- 1School of Marine Sciences, Zhuhai Key Laboratory of Marine Bioresources and Environment, Guangdong Provincial Key Laboratory of Marine Resources and Coastal Engineering, Sun Yat-Sen University, and Southern Marine Science and Engineering Guangdong Laboratory (Zhuhai), Zhuhai, China
- 2Department of Biology, Center for Environment, Biodiversity and Conservation, The University of Texas at Tyler, Tyler, TX, United States
Reserve planning based on the investigation of the core habitat distribution of wild cetaceans is a powerful conservation strategy for protecting target species. However, studies on core habitats and their variations at the large-scale distribution of cetaceans are limited. In this study, we conducted a seven years (2015-2021) boat-based field observation surveys with highly applicable and generalized methods to analyze the habitat changes and how these changes influenced the largest known Indo-Pacific humpback dolphin (Sousa chinensis) population in the Pearl River Estuary (PRE), China. Our findings revealed that there is 3204 km2 of dolphin habitat in the PRE, and dolphin distribution preferences are related to nutrients and biological resources. The decreasing trend of dolphin sighting rates in most areas (73.2%) indicated that the dolphin habitat had declined, and the annual habitat decline rate (2.83%; 95% confidence interval: 0.58%-5.08%) was consistent with the population decline rate. The annual habitat decline rate in the hypoxic zone revealed that low dolphin sighting rates from March to October in the hypoxic zone was 0.43% faster than that in the non-hypoxic zone, indicating long-term sustained effects of summer hypoxia on the dolphin population. Existing reserves have partially prevented habitat decline. However, more than 82.3% of the habitat has not been effectively protected and encounters the threats of pollution and hypoxic stress. This study offers new scientific evidence for developing effective monitoring strategies for humpback dolphin populations in the PRE, and also help establish spatial planning and management measures for reserve habitats.
Introduction
Habitat is a natural environment that wild animal populations depend on for survival and reproduction, and ensuring the integrity of a habitat, including size, mass structure, and connectivity, is key to maintaining the long-term survival of the population (Doak, 1995; Griffen and Drake, 2008). Compared to the pelagic cetaceans, nearshore dolphins are more susceptible to various human disturbances, especially the development of coastal infrastructure, and other environmental changes (Thompson et al., 2000; Stockin et al., 2008). Many species living in coastal waters face the danger of declining populations due to habitat deterioration and loss, which has become an important factors in wildlife extinction (Fahrig, 2003; Bearzi et al., 2004; Ross et al., 2011). Identifying the core habitats of wildlife populations can provide essential foundational information for planning and managing nature reserves (a powerful conservation strategy) (Bailey and Thompson, 2009; Fahrig and Triantis, 2013). Therefore, understanding the habitat dynamics of endangered cetaceans and identifying the main factors influencing population conservation is important.
The Pearl River Estuary (PRE) and its adjacent waters are home to the largest known population (> 2000 individuals) of Indo-Pacific humpback dolphins (Sousa chinensis) worldwide, which is of great significance for the preservation of precious resources of this species (Chen et al., 2010; Jefferson and Smith, 2016). Currently the species S. chinensis has been classified as Vulnerable (VU) on the IUCN Red Species List, but the PRE population has not yet been officially evaluated on the Red Species List. Variuos studies have indicated that this population could reach the status of Endangered (EN) or even Critically Endangered (CR) (Huang et al., 2012; Chan, 2019). Several previous studies have investigated the life history, population size, survival rates, age structure, feeding habits, socio-behavioral dynamics, and pollutant accumulation in Indo-Pacific humpback dolphins in the PRE (Chen et al., 2010; Jefferson et al., 2012; Lin et al., 2021b; Sun et al., 2022). Since cetaceans have a fully aquatic lifestyle and are difficult to observe, surveys of their habitats are often subject to various limitations, such as weather conditions, and human and material resources. Sighting surveys are also time consuming for collecting sufficient data. The dynamics of core habitats of humpback dolphins on continuous time scales and the identification their environmental characteristics have rarely been considered in the PRE, to date, there have been no published studies on the core habitat distribution.
Indo-Pacific humpback dolphins mainly live along the coast from tropical to warm temperate zones (from central China in the east, southward throughout Southeast Asia, and westward around the coastal rim of the Bay of Bengal to at least the Orissa coast of eastern India), including bays, lagoons, estuaries, and other water regions, with depths of no more than 30 m (Karczmarski et al., 2015; Jefferson and Smith, 2016). Oceanographic variables (e.g., distance from shore, water depth, chlorophyll-a concentration, and salinity) and ecological conditions (e.g., prey distribution and abundance) and determine the spatial patterns of humpback dolphins (Wu et al., 2017a; Lin et al., 2021a). However, the inshore distribution of humpback dolphins makes their habitats extremely vulnerable to human activity. Their habitats may also change over time, depending on the variability of the population, dietary availability, and deterioration of their living environment (Karczmarski et al., 2015). Therefore, habitat changes of humpback dolphins in the PRE and the related influencing factors deserve further study.
The PRE is a rapidly developing metropolitan area that is influenced by some of the most intense human activity (Halpern et al., 2015). Phytoplankton blooms and episodic events of hypoxia (dissolved oxygen concentrations of < 2 mg L-1 or 62.5 μmol L-1) occur frequently in the PRE and adjacent coastal waters (Li et al., 2020b). Coastal hypoxia not only alters sedimentary structures and biogeochemical cycles but also deteriorates the sustainability of marine ecosystems, including increased biological mortality, loss of reproductive capacity, and changing benthic communities (Conley et al., 2010; Rabalais et al., 2010; Breitburg et al., 2018). Previous studies have reported reported the potential ecological effects of hypoxia on a variety of low-trophic marine organisms, including zooplankton, crustaceans, macro-invertebrates, and fish (Levin et al., 2009; Roman et al., 2019). However, the effects of hypoxia on the habitats of marine mammals such as cetaceans have rarely been studied.
In this study, the distribution and changes in the core habitat of the PRE humpback dolphin population were studied over seven years (2015-2021) using boat-based field observation surveys by employing a generalized analysis method. We investigated the relationships between different dolphin sightings and the characteristics of the water environment using factor analyses. We further compared the habitat change trends in different areas each year during the surveys to explore the impact of existing reserves and hypoxic zones on dolphin habitats. Our findings provide a new scientific perspective and an important approach to explore effective monitoring programs, spatial planning and adjustment managements of natural resource conservations.
Materials and methods
Study area
The Pearl River Estuary (21°30′-23°30′ N and 112°30′-114°30′E) is one of the most industrialized and densely urbanized areas in the world, encircled by cities including Hong Kong, Shenzhen, Dongguan, Guangzhou, Zhongshan, Zhuhai, and Macao (Figure 1). The water depth is < 30 m and the annual average water surface temperature averages is 24°C (Liu et al., 2020). A multi-year mean runoff (freshwater containing many nutrients) amounts to 3.3 × 1011 m3 in the PRE with eight waterways which receives annual ammonia, nitrate, and phosphate loads of 2.64 × 104, 4.30× 105, and 2.55 × 104 tons, respectively (Cui et al., 2019; Li et al., 2020a). Hypoxia in summertime sporadically occurred in the lower PRE for more than three decades and showed a similar pattern every year (Cui et al., 2019; Li et al., 2020a). Previous studies have indicated that, the eastern boundary of the PRE Indo-Pacific humpback dolphin population was Hong Kong waters, while the western boundary might extend westward to Mangzhou Island (Chen et al., 2010; Li et al., 2019). Based on the background information provided by early studies, the current nature reserves have been stablished and include two major parts: the highlighted Lingding Bay (460 km2) in the eastern PRE extending from the west coast of Hong Kong to the east coast of Macao, and the region near Dajin Island (108 km2) in the western PRE (Figure 1).
