- 1Marine Data Technology Hub, College of Science and Engineering, James Cook University, Townsville, QLD, Australia
- 2Centre for Tropical Water and Aquatic Ecosystem Research (TropWATER), College of Science and Engineering, James Cook University, Townsville, QLD, Australia
Urban-industrial seascapes are prevalent around the world, yet we lack a basic understanding of how the mosaic of different habitats in these areas are used by mobile marine fauna, including features such as industrial ports and marinas. Urbanised areas have been alternately characterised in scientific literature as degraded, depauperate, or in some cases diverse and abundant. To advance our spatial and temporal understanding of the community of mobile marine fauna in these areas, we used repeated sonar image sampling over large swathes of two urban-industrial seascapes, combined with underwater video. 2,341 sonar segments were captured across Mackay Harbour and the Pioneer River estuary (North Queensland, Australia). We used this information to generate a preliminary understanding of the ecology of these locations. We found that overall, urban-industrial seascapes can contain counts of mobile marine fauna similar to natural areas, and that these seascapes are characterised by dynamic diel shifts in the spatial arrangement of mobile marine fauna in the water column. At night, large fish are prevalent in the water column, while during the day, assemblages are dominated by small fish. Within these urban-industrial seascapes, deeper areas containing heavy infrastructure such as ports can harbour large densities of fish, including heavily targeted fisheries species. These areas deserve recognition as marine habitat, are of consequence to fisheries, and have the potential to influence surrounding ecosystems. Important research questions remain regarding their impact on food webs and animal movement at larger scales.
1 Introduction
Human activities are reshaping coastal ecosystems in diverse ways, changing the ecosystem services they provide (Worm et al., 2006). Marine urbanisation is proceeding rapidly (Bulleri and Chapman, 2010; Waltham and Sheaves, 2015). This includes ocean sprawl (sensu Todd et al., 2019); the extension of urban development and industrial infrastructure into the marine environment, usually through the reshaping and linearization of shorelines, and addition of hard and complex structures to estuaries, bays and beaches (Lai et al., 2015; Bishop et al., 2017). This leads to a mosaic of artificial structures like rock walls, ports, tidal turbines or pipelines, intermixed with depositional or dredged soft bottom habitats and remanent or colonised vegetated habitats (Gill, 2005; Airoldi et al., 2020; Claassens et al., 2022).
There are conflicting ideas in the scientific literature around the nature of the new urban marine environments we are creating; these areas could reasonably be understood as degraded and depauperate locations, similar to natural areas, or, alternatively, sites of enhanced productivity and biodiversity (Airoldi et al., 2020). In the process of marine urbanisation, ocean sprawl is often accompanied by increased resource exploitation and new pollution pathways (Burt and Bartholomew, 2019; Todd et al., 2019). Marine urbanisation has a wide range of effects on marine ecosystems, including homogenisation of environment, changes in biodiversity and productivity, the creation of novel assemblages, and the proliferation of species that benefit from human modification of the environment, such as ruderal species, invasive species and synanthropes (Todd et al., 2019). Some of the most serious threats to coastal ecosystems stem from marine urbanisation (Sheppard et al., 2010; Van Lavieren et al., 2011). But, along with the negative effects, urban and industrial habitats can also provide the foundation for diverse and abundant marine communities (Feary et al., 2011; Burt and Bartholomew, 2019), support endangered species (Claassens, 2016), and enhance fish recruitment (Pastor et al., 2013). The kind of habitats these new areas form is entirely context-dependent (sensu Bradley et al., 2020), and can result in heavily degraded and depauperate systems, novel systems with enhanced diversity and productivity, or something in between (Todd et al., 2019). This variety of responses complicates simple negative ideas around novel anthropogenic ecosystems, which can provide vital habitat (Maclagan et al., 2018), and reorder relationships across whole landscapes in complex ways (Plaza and Lambertucci, 2017).
Large industrial infrastructure such as ports, which often form part of urbanised seascapes, represent a gap in our ecological knowledge of ocean sprawl. Our understanding of these areas can only be inferred from studies of other urban-industrial environments (e.g. marinas, jetties), purpose-built artificial reefs, fish aggregating devices, and dec-commissioned oil rigs (Clynick, 2008; McLean et al., 2022). Depending on the local context, large, complex, artificial structures in the marine environment are often recognised as locations of high productivity and diversity. Pilings and pontoons have a strong attractive effect on the distribution of mobile fauna such as fish (Lindegarth, 2001; Clynick, 2008; Waltham et al., 2022). Artificial reefs tend to support similar levels of faunal biomass and diversity as natural reefs, and can have high rates of secondary productivity (Smith et al., 2016; Paxton et al., 2020). Oil and gas infrastructure can also support high levels of biodiversity and secondary productivity, due to high levels of recruitment, growth and retention of fauna (Claisse et al., 2014; Torquato et al., 2017). Heavy infrastructure, like ports, which provide structural complexity at dimensions that far exceed natural habitats, have the potential to create novel and productive ecosystems, but are also likely to be negatively impacted by urbanisation (Brook et al., 2018). Ports situated within highly modified urban seascapes sit outside our current understanding of artificial structures as available habitat for marine species, with only a few studies that have examined the biodiversity values and services provided by these obvious habitat features in the coastal zone (Francis et al., 2005; Selfati et al., 2018; Adams et al., 2021).
