- 1Key Laboratory of Mariculture, Ministry of Education, Ocean University of China, Qingdao, China
- 2Administration Department, Weihai Taifeng Seawater Seedling Co., LTD, Weihai, China
Introduction: As a unique type of immunological process, pregnancy and subsequent parturition are associated with a series of inflammatory events. As an anti-inflammatory cytokine, IL-22 has been proven to participate in the parturition process in mammals. However, less is known about ovoviviparous teleosts. To investigate the role of IL-22 in fish parturition, black rockfish, an ovoviviparous teleost, was used. This fish is unique in that it provides over 60% nutrition supply for over 50 thousand embryos at the same time though a placenta-like structure during pregnancy while delivering all fries in two hours.
Methods: Sequence alignment, phylogenetic tree analysis and homology modeling were performed on IL-22 and its receptor. In situ hybridization demonstrated ovarian localization of il22 and il22r1. The protein of rIL-22 was obtained through the prokaryotic expression. RNA-seq analysis was performed on black rockfish ovarian cells treated with rIL-22.
Results: In situ hybridization results showed that both il22 and il22ra1 were localized in the perinatal follicle layer and embryonic envelope. The il22 expression level was significantly increased during parturition compared to before and after parturition (P<0.05). To further understand the mechanism of IL-22 in parturition, rIL-22 was obtained. The downstream variation genes in primary cultured ovarian cells in the perinatal period were analyzed according to the transcriptomic results. A total of 168 differentially expressed genes (DEGs) were identified in the rIL-22 group compared with the PBS control group (padj < 0.05 and |log2FoldChange| 1). Of those, 134 DEGs were identified in the LPS and rIL-22 treatment groups, including chemokine, cytokine, and PG synthesis. 34 DEGs were mainly identified in immune-related pathways and reorganization of the cytoskeleton responded only to rIL-22 stimulation but not LPS. KEGG pathways mainly included immune response, PG synthesis, cell death and angiogenesis (p value < 0.05).
Discussion: Taken together, our results indicated that IL-22 plays an important role in the parturition of black rockfish by upregulating PG synthesis and increasing chemokine, proinflammatory factor, as well as PG synthetase levels.
1 Introduction
Teleost, the most abundant vertebrate, exhibits various reproductive strategies, including viviparity, oviparity and ovoviviparity. As the transitional strategy between viviparity and oviparity, ovoviviparity presents a lecithotrophic source homologous to egg-laying oviparous teleosts. And the internal fertilization in ovoviviparous species is similar to viviparous species. Furthermore, ovoviviparous teleosts also exhibit parturition behavior similar to mammals (Venkatesh et al., 1992; Lyu et al., 2021; Lyu et al., 2022). Parturition is the period and process when the fetus separates from its mother and becomes an independent individual. Parturition is a crucial reproductive event for the continuation of species. As an intriguing immunological event, the onset of parturition expresses a series of immunomodulatory effects during pregnancy. Research in the field of immunology of pregnancy has investigated the possibility that cellular immune effectors participate in these pregnancy complications. In particular, the effects that cytokines have on the fetus and thus on the success and failure of pregnancy have been investigated as the triggers of inflammation (Mazaki-Tovi et al., 2007; Raghupathy and Kalinka, 2008). In human parturition, the levels of proinflammatory cytokines were significantly increased without any infection in the amniotic fluid, uterine tissue, membranes, or maternal serum (Hadley et al., 2018). This indicated that these proinflammatory factors have a vital function in the initiation of parturition, in addition to inducing immunological responses. During pregnancy, the mother undergoes a state of immunosuppression to protect the fetus from maternal immune rejection (Abu-Raya et al., 2020). At the end of pregnancy, the changes to parturition in mammals are accompanied by the transition of the endometrium and the amniotic membrane from a static state to a contractile state. One leading reason is that the uterine environment gradually changes to a proinflammatory environment (Conde-Agudelo and Romero, 2014).
Interleukins (ILs) are regarded as local inflammatory mediators in gestational tissues during pregnancy and parturition. Studies have shown that IL-1 is an essential mediator in embryo implantation and the establishment of pregnancy in mammals (Paulesu et al., 2005). It was also revealed that IL-6 was crucial for controlling the progression of parturition by regulating genes involved in the prostaglandin (PG)-mediated uterine activation cascade (Robertson et al., 2010). In addition, ILs have been shown to play a role in the regulation of ovarian functions in teleosts. It is well established that ovulation is similar to inflammatory responses (Liu et al., 2017; Chatterjee et al., 2020). Treatment with IL-6 could induce a marked increase in the ovulation rate by activating matrix metalloproteinase (MMP) and PG synthesis in climbing perch (Anabas testudineus) (Chatterjee et al., 2020). Studies on orange-spotted grouper (Epinephelus coioides) and sea bass (Dicentrarchus labrax L.) have shown that recombinant IL-1β (r IL-1β) can also promote the expression of prostaglandin G/H synthase 2 (ptgs2), a key enzyme in PG synthesis (Buonocore et al., 2005; Lu et al., 2008).
IL-22 is a novel cytokine in the IL-10 family produced by T cells and natural killer (NK) cells (Dudakov et al., 2015). IL-22 exhibited both proinflammatory and anti-inflammatory responses. The double responses depend on the tissue microenvironment including the cytokine environment (Alabbas et al., 2018). IL-22 has been observed in both mice and humans to influence the production of other proinflammatory cytokines, including IL-6, IL-8 and tumor necrosis factor alpha (TNF-α), to coordinate the inflammatory response (Andoh et al., 2005; Kong et al., 2012). In addition, IL-22 plays a dual role in pregnancy and parturition. It has been shown that the increased expression of il22 in unexplained recurrent pregnancy loss (uRPL) patients may be the cause of endometrial homeostasis disorder and recurrent miscarriage (Heidari et al., 2021). In endometriosis, the expression of il22 is significantly upregulated and induces C-C motif chemokine 2 (CCL2) production to recruit macrophages (Mei et al., 2019). More recently, IL-22 was found to cause fetal injury in the amniotic cavity leading to neonatal death. However, it may also participate in host defense against microbial invasion of the amniotic cavity (Gershater et al., 2022). In mice, IL-22 acts as an anti-inflammatory cytokine and prevents premature parturition by inhibiting placental cell death (Dambaeva et al., 2018). In the human uterus, IL-22 secreted by decidual stromal cells (DSCs) and decidual natural killer (dNK) cells promotes the survival of trophoblasts and maintains pregnancy by combining with the receptor (Wang et al., 2013). These studies suggest that IL-22 has the dual role of promoting miscarriage and maintaining pregnancy. Although there have been some studies of IL-22 in mammalian pregnancy and parturition, most of them focus on the molecular level. Therefore, research on nonmammals and the addition of bioinformatics can provide a broader basis for the role of IL-22 in parturition.
Black rockfish (Sebastes schlegelii) is an important commercial aquaculture species and performs an ovoviviparous reproductive strategy (Zhang et al., 2020). Females mate with males and store sperm inside the ovary from December until April of the following year when the oocytes mature and fertilization is complete (Wang et al., 2021). The female then gives birth after approximately one month of gestation (Liu et al., 2019). During late gestation and the final parturition period, female individuals always suffer from inflammatory symptoms in the ovary and cloaca, including hyperemia and swelling (Lyu et al., 2022). In the present study, to clarify the possible role of IL-22 in the parturition of black rockfish. We first investigated the tissue expression pattern and ovarian localization of IL-22 in black rockfish. We also analyzed the rna-seq obtained from ovarian cells treated with recombinant IL-22 (rIL-22). Our findings demonstrated the function of IL-22 in ovoviviparous teleosts for the first time and provided evidence for the conserved function of cytokines in the reproductive system during evolution.
2 Materials and methods
2.1 Fish and sampling
All animal experiments in this research were approved by the Animal Research and Ethics Committees of Ocean University of China prior to the initiation of the study. No endangered or protected species were involved in this experiment. All experiments were performed in accordance with the relevant guidelines and regulations.
Experimental individuals were anesthetized with 100 ng/mL 3-aminobenzoate methanesulfonic acid (MS-222) to reduce pain before sacrifice and treatment. The fish used in the experiment were from the aquaculture population maintained in marine cages offshore of Rushan, Shandong, China (36.92°N, 121.54°E). A total of 26 female black rockfish were selected randomly for the experiment (over 3 years old, body weight: 1.0 ± 0.4 kg, body length: 24.3 ± 1.3 cm). All the animals were housed for 2 days in indoor cement pools with a culvert system before the experiment. Whole tissue samples, including heart, liver, spleen, kidney, head kidney, intestine, gill, skin, pituitary, muscle, brain and ovary samples, were collected from three females in December 2021. In April 2022, spleen and ovarian stroma cell cultures were performed on gestational female individuals, and parts of the ovary samples were fixed in 4% paraformaldehyde for in situ hybridization. The ovarian tissues were sampled at time points of 24 hours before delivery (n=5), during delivery (n=3), and 24 hours after delivery (n=4). For the “before parturition” samples, the swollen cloaca of maternal fish was full of squeezed fries (Lyu et al., 2022). Since a large number of fries (approximately 50,000 fries) will be delivered, the parturition process normally takes approximately one hour in which we collected the “during parturition” sample. For the “after parturition” samples, we recorded the parturition start time of each maternal fish and collected ovary samples 24 hours later.
2.2 Total RNA extraction and reverse transcription
Total RNA was extracted from tissues using TRIzol reagent (Invitrogen, America) according to the manufacturer’s instructions. RNA quantity and purity were assessed by a Biodropsis BD-1000 nucleic acid analyzer (OSTC, China) and electrophoresis using a 1% agarose gel. cDNA was prepared using the Prime ScriptTM RT Real Time Kit with gDNA Eraser (Perfect Real Time) (TAKARA, Japan) according to the manufacturer’s instructions.
2.3 Quantitative real-time PCR
The ChamQTM SYBR Color qPCR Master Mix (High Rox Premixed) kit (Vazyme, China) was used for qPCR according to the reagent instructions. The qPCR procedure was 95°C for 30 s, followed by 40 cycles of 95°C for 10 s and 60°C for 30 s. The threshold circulation (CT) values of each sample were measured using 18S rRNA (Accession number: KF430619.1) as an internal reference gene (Liman et al., 2013). Three replications of qPCR were performed to confirm the results using the 2-ΔΔCT method. The primers for qPCR are shown in Table 1.