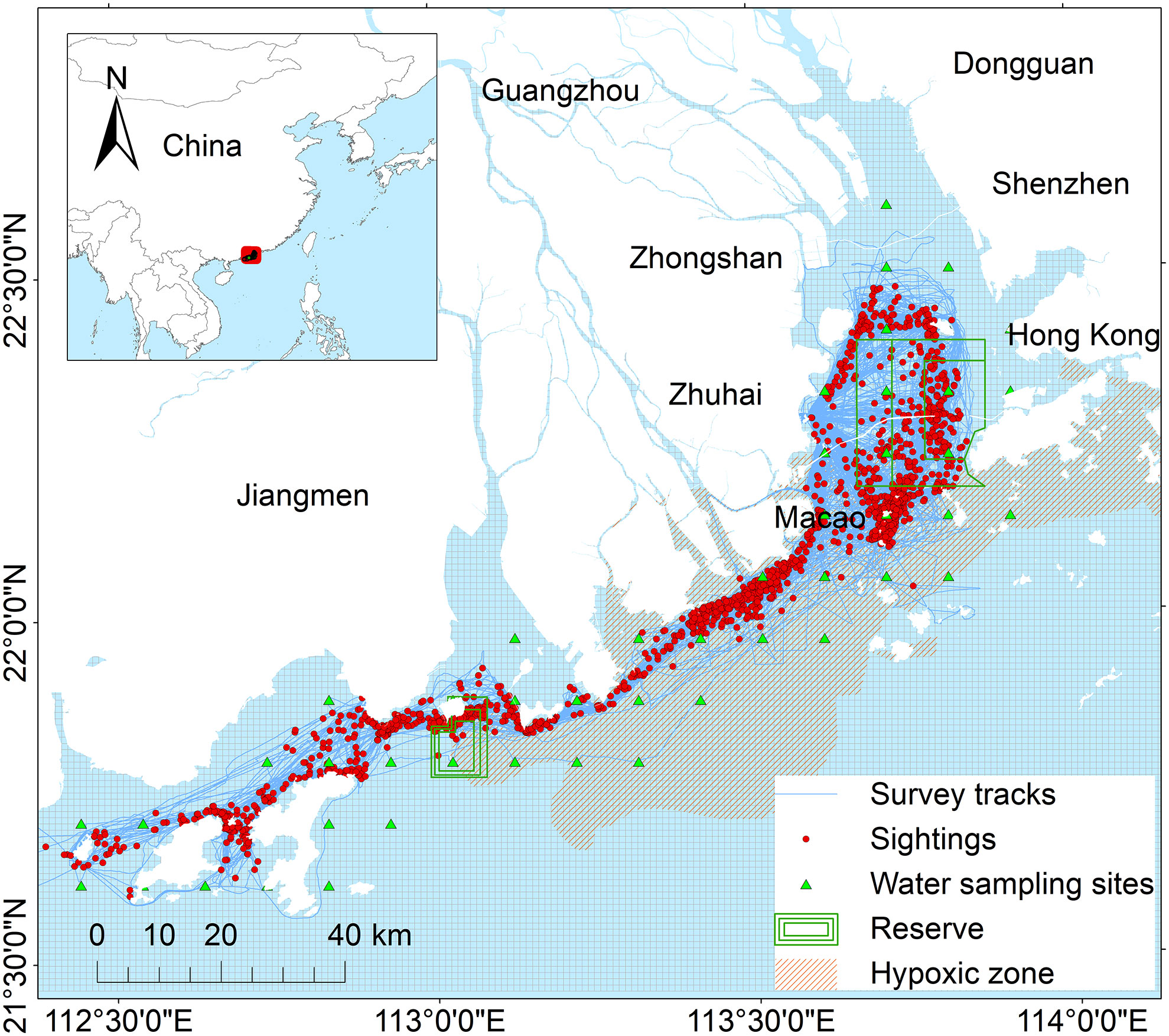
Figure 1 The study area embedded with a fishnet (Each cell size represents 1 ×1 km) in the Pearl River Estuary during 2015-2021. Legends include locations of Indo-Pacific humpback dolphin sightings (red dots), survey tracks (blue lines), water sampling sites (green triangles), reserve area (green boxes) and hypoxic zone (orange shaded area).
Data collection
Data on humpback dolphins were collected from 2015–2021 using boat-based observational surveys. The observation surveys were conducted using small boats equipped with Suzuki 90-120 4-stroke outboard engines, and the cruise surveys generally ranged from 7.8 – 8.2 m, with more surveys in the east than in the west PRE. Survey protocols, including field sampling methods, have beene described in previous studies (Chan and Karczmarski, 2017; Guo et al., 2020). Briefly, the survey team consisted of at least three observers continuously scanning the front and sides of the boat with the naked eyes. Once sighted, the dolphins were approached from the side at a low boat speed, and waypoints were recorded using a Garmin handheld GPS map78s at the sighting location. The survey tracks and sighted humpback dolphins are shown in Figure 1.
We collected environmental data in the study area, including offshore distance and distance to the estuary, water quality parameters, and hypoxic zone distribution. The offshore distance and distance to estuary were calculated by the tool “Near” of ArcMap v10.2 The 26 water quality indices included depth, temperature, salinity, pH, dissolved oxygen (DO), chemical oxygen demand (COD), ammonia nitrogen, nonionic ammonia, fecal coliform, chlorophyll-a, suspended solids, inorganic nitrogen, petroleum, active phosphate, total phosphorus, total chromium, total nitrogen, nitrogen nitrite, nitrogen nitrate, As, Cu, Cr, Cd, Pb, Hg, and Zn during the investigation period, which were obtained from the Department of Ecology and Environment of Guangdong Province in China (http://gdee.gd.gov.cn/jhszl/index.html). Data on the hypoxic zone distribution during the investigation period were obtained according to published databases (Li et al., 2020a) and (Cui et al., 2019). The water sampling sites and hypoxic zone distribution are shown in Figure 1.
Data analyses
The total humpback dolphin ranging area could be ≤ 5000 km2, and its east-west distribution spans approximately 200 km (Chen et al., 2010). Considering that it is difficult to evenly cover the entire distribution range of humpback dolphins using the classic and widely used boat-based survey methods of marine ecological surveys and effort in various regions may not be uniform, a kernel density analysis method that could eliminate the impacts of effort was used to estimate the core habitat distribution of humpback dolphins in this study. The proposed analysis method may provide an important reference for the habitat study of cetacean populations with wide distribution areas and difficulty in regional survey investigations. On the survey map (Figure 1), fishnet grips with a cell size of 1 ×1 km in the study area were created using the ArcMap v10.2 toolbox. By counting the sightings and surveys of each cell, we calculated the sighting rate (sightings/surveys/km2) for each cell from 2015 – 2021. Using the kernel density of the ArcMap v10.2 toolbox, spatial analyses were conducted according to the sighting locations, selecting the population as the sighting rate and setting the search radius to 5 km. The geographic coordinate system used in this process was WGS 1984 and the projected coordinate system was WGS 1984 UTM Zone 49N. We calculated Kendall’s tau coefficients of the sightings rates of humpback dolphins and the year of each cell using MATLAB (MathWorks, R2020b) to evaluate the increased sightings rate (Kendall’s tau > 0) or decreased one (Kendall’s tau < 0) of humpback dolphins in each cell (p < 0.05 significance level).
Based on the distribution of water sampling sites, this study explored the kriging interpolation method by integrating water sampling sites with dolphin sighting lofcations and retrieved 26 water quality parameters per cell in humpback dolphin habitats. We calculated Spearman's correlations between different sighting rates and environmental factors, selected the factors with a significant correlation (p < 0.05) with dolphin sighting rates for factor analyses, and obtained the relationship between dolphin sighting rates and different factors in RStudio (RStudio 2022). Similarly, we selected the environmental factors that had significant differences (Mann-Whitney U-test, p < 0.05) in the areas where dolphin sightings increased significantly (Kendall’s tau > 0, p < 0.05) and decreased significantly (Kendall’s tau < 0, p < 0.05), and incorporated these factors into factor analyses to explore the relationships between environmental factors and the changes in dolphin sighting rates using RStudio (RStudio 2022).
In addition, we calculated the sighting area rates (annual sighting area/annual survey area) of humpback dolphins in different regions (on or off the reserve, and in hypoxic and non-hypoxic zones) of each year, and fitted the linear relationships between the sighting area rate and the year with its 95% confidence interval (CI), which could indicate whether the distribution of humpback dolphins changed in recent years. Considering the seasonal occurrence of hypoxic phenomena, we further compared the differences (Mann-Whitney U-test) in dolphin sighting rates in the same month and the consistency (two-sample Kolmogorov-Smirnov test) of the monthly average sighting rates between the hypoxic and non-hypoxic zones to explore the influence of the hypoxic zone on dolphin distribution.
Results
Three hundred and seventy boat-based surveys were conducted during the investigation period from 2015-2021. The total survey time was 1900 h with a survey distance of over 37,000 km, and a total of 1775 humpback dolphin groups were sighted (Table 1). On average, 53 (SD ± 11) surveys were conducted and approximately 254 (SD ± 96) groups of humpback dolphins were sighted per year, with an annual investigation time of approximately 275 h (SD ± 70) h and a survey distance of approximately 5345 km (SD ± 1009).
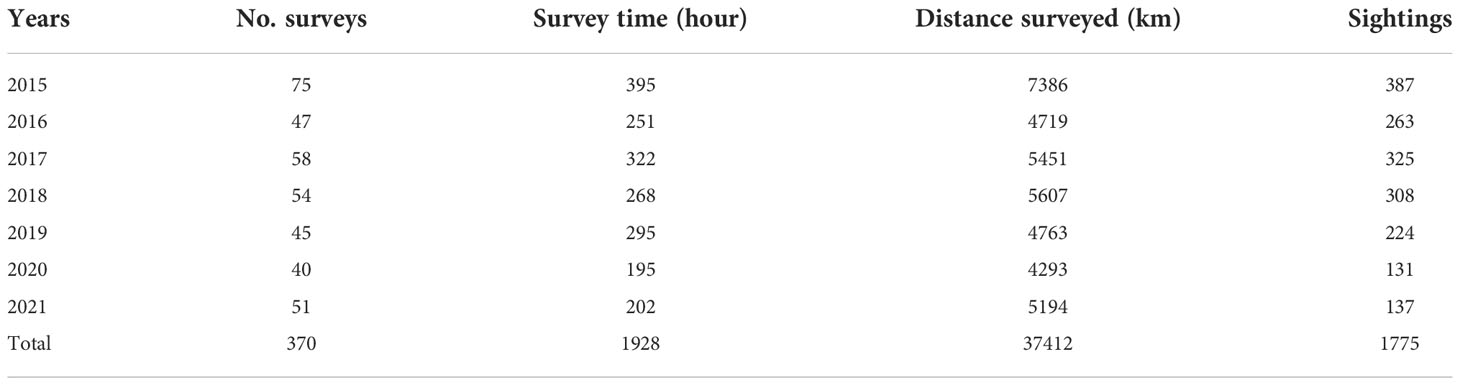
Table 1 Survey number, time, distance, and the sighting number of Indo-Pacific humpback dolphins recorded in the Pearl River Estuary from 2015 – 2021.
