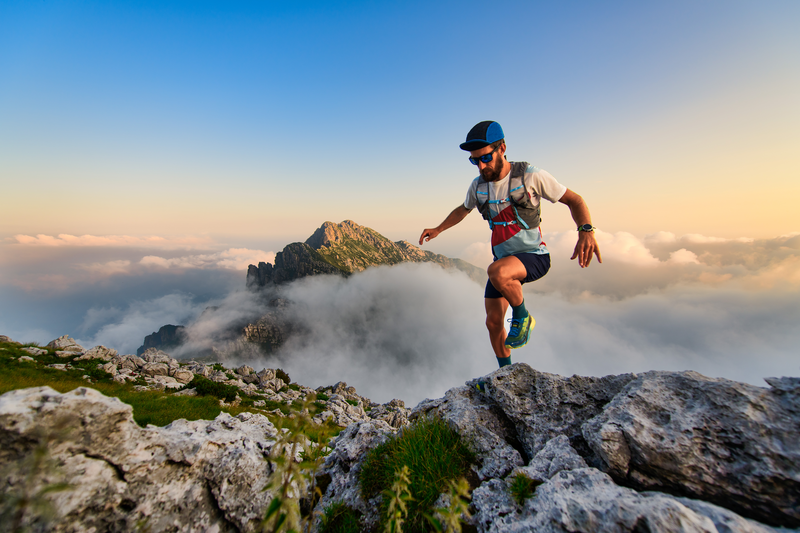
95% of researchers rate our articles as excellent or good
Learn more about the work of our research integrity team to safeguard the quality of each article we publish.
Find out more
ORIGINAL RESEARCH article
Front. Mar. Sci. , 14 October 2022
Sec. Marine Fisheries, Aquaculture and Living Resources
Volume 9 - 2022 | https://doi.org/10.3389/fmars.2022.1044990
Vibrio parahaemolyticus is a common pathogen of marine crustaceans and is responsible for large losses in aquaculture. Salinity is an important environmental factor, and abrupt changes in salinity can affect the immunity of crustaceans. In this study, we carried out a transcriptomic analysis under pathogenic infection and low salt stress, and conducted a comparative analysis of the differentially expressed genes (DEGs) after stimulation by the two factors. Illumina sequencing technology was used for the transcriptome sequencing of 27 hepatopancreas samples, and 178.77 G sequencing data and an average of 44,142,119 clean reads per sample were obtained. A total of 3,047 and 3,710 DEGs were found after V. parahaemolyticus infection and low salt stress, which included a number of innate immunity genes, such as Toll-like receptor (TLR), anti-lipopolysaccharide factor (ALF), lectin, and hemocyanin. In addition, 2,016 common DEGs were found, accounting for 42.52% of the total DEGs. Among these, 169 DEGs were up-regulated after pathogenic infection and down-regulated after low salt stress, and were mainly enriched in starch and sucrose metabolism, nitrogen metabolism, amino sugar and nucleotide sugar processes, and other pathways. Collectively, these results provide data support for the analysis of the immune mechanism of crabs against V. parahaemolyticus and will also help to clarify the molecular mechanism by which salinity affects immunity.
The swimming crab (Portunus trituberculatus, Crustacea: Decapoda: Brachyura) is widely distributed in the coastal waters of China, Korea, Japan, and Southeast Asia (Lv et al., 2017). Because of its rapid growth and high nutritional value, it has become an important crustacean species in mariculture in China. In 2019, the domestic production of swimming crab reached 113,810 tons (China Fishery Statistics Yearbook, 2020), and it is therefore of great economic value.
