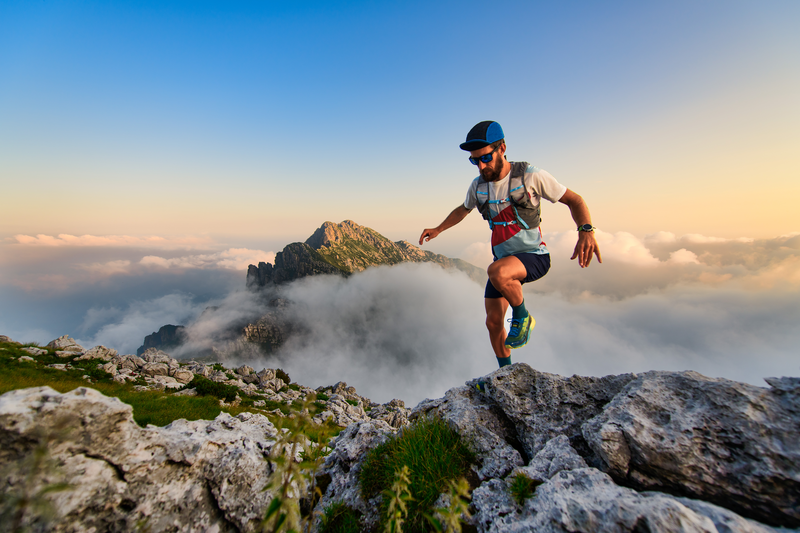
94% of researchers rate our articles as excellent or good
Learn more about the work of our research integrity team to safeguard the quality of each article we publish.
Find out more
ORIGINAL RESEARCH article
Front. Mar. Sci. , 21 November 2022
Sec. Marine Fisheries, Aquaculture and Living Resources
Volume 9 - 2022 | https://doi.org/10.3389/fmars.2022.1057201
This article is part of the Research Topic Exploration and Utilization of Marine and Freshwater High-Value Biological Resources View all 12 articles
Introduction: As a pigment protein, phycocyanin has been widely used in the fields of food, nutraceutical and biotechnology due to its excellent biological activities of antioxidant. So far, Arthrospira has been generally considered as a great species for phycocyanin production.
Methods: In this study, independent and interactive effect of three factors, NaCl, Bainengsi (BS) and Bainengtai (BT) on microalgae growth and phycocyanin production were explored by response surface methodology (RSM). Using Box-Behnken design (BBD) method, the well-fitting quadratic models were established based on experimental results.
Results: Moreover, the maximum phycocyanin concentration reached 704.66 mg L-1 at the optimal condition (185 mM NaCl, 200 mg L-1 BS, and 20 mg L-1 BT), while the maximum phycocyanin content of 19.03% was obtained at another optimal condition (136 mM NaCl, 200 mg L-1 BS, and 50 mg L-1 BT). Compared to control, the concentration and content of phycocyanin were increased by 22.98% and 16.73%, respectively.
Discussion: Overall, this study demonstrated that addition of exogenous substances (BS, BT) into culture medium optimized by RSM was an effective approach to increase phycocyanin production, which paved a potential way to realizing high efficient production of algal biomass and bioactive substances.
Microalgae have been extensively considered as renewable and sustainable resources for the production of various bioactive substances, such as phycobiliproteins (Manirafasha et al., 2016), carotenoids (Novoveská et al., 2019), polyunsaturated fatty acids (Lu et al., 2021), and polysaccharide (Sathasivam et al., 2019). Phycobiliprotein is a kind of pigment protein that widely exists in cyanobacteria, rhodophyta, cryptophyta, and glaucophyta (Lawrenz et al., 2011; Yu et al., 2017). In cyanobacteria, phycobiliprotein can account for 40% of the total soluble protein (Pandey et al., 2013). Generally, there are four types of phycobiliprotein, namely phycoerythrin, phycocyanin, phycoerythrocyanin, and allophycocyanin (Pagels et al., 2019). Phycocyanin shows great antioxidant and radical scavenging activity (Fratelli et al., 2021; Li, 2022). Besides, phycocyanin has other biological activities, like anti-inflammatory, anti-cancer, anti-diabetes, neuroprotective and hepatoprotective effects (Romay et al., 2003; Jiang et al., 2017; Prabakaran et al., 2020). Thereby, phycocyanin nowadays is widely used in food, nutraceutical, cosmetics, and pharmaceutical industries (Manirafasha et al., 2016; Khazi et al., 2020). Presently, the commercial price of phycocyanin varies enormously depending on its purity. It was reported that the price of food-grade phycoerythrin was approximately $130 g-1 (Hsieh-Lo et al., 2019). Moreover, the market demands for phycoerythrin continuously increased in the past few years due to its natural and functional properties. Specifically, the global market size of phycocyanin was valued at $155.3 million in 2020 and it is estimated to reach $409.8 million by 2030 (Thevarajah et al., 2022).
Arthrospira, commonly known as Spirulina, is a multicellular and filamentous cyanobacteria (Soni et al., 2017). It is generally rich in protein, carbohydrates, phycocyanin, fatty acids, and chlorophyll (Anvar and Nowruzi, 2021). Arthrospira is well considered as an excellent source of the production of phycocyanin (CPC) (Nethravathy et al., 2019; Silva et al., 2020). To date, numerous studies have been conducted to improve the phycocyanin productivity and content of Arthrospira. The main strategies includes culture medium improvement, exogenous substances addition (Manirafasha et al., 2018), environmental condition optimization (Bachchhav et al., 2016), and changing of culture mode (Chen et al., 2006). The optimization of culture medium is always a basic work to enhance microalgal growth and phycocyanin production. Nitrogen is one of the primary nutrient requirements for algal cell growth. With the nitrogen (NaNO3) concentration in the medium increased from 0.63 to 2.5 g L-1, the biomass concentration of Arthrospira highly increased from 0.83 to 1.04 g L-1 (Can et al., 2017). In addition to nitrogen concentration, microalgae growth and phycocyanin production are also affected by type of nitrogen source. Mousavi et al. (2022) found that Arthrospira cultured in the medium containing (NH4)2SO4 produced the largest amount of phycocyanin (118 mg L-1) while the medium with KNO3 as the nitrogen source resulted in the highest biomass output (1.18 g L-1). In addition, salinity of culture medium significantly influences algal cell growth and phycocyanin accumulation. The Zarrouk medium with 0.1 M NaCl considerably raised the biomass productivity from 139.5 mg L-1 d-1 at the normal condition (0.02 M NaCl) to 221.33 mg L-1 d-1, and the total phycobiliprotein content increased from 11.08% to 15.63% (Abd El-Baky and El-Baroty, 2012). However, the impact of salinity on cell growth and phycobiliprotein production is highly related to NaCl concentration. The tremendously high NaCl concentration would result in very low biomass and phycobiliprotein productivity (Chen et al., 2016).