2.4 Molecular characterization of il22 and its receptor cDNAs
The cDNA sequences of the black rockfish il22, il22 receptor alpha 1 (il22ra1) and il22 receptor alpha 2 (il22ra2 or il22 bp) genes were cloned and obtained from black rockfish genomic data (PRJNA516036). Primers for cloning il22 and il22r were designed using Primer5 software (Premier, Canada). The primers for the full-length cDNA sequences of il22 and il22r are shown in Table 1. The 2×Phanta Max Master Mix (Dye Plus) (Vazyme, China) was used for cloning, and the PCR program used was 95°C for 3 min, 35 cycles of 95°C for 15 s, 60°C for 15 s, and 72°C for 2 min, followed by 72°C for 5 min, with a final hold at 4°C. The PCR product was purified and cloned into the pCE2 TA/Blunt-Zero vector (Vazyme, China) for subsequent sequencing. The cleavage sites of the signal peptides were predicted using the SignalP 6.0 program (http://www.cbs.dtu.dk/services/SignalP/). The molecular weight and isoelectric point were predicted using the ExPASy Compute pI/MW tool (http://web.expasy.org/compute_pi/). Multiple sequence alignments were generated by the ClustalX 2.1 and Espript 3.0 programs (https://espript.ibcp.fr/ESPript/cgi-bin/ESPript.cgi). The phylogenetic tree was reconstructed from the multiple alignments of the amino acid sequences with the neighbor-joining method using MEGA11 (Tamura et al., 2021). The values on the trees represent bootstrap scores of 1,000 iterations, indicating the credibility of each branch. The 3D structures of black rockfish IL-22/IL-22R1/IL-22R2 were modeled using SWISS-Model (https://swissmodel.expasy.org/).
2.5 Recombinant expression of IL-22
rIL-22 was expressed by the Escherichia coli Rosetta-gami B (DE3)/pET series vector (Novagen, Germany) system and purified by a Ni-NTA column (Beyotime, China). The primers with overlaps for IL-22 were designed to amplify the sequence encoding IL-22 (Table 1). After digestion with BamH I and Kpn I, the amplicon was cloned into the pET-C-His expression vector, and the constructed plasmid was subsequently transformed into E. coli Rosetta-gami B (DE3). See Supplementary 1 for details.
The positive clone of E. coli Rosetta-gami B (DE3) was grown in Luria Broth (LB) medium with glucose (2 g/L, Sigma−Aldrich), ampicillin (100 mg/L, Sigma−Aldrich, St. Louis, MO, USA) and chloramphenicol (34 mg/L, Sangon Biotech, Shanghai, China) in a shock incubator at 37°C for 4 h. When the OD600 ranged from 0.4 to 0.6, isopropyl-β-D-thiogalactopyranoside (IPTG, Sigma−Aldrich) was added at a final concentration of 0.4 mM, and the temperature was lowered to 16 °C. After 14 h of induction, the cells were collected by centrifugation at 3,500 × g for 10 min at 4°C. The cells were resuspended in lysis buffer (500 mM NaCl, 20 mM phosphate buffer, 10 mM imidazole, and pH 7.4). The sample was ultrasonically decomposed on ice, followed by centrifugation at 12,000 g for 10 min at 4°C to retain the supernatant. The supernatant was collected and filtered through a 0.22 μm filter. The rIL-22 in the supernatant was purified on a Ni-NTA column. The concentration of rIL-22 was determined by a BCA kit (Beyotime Biotechnology, Shanghai, China), and the purified protein was analyzed by SDS−PAGE. The purified rIL-22 was frozen in liquid nitrogen and stored at -80°C.
2.6 In situ hybridization
Following the manufacturer’s instructions, the 2 × Phanta® Max Master Mix (Dye Plus) kit (Vazyme, China) was used for PCR, and the cDNA of the ovary was used as a template. Following the manufacturer’s instructions, the DIG RNA Labeling Kit (SP6/T7) (Roche, Switzerland) was used to synthesize antisense or sense probes for the in vitro transcription of il22 and il22ra1 mRNA. The specific process of ISH was performed as previously reported (Wang et al., 2022).
2.7 Culture of ovarian cells
We obtained ovarian cells by removing the ovarian parietal membrane, embryo, fries and large oocyte with forceps and scissors. The cells were cultured for 48 h in L15 (G-Clone, China, Beijing) complete medium with 10% fetal bovine serum (FBS) (G-Clone, China, Beijing) and 1% penicillin−streptomycin-gentamicin solution (Absin, China, Shanghai). Subsequently, L15 starvation medium without FBS but containing 1% penicillin−streptomycin-gentamicin solution was used to culture the cells for 12 h under low-transcription conditions. Finally, complete medium with PBS (solvent control, n=3), lipopolysaccharides (LPS, final concentration: 100 ng/mL, n=3), and rIL-22 (final concentration: 500 ng/mL, n=3) was used to treat cells for 6 h. The LPS group was used as a positive control to induce inflammation in ovarian cells. Subsequently, the cells were harvested for RNA extraction.
2.8 RNA isolation and library construction
RNA isolation of each cell sample was performed as shown previously. Qualities and concentrations of total RNA were evaluated by a NanoDrop (Thermo Fisher Scientific, USA) and an Agilent 2100 bioanalyzer system (Agilent Technologies, USA). The RIN (RNA Integrity Number) value of each sample was above 9. The NEBNext® Ultra™ RNA Library Prep Kit for Illumina® (NEB, USA) was employed to generate 9 sequencing libraries according to the manufacturer’s instructions. The samples were sequenced on an Illumina HiSeq X Ten platform, and 150-bp paired-end reads were generated.
The transcriptomic data obtained by removing the reads of adaptors were aligned to the reference Sebastes schlegelii genome (PRJNA516036) with HISAT2 (Kim et al., 2015a). Assemble and quantification analyses were accomplished with the StringTie package (Pertea et al., 2016). The multiple mapped reads were removed, and the count numbers of unique mapped reads and FPKM (Fragments Per Kilobase Per Million) were retrieved and normalized with previous references (Anders et al., 2015). Principal component analysis (PCA) was performed by the ggplot2 package.
Based on the DESeq2 package, statistical analysis of transcripts with a cutoff “padj” < 0.05 and absolute fold change values greater than 1 were marked as significantly differentially expressed genes (DEGs). DEG annotation was based on the reference Sebastes schlegelii genome. Thereafter, DEGs were assigned to Gene Ontology (GO) classification by the aid of the Blast2GO program with the p value threshold < 0.05 (Götz et al., 2008). Kyoto Encyclopedia of Genes and Genomes pathway enrichment analysis (KEGG, a database of biological systems, http://www.genome.jp/kegg/) was performed on significant pathway enrichment analysis. The clusterProfiler R package was employed to test the statistical enrichment of DEGs in KEGG pathways with the p value threshold < 0.05 (Kanehisa et al., 2017). The STRING database was used to construct a protein−protein interaction (PPI) network with Homo sapiens used as a reference species.
2.9 Statistical analysis
All the data are shown as the mean ± standard deviation (SD). Statistical analysis was performed by one-way ANOVA followed by Tukey’s and Sidak’s multiple range tests. Differences were considered significant at P < 0.05. All statistical procedures and graphs were generated with GraphPad Prism 9 (GraphPad Software, USA).
3 Results
3.1 Molecular cloning and characterization of il22 and its receptors
The cDNA sequences of il22 (Accession number: OP331275), il22ra1 (Accession number: OP331276) and il22ra2 (Accession number: OP331277) were identified and cloned based on black rockfish genomic data. The ORFs of il22, il22ra1 and il22ra2 were 567 bp, 1671 bp and 612 bp, encoding 188 amino acids, 556 amino acids and 203 amino acids, respectively. The physicochemical properties of the IL-22 protein are as follows: the molecular weight is 18.17 kDa, and the theoretical isoelectric point is 6.62. According to amino acid sequence analysis, IL-22 from black rockfish has six α-helices and two adjacent disulfide bridges. Meanwhile, the amino acid homology of IL-22 in black rockfish is high compared with that in Nile tilapia (Oreochromis niloticus) (Figure 1A). However, the amino acid sequences of IL-22RA1 and IL-22RA2 were not conserved (Figure 1B). The phylogenetic analysis showed that IL-22 and its receptors were clustered with teleost and differed from other vertebrates (Figures 1C, D).
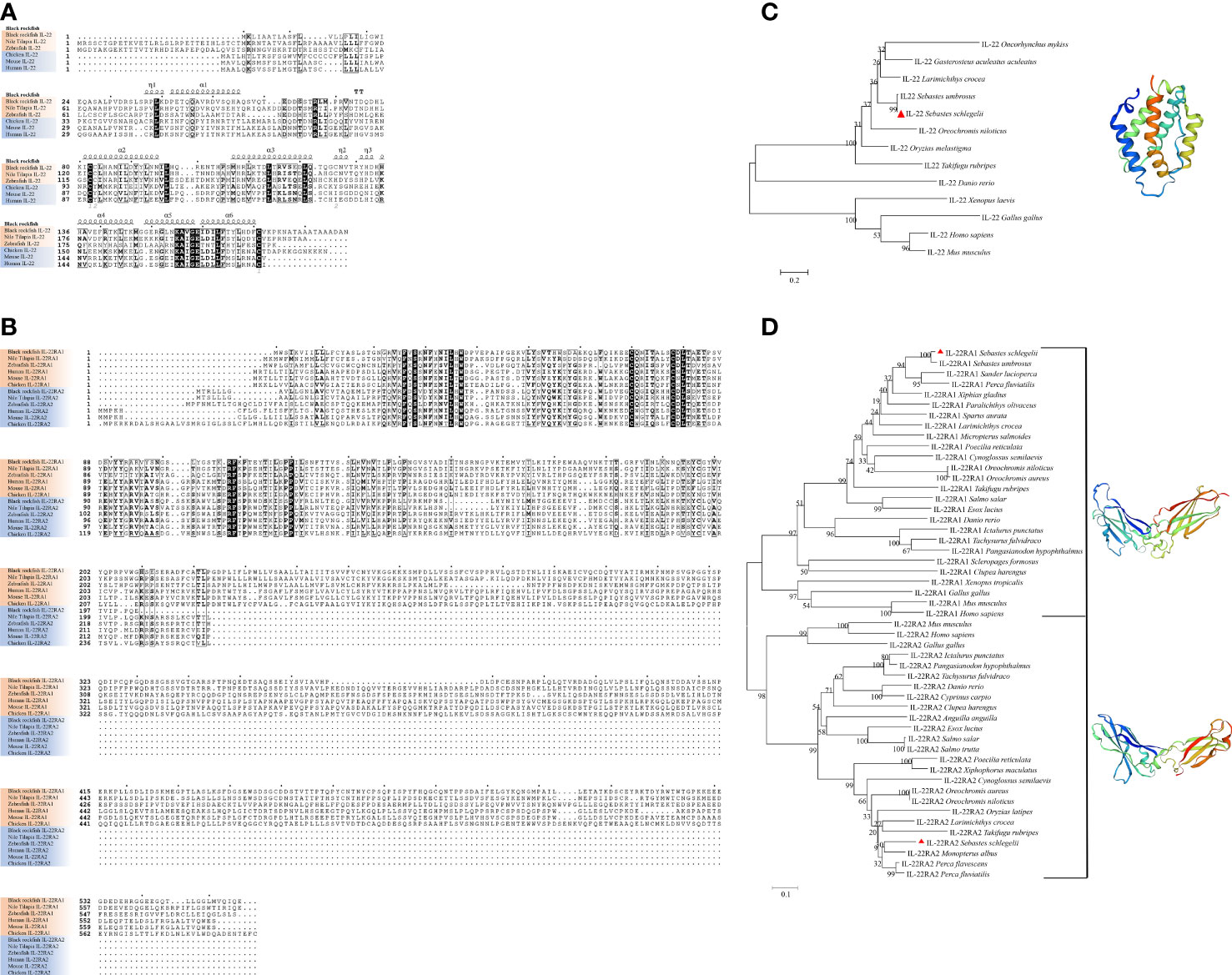
Figure 1 Multiple sequence alignment of IL-22 (A), IL-22RA1 and IL-22RA2 (B) in black rockfish, nile tilapia, zebrafish, chicken, mouse, and human. Phylogenetic tree of IL-22 (C), IL-22RA1 and IL-22RA2 (D) in black rockfish and other species. Data were resampled with 1,000 bootstrap replicates. The access number of the sequences used to generate the multiple sequence alignment and phylogenetic tree were listed in Supplementary 2.