High-throughput sequencing together with gene expression analysis has become a powerful tool to identify the essential genes controlling a particular biological process. Recently, molecular mechanisms such as immune defense (Liu et al., 2020; Kong et al., 2020), stress response (Lou et al., 2019; Lee et al., 2022), growth and development (Fang et al., 2021), and sex determination (Lin et al., 2019; Xu et al., 2021) have been resolved by transcriptome sequencing in aquatic crustaceans. The hepatopancreas in crabs is an important immune organ (Xu et al., 2020a). Innate immunity is an important way for crustaceans to resist pathogen infection, and immune-related genes play an important role in this process (Junprung et al., 2021). Some immune-related genes in crustaceans were identified by comparative transcriptomics, such as Toll-like receptor (TLR), anti-lipopolysaccharide factor (ALF), lysosome and other important immune factors, which provide a solid foundation for studying immune mechanisms in crustaceans.
Vibrio parahaemolyticus is an important pathogen of P. trituberculatus (Yan et al., 2010). Infection will lead to multiple tissue damage and slow growth, resulting in huge economic losses (Yan et al., 2010). In previous studies, some genes may have played a role in warding off the original infection. However, due to the lack of relevant comparative transcriptomic studies, it is difficult to fully understand the molecular mechanism of P. trituberculatus resistance to the invasion of V. parahaemolyticus. In addition, sudden changes in salinity can affect the immunity of crustaceans (Chen et al., 2020; Long et al., 2021), making them more sensitive to pathogens. However, it is not clear through which genes or pathways salinity stress affects immunity.
A comparative transcriptome study was performed on the hepatopancreas before and after V. parahaemolyticus infection and low salt stress. The differentially expressed genes (DEGs) of the two types of stress were thoroughly investigated. Furthermore, the common DEGs were further screened and verified by a Kyoto Encyclopedia of Genes and Genomes (KEGG) enrichment analysis and quantitative polymerase chain reaction (qPCR). The results were used to clarify the immune mechanism of P. trituberculatus as well as the molecular mechanism of salinity affecting immunity.
Juvenile swimming crabs (80 days old; body weight = 35.5 ± 2.8 g) were obtained from the Haifeng Company (Weifang, China). After being transferred to the laboratory, 180 crabs were randomly placed into three 7 m3 cisterns at 26°C and acclimated for one week. After acclimation,180 individuals were divided into three groups. The control group was injected with 100 ml of sterile normal saline for marine crustaceans. The infection group was injected with V. parahaemolyticus (105 CFU/ml, 1 µl/g). The low-salinity group was transferred from normal seawater (26 ppt) to a low-salinity seawater environment (11 ppt). At 12, 24, 48, and 72 h infection and under low salt stress, the hepatopancreas was dissected, immediately frozen in liquid nitrogen, and stored at -80˚C. For each group, three samples at each time point were used for a transcriptome analysis, and each sample was a mixture of the hepatopancreas from 3–5 individuals. All experimental procedures were approved by the Yellow Sea Fisheries Research Institute’s animal care and use committee.
Total RNA was extracted from the samples using TRIzol Reagent (Invitrogen, Carlsbad, CA, USA) following the manufacture’s instruction. RNA integrity was detected using Agilent 2100 BioAnalyzer (Agilent Technologies, CA, USA) and the concentration was measured using Qubit® RNA Assay Kit in Qubit® 2.0 Flurometer (Life Technologies, CA, USA). 1.5 μg total RNA was used as the input material for libraries construction and the NEBNext®Ultra™ RNA Library Prep Kit for Illumina ® (NEB, USA) was used to construct RNA-Seq libraries according to the manufacturer’s instructions. Subsequently, the twenty-seven libraries were sequenced on the Illumina Nova seq 6000 platform and paired-end reads were generated.
Differential expression analysis between two groups, Adjusted padj ≤ 0.05 and |log2 (FoldChange)| ≥ 1 were set as the threshold for significantly differential expression. The DEGs of the two groups were analyzed by cluster analysis. DEGs were classified to signaling pathways by KEGG pathway analysis. Based on the KEGG annotation results and the official classification, The functions of DEGs in which signal pathways with false discovery rate (FDR) less than 0.05 were considered as the enriched significantly ones. The above analysis is based on the website of https://magic.novogene.com/customer/main/login.