Besides, the addition of exogenous substances is also an efficient approach to promote the biomass and phycocyanin accumulation. The supplements of mineral elements, organic acids, and vitamins in culture medium have been reported to enhance biomass and phycocyanin production (Jung et al., 2014; Bachchhav et al., 2016; Mogany et al., 2018). Stepwise addition of selenium (Se) during the exponential growth phase of Arthrospira. platensis grown mixotrophically increased the phycocyanin production by 21.2% compared to control (Chen et al., 2006). The metabolic stress conditions induced by nutrient enrichment through additionally adding sodium glutamate and succinic acid strongly boosted the biomass production and phycocyanin concentration of Arthrospira platensis (Bachchhav et al., 2016). Hence, the addition of suitable substance in culture medium at an appropriate range is critical to efficient production of phycocyanin.
Response surface methodology (RSM) is a set of mathematical and statistical techniques that are based on fitting a polynomial equation to experimental data (Bezerra et al., 2008). Currently, the RSM has been used to optimize the culture process of microalgae. To maximize the biomass production of Chlorella sp. as biofuel production stock, the optimal concentration of nitrogen and glycerol (0.114 g L-1 and 2.70 g L-1) were determined by the RSM, and the highest biomass reached 2.41 g L-1 at this condition (Skorupskaite et al., 2015). Verma et al. (2020) performed the culture experiments to improved medium using the RSM, and obtained the highest biomass productivity of 0.86 g L-1 d-1 at the optimal conditions. Undoubtedly, RSM is an efficient and effective means to optimize the culture medium.
There are commercial products of mineral element complexes and peptide complexes, commonly called Bainengsi (BS) and Bainengtai (BT), respectively, in China. BS contains many mineral elements including Si, Mo, Se, Ni, Bo, K and so on. BT is composed of enzymatic hydrolysates of high-quality proteins sourced from plants. BS and BT have been used in agriculture and feed industries to enhance the plant and animal growth. In the present study, we consider BS and BT as the additional supplements for Arthrospira maxima culture. The main aim of the research is to evaluate the independent and interaction effect of three factors (BS, BT, and NaCl concentrations) on biomass and phycocyanin production of Arthrospira maxima, and to determine the optimal addition amounts of these substances using RSM to maximize the productivity and content of phycocyanin.
Arthrospira maxima (FACHB-438) was obtained from the Freshwater Algae Culture Collection at the Institute of Hydrobiology (FACHB), China. For stock culture preparation, the microalgal cells were cultivated in Zarrouk medium (Zarrouk, 1996) at 31°C and light intensity of 80 μ mol m-2 s-1.
Two types of exogenous substances (BS and BT) were added into culture medium to explore their impacts on the growth and phycocyanin production of Arthrospira maxima. BS and BT were purchased from Jiangsu Rishengchang Biotechnology Company, China. BS is the mineral element complexes and composed of various inorganic microelements. Based on element analysis via the method of inductively coupled plasma optical emission spectrometer (ICP-OES), the contents of Na, K, Cl, Fe, Mn, Mg, Mo, Ba, S, B, Al, Ti, Zn, Rb, Ni and Ir were 34.24, 13.35, 7.36, 1.37, 0.84, 0.44, 0.23, 0.21, 0.15, 0.12, 0.11, 0.09, 0.06, 0.04, 0.03 and 0.01 mg kg-1, respectively. BT is a mixture of amino acids, polypeptide and proteins, and generally recognized as polypeptides complexes. According to the formula provided by the company, it contains 5.1% amino acids (<180 Da), 34.2% small peptides (180~6000 Da), 31.6% polypeptide (6000~30000 Da) and 29.1% proteins (>30000 Da), respectively. The major types of amino acids were arginine, phenylalanine, valine, lysine, leucine, isoleucine, threonine and methionine. The commercial prices of BS and BT are $4.83 kg-1 and $4.14 kg-1, respectively.
RSM using the Box-Behnken Design (BBD) method was employed to optimize the culture medium with the supplement of BS and BT. The effects of three independent variables (concentrations of NaCl, BS and BT) at different ranges on biomass concentration and phycocyanin production were assessed. For this, a three-factor three-level experimental design was employed (Table 1). Ranges of variables were chosen based on our preliminary studies. According to single-factor experiments, the three factors had a positive effect on biomass and phycocyanin accumulation at low concentration, and showed an inhibitory effect at high concentration. The optimal concentration of each factor was selected as the central point of the BBD. The quantitative relationship between dependent [Y1: biomass concentration, Y2: phycocyanin concentration (CPCconc) and Y3: phycocyanin content (CPCcont)] and independent variables (A: NaCl, B: BS and C: BT) was determined. The experimental design, mathematical model and statistical analysis were carried out using Design-Export software (13.0.1.0).
Table 1 Independent variables (NaCl, BS and BT concentration) and their levels used in the Box-Behnken design.
According to BBD, the total experiments included 17 groups (Table 2). The medium of each experimental group differed by varying addition amounts of NaCl, BS, and BT into Zarrouk medium. All media were autoclaved at 121°C for 20 min after the initial pH was adjusted to 9.5 with 1 M NaOH. Microalgae cultivated at logarithmic growth phase were inoculated into 250 mL flasks with 100 mL culture medium at 10% (v/v) inoculation rate after the medium cooled to room temperature. Arthrospira maxima were cultivated at 31°C in shake flasks with rotation rate of 120 rpm, and illuminated by 6 light tubes at light intensity of 144 μ mol m−2 s−1 for 16 days. Then, microalgae cells were harvested by filtration to measure the biomass concentration, CPCconc and CPCcont. Each experiment was conducted in triplicate. Taking the maximum value of CPCconc and CPCcont as targets, respectively, validation experiments were conducted based on the optimal conditions predicted by the model.