3.2 Expression patterns and localization of il22 and its receptors
Tissue distribution analysis showed that il22 and its receptors were widely expressed in various tissues. In particular, il22 was highly expressed in the intestines, gills and skin of black rockfish. In addition, a certain amount of il22 was detected in the ovary (Figure 2A1). The tissue expression patterns of il22ra1 and il22ra2 were similar to il22. High il22ra1 mRNA levels were observed in the intestines, gills, skin, and ovary (Figure 2A2). The il22ra2 transcript is widely expressed in different tissues. Compared with il22ra1, il22ra2 was highly expressed in the kidney and head kidney (Figure 2A3). The il22 expression pattern in the black rockfish perinatal period showed that il22 was significantly increased during parturition (P<0.05) (Figure 2B).
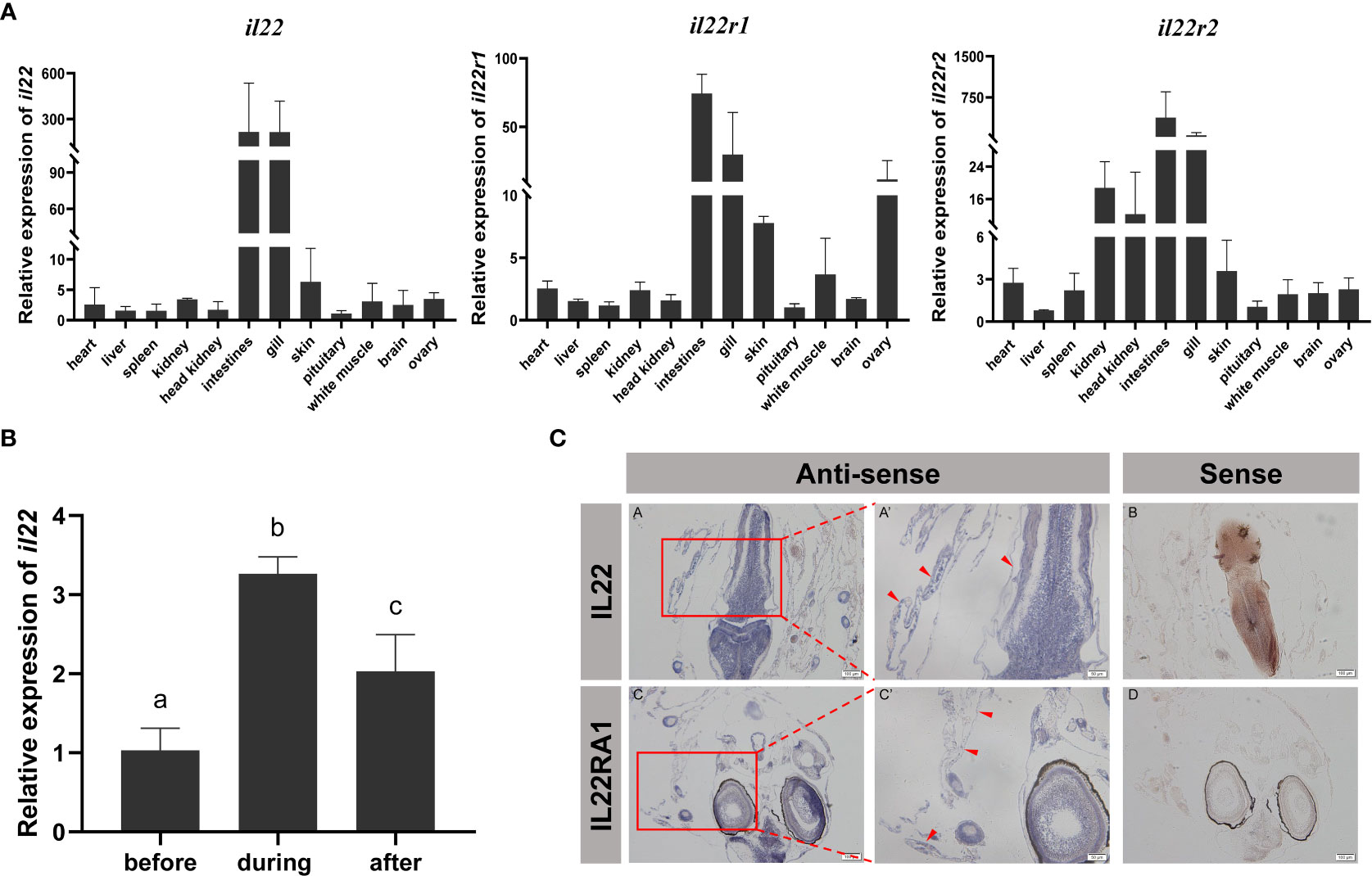
Figure 2 Relative expression levels of il22 (A1), il22ra1 (A2) and il22ra2 (A3) in different organs (heart, liver, spleen, kidney, head kidney, intestine, gill, skin, pituitary, white muscle, brain, and ovary) (n = 3). The X axis indicates different tissues. The Y axis indicates the relative expression normalized to 18s rRNA. (B) Relative expression levels of il22 in black rockfish before parturition (n = 5), during parturition (n = 3), and 24 h after parturition (n = 4). The X axis indicates different parturition stage of black rockfish. The Y axis indicates the relative expression normalized to 18s rRNA. The data are presented as the mean ± SD. Different letters indicate significant differences (P < 0.05). (C) Localization of il22 and il22ra1 mRNA in ovary of black rockfish via in situ hybridization. The red arrows indicate positive signals. Scale bars (A–D) = 100 μm. Scale bars (A’, C’) = 50 μm.
The embryo development and mastery exchange in black rockfish was determined by the maternal-fetal interface. The maternal-fetal interface consists of the follicle layer, embryonic envelope and the liquid between them. We performed in situ hybridization of il22 and il22ra1 on ovaries during the perinatal period. The results showed that il22 and il22ra1 positive signals were observed in the follicle layer and embryonic envelope. In embryos, extensive positive signals of il22 and il22ra1 were also detected in various tissues, especially in gill, intestine and skin tissues (Figure 2C).
3.3 Prokaryotic expression, purification and functional verification of rIL-22
To further study the molecular function of IL-22, rIL-22 was generated through a prokaryotic expression system. We obtained rIL-22 with a c-terminal 6× His tag soluble in the supernatant after IPTG-induced expression. A single band of approximately 18 kDa was observed on the SDS−PAGE gel after purification by the Ni-NTA column (Figure 3A). The protein concentration of rIL-22 was 0.25 mg/mL, as determined by a BCA kit. Primary cells from black rockfish spleen were cultured to verify the bioactivity of rIL-22. As shown in Figure 3C, the rIL-22-treated group did not differ from the control group, but rIL-22 significantly reduced LPS-induced il6 and ptgs2 levels when combined with LPS (P<0.05). These results indicated that rIL-22 was functionally active in vitro.
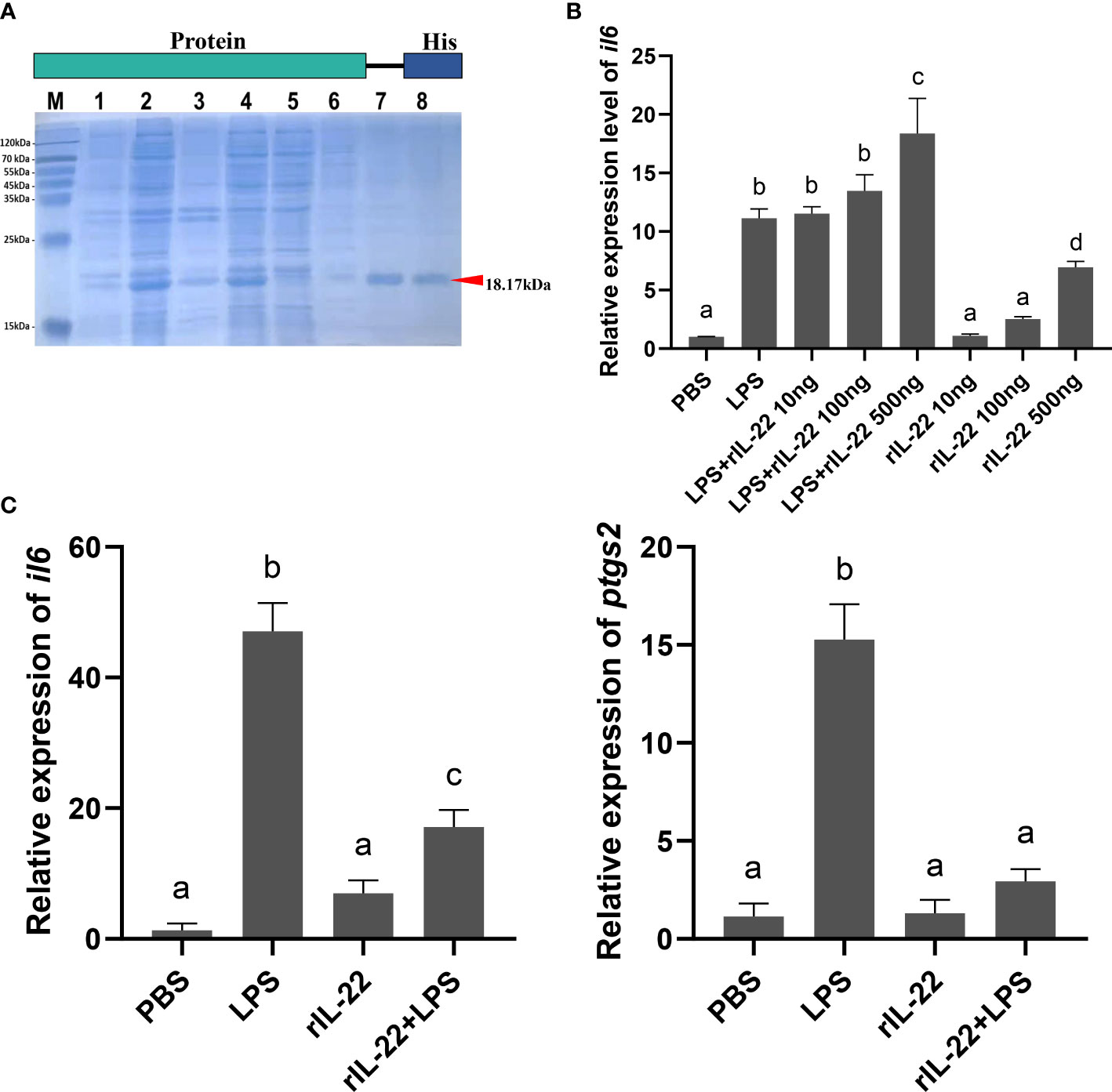
Figure 3 (A) SDS-PAGE analysis of purified rIL-22 (M: Marker; lane 1: Total protein in the thallus before induction; lane 2: Total protein in thallus after induction; lane 3: Protein in the precipitate after induction; lane 4: Protein in the supernatant after induction; lane 5, 6: The non-target proteins are washed down; lane 7, 8: Purified rIL-22 (18.17 kDa)). (B) Relative expression of il6 under PBS and different concentrate LPS and rIL-22 stimulation (n=3). (C) Relative expression of il6 and ptgs2 after PBS, LPS, rIL-22 and rIL-22 + LPS incubation, respectively (n=3). The X axis indicates different treatments. The Y axis indicates the relative expression normalized to 18s rRNA. All data are presented as the mean ± SD. Different letters indicate significant differences (P < 0.05).
