- 1Chongqing Key Laboratory of Nonlinear Circuits and Intelligent Information Processing, College of Electronic and Information Engineering, Southwest University, Chongqing, China
- 2Chinese Academy of Sciences (CAS) Center for Excellence in Nanoscience Beijing Key Laboratory of Micro-nano Energy and Sensor, Beijing Institute of Nanoenergy and Nanosystems, Chinese Academy of Sciences, Beijing, China
- 3Shanxi Key Laboratory of Advanced Manufacturing Technology, North University of China, Taiyuan, Shanxi, China
Introduction
With the worldwide aggravations caused by resource consumption and environmental pollution in recent years, it is imperative to reduce carbon emissions, achieve carbon neutrality, and find pollution-free sources of renewable energy (Gielen et al., 2016). Solar energy, wind energy, and blue energy are the main clean energy sources currently being explored (Jacobson et al., 2015). Moreover, owing to the wide distribution of oceans, the development, and application of ocean energy have become a vital approach to solving increasingly serious energy problems (Wang, 2017a).
A triboelectric nanogenerator (TENG) was invented by Z. L. Wang in 2012, based on coupling the effects of contact electrification and electrostatic induction (Wang, 2017b). In an ocean wave pushing the device, cyclical contact-separation or sliding motion occurs, which then generates the electrical output. The working mechanism depends on the different attraction to electrons of the materials used. When two materials are close, an electric charge is induced on the surface, generating a potential difference. Electrons are then transferred to the more electronegative material. When these two materials are separate, electrons flow back through the external circuit to balance the potential difference (Figure 1A). Therefore, in the theoretical calculation, the V- x relationship (Wang et al., 2012) is given by the following equations:
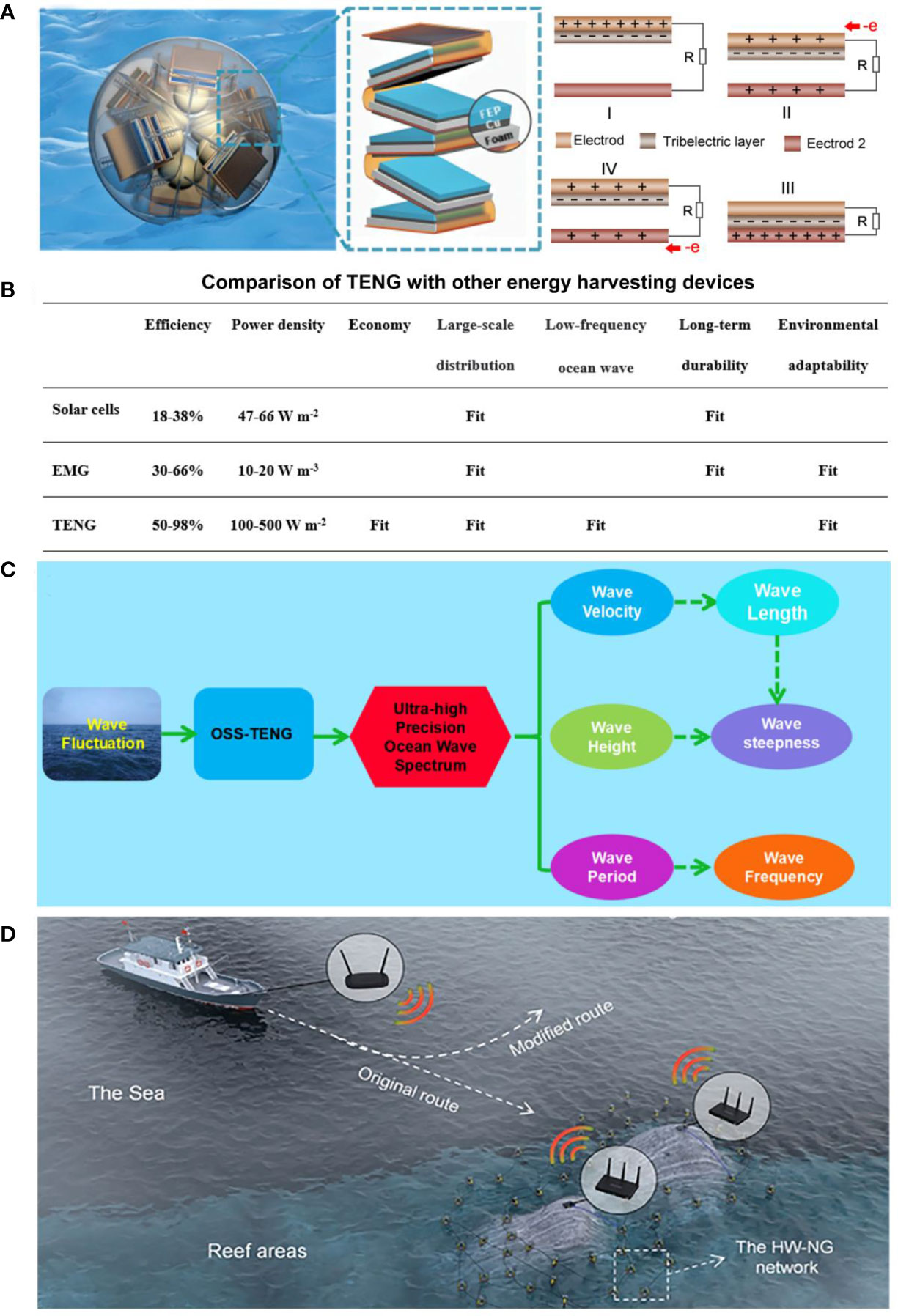
Figure 1 (A)The working mechanism of TENG (Hao et al., 2022). (B) Comparison of TENG with other energy harvesters (Hu et al., 2022) (Miller and Keith, 2018) (Wang et al., 2017) (Green, 2020). (C) Flowchart of TOSS acquiring data on ocean-wave spectrum and multi parameters (Zhang et al., 2020). (D) Schematic diagram of the self-powered automatic RAW system for ocean navigation based on HW-NG network (Ren et al., 2021).
Here, σ is the charge density, S is the metal area, ϵ0 is the permittivity of free space, d0 is the effective triboelectric thickness, and x(t) is the distance between these two triboelectric layers.