Total RNA was extracted from the hepatopancreas with TRIzol reagents (Invitrogen, USA) based on the manufacturer’s protocols. Subsequently, the RNA was reverse-transcribed to first-strand cDNA using the PrimeScript™ RT reagent kit (TaKaRa, Japan). Eight genes candidates selected after transcriptomic screening were subjected to qPCR validation. qPCR was performed with a 7500Fast machine (Applied Biosystems, USA) using a SYBR green kit (Takara) based on a previously established method (Min et al., 2019). β-actin was employed as a reference gene, and the relative transcription level was calculated by the 2−ΔΔCt method. All data are presented as the mean ± SD of three samples with triplet repetitions. Differences between means were tested by ANOVA followed by Duncan’s post hoc test using SPSS software. Significant differences were accepted when the Padj ≤ 0.05.
The transcriptome sequencing and quality control of 27 hepatopancreas samples from pathogen and low salt stress at 0–72 h were performed using Illumina Hiseq sequencing technology. A total of 178.77 G of raw data was obtained (available on BIG sub https://ngdc.cncb.ac.cn/gsub/, GSA: CRA006377), and the average clean reads per sample was 44,142,119. The quality control results revealed that the average Q20 and Q30 values were above 97% and 93%, respectively, and the sequencing quality was excellent. The reference genome was compared using Clean Read, and the average mapping rate was 90.76% (Table S1).
Based on the analysis of DEGs (| log2 (FoldChange) | ≥ 1 & padj ≤ 0.05), after V. parahaemolyticus infection 12–72 h, a total of 3,047 DEGs were screened, with most screened at 72 h, accounting for 65.15% of the total DEGs (Figure 1). A total of 3,710 DEGs were screened from 12 to 72 h after low salt stress, with most DEGs screened at 12 h after low salt stress, accounting for 70.40% of the total DEGs (Figure 2). Following infection and low salt stress, there were 2,016 common DEGs, accounting for 42.52% of the total DEGs (Figure 3). According to the KEGG enrichment analysis, these common genes were primarily enriched in starch and sucrose metabolism, galactose metabolism, fructose and mannose metabolism (Figure 4).
A cluster analysis was performed on the DEGs in the infection and low salt groups, and the results revealed that the two groups of DEGs were clustered into six categories each. Subcluster 1 had the most DEGs in the infection group, with an initial down-regulated expression trend and then the DEGs were up-regulated and then down-regulated. Subcluster 2 was the largest cluster of DEGs in the low salt group, with an overall expression trend of down-regulation. The cluster analysis revealed that four groups (subclusters 2, 3, 4, and 5) generally showed an up-regulated expression trend, while two groups (subclusters 1 and 6) generally showed an down-regulated expression trend, (Figure 5). Under salinity stress, three groups (subclusters 3, 4, and 6) generally showed an up-regulated expression trend, while three groups (subclusters 1, 2, and 5) generally showed an down-regulated expression trend (Figure 6).
We screened DEGs whose expression patterns displayed the opposite trend after pathogen infection and low salt stress based on the results of the cluster analysis. Among them, 169 DEGs were up-regulated following pathogen infection and down-regulated following low salt stress (Fup&Sdown), accounting for 8.39% of all genes with common DEGs. According to the KEGG enrichment analysis, the roots of the Fup&Sdown group primarily participate in starch and sucrose metabolism, nitrogen metabolism, and amino sugar and nucleotide sugar metabolism processes. The Fdown&Sup group consisted primarily of arginine biosynthesis, 2-oxocarboxylic acid metabolism, and the ABC transporters pathway (Table S2).
A qPCR assay was used to test six DEGs in the three major pathways that were found to be enriched in the Fup&Sdown group (padj0.05). The majority of these genes were up-regulated 12 h after pathogen infection and peaked between 24 and 72 h. In contrast, the overall expression trend was down-regulated after salinity stress, with the lowest expression level at 72 h (Figure 7). The qPCR validation results were largely consistent with the trend of the transcriptome results (Figure 8), indicating that the DEG analysis in this study was highly accurate.