Biomass concentration was determined by dry weight method. In brief, algal cells were harvested using 500 mesh screens, then washed with deionized water 2 times, and transferred to a pre-dried weighing disc, followed by oven drying at 75°C until constant weight. The biomass concentration (X) and productivity (Pbiomass) (Skorupskaite et al., 2015) were calculated using the following equations:
where m1 represents the weight (g) of dried weighing disc containing the sample, and m0 (g) represents the weight of pre-dried empty weighing disc. Vcul (L) is the sampling volume.
where X1 and X0 represent the biomass concentration (g L−1) on days t1 and t0, respectively.
Phycocyanin was extracted from Arthrospira maxima by using modified wet extraction method (Khazi et al., 2018). Specifically, 5 mL culture was filtered with 500 mesh screens to obtain wet biomass. It was washed by deionized water, re-suspended in 20 mL of 100 mM sodium phosphate buffer (pH 7), and subjected a freeze of -20°C for 12h. Thereafter, the sample was centrifuged at 8000×g for 10 min and the blue supernatant was collected. The CPCconc in the supernatant was determined by measuring the absorbance at 620 and 652 nm using ultraviolet-visible spectrophotometer (UV-5200) (Mogany et al., 2018; Novoveská et al., 2019). The concentration and the content of CPC were calculated as follows:
Where CPCconc was the concentration of CPC in the solution of microalgae, A620 and A652 were optical density of extracting solution at 620 and 652 nm, respectively. Vextr (L) and Vcul (L) were the volume of extracting solution and culture sample, respectively.
where CPCconc was the concentration of CPC (mg L-1) obtained from Eq. (3), and X was the biomass concentration (g L-1) obtained from Eq. (1).
The gross volumetric productivity of CPC was calculated using Eq. (5).
where CPCconc_end was the final CPC concentration (mg L-1), and t was the total duration of the cultivation (d).
After performing all experiments, the quadratic second-order equation with interaction terms was used to model the relationship between dependent and independent variables:
where yi, and β0 represent response variable and interception coefficient, respectively, and bi, bii, and bij are regression coefficients, n is the number of studied variables, xi and xj represent independent variables.
The statistical differences among the experiments were assessed by the analysis of variance (ANOVA) via the Design-Export software (13.0.1.0). The main effects and interaction effects of each factor were analyzed. All analyses are significant at p<0.05.
In order to achieve the maximal biomass concentration and phycocyanin accumulation, the relationship between the three independent and dependent variables was analyzed. The response surface models were identified for the coded factors and the quadratic second-order equations with interaction terms were used for data fitting. The final model equations and the variance analysis for the responses were shown in Table 3. The significance of the models was specified by low p-values (p<0.05) (Nemanja et al., 2019). The p-values of the fitting models of biomass concentration, phycocyanin concentration and content were 0.0007,<0.0001 and 0.001, respectively, indicating that all generated models were significant. Meanwhile, the high values of R2 (>0.9481) represented that all models were well fitting. For each model, the adequate precision was more than 4, which was desirable for good discrimination and indicated an adequate signal for the models to be used to navigate design space. Moreover, the low variation coefficients (1.33 to 2.28%) and the insignificant lack of fit (p>0.05) implied a high experimental reliability and a good correlation between the responses and the independent variables.
The biomass concentration and productivity of Arthrospira maxima under various cultivation conditions were summarized in Table 4. Compared with the control, the growth performance of most experimental groups decreased after adding three exogenous substances synchronously. There were only four treatments (run 3, 4, 10 and 11) in which the biomass concentrations and productivities were higher than that of the control. Among them, the run 10 showed the best microalgae growth, attaining the highest biomass concentration of 3.8 g L-1 and productivity of 0.22 g L-1 d-1. The common feature of these four treatments was the addition of middle-high concentration of NaCl (115 and 220 mM) and low-middle concentration of BT (20 and 50 mg L-1). Moreover, the perturbation plot revealed that the biomass was sensitive to NaCl and BT (Figure 1A). Hence, it can be speculated that adding a relative high concentration of NaCl and a small amount of BT can help to increase the biomass concentration. 3D response surfaces and contour plots showed more details about the relationships between the three variables and responses (Figure 2). The effect of exogenous substances at different concentration levels on the biomass concentration was shown in Figures 2A–C. The curvatures indicated that there was a strong interactive effect between the NaCl and BS concentration, as well as the NaCl and BT concentration with the low p-values of 0.0026 and 0.090, respectively (Table S1). Overall, the biomass concentration first increased and then declined with the increase of NaCl concentration at the fixed concentration of BT (50 mg L-1). However, the point of trend change depended on the BS concentration. For the combined impact of NaCl and BT, the maximal biomass concentration was achieved at the high NaCl and low BT concentration. The interaction between BS and BT was not significant for the growth of Arthrospira maxima (p>0.05, Table S1).
Figure 1 Perturbation plots of the dependent variables. (A) biomass concentration, (B) CPCconc, and (C) CPCcont. (A) NaCl concentration; (B) BS concentration; (C) BT concentration.
Figure 2 Response surface plots visualizing the interactive effects among the NaCl, BS and BT concentration for responses. (A–C) biomass concentration, (D–F) CPCconc, and (G–I) CPCcont.
Likewise, the relationships between the phycocyanin concentration and three independent variables were analyzed. The ANOVA results of the model showed that the single factors (A, C), interaction terms (AB, AC), and secondary terms (A2, B2) had significant effects on phycocyanin concentration and the p-values of these factors were all less than 0.05 (Table S2). The phycocyanin concentration was more sensitive to NaCl concentration than BS and BT concentrations (Figure 1B), which was confirmed by the linear model coefficients (Table 3). The interactive effect of NaCl and BS concentration was similar to that on biomass concentration (Figures 2A, D). Specifically, the effect of NaCl concentration on CPCconc was dependent on the BS concentration. The high CPCconc were obtained at the extreme low BS concentration (200 mg L-1) and NaCl concentration ranged from 125-220 mM (Figure 2E). The interactive effect of NaCl and BT concentration was significant but not strong. The CPCconc increased first and then decreased with the increase of NaCl concentration. The high concentration of BT reduced the CPCconc. A combination of a high concentration of NaCl (100-200 mM) and a low concentration of BT (20-25 mg L-1) was beneficial to the CPC accumulation. The interaction between BS and BT is not significant (p>0.05, Table S2). Both low concentrations of BT and BS is beneficial to CPC synthesis.