Habitat distribution and changes
During survey from 2015-2021, humpback dolphins were observed in 736 km2 of the 2882 km2 surveyed area. Most humpback dolphins were distributed with a distance of 5 km offshore with a depth of less than 20 m, and preferred to gather in the waters near the estuaries. The spatial distribution within the 3204 km2 area of humpback dolphins by kernel density estimation was well-defined, indicating that humpback dolphins were found in waters offshore with the core areas mainly clustered around near-shore islands in the PRE (Figure 2A). This study demonstrated that the core habitats of humpback dolphins were located in the surrounding waters on the northeast side of Qiao Island, the northwest side of Neilingling Island, west side of Lantau Island and surrounding Sanjiao Island in the eastern PRE (Figure 2B). In the middle region of the western PRE, the dolphins were mainly distributed in the water from the south side of Hengqin to the southeast side of Sanzao (Figure 2C). In the western PRE, the core areas of the humpback dolphins were near the waters to the south of Dajin Island to the north of Shangchuan Island (Figure 2D), the waters between the Shangchuan and Xiachuan Islands and the surrounding area of Mangzhou Island (Figure 2E). Surprisingly, more than 82.3% (2636 km2) of all humpback dolphin habitats were found beyond the existing reserves, and 39.7% (1273 km2) of the region showed hypoxic occurrences during the survey period in the PRE (Figure 2A).
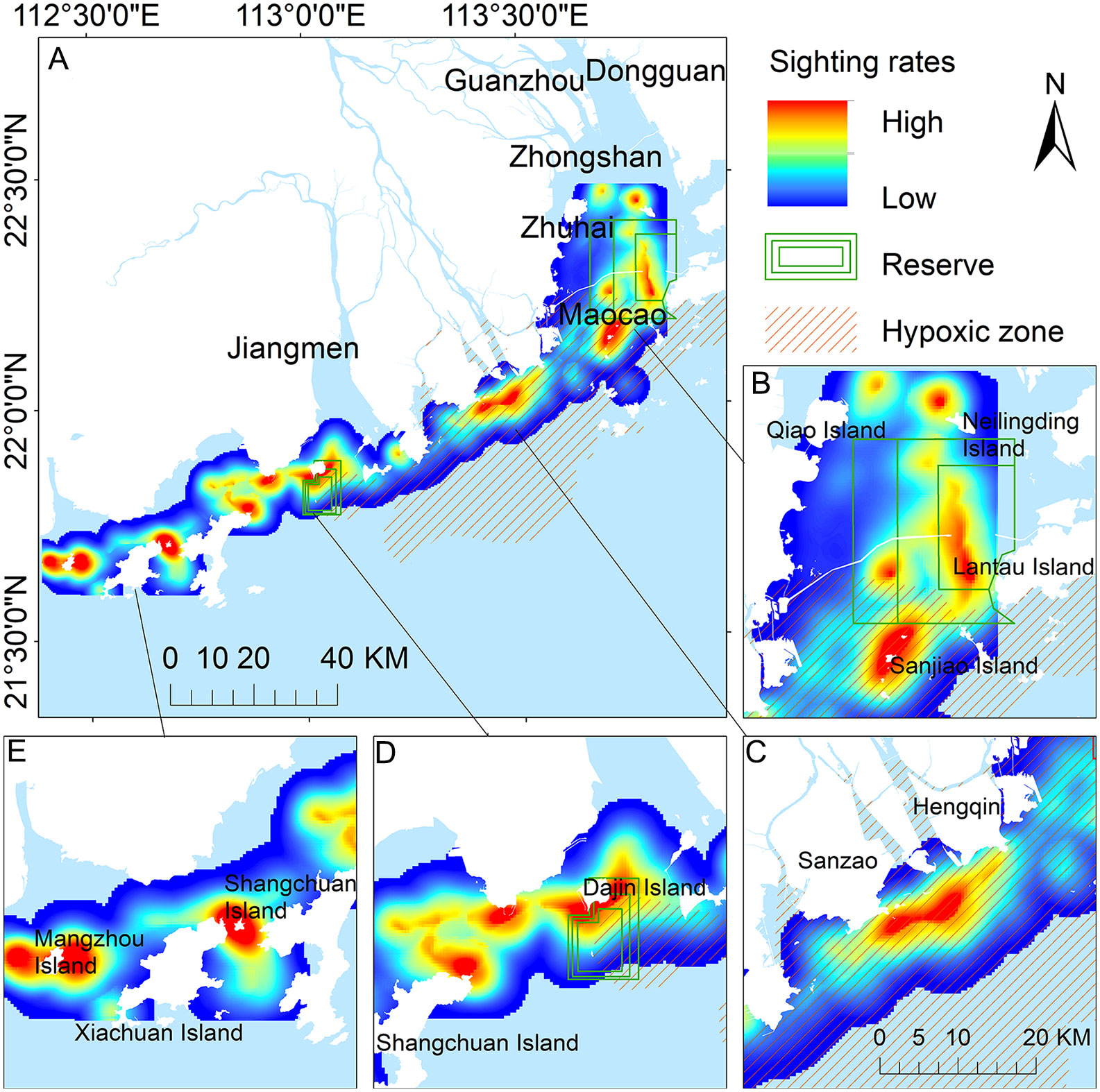
Figure 2 Spatial distribution patterns of sighting rates (sightings/surveys/km2) of Indo-Pacific humpback dolphin by kernel density analyses in the Pearl River Estuary (PRE) from 2015–2021. Current reserve area (green boxes); hypoxic zone (orange shaded area); (A) entire PRE; (B) eastern PRE; (C) middle PRE; (D) Dajin and Shangchuan Islands in western PRE; (E) Shangchuan, Xiachuan,and Mangzhou Islands in western PRE.
By calculating the Kendall’s tau correlation coefficients between the sighting rates and year of each cell over time, most areas of the PRE (73.2%; 2345 km2) showed a downward trend (Kendall’s tau < 0) in the sighting rates of humpback dolphins, and 200 km2 of them decreased significantly (p < 0.05) (Figure 3A). The main areas with decreased dolphin sighting rates were in the waters surrounding the southern side of Neilingling Island and Sanjiao Island in the eastern PRE (Figure 3B). A downward trend of dolphin sighting rates was also observed in the southern waters from Sanzao (Figure 3C) to Dajin Island (Figure 3D) and inshore waters of Shangchuan Island (Figure 3E). Only a few areas (24.1%, 771 km2) showed an increasing trend in the sightings rates of humpback dolphins, among which the humpback dolphin sighting rates over 34 km2 increased significantly (p < 0.05).