Figure 7 Clustering heatmap of DEGs in three pathways. Each column represented a sample, each row represents a gene. Colors represented the gene expression in a single sample, red represented higher expression, while blue represented lower expression. CL was the hepatopancreas tissue of the control group, FL was the hepatopancreas tissue of the V.parahaemolyticus infection group, LL was the hepatopancreas tissue under low salt stress group.
Figure 8 qPCR validations of transcriptomic data. Black was for V.parahaemolyticus infection group, gray was for low salt stress group. Different letter (a,b) in the picture indicate significant difference (P<0.05).
The main pathogen of P.trituberculatus is V. parahaemolyticus, which can cause acute hepatopancreas necrosis (AHPND) (Lee et al., 2015; Theethakaew et al., 2017), resulting in significant cultural loss. Salinity is an important environmental factor, and sudden changes in salinity can affect crustacean immunity (Wang and Chen, 2006; Chen et al., 2020). In crustaceans, the hepatopancreas is the primary tissue for energy storage, detoxification metabolism, and immune regulation, and it plays an important role in immunity and stress resistance (Rőszer, 2014; Xu et al., 2020b). In this study, a hepatopancreas transcriptome analysis was performed following V. parahaemolyticus infection and low salt stress, with the results being useful for determining the immune mechanisms of this species.
A total of 4,741 DEGs were discovered in this study after pathogen and salinity stress, including 3,047 pathogenic DEGs and 3,710 salinity stress DEGs. There were so many DEGs indicated that it was clear that the crab made a substantial response to the two stress factors. In addition, the pattern of the hepatopancreas response to the two kinds of DEGs was different, with the highest differential gene expression after pathogen stimulation at 72 h and the highest differential gene expression after low salt stress at 12 h. Among them, 2,016 DEGs were common to both types of stress, accounting for 42.52% of the total DEGs that were enriched in the starch and sucrose metabolism, galactose metabolism, fructose and mannose metabolism, and other pathways, indicating that these pathways may play an important role in disease resistance and salinity adaptation, or the process would be susceptible to pathogens and low salt stress.
In crustaceans, genes associated with innate immunity against pathogen invasion have been reported. For example, under the attack of V. algininaniae and white spot syndrome virus (WSSV, Candida lusitaniae), the Toll gene showed different up-regulation reactions, indicating that it was involved in the immune response of the body (Zhou et al., 2015). The expression of ALF was significantly down-regulated after WSSV stimulation in Macrobrachium nipponense, indicating that it had a strong immune response to the pathogen (Jiang et al., 2022). The plasma hemocyanin cyanogen concentration, hepatopancreas hemocyanin mRNA, and subunit expression of Litopenaeus vannamei were significantly affected by Vibrio harveyi and Staphylococcus aureus stress (Pan et al., 2019). In this study, Lectin, hemocyanin, TLR, ALF, and other innate immune genes were all expressed differently after pathogen stress, indicating that these genes also play a role in resistance to V. parahaemolyticus invasion in the hepatopancreas.
Heavy rain during production often causes a large number of shrimp and crab deaths (Le Moullac and Haffner, 2000; Ye et al., 2008; Yan et al., 2010). We also found that the P. trituberculatus in the low-salt environment was more sensitive to V. parahaemolyticus, indicating that the low-salt environment led to a decline in the body’s immunity to some extent in Crustacea (Chen et al., 2020; Long et al., 2021). Based on the cluster analysis results, we screened the reverse expression genes (Fup&Sdown) of the two factors after stress to investigate the mechanism of low salt stress on immunity. A total of 169 DEGs were found to be up-regulated after pathogen infection and down-regulated after low salt stress, as well as being enriched in starch and sucrose metabolism, nitrogen metabolism, amino sugar, and nucleotide sugar metabolism. Several genes were screened for qPCR verification in these processes, and the verification results were consistent with the transcriptome data. The metabolism of starch and sucrose is related to energy absorption and release (Tang et al., 2015). Nitrogen metabolism is linked to the detoxification metabolism in the body (Meng et al., 2021). The metabolism of amino and nucleotide sugars has been reported to be associated with fungal sporogenesis (Ding et al., 2019). These processes are activated after pathogen stress, but are inhibited under low salt stress, which indicates that it may be an important way for salinity to affect immunity.
