- 1Key Laboratory of Marine Ecosystem Dynamics, Second Institute of Oceanography, Ministry of Natural Resources, Hangzhou, China
- 2Observation and Research Station of Yangtze River Delta Marine Ecosystems, Ministry of Natural Resources, Hangzhou, China
- 3State Key Laboratory of Satellite Ocean Environment Dynamics, Second Institute of Oceanography, Ministry of Natural Resources, Hangzhou, China
Water quality in Hangzhou Bay is inferior (grade 5) because of discharged from Yangtze River and Qiantang River, and from factories around the bay. This study analyzed relationships between the environment and macrobenthic communities at 44 stations throughout Hangzhou Bay, based on samples collected in 2006, from 2016–2018, and in 2021. Long-term spatial and temporal changed in macrobenthos, and the influence of secondary water fronts on community composition, were discussed. Macrobenthos in Qiantang River Estuary was numerically, primarily dominated by crustaceans, and elsewhere by polychaetes. Species that differ most in abundance between four identified regions in the bay were the amphipod Corophium sinensis, clam Potamocorbula laevis, and polychaete Amaeana occidentalis. The abundance and biomass of macrobenthos in different regions differs significantly. Taxa were allocated to six feeding guilds: filter feeders and omnivores predominate in Qiantang River Estuary and south of Hangzhou Bay, while surface and subsurface deposit feeders predominate in sediments north of Hangzhou Bay and in the bay mouth area. Negative correlations were apparent between the abundances of polychaetes Nephtys polybranchia, Magelona japonica, Heterospio sinica, Sabella sp. and A. occidentalis and salinity and pH, while positive correlations were apparent between those of Sternaspis chinensis and Capitellidae indet. Fine-grained fraction (silt and clay) and mid-salinity were more suitable for macrobenthos. The numbers and biomasses of macrobenthic species in the Hangzhou Bay frontal area increased significantly relative to values in adjacent areas. The difference of each area near the front was more than 94%, and the main difference species were polychaetes and mollusks. Our baseline data for this region might ultimately contribute towards improved conservation of regional macrobenthos, and a better understanding of ecosystem health in this highly polluted bay.
1 Introduction
Estuaries, transition zones between the land and sea, were characterized by salinity gradients that extend from river mouths into the sea that were controlled by tides and river discharge (Laprise and Dodson, 1993). Plentiful nutrients and organic matter often render these environments highly productive, with high rates of primary productivity (Dolbeth et al., 2007), and they had important ecological functions. However, estuarine environments and biodiversity were increasingly changing because of anthropogenic disturbance, such as occurs with power plant construction, and factory wastewater and pesticide discharged (Ecoutin et al., 2010; González-Ortegón et al., 2012; Calle et al., 2018; Azovsky and Kokarev, 2019).
Hangzhou Bay, Yangtze River Delta, eastern China, was a densely populated and highly industrialized area (Le Gallo and Ertur, 2003). According to China’s marine ecological environment in 2020, waters in this area were of inferior quality (class IV), and they had been for two decades. Bay hydrodynamics were mainly controlled by the Yangtze and Qiantang rivers, and the East China Sea current. A plume of freshwater from the Yangtze River extended to the northern shores of the bay, where it encounters a freshwater plume from the Qiantang River which extended to the upper reaches of the bay, and an intrusion of the East China Sea current. The convergence of these three water masses created a secondary frontal zone. The secondary front may further impacted the marine ecosystem by increasing the deposition of organic matter and detritus from plankton onto the seabed through a “biological pump” action, thereby affecting macrobenthos communities (Longhurst and Glen Harrison, 1989).
Macrobenthos, a main component of estuaries, played an important role in material circulation and food-chain energy flow (Herman et al., 1999; Ghodrati Shojaei et al., 2016). Although movement of macrobenthic organisms was typically limited, their life spans were relatively long, and they were sensitive to environmental change (Patrício et al., 2012). Therefore, their study was well suited to monitoring environmental change, such as that from climate or anthropogenic disturbance (Azovsky and Kokarev, 2019). Changes in estuarine factors such as salinity, sediment quality, and turbidity also directly affected macrobenthos community structures.
Between 2012 and 2019, macrobenthos assemblages in Hangzhou Bay were reported to be mainly controlled by sediment disturbance and salinity gradients (Meng et al., 2021). Sediment toxicity had previously caused half of the species living in this bay to disappear between 2003 and 2015 (Bao et al., 2021). Therefore, long-term monitoring of macrobenthos can signal changed in ecosystems (Paul and Kari, 2004). Relationships between a secondary frontal system in Hangzhou Bay and macrobenthos were unknown. This study examined long-term changes in community structure and macrobenthic functional groups, and the effected of a secondary front on macrobenthos community structure in Hangzhou Bay.
In this study, we hypothesized that variations in salinity and sedimentation would have an impact on macrobenthic community structure, while the secondary front may influence macrobenthic distribution. To test this hypothesis, this study surveyed the macrobenthos in 2006, 2016-2018, 2021. And four zones have been divided based on salinity and sedimentation, while selected part of stations to analyze impact of secondary fronts. The results of this study could provide scientific support for studying the relationship between fronts and benthic organisms.
2 Materials and methods
2.1 Study area
Hangzhou Bay, ~4800 km2 in area, was funnel shaped, ~100 km wide at its mouth and ~20 km at its upstream reach (Xie et al., 2017). At an ~9 m tidal range, this bay had one of the largest tidal ranges in China (Hu et al., 2019). Tides were semidiurnal, and flowed in from the north, and flowed out past the southern Zhoushan Islands (Su and Wang, 1989). This bay was affected by alternating dry and wet monsoon winds, had plenty of sunlight, a humid climate, and abundant rainfall.
2.2 Sample collection and processing
Alternating runoff and tides created a bottom-water salinity gradient in Hangzhou Bay, where salinity gradually increased from the estuary to the mouth of bay (Wu et al., 2019). NOAA remote-sensed salinity data reveals water from Qiantang River to extend into mid-Hangzhou Bay in a tongue shaped plume. Bottom-water salinity in northern areas of the bay was generally low, and that in southern areas occurs in strips (Figure 1A).
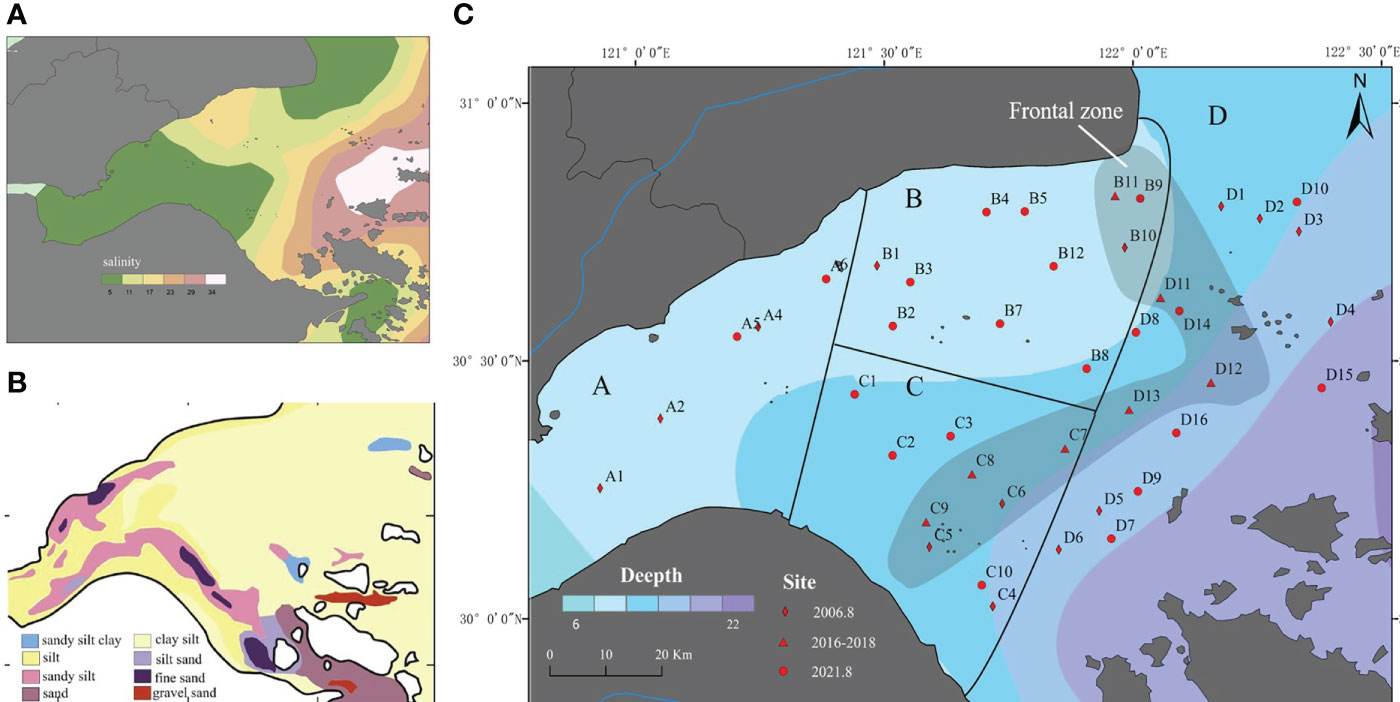
Figure 1 (A)Salinity at 10 m depth, Hangzhou Bay (data from NOAA), (B) Surface sediments, Hangzhou Bay from Xie et al. (2013), (C) Sampling stations, Hangzhou Bay.