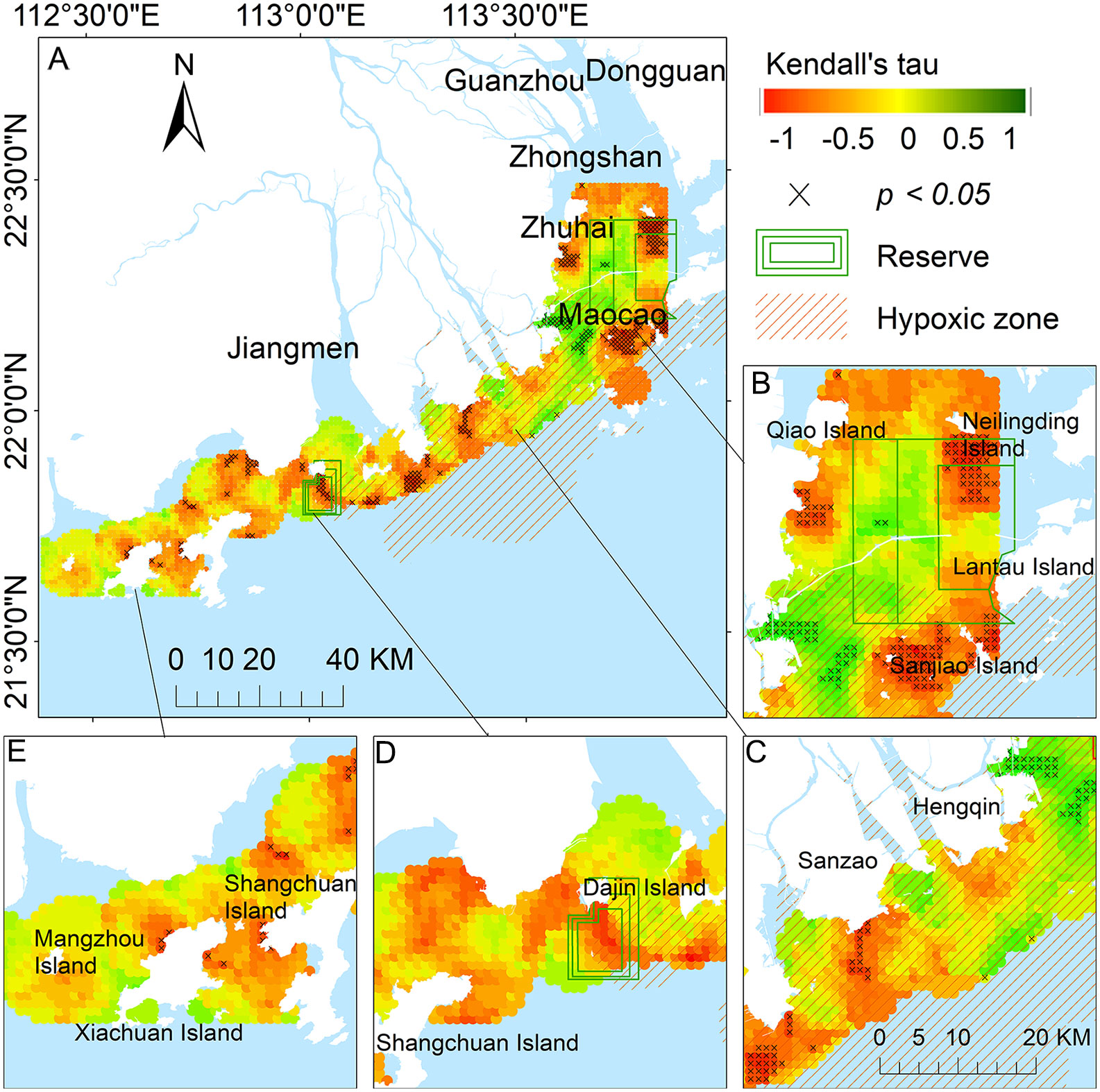
Figure 3 Spatial distribution pattern changes of sighting rates (sightings/surveys/km2) of Indo-Pacific humpback dolphins using Kendall’s tau correlation coefficients of sighting rates (increased: Kendall’stau>0; decreased: Kendall'stau<0; ×: p<0.05 significance level) with year for each cell in the Pearl River Estuary (PRE; 2015-2021). Current reserve area (green boxes); hypoxic zone (orange shaded area); (A) entire PRE; (B) eastern PRE; (C) middle PRE; (D) Dajin and Shangchuan Islands in western PRE; (E) Shangchuan, Xiachuan, and Mangzhou Islands in western PRE.
Environmental characteristics
By analyzing the Spearman's correlation coefficients between the dolphin sighting rates and environmental factors, dolphin sighting rates showed a significant positive correlation with DO, chlorophyll-a, As, Pb and Zn, while presenting a significant negative correlation with fecal coliform and suspended solids (Figure 4A), respectively. These seven water quality indices were further employed for factor analyses, and parallel analyses revealed that the number of factors as 1 (Pa1) showed a positive correlation (R2 = 0.86, p < 0.001) with dolphin sighting rates (Figure 4B).
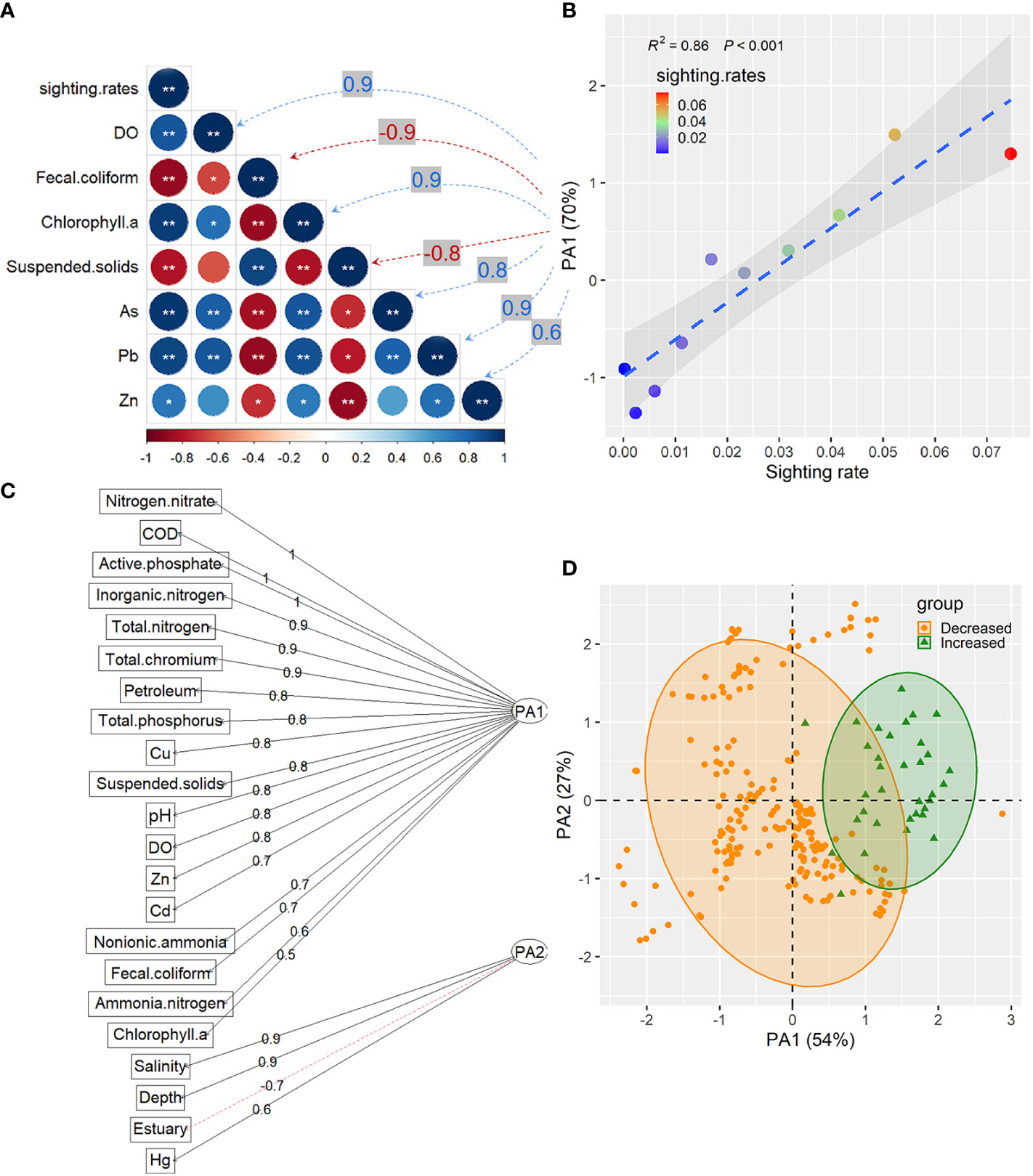
Figure 4 Factor analysis plot of water quality indicators in different Indo-Pacific Humpback dolphin habitats. (A) Correlation matrix between seven factors and dolphin sighting rates (sightings/surveys/km2); (B) Linear fitting of dolphin sighting rates with Pa1 obtained through parallel analyses; (C) Correlation of water quality indicators with significant differences between the declined dolphin sighting rates and increased habitat areas and two factors (Pa1 and Pa2) obtained through parallel analyses; (D) Factor analysis plot based on the scores of Pa1 and Pa2 from the evaluation of the declined dolphin sighting rates and increased areas.
Significant differences in the 22 environmental factors (Figure 4B) were found between areas with significantly increased dolphin sighting rates and areas with significantly decreased dolphin sighting rates (Mann-Whitney U-test, p < 0.05). Parallel analyses with these 22 environmental factors indicated that Pa1 was positively correlated with nitrogen nitrate, COD, active phosphate, and total nitrogen, mostly as nutrient parameters in water. Pa2 was positively correlated with salinity, depth, and Hg, and negatively correlated with estuary location (Figure 4C). Compared with the areas with decreased dolphin sighting rates, the areas with increased dolphin sighting rates were mainly located in areas with higher Pa1 (Figure 4D), indicating that the distribution areas with increased dolphin sighting rates might contain more abundant nutrients required by dolphins.
Regional variations
The regression analyses by linear fitting (r2 = 0.68, p < 0.05) of the sighting area rates (annual sighting area/annual survey area) over the survey years showed that the humpback dolphin habitat in the study area might have been declining at a rate of 2.83% (95% CI: 0.58% ~ 5.08%; p < 0.05) (Figure 5A). There were no significant (r2 = 0.01; p > 0.05) downward sighting area rates trends of humpback dolphins in the reserve, but the decline rates of humpback dolphins sighting area rates outside the reserve reached 3.94% (95% CI: 1.80% ~ 6.09%) (Figure 5A). In addition, the hypoxic zone might accelerate the decline of humpback dolphin occurrences, and the sighting area rates of humpback dolphins that declined in the hypoxic zone (3.16%, 95% CI: 0.67% – 5.65%, p < 0.05) was 0.46% faster than that in the non-hypoxic zone (2.70%; 95% CI: 0.49% – 4.91%, p < 0.05) (Figure 5A).