We performed a comparative transcriptome analysis of P. trituberclatus hepatopancreas under V. parahaemolyticus infection and low salt stress. We found a total of 4,741 DEGs, including 2,016 DEGs shared by the two stresses, indicating that a large number of genes may play roles in both disease resistance and adaptation. Following stimulation by the two factors, 169 DEGs were discovered, which were primarily enriched in starch and sucrose metabolism, nitrogen metabolism, amino sugar, and nucleotide sugar metabolism processes. These findings will improve our understanding of the P. trituberculatus immune mechanism against V. parahaemolyticus, as well as the molecular mechanism of salinity affecting immunity.
The datasets presented in this study can be found in online repositories. The names of the repository/repositories and accession number(s) can be found in the article/Supplementary Material.
The studies involving human participants were reviewed and approved by Yellow Sea Fisheries Research Institute’s animal care and use committee. The patients/participants provided their written informed consent to participate in this study. The animal study was reviewed and approved by Yellow Sea Fisheries Research Institute’s animal care and use committee. Written informed consent was obtained from the owners for the participation of their animals in this study.
YL: Conducted experiments, Analyzed data and results, Paper writing. JW: Analyzed data and results, Paper revising. BG: Experiment designed, collected the samples, reviewed and edited draft paper. PL: Reviewed and edited draft paper. JL: Experiment designed, Reviewed and edited draft paper. All authors contributed to the article and approved the submitted version.
This research was supported by the National Key R&D Program of China (2018YFD0900303), the National Natural Science Foundation of China (42076116), the earmarked fund for CARS-48 and the Central Public-interest Scientific Institution Basal Research Fund, CAFS (NO. 2020TD46).
The authors declare that the research was conducted in the absence of any commercial or financial relationships that could be construed as a potential conflict of interest.
All claims expressed in this article are solely those of the authors and do not necessarily represent those of their affiliated organizations, or those of the publisher, the editors and the reviewers. Any product that may be evaluated in this article, or claim that may be made by its manufacturer, is not guaranteed or endorsed by the publisher.
The Supplementary Material for this article can be found online at: https://www.frontiersin.org/articles/10.3389/fmars.2022.1044990/full#supplementary-material
Chen Q., Zhang Y., Zhao Q. (2020). Expression analysis of immune-associated genes in hemocytes of mud crab Scylla paramamosain under low salinity challenge. Fish Shellfish Immunol. 107, 16–25. doi: 10.1016/j.fsi.2020.09.021
Ding Z. J., Yan-Xiang Q. I., Zeng F. Y., Peng J., Xie Y. X., Zhang X.. (2019). Amino sugar metabolism pathway involved in chlamydospore formation of fusarium oxysporum f. sp. cubense. Mycosystema 38(04). doi: 10.13346/j.mycosystema.180279
Fang F., Yuan Y., Jin M., Shi B., Zhu T., Luo J., et al. (2021). Hepatopancreas transcriptome analysis reveals the molecular responses to different dietary n-3 PUFA lipid sources in the swimming crab portunus trituberculatus. Aquaculture 543, 737016. doi: 10.1016/j.aquaculture.2021.737016