A close relationship between salinity and abundance of macrobenthos exists (Rethinam Subramanian et al., 2021). Hangzhou Bay sediments primarily comprised silts with an admixture of sand and clay of 20–40 um size. These sediments were mainly transported in suspension (Xie et al., 2017) (Figure 1B). Different-sized particles settled in different areas depending on water flow. Because sediments were an important foundation of marine food webs (Bao et al., 2021), based on their distribution and that of salinity, this study divides Hangzhou Bay into an estuary region (A), and an inner and outer bay region (D), and further divided the inner bay area into northern (B) and southern (C) regions (Figure 1C). Our data from 44 stations were for the years 2006, 2016–2018, and 2021. All samples were collected in summer. In this study, the five years of the station were analyzed together.
At each station, duplicate Van Veen grab sediment samples (0.1 m2) were collected and sieved through a 0.5 mm mesh sieve. Macrobenthos preserved in 5% formalin solution, identified to the lowest possible taxonomic level beneath a stereo microscope. Species were counted, expressed as individuals per square meter (ind m−2), and weighed, expressed as wet weight per square meter (g m−2).
Species were attributed to feeding guilds (Ysebaert et al., 1998; Macdonald et al., 2010; Telesh and Khlebovich, 2010; Jumars et al., 2015a; Yang et al., 2017): herbivores (H), surface deposit feeders (SDF), filter feeders (FF), carnivores (CA), subsurface deposit feeders (SSDF), and omnivores (O). Where the feeding mode of a particular species was unknown, this study assumed that it fed in a manner similar to that of congeneric or confamilial taxa.
Environmental data was collected in 2006 only. Temperature (T) and pH were measured onsite using a Yellow Springs Instruments water quality meter. Nutrient concentration was determined colorimetrically using cadmium column-diazotization and phenol-indophenol methods for dissolved inorganic nitrogen (DIN), and a molybdate method for PO3− and SiO− with a UV-Vis spectrophotometer. Dissolved oxygen (DO) was determined by iodimetry, and total organic carbon (TOC) by carbon analyzer. Suspended solids (SS) were determined by filtering an aliquot of water through a pre-weighed 47 mm diameter polycarbonate membrane filter with 0.45 μm pore size.
2.3 Data analyses
Macrobenthos diversity was assessed using the Shannon–Wiener diversity (H′), Pielou’s evenness (J′), and Margalef’s richness (d) indexes, as follows:
where Pi is the ratio of individual number or weight of species i to the total number of individuals or total biomass of benthos, S is the species number per station for each sampling period, and N is the total number of individuals or biomass for each sampling period.
This study selected 12 stations in the frontal area, and 12 upper front (B1–B5, B7, B8, B12, C1–3, D8) and 9 lower front (C4, C10, D1, D5–7, D9, D15, D16) stations to assess spatial and temporal differences in macrobenthos communities throughout Hangzhou Bay.
The top three species in each region (total 13 species) were selected and their abundance related to environmental data in 2006. The CCA analysis only analyzed the relationship between environmental data in 2006 and benthos in that year. Since the average water depth in Hangzhou Bay was 10 m and does not vary much from station to station, no analysis is done.
A sampling station map was prepared in ArcMap 10.6 (Environment System Research Institute, US). Cluster analysis was applied to analyze the macrobenthos community by using Primer 6.0 (Plymouth Marine Laboratory, UK). Before analyses, a Bray-Curtis similarity matrix was constructed, and species abundance were transformed using the fourth-root method. One-way analysis of similarity (ANOSIM) was employed to test for any significant differences among ecological groups. Similarity percentage (SIMPER) was used to identify the dominant species contributing most to the similarity within each ecological group. Canonical correspondence analysis (CCA) using Canoco 4.5 (Microcomputer Power, US), and table mapping using Origin 8.0 (OriginLab, US). Nonparametric tests (Kruskal–Wallis) were performed to evaluate spatial and temporal variation in macrobenthos abundance and biomass, and temporal variation in environmental variables, using IBM SPSS Statistics 22.
3 Results
3.1 Spatial and temporal macrobenthic distribution
3.1.1 Spatial distribution in macrobenthos community structure
This study attribute the 80 identified taxa to 41 families, 11 classes, and 10 phyla. Polychaetes were most species, with 35 taxa (43.75% of all taxa), followed by crustaceans (24 taxa, 30%), mollusks (7 taxa, 8.75%), echinoderms (5 taxa, 6.25%), and 9 taxa (11.25%) belonging to other phyla. And they were classified to 6 feeding guilds: SDF (9 taxa), SSDF (7), CA (28), H (4), FF (14), and O (18).
The results of site location clustering analysis of macrobenthos during the four months in Hangzhou Bay was consistent (Figure 2). From the results of the hierarchical clustering analysis, there were not much similarity between survey stations, but part of stations that in the different regions similarity more than 60%. ANOSIM results revealed significant differences in macrobenthos communities throughout Hangzhou Bay (Global R = 0.087, significance level of sample statistic P = 0.015). Significant differences exist between communities in regions A and D, and regions B and D.
The dominant species in each region were revealed using Simper analysis (Table 1). The main contributor(s) to dissimilarity in samples within region A was the amphipod Corophium sinensis; B the clam Potamocorbula laevis, polychaetes Sternaspis scultata and Nephtys oligobranchia; C the polychaetes Sternaspis chinensis and Heteromastus filiformis, and crustaceans Malacostraca; and D, the polychaetes Amaeana occidentalis, Sternaspis chinensis, and Nephtys polybranchia.
All average dissimilarities exceeded 90%, indicating strong spatial variation in the composition of macrobenthos communities (Table 1). The greatest difference in regions A and D was 96.17%, with the species contributing most to difference being the amphipod C. sinensis. In regions A and C, the difference was 95.12%, with C. sinensis again contributing most to differences. For regions A and B, B and D, and B and C, the species contributing most to dissimilarities was the clam Potamocorbula laevis. The lowest difference (90.98%) was between regions C and D, with the polychaete A. occidentalis contributing most to differences.
Percentage values (%) of macrobenthos abundance and biomass in different regions of Hangzhou Bay are presented in Figure 3A. For region A, crustaceans were most abundant (the amphipod Corophium sinensis reached 80 ind m−2), and mollusk biomass was highest (bivalve biomass reached 0.4 g m−2). The abundance ratio of polychaetes in regions B, C and D was highest, with the most-abundant species being Magelona cincta, Sternaspis chinensis, and Amaeana occidentalis. In region C, polychaetes, especially S. chinensis, also contributed most to biomass.
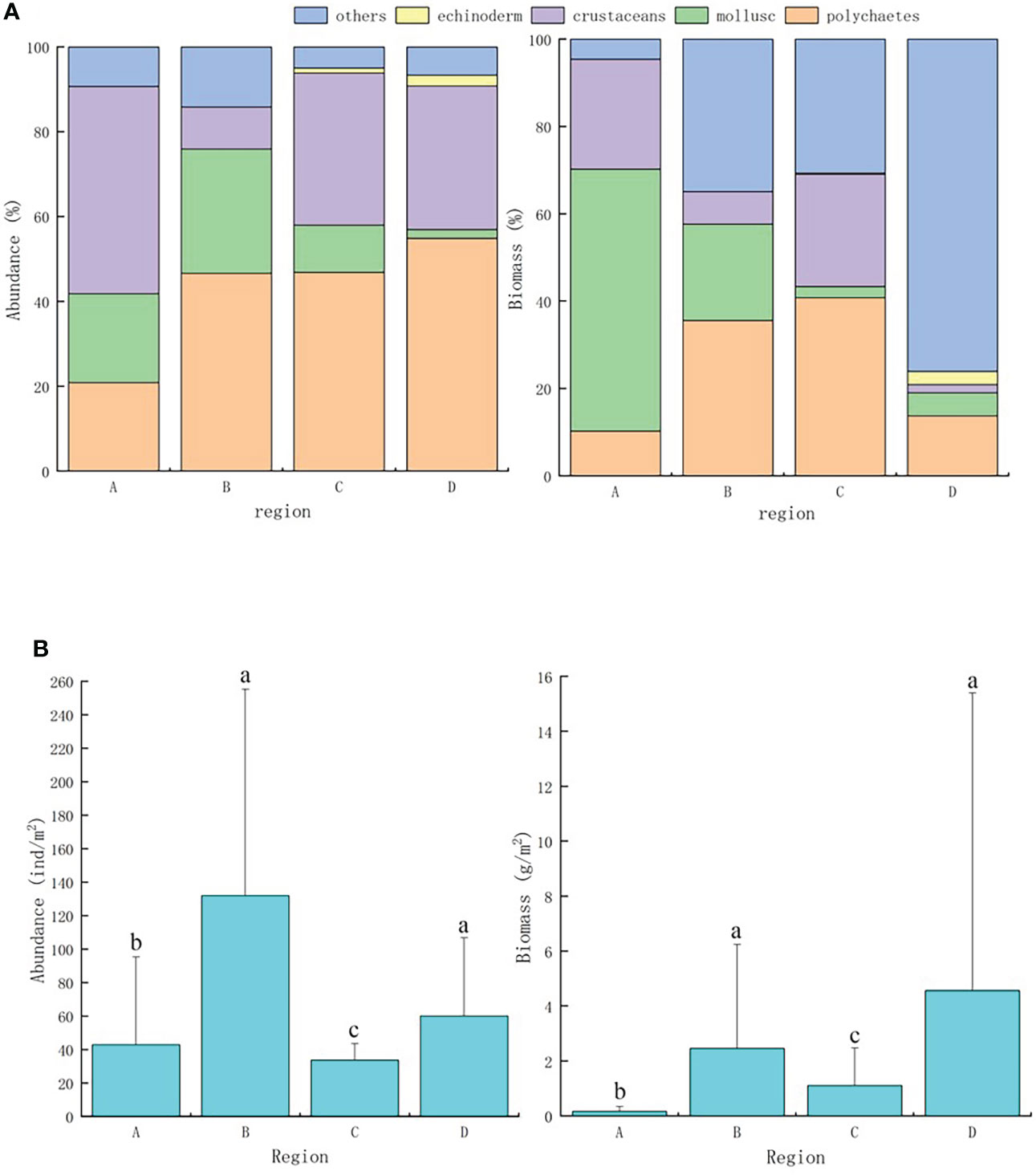
Figure 3 (A)Abundance and biomass precent of macrobenthos throughout Hangzhou Bay, (B) Abundance and biomass of macrobenthos throughout Hangzhou Bay. a, b, c represent the difference of multiple comparisons.
