Eléments d’analyse et enjeux pour la petite pêche côtière en France métropolitaine. Available at: https://www.franceagrimer.fr/fam/content/download/64934/document/ETU-MER-PPC-2020.pdf?version=6.
Friedman K. J., Bell J. D. (2000). Shorter immersion times increase yields of the blacklip pearl oyster, Pinctada margaritifera (Linne.), from spat collectors in Solomon islands. Aquaculture 187 (3), 299–313. doi: 10.1016/S0044-8486(00)00311-2
Gardner C., Frusher S., Mills D., Oliver M. (2006). Simultaneous enhancement of rock lobster fisheries and provision of puerulus for aquaculture. Fish. Res. 80 (1), 122–128. doi: 10.1016/j.fishres.2006.03.025
Garlock T. M., Camp E. V., Lorenzen K. (2017). Using fisheries modeling to assess candidate species for marine fisheries enhancement. FishRes 186, 460–467. doi: 10.1016/j.fishres.2016.08.024
Goatley C. H. R., Bellwood D. R. (2016). Body size and mortality rates in coral reef fishes: a three-phase relationship. Proc. R Soc. B Biol. Sci. 283 (1841), 20161858. doi: 10.1098/rspb.2016.1858
Green B. S., Pederson H., Gardner C. (2013). Overlap of home ranges of resident and introduced southern rock lobster after translocation. Rev. Fish. Sci. 21 (3–4), 258–266. doi: 10.1080/10641262.2013.799389
Hair C. A., Bell J. D., Doherty P. J. (2002). The use of wild-caught juveniles in coastal aquaculture and its application to coral reef fishes. Resp. Mar. Aquac. CAB Int., 327–353. doi: 10.1079/9780851996042.0327
Herrnkind W. F., Butler M. J. IV, Hunt J. H. (1999). A case for shelter replacement in a disturbed spiny lobster nursery in Florida: why basic research had to come first. In Am. Fish. Soc. Symp. 22, 421–437).
Hilborn R. (2004). Population management in stock enhancement and sea ranching. Stock. Enhanc. Sea Ranching Dev. Pitfalls Oppor., 201–209. doi: 10.1002/9780470751329.ch17
Hilborn R., Amoroso R. O., Anderson C. M., Baum J. K., Branch T. A., Costello C., et al. (2020). Effective fisheries management instrumental in improving fish stock status. Proc. Natl. Acad. Sci. 117 (4), 2218–2224. doi: 10.1073/pnas.1909726116
Holbrook S. J., Schmitt R. J. (1997). “Settlement patterns and process in a coral reef damselfish: in situ nocturnal observations using infrared video,” in Proceedings of the 8th international coral reef symposium. Balboa, Panama: Smithsonian Tropical Research Institute, Vol. 2. 1143–1148.
Humphrey J. D. (1995). “Perspectives in aquatic exotic species management in the pacific islands,” in Vol.II introductions of aquatic animals to the pacific islands: disease threats and guidelinesfor quarantine, vol. 8, 53. (South Pacific Commission, Inshore Fisheries Research Project).
Iacarella J. C., Saheed D., Dunham A., Ban N. C. (2019). Non-native species are a global issue for marine protected areas. Front. Ecol. Environ. 17 (9), 495–501. doi: 10.1002/fee.2100
Kieffer J. D., Colgan P. W. (1992). The role of learning in fish behaviour. Rev. Fish. Biol. Fish. 2 (2), 125–143. doi: 10.1007/BF00042881
Kindsvater H. K., Mangel M., Reynolds J. D., Dulvy N. K. (2016). Ten principles from evolutionary ecology essential for effective marine conservation. Ecol. Evol. 6 (7), 2125–2138. doi: 10.1002/ece3.2012
Kitada S. (2018). Economic, ecological and genetic impacts of marine stock enhancement and sea ranching: A systematic review. Fish. Fish. 19 (3), 511–532. doi: 10.1111/faf.12271
Kitada S. (2020) Lessons from Japan marine stock enhancement and sea ranching programmes over 100 years. Rev. Aquac. 3, 1944–1969. doi: 10.1111/raq.12418
Langdon J. S. (1989). Experimental transmission and pathogenicity of epizootic haematopoietic necrosis virus (EHNV) in redfin perch, perca fluviatilis l., and 11 other teleosts. J. Fish Dis. 12 (4), 295–310. doi: 10.1111/j.1365-2761.1989.tb00318.x
Lecaillon G. (2012). “Postlarval fish capture and culture for restoring fisheries,” in Innov methods mar ecosyst restor, 1st ed. Eds. Goreau T. J., Trench R. K. (Boca Raton: CRC Press), 312.