Conflicting ideas of degradation vs enhancement in urban-industrial seascapes need to be resolved. Depending on the extent of modification, these urban-industrial seascapes likely present hybrid or novel ecosystems (sensu Hobbs et al., 2009), so in contrast to well-studied natural ecosystems, we lack the background information to generate reasonable hypotheses, and understand new data (Sheaves et al., 2021a). While understanding the ecosystem is the overarching task, here we examine the community of mobile marine fauna in these areas, with a focus on mobile vertebrates including bony fish, elasmobranchs, reptiles and mammals. Our understanding of mobile marine fauna in these seascapes is often limited by sampling approaches (e.g. diver surveys or remote underwater videos) which provide detailed information over a spatially limited area, with limited temporal representation, and often excluding most of the water column. Before fine-scale data can be understood, we need answers to basic questions, such as: ‘Do these areas usually contain fish? How does their presence vary over time? In what way does animal presence relate to local habitat features, or do factors at a larger scale influence animal presence?’ We used repeated sonar imaging over large swathes of two tropical Australian urban-industrial seascapes to rapidly assess spatial and temporal occupation by mobile marine fauna. We use this data to characterize the community and generate testable hypotheses about the ecology of these types of seascapes.
2 Materials and methods
2.1 Study location
This study was conducted across two locations, Mackay Harbour and the Pioneer River estuary, within the urban-industrial landscape of Mackay, a city on the tropical east coast of northern Australia (Figure 1). The coastline of Mackay borders the Great Barrier Reef World Heritage Area, and the coastal and barrier reef ecosystems in this region are intimately connected through the movement of productivity, nutrients, sediments and fauna (Alongi and McKinnon, 2005), with many animals completing their lifecycles across this interconnected mosaic of habitats (e.g. Sheaves and Molony, 2000; Bradley et al., 2019). The area experiences a maximum tidal range greater than 6m, and experiences highly seasonal rainfall between December and March. Sampling occurred between November 2021 (pre-wet) and April 2022 (post-wet).
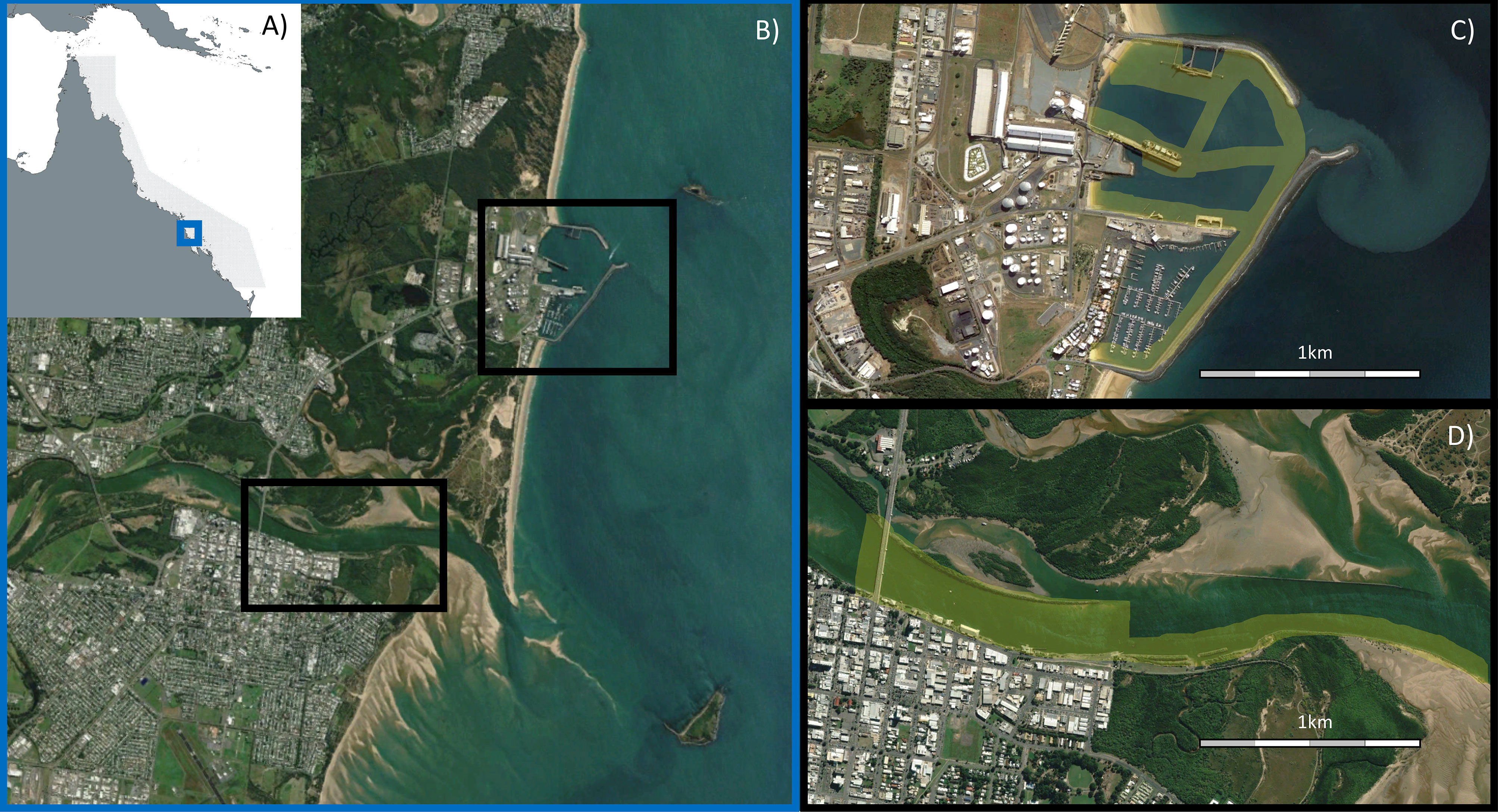
Figure 1 (A) Blue box shows location of Mackay along the Queensland coast (21°07’ S, 149°12’ E), adjacent to the Great Barrier Reef World Heritage Area (grey shaded area); (B) Black boxes show location of the two urban-industrial areas examined within Mackay; (C) Mackay Harbour; (D) Pioneer River. In (C, D), yellow shading shows areas sampled using sonar. Images sourced from Google Earth, CNES/Airbus.
2.1.1 Mackay Harbour