Compared with solar energy, TENG possesses continuity of working, low cost, and strong environmental adaptability. TENG can also adapt better to low-frequency wave energy than EMG, which is more suitable for high-entropy energy harvesting. The characteristics of TENG in ocean energy harvesting are illustrated in Figure 1B (Hu et al., 2022, (Miller and Keith, 2018, (Wang et al., 2017, (Green, 2020), which is an emerging technology that can convert mechanical stimulation into electrical energy and display extensive applications in several scientific fields (Yang et al., 2013), such as modern intelligent sports (Hao et al., 2022), self-powered sensing (Han et al., 2019), physiological information monitoring (Yang et al., 2022), and human-computer interaction (Zhu et al., 2020).
Research on marine science has entered a new era, as part of which the multitudinous rational and efficient utilization of ocean energy has received increasing, extensive attention from researchers. TENG has been demonstrated as a promising harvester of ocean energy (Zhang et al., 2018). In addition, TENG can be also used as distributed sensors to monitor ocean conditions in the long term (Chen et al., 2015), through large-scale installation to realize environmental monitoring such as hydrologic observation (Rui et al., 2020), and can be extended to liquid composition determination (Zhao et al., 2021), even acting as an artificial electroreceptor to detect the target (Guo et al., 2022), which is a significant development for the modernization of ocean monitoring.
This paper introduces applications of TENG for energy harvesting and self-powered sensing in the ocean. The work of TENG in blue energy harvesting is summarized first, concentrating on the energy harvesting characteristics of TENG with different working modes. Second, the application field and advantages of TENG are generalized. Finally, this paper discusses challenges and feasible plans in marine science.
Research and application of TENG in marine scientific research
Ocean energy harvesting
The oceans contain a great deal of energy, but the situation of accessing that energy is complicated. Large-scale energy harvesting challenges the traditional utilization of ocean energy. (Xu et al., 2022). Due to high harvest efficiency, the convenience of installation, low price, and adaptability to different environments (Chen et al., 2018), TENG is considered to be a new idea in ocean energy harvesting. Zhang et al. (Zhang et al., 2021) designed an active resonant triboelectric nanogenerator system fabricated by a pendulum, a tumbler, and a flexible ring TENG based on the contact-separation mode. Due to the resonance effect of the multilayer structure, the device can harvest ocean energy with characteristics of omnidirectional and varying frequency. Thanks to the high-frequency damping effect of the single pendulum and the tumbler, the energy harvesting efficiency of the device in low-frequency water waves can be ameliorated. This device demonstrates the feasibility of triboelectric nanogenerators as an ocean energy harvester.