It was observed that a trend of first dramatically increasing and then decreasing phycocyanin content as the NaCl concentration increased from 50 mM to 220 mM (Figure 2G). The maximum CPCconc was found when the NaCl concentration ranged from 100 to 180 mM. The variation in BT concentration, however, had slight impact on the content of phycocyanin when the NaCl concentration was ranged from about 10-40 mM. The perturbation plot demonstrated that the CPCcont was sensitive to NaCl and BT concentrations (Figure 1C), and their interaction was significant (p<0.05, Table S3). 3D model presented that CPCcont was low at the extreme concentrations of NaCl and BT. When the concentration of NaCl or BT is constant, the CPCcont significantly increased first and then declined sharply with the increase of another factor. The sensitivity of CPCcont to BT was also reflected in the interaction between BS and BT (Figure 2I). As the increase of BT concentration from 20 to 80 mg L-1, the CPCcont increased first and then decreased, and the optimum concentration of BT was about 50 mg L-1. With the variation in BS concentration, the CPCcont did not change obviously, indicating that the CPCcont was not sensitive to BS, which was consistent with the results shown in the perturbation plots (Figure 1C).
The comparison between the actual experimental and the predicted data using the quadratic regression model was shown in Figure 3. The strong linear relationship indicated that there was a good agreement between the predicted and experimental results, demonstrating this model can be used in prediction and optimization. According to the model predication, the maximum CPCconc was achieved when the concentrations of NaCl, BS and BT were 185 mM, 200 mg L-1 and 20 mg L-1, respectively. However, it should be noted that the CPCcont was not maximum at this condition. The optimal condition estimated for obtaining the maximum CPCcont were 136 mM NaCl, 200 mg L-1of BS and 50 mg L-1 of BT. Subsequently, the validation experiments were performed at the optimal conditions. The experimental results (CPCconc of 704.66 mg L-1, CPCcont of 19.03%) were very close to the predicted data (CPCconc of 710.13 mg L-1, CPCcont of 18.63%) at the respective optimal condition (Figure 4).
Figure 3 Comparison between experimental and predicted values of (A) biomass concentration, (B) CPCconc, and (C) CPCcont.
Three factors (NaCl, BS, and BT) were chosen in this study to evaluate their impacts on the growth and phycocyanin production of Arthrospira maxima. The RSM model of algal biomass revealed that two of the factors, NaCl and BT, had a more substantial impact in comparison with BS concentration. The results of microalgae growth indicated that higher NaCl concentrations (100-220 mM) were advantageous for microalgae growth, which was basically consistent with previous studies (Abd El-Baky and El-Baroty, 2012). They reported that the medium containing 100 mM of NaCl can dramatically increase the growth of Arthrospira maxima as compared to control (20 mM NaCl), and the corresponding specific growth rate increased from 0.089 to 0.097 d-1. Nevertheless, cell proliferation of Arthrospira platensis was sharply slowed down when the concentration of NaCl raised above 500 mM because of more severe detrimental effect of salt stress (Chen and Tang, 2012). The content of chlorophyll and carotenoid in algal cell was also decreased, which consequentially resulted in declining of photosynthetic rate. The culture experiment results demonstrated that the addition of BT into medium was beneficial to microalgae growth. BT can be considered as nitrogen source as the primary component of BT is polypeptide. Nitrogen source is essential for cell growth and involved in the synthesis of amino acids, purines, pyrimidines, and other compounds used in cellular metabolism (Li et al., 2018). Hence, the increase of nitrogen concentration in a certain range can efficiently encourage the growth of microalgae (Mousavi et al., 2022). As the nitrogen concentration (NaNO3) increased from 0.625 to 2.5 g L-1, the biomass of Arthrospira platensis enhanced by 1.25 times at the culture temperature of 35°C (Can et al., 2017). In addition to nitrogen concentration, the type of nitrogen source was also highly affected the microalgae growth. Costa et al. (2001) observed that the optimal order of the nitrogen type for the growth of Arthrospira platensis was sodium nitrate, ammonium nitrate, acid ammonium phosphate, urea, ammonium sulphate, and ammonium chloride, at the same concentration of 10 mM. Interestingly, this order greatly changed when the nitrogen source concentration increased up to 30 and 50 mM, indicating the effect of nitrogen source on microalgae growth depended on its concentration. Besides, exogenous addition of organic nitrogen source into culture medium is a effective approach to enhance microalgae growth. Shanthi et al. (2018) found that the biomass productivity of Arthrospira platensis increased to 175, 153 and 144 mg L-1 d-1 by addition of L-Arginine, L-Asparagine and L-Glutamine, respectively. These growth rates were significantly higher than 114 mg L-1 d-1 obtained in the control group. And, these findings also confirmed that the type of nitrogen source (structure of amino acids) influenced the microalgae growth. Manirafasha et al. (2018) examined the effect of various concentration of sodium glutamate additionally added into Zarrouk medium on the growth of Arthrospira platensis, and found that the biomass concentration at 4 mM concentration of sodium glutamate reached 2.54 g L-1 that is much higher than ~1.4 g L-1 obtained in the control. In the present study, overall, algal biomass was gradually increased as the BT concentration increased, which is probably due to the beneficial impact of multiple amino acids degraded from polypeptide. In contrast, there was a little influence of BS on microalgae growth (p>0.05), which was possibly attributed to the sufficient minerals already existed in the Zarrouk medium. Further increased in minerals concentration did not allow the increase of microalgae growth.
NaCl had a huge impact on phycocyanin accumulation, as seen by the 3D surface plots and contour plots (Figures 2D–F). The phycocyanin concentration peaked when the NaCl concentration was 136 mM. Enhanced production of phycocyanin by NaCl is mostly related to the growth performance of microalgae and the reflection of phycocyanin synthesis metabolism to salt stress. On one hand, the increase in phycocyanin concentration caused by the ongoing accumulation of biomass in the region of 100-200 mM NaCl concentration. On the other hand, the phycocyanin concentration was associated with intracellular phycocyanin content. The increase of phycocyanin content can facilitate the production of phycocyanin. Phycocyanin can be generated in greater numbers like some antioxidant enzyme to resist damage from reactive oxygen radicals caused by environmental stresses (Singh et al., 2018; Gauthier et al., 2020). But an excessive concentration of NaCl will inhibit the synthesis of phycocyanin. Electron transport is hampered by extreme salt stress, which could halt the photosystem II activity and cause the amount of phycocyanin to decrease (Kirst, 1990).