Lecaillon G., Lourié S. M. (2007). Current status of marine post-larval collection: Existing tools, initial results, market opportunities and prospects. SPC Live. Reef Fish. Bull. 17, 3–10.
Lenfant P. (2015). SUBLIMO – suivi de la biodiversité de post-larves ichtyques en méditerranée occidentale LIFE10 NAT/FR/000200 (Perpignan: Université de Perpignan).
Lloret J., Zaragoza N., Caballero D., Riera V. (2008). Biological and socioeconomic implications of recreational boat fishing for the management of fishery resources in the marine reserve of cap de creus (NW Mediterranean). Fish. Res. 91 (2), 252–259. doi: 10.1016/j.fishres.2007.12.002
Loneragan N. R., Jenkins G. I., Taylor M. D. (2013). Marine stock enhancement, restocking, and sea ranching in Australia: Future directions and a synthesis of two decades of research and development. Rev. Fish. Sci. 21 (3-4), 222–236. doi: 10.1080/10641262.2013.796810
Lorenzen K. (2005). Population dynamics and potential of fisheries stock enhancement: practical theory for assessment and policy analysis. Philos. Trans. R Soc. B Biol. Sci. 360 (1453), 171–189. doi: 10.1098/rstb.2004.1570
Lorenzen K. (2006). Population management in fisheries enhancement: Gaining key information from release experiments through use of a size-dependent mortality model. Fish. Res. 80 (1), 19–27. doi: 10.1016/j.fishres.2006.03.010
Lorenzen K. (2008). Understanding and managing enhancement fisheries systems. Rev. Fish. Sci. 16 (1–3), 10–23. doi: 10.1080/10641260701790291
Lorenzen K., Beveridge M. C., Mangel M. (2012). Cultured fish: Integrative biology and management of domestication and interactions with wild fish. Biol. Rev. 87 (3), 639–660. doi: 10.1111/j.1469-185X.2011.00215.x
Lorenzen K. (2014). Understanding and managing enhancements: why fisheries scientists should care. J. Fish. Biol. 85 (6), 1807–1829. doi: 10.1111/jfb.12573
Lorenzen K. (2022). Size-and age-dependent natural mortality in fish populations: Biology, models, implications, and a generalized length-inverse mortality paradigm. Fish. Res. 255, 106454. doi: 10.1016/j.fishres.2022.106454
Lorenzen K., Agnalt A.-L., Blankenship H. L., Hines A. H., Leber K. M., Loneragan N. R., et al. (2013). Evolving context and maturing science: Aquaculture-based enhancement and restoration enter the marine fisheries management toolbox. Rev. Fish. Sci. 21 (3–4), 213–221. doi: 10.1080/10641262.2013.837358
Lorenzen K., Enberg K. (2002). Density-dependent growth as a key mechanism in the regulation of fish populations: evidence from among-population comparisons. Proc. R Soc. London Ser. B Biol. Sci. 269 (1486), 49–54. doi: 10.1098/rspb.2001.1853
Lorenzen K., Leber K. M., Blankenship H. L. (2010). Responsible approach to marine stock enhancement: An update. Rev. Fish. Sci. 18 (2), 189–210. doi: 10.1080/10641262.2010.491564
Lorenzen K., Camp E. V. (2019). Density-dependence in the life history of fishes: when is a fish recruited? Fisheries Res. 217, 5–10. doi: 10.1016/j.fishres.2018.09.024
Lovatelli A., Holthus P. F. (2008). Capture-based aquaculture: global overview (Rome: Food and Agriculture Organization of the United Nations Rome).