To understand the role of IL-22 in ovarian cells during the perinatal period, we treated ovarian cells with different concentrations of rIL-22 with or without LPS. In contrast to the results in the spleen, the il6 level in ovarian cells was increased along with the rIL-22 concentration with or without LPS Figure 3B).
3.4 RNA-seq revealed the function of IL-22 in black rockfish ovaries
To verify the response mechanism of IL-22 in black rockfish ovaries during the perinatal period, nine groups of cDNA libraries were constructed and sequenced on the Illumina platform (Accession number: PRJNA876649). By high-throughput sequencing, 44,521,866, 46,205,972 and 48,020,019 average raw reads were obtained from the PBS, LPS, and IL-22 groups, respectively. After filtration, the average clean reads of the control and treatment groups used in subsequent analysis were 43,498,905, 45,496,249 and 47,222,700, with Q30 average percentages of 92.67, 91.93 and 91.76%, respectively. By mapping these clean reads with black rockfish genomes, the average total map of the PBS, LPS, and IL-22 groups was obtained as 40,352,149.33 (92.74%), 42,316,499 (93.01%), and 43,825,848 (92.80%), respectively.
The PCA showed significant differences among the PBS, LPS, and IL-22 groups (Figure 4A). We identified 760 significant DEGs in the LPS group compared to the PBS group, including 528 upregulated DEGs and 232 downregulated DEGs. Simultaneously, 168 significant DEGs were identified in the IL-22 group compared with the PBS group, including 143 upregulated DEGs and 25 downregulated DEGs. In addition, 66 significant DEGs were detected in the IL-22 group compared to the LPS group, including 51 upregulated DEGs and 15 downregulated DEGs (Figure 4B–D).
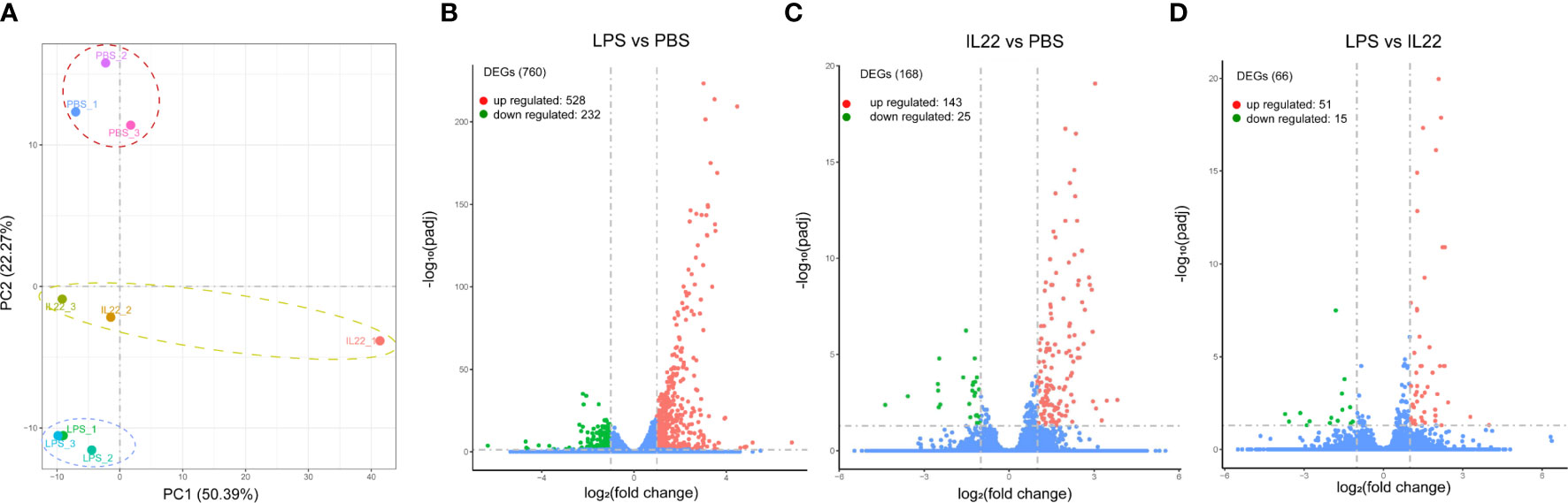
Figure 4 (A) PCA of the nine libraries treated with PBS (PBS_1, PBS_2, PBS_3), LPS (LPS_1, LPS_2, LPS_3), and IL-22 (IL22_1, IL22_2, IL22_3), respectively. Volcano plots represented the DEGs in LPS vs. PBS group (B), IL-22 vs. PBS group (C), and LPS vs. IL-22 group (D), respectively.
A total of 134 DEGs were clustered in the intersection between the LPS vs. PBS groups and IL-22 vs. PBS groups, which stood for the coactivated genes of both LPS and IL-22 (Figure 5A). The heatmap indicated that the expression patterns of these 134 DEGs were similar in the IL-22 and LPS groups (Figure 5B). Further annotation of these 134 DEGs is presented in Figures 5C, D. KEGG enrichment analysis identified 20 pathways, which were considered to be related to the following: immune response (13 pathways), PG synthesis (3 pathways), angiogenesis (2 pathways) and cell death (2 pathways) (Figure 5C). GO enrichment analysis showed that the top 10 GO terms in the Biological Process were classified into four categories: immune response (GO: 0006955, 0002376), fatty acid metabolism (GO: 0006633, 0006631, 0044255), stress response (GO: 0006979, 0006950), and monocarboxylic acid (GO: 0072330, 0032787) (Figure 5D). The PPI analysis revealed a complex network of interactions among 134 DEGs in which chemokines, cytokines and PG synthesis-related proteins were the main focus (Figure 6A). In addition, 34 DEGs were identified and responded only to IL-22 stimulation. There were particular interactions between the 34 DEGs through the PPI analysis (Figure 6B). They are mainly classified as immune-related and cytoskeleton reorganization-related. To verify the expression levels of genes in transcriptome data, 7 DEGs were randomly selected for qPCR. As shown in Figure 6C, the gene expression levels obtained by qPCR were basically consistent with the transcriptomic data.
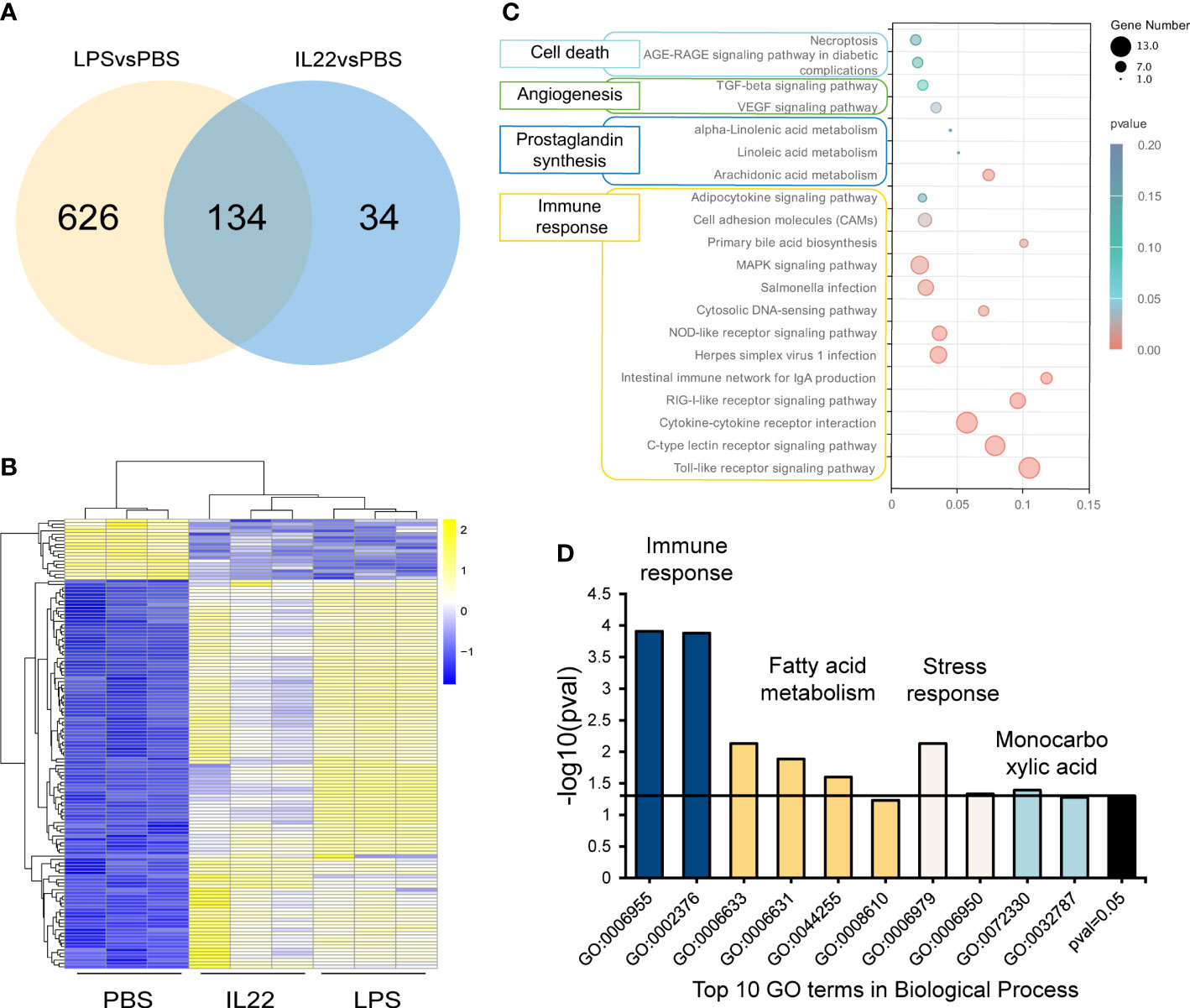
Figure 5 (A) Venn diagram of the DEGs of LPS vs PBS group and IL-22 vs PBS group. (B) Heatmap of 134 DEGs in the three groups (PBS, LPS, and IL-22). Yellow and blue colors indicated up- and down-regulated transcripts, respectively. (C) KEGG analysis of the 134 DEGs. Enriched pathways were classified into four classifications including Immune response, PG synthesis, Angiogenesis, and Cell death. (D) The top 10 GO terms of the 134 DEGs were classified into Immune response, Fatty acid metabolism, Stress response, and Monocarboxylic acid.