However, the adaptability and domestication of Arthrospira can be used to overcome the challenge of high concentrations of NaCl impeding microalgae growth and phycocyanin. (Tredici et al., 1986; Hagemann, 2011). Liu et al. (2016) domesticated two strains of Arthrospira platensis (SP843 and SP972) using 200-800 mM of NaCl, and observed that they grew well in the medium containing 200 and 400 mM of NaCl, respectively. Meanwhile, the concentrations of phycocyanin increased by 180% and 157%, respectively, in comparison to the control. After salt acclimation, Arthrospira has a better capacity to produce phycocyanin at high salt concentration. Thereby, Arthrospira can be domesticated to increase its tolerance to salt stress, which can help Arthrospira culture in locations where excessive salinity makes it difficult.
As a nitrogen source, BT was conducive to the microalgae growth and phycocyanin production. In addition to affecting the growth of Arthrospira, the nitrogen source also affected the synthesis of phycocyanin. Nitrogen deficiency will limit the production of proteins associated with photosynthetic activity such as phycocyanin (Li et al., 2018). Thus, the increase of nitrogen concentration will improve the production of phycocyanin. The types of nitrogen sources also had an impact on phycocyanin accumulation. Gladfelter et al. (2022) discovered that compared to nitrate, the reduced nitrogen forms (ammonium/ammonia and urea) can much more promote algae growth and phycocyanin accumulation. In addition, in a research on improving the sources of nitrogen in the culture medium, the phycocyanin content was increased by 23% after optimizing the medium that included soda ash production wastes, NaNO3, KCl and KH2PO4 (Xie and Wang, 2017).
In this work, the addition of BS into the medium did not exert a obvious positive effect to the phycocyanin production. This result slightly differed from the findings of the other previous study. Mostly researches showed that the addition of mineral components can promote the phycocyanin accumulation. Compared to the control (Zarrouk medium), phycocyanin concentration increased by 1.6 times when the modified Zarrouk medium containing more Ca2+ from oyster shell and soil extract was used (Jung et al., 2014). A low concentration (<0.3 μg mL-1) of Ni increased the content of phycocyanin in Microcystis aeruginosa (Liu et al., 2005). It was reported that the dual effects of Se on promoting growth at low concentrations while also being toxic at high concentrations to Arthrospira (Chen et al., 2007). The addition of Se increased the phycocyanin concentration of Arthrospira maxima from 226 to 274 mg L-1 which was 21.2% higher than that in the control group (Chen et al., 2006). Most studies on the influence of mineral elements on phycocyanin presently focused on a single factor, such as Ni, Ca and Fe (Sloth et al., 2006; Velu et al., 2019; Rawat et al., 2022; Yang et al., 2022), and showed a good effect on phycocyanin. Arthrospira requires a small number of mineral components to grow. It is possibly that the conventional Zarrouk medium has enough minerals, to support the cell growth and phycocyanin production. Thus, the extra-addition of BS, a complex of different mineral elements, cannot generate significant positive impact, even showed inhibitory effect on phycocyanin production at a high concentration because of the resulted toxic of some metal elements.
In order to optimize the Arthrospira medium, we established the predicted models of algal biomass and phycocyanin production using response surface method. The maximum concentration (704.66 mg L-1) and content (19.03%) of phycocyanin were successfully achieved using the ideal conditions predicted by this model. These values were 22.98% and 16.73% higher than those of the control group.
At present, there have been many studies on improving the production of phycocyanin, and the corresponding results are summarized in Table 5. It can be seen that the phycocyanin concentration varied greatly, which ranged from 100-1500 mg L-1, with most of it concentrated in the range of 200-500 mg L-1. Also, phycocyanin content had a large range that from ~5% to ~20%. This great variation in concentration and content of CPC was probably caused by the different culture condition employed in the different literature, such as algal strains, culture medium, reactor type, culture mode, temperature and light intensity. In the current study, the maximum CPCconc and CPCcont reached 704.66 mg L-1 and 19.03%, respectively, and these values were comparable to or even higher than the most results obtained in the previous studies. It is noteworthy, in the study conducted by Chaiklahan et al. (2022), that the phycocyanin content reached 19.88% that is slightly higher than our result (19.03%), whereas the phycocyanin productivity (123.66 mg L-1 d-1) is much greater than 44.04 mg L-1 d-1 achieved by our study. The main reason was that they used the semi-continuous culture mode, in which the regular addition of fresh medium greatly ensured the nutrients required for the growth of microalgae, achieving a high biomass productivity (0.62 g L-1 d-1) and consequent high phycocyanin productivity. In contrast, the batch mode was employed in the present study and the resulted biomass productivity was only 0.23 g L-1 d-1. Surprisingly, the extreme high level of phycocyanin concentrations (>1000 mg L-1) was attained by Zeng et al. (2012) and Xie et al. (2015). In the former study, the intermittent supply of CO2 provided sufficient carbon source to microalgae growth, thus achieving a very high biomass concentration (7.26 g L-1). Xie et al. (2015) employed a feed-batch mode which is more efficient in terms of biomass accumulation compared to the batch mode, leading to a high biomass concentration (6.78 g L-1) and consequent high phycocyanin concentration (1034.8 mg L-1).
Table 5 Summary of algal growth and phycocyanin production of Arthrospira reported in the literature and this study.
It should be noted that the conecntration of NaCl in the culture medium was increased and additional BS and BT were added after optimizaiton, which will increase the produce cost. Thereby, we briefly conducted a economic analysis as it is very important and essential. At the optimal condition achieving the maxiumum phycocyanin concentration, the phycocyanin production will additionally increase 0.13 g L-1 compared to the control. However, the extra additon of these substances in 1 L culture medium brought extra cost (~$0.03) that was calculated through multipling the additional quantities of subtansces (NaCl, BS and BT) by their commerical prices. In terms of the market price of food-grade phycocyanin ($0.5 g-1) (Güroy et al., 2017), the extra production of phycocyanin per liter (0.13 g) valued approximately $0.07, which exceeded the input cost ($0.03). Therefore, it would be cost-effective and economically feasible for the phycocyanin production after optimizaiton.
Overall, a high level of phycocyanin production was achieved by optimization of culture medium added by BT and BS based on RSM. However, this study used the mode of batch culture. Moreover, during the process, there was no additional carbon (such as CO2) supplied into the medium. Therefore, more work could be performed in the next step to further increase the phycocyanin productivity by using feed-batch or semi-continuous mode, as well as providing additional carbon source based on the optimized medium.