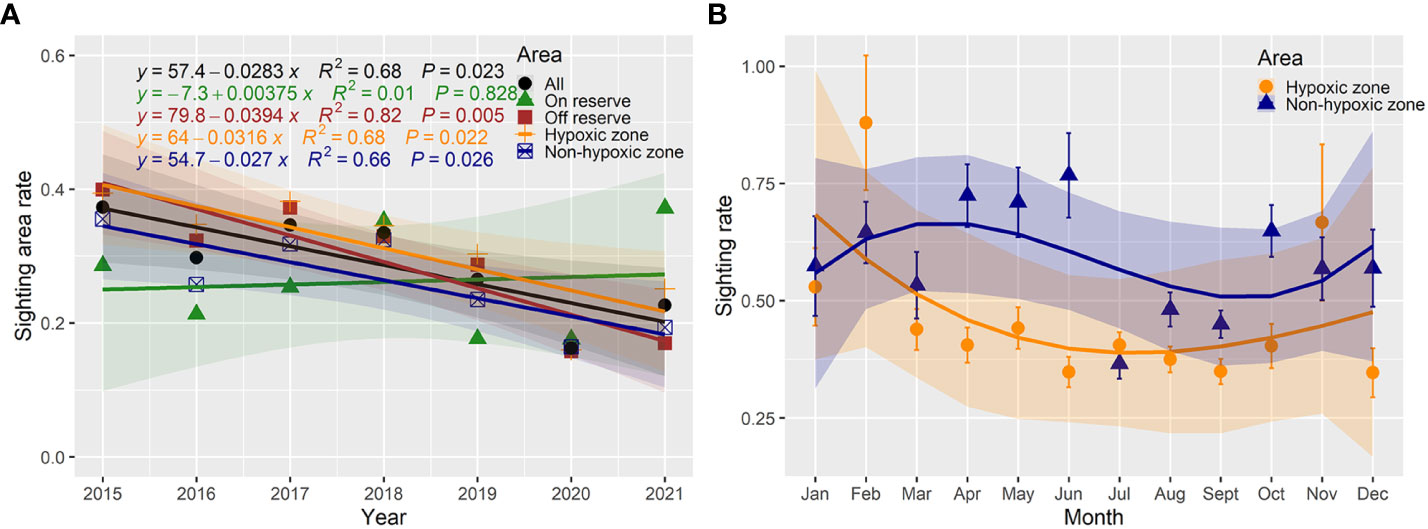
Figure 5 Variations of sighting area rates (annual sighting area/annual survey area) and sighting rates (sightings/surveys/km2) of Indo-Pacific humpback dolphins in the Pearl River Estuary. (A) Linear correlation fitting of sighting area rates with year in different areas from 2015 to 2021. (B) Variations of monthly mean dolphin sighting rates in the hypoxic and non-hypoxic zones.
In comparison, the sighting rates of dolphins in the hypoxic zone from April to December (excluding July and November) were significantly lower (Mann-Whitney U-test, p < 0.05) than those in the non-hypoxic zone (Figure 5B). The variation pattern of the monthly average sighting rates of dolphins throughout the year was not the same in different regions (two-sample Kolmogorov-Smirnov test, p < 0.01) (Figure 5B). The peak dolphin sighting rates in the non-hypoxic zone occurred from April to June, and the trough of low sighting rates occurred from July to September. In the hypoxic zone, dolphin sighting rates peaked in February and November, while continuous low sighting rates occurred from March to October (Figure 5B).
Discussion
Habitat distribution and environmental characteristics
The distribution of core habitats of wild cetaceans is fundamental and critical information for protection of target species' (Doak, 1995; Griffen and Drake, 2008). However, comprehensive distribution data of many cetacean populations are difficult to obtain, and large knowledge gaps remain in the study of the core habitats of the populations, which makes it difficult to determine optimum conservation strategy of wild cetacean populations (Peters et al., 2012; Wu et al., 2017b). This exploration of the habitat and changes in the largest known population of Indo-Pacific humpback dolphins in recent years likely appropriately complements the important gaps in current research. Our findings provide an important reference for researchers to develop effective humpback dolphin population monitoring strategies, and also help relevant agencies promptly adjust the spatial planning and management scheme of the protected area.
The identification of the core habitat of cetaceans is mainly based on the distribution density of animals, which are the most traditional and widely used methods for investigating the distribution of cetaceans because they can collect rich and valuable data, including photographs (Chan and Karczmarski, 2019; Guo et al., 2020; Tang et al., 2021), group size (Chen et al., 2010; Chan and Karczmarski, 2017), behaviors (Koper et al., 2016; Félix et al., 2022), environmental parameters (Wu et al., 2017a), and acoustic recording (Dong et al., 2021). These data are difficult to collect using other methods and are essential for studying of cetacean behaviors, habitat preferences, and population dynamics. Cetaceans are generally widely distributed, thus, surveys of cetaceans using boat-based survey methods might result in uneven study efforts in different investigation areas (Becker et al., 2022; Félix et al., 2022). Therefore, although field observation data from the visual survey is the most direct evidence that can represent the presence/habitation of cetaceans in a particular area, kernel density analyses using dolphin sighting rates obtained from boat-based surveys may directly affect the distribution of core habitats of the population. In this study, we used methods that could largely eliminate the effects of uneven efforts on the identification of dolphin core habitats using a seven years (2015-2021) boat-based field observation surveys.
The seven years boat-based surveys on humpback dolphins indicated that the distribution range of humpback dolphins in the PRE waters exceeded 3000 km2. Most of humpback dolphins frequented narrow water depths within 20 m and shallower depths within 5 km offshore for many years. Previous studies have revealed that the distribution preference of this species was within 10 km of the coast and within 30 m of the water depth (Karczmarski et al., 2015; Jefferson and Smith, 2016). However, our findings showed that the distribution of humpback dolphins in the PRE was narrower, and their suitable habitat range could be smaller than previously expected in the PRE. The distribution of cetaceans is likely related to the internal structure of the population (Nicholson et al., 2021), and many studies have shown that the humpback dolphins population in the PRE has spatial heterogeneity in their survival rate, age compositions, nutrient status and pollutant accumulation (Gui et al., 2017; Chan, 2019; Zhang et al., 2019; Guo et al., 2020). The multiple dispersed core habitat areas of the dolphin populations recorded based on the identification of the dolphin distribution density in this study, indicates that number and distribution of the dolphin community structures (Chan, 2019) requires further exploration.
Cetaceans typically live in areas with specific environmental characteristics, and previous studies have shown that ecological conditions, such as distance from the coast, water depth, chlorophyll-a concentration, salinity, and prey distribution and abundance might determine the spatial pattern of humpback dolphins (Wu et al., 2017a; Lin et al., 2021a). Our study further showed that the distribution of dolphins in the PRE was significantly associated with DO and heavy metals such as, As, Pb, and Zn. First, the results of dolphin distribution in relation to DO supports previous reports that many cetaceans living in tropical regions such as sperm and beaked whales, Globicephalinae and Delphininae (including the genera Sousa to which the PRE dolphin belongs), exhibited distribution characteristics associated with DO (Mannocci et al., 2015). Second, the correlation between the distribution of dolphins and As, Pb, and Zn could be due to the close relationship between the distribution characteristics of heavy metals and the activities of organisms in the area. In the aquatic environments, sediments are the most important reservoirs or sinks of heavy metals and other pollutants (Boudet et al., 2011; Rakib et al., 2022). Biological disturbances such as the foraging and swimming activities of benthic fishes and dolphins can induce disturbances in the sediment–water interface (Fang et al., 2019; Yang et al., 2022), and water with high activity of demersal fish and dolphins might contain higher levels of heavy metals. Zn is an essential element for the growth of organisms, and can participate in the material energy cycle of organisms, whereas Pb and As are not essential elements of organisms, but they affect the seawater environment and the life activities of organisms, along the food chain and food network enriched into higher trophic levels (Gui et al., 2017; Fang et al., 2019). With the excretion and death of organisms, these debris are absorbed and degraded by microorganisms, and the heavy metal elements in them re-enter the aquatic environment. Therefore, part of heavy metals source in the seawater with high dolphin sightings may be the dolphins’ own excrement and other biological sources. In addition to heavy metals, N and P, as a nutrient salts, are positively correlated with chlorophyll-a, and the N and P contents in water can be used to indicate the distribution of nutrients and biological resources in water (Al-Akhaly et al., 2020). Factors such as N, P, and heavy metals in the water of dolphin habitats where dolphin sighting rates decreased differed from those of dolphins with increased sighting rates, indicating that adequate nutrition and rich biological resources are indispensable for the preferred habitats of dolphins.
High concentrations of heavy metals, and eutrophication of water bodies are likely to pose a threat to dolphins. The hepatic levels of As in humpback dolphins are among the highest reported for cetaceans globally, and the levels of Zn are high enough to cause toxicological effects in some animals (Gui et al., 2017). Eutrophication of coastal waters can introduce pathogenic agents that cause epidermal lesions in dolphins (Chan and Karczmarski, 2019). However, the individual migration of dolphins is very limited (Hung and Jefferson, 2004), making it difficult for them to search for other suitable habitats. It seems that dolphins in the PRE could not avoid the threat of water pollution, which remains an important factor threatening the survival of this population.