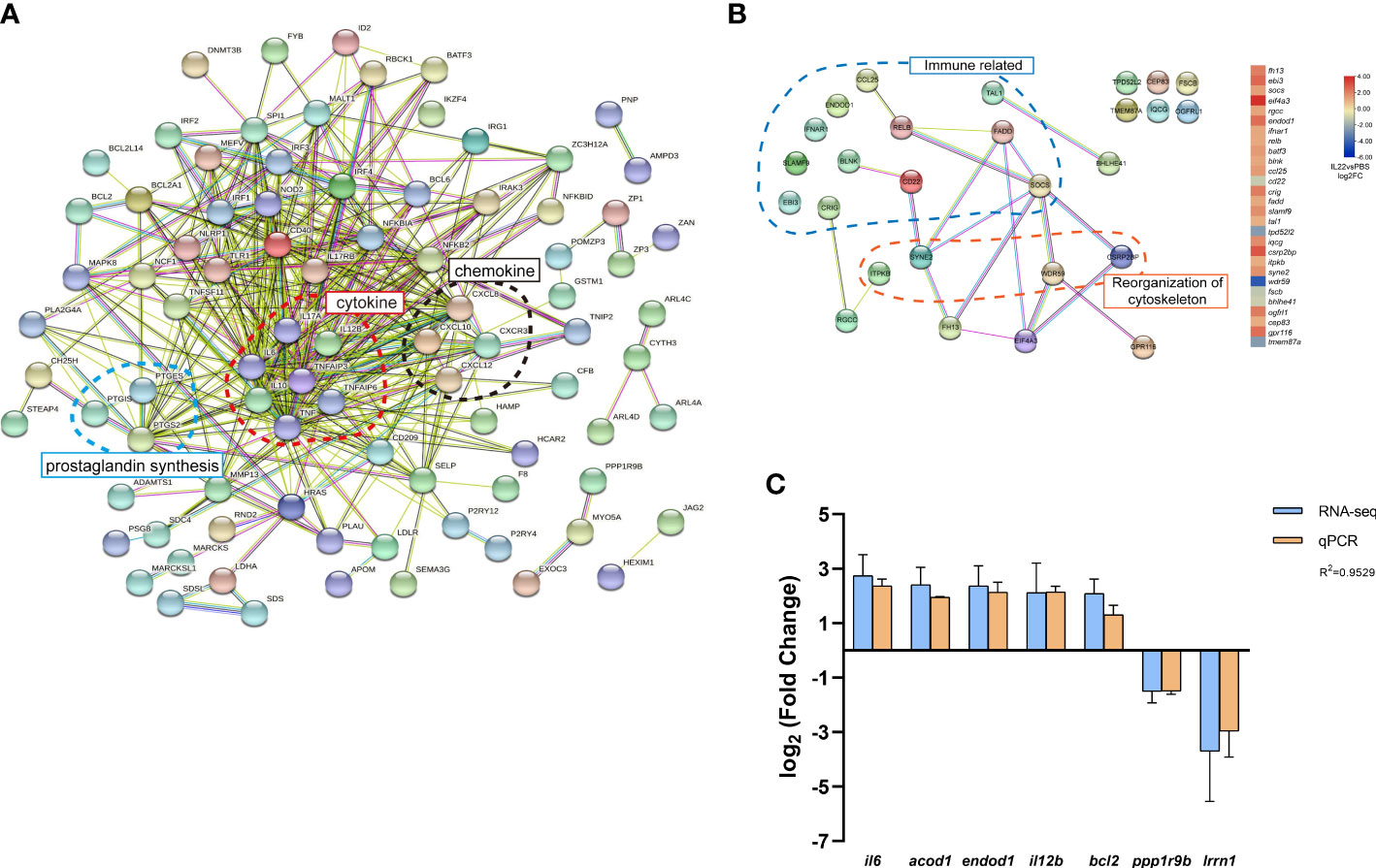
Figure 6 (A) The PPI analysis of the 134 DEGs enriched from the intersection of LPS vs. PBS group and IL-22 vs. PBS group. Red circle represented DEGs related to cytokine. Black circle represented DEGs related to chemokine. Blue circle represented DEGs related to prostaglandin synthesis. (B) The PPI analysis of the 34 DEGs only expressed in IL-22 vs. PBS group. Red circle represented DEGs related to reorganization of cytoskeleton. Blue circle represented DEGs related to immune related. (C) The qPCR validation of seven DEGs generated from the RNA-Seq results. These genes include il6, Cis-aconitate decarboxylase (acod1), endod1, il12b, B cell lymphoma 2 (bcl2), protein phosphatase 1 regulatory subunit 9B (ppp1r9b), and leucine rich repeat neuronal 1 (lrrn1).
4 Discussion
IL-22 is a member of the IL-10 cytokine family. Protein modeling revealed that the six α-helices in IL-22 were similar to those in other IL-10 family members (Wolk et al., 2010). The sequence alignment results showed that IL-22 of black rockfish had two adjacent disulfide bridges, which is consistent with a study in teleosts (Siupka et al., 2014). The phylogenetic trees showed that IL-22 congregated with teleosts and differed from mammals. IL-22 acts via the IL-22 receptor, which is a heterodimer consisting of IL-22RA1 and IL-10RB (Trevejo-Nunez et al., 2016). The IL-22 receptor complex is a transmembrane complex associated with JAK and TYK. It triggers a cascade of downstream reactions when IL-22 binds to its receptor complex, including phosphorylation of JAK and TYK2 and activation of STAT3 (Wu et al., 2020). In addition, other molecules include P38, ERK, JNK, and PI3K (Sabat et al., 2014). IL-22 also possesses the soluble receptor IL-22RA2, also known as IL-22BP, which can competitively combine with IL-22.
In humans, pregnancy is thought to be a state of immunosuppression, whereas delivery is a reactivation of local inflammation (Osman et al., 2003). Studies have shown that the volume of the uterus occupied by the embryo acts as a signal that is sensed by the uterus (Shynlova et al., 2013a). The plateau reached in embryonic growth may act as a signal to start parturition. In mice, the myometrium cells can sense mechanical stretching signals from the growing embryo (Shynlova et al., 2008). Meanwhile, it has been reported that mechanical stretching can stimulate uterine chemokine expression, such as CCL2 (Shynlova et al., 2008), C-X-C motif chemokine 1 (CXCL1) (Hilscher et al., 2019), CXCL5 (Bollapragada et al., 2009), and CXCL8 (Lei et al., 2011), which aggregate immune cells. The immune cells that accumulate include T cells (Hamilton et al., 2012), neutrophils and macrophages (Gimeno-Molina et al., 2022). These immune cells are recruited from the peripheral circulation to cause decidual infiltration (Shynlova et al., 2013b). The large accumulation of immune cells at the maternal-fetal interface in turn produces extracellular matrix (ECM) degradation proteases such as MMP to promote cervical relaxation (Kelly, 2002), and are also a source of cytokines such as IL-22, IL-1β, IL-6 and TNF-α. IL-22 promotes chemokine and cytokine production. IL-1β could promote prostaglandin E2 (PGE2) synthesis in uterine tissues harvested from pigs (Franczak et al., 2010). IL-6 and TNF-α upregulate the expression of ptgs2 in different cell types (Honda, 2011; McHale et al., 2018; Koyama et al., 2021). PGE2 is an inducer of parturition due to its increased levels in the uterus during parturition and its ability to cause strong uterine contractions (Sugimoto et al., 2015). During the complex process of parturition, inflammatory cytokines play a very important role.
The il22 level was significantly increased during the parturition of black rockfish, suggesting that IL-22 plays an important role in parturition. According to the transcriptomic results, 134 DEGs involved in chemokine, cytokine, and PG synthesis were identified in both the IL-22 and LPS groups. A similar expression pattern of these DEGs was also found in the transcriptomic data of the human myometrium (Mittal et al., 2010). These results indicated a conserved mechanism in parturition between mammals and ovoviviparous fish. In our results, IL-22 induced the production of chemokine-related genes such as CXCL10/CXCR3, CXCL12 and CXCL8 in the ovary at parturition. In human preterm parturition, the CXCL10/CXCR3 system can recruit T lymphocytes to cause placental infiltration (Romero et al., 2017). CXCL12 is widely present in placental cells and is considered a key component of fetal outcome (Ao et al., 2020). Higher levels of CXCL8 have been reported to be associated with preterm parturition (Rode et al., 2012). These chemokines recruit immune cells to the maternal-fetal interface to amplify inflammatory signaling cascades.
IL-6 and TNF-α are considered to be involved in parturition through the PG signaling pathway (Keelan et al., 2003). In mice, IL-6 promotes the expression of genes that control PG synthesis and signaling in isolated uterine cells (Robertson et al., 2010). Meanwhile, TNF-α promotes the production of ptgs2, which leads to the upregulation of prostaglandin F2a (PGF2a) in bovine endometrial stromal cells (Sakai et al., 2021). In our results, proinflammatory cytokines (IL-6, TNF-α and IL-17) and PG synthesis-related genes (ptgs2, ptgis, and ptges) were upregulated, which is consistent with the results in mammals. The fertilization process of black rockfish occurs in situ, and the parturition process is similar to ovulation in oviparous teleosts. Studies on oviparity ovulation have proven that PGs can activate ovulation in medaka (Oryzias latipes) (Fujimori et al., 2011), pacu (Piaractus mesopotamicus) (Criscuolo-Urbinati et al., 2012), goldfish (Carassius auratus) (Sorensen et al., 2018), catfish (Heteropneustes fossilis) (Joy and Singh, 2013), and longchin goby (Chasmichthys dolichognathus) (Baek and Lee, 2019). In ovoviviparous guppies (Poecilia reticulata), intraperitoneal administration of PGF2a significantly induces parturition (Lyu et al., 2021). In a previous study on black rockfish, ptgs2, a key enzyme in PG synthesis, was also proven to be related to parturition (Lyu et al., 2022). Taken together, IL-22 was involved in parturition by promoting PG synthesis in black rockfish. Meanwhile, rIL-22 significantly upregulated the expression of ptgs2 and il6 in the spleen and ovary. The above processes of parturition induced by IL-22 appear to be similar to those induced by pathogenic bacterial infections.