Kruskal–Wallis tests revealed significant differences (P< 0.05) in macrobenthos abundance and biomass between region A and elsewhere, and for significant differences to exist in abundance and biomass between regions B and C, and C and D (Figure 3B).
The distributions of macrobenthos abundance and biomass throughout Hangzhou Bay were depicted in Figures 4A, B. High abundance and biomass stations occurred mainly in region B and near the bay mouth. Abundance and biomass generally, gradually increased from the estuary to the mouth, and decreased outside of the bay. Abundance and biomass were greater at more northern stations than they were at southern stations.
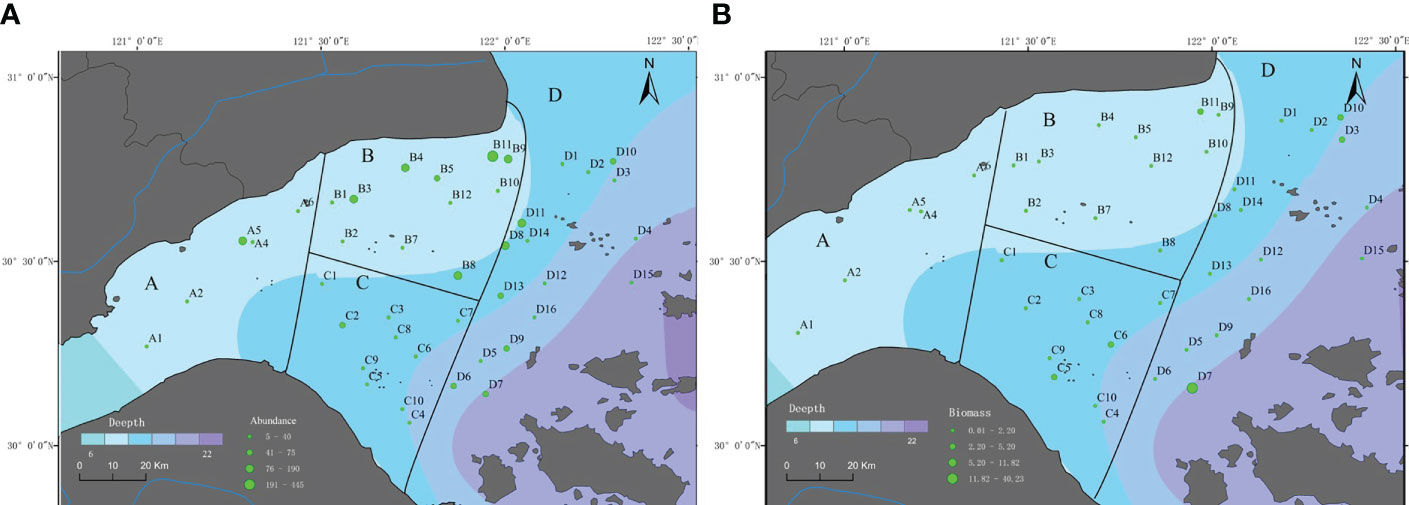
Figure 4 (A) Macrobenthos abundance (ind m−2), Hangzhou Bay, (B) Macrobenthos biomass (g m−2), Hangzhou Bay.
Macrobenthos community diversity throughout Hangzhou Bay was shown in Figure 5. Lowest H′, J′, and d values occurred in region A. Average H′ and J′ values in region C were higher than elsewhere. There were no significant differences in diversity indexes between regions.
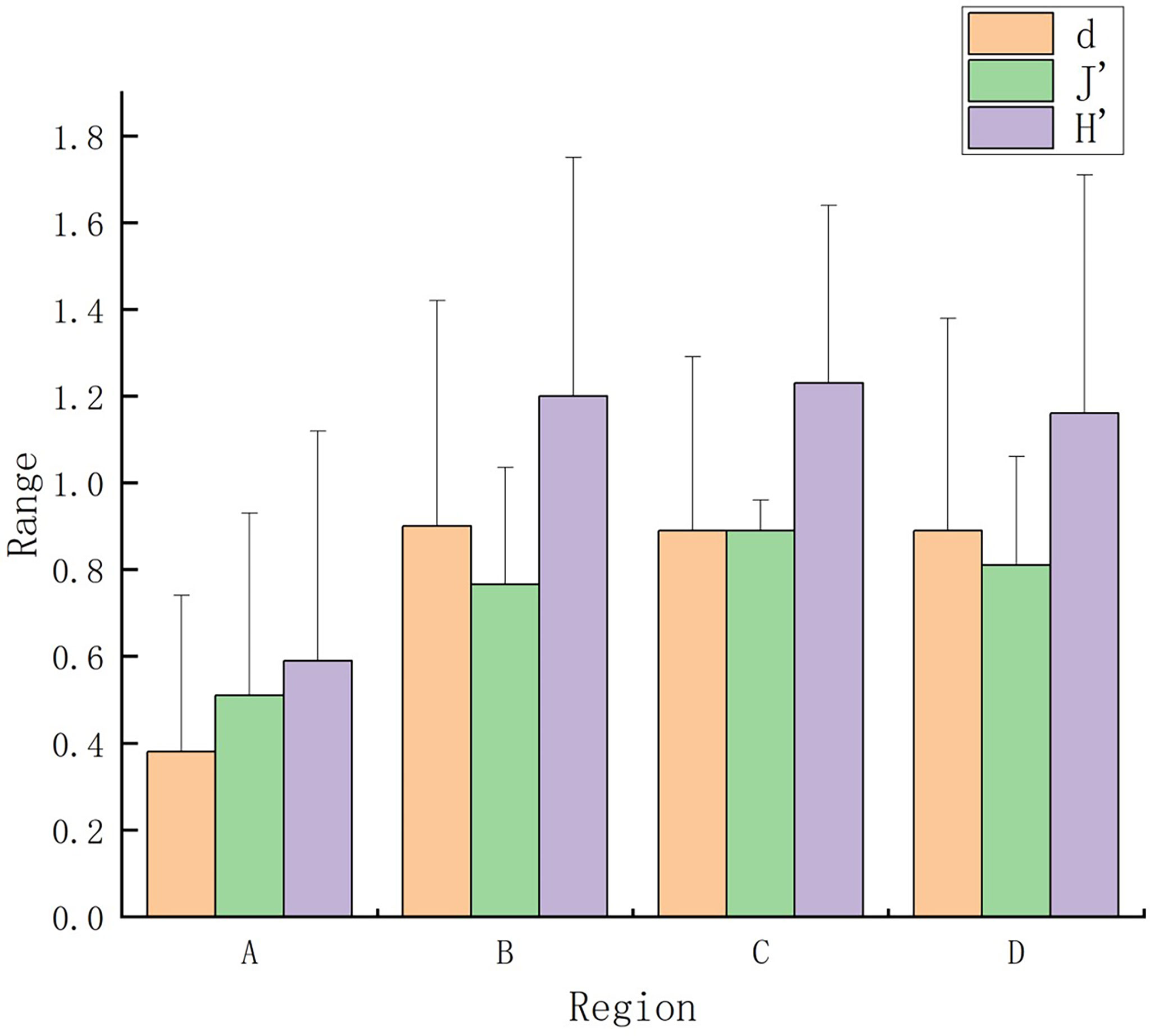
Figure 5 Margalef’s richness (d), Pielou’s evenness (J′), and Shannon–Wiener diversity (H′) indexes for macrobenthos communities in Hangzhou Bay.