Diverse energy sources can be found in the ocean, such as wave energy, ocean current energy, tidal energy, and ocean thermal energy (Melikoglu, 2018). To probe deeper into ocean current energy, Wang et. al. (Wang et al., 2021) have presented a structure design: a flexible underwater flag-like TENG (UF-TENG) system based on a contact-separation mode for harvesting ocean current energy, which consists of two conductive ink-coated PET membranes and a waterproof PTFE membrane. While harvesting deep current, the vortex street will be induced by the cylinder, enhancing the vibration of the device, and improving the electrical output significantly at lower flow velocities. The experimental results prove that the scheme can not only improve the utilization rate of ocean energy but also provide a new idea for harvesting current energy. Ocean energy is a sort of high-entropy energy characterized by irregularity. To overcome this problem, Wang et al. (Wang et al., 2015) propose a rolling-structured, freestanding triboelectric-layer-based nanogenerator (RF-TENG) to improve the output of the TENG under random wave motions from all directions. A high charge transfer efficiency can be obtained by using nylon balls in contact with the PI film inside the closed shell, even under low-amplitude vibration. The rolling design also effectively reduces wear and achieves resonance with the waves, improving output performance greatly. Chen et al. (Chen et al., 2021) combined natural animal furs with TENG based on a free-standing mode and designed a fur-brush TENG (FB-TENG). Owing to the extremely low wear involved, the performance of the device was only reduced by 5.6% after 300,000 continuous operation cycles, and the output current was elevated by 30%, which was obtained in ambient conditions. Moreover, FB-TENG maintains a high output performance of the relative humidity increased up to 90%. The rotation structure harvests energy to provide a sustainable output to the device with fewer frictional losses and reduces material wear and heat generation during friction. The experimental result exhibits a structure of reducing wear on the premise of maintaining a high performance and can be applied in a high-humidity marine environment for a long time.
Owing to the limitations imposed by interfacial effects, the peak power densities of TENG are less than one watt per square meter. Xu et al. (Xu et al., 2020) introduce a droplet energy harvester based on liquid–solid interface contact electrification. Water droplets hit a PTFE film attached to the ITO substrate, and spread across the device after impact, then connect with the originally disconnected components. This transfers the conventional interface effect into the bulk effect, heightening the instantaneous power density by several orders of magnitude. The FB-TENG reduces the requirement for the hermeticity of the device and eliminates the water screening effect, greatly elevating the operational stability of the device under extreme conditions. The above cases provide an ideal effective energy conversion for the utilization of ocean energy. The open-circuit voltage and short-current current of TENG can reach 10 kV and 1.5mA respectively. Power density hit a peak of 500 W m-2 (Wu et al., 2019) and the maximum efficiency of the device was up to 90% (Tang et al., 2015). In light of its high output performance, TENG is a promising energy conversion method for low-frequency, low-amplitude, and omnidirectional ocean energy, and could provide an innovative and effective approach for large-scale ocean energy harvesting.
Self-powered sensing and application in marine
Water wave spectrum, ocean temperature, relative humidity, and sea-level air pressure are vital parameters for marine safety and nautical communication (Depalo et al., 2021). By identifying pressure and material, TENG possesses the sensing characteristics of being widely distributed, having low power consumption, and a high sensitivity of 346.5 pC N-1 (Zhang et al., 2022), as well as a high accuracy of up to 97.8% (Wu et al., 2018), which can transmit signals directly or transmit electrical signals using nodes.