Jiang Z., Zhou C., Wang H., Liu B., Qin W., Huang X., et al. (2022). Anti-lipopolysaccharide factors regulated by stat, dorsal, and relish are involved in anti-WSSV innate immune defense in macrobrachium nipponense. Fish Shellfish Immunol. 121, 342–350. doi: 10.1016/j.fsi.2022.01.016
Junprung W., Supungul P., Tassanakajon A. (2021). Structure, gene expression, and putative functions of crustacean heat shock proteins in innate immunity. Dev. Comp. Immunol. 115, 103875. doi: 10.1016/j.dci.2020.103875
Kong T., Lin S., Ren X., Li S., Gong Y. (2020). Transcriptome and metabolome integration analysis of mud crab Scylla paramamosain challenged to vibrio parahaemolyticus infection. Fish Shellfish Immunol. 103, 430–437. doi: 10.1016/j.fsi.2020.05.069
Lee C.-T., Chen I.-T., Yang Y.-T., Ko T.-P., Huang Y.-T., Huang J.-Y., et al. (2015). The opportunistic marine pathogen vibrio parahaemolyticus becomes virulent by acquiring a plasmid that expresses a deadly toxin. Proc. Natl. Acad. Sci. U.S.A 112 (34), 10798–803. doi: 10.1073/pnas.1503129112
Lee J., Cho B. C., Park J. S. (2022). Transcriptomic analysis of brine shrimp artemia franciscana across a wide range of salinities. Mar. Genomics 61, 100919. doi: 10.1016/j.margen.2021.100919
Le Moullac G., Haffner P. (2000). Environmental factors affecting immune responses in Crustacea. Aquaculture 191 (1), 121–131. doi: 10.1016/S0044-8486(00)00422-1
Lin J., Shi X., Fang S., Zhang Y., You C., Ma H., et al. (2019). Comparative transcriptome analysis combining SMRT and NGS sequencing provides novel insights into sex differentiation and development in mud crab (Scylla paramamosain). Aquaculture 513, 734447. doi: 10.1016/j.aquaculture.2019.734447
Liu M., Jiang X., Chen A., Chen T., Cheng Y., Wu X.. (2020). Transcriptome analysis reveals the potential mechanism of dietary carotenoids improving antioxidative capability and immunity of juvenile Chinese mitten crabs eriocheir sinensis. Fish Shellfish Immunol. 104, 359–373. doi: 10.1016/j.fsi.2020.06.033
Long J., Cui Y., Wang R., Chen Y., Zhao N., Wang C., et al. (2021). Combined effects of high salinity and ammonia-n exposure on the energy metabolism, immune response, oxidative resistance and ammonia metabolism of the pacific white shrimp litopenaeus vannamei. Aquaculture Rep. 20, 100648. doi: 10.1016/j.aqrep.2021.100648
Lou F., Gao T., Han Z. (2019). Effect of salinity fluctuation on the transcriptome of the Japanese mantis shrimp oratosquilla oratoria. Int. J. Biol. Macromolecules 140, 1202–1213. doi: 10.1016/j.ijbiomac.2019.08.223
Lv J. J., Gao B. Q., Liu P., Li J., Meng X. L.. (2017). Linkage mapping aided by de novo genome and transcriptome assembly in portunus trituberculatus: Applications in growth-related QTL and gene identification. Sci. Rep. 7 (1), 7874. doi: 10.1038/s41598-017-08256-8
Meng X., Jayasundara N., Zhang J., Ren X., Gao B., Li J., et al. (2021). Integrated physiological, transcriptome and metabolome analyses of the hepatopancreas of the female swimming crab portunus trituberculatus under ammonia exposure. Ecotoxicology Environ. Saf. 228, 113026. doi: 10.1016/j.ecoenv.2021.113026
Wei M., Xu W.-t., Gan T., Wang L., Zhang H.-x., Zhao F.-z., et al. (2019). Cloning, expression prolife, and immune characterization of a novel stat family member (stat5bl) in Chinese tongue sole (Cynoglossus semilaevis) - ScienceDirect. Fish Shellfish Immunol. 84, 962–969. doi: 10.1016/j.fsi.2018.10.030
Pan L., Zhang X., Yang L., Pan S.. (2019). Effects of vibro harveyi and staphyloccocus aureus infection on hemocyanin synthesis and innate immune responses in white shrimp litopenaeus vannamei. Fish Shellfish Immunol. 93, 659–668. doi: 10.1016/j.fsi.2019.08.016