3.1.2 Spatial and temporal distribution in macrobenthos feeding guilds
Percentage values (%) of feeding guild abundances and biomasses were presented in Figure 6. Guilds FF and O were most abundant in regions A and B, and SSDF in regions C and D. The highest proportional biomasses of FF occurred in region A, SDF in regions B and D, and SSDF in region C. Kruskal–Wallis tests reveal significant differences in the abundance of feeding guilds in region C only. Within a feeding guild, only the abundance of CA differed significantly between regions.
3.2 Relationships between macrobenthos and environmental parameters
The 13 most-abundant species were selected for CCA analysis to identify environmental variables that most-affect macrobenthos community composition (Figure 7). Of measured parameters, salinity, pH, SiO−, and DO most strongly influenced community structure. The first axis correlates positively with pH (correlation coefficient 0.428) and negatively with DO (correlation coefficient −0.578). The second axis correlates positively with salinity (correlation coefficient 0.546) and negatively with SiO− (correlation coefficient −0.4394). Eigenvalues for the first two axes, 0.942 and 0.712, account for 65.3% of all eigenvalues, indicating that these axes explain most of the variation in abundance of the 13 most-abundant species. For polychaetes, the abundances of N. polybranchia, M. japonica, Heterospio sinica, A. occidentalis and Sabella sp. correlated negatively with salinity and pH, while those of S. chinensis and Capitellidae indet correlated positively. The abundance of the branded goby Chaeturichthys stigmatias and clam Nucula izushotoensis correlated positively with suspended solids, but negatively with salinity. In this study, no strong correlation was found between the abundance of croaker Johnius sp. and shrimp Acetes chinensis and the environmental factors investigated.
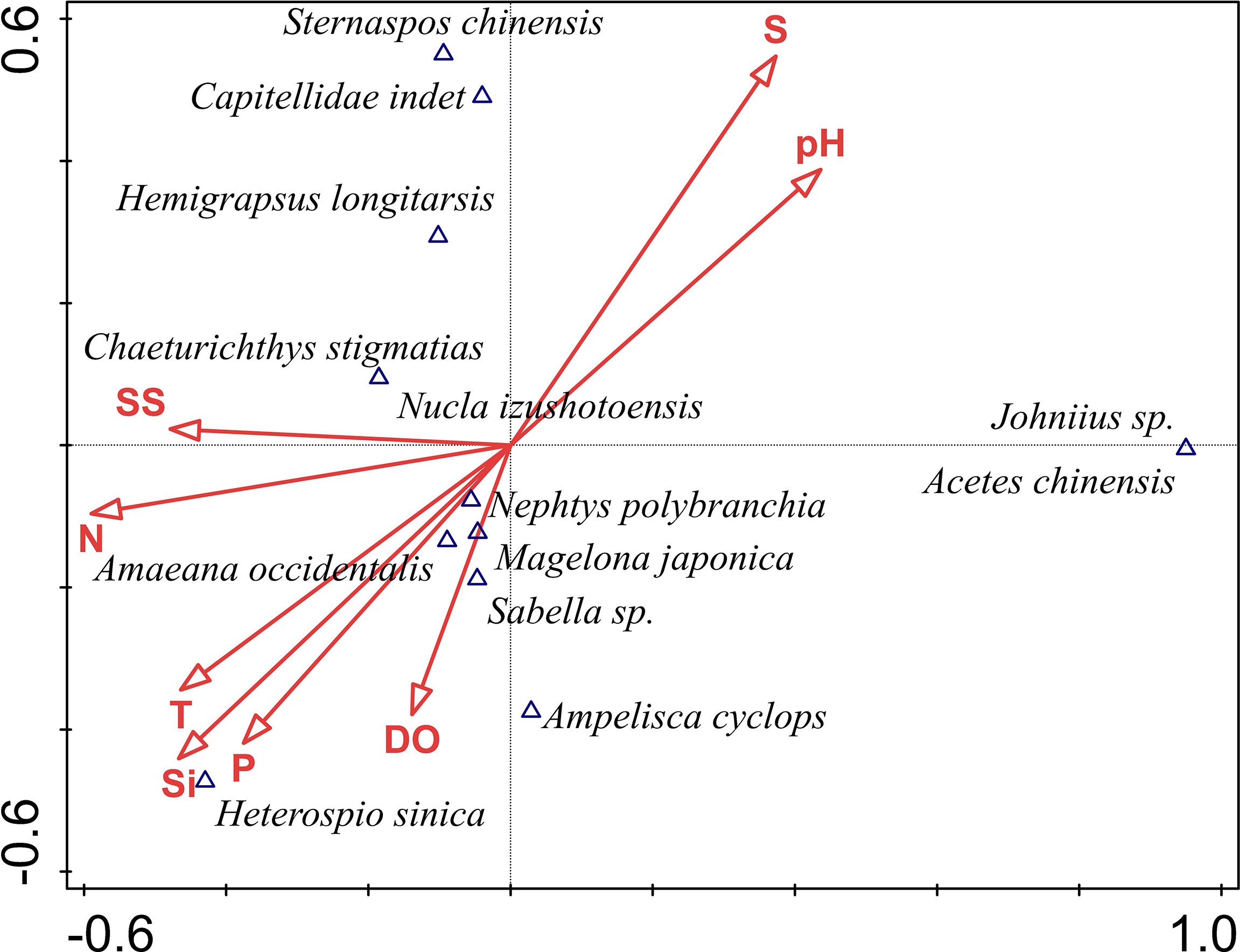
Figure 7 CCA ranking of macrobenthos and environmental factors in Hangzhou Bay. P: ; T: temperature; SS: suspended solids; DO: dissolved oxygen; S: salinity; N: dissolved inorganic nitrogen; Si: SiO−.
3.3 The impact of the secondary front on macrobenthos
Of 44 species in the frontal zone, the most speciose taxon was Polychaeta with 21 taxa, followed by crustaceans (12 taxa), mollusks (4 taxa), echinoderms (1 taxon), and other phyla (6 taxa); 33 species occurred in the upper front and 22 species in the lower front (Figure 8).
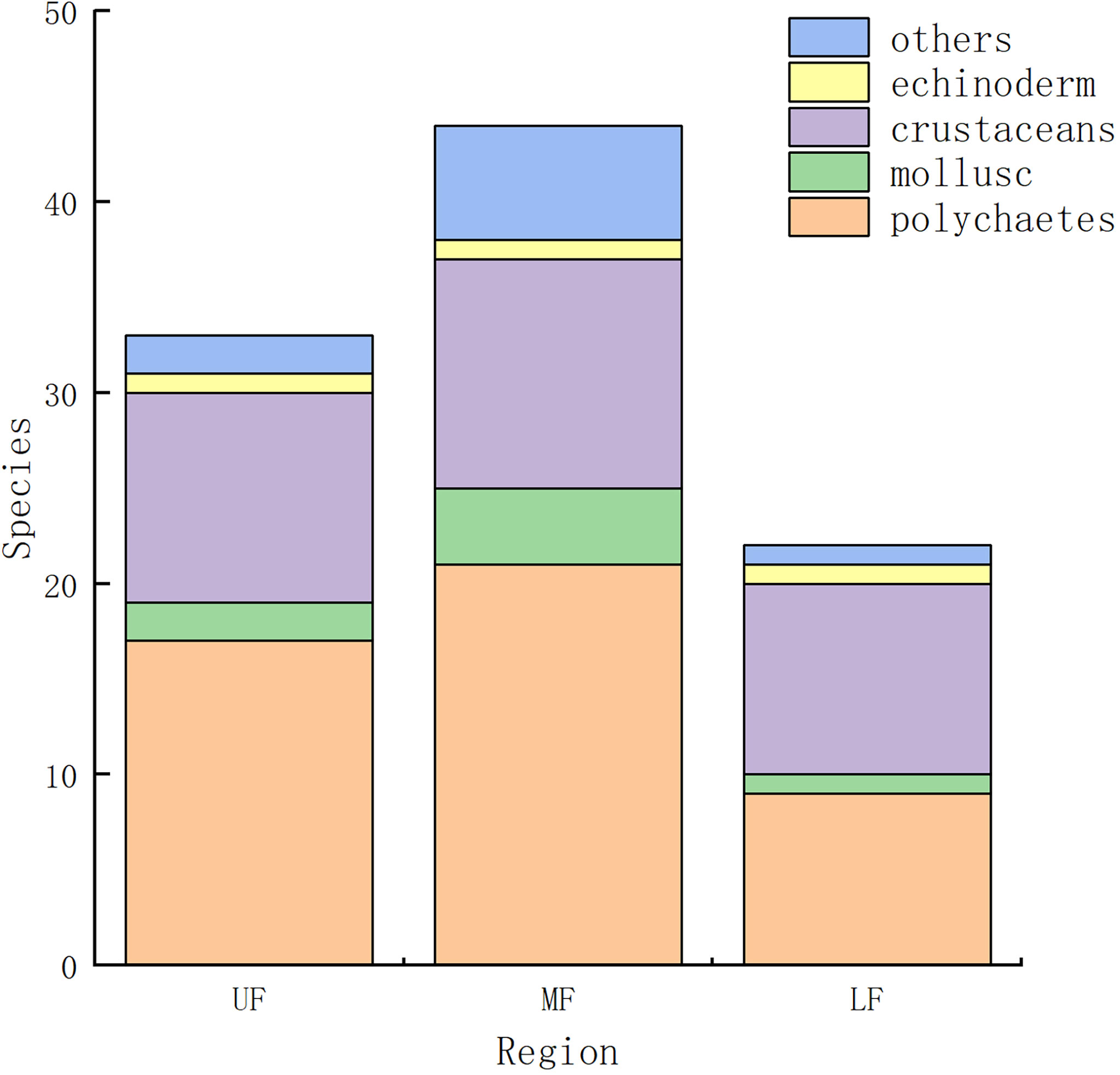
Figure 8 Macrobenthos composition in and adjacent to the secondary front, Hangzhou Bay. (UF, upper front; MF, middle front; LF, lower front).