Zhang et al. (Zhang et al., 2020) have presented a self-powered and high-performance triboelectric ocean-wave spectrum sensor (TOSS) fabricated with a tubular TENG and hollow ball buoy (Figure 1C). Due to the ultrahigh sensitivity of 2530 mV/mm and the tiny monitoring error of 0.1%, six basic ocean wave parameters (wave height, wave period, wave frequency, wave speed, wavelength, and wave steepness), as well as wave speed spectrum and mechanical energy spectrum can be recognized from the electrical signals of TOSS. The predominant advantage of the proposed method is that it can achieve active ocean-wave sensing without any additional power source, which supplies significant and accurate data support for intelligent marine monitoring. To ensure the safe navigation of ships sailing in bad weather, Ren et al. (Ren et al., 2021) proposed a hybrid wave energy harvesting nanogenerator (HW-NG) as a power source for long-distance wireless transmission, which is assembled with an electromagnetic generator (EMG) integrated by a pendulum structure. As depicted in Figure 1D, HW-NGs established long-distance (1.5 km) communication nodes in the sea, and the wireless forewarning network is composed of hundreds of HW-NGs, which can realize real-time forewarning through automatic switching modules. Navigation safety is effectively ameliorated with the support of large-scale array installation.
Ocean waves contain a variety of information, which are normally difficult to quantify precisely. Numerous natural resources are available as the exploration of the ocean progresses, and the corresponding underwater communication technology has also become a huge challenge. Zhao et al. (Zhao et al., 2022) have developed an underwater wireless communication system through Maxwell displacement currents generated by TENG. Maxwell’s equations as the basis of modern wireless electromagnetic communication, electricity and magnetism are integrated by displacement current corresponding to ∂D/∂t in Maxwell’s equations, respectively. The permittivity in a vacuum is ϵ0, and Maxwell’s displacement current density (Cao et al., 2018) can be defined as
The electrical output is generated by the variation of the electric field built in the TENG, which is directly correlated with the second term (∂P/∂t) in Maxwell’s displacement current. E0 is induced in water as the TENG works, and P0 is the polarization electric field generated from the electric field E0. Where the second term Jp (Wang, 2020) (generated by the polarization electric field) is
An underwater electric field can be generated via a TENG, thereby the corresponding time-varying current can be measured at a distance. The electric signal has a strong anti-interference ability, which is obtained in the underwater environment, without any distortion of waveform even after a 100 meter long spiral water pipe, the text and image signals can still be transmitted at 16 bits/s. The results reveal the principle and process of Maxwell displacement currents in underwater communication, demonstrating the potential of TENG for marine information gathering and sensing. Due to the randomness of energy in the environment, the output of TENG is irregular, with the characteristics of high impedance and pulsed output. Efficient and stable energy management is essential for self-powered systems. One feasible approach designed power converters to achieve impedance matching (Niu et al., 2015) with 60% energy storage efficiency achieved. Another practicable strategy involved the design of a rational charging cycle (Zi et al., 2016), which allows for a theoretical increase of 50% in maximum energy storage efficiency.
Discussion
TENG has been widely applied in contemporary marine science and numerous types of research in marine science have been carried out to date. Nevertheless, due to the complexity of ocean conditions, there are still many challenges in these fields. Through the optimization of the structure, the efficiency of energy harvesting of TENG has been greatly improved. Nonetheless, it is difficult to form a unified standard in many types of structures. As a consequence, the structure of TENG should be optimized and classified, and modular design carried out to reduce the costs involved. Through analysis and comparison of TENGs fabricated based on different operating modes, their advantages and application area should also be classified. Furthermore, corresponding modifications should be conducted in line with this model when in specific applications. This approach could reduce the research period and lessen the research and development costs involved. In addition, Although TENG is can harvest low-frequency, low-amplitude, and omnidirectional wave energy, the efficiency of energy harvesting is limited in severe weather conditions. A combination of other types of generators allows the harvester to be efficiently applied in different ocean conditions.
Secondly, on account of its high sensitivity and low price, TENG can be applied as a self-powered sensor in large-scale marine information sensing. Moreover, TENG could provide sensing signals for marine meteorological monitoring, safety monitoring, and signal communication. However, it is easily affected by environmental factors, so a high-resolution sensing analysis system is indispensable. The sensor network is constructed by a large-scale distribution of TENG, to increase the number of samples, reduce the error of the sensor system, and determine more accurate information. Multidimensional analysis of data is carried out to reduce the impact of measurement errors on real data. The above assumption can be realized by embedding corresponding software to collect and process sensing information in an integrated way.