Rőszer Tamás (2014). The invertebrate midintestinal gland ("hepatopancreas") is an evolutionary forerunner in the integration of immunity and metabolism. Cell Tissue Res. 358 (3), 685–695. doi: 10.1007/s00441-014-1985-7
Tang X., Wang H., Shao C., Shao H. (2015). KEGG pathway visualization of starch and sucrose metabolism changes under salt stress. PLOS ONE. doi: 10.1371/journal.pone.0124421.g006
Theethakaew C., Nakamura S., Motooka D., Matsuda S., Kodama T., Chonsin K., et al. (2017). Plasmid dynamics in vibrio parahaemolyticus strains related to shrimp acute hepatopancreatic necrosis syndrome (AHPNS). Infection Genet. Evol. J. Mol. Epidemiol. Evolutionary Genet. Infect. Dis. 51, 211. doi: 10.1016/j.meegid.2017.04.007
Wang F. I., Chen J.-C. (2006). Effect of salinity on the immune response of tiger shrimp penaeus monodon and its susceptibility to photobacterium damselae subsp. damselae. Fish Shellfish Immunol. 20 (5), 671–681. doi: 10.1016/j.fsi.2005.08.003
Xu W., Cui Z., Wang N., Zhang M., Wang J., Xu X., et al. (2021). Transcriptomic analysis revealed gene expression profiles during the sex differentiation of Chinese tongue sole (Cynoglossus semilaevis). Comp. Biochem. Physiol. Part D: Genomics Proteomics 40, 100919. doi: 10.1016/j.cbd.2021.100919
Xu Z., Wei Y., Guo S., Lin D., Ye H. (2020a). B-type allatostatin modulates immune response in hepatopancreas of the mud crab Scylla paramamosain. Dev. Comp. Immunol. 110, 103725. doi: 10.1016/j.dci.2020.103725
Xu Z., Wei Y., Guo S., Lin D., Ye H. (2020b). Short neuropeptide f enhances the immune response in the hepatopancreas of mud crab (Scylla paramamosain). Fish Shellfish Immunol. 101, 244–251. doi: 10.1016/j.fsi.2020.04.007
Yan B., Liang L., Zhang X. (2010). Research progress on main diseases of portunus tricolata. Fisheries Sci. Technol. Inf. 1, 6. doi: CNKI:SUN:SCKJ.0.2010-01-008
Ye J. S., Wang X. Q., Ma Y., et al. (2008). Effects of salinity mutation on non-specific immune factors of litopenaeus vannamei. Mar. Fisheries Res. 29 (1), 6. doi: CNKI:SUN:HYSC.0.2008-01-007
Keywords: Portunus trituberculatus, Vibrio parahaemolyticus, salt, transcriptome, immunity
Citation: Li Y, Lv J, Wu J, Liu P and Gao B (2022) Comparative transcriptome analysis of Portunus trituberculatus in response to Vibrio parahaemolyticus and low salinity. Front. Mar. Sci. 9:1044990. doi: 10.3389/fmars.2022.1044990
Received: 15 September 2022; Accepted: 28 September 2022;
Published: 14 October 2022.
Edited by:
Bin Xia, Qingdao Agricultural University, ChinaCopyright © 2022 Li, Lv, Wu, Liu and Gao. This is an open-access article distributed under the terms of the Creative Commons Attribution License (CC BY). The use, distribution or reproduction in other forums is permitted, provided the original author(s) and the copyright owner(s) are credited and that the original publication in this journal is cited, in accordance with accepted academic practice. No use, distribution or reproduction is permitted which does not comply with these terms.
*Correspondence: Baoquan Gao, Z2FvYnFAeXNmcmkuYWMuY24=
†These authors have contributed equally to this work
Disclaimer: All claims expressed in this article are solely those of the authors and do not necessarily represent those of their affiliated organizations, or those of the publisher, the editors and the reviewers. Any product that may be evaluated in this article or claim that may be made by its manufacturer is not guaranteed or endorsed by the publisher.
Research integrity at Frontiers
Learn more about the work of our research integrity team to safeguard the quality of each article we publish.