Proportional macrobenthos abundances and biomasses in the secondary front and adjacent areas of Hangzhou Bay were presented in Figure 9. In the upper and lower front, polychaetes dominated numerically (36.1% of all taxa), followed by crustaceans (25.5%) and mollusks (22.35%); community composition was relatively uniform. Biomass of other species gradually increased from the upper to lower front, with crustacean biomass gradually decreasing. Polychaete biomass was highest in the upper front (34.15%). Averaged abundances and biomasses in the frontal area were highest (Table 2). A significant difference existed between abundances of macrobenthos in the frontal area and the lower frontal area, and a significant difference in biomass between the frontal area and the other two areas.
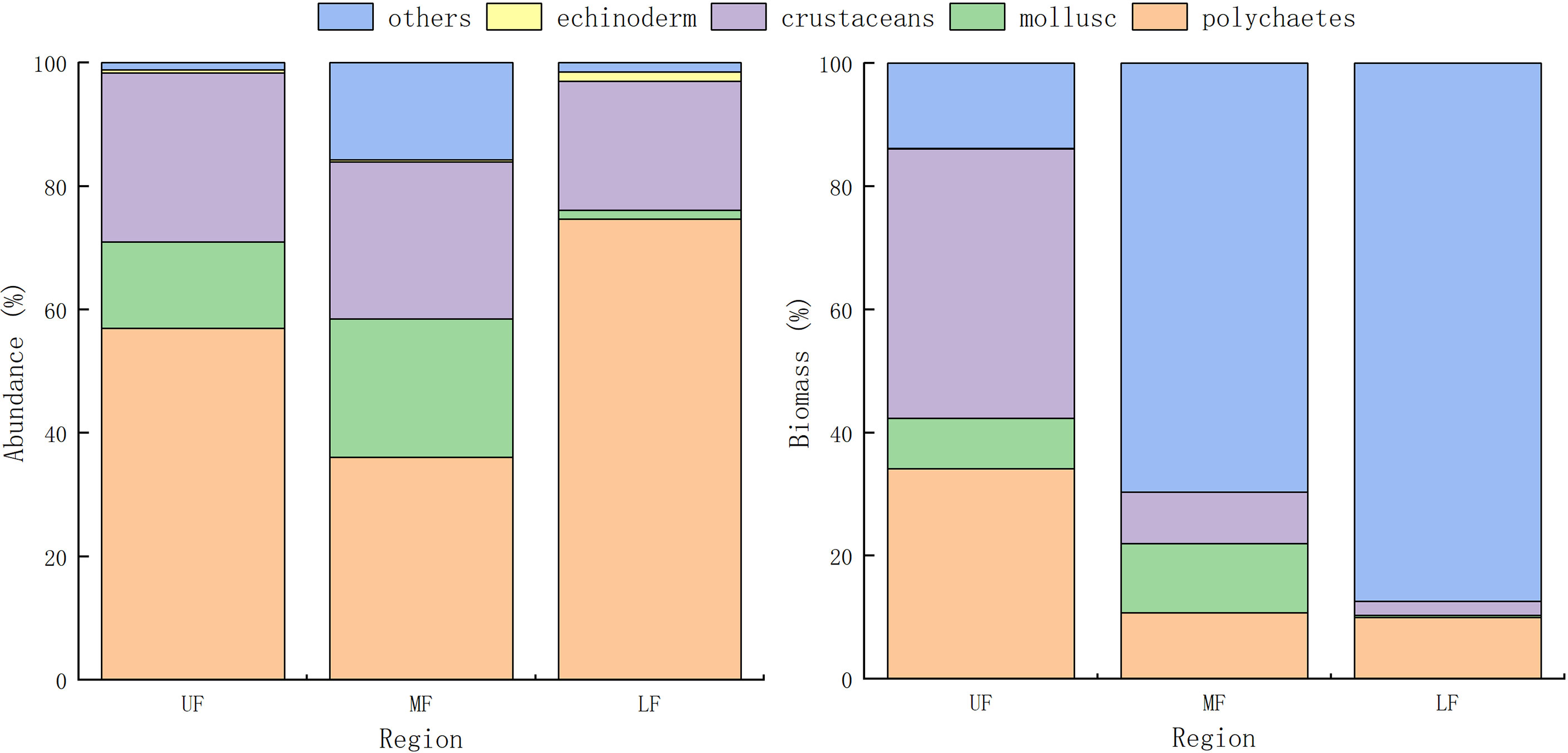
Figure 9 Abundance and biomass precent of macrobenthos in and adjacent to the secondary front, Hangzhou Bay.
SIMPER results for macrobenthos communities in and adjacent to the secondary front were presented in Table 3. Average dissimilarity between regions exceeded 94%, with polychaetes and mollusks contributing most to differences. Species that differ most between the upper- and mid-frontal areas were the polychaetes S. chinensis and M. cincta, and clam P. laevis. Species that differ most between the upper- and lower-frontal areas were the polychaetes A. occidentalis and N. polybranchia, and clam P. laevis. Species that differ most between the mid- and lower-frontal areas were the polychaetes A. occidentalis, S. chinensis, and N. polybranchia.
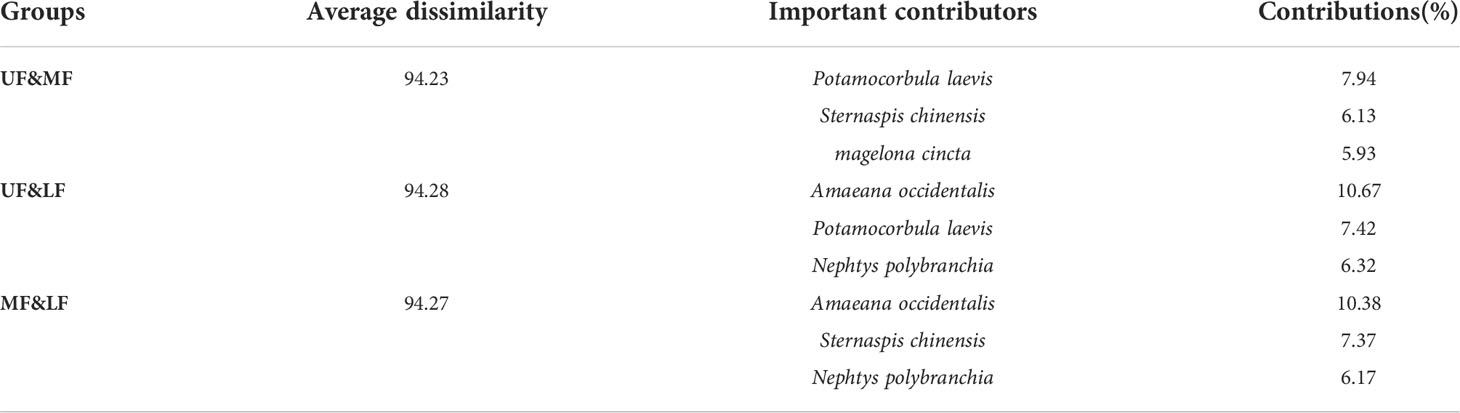
Table 3 Average dissimilarity in community structure, and the three most important contributors in and adjacent to the secondary front, Hangzhou Bay.
ANOSIM results reveal significant differences in community structure in and adjacent to the secondary front (Global R = 0.253, significance level of sample statistic: P = 0.001), and between regions. The difference in community structure between upper- and mid-frontal areas was 0.002, between upper- and lower-frontal areas was 0.001, and between mid- and lower-frontal areas was 0.005.
4 Discussion
4.1 Spatiotemporal variation in Hangzhou Bay macrobenthos communities
Dominant species usually control energy flow and material circulation in communities, and their distributions dominate patterns in community distribution. For example the polychaete S. chinensis was the main contributing species to assemblage structure in B, C, D regions, and had been repeatedly reported as a dominant species in Hangzhou Bay (Yang et al., 2017; Meng et al., 2021). All three regions have a higher percentage of polychaete than region A. The temperature and salinity tolerance of this species is wide, and it has an extensive bathymetric range, occurring in fine sediments from shallow waters to the deep sea (Jumars et al., 2015b).