TENG has great potential in self-powered systems. On the one hand, on account of this approach to energy harvesting being unique and simple, TENG can provide novel ideas for sustainable clean energy. On the other hand, since TENG takes into account the application characteristics of energy harvesting and sensing, a continuously sensing combination can be formed by sending the data gathered by high-performance self-powered devices.
At the same time, blue energy harvesting enters the era of the intelligent internet of things, whether in disaster prevention, navigation information prediction, or marine resource detection. It requires the provision of sustainable energy to convert ocean energy into electricity for large-scale sensors. Based on these viewpoints, a digital energy revolution is currently being led by TENG, which integrates energy harvesting and self-powered sensing of marine information. By combining big data analysis, cloud computing could further improve our understanding of the ocean and provide a new approach to harnessing ocean energy.
Author contributions
Writing—original draft, YH; writing—review and editing,YH, XL, BC, and ZZ. All authors contributed to the article and approved the submitted version.
Funding
This project was supported by the Opening Project of the Shanxi Key Laboratory of Advanced Manufacturing Technology (No. XJZZ202106) and the State key laboratory of precision measuring technology and instruments (No. pilab2208).
Conflict of interest
The authors declare that the research was conducted in the absence of any commercial or financial relationships that could be construed as a potential conflict of interest.
Publisher’s note
All claims expressed in this article are solely those of the authors and do not necessarily represent those of their affiliated organizations, or those of the publisher, the editors and the reviewers. Any product that may be evaluated in this article, or claim that may be made by its manufacturer, is not guaranteed or endorsed by the publisher.
References
Cao X., Zhang M., Huang J., Jiang T., Zou J., Wang N., et al. (2018). Inductor-free wireless energy delivery via maxwell’s displacement current from an electrodeless triboelectric nanogenerator. Adv. Mater. 30 (6), 1404011. doi: 10.1002/adma.201704077
Chen P., An J., Shu S., Cheng R., Nie J., Jiang T., et al. (2021). Super-durable, low-Wear, and high-performance fur-brush triboelectric nanogenerator for wind and water energy harvesting for smart agriculture. Adv. Energy Mater. 11 (9), 2003066. doi: 10.1002/aenm.202003066
Chen B. D., Tang W., He C., Deng C. R., Yang L. J., Zhu L. P., et al. (2018). Water wave energy harvesting and self-powered liquid-surface fluctuation sensing based on bionic-jellyfish triboelectric nanogenerator. Mater. Today 21 (1), 88–97. doi: 10.1016/j.mattod.2017.10.006
Chen J., Yang J., Li Z., Fan X., Zi Y., Jing Q., et al. (2015). Networks of triboelectric nanogenerators for harvesting water wave energy: A potential approach toward blue energy. ACS Nano. 9 (3), 3324–3331. doi: 10.1021/acsnano.5b00534
Depalo F., Wang S., Xu S., Guedes Soares C. (2021). Design and analysis of a mooring system for a wave energy converter. J. Mar. Sci. Eng. 9 (7), 782. doi: 10.3390/jmse9070782
Gielen D., Boshell F., Saygin D. (2016). Climate and energy challenges for materials science. Nat. Mater. 15 (2), 117–120. doi: 10.1038/nmat4545
Green M. A. (2020). Tracking solar cell conversion efficiency. Nat. Rev. Phys. 2 (4), 172–173. doi: 10.1038/s42254-020-0163-y
Guo Z., Wang H., Shao J., Shao Y., Jia L., Li L., et al. (2022). Bioinspired soft electroreceptors for artificial precontact somatosensation. Sci. Adv. 8, eabo5201. doi: 10.1126/sciadv.abo5201