In addition, our transcriptomic data showed that 34 DEGs were mainly enriched in immune-related and cytoskeleton reorganization-related genes that respond to IL-22 stimulation but not LPS. The immune-related genes included fas associated via death domain (fadd), transcription factor RelB (relb), endonuclease domain containing 1 (endod1), and suppressor of cytokine signaling 1 (socs1), etc. As a ubiquitous adaptor protein, FADD, regulates cell apoptosis and autophagy to maintain homeostasis. Meanwhile, FADD inhibits typical cell death, such as necroptosis, which can trigger inflammatory responses (Mouasni and Tourneur, 2018). Therefore, we speculated that FADD may maintain ovarian homeostasis by regulating cell death. RELB can activate NF-κB through both classical and nonclassical pathways as a key molecule (Madge and May, 2011). Our results showed that RELB was upregulated, suggesting that IL-22 could activate NF-κB through a nonclassical pathway in black rockfish parturition. Studies have shown that RELB can limit acute inflammation and repress innate immunity by blocking or replacing RELA in the classical pathway (Millet et al., 2013). Therefore, we hypothesized that IL-22 may play a limiting role in preventing the expansion of inflammation during the local inflammatory reaction of black rockfish. Our results above showed that inflammation in black rockfish parturition is similar to the inflammation caused by recognition of pathogen-associated molecular patterns (PAMPs). However, ENDOD1 can participate in the degradation of neutrophil extracellular traps (NETs) after pathogen clearance to protect the host from autoimmune damage (Lyu et al., 2016). In addition, SOCS1 is a representative negative regulator of JAK/STAT-mediated cytokine signaling and strictly regulates pathways related to inflammation (Yoshimura et al., 2021). These results suggested that IL-22 may protect the ovary from autoimmune damage and play a limiting role in inflammatory pathways during parturition in black rockfish. The reorganization of cytoskeletal genes, including inositol-trisphosphate 3-kinase B (itpkb), WD repeat domain 59 (wdr59), and spectrin repeat containing nuclear envelope protein 2 (syne2), was also enriched in the IL-22 vs. PBS groups. Studies have shown that the F-actin binding domain of the ITPKB isoenzyme exhibits the ability to reorganize cytoskeletal networks to influence cell movement and migration (Erneux et al., 2016). An important cause of parturition events is ovary contraction due to cytoskeletal remodeling and motility (Taggart and Morgan, 2007). In our results, the wdr59 gene was significantly downregulated. WDR59 is a component of the GATOR2 protein complex that can indirectly promote mammalian target of rapamycin C1 (mTORC1) activity (Kim et al., 2015b). Activation of mTORC1 can promote cell motility and invasion (Zhou and Huang, 2011). The mechanical connection between the nucleus and the cytoskeleton is provided by nesprins, including syne2 (also called nesprin2). SYNE2 can bind to the cytoskeleton and work with actin to drive cellular nuclei and movement (Davidson et al., 2020). Fertilization and embryo development of black rockfish was processed inside the ovary. It is also indicated that the process of parturition is similar to oviparity ovulation. The process of parturition involves follicular layer and egg envelope rupture, which are all associated with cytoskeletal remodeling. In our results, DEGs regulated by IL-22 were associated with cytoskeletal remodeling in ovarian cells, suggesting that IL-22 may function in ovarian cell migration or motility during parturition in black rockfish.
IL-22 activates different important pathways in black rockfish parturition. The transcriptomic results included pathways related to the immune response, PG synthesis, angiogenesis and cell death. The activation of the immune response plays an important role during parturition. The Toll-like receptor (TLR) signaling pathway regulates the downstream inflammatory response by activating the transcription factors NF-κB and JNK through the MyD88 adaptor (Takeda and Akira, 2004). It has been shown that TLR4 expression is significantly increased in human pregnancy tissues during preterm and term parturition (Chen et al., 2020). In this study, TLR signaling pathway-related genes were upregulated, similar to research results seen in humans. The TLR signaling pathway may play a role in the activation of inflammatory cytokines in the ovarian stroma during black rockfish parturition. The cytokine−cytokine receptor interaction was also significantly upregulated, which was expected. C-type lectin receptors (CLRs) can trigger a variety of signaling pathways that broadly lead to the activation or inhibition of cellular functions. CLRs can induce a large number of cytokines and chemokines. In general, CLRs induce a proinflammatory response, which can directly or indirectly induce the production of IL-1β, IL-6, TNF and leukotrienes (LTs) (Brown et al., 2018). The CLRs may be involved in the production of cytokines in the parturient period of black rockfish. PG is a key factor in parturition. Correspondingly, PG synthesis was significantly enriched in our results. The results included linoleic acid metabolism and arachidonic acid metabolism. Arachidonic acid can form PGE2 in the presence of ptgs2 (Wang et al., 2019). However, linoleic acid is an upstream provider that generates arachidonic acid (Szczuko et al., 2020). Research has shown that both full-term spontaneous parturition and preterm parturition lead to a high expression of genes related to angiogenesis (Haddad et al., 2008). Similarly, vascular endothelial growth factor (VEGF) signaling pathway-related genes were significantly upregulated in our results. In human research, VEGF not only plays an important role in decidua growth and maintenance but also may stimulate leukocyte extravasation into the decidua and promote decidua inflammation (Christiaens et al., 2008). The transforming growth factor beta (TGF-β) signaling pathway is a multifunctional cellular pathway. TGF-β in bovine placenta may play an important role in fetal membrane dissection after parturition through cell inhibitory activity and ECM remodeling (Hirayama et al., 2015). Because black rockfish expel their larvae directly at parturient time, TGF-β may play a potential role in the process of the larvae leaving the ovary. Intracellular components released by cell death are one of the stimuli that trigger the proinflammatory gene expression program in innate immune cells (Pasparakis and Vandenabeele, 2015). In our results, cell death-related pathways such as the necroptosis pathway were significantly enriched.
In summary, we propose that IL-22 plays an important role in the parturition of black rockfish by upregulating PG synthesis and promoting parturition-related inflammatory signals. On the one hand, chemokines (CXCL10/CXCR3, CXCL12, and CXCL8) and proinflammatory cytokines (IL-6, TNF-α, and IL-17) caused the destruction of the immune tolerance balance in the ovary and further increased PG synthesis. On the other hand, IL-22 also activated PG synthesis directly by regulating related PG synthetase levels. In addition, IL-22 may play a protective role by maintaining ovarian homeostasis and limiting inflammatory amplification and cytoskeletal remodeling.
Data availability statement
The datasets presented in this study can be found in online repositories. The names of the repository/repositories and accession number(s) can be found below: BioProject, PRJNA876649.
Ethics statement
The animal study was reviewed and approved by Institutional Animal Care and Use Committee of Ocean University of China.
Author contributions
HW, XQ and YL designed the study. SY performed the experiment. ZW provided all the fish for sampling. SY, LL, XW, JL, YY, CZ and SX performed in samples collection. SY wrote the manuscript, XQ and LL provided manuscript editing and feedback. All authors contributed to the article and approved the submitted version.
Funding
This study was supported by grants from The National Natural Science Foundation of China (41976089).
Acknowledgments
We thank ZW for providing us with all the fish for sampling.
Conflict of interest
Author ZW was employed by the company Weihai Taifeng Seawater Seedling Co.
The remaining authors declare that the research was conducted in the absence of any commercial or financial relationships that could be construed as a potential conflict of interest.
Publisher’s note
All claims expressed in this article are solely those of the authors and do not necessarily represent those of their affiliated organizations, or those of the publisher, the editors and the reviewers. Any product that may be evaluated in this article, or claim that may be made by its manufacturer, is not guaranteed or endorsed by the publisher.
Supplementary material
The Supplementary Material for this article can be found online at: https://www.frontiersin.org/articles/10.3389/fmars.2022.1041681/full#supplementary-material
Supplementary 1 | Details of the prokaryotic expression vector.
Supplementary 2 | The GenBank accession numbers and sequences of IL-22, IL-22RA1 and IL-22RA2 screened by the phylogenetic tree.