Spatial heterogeneity in macrobenthos community structure in estuaries was usually affected by sediment composition (Ysebaert et al., 1998) and, especially, salinity (Telesh and Khlebovich, 2010). The macrobenthic distribution taxa and community composition along a salinity gradient was affected mostly by taxon salinity tolerance (Remane, 1934). Across the whole sampling period the bottom-water salinity in Hangzhou Bay was affected by the Qiantang and Yangtze rivers, and tides of the East China Sea, with an obvious salinity gradient existing in bottom water layers. According to Shadrin et al., 2019, macrobenthos abundance has a negative correlation with salinity. In this study, the results of CCA analysis showed Nephtys polybranchia, Magelona japonica, Sabella sp., Heterospio sinica and Amaeana occidentalis have a negative correlation with salinity. The stations with high macrobenthos abundance were mainly concentrated in region B. However, the biomass of macrobenthos was higher in regions with high salinity. So macrobenthos in relation to salinity was that abundance decreases with increasing salinity gradient but biomass increases, increased species and larger individuals.
Sediment grain size affected macrobenthos assemblage structure (Cranfield et al., 2004). Sandy silts were beneficial to the growth and reproduction of some mollusk taxa (Donohue and Irvine, 2003). Sediments in region A were predominantly sandy silts, and mollusk biomass is proportionally high (to 0.4 g m−2). This region was also located in the Qiantang River estuary where water flow was relatively high and many sediment types occur (sediments elsewhere are primarily clay silts). Among all types of sediment, fine-grained fraction (silt and clay) was most sensitive to the impact of benthos (Arlinghaus et al., 2021). In this study silt and clay were mainly distributed in region A, B, and D. The abundance of macrobenthos was higher in all three regions than in region C. Organic carbon contained in sediments represents a food source for estuarine benthos. Soft-sediment communities generally contain species in high densities, and their populations were maintained by high levels of organic matter (Lenihan and Micheli, 2001), with a positive correlation existing between species abundance and organic matter content (Kon et al., 2015). Abundance of species was greatest in region B, then D, and lowest in region A. Low-salinity Yangtze River fresh water, high in suspended solids and nutrients, flows southward from the Yangtze River estuary into regions B and D.
4.2 Feeding guild distributions in Hangzhou Bay
The functional characteristics of macrobenthos were the comprehensive response of marine habitat and environmental quality (Dimitriadis et al., 2012). Macrobenthos communities in Hangzhou Bay were numerically dominated by SSDF feeding-guild taxa. Water quality in Hangzhou Bay was inferior (class IV), had been for two decades, and the environment was seriously eutrophic. Because SDF and SSDF taxa can exploit organic matter in sediments, they often occur in heavily polluted areas enriched in organic matter (Yokoyama, 2002). In regions B, C, and D, the abundance of SDF and SSDF was higher than 38% in all regions. However, in region A, the highest percentage of FF abundance was influenced by the runoff of Qiantang River. CA taxa do not dominated any region. Yan et al., 2021 reported CA feeding-guild taxa to be most abundant in Hangzhou Bay, this probably because they had a larger sampling scale than this study did.
According to Gaudêncio and Cabral (2007), filter feeders and detritivores dominated in the mesohaline area. In this study, FF feeding-guild taxa were particularly abundant in the upper reaches and the north of Hangzhou Bay, where they dominated in mid-salinity areas; their distribution was also related to the mud content of sediments (Gaudêncio and Cabral, 2007).
In several estuarine systems in the North Sea, Hummel et al. (1988); Hamerlynck et al. (2003) and Ysebaert et al. (2003) reported that the trophic structure of benthic assemblages was dominated by suspension feeders in the polyhaline areas and by deposit feeder in the meohaline areas. In the Hangzhou Bay, region A had the fastest flow rate and therefore the highest FF abundance. In regions C and D, where tidal flow velocities were relatively slow, suspended solids deposit onto the seabed. In region C, the abundance and biomass proportion of SSDF feeding-guild taxa was greatest, mainly because the environment most-suitable for this feeding guild was characterized by heterogeneous sediments. Because of current velocities and water-borne suspended matter, of all feeding guilds, herbivores in Hangzhou Bay were both the least abundant and have the lowest biomass. Detritus usually greatly affected food chains in estuaries (García-Arberas and Rallo, 2002), so this study speculate that the ecosystem in Hangzhou Bay was dominated by food webs based on detritus.
It is difficult to identify relationships between patterns in the spatial distribution of macrobenthos feeding guilds and environmental factors, especially to differentiate natural from anthropogenic changes. While hydrodynamic conditions and sediment composition (Probert, 1984; Gaston and Russell, 1987), especially the mud content of sediments, were key factors that affect benthic animal community structure (Eleftheriou and Basford, 1989), the specific combinations of these factors may produce unexplained patterns.
Relationships between the dominance of feeding guilds and food availability were obvious in macrobenthic communities of Hangzhou Bay. The abundance of FF feeding-guild taxa was greatest in the most-turbid environments, and they numerically dominated in estuarine areas with the highest particulate organic carbon levels (Galenne, 1990). However, when suspended solid concentrations in estuarine waters reached 80 mg l−1, mortality of FF feeding-guild taxa increased, their feeding was hindered; Echinoidea and tubular polychaetes feeding on sediments were also negatively affected. High turbidity reduced photosynthesis and the growth capacity of macrobenthos, indirectly affecting SDF and SSDF feeding-guild taxa in high-depositional areas (Kromkamp et al., 1995). In Hangzhou Bay coastal shelf sediments, the spatial distribution of dominant nutrient groups may be related to food supply. SDF and SSDF taxa can consume organic matter from the seabed, and often dominated in organically polluted areas (Yokoyama, 2002). CA feeding-guild taxa do not numerically dominate sediments in Hangzhou Bay, possibly because of tidal current and sediment instability, neither of which is conducive to their survival (Yan et al., 2021). Relatively highly mobile SDF, SSDF and CA feeding-guild taxa can survive in relatively unstable muddy sediments because they can maintain favorable feeding positions (Aller and Stupakoff, 1996).
4.3 Effects of the secondary front on macrobenthos communities in Hangzhou Bay
In the early 1970s, Simpson and Hunter (1974) proposed that a shallow water ocean front in the Irish Sea during summer formed through tidal mixing. Wang and Su. (1993) reported a northeast–southwest front at the mouth of Hangzhou Bay, primarily composed of runoff from the Yangtze and Qiantang rivers, and East China Sea coastal currents. Oceanographic frontal systems were narrow zones with strong physical gradients, which usually have high productivity and can change food supplies in marine food webs (Longhurst, 2010; Mann and Lazier, 2013). A increase in diversity and productivity was a general characteristic of communities associated with these fronts. The species composition in the Hangzhou Bay frontal area was more species rich, and the community structure also differs, it may be influenced by similar processes.
The dominant species were identified for frontal zone and its adjacent area. The polychaetes S. chinensis and H. filiformis, and gammaridean amphipods dominated in the frontal zone, whereas that of the upper frontal area was dominated by the clam P. laevis, amphipod C. sinensis, and other decapod crustaceans, and that of the lower frontal area was dominated by the polychaetes A. occidentalis, N. polybranchia, and M. japonica. The numbers of macrobenthic species, and their total abundance and biomass in the frontal area were higher than adjacent area. Owen (1981); Bakun (2006); Cao et al. (2021) pointed out that owing to frontogenesis of the fronts and eddies in coastal oceans, the nutrient input into the coastal and bay aera seasonally and induced higher production of food organisms (such as phytoplankton and zooplankton), which had a stronger influence on the macrobenthos diversity, abundance and assemblage structure.
Compared with the secondary front in Hangzhou Bay, the study of community differences between the frontal system and surrounding areas was usually performed at a higher scale (de Nooijer et al., 2008). Differences in community structure and biomass in these areas may be more obvious at larger scales because of differences in food supply (Dauwe et al., 1998). The interaction of plankton at the sub-mesoscale frontal area may be very important for building the biodiversity and community composition of planktonic ecosystems (Liu et al., 2018), while plankton and macrobenthos were closely related in shallow water areas (Gascón et al., 2007). Organic matter and detritus can be exported from and settled on the seabed through the follow-up path of phytoplankton and “biological pump” (Longhurst and Glen Harrison, 1989). Therefore, the impact of the frontal area on plankton and water temperature environment will be transmitted to macrobenthos communities.
Marine physical processes, such as the formation of fronts, affected the patterns in distribution of marine organisms at all trophic levels. As organisms grow in size, they occupied higher nutrient levels, and behavioral habits became increasingly important (McManus and Woodson, 2012). In the frontal area, non-or only weakly swimming organisms such as plankton can passively accumulate and grow because of highly abundant nutritional foods (Woodson and McManus, 2007). There was a strong coupling between the front and plankton. However, this association becomes more complex at higher trophic levels. The ability of biological behavior and availability in the high trophic level may determine the distribution pattern (McManus and Woodson, 2012). Primary producers respond directly to the front because of highly nutritious food sources and light conditions, but at higher trophic levels, the sensory inducements of biological behavior (such as temperature, salinity, light conditions and trace substances) will find the front structure.
5 Conclusion
Four regions were divided according to salinity and sediment distribution and picked out the frontal zone and adjacent areas. The spatial pattern of macrobenthos in Hangzhou Bay was that abundance of macrobenthos was highest in the mid-salinity region and decreased with increasing salinity in Hangzhou Bay. However, increasing biomass and higher abundance of macrobenthos were observed in silt and clay sediments comparing to the other sediment grain. The highest percentage of FF feeding groups was found in the areas with the most rapid currents (region A). SDF and SSDF accounted for the greatest abundance in region B, C, D. The number of macrobenthic species, abundance and biomass were higher in the frontal zone than in adjacent areas.