A growing number of less manpower-intensive and cost-effective methods are currently being applied to cetacean research and conservation programs, including passive acoustic monitoring and multidimensional habitat modeling tools (Marini et al., 2015; Fang et al., 2020; Becker et al., 2022; Liu et al., 2022). Passive acoustics monitoring can acquire continuous data at large spatial and temporal scales, regardless of harsh weather and visual conditions; however, the arrangement of these instruments often requires visual observation data as a basis (Fang et al., 2020). The findings of this study on the distribution and variation of humpback dolphin core habitats provides a reliable reference for deployment sites monitored by these passive acoustic instruments, which can make the design of the experimental protocol more rational and efficient for relevant researchers to obtain the required data. The exploration of the environmental characteristics of humpback dolphin habitats can provide more accurate and abundant parameters for habitat modeling and improve the accuracy of model identification of potential habitats for populations (Chen et al., 2020; Lin et al., 2021a).
Habitat decline and regional variations
Reducing the population size would lead to shrinkage in the habitat of dolphin (Boyce et al., 2016). The calculation of the annual decline rate (2.83%) of dolphin habitats in this study is similar to the previously estimated annual decline rate (2.46%–3.17%) of the population (Huang et al., 2012; Chan, 2019), indicating the reliability of our research method, and further confirming the feasibility of using habitat changes to estimate population changes (Boyce et al., 2016). However, sighting rates are not a great way to measure changes in dolphin abundance or density, as they are affected by other factors (e.g., weather, sighting conditions, and observer efficiency). A much better way to examine such issues is to use systematic sighting surveys and distance sampling methods, that are explicitly designed to evaluate density and abundance. Therefore, the habitat changes of PRE dolphins shown in this study provide a reference for population distribution changes, and cannot directly indicate changes in population size.
By estimating habitat changes within the existing reserves, we confirmed that existing protected areas play a role in inshore cetacean habitat conservation. Strict management and restriction of human activities in reserves, such as restrictions on fishing activities, aquaculture, and speed limits for transport vessels, provide some help for dolphins to have sufficient living space and food resources in this estuarine area (Ross et al., 2011). However, we also need to point out that existing reserves were poorly planned. Many dolphin core habitats are not effectively protected, especially in the middle and western seas with a high density of dolphins, but the dolphin sighting rates in these areas have decreased significantly in recent years. In addition, the extent of the reserve in the eastern PRE does not protect dolphin habitats in the northern and southern PRE. This lack of conservation may lead to the gradual loss of local habitats, fragmentation of the original continuous habitat, and degenerated integrity of the habitat including size, quality, structure, and connectivity, which are not conducive to the long-term development of the population (Wiegand et al., 2005). Therefore, we strongly recommend adjusting the scope of the eastern reserve and increasing protected areas in the middle and western parts of PRE. The protection of dolphin habitats in this area cannot be delayed.
In recent decades, the decline in DO concentrations in most of the bay, estuary, and adjacent sea areas has been frequently observed, which is to some extent affected by human activities in coastal areas, resulting in an increased nutrient discharge into the sea. Excess nutrient input aggravates the degree of eutrophication in estuaries and offshore waters, coupled with global warming, and leads to an increase in the occurrence and intensity of hypoxic conditions (Breitburg et al., 2018). Since 1950, more than 500 coastal systems of hypoxia have been identified, with a total area of more than 245,000 km2 (Diaz and Rosenberg, 2008; Breitburg et al., 2018), including the Gulf of Mexico (Del Giudice et al., 2019), Chesapeake Bay (Da et al., 2018), Lower St. Lawrence Estuary (Lefort et al., 2012), and the Yangtze River Estuary (Tian et al., 2021). The lack of oxygen in the sea area has gradually developed into a major global ecological and environmental problem. This study suggests that the emergence of hypoxic zones in the PRE might have a negative impact on dolphin habitats, as dolphin habitats in the hypoxic zone declined 0.46% faster than those in the non-hypoxic zone. Hypoxia often occurs in the PRE in the summer (April to September) (Cui et al., 2019; Li et al., 2020b), and the persistent low sighting rates of dolphins in the hypoxic zone from March to October indicated that the negative impact of hypoxia was continuous, starting from the month before the onset of hypoxia. The low sighting rates of dolphins in the hypoxic zone continued until one month after the end of the hypoxia. In July, August and September, the seasonally low sighting rates of dolphins across the study area (hypoxic and non-hypoxic zones) were likely be associated with the abundance and range of the summer dolphin prey (Chen et al., 2010). Because of the annual ban on fishing in the PRE from May to August, the fish resources during this period are relatively abundant. Abundant fish resources might increase the distribution range of dolphins, thus reducing their sighting rates (Wang et al., 2021).
Cetaceans, as air-breathing species, are not directly affected by hypoxia. However, the occurrence of hypoxia usually directly affects the survival of demersal fish, which indirectly influences dolphins whose main food source is fish (Craig et al., 2001). Recent studies suggest a dietary shift in the PRE humpback dolphins, from primarily demersal to greater intake of neritic and pelagic fish (Lin et al., 2021b). It is reasonable to speculate that hypoxia could be one of the reasons for the shift in the feeding habits of dolphins. Dietary changes reduces the nutritional level of dolphins, which is not conducive to their long-term survival (Zhang et al., 2019). In the middle of the PRE, which was mainly covered by the hypoxic zone, the dolphins tended to migrate to both sides, which further indicated the negative effects of the hypoxic zone (Chan, 2019; Guo et al., 2020). The impact analyses of hypoxic zones on inshore cetacean habitats in this study might provide additional evidence and an approach basis for a better assessment of environmental threats to wild cetacean populations. This study strongly suggests that the potential damage and risk of hypoxia in cetacean habitats should be investigated further to promote the conservation of wild cetacean habitats.
There is no doubt that there are many other causes of habitat decline in offshore cetacean populations, such as land reclamation, alteration of coastlines, coastal fisheries, noise and disturbance due to marine construction, which have not been included in our analysis (Karczmarski et al., 2015; Jefferson and Smith, 2016). Further studies are recommended to evaluate and quantify the impact of different environmental factors on population habitat decline, to better protect the population habitat and avoid its decline.
Conclusion
Using a generalized and highly applicable approach, this study explored the habitat changes of the world’s largest known population of Sousa chinensis in the poorly protected PRE region interspaced with the hypoxic zone in the past seven years. These findings indicated that the distribution of PRE dolphins was closely related to estuarine nutrients and biological resources, and the habitat changes of humpback dolphins were consistent with the population decline in the PRE. Although the existing reserves have effectively reduced certain habitat area declines, the activity areas in the middle and western PRE of the population beyond the current reserve, lacks efficient protection. A large gap still exists in the understanding of the threat of the hypoxic zone to the protection of this population habitat. Further studies on population dynamics and thier influencing factors are warranted.
Data availability statement
The original contributions presented in the study are included in the article/supplementary material. Further inquiries can be directed to the corresponding author.
Ethics statement
The animal study was reviewed and approved by Sun Yat-Sen University.
Author contributions
LG: Data curation and Investigation, Funding acquisition, Conceptualization, Methodology, Software and Visualization, and Writing – original draf. DL: Data curation and Investigation, Software and Visualization, Writing – review & editing; R-QY: Investigation, Writing – review & editing; CZ, NH and HW: Data curation and Investigation; YW: Project administration, Supervision and Funding acquisition. All authors contributed to the article and approved the submitted version.
Funding
This work was supported by the National Key Research and Development Program of China (2022YFF1301603), National Natural Science Foundation of China (32201290), the 71st batch of China Postdoctoral Science Funding (2022M713560), and Innovation Group Project of Southern Marine Science and Engineering Guangdong Laboratory (Zhuhai) (311021004 and 311020003).
Acknowledgments
We greatfully acknowledge Dr. Wenzhi Lin for his help in the field investigation, and thank each members of the marine cetacean and environmental protection research team of Sun Yat-Sen University, the Guangdong Pearl River Estuary Chinese White Dolphin Reserve and Guangdong Jiangmen Chinese White Dolphin Provincial Nature Reserve for their help in promoting this study.
Conflict of interest
The authors declare that the research was conducted in the absence of any commercial or financial relationships that could be construed as a potential conflict of interest.
Publisher’s note
All claims expressed in this article are solely those of the authors and do not necessarily represent those of their affiliated organizations, or those of the publisher, the editors and the reviewers. Any product that may be evaluated in this article, or claim that may be made by its manufacturer, is not guaranteed or endorsed by the publisher.