Han Y., Yi F., Jiang C., Dai K., Xu Y., Wang X., et al. (2019). Self-powered gait pattern-based identity recognition by a soft and stretchable triboelectric band. Nano. Energy 56, 516–523. doi: 10.1016/j.nanoen.2018.11.078
Hao Y., Wen J., Gao X., Nan D., Pan J., Yang Y., et al. (2022). Self-rebound cambered triboelectric nanogenerator array for self-powered sensing in kinematic analytics. ACS Nano. 16 (1), 1271–1279. doi: 10.1021/acsnano.1c09096
Hu C., Yang Y., Wang Z. L. (2022). Quantitative comparison between the effective energy utilization efficiency of triboelectric nanogenerator and electromagnetic generator post power management. Nano. Energy 103, 107760. doi: 10.1016/j.nanoen.2022.107760
Jacobson M. Z., Delucchi M. A., Bazouin G., Bauer Z. A. F., Heavey C. C., Fisher E., et al. (2015). 100% clean and renewable wind, water, and sunlight (WWS) all-sector energy roadmaps for the 50 united states. Energy Environ. Sci. 8 (7), 2093–2117. doi: 10.1039/c5ee01283j
Melikoglu M. (2018). Current status and future of ocean energy sources: A global review. Ocean Eng. 148, 563–573. doi: 10.1016/j.oceaneng.2017.11.045
Miller L. M., Keith D. W. (2018). Observation-based solar and wind power capacity factors and power densities. Environ. Res. Letters 13 (10), 104008. doi: 10.1088/1748-9326/aae102
Niu S., Wang X., Yi F., Zhou Y. S., Wang Z. L. (2015). A universal self-charging system driven by random biomechanical energy for sustainable operation of mobile electronics. Nat. Commun. 6, 8975. doi: 10.1038/ncomms9975
Ren Z., Liang X., Liu D., Li X., Ping J., Wang Z., et al. (2021). Water-wave driven route avoidance warning system for wireless ocean navigation. Adv. Energy Mater. 11 (31), 2101116. doi: 10.1002/aenm.202101116
Rui P., Zhang W., Zhong Y., Wei X., Guo Y., Shi S., et al. (2020). High-performance cylindrical pendulum shaped triboelectric nanogenerators driven by water wave energy for full-automatic and self-powered wireless hydrological monitoring system. Nano. Energy 74, 104937. doi: 10.1016/j.nanoen.2020.104937
Tang W., Jiang T., Fan F. R., Yu A. F., Zhang C., Cao X., et al. (2015). Liquid-metal electrode for high-performance triboelectric nanogenerator at an instantaneous energy conversion efficiency of 70.6%. Adv. Funct. Mater. 25 (24), 3718–3725. doi: 10.1002/adfm.201501331
Wang Z. L. (2017b). On maxwell’s displacement current for energy and sensors: the origin of nanogenerators. Mater. Today 20 (2), 74–82. doi: 10.1016/j.mattod.2016.12.001
Wang Z. L. (2020). On the first principle theory of nanogenerators from maxwell’s equations. Nano. Energy 68, 104272. doi: 10.1016/j.nanoen.2019.104272
Wang Z. L., Jiang T., Xu L. (2017). Toward the blue energy dream by triboelectric nanogenerator networks. Nano. Energy 39, 9–23. doi: 10.1016/j.nanoen.2017.06.035
Wang S., Lin L., Wang Z. L. (2012). Nanoscale triboelectric-effect-enabled energy conversion for sustainably powering portable electronics. Nano. Lett. 12 (12), 6339–6346. doi: 10.1021/nl303573d
Wang Y., Liu X., Chen T., Wang H., Zhu C., Yu H., et al. (2021). An underwater flag-like triboelectric nanogenerator for harvesting ocean current energy under extremely low velocity condition. Nano. Energy 90, 106503. doi: 10.1016/j.nanoen.2021.106503
Wang X., Niu S., Yin Y., Yi F., You Z., Wang Z. L. (2015). Triboelectric nanogenerator based on fully enclosed rolling spherical structure for harvesting low-frequency water wave energy. Adv. Energy Mater. 5 (24). doi: 10.1002/aenm.201501467
Wu C., Ding W., Liu R., Wang J., Wang A. C., Wang J., et al. (2018). Keystroke dynamics enabled authentication and identification using triboelectric nanogenerator array. Mater. Today 21 (3), 216–222. doi: 10.1016/j.mattod.2018.01.006
Wu C., Wang A. C., Ding W., Guo H., Wang Z. L. (2019). Triboelectric nanogenerator: A foundation of the energy for the new era. Adv. Energy Mater. 9 (1). doi: 10.1002/aenm.201802906