Macpherson E. (1998). Ontogenetic shifts in habitat use and aggregation in juvenile sparid fishes. J. Exp. Mar. Biol. 220 (1), 127–150. doi: 10.1016/S0022-0981(97)00086-5
Macpherson E., Biagi F., Francour P., García-Rubies A., Harmelin J., Harmelin-Vivien M., et al. (1997). Mortality of juvenile fishes of the genus Diplodus in protected and unprotected areas in the western Mediterranean Sea. Mar. Ecol. Prog. Ser. 160, 135–147. doi: 10.3354/meps160135
Masuda R., Tsukamoto K. (1998). Stock enhancement in Japan: review and perspective. Bull. Mar. Sci. 62 (2), 337–358.
Medley P. A. H., Lorenzen K. (2006). EnhanceFish: A decision support tool for aquaculture-based fisheries enhancement. (Software package: London: Imperial College).
Mercader M., Rider M., Cheminée A., Pastor J., Zawadzki A., Mercière A., et al. (2018). Spatial distribution of juvenile fish along an artificialized seascape, insights from common coastal species in the northwestern Mediterranean Sea. Mar. Environ. Res. 137, 60–72. doi: 10.1016/j.marenvres.2018.02.030
Mihalitsis M., Bellwood D. R. (2017). A morphological and functional basis for maximum prey size in piscivorous fishes. PloS One 12 (9), e0184679. doi: 10.1371/journal.pone.0184679
Mills D. J., Gardner C., Johnson C. R. (2006). Experimental reseeding of juvenile spiny lobsters (Jasus edwardsii): Comparing survival and movement of wild and naïve lobsters at multiple sites. Aquaculture 254 (1), 256–268. doi: 10.1016/j.aquaculture.2005.09.007
Moore J. K., Fu W., Primeau F., Britten G. L., Lindsay K., Long M., et al. (2018). Sustained climate warming drives declining marine biological productivity. Science 359 (6380), 1139–1143. doi: 10.1126/science.aao6379
Munro J. L., Bell J. D. (1997). Enhancement of marine fisheries resources. Rev. Fish. Sci. 5 (2), 185–222. doi: 10.1080/10641269709388597
Näslund J. (2021). Reared to become wild-like: addressing behavioral and cognitive deficits in cultured aquatic animals destined for stocking into natural environments–a critical review. Bull. Mar. Sci. 97 (4), 489–538. doi: 10.5343/bms.2020.0039
Neto J., Vieira D., Abecasis D., Marques J., Gordo L J. I. R., Bispo R., et al. (2019). Facultative cleaning behaviour of juvenile Diplodus sargus (Sparidae) and its ecological role in marine temperate waters. Mar. Ecol. Prog. Ser. 629, 165–177. doi: 10.3354/meps13105
Neubauer P., Jensen O. P., Hutchings J. A., Baum J. K. (2013). Resilience and recovery of overexploited marine populations. Science 340 (6130), 347–349. doi: 10.1126/science.1230441
Pauly D., Zeller D. (2016). Catch reconstructions reveal that global marine fisheries catches are higher than reported and declining. Nat. Commun. 7 (1), 10244. doi: 10.1038/ncomms10244
Perry A. L., Low P. J., Ellis J. R., Reynolds J. D. (2005). Climate change and distribution shifts in marine fishes. Science 308 (5730), 1912–1915. doi: 10.1126/science.1111322
Phillips B. F., Melville-Smith R., Cheng Y. W., Rossbach M. (2001). Testing collector designs for commercial harvesting of western rock lobster (Panulirus cygnus) puerulus. Mar. Freshw. Res. 52 (8), 1465–1473. doi: 10.1071/MF01069
Planes S., Jouvenel J.-Y., Lenfant P. (1998). Density dependence in post-recruitment processes of juvenile sparids in the littoral of the Mediterranean Sea. Oikos 83 (2), 293–300. doi: 10.2307/3546840
Planes S., Lecaillon G. (2001). Caging experiment to examine mortality during metamorphosis of coral reef fish larvae. Coral Reefs 20 (3), 211–218. doi: 10.1007/s003380100161
Portz D. E., Woodley C. M., Cech J. J. (2006). Stress-associated impacts of short-term holding on fishes. Rev. Fish. Biol. Fish. 16 (2), 125–170. doi: 10.1007/s11160-006-9012-z
Raventós N., Macpherson E. (2001). Planktonic larval duration and settlement marks on the otoliths of Mediterranean littoral fishes. Mar. Biol. 138 (6), 1115–1120. doi: 10.1007/s002270000535
Sadovy de Mitcheson Y., Liu M. (2008). “Environmental and biodiversity impacts of capture-based aquaculture,” in Capture-based aquaculture. Global overview. FAO Fisheries Technical Paper. Eds. Lovatelli A., Holthus P. F. (Rome: FAO), 5–39.