Mackay Harbour is entirely man-made, constructed by partially enclosing a section of natural beach in the mid-1900s. There are no significant freshwater inputs, except during rainfall where stormwater runoff drains the surrounding hardstand area of this facility. Mackay Harbour contains a marina and port with four shipping wharves, semi-enclosed by a rock seawall. The marina contains >400 berths and has a typical depth of 5m, while the multi-cargo import and export port features sugar and fuel terminals as well as break-bulk wharves. Port waters have a typical depth of 12m, down to 20m at each wharf. In the marine environment these facilities create a mosaic of extensive rock walls, open areas of soft sediment and clusters of concrete marine pilings (referred to generically as ‘pylons’ in this article) at different densities.
2.1.2 Pioneer River estuary
The Pioneer River drains a large catchment (>1000km2) dominated by sugarcane agriculture, before flowing through the city of Mackay and entering the Coral Sea (Lewis et al., 2021). We surveyed the lower, tidally dominated section of the river estuary from the city downstream towards the mouth. This area comprises long sections of engineered rock wall, armoured urban shoreline, bridge pylons, deeper channels (max depth surveyed 7m), and large areas of intertidal sand flats and mangrove forests. This section is connected to less urbanised areas of the estuary, upstream freshwater habitats and downstream marine habitats.
2.2 Sonar imaging
Sonar imaging was used to identify and quantify mobile marine fauna, with a focus on vertebrates including bony fish, elasmobranchs, reptiles and mammals. A sonar pulse travels through the water column and is reflected off underwater objects, to produce a 2-dimensional image. See Daniel et al. (1998) for detailed description. This technology is commonly used to map benthic habitats (e.g. Durá et al., 2004) but is also used to estimate fish densities (e.g. Gerlotto et al., 2000; Vine et al., 2019), particularly in species specific surveys (e.g. Gonzalez-Socoloske et al., 2009; Papastamatiou et al., 2020). The utility of sonar imaging in surveying mobile marine fauna has been trailed and tested successfully, including on known tethered or captive animal targets, demonstrating that consumer grade side-scan sonar can detect entire body structures of fish and sharks, including fine structures like caudal, pelvic and pectoral fins (Fleming et al., 2018; Barnett et al., 2022). This technique is unlikely to provide effective detection of smaller animals, invertebrates, and highly bottom associated mobile marine fauna. Sonar surveys in stocked ponds can yield fish abundance estimates within 10% of traditional mark-recapture estimates (Fleming et al., 2018). A preliminary methodology for mobile marine fauna community surveys has recently been developed (Barnett et al., 2022). A Garmin Echomap Ultra 125 side-imaging device was used at 1070 kHz, at a boat speed of 4-6 knots. Both side-imaging and down-imaging were captured. The resulting image data is time-stamped and geolocated. Side-imaging provides wide coverage of benthic topography, allowing midwater objects to cast accurate shadows. Down-imaging provides a high-definition coverage of the water column immediately underneath the boat. This technique provides limited detail on faunal identity (see sonar processing and interpretation below), but allows for a record of the entire water column over large spatial scales (kilometres).