In this study, the forecasting models has been established based on RSM, aiming to optimize culture conditions to enhance algal biomass and phycocyanin accumulation. According to the models, it was observed that NaCl was the most important factor to the responses. Meanwhile, the interactive effect of NaCl and BT had the greatest effect on phycocyanin production. The maximum values of phycocyanin concentration and content (704.66 mg L-1 and 19.03%) were reached at the respective optimal conditions determined by the models. Hence, BS and BT can be potentially considered as the great exogenous additives to promote Arthrospira maxima growth and phycocyanin synthesis. In the next step, semi-continuous or feed-batch mode as well as the supply of CO2 can be used to further increase the productivity of algal biomass and phycocyanin.
The original contributions presented in the study are included in the article/Supplementary Material. Further inquiries can be directed to the corresponding authors.
TY: conceptualization, methodology, data curation, formal analysis, visualization, and writing-original draft. JH: conceptualization, methodology, data curation, formal analysis, investigation, supervision and writing-review and editing, funding acquisition. BS: investigation, data curation, formal analysis. LW: methodology, data curation, formal analysis. A-HZ: conceptualization, writing-review and editing, funding acquisition. D-FZ: funding acquisition, project administration. YZ: project administration, resources. GM: project administration, resources. All authors contributed to the article and approved the submitted version.
The study was supported by Nantong social livelihood science and technology plan (MS12021024), and National Natural Science Foundation of China (32273157).
YZ and GM were employed by Jiangsu Coast Development Group Co., Ltd.
The remaining authors declare that the research was conducted in the absence of any commercial or financial relationships that could be construed as a potential conflict of interest.
All claims expressed in this article are solely those of the authors and do not necessarily represent those of their affiliated organizations, or those of the publisher, the editors and the reviewers. Any product that may be evaluated in this article, or claim that may be made by its manufacturer, is not guaranteed or endorsed by the publisher.
The Supplementary Material for this article can be found online at: https://www.frontiersin.org/articles/10.3389/fmars.2022.1057201/full#supplementary-material
Abd El-Baky H. H., El-Baroty G. S. (2012). Characterization and bioactivity of phycocyanin isolated from spirulina maxima grown under salt stress. Food Funct. 3 (4), 381–388. doi: 10.1039/c2fo10194g
Anvar A. A., Nowruzi B. (2021). Bioactive properties of spirulina: A review. Microbial Bioactives 4 (1), 134–142. doi: 10.25163/microbbioacts.412117B0719110521
Bachchhav M. B., Kulkarni M. V., Ingale A. G. (2016). Enhanced phycocyanin production from spirulina platensis using light emitting diode. J. Institution Engineers (India): Ser. E 98 (1), 41–45. doi: 10.1007/s40034-016-0090-8
Bezerra M. A., Santelli R. E., Oliveira E. P., Villar L. S., Escaleira L. A. (2008). Response surface methodology (RSM) as a tool for optimization in analytical chemistry. Talanta 76 (5), 965–977. doi: 10.1016/j.talanta.2008.05.019
Can S. S., Koru E., Cirik S. (2017). Effect of temperature and nitrogen concentration on the growth and lipid content of spirulina platensis and biodiesel production. Aquaculture Int. 25 (4), 1485–1493. doi: 10.1007/s10499-017-0121-6
Chaiklahan R., Chirasuwan N., Srinorasing T., Attasat S., Nopharatana A., Bunnag B. (2022). Enhanced biomass and phycocyanin production of arthrospira (Spirulina) platensis by a cultivation management strategy: Light intensity and cell concentration. Bioresour Technol. 343, 126077. doi: 10.1016/j.biortech.2021.126077
Chen C. Y., Kao P. C., Tan C. H., Show P. L., Cheah W. Y., Lee W. L., et al. (2016). Using an innovative pH-stat CO2 feeding strategy to enhance cell growth and c-phycocyanin production from spirulina platensis. Biochem. Eng. J. 112, 78–85. doi: 10.1016/j.bej.2016.04.009
Chen M., Tang Y. L. (2012). Investigation on the detrimental effects of salt stress on photosynthesis of spirulina platensis. Agric. Sci. & Technol. 8), 1625–1627+1770. doi: 10.16175/j.cnki.1009-4229.2012.08.042
Chen S. J., Yang F., Zheng W. J., Bai Y., Huang Z., Zhou Y. H. (2007). Effects of selenium stress on antioxidase system of spirulina platensis. Acta Hydrobiologica Sin. 5), 706–711. doi: 10.1016/S1872-2032(08)60016-5
Chen T. F., Zheng W. J., Yang F., Bai Y., Wong Y. S. (2006). Mixotrophic culture of high selenium-enriched spirulina platensis on acetate and the enhanced production of photosynthetic pigments. Enzyme Microbial Technol. 39 (1), 103–107. doi: 10.1016/j.enzmictec.2005.10.001
Chini Z. G., Mugnai G., Milia M., Cicchi B., Silva B. A.M., Angioni A., et al (2022). Effects of blue, orange and white lights on growth, chlorophyll fluorescence, and phycocyanin production of Arthrospira platensis cultures. Algal Res 61, 102583. doi: 10.1016/j.algal.2021.102583
Costa J. A. V., Cozza K. L., Oliveira L., Magagnin G. (2001). Different nitrogen sources and growth responses of spirulina platensis in microenvironments. World J. Microbiol. Biotechnol. 17 (5), 439–442. doi: 10.1023/a:1011925022941
Fratelli C., Burck M., Amarant M. C. A., Braga A. R. C. (2021). Antioxidant potential of nature's "something blue": Something new in the marriage of biological activity and extraction methods applied to c-phycocyanin. Trends Food Sci. Technol. 107, 309–323. doi: 10.1016/j.tifs.2020.10.043