References
Al-Akhaly I. A., Al-Shwafi N. A., Al-Kabsh S. A. (2020). Distribution of nutrient salts and chlorophyll-a in surface water along the gulf of Aden and Arabian Sea coast, Yemen. Sultan Qaboos Univ. J. Sci. [SQUJS] 25, 17–25. doi: 10.24200/squjs.vol25iss1pp17-25
Bailey H., Thompson P. M. (2009). Using marine mammal habitat modelling to identify priority conservation zones within a marine protected area. Mar. Ecol. Prog. Ser. 378, 279–287. doi: 10.3354/meps07887
Bearzi G., Holcer D., Notarbartolo di Sciara G. (2004). The role of historical dolphin takes and habitat degradation in shaping the present status of northern Adriatic cetaceans. Aquat. Conservation: Mar. Freshw. Ecosyst. 14, 363–379. doi: 10.1002/aqc.626
Becker E. A., Forney K. A., Miller D. L., Barlow J., Rojas-Bracho L., Urbán R. J., et al. (2022). Dynamic habitat models reflect interannual movement of cetaceans within the California current ecosystem. Front. Mar. Sci. 9, 829523. doi: 10.3389/fmars.2022.829523
Boudet L. C., Escalante A., Haeften G. V., Moreno V., Gerpe M. (2011). Assessment of heavy metal accumulation in two aquatic macrophytes: A field study. J. Braz. Soc. Ecotoxicol. 5 (2), 1–13. doi: 10.5132/jbse.2010.02.012
Boyce M. S., Johnson C. J., Merrill E. H., Nielsen S. E., Solberg E. J., Moorter B. V.. (2016). REVIEW: Can habitat selection predict abundance? J. Anim. Ecol. 85, 11–20. doi: 10.1111/1365-2656.12359
Breitburg D., Levin L. A., Oschlies A., Gregoire M., Chavez F. P., Conley D. J., et al. (2018). Declining oxygen in the global ocean and coastal waters. Science 359 (6371): eaam7240. doi: 10.1126/science.aam7240
Chan S. C. Y. (2019). Demography and socio-ecology of indo-pacific humpback dolphin (Sousa chinensis) metapopulation in the pearl river estuary Ph.D. Thesis (China: University of Hong Kong, Pokfulam, Hong Kong SAR, China).
Chan S. C. Y., Karczmarski L. (2017). Indo-pacific humpback dolphins (Sousa chinensis) in Hong Kong: Modelling demographic parameters with mark-recapture techniques. PloS One 12, e0174029. doi: 10.1371/journal.pone.0174029
Chan S. C. Y., Karczmarski L. (2019). Epidermal lesions and injuries of coastal dolphins as indicators of ecological health. EcoHealth 16, 576–582. doi: 10.1007/s10393-019-01428-0
Chen B., Hong Z., Hao X., Gao H., et al. (2020). Environmental models for predicting habitat of the indo-pacific humpback dolphins in fujian, China. Aquat. Conservation: Mar. Freshw. Ecosyst. 30, 787–793. doi: 10.1002/aqc.3279
Chen T., Hung Samuel K., Qiu Y., Jia X, Jefferson TA.. (2010). Distribution, abundance, and individual movements of indo-pacific humpback dolphins (Sousa chinensis) in the pearl river estuary, China. Mammalia 74, 117. doi: 10.1515/mamm.2010.024
Conley D. J., Carstensen J., Vaquer-Sunyer R., Duarte C.M.. (2010). Ecosystem thresholds with hypoxia. Hydrobiologia 629, 21–29. doi: 10.1007/s10750-009-9764-2
Craig J. K., Crowder L. B., Gray C. D., McDaniel C. J, Henwood T. A, Hanifen J. G. (2001). Ecological Effects of Hypoxia on Fish, Sea Turtles, andMarine Mammals in the Northwestern Gulf of Mexico. Coastal hypoxia: Consequences for living resources and ecosystems. 58, 269–291. doi: 10.1029/CE058p0269
Cui Y., Wu J., Ren J., Xu J. (2019). Physical dynamics structures and oxygen budget of summer hypoxia in the pearl river estuary. Limnology Oceanography 64, 131–148. doi: 10.1002/lno.11025
Da F., Friedrichs M. A. M., St-Laurent P. (2018). Impacts of atmospheric nitrogen deposition and coastal nitrogen fluxes on oxygen concentrations in Chesapeake bay. J. Geophysical Research: Oceans 123 (7): 5004-5025. doi: 10.1029/2018JC014009.
Del Giudice D., Matli V., Obenour D. (2019). Bayesian Mechanistic modeling characterizes gulf of Mexico hypoxia: 1968–2016 and future scenarios. Ecol. Appl. 30, e02032. doi: 10.1002/eap.2032
Diaz R. J., Rosenberg R. (2008). Spreading dead zones and consequences for marine ecosystems. Science 321, 926–929. doi: 10.1126/science.1156401
Doak D. F. (1995). Source-sink models and the problem of habitat degradation: General models and applications to the Yellowstone grizzly. Conserv. Biol. 9, 1370–1379. doi: 10.1046/j.1523-1739.1995.09061370.x
Dong L., Dong J., Caruso F., Zhao L., Li S.. (2021). Temporal variation of the underwater soundscape in jiaotou bay, an indo-pacific humpback dolphin (Sousa chinensis) habitat off hainan island, China. Integr. zoology 0, 1–22. doi: 10.1111/1749-4877.12530
Fahrig L. (2003). Effects of habitat fragmentation on biodiversity. Annu. Rev. Ecology Evolution Systematics 34, 487–515. doi: 10.1146/annurev.ecolsys.34.011802.132419
Fahrig L., Triantis K. (2013). Rethinking patch size and isolation effects: the habitat amount hypothesis. J. Biogeography 40, 1649–1663. doi: 10.1111/jbi.12130
Fang L., Lin W., Guo L., Cai H., Pine M. K., Wu Y. (2020). Monitoring indo-pacific humpback dolphin occurrences in a highly urbanized estuary for informing conservation and management. Aquat. Conservation: Mar. Freshw. Ecosyst. 31, 685–695. doi: 10.1002/aqc.3475
Fang T., Lu W., Cui K., Li J., Yang K., Zhao X. (2019). Distribution, bioaccumulation and trophic transfer of trace metals in the food web of chaohu lake, anhui, China. Chemosphere 218, 1122–1130. doi: 10.1016/j.chemosphere.2018.10.107
Félix F., Fernández J. E., Paladines A., Centeno R., Romero J., Burneo SF. (2022). Habitat use of the common bottlenose dolphin (Tursiops truncatus) in the gulf of guayaquil, Ecuador: Management needs for a threatened population. Ocean Coast. Manage. 223, 106174. doi: 10.1016/j.ocecoaman.2022.106174
Griffen B. D., Drake J. M. (2008). Effects of habitat quality and size on extinction in experimental populations. Proc. Biol. Sci. 275, 2251–2256. doi: 10.1098/rspb.2008.0518
Gui D., Yu R. Q., Karczmarski L., Ding Y., Zhang H., Sun Y. (2017). Spatiotemporal trends of heavy metals in indo-pacific humpback dolphins (Sousa chinensis) from the Western pearl river estuary, China. Environ. Sci. Technol. 51, 1848–1858. doi: 10.1021/acs.est.6b05566
Guo L., Lin W., Zeng C., Luo D., Wu Y. (2020). Investigating the age composition of indo-pacific humpback dolphins in the pearl river estuary based on their pigmentation pattern. Mar. Biol. 167 (4), 1–12. doi: 10.1007/s00227-020-3650-x.
Halpern B. S., Frazier M., Potapenko J., Casey KS., Koenig K., Longo C., et al. (2015). Spatial and temporal changes in cumulative human impacts on the world's ocean. Nat. Commun. 6, 7615. doi: 10.1038/ncomms8615
Huang S., Karczmarski L., Chen J., Zhou R., Lin W., Zhang H., et al. (2012). Demography and population trends of the largest population of indo-pacific humpback dolphins. Biol. Conserv. 147, 234–242. doi: 10.1016/j.biocon.2012.01.004
Hung S. K., Jefferson T. A. (2004). Ranging patterns of indo-pacific humpback dolphins (Sousa chinensis) in the pearl river estuary, people's republic of China. Aquat. Mammals 30, 159–174. doi: 10.1578/AM.30.1.2004.159
Jefferson T. A., Hung S. K., Robertson K. M., Archer F. I. (2012). Life history of the indo-pacific humpback dolphin in the pearl river estuary, southern China. Mar. Mammal Sci. 28, 84–104. doi: 10.1111/j.1748-7692.2010.00462.x
Jefferson T. A., Smith B. D. (2016). Re-assessment of the conservation status of the indo-pacific humpback dolphin (Sousa chinensis) using the IUCN red list criteria. Adv. Mar. Biol. 73, 1–26. doi: 10.1016/bs.amb.2015.04.002
Karczmarski L., Huang S.-L., Or C. K. M., Gui D., Chan S. C. Y., Lin W., et al. (2015). Humpback dolphins in Hong Kong and the pearl river delta: status, threats and conservation challenges. Adv. Mar. Biol. 73, 27–64. doi: 10.1016/bs.amb.2015.09.003
Koper R. P., Karczmarski L., Preez D., Plön S. (2016). Sixteen years later: Occurrence, group size, and habitat use of humpback dolphins (Sousa plumbea) in algoa bay, south Africa. Mar. Mammal Sci. 32, 490–507. doi: 10.1111/mms.12279
Lefort S., Gratton Y., Mucci A., Dadou I., Gilbert D. (2012). Hypoxia in the lower st. Lawrence estuary: How physics controls spatial patterns. J. Geophys. Res. 117, C07018. doi: 10.1029/2011JC007751.