References
Abu-Raya B., Michalski C., Sadarangani M., Lavoie P. M. (2020). Maternal Immunological Adaptation During Normal Pregnancy. Clin. Transl. Immunol. 11, 575197. doi: 10.3389/fimmu.2020.575197
Alabbas S. Y., Begun J., Florin T. H., Oancea I. (2018). The role of IL-22 in the resolution of sterile and nonsterile inflammation. Clin. Transl. Immunol. 7 (4), e1017. doi: 10.1002/cti2.1017
Anders S., Pyl P. T., Huber W. (2015). HTSeq–a Python framework to work with high-throughput sequencing data. Bioinformatics 31 (2), 166–169. doi: 10.1093/bioinformatics/btu638
Andoh A., Zhang Z., Inatomi O., Fujino S., Deguchi Y., Araki Y., et al. (2005). Interleukin-22, a member of the IL-10 subfamily, induces inflammatory responses in colonic subepithelial myofibroblasts. Gastroenterology 129 (3), 969–984. doi: 10.1053/j.gastro.2005.06.071
Ao D., Li D. J., Li M. Q. (2020). CXCL12 in normal and pathological pregnancies: A review. Am. J. Reprod. Immunol. 84 (3), e13280. doi: 10.1111/aji.13280
Baek H. J., Lee D. S. (2019). Prostaglandin affects in vitro ovulation and 17α, 20β-dihydroxy- 4-pregnen-3-one production in longchin goby, Chasmichthys dolichognathus oocytes. Dev. Reprod. 23 (2), 111–117. doi: 10.12717/dr.2019.23.2.111
Bollapragada S., Youssef R., Jordan F., Greer I., Norman J., Nelson S. (2009). Term labor is associated with a core inflammatory response in human fetal membranes, myometrium, and cervix. Am. J. Obstet. Gynecol. 200 (1), 104.e101–111. doi: 10.1016/j.ajog.2008.08.032
Brown G. D., Willment J. A., Whitehead L. (2018). C-type lectins in immunity and homeostasis. Nat. Rev. Immunol. 18 (6), 374–389. doi: 10.1038/s41577-018-0004-8
Buonocore F., Forlenza M., Randelli E., Benedetti S., Bossù P., Meloni S., et al. (2005). Biological activity of sea bass (Dicentrarchus labrax l.) recombinant interleukin-1beta. Mar. Biotechnol. (NY) 7 (6), 609–617. doi: 10.1007/s10126-004-5131-5
Chatterjee A., Guchhait R., Maity S., Mukherjee D., Pramanick K. (2020). Functions of interleukin-6 in ovulation of female climbing perch, Anabas testudineus. Anim. Reprod. Sci. 219, 106528. doi: 10.1016/j.anireprosci.2020.106528
Chen Z., Liu Q., Zhu Z., Xiang F., Wu R., Kang X. (2020). Toll-like receptor 4 contributes to uterine activation by upregulating pro-inflammatory cytokine and CAP expression via the NF-κB/P38MAPK signaling pathway during pregnancy. J. Cell Physiol. 235 (1), 513–525. doi: 10.1002/jcp.28991
Christiaens I., Zaragoza D. B., Guilbert L., Robertson S. A., Mitchell B. F., Olson D. M. (2008). Inflammatory processes in preterm and term parturition. J. Reprod. Immunol. 79 (1), 50–57. doi: 10.1016/j.jri.2008.04.002
Conde-Agudelo A., Romero R. (2014). Prediction of preterm birth in twin gestations using biophysical and biochemical tests. Am. J. Obstet. Gynecol. 211 (6), 583–595. doi: 10.1016/j.ajog.2014.07.047
Criscuolo-Urbinati E., Kuradomi R. Y., Urbinati E. C., Batlouni S. R. (2012). The administration of exogenous prostaglandin may improve ovulation in pacu (Piaractus mesopotamicus). Theriogenology 78 (9), 2087–2094. doi: 10.1016/j.theriogenology.2012.08.001
Dambaeva S., Schneiderman S., Jaiswal M. K., Agrawal V., Katara G. K., Gilman-Sachs A., et al. (2018). Interleukin 22 prevents lipopolysaccharide- induced preterm labor in mice. Biol. Reprod. 98 (3), 299–308. doi: 10.1093/biolre/iox182
Davidson P. M., Battistella A., Déjardin T., Betz T., Plastino J., Borghi N., et al. (2020). Nesprin-2 accumulates at the front of the nucleus during confined cell migration. EMBO Rep. 21 (7), e49910. doi: 10.15252/embr.201949910
Dudakov J. A., Hanash A. M., van den Brink M. R. (2015). Interleukin-22: immunobiology and pathology. Annu. Rev. Immunol. 33, 747–785. doi: 10.1146/annurev-immunol-032414-112123
Erneux C., Ghosh S., Koenig S. (2016). Inositol(1,4,5)P3 3-kinase isoenzymes: Catalytic properties and importance of targeting to f-actin to understand function. Adv. Biol. Regul. 60, 135–143. doi: 10.1016/j.jbior.2015.09.004
Franczak A., Zmijewska A., Kurowicka B., Wojciechowicz B., Kotwica G. (2010). Interleukin 1β-induced synthesis and secretion of prostaglandin E2 in the porcine uterus during various periods of pregnancy and the estrous cycle. J. Physiol. Pharmacol. 61 (6), 733–742.
Fujimori C., Ogiwara K., Hagiwara A., Rajapakse S., Kimura A., Takahashi T. (2011). Expression of cyclooxygenase-2 and prostaglandin receptor EP4b mRNA in the ovary of the medaka fish, Oryzias latipes: possible involvement in ovulation. Mol. Cell Endocrinol. 332 (1-2), 67–77. doi: 10.1016/j.mce.2010.09.015
Gershater M., Romero R., Arenas-Hernandez M., Galaz J., Motomura K., Tao L., et al. (2022). IL-22 plays a dual role in the amniotic cavity: Tissue injury and host defense against microbes in preterm labor. J. Immunol. 208 (7), 1595–1615. doi: 10.4049/jimmunol.2100439
Gimeno-Molina B., Muller I., Kropf P., Sykes L. (2022). The role of neutrophils in pregnancy, term and preterm labour. Life (Basel) 12 (10), 1512. doi: 10.3390/life12101512
Götz S., García-Gómez J. M., Terol J., Williams T. D., Nagaraj S. H., Nueda M. J., et al. (2008). High-throughput functional annotation and data mining with the Blast2GO suite. Nucleic Acids Res. 36 (10), 3420–3435. doi: 10.1093/nar/gkn176
Haddad R., Romero R., Gould B. R., Tromp G., Gotsch F., Edwin S. S., et al. (2008). Angiogenesis gene expression in mouse uterus during the common pathway of parturition. Am. J. Obstet. Gynecol. 198 (5), 539.e531–538. doi: 10.1016/j.ajog.2007.11.021
Hadley E. E., Richardson L. S., Torloni M. R., Menon R. (2018). Gestational tissue inflammatory biomarkers at term labor: A systematic review of literature. Am. J. Reprod. Immunol. 79 (2), 10. doi: 10.1111/aji.12776
Hamilton S., Oomomian Y., Stephen G., Shynlova O., Tower C. L., Garrod A., et al. (2012). Macrophages infiltrate the human and rat decidua during term and preterm labor: Evidence that decidual inflammation precedes labor. Biol. Reprod. 86 (2), 39. doi: 10.1095/biolreprod.111.095505
Heidari Z., Moudi B., Sheibak N., Asemi-Rad A., Keikha N., Mahmoudzadeh-Sagheb H., et al. (2021). Interleukin 22 expression during the implantation window in the endometrium of women with unexplained recurrent pregnancy loss and unexplained infertility compared to healthy parturient individuals. J. Interferon Cytokine Res. 41 (12), 461–468. doi: 10.1089/jir.2021.0160
Hilscher M. B., Sehrawat T., Arab J. P., Zeng Z., Gao J., Liu M., et al. (2019). Mechanical stretch increases expression of CXCL1 in liver sinusoidal endothelial cells to recruit neutrophils, generate sinusoidal microthombi, and promote portal hypertension. Gastroenterology 157 (1), 193–209.e199. doi: 10.1053/j.gastro.2019.03.013
Hirayama H., Koyama K., Sawai K., Fujii T., Naito A., Fukuda S., et al. (2015). Localization of TGF-β and TGF-β receptor in bovine term placentome and expression differences between spontaneous and induced parturition. Placenta 36 (11), 1239–1245. doi: 10.1016/j.placenta.2015.09.003
Honda K. (2011). Interleukin-6 and soluble interleukin-6 receptor suppress osteoclastic differentiation by inducing PGE(2) production in chondrocytes. J. Oral. Sci. 53 (1), 87–96. doi: 10.2334/josnusd.53.87
Joy K. P., Singh V. (2013). Functional interactions between vasotocin and prostaglandins during final oocyte maturation and ovulation in the catfish Heteropneustes fossilis. Gen. Comp. Endocrinol. 186, 126–135. doi: 10.1016/j.ygcen.2013.02.043
Kanehisa M., Furumichi M., Tanabe M., Sato Y., Morishima K. (2017). KEGG: new perspectives on genomes, pathways, diseases and drugs. Nucleic Acids Res. 45 (D1), D353–d361. doi: 10.1093/nar/gkw1092
Keelan J. A., Blumenstein M., Helliwell R. J., Sato T. A., Marvin K. W., Mitchell M. D. (2003). Cytokines, prostaglandins and parturition–a review. Placenta 24 (Suppl A), S33–S46. doi: 10.1053/plac.2002.0948
Kelly R. W. (2002). Inflammatory mediators and cervical ripening. J. Reprod. Immunol. 57 (1-2), 217–224. doi: 10.1016/s0165-0378(02)00007-4
Kim D., Langmead B., Salzberg S. L. (2015a). HISAT: a fast spliced aligner with low memory requirements. Nat. Methods 12 (4), 357–360. doi: 10.1038/nmeth.3317
Kim J. S., Ro S. H., Kim M., Park H. W., Semple I. A., Park H., et al. (2015b). Sestrin2 inhibits mTORC1 through modulation of GATOR complexes. Sci. Rep. 5, 9502. doi: 10.1038/srep09502
Kong X., Feng D., Wang H., Hong F., Bertola A., Wang F. S., et al. (2012). Interleukin-22 induces hepatic stellate cell senescence and restricts liver fibrosis in mice. Hepatology 56 (3), 1150–1159. doi: 10.1002/hep.25744
Koyama T., Uchida K., Fukushima K., Ohashi Y., Uchiyama K., Inoue G., et al. (2021). Elevated levels of TNF-α, IL-1β and IL-6 in the synovial tissue of patients with labral tear: a comparative study with hip osteoarthritis. BMC Musculoskelet. Disord. 22 (1), 33. doi: 10.1186/s12891-020-03888-w
Lei K., Chen L., Cryar B. J., Hua R., Sooranna S. R., Brosens J. J., et al. (2011). Uterine stretch and progesterone action. J. Clin. Endocrinol. Metab. 96 (6), E1013–E1024. doi: 10.1210/jc.2010-2310
Liman M., Wenji W., Conghui L., Haiyang Y., Zhigang W., Xubo W., et al. (2013). Selection of reference genes for reverse transcription quantitative real-time PCR normalization in black rockfish (Sebastes schlegeli). Mar. Genomics 11, 67–73. doi: 10.1016/j.margen.2013.08.002
Liu D. T., Brewer M. S., Chen S., Hong W., Zhu Y. (2017). Transcriptomic signatures for ovulation in vertebrates. Gen. Comp. Endocrinol. 247, 74–86. doi: 10.1016/j.ygcen.2017.01.019
Liu Q., Wang X., Xiao Y., Zhao H., Xu S., Wang Y., et al. (2019). Sequencing of the black rockfish chromosomal genome provides insight into sperm storage in the female ovary. DNA Res. 26 (6), 453–464. doi: 10.1093/dnares/dsz023
Lu D. Q., Bei J. X., Feng L. N., Zhang Y., Liu X. C., Wang L., et al. (2008). Interleukin-1beta gene in orange-spotted grouper, Epinephelus coioides: molecular cloning, expression, biological activities and signal transduction. Mol. Immunol. 45 (4), 857–867. doi: 10.1016/j.molimm.2007.08.009