Xu S., Rezanejad K., Gadelho J. F. M., Guedes Soares C. (2022). Influence of the power take-off damping of a dual chamber floating oscillating water column on the mooring fatigue damage. Ocean Eng., 249, 110832. doi: 10.1016/j.oceaneng.2022.110832
Xu W., Zheng H., Liu Y., Zhou X., Zhang C., Song Y., et al. (2020). A droplet-based electricity generator with high instantaneous power density. Nature 578 (7795), 392–396. doi: 10.1038/s41586-020-1985-6
Yang J., An J., Sun Y., Zhang J., Zu L., Li H., et al. (2022). Transparent self-powered triboelectric sensor based on PVA/PA hydrogel for promoting human-machine interaction in nursing and patient safety. Nano. Energy 97, 107199. doi: 10.1016/j.nanoen.2022.107199
Yang Y., Zhang H., Liu R., Wen X., Hou T.-C., Wang Z. L. (2013). Fully enclosed triboelectric nanogenerators for applications in water and harsh environments. Adv. Energy Mater. 3 (12), 1563–1568. doi: 10.1002/aenm.201300376
Zhang C., He L., Zhou L., Yang O., Yuan W., Wei X., et al. (2021). Active resonance triboelectric nanogenerator for harvesting omnidirectional water-wave energy. Joule 5 (6), 1613–1623. doi: 10.1016/j.joule.2021.04.016
Zhang C., Liu L., Zhou L., Yin X., Wei X., Hu Y., et al. (2020). Self-powered sensor for quantifying ocean surface water waves based on triboelectric nanogenerator. ACS Nano. 14 (6), 7092–7100. doi: 10.1021/acsnano.0c01827
Zhang S. L., Xu M., Zhang C., Wang Y.-C., Zou H., He X., et al. (2018). Rationally designed sea snake structure based triboelectric nanogenerators for effectively and efficiently harvesting ocean wave energy with minimized water screening effect. Nano. Energy 48, 421–429. doi: 10.1016/j.nanoen.2018.03.062
Zhang J., Yao H., Mo J., Chen S., Xie Y., Ma S., et al. (2022). Finger-inspired rigid-soft hybrid tactile sensor with superior sensitivity at high frequency. Nat. Commun. 13 (1), 5076. doi: 10.1038/s41467-022-32827-7
Zhao J., Wang D., Zhang F., Liu Y., Chen B., Wang Z. L., et al. (2021). Real-time and online lubricating oil condition monitoring enabled by triboelectric nanogenerator. ACS Nano. 15 (7), 11869–11879. doi: 10.1021/acsnano.1c02980
Zhao H., Xu M., Shu M., An J., Ding W., Liu X., et al. (2022). Underwater wireless communication via TENG-generated maxwell’s displacement current. Nat. Commun. 13 (1), 3325. doi: 10.1038/s41467-022-31042-8
Zhu M., Sun Z., Zhang Z., Shi Q. (2020). Haptic-feedback smart glove as a creative human-machine interface (HMI) for virtual/augmented reality applications. Sci. Adv. 6 (19), eaaz8693. doi: 10.1126/sciadv.aaz8693
Keywords: triboelectric nanogenerator, ocean energy harvesting, structure design, self-powered sensing, marine information
Citation: Hao Y, Li X, Chen B and Zhu Z (2022) Marine monitoring based on triboelectric nanogenerator: Ocean energy harvesting and sensing. Front. Mar. Sci. 9:1038035. doi: 10.3389/fmars.2022.1038035
Received: 06 September 2022; Accepted: 07 November 2022;
Published: 30 November 2022.
Edited by:
Sheng Xu, Jiangsu University of Science and Technology, ChinaReviewed by:
Steven Zhang, Max Planck Institute for Intelligent Systems, GermanyYiqiang Fu, Hong Kong Polytechnic University, Hong Kong SAR, China
Copyright © 2022 Hao, Li, Chen and Zhu. This is an open-access article distributed under the terms of the Creative Commons Attribution License (CC BY). The use, distribution or reproduction in other forums is permitted, provided the original author(s) and the copyright owner(s) are credited and that the original publication in this journal is cited, in accordance with accepted academic practice. No use, distribution or reproduction is permitted which does not comply with these terms.
*Correspondence: Baodong Chen, Y2hlbmJhb2RvbmdAYmlubi5jYXMuY24=; Zhiyuan Zhu, enl1YW56aHVAc3d1LmVkdS5jbg==