Data availability statement
The original contributions presented in the study are included in the article/Supplementary Material. Further inquiries can be directed to the corresponding authors.
Ethics statement
Ethical review and approval was not required for this study as only field surveys were undertaken.
Author contributions
KJ made substantial contributions to draft the manuscript. YT made substantial contributions to the data analysis. QL and RZ made substantial contributions to participate the manuscript discussion. YL, LS, JZ, QC, and JC made substantial contributions to the identification samples and data acquisition of environmental factors. All authors contributed to the article and approved the submitted version.
Funding
The present work was supported financially by the Scientific Research Fund of the Second Institute of Oceanography, MNR,China (SZ2001, JG2209), the Key R&D Program of Zhejiang (Grant No. 2022C03044), the National Key Research and Development Program of China (No. 2021YFC3101702), National Programme on Global Change and Air-Sea Interaction (Phase II)—Hypoxia and Acidification Monitoring and Warning Project in the Changjiang Estuary and the Natural Science Foundation of Zhejiang Province under contract No. LQ19D060004.
Acknowledgments
We thank Steve O’Shea, PhD, from Edanz (https://www.edanz.com/ac), for editing a draft of this manuscript. We thank the Observation and Research Station of Yangtze River Delta Marine Ecosystems of Ministry of Natural Resources (http://yangtzeriverestuary.soed.org.cn/) for providing the crew members to conduct the surveys.
Conflict of interest
The authors declare that the research was conducted in the absence of any commercial or financial relationships that could be construed as a potential conflict of interest.
Publisher’s note
All claims expressed in this article are solely those of the authors and do not necessarily represent those of their affiliated organizations, or those of the publisher, the editors and the reviewers. Any product that may be evaluated in this article, or claim that may be made by its manufacturer, is not guaranteed or endorsed by the publisher.
Supplementary material
The Supplementary Material for this article can be found online at: https://www.frontiersin.org/articles/10.3389/fmars.2022.1037287/full#supplementary-material
References
Aller J. Y., Stupakoff I. (1996). The distribution and seasonal characteristics of benthic communities on the Amazon shelf as indicators of physical processes. Continental Shelf Res. 16 (5-6), 717–751. doi: 10.1016/0278-4343(96)88778-4
Arlinghaus P., Zhang W., Wrede A., Schrum C., Neumann A. (2021). Impact of benthos on morphodynamics from a modeling perspective. Earth-Sci. Rev. 221, 103803. doi: 10.1016/j.earscirev.2021.103803
Azovsky A. I., Kokarev V. N. (2019). Stable but fragile: long-term dynamics of arctic benthic macrofauna in baydaratskaya bay (the kara Sea). Polar Biol. 42 (7), 1307–1322. doi: 10.1007/s00300-019-02519-y
Bakun A. (2006). Fronts and eddies as key structures in the habitat of marine fish larvae: opportunity, adaptive response and competitive advantage. Scientia Marina 70 (S2), 105–122. doi: 10.3989/scimar.2006.70s2105
Bao C., Huang B., Shao J., Cai Q., Mu Q., Murk A. J. (2021). Spatial and temporal distribution of toxic compounds in sediments and potential ecological effects on macrobenthic faunal species in hangzhou bay from 2003 to 2015. Mar. Pollut. Bull. 172, 112816. doi: 10.1016/j.marpolbul.2021.112816
Calle P., Monserrate L., Medina F., Calle Delgado M., Tirapé A., Montiel M., et al. (2018). Mercury assessment, macrobenthos diversity and environmental quality conditions in the salado estuary (Gulf of guayaquil, Ecuador) impacted by anthropogenic influences. Mar. Pollut. Bull. 136, 365–373. doi: 10.1016/j.marpolbul.2018.09.018
Cao L., Tang R., Huang W., Wang Y. (2021). Seasonal variability and dynamics of coastal sea surface temperature fronts in the East China Sea. Ocean Dynam. 71 (2), 237–249. doi: 10.1007/s10236-020-01427-8
Cranfield H. J., Rowden A. A., Smith D. J., Gordon D. P., Michael K. P. (2004). Macrofaunal assemblages of benthic habitat of different complexity and the proposition of a model of biogenic reef habitat regeneration in foveaux strait, new Zealand. J. Sea Res. 52 (2), 109–125. doi: 10.1016/j.seares.2003.12.003
Dauwe B., Herman P., Heip C. (1998). Community structure and bioturbation potential of macrofauna at four north Sea stations with contrasting food supply. Mar. Ecol. Prog. Ser. 173, 67–83. doi: 10.3354/meps173067
de Nooijer L. J., Duijnstee I., Bergman M., van der Zwaan G. (2008). The ecology of benthic foraminifera across the Frisian front, southern north Sea. Estuarine Coast. Shelf Sci. 78 (4), 715–726. doi: 10.1016/j.ecss.2008.02.015
Dimitriadis C., Evagelopoulos A., Koutsoubas D. (2012). Functional diversity and redundancy of soft bottom communities in brackish waters areas: Local vs regional effects. J. Exp. Mar. Biol. Ecol. 426-427, 53–59. doi: 10.1016/j.jembe.2012.05.016
Dolbeth M., Cardoso P. G., Ferreira S. M., Verdelhos T., Raffaelli D., Pardal M. A. (2007). Anthropogenic and natural disturbance effects on a macrobenthic estuarine community over a 10-year period. Mar. pollut. Bull. 54 (5), 576–585. doi: 10.1016/j.marpolbul.2006.12.005
Donohue I., Irvine K. (2003). Effects of sediment particle size composition on survivorship of benthic invertebrates from lake Tanganyika, Africa. Archiv Fur Hydrobiol. 157 (1), 131–144. doi: 10.1127/0003-9136/2003/0157-0131
Ecoutin J. M., Simier M., Albaret J. J., La R., Morais L. (2010). Changes over a decade in fish assemblages exposed to both environmental and fishing constraints in the sine saloum estuary (Senegal). Estuar. Coast. Shelf Sci. 87 (2), 284–292. doi: 10.1016/j.ecss.2010.01.009
Eleftheriou A., Basford D. J. (1989). Abundance and biomass of macrobenthic infauna of the offshore northern north Sea. Journal of the Marine Biological Association of the United Kingdom 69 (1), 123–143. doi: 10.1017/S0025315400049158
Galenne G. (1990). Proceedings of the international seminar on the environmental aspects of dredging activities Nantes (France), 27 November - 1 December 1989. J. Pharm. 3 (3), 2460–2462.