Gauthier M. R., Senhorinho G. N. A., Scott J. A. (2020). Microalgae under environmental stress as a source of antioxidants. Algal Res. 52, 102104. doi: 10.1016/j.algal.2020.102104
Gladfelter M. F., Buley R. P., Belfiore A. P., Fernandez-Figueroa E. G., Gerovac B. L., Baker N. D., et al. (2022). Dissolved nitrogen form mediates phycocyanin content in cyanobacteria. Freshw. Biol. 67 (6), 954–964. doi: 10.1111/fwb.13892
Güroy B., Karadal O., Mantoğlu S., Cebeci O. I. (2017). Effects of different drying methods on c-phycocyanin content of spirulina platensis powder. Ege J. Fisheries Aquat. Sci. 34 (2), 129–132. doi: 10.12714/egejfas.2017.34.2.02
Hagemann M. (2011). Molecular biology of cyanobacterial salt acclimation. FEMS Microbiol. Rev. 35 (1), 87–123. doi: 10.1111/j.1574-6976.2010.00234.x
Hsieh-Lo M., Castillo G., Ochoa-Becerra M. A., Mojica L. (2019). Phycocyanin and phycoerythrin: Strategies to improve production yield and chemical stability. Algal Res. 42, 101600. doi: 10.1016/j.algal.2019.101600
Jiang L. Q., Wang Y. J., Yin Q. F., Liu G. X., Liu H. H., Huang Y. J., et al. (2017). Phycocyanin: A potential drug for cancer treatment. J. Cancer 8 (17), 3416–3429. doi: 10.7150/jca.21058
Jung J. Y., Kim S., Lee H., Kim K., Kim W., Park M. S., et al. (2014). Use of extracts from oyster shell and soil for cultivation of spirulina maxima. Bioprocess Biosyst. Eng. 37 (12), 2395–2400. doi: 10.1007/s00449-014-1216-3
Khazi M. I., Demirel Z., Conk Dalay M. (2018). Enhancement of biomass and phycocyanin content of spirulina platensis. Front. bioscience (Elite edition) 10 (2), 276–286. doi: 10.2741/e822
Khazi M. I., Demirel Z., Liaqat F., Dalay M. C. (2020). Analytical grade purification of phycocyanin from cyanobacteria. Methods Mol. Biol. (Clifton N.J.) 1980, 173–179. doi: 10.1007/7651_2018_202
Kirst G. O. (1990). Salinity tolerance of eukaryotic marine algae. Annu. Rev. Plant Biol. 41 (21), 53. doi: 10.1146/annurev.pp.41.060190.000321
Lawrenz E., Fedewa E. J., Richardson T. L. (2011). Extraction protocols for the quantification of phycobilins in aqueous phytoplankton extracts. J. Appl. Phycology 23 (5), 865–871. doi: 10.1007/s10811-010-9600-0
Li Y. (2022). The bioactivities of phycocyanobilin from spirulina. J. Immunol. Res. 2022, 4008991. doi: 10.1155/2022/4008991
Li X., Li W., Zhai J., Wei H. (2018). Effect of nitrogen limitation on biochemical composition and photosynthetic performance for fed-batch mixotrophic cultivation of microalga spirulina platensis. Bioresour Technol. 263, 555–561. doi: 10.1016/j.biortech.2018.05.046
Liu J., Chang X. X., Huang L. J., Ma Z. G. (2005). Effects of Ni on the growth, absorption spectrum and phycobiliprotein content of microcystis aeruginosa. J. Yunnan Univ. (Natural Science) 27 (4), 365–368. doi: 10.3321/j
Liu C., Li L. J., Wu C. Y., Guo K. N., Li J. H. (2016). Growth and antioxidant production of spirulina in different NaCl concentrations. Biotechnol. Lett. 38 (7), 1089–1096. doi: 10.1007/s10529-016-2087-2
Lu Q., Li H. K., Xiao Y., Liu H. (2021). A state-of-the-art review on the synthetic mechanisms, production technologies, and practical application of polyunsaturated fatty acids from microalgae. Algal Research-Biomass Biofuels Bioproducts 55. doi: 10.1016/j.algal.2021.102281
Manirafasha E., Murwanashyaka T., Ndikubwimana T., Rashid Ahmed N., Liu J., Lu Y., et al. (2018). Enhancement of cell growth and phycocyanin production in arthrospira (Spirulina) platensis by metabolic stress and nitrate fed-batch. Bioresour Technol. 255, 293–301. doi: 10.1016/j.biortech.2017.12.068
Manirafasha E., Ndikubwimana T., Zeng X. H., Lu Y. H., Jing K. J. (2016). Phycobiliprotein: Potential microalgae derived pharmaceutical and biological reagent. Biochem. Eng. J. 109, 282–296. doi: 10.1016/j.bej.2016.01.025
Mogany T., Kumari S., Swalaha F. M., Bux F. (2018). Extraction and characterisation of analytical grade c-phycocyanin from euhalothece sp. J. Appl. Phycology 31 (3), 1661–1674. doi: 10.1007/s10811-018-1661-5
Mousavi M., Mehrzad J., Najafi M. F., Zhiani R., Shamsian S.A.I A. (2022). Nitrate and ammonia: two key nitrogen sources for biomass and phycocyanin production by arthrospira (Spirulina) platensis. J. Appl. Phycology. 5, 2271–2281. doi: 10.1007/s10811-021-02664-0
Nemanja T., Nemanja B., Dušan R., Aleksandar T., Zoran Z., Aleksandar F., et al. (2019). Defatted wheat germ as source of polyphenols–optimization of microwave-assisted extraction by RSM and ANN approach. Chem. Eng. Process. - Process Intensification 143, 107634. doi: 10.1016/j.cep.2019.107634
Nethravathy, Mehar J. G., Mudliar S. N., Shekh A. Y. (2019). Recent advances in microalgal bioactives for food, feed, and healthcare products: Commercial potential, market space, and sustainability. Compr. Rev. Food Sci. Food Saf. 18 (6), 1882–1897. doi: 10.1111/1541-4337.12500
Novoveská L., Ross M. E., Stanley M. S., Pradelles R., Wasiolek V., Sassi J. F. (2019). Microalgal carotenoids: A review of production, current markets, regulations, and future direction. Mar. Drugs 17 (11), 640–661. doi: 10.3390/md17110640
Pagels F., Guedes A. C., Amaro H. M., Kijjoa A., Vasconcelos V. (2019). Phycobiliproteins from cyanobacteria: Chemistry and biotechnological applications. Biotechnol. Adv. 37 (3), 422–443. doi: 10.1016/j.biotechadv.2019.02.010
Pandey V. D., Pandey A., Sharma V. (2013). Biotechnological applications of cyanobacterial phycobiliproteins. int.j.curr.microbiol.app.sci. 2, 89–97.