Levin L. A., Ekau W., Gooday A. J., Jorissen F, Middelburg J. J., Naqvi S. W. A.. (2009). Effects of natural and human-induced hypoxia on coastal benthos. Biogeosciences 6, 2063–2098. doi: 10.5194/bg-6-2063-2009
Li D., Gan J., Hui R., Liu Z., Yu L., Lu Z., et al. (2020a). Vortex and biogeochemical dynamics for the hypoxia formation within the coastal transition zone off the pearl river estuary. J. Geophysical Research: Oceans 125. doi: 10.1016/j.marpolbul.2019.110814.
Li X., Lu C., Zhang Y., Zhao H., Wang J., Liu H., et al. (2020b). Low dissolved oxygen in the pearl river estuary in summer: Long-term spatio-temporal patterns, trends, and regulating factors. Mar. pollut. Bull. 151, 110814. doi: 10.1016/j.marpolbul.2019.110814.
Lin W., Karczmarski L., Zhou R., Mo Y., Guo L., Yiu S. K. F., et al. (2021b). Prey decline leads to diet shift in the largest population of indo-pacific humpback dolphins? Integr. zoology 16, 548–574. doi: 10.1111/1749-4877.12548
Lin M., Liu M., Lek S., et al. (2021a). Modelling habitat suitability of the indo-pacific humpback dolphin using artificial neural network: The influence of shipping. Ecol. Inf. 62, 101274. doi: 10.1016/j.ecoinf.2021.101274.
Liu G., He W., Cai S. (2020). Seasonal variation of dissolved oxygen in the southeast of the pearl river estuary. Water 12, 2475. doi: 10.3390/w12092475
Liu M., Lin M., Dong L., Caruso F., Li S. (2022). An integrated strategy for monitoring cetaceans in data-poor regions. Biol. Conserv. 272, 109648. doi: 10.1016/j.biocon.2022.109648
Li M., Wang X., Hung S. K., Xu Y., Chen T. (2019). Indo-pacific humpback dolphins (Sousa chinensis) in the moyang river estuary: The western part of the world's largest population of humpback dolphins. Aquat. Conservation: Mar. Freshw. Ecosyst. 2019, 1–11. doi: 10.1002/aqc.3055
Mannocci L., Monestiez P., Spitz J., Ridoux V. (2015). Extrapolating cetacean densities beyond surveyed regions: habitat-based predictions in the circumtropical belt. J. Biogeography 42, 1267–1280. doi: 10.1111/jbi.12530
Marini C., Fossa F., Paoli C., Bellingerib M., Gnoneb G., Vassallo P., et al. (2015). Predicting bottlenose dolphin distribution along liguria coast (northwestern Mediterranean Sea) through different modeling techniques and indirect predictors. J. Environ. Manage. 150, 9–20. doi: 10.1016/j.jenvman.2014.11.008
Nicholson K., Loneragan N., Finn H., Bejder L. (2021). Social, spatial and isotopic niche partitioning identify an estuarine community of bottlenose dolphins as a discrete management unit. Aquat. Conservation: Mar. Freshw. Ecosyst. 31, 3526–3542. doi: 10.1002/aqc.3736
Peters K. J., Parra G. J., Skuza P. P., Möller L. (2012). First insights into the effects of swim-with-dolphin tourism on the behavior, response, and group structure of southern Australian bottlenose dolphins. Mar. Mammal Sci. 29, E484–E497. doi: 10.1111/mms.12003
Rabalais N. N., Díaz R. J., Levin L. A., Turner RE., Gilbert D., Zhang J. (2010). Dynamics and distribution of natural and human-caused hypoxia. Biogeosciences 7, 585–619. doi: 10.5194/bg-7-585-2010
Rakib M. R. J., Rahman M. A., Onyena A. P., Kumar R., Sarker A., Hossain MB., et al. (2022). A comprehensive review of heavy metal pollution in the coastal areas of Bangladesh: abundance, bioaccumulation, health implications, and challenges. Environ. Sci. pollut. Res. Int 29, 67532–67558. doi: 10.1007/s11356-022-22122-9
Roman M. R., Brandt S. B., Houde E. D., Pierson J. J., et al. (2019). Interactive effects of hypoxia and temperature on coastal pelagic zooplankton and fish. Front. Mar. Sci. 6. doi: 10.3389/fmars.2019.00139
Ross P. S., Barlow J., Jefferson T. A., Hickie BE., Lee T., MacFarquhar C., et al. (2011). Ten guiding principles for the delineation of priority habitat for endangered small cetaceans. Mar. Policy 35, 483–488. doi: 10.1016/j.marpol.2010.11.004
Stockin K. A., Lusseau D., Binedell V., Wiseman N., Orams M. B., et al. (2008). Tourism affects the behavioural budget of the common dolphin delphinus sp. in the hauraki gulf, new Zealand. Mar. Ecol. Prog. Ser. 355, 287–295. doi: 10.3354/meps07386
Sun X., Guo L., Luo D., Yu R.-Q., Yu X., Liang Y., et al. (2022). Long-term increase in mortality of indo-pacific humpback dolphins (Sousa chinensis) in the pearl river estuary following anthropic activities: Evidence from the stranded dolphin mortality analysis from 2003 to 2017. Environ. pollut. 307, 119526. doi: 10.1016/j.envpol.2022.119526
Tang X., Lin W., Karczmarski L., Lin M., Chan S. C. Y., Liu M., et al. (2021). Photo-identification comparison of four indo-pacific humpback dolphin populations off southeast China. Integr. zoology 0, 1–8. doi: 10.1111/1749-4877.12537
Thompson P. M., Wilson B., Grellier K. (2000). Combining power analysis and population viability analysis to compare traditional and precautionary approaches to conservation of coastal cetaceans. Conserv. Biol. 14, 1253–1263. doi: 10.1046/j.1523-1739.2000.00099-410.x
Tian D., Zhou F., Zhang W., Ma X., Gou X., et al. (2021). Effects of dissolved oxygen and nutrients from the kuroshio on hypoxia off the changjiang river estuary. J. Oceanology Limnology. 40(2): 515–529. doi: 10.1007/s00343-021-0440-3
Wang C. C., Xu Y., Li N., Peng C., Wu H., Huang S.-L., et al. (2021). Seasonal distribution of the indo-pacific humpback dolphins: Implications for coastal habitat management. Aquat. Conservation: Mar. Freshw. Ecosyst. 31, 696–707. doi: 10.1002/aqc.3479
Wiegand T., Revilla E., Moloney K. A. (2005). Effects of habitat loss and fragmentation on population dynamics. Conserv. Biol. 19, 108–121. doi: 10.1111/j.1523-1739.2005.00208.x
Wu H., Jefferson T. A., Peng C., Liao Y., Huang H., Lin M., et al. (2017a). Distribution and habitat characteristics of the indo-pacific humpback dolphin (Sousa chinensis)in the northern beibu gulf, China. Aquat. Mammals 43, 219–228. doi: 10.1578/AM.43.2.2017.219
Wu H., Xu Y., Peng C., Liao Y., Wang X., Jefferson T. A., et al. (2017b). Long-term habitat loss in a lightly-disturbed population of the indo-pacific humpback dolphin, Sousa chinensis. Aquat. Conserv. Mar. Freshw. Ecosyst. 27, 1–11. doi: 10.1002/aqc.2778
Yang F., Zhang H., Xie S., Wei C., Yang X.. (2022). Concentrations of heavy metals in water, sediments and aquatic organisms from a closed realgar mine. Environ. Sci. pollut. Res. Int. doi: 10.1007/s11356-022-22563-2
Keywords: habitat decline, Indo-Pacific humpback dolphins, Pearl River Estuary, reserve, hypoxic zone
Citation: Guo L, Luo D, Yu R-Q, Zeng C, Huang N, Wang H and Wu Y (2022) Habitat decline of the largest known Indo-Pacific humpback dolphin (Sousa chinensis) population in poorly protected areas associated with the hypoxic zone. Front. Mar. Sci. 9:1048959. doi: 10.3389/fmars.2022.1048959
Received: 20 September 2022; Accepted: 31 October 2022;
Published: 24 November 2022.
Edited by:
SM Sharifuzzaman, University of Chittagong, BangladeshReviewed by:
Thomas A. Jefferson, Clymene Enterprises, United StatesSalvatore Siciliano, Escola Nacional de Saúde Pública Sergio Arouca, Fundação Oswaldo Cruz (Fiocruz), Brazil
Copyright © 2022 Guo, Luo, Yu, Zeng, Huang, Wang and Wu. This is an open-access article distributed under the terms of the Creative Commons Attribution License (CC BY). The use, distribution or reproduction in other forums is permitted, provided the original author(s) and the copyright owner(s) are credited and that the original publication in this journal is cited, in accordance with accepted academic practice. No use, distribution or reproduction is permitted which does not comply with these terms.
*Correspondence: Yuping Wu, exwyp@mail.sysu.edu.cn