Lyu L. K., Li J. S., Wang X. J., Yao Y. J., Li J. F., Li Y., et al. (2021). Arg-vasotocin directly activates isotocin receptors and induces COX2 expression in ovoviviparous guppies. Front. Endocrinol. (Lausanne) 12. doi: 10.3389/fendo.2021.617580
Lyu L., Wang R., Wen H., Li Y., Li J., Wang X., et al. (2022). Cyclooxygenases of ovoviviparous black rockfish (Sebastes schlegelii): Cloning, tissue distribution and potential role in mating and parturition. Comp. Biochem. Physiol. B Biochem. Mol. Biol. 257, 110677. doi: 10.1016/j.cbpb.2021.110677
Lyu Z. Z., Zhao B. B., Koiwai K., Hirono I., Kondo H. (2016). Identification of endonuclease domain-containing 1 gene in Japanese flounder Paralichthys olivaceus. Fish Shellfish Immunol. 50, 43–49. doi: 10.1016/j.fsi.2016.01.017
Madge L. A., May M. J. (2011). The NFκB paradox: RelB induces and inhibits gene expression. Cell Cycle 10 (1), 6–7. doi: 10.4161/cc.10.1.14291
Mazaki-Tovi S., Romero R., Kusanovic J. P., Erez O., Pineles B. L., Gotsch F., et al. (2007). Recurrent preterm birth. Semin. Perinatol. 31 (3), 142–158. doi: 10.1053/j.semperi.2007.04.001
McHale C., Mohammed Z., Deppen J., Gomez G. (2018). Interleukin-6 potentiates FcϵRI-induced PGD(2) biosynthesis and induces VEGF from human in situ-matured skin mast cells. Biochim. Biophys. Acta Gen. Subj. 1862 (5), 1069–1078. doi: 10.1016/j.bbagen.2018.01.020
Mei J., Zhou W. J., Li S. Y., Li M. Q., Sun H. X. (2019). Interleukin-22 secreted by ectopic endometrial stromal cells and natural killer cells promotes the recruitment of macrophages through promoting CCL2 secretion. Am. J. Reprod. Immunol. 82 (4), e13166. doi: 10.1111/aji.13166
Millet P., McCall C., Yoza B. (2013). RelB: an outlier in leukocyte biology. J. Leukoc. Biol. 94 (5), 941–951. doi: 10.1189/jlb.0513305
Mittal P., Romero R., Tarca A. L., Gonzalez J., Draghici S., Xu Y., et al. (2010). Characterization of the myometrial transcriptome and biological pathways of spontaneous human labor at term. J. Perinat Med. 38 (6), 617–643. doi: 10.1515/jpm.2010.097
Mouasni S., Tourneur L. (2018). FADD at the crossroads between cancer and inflammation. Trends Immunol. 39 (12), 1036–1053. doi: 10.1016/j.it.2018.10.005
Osman I., Young A., Ledingham M. A., Thomson A. J., Jordan F., Greer I. A., et al. (2003). Leukocyte density and pro-inflammatory cytokine expression in human fetal membranes, decidua, cervix and myometrium before and during labour at term. Mol. Hum. Reprod. 9 (1), 41–45. doi: 10.1093/molehr/gag001
Pasparakis M., Vandenabeele P. (2015). Necroptosis and its role in inflammation. Nature 517 (7534), 311–320. doi: 10.1038/nature14191
Paulesu L., Romagnoli R., Bigliardi E. (2005). Materno-fetal immunotolerance: is interleukin-1 a fundamental mediator in placental viviparity? Dev. Comp. Immunol. 29 (5), 409–415. doi: 10.1016/j.dci.2004.09.007
Pertea M., Kim D., Pertea G. M., Leek J. T., Salzberg S. L. (2016). Transcript-level expression analysis of RNA-seq experiments with HISAT, StringTie and ballgown. Nat. Protoc. 11 (9), 1650–1667. doi: 10.1038/nprot.2016.095
Raghupathy R., Kalinka J. (2008). Cytokine imbalance in pregnancy complications and its modulation. Front. Biosci. 13, 985–994. doi: 10.2741/2737
Robertson S. A., Christiaens I., Dorian C. L., Zaragoza D. B., Care A. S., Banks A. M., et al. (2010). Interleukin-6 is an essential determinant of on-time parturition in the mouse. Endocrinology 151 (8), 3996–4006. doi: 10.1210/en.2010-0063
Rode L., Klein K., Larsen H., Holmskov A., Andreasen K. R., Uldbjerg N., et al. (2012). Cytokines and the risk of preterm delivery in twin pregnancies. Obstet. Gynecol. 120 (1), 60–68. doi: 10.1097/AOG.0b013e31825bc3cd
Romero R., Chaemsaithong P., Chaiyasit N., Docheva N., Dong Z., Kim C. J., et al. (2017). CXCL10 and IL-6: Markers of two different forms of intra-amniotic inflammation in preterm labor. Am. J. Reprod. Immunol. 78 (1), e12685. doi: 10.1111/aji.12685
Sabat R., Ouyang W., Wolk K. (2014). Therapeutic opportunities of the IL-22-IL-22R1 system. Nat. Rev. Drug Discovery 13 (1), 21–38. doi: 10.1038/nrd4176
Sakai S., Yagi M., Fujime N., Kuse M., Sakumoto R., Yamamoto Y., et al. (2021). Heat stress influences the attenuation of prostaglandin synthesis by interferon tau in bovine endometrial cells. Theriogenology 165, 52–58. doi: 10.1016/j.theriogenology.2021.02.005
Shynlova O., Lee Y. H., Srikhajon K., Lye S. J. (2013a). Physiologic uterine inflammation and labor onset: integration of endocrine and mechanical signals. Reprod. Sci. 20 (2), 154–167. doi: 10.1177/1933719112446084
Shynlova O., Nedd-Roderique T., Li Y., Dorogin A., Nguyen T., Lye S. J. (2013b). Infiltration of myeloid cells into decidua is a critical early event in the labour cascade and post-partum uterine remodelling. J. Cell Mol. Med. 17 (2), 311–324. doi: 10.1111/jcmm.12012
Shynlova O., Tsui P., Dorogin A., Lye S. J. (2008). Monocyte chemoattractant protein-1 (CCL-2) integrates mechanical and endocrine signals that mediate term and preterm labor. J. Immunol. 181 (2), 1470–1479. doi: 10.4049/jimmunol.181.2.1470
Siupka P., Hamming O. J., Frétaud M., Luftalla G., Levraud J. P., Hartmann R. (2014). The crystal structure of zebrafish IL-22 reveals an evolutionary, conserved structure highly similar to that of human IL-22. Genes Immun. 15 (5), 293–302. doi: 10.1038/gene.2014.18
Sorensen P. W., Appelt C., Stacey N. E., Goetz F. W., Brash A. R. (2018). High levels of circulating prostaglandin F(2α) associated with ovulation stimulate female sexual receptivity and spawning behavior in the goldfish (Carassius auratus). Gen. Comp. Endocrinol. 267, 128–136. doi: 10.1016/j.ygcen.2018.06.014
Sugimoto Y., Inazumi T., Tsuchiya S. (2015). Roles of prostaglandin receptors in female reproduction. J. Biochem. 157 (2), 73–80. doi: 10.1093/jb/mvu081
Szczuko M., Kikut J., Komorniak N., Bilicki J., Celewicz Z., Ziętek M. (2020). The role of arachidonic and linoleic acid derivatives in pathological pregnancies and the human reproduction process. Int. J. Mol. Sci. 21 (24), 9628. doi: 10.3390/ijms21249628
Taggart M. J., Morgan K. G. (2007). Regulation of the uterine contractile apparatus and cytoskeleton. Semin. Cell Dev. Biol. 18 (3), 296–304. doi: 10.1016/j.semcdb.2007.05.006
Takeda K., Akira S. (2004). TLR signaling pathways. Semin. Immunol. 16 (1), 3–9. doi: 10.1016/j.smim.2003.10.003
Tamura K., Stecher G., Kumar S. (2021). MEGA11: Molecular evolutionary genetics analysis version 11. Mol. Biol. Evol. 38 (7), 3022–3027. doi: 10.1093/molbev/msab120
Trevejo-Nunez G., Elsegeiny W., Conboy P., Chen K., Kolls J. K. (2016). Critical role of IL-22/IL22-RA1 signaling in pneumococcal pneumonia. J. Immunol. 197 (5), 1877–1883. doi: 10.4049/jimmunol.1600528
Venkatesh B., Tan C. H., Lam T. J. (1992). Prostaglandin synthesis in vitro by ovarian follicles and extrafollicular tissue of the viviparous guppy (Poecilia reticulata) and its regulation. J. Exp. Zool. 262, 405–413. doi: 10.1002/jez.1402620406
Wang T., Fu X., Chen Q., Patra J. K., Wang D., Wang Z., et al. (2019). Arachidonic acid metabolism and kidney inflammation. Int. J. Mol. Sci. 20 (15), 3683. doi: 10.3390/ijms20153683
Wang X., Meng W., Qi X., Li Y., Li J., Lyu L., et al. (2022). Molecular characterization and expression patterns of glucocorticoid receptors in the viviparous black rockfish Sebastes schlegelii. Gen. Comp. Endocrinol. 316, 113947. doi: 10.1016/j.ygcen.2021.113947
Wang X., Wen H., Li Y., Lyu L., Song M., Zhang Y., et al. (2021). Characterization of CYP11A1 and its potential role in sex asynchronous gonadal development of viviparous black rockfish Sebastes schlegelii (Sebastidae). Gen. Comp. Endocrinol. 302, 113689. doi: 10.1016/j.ygcen.2020.113689
Wang Y., Xu B., Li M. Q., Li D. J., Jin L. P. (2013). IL-22 secreted by decidual stromal cells and NK cells promotes the survival of human trophoblasts. Int. J. Clin. Exp. Pathol. 6 (9), 1781–1790.
Wolk K., Witte E., Witte K., Warszawska K., Sabat R. (2010). Biology of interleukin-22. Semin. Immunopathol. 32 (1), 17–31. doi: 10.1007/s00281-009-0188-x
Wu Y., Min J., Ge C., Shu J., Tian D., Yuan Y., et al. (2020). Interleukin 22 in liver injury, inflammation and cancer. Int. J. Biol. Sci. 16 (13), 2405–2413. doi: 10.7150/ijbs.38925
Yoshimura A., Ito M., Mise-Omata S., Ando M. (2021). SOCS: negative regulators of cytokine signaling for immune tolerance. Int. Immunol. 33 (12), 711–716. doi: 10.1093/intimm/dxab055
Zhang Y., Wen H. S., Li Y., Lyu L. K., Zhang Z. X., Wang X. J., et al. (2020). Melanocortin-4 receptor regulation of reproductive function in black rockfish (Sebastes schlegelii). Gene 741, 144541. doi: 10.1016/j.gene.2020.144541
Keywords: interleukin-22, parturition, black rockfish, RNA-seq, cytokine
Citation: Yan S, Lyu L, Wang X, Wen H, Li Y, Li J, Yao Y, Zuo C, Xie S, Wang Z and Qi X (2022) The anti-inflammatory cytokine IL-22 exhibited a proinflammatory effect in the ovary aiding with the parturition of ovoviviparous black rockfish (Sebastes schlegelii). Front. Mar. Sci. 9:1041681. doi: 10.3389/fmars.2022.1041681
Received: 11 September 2022; Accepted: 28 November 2022;
Published: 14 December 2022.
Edited by:
Rui Jia, Freshwater Fisheries Research Center, Chinese Academy of Fishery Sciences, ChinaCopyright © 2022 Yan, Lyu, Wang, Wen, Li, Li, Yao, Zuo, Xie, Wang and Qi. This is an open-access article distributed under the terms of the Creative Commons Attribution License (CC BY). The use, distribution or reproduction in other forums is permitted, provided the original author(s) and the copyright owner(s) are credited and that the original publication in this journal is cited, in accordance with accepted academic practice. No use, distribution or reproduction is permitted which does not comply with these terms.
*Correspondence: Xin Qi, qx@ouc.edu.cn
†These authors have contributed equally to this work