García-Arberas L., Rallo A. (2002). The intertidal soft-bottom infaunal macrobenthos in three Basque estuaries (Gulf of biscay): a feeding guild approach. Hydrobiologia 475-476 (1), 457–468. doi: 10.1007/978-94-017-2464-7_35
Gascón S., Brucet S., Sala J., Boix D., Quintana X. D. (2007). Comparison of the effects of hydrological disturbance events on benthos and plankton salt marsh communities. Estuarine Coast. Shelf Sci. 74 (3), 419–428. doi: 10.1016/j.ecss.2007.04.031
Gaston, Russell G. (1987). Benthic polychaeta of the middle Atlantic bight: Feeding and distribution. Mar. Ecol. Prog. 36 (3), 251–262. doi: 10.3354/meps036251
Gaudêncio M. J., Cabral H. N. (2007). Trophic structure of macrobenthos in the tagus estuary and adjacent coastal shelf. Hydrobiologia 587 (1), 241–251. doi: 10.1007/s10750-007-0686-6
Ghodrati Shojaei M., Gutow L., Dannheim J., Rachor E., Schröder A., Brey T. (2016). Common trends in German bight benthic macrofaunal communities: Assessing temporal variability and the relative importance of environmental variables. J. Sea Res. 107, 25–33. doi: 10.1016/j.seares.2015.11.002
González-Ortegón E., Subida M.D., Arias A.M., Baldó F., Cuesta J.A., Fernández-Delgado C., et al. (2012). Nekton response to freshwater inputs in a temperate European estuary with regulated riverine inflow. Sci. Total Environ. 440 (1), 261–271. doi: 10.1016/j.scitotenv.2012.06.061
Hamerlynck O., Mees J., Craeymeersch J., Soetaert K., Hostens K., Cattrijsse A., et al. (2003). The westerschelde estuary: two food webs and a nutrient rich desert. Progress in Belgian Oceanographic Research, 217. doi: 10.1016/S0065-2504(08)60194-4
Herman P., Middelburg J., Van de Koppel J., Heip C. (1999a) Ecology of estuarine macrobenthos. Adv. Ecol. Re. 29 (780), 195–240. doi: 10.1016/s0065-2504(08)60194-4
Hummel H., Moerland G., Bakker C. (1988). The concomitant existence of a typical coastal and a detritus food chain in the westerschelde estuary. Hydrobiol. Bull. 22 (1), 35–41. doi: 10.1007/bf02256780
Hu Y., Yu Z., Zhou B., Li Y., Yin S., He X., et al. (2019). Tidal-driven variation of suspended sediment in hangzhou bay based on GOCI data. Int. J. Appl. Earth Observation Geoinfo. 82, 101920. doi: 10.1016/j.jag.2019.101920
Jumars P. A., Dorgan K. M., Lindsay S. M. (2015a). Diet of worms emended: An update of polychaete feeding guilds. Annu. Rev. Mar. Sci. 7 (1), 497. doi: 10.1146/annurevmarine-010814-020007
Jumars P. A., Dorgan K. M., Lindsay S. M. (2015b). Diet of worms emended: an update of polychaete feeding guilds. Ann. Rev. Mar. Sci. 7, 497–520. doi: 10.1146/annurev-marine-010814-020007
Kon K., Tsuchiya Y., Sato T., Shinagawa H., Yamada Y. (2015). Role of microhabitat heterogeneity in benthic faunal communities in sandy bottom sediments of oura bay, shimoda, Japan. Regional Stud. Mar. Sci. 2, 71–76. doi: 10.1016/j.rsma.2015.05.007
Kromkamp J., Peene J., Rijswijk P., Sandee A., Goosen N. (1995). Nutrients, light and primary production by phytoplankton and microphytobenthos in the eutrophic, turbid westerschelde estuary (The Netherlands). Hydrobiologia 311 (1-3), 9–19. doi: 10.1007/BF00008567
Laprise R., Dodson J. J. (1993). Nature of environmental variability experienced by benthic and pelagic animals in the st. Lawrence estuary, Canada. Mar. Ecol. Prog. Ser. 94 (2), 129–139. doi: 10.3354/meps094129
Le Gallo J., Ertur C. (2003). Exploratory spatial data analysis of the distribution of regional per capita GDP in Europe 1980–1995. Pap. regional Sci. 82 (2), 175–201. doi: 10.1111/j.1435-5597.2003.tb00010.x
Liu J., Cao K., Yin P., Gao F., Chen X., Zhang Y., et al. (2018). The sources and transport patterns of modern sediments in hangzhou bay: Evidence from clay minerals. J. Ocean Univ. China 17 (6), 1352–1360. doi: 10.1007/s11802-018-3710-8
Longhurst A. R. (1989). The biological pump: Profiles of plankton production and consumption in the upper ocean. Prog. Oceanogr. 22 (1), 47–123. doi: 10.1016/0079-6611(89)90010-4
Macdonald T. A., Burd B., Macdonald V., Van Roodselaar A. (2010). Taxonomic and feeding guild classification for the marine benthic macroinvertebrates of the strait of Georgia, British columbia. Fish. Oceans Canada= Pêches océans Canada.
Mann K. H., Lazier J. R. (2013). Dynamics of marine ecosystems: biological-physical interactions in the oceans (British: John Wiley & Sons, Mann).
McManus M. A., Woodson C. B. (2012). Plankton distribution and ocean dispersal. J. Exp. Biol. 215 (6), 1008–1016. doi: 10.1242/jeb.059014
Meng Z., Han Q., Wang X. (2021). Distribution pattern of macrobenthic composition, diversity and secondary production in hangzhou bay, northern East China Sea. Regional Stud. Mar. Sci. 10), 101956. doi: 10.1016/j.rsma.2021.101956
Owen R. W. (1981). Fronts and eddies in the sea: mechanisms, interactions and biological effects. Anal. Mar. Ecosyst. 197–233. doi: 10.1.1.464.9887
Patrício J., Adão H., Neto J. M., Alves A. S., Traunspurger W., Marques J. C. (2012). Do nematode and macrofauna assemblages provide similar ecological assessment information? Ecol. Indic. 14 (1), 124–137. doi: 10.1016/j.ecolind.2011.06.027
Paul K. S. S., Kari E. E. (2004). Spatial patterns of soft-sediment benthic diversity in subtropical Hong Kong waters. Mar. Ecol. Prog. Ser. 276, 25–35. doi: 10.3354/meps276025
Probert P. K. (1984). Disturbance, sediment stability, and trophic structure of soft-bottom communities. J. Mar. Res. 42 (4), 893–921. doi: 10.1357/002224084788520837
Remane A. (1934). Die brackwasserfauna. Mit besonderer Berücksichtigung der Ostsee. (Zoologischer Anzeiger).
Rethinam Subramanian >P.R., Retnamma J., Nagarathinam A., Loganathan J., Singaram P., Chandrababu V. (2021). Seasonality of macrobenthic assemblages and the biotic environmental quality of the largest monsoonal estuary along the west coast of India. Environ. Sci. pollut. Res. 28 (28), 37262–37278. doi: 10.1007/s11356-021-13144-w
Shadrin N., Kolesnikova E., Revkova T., Latushkin A., Chepyzhenko A., Dyakov N., et al. (2019). Macrostructure of benthos along a salinity gradient: The case of sivash bay (the Sea of Azov), the largest hypersaline lagoon worldwide. J. Sea Res. 154, 101811. doi: 10.1016/j.seares.2019.101811
Simpson J. H., Hunter J. R. (1974). Fronts in the Irish Sea. Nature 250 (5465), 404–406. doi: 10.1038/250404a0
Su J. L., Wang K. S. (1989). Changjiang river plume and suspended sediment transport in Hangzhou Bay. Continental Shelf Research 9 (1), 93–111. doi: 10.1016/0278-4343(89)90085-X
Telesh I. V., Khlebovich V. V. (2010). Principal processes within the estuarine salinity gradient: A review. Mar. pollut. Bull. 61 (4), 149–155. doi: 10.1016/j.marpolbul.2010.02.008
Wang KS., Su J. L. (1993). Hydrographic features of the plume front in the Hangzhou Bay. Acta oceanologica sinica (1), 12 .
Woodson C., McManus M. (2007). Foraging behavior can influence dispersal of marine organisms. Limnol. Oceanogr. 52 (6), 2701–2709. doi: 10.4319/lo.2007.52.6.2701
Wu B., Jin H., Gao S., Xu J., Chen J. (2019). Nutrient budgets and recent decadal variations in a highly eutrophic estuary: Hangzhou bay, China. J. Coast. Res. 36 (1), 63–71. doi: 10.2112/JCOASTRES-D-18-00071.1
Xie D.-f., Gao S., Wang Z.-b., Pan C.-h. (2013). Numerical modeling of tidal currents, sediment transport and morphological evolution in hangzhou bay, China. Int. J. Sediment Res. 28 (3), 316–328. doi: 10.1016/s1001-6279(13)60042-6
Xie D., Pan C., Wu X., Gao S., Wang Z. B. (2017). Local human activities overwhelm decreased sediment supply from the changjiang river: Continued rapid accumulation in the hangzhou bay-qiantang estuary system. Mar. Geol. 392, 66–77. doi: 10.1016/j.margeo.2017.08.013
Yang L., Huang R., Lu Z., Xu K., Wang Z., Zhou Y., et al (2017). The distribution of macrobenthos and the relationship with environmental factors in the waters around Jintang Island. J. Zhejiang Ocean University. 36 (4), 295–301. doi: 10.3969/j.issn.1008-830X.2017.04.003
Yan R., Wang X., Wang C., Han Q. (2021). Spatial and temporal distributions of macrobenthic feeding guilds and their influencing factors in hangzhou bay and its adjacent areas. Regional Stud. Mar. Sci. 48, 102029. doi: 10.1016/j.rsma.2021.102029
Yokoyama H. (2002). Impact of fish and pearl farming on the benthic enviroments in gokasho bay: Evaluation from seasonal fluctuations of the macrobenthos. Fish. Sci. 68 (2), 258–268. doi: 10.1046/j.1444-2906.2002.00420.x
Ysebaert T., Herman P., Meire P., Craeymeersch J., Verbeek H., Heip C., et al (2003). Large-scale spatial patterns in estuaries: estuarine macrobenthic communities in the Schelde estuary, NW Europe. Estuarine, coastal and shelf Science 57 (1–2), 335–355. doi: 10.1016/S0272-7714(02)00359-1
Keywords: macrobenthos, spatial-temporal distribution, feeding guild, Hangzhou Bay, marine front
Citation: Jia K, Tang Y, Liu Q, Zhang R, Liao Y, Shou L, Zeng J, Chen Q and Chen J (2022) Spatial and temporal distribution of macrobenthos communities and their relationship with secondary front in Hangzhou Bay. Front. Mar. Sci. 9:1037287. doi: 10.3389/fmars.2022.1037287
Received: 05 September 2022; Accepted: 13 October 2022;
Published: 28 October 2022.
Edited by:
Luzhen Chen, Xiamen University, ChinaCopyright © 2022 Jia, Tang, Liu, Zhang, Liao, Shou, Zeng, Chen and Chen. This is an open-access article distributed under the terms of the Creative Commons Attribution License (CC BY). The use, distribution or reproduction in other forums is permitted, provided the original author(s) and the copyright owner(s) are credited and that the original publication in this journal is cited, in accordance with accepted academic practice. No use, distribution or reproduction is permitted which does not comply with these terms.
*Correspondence: Yibo Liao, bGlhb3liQHNpby5vcmcuY24=; Lu Shou, c2hvdWx1OTgxQHNpby5vcmcuY24=