Saleh M. (2008). Capture-based aquaculture of mullets in egypt. capture-based aquac glob overview. FAO Fish. Tech. Pap. 508, 109–126.
Taylor M. D., Chick R. C., Leber K. M., Haegen G.V., Loneragan N. R. (2017). Fisheries enhancement and restoration in a changing world. Fish. Res 186, 407–412. doi: 10.1016/j.fishres.2016.10.004
Taylor M. D., Fairfax A. V., Suthers I. M. (2013). The race for space: Using acoustic telemetry to understand density-dependent emigration and habitat selection in a released predatory fish. Rev. Fish. Sci. 21 (3–4), 276–285. doi: 10.1080/10641262.2013.796813
Tuan L. A., Nho N. T., Hambrey J. (2000). “Status of cage mariculture in Vietnam,” in 1 Int Symp Cage Aquac Asia, Tungkang, Pintung (Taiwan, 2-6 Nov 1999, AFS: WAS-SC.
Uki N. (2006). Stock enhancement of the Japanese scallop Patinopecten yessoensis in Hokkaido. Fish. Res. 80 (1), 62–66. doi: 10.1016/j.fishres.2006.03.013
Wang Y.-G., Haywood M. D. E. (1999). Size-dependent natural mortality of juvenile banana prawns Penaeus merguiensis in the gulf of carpentaria, Australia. Mar. Freshw. Res. 50 (4), 313–317. doi: 10.1071/MF97229
Whitmarsh D. (2001). Economic analysis of marine ranching (Centre for the Economics and Management of Aquatic Resources, University of Portsmouth).
Keywords: capture-based fisheries, fisheries management, mariculture, wild-caught post-larval fish, restoration, stock enhancement
Citation: Richardson LE, Lenfant P, Clarke LJ, Fontcuberta A, Gudefin A, Lecaillon G, Le Vay L, Radford AN and Simpson SD (2023) Examining current best-practices for the use of wild post-larvae capture, culture, and release for fisheries enhancement. Front. Mar. Sci. 9:1058497. doi: 10.3389/fmars.2022.1058497
Received: 30 September 2022; Accepted: 19 December 2022;
Published: 17 January 2023.
Edited by:
Brett W. Molony, Oceans and Atmosphere (CSIRO), AustraliaReviewed by:
Ginny Eckert, University of Alaska Fairbanks, United StatesChongliang Zhang, Ocean University of China, China
Copyright © 2023 Richardson, Lenfant, Clarke, Fontcuberta, Gudefin, Lecaillon, Le Vay, Radford and Simpson. This is an open-access article distributed under the terms of the Creative Commons Attribution License (CC BY). The use, distribution or reproduction in other forums is permitted, provided the original author(s) and the copyright owner(s) are credited and that the original publication in this journal is cited, in accordance with accepted academic practice. No use, distribution or reproduction is permitted which does not comply with these terms.
*Correspondence: Leo J. Clarke, l.clarke@bangor.ac.uk