Prabakaran G., Sampathkumar P., Kavisri M., Moovendhan M. (2020). Extraction and characterization of phycocyanin from spirulina platensis and evaluation of its anticancer, antidiabetic and antiinflammatory effect. Int. J. Biol. Macromol 153, 256–263. doi: 10.1016/j.ijbiomac.2020.03.009
Rawat D., Sharma U., Poria P., Finlan A., Parker B., Sharma R. S., et al. (2022). Iron-dependent mutualism between chlorella sorokiniana and ralstonia pickettii forms the basis for a sustainable bioremediation system. ISME Commun. 2 (1), 83–96. doi: 10.1038/s43705-022-00161-0
Romay C., Gonzalez R., Ledon N., Remirez D., Rimbau V. (2003). C-phycocyanin: A biliprotein with antioxidant, anti-inflammatory and neuroprotective effects. Curr. Protein Pept. Sci. 4 (3), 207–216. doi: 10.2174/1389203033487216
Sathasivam R., Radhakrishnan R., Hashem A., Abd Allah E. F. (2019). Microalgae metabolites: A rich source for food and medicine. Saudi J. Biol. Sci. 26 (4), 709–722. doi: 10.1016/j.sjbs.2017.11.003
Shanthi G., Premalatha M., Anantharaman N. (2018). Effects of l-amino acids as organic nitrogen source on the growth rate, biochemical composition and polyphenol content of spirulina platensis. Algal Res. 35, 471–478. doi: 10.1016/j.algal.2018.09.014
Silva S. C., Ferreira I. C. F. R., Dias M. M., Barreiro M. F. (2020). Microalgae-derived pigments: A 10-year bibliometric review and industry and market trend analysis. Molecules 25 (15), 3406–3428. doi: 10.3390/molecules25153406
Singh R., Upadhyay A. K., Chandra P., Singh D. P. (2018). Sodium chloride incites reactive oxygen species in green algae chlorococcum humicola and chlorella vulgaris: Implication on lipid synthesis, mineral nutrients and antioxidant system. Bioresour Technol. 270, 489–497. doi: 10.1016/j.biortech.2018.09.065
Skorupskaite V., Makareviciene V., Levisauskas D. (2015). Optimization of mixotrophic cultivation of microalgae chlorella sp for biofuel production using response surface methodology. Algal Research-Biomass Biofuels Bioproducts 7, 45–50. doi: 10.1016/j.algal.2014.12.001
Sloth J. K., Wiebe M. G., Eriksen N. T. (2006). Accumulation of phycocyanin in heterotrophic and mixotrophic cultures of the acidophilic red alga galdieria sulphuraria. Enzyme Microbial Technol. 38 (1-2), 168–175. doi: 10.1016/j.enzmictec.2005.05.010
Soni R. A., Sudhakar K., Rana R. S. (2017). Spirulina – from growth to nutritional product: A review. Trends Food Sci. Technol. 69, 157–171. doi: 10.1016/j.tifs.2017.09.010
Thevarajah B., Nishshanka G. K. S. H., Premaratne M., Nimarshana P. H. V., Nagarajan D., Chang J. S., et al. (2022). Large-Scale production of spirulina-based proteins and c-phycocyanin: A biorefinery approach. Biochem. Eng. J. 185, 108541. doi: 10.1016/j.bej.2022.108541
Tredici M. R., Papuzzo T., Tomaselli L. (1986). Outdoor mass culture ofSpirulina maximain sea-water. Appl Microbiol Biot. 24, 1, 47–50. doi: 10.1007/BF00266284
Velu C., Cires S., Brinkman D. L., Heimann K. (2019). Effect of CO2 and metal-rich waste water on bioproduct potential of the diazotrophic freshwater cyanobacterium, tolypothrix sp. Heliyon 5 (4), e01549. doi: 10.1016/j.heliyon.2019.e01549
Verma R., Kumari K. V. L. K., Srivastava A., Kumar A. (2020). Photoautotrophic, mixotrophic, and heterotrophic culture media optimization for enhanced microalgae production. J. Environ. Chem. Eng. 8 (5), 104199. doi: 10.1016/j.jece.2020.104149
Xie Y., Jin Y., Zeng X., Chen J., Lu Y., Jing K. (2015). Fed-batch strategy for enhancing cell growth and c-phycocyanin production of arthrospira (Spirulina) platensis under phototrophic cultivation. Bioresour Technol. 180, 281–287. doi: 10.1016/j.biortech.2014.12.073
Xie L. D., Wang S. Y. (2017). Optimization of Spirulina media by response surface methodology. Acta Agriculturae Zhejiangensis 29 (2), 307–314. doi: 10.3969/j.issn.1004-1524.2017.02.18
Yang J., Li W., Xing C., Xing G., Guo Y., Yuan H. (2022). Ca(2+) participates in the regulation of microalgae triacylglycerol metabolism under heat stress. Environ. Res. 208, 112696. doi: 10.1016/j.envres.2022.112696
Yu P., Wu Y. T., Wang G. W., Jia T. M., Zhang Y. S. (2017). Purification and bioactivities of phycocyanin. Crit. Rev. Food Sci. Nutr. 57 (18), 3840–3849. doi: 10.1080/10408398.2016.1167668
Zarrouk C. (1996). Contribution to the study of a cyanophyceae: Influence of various physical and chemical factors on the growth and photosynthesis of Spirulina maxima. PhD Thesis, University of Paris, Paris.
Keywords: Arthrospira maxima, phycocyanin, response surface methodology, mineral elements complexes, polypeptides, medium optimization
Citation: Yao T, Huang J, Su B, Wei L, Zhang A-H, Zhang D-F, Zhou Y and Ma G (2022) Enhanced phycocyanin production of Arthrospira maxima by addition of mineral elements and polypeptides using response surface methodology. Front. Mar. Sci. 9:1057201. doi: 10.3389/fmars.2022.1057201
Received: 29 September 2022; Accepted: 31 October 2022;
Published: 21 November 2022.
Edited by:
Liandong Zhu, Wuhan University, ChinaReviewed by:
Hongli Cui, Shanxi Agricultural University, ChinaCopyright © 2022 Yao, Huang, Su, Wei, Zhang, Zhang, Zhou and Ma. This is an open-access article distributed under the terms of the Creative Commons Attribution License (CC BY). The use, distribution or reproduction in other forums is permitted, provided the original author(s) and the copyright owner(s) are credited and that the original publication in this journal is cited, in accordance with accepted academic practice. No use, distribution or reproduction is permitted which does not comply with these terms.
*Correspondence: Jianke Huang, amtodWFuZ0BoaHUuZWR1LmNu; Ai-Hua Zhang, YWh6QGhodS5lZHUuY24=
Disclaimer: All claims expressed in this article are solely those of the authors and do not necessarily represent those of their affiliated organizations, or those of the publisher, the editors and the reviewers. Any product that may be evaluated in this article or claim that may be made by its manufacturer is not guaranteed or endorsed by the publisher.
Research integrity at Frontiers
Learn more about the work of our research integrity team to safeguard the quality of each article we publish.