2.3 Sonar sampling
Sonar runs were determined by geolocated tracks, and sonar imaging was collected continuously over large distances (kilometres) and then broken into standardised segments for analysis. Multiple (non-independent) segments were carried out in succession. Each standardised segment was 20m in length. Sonar runs were conducted on multiple occasions during each sampling trip, with each site sampled twice (in opposite directions) during each sampling event, and across day and night. Due to logistical constraints such as ship movements, totally orthogonal sampling was not possible for every location. Subsets of the total data set were examined to answer specific questions (see Supplementary Tables S1-S4).
2.4 Sonar processing and interpretation
Sonar imaging recordings were visually inspected to identify detected animals, count them, and record their schooling behaviour and location within the water column. Garmin HomePort™ software was used to display traditional, down-view and side-view images, and to geolocate this information. Sonar imagery of the water column (both traditional down-imaging and side-scanning sonar) were visually inspected to detect mobile marine fauna within a segment. Only fauna in the water column directly under the boat were recorded, side imaging was not used to detect fauna in the swath on either side of the boat, as these are not easily distinguished from the benthos. Following the broad typologies developed in Barnett et al. (2022), we used the following categories; small fish, large fish and megafauna (see Figure 2). The movement and orientation of the animal, gas in the swim bladder and boat speed, will all affect the size and intensity of returned signal (Horne, 2000; Fleming et al., 2018), so only broad categories like these can be used with confidence. Individuals in each size category were manually counted, acknowledging that when present in high densities this would result in underestimation. Mobile marine fauna detected in the water column were further classified according to their apparent grouping behaviour (schooling or scattered) and their location within the water column (bottom associated vs water column associated). This provided an assessment of the assemblage of mobile marine fauna and their spatial arrangement in the water column. We only distinguished between animal types based very coarsely on their size, and between obviously different spatial arrangements of those animal types in the water column. Thus, while we could not determine, for example, whether large fish scattered on the bottom were of a different species to large fish schooling in the water column, we could determine that the spatial arrangement of the assemblage of mobile marine fauna in the water column was different – if these differences are consistent, this implies meaningful ecological difference, regardless of species identity.
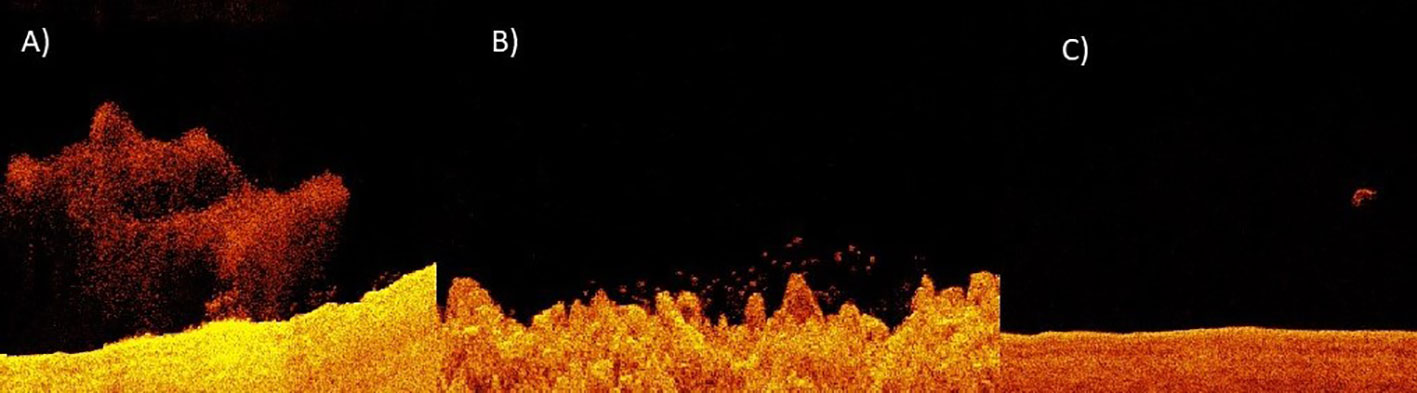
Figure 2 Sonar imaging depicting (A) an example of small schooling fish, defined by water column detections that are dimensionless; (B) large fish, defined by water column detections that are large enough to exhibit a distinctive shape; and (C) megafauna, defined by solid water column detections that appeared to exceed 1m in any direction. Often it is not possible to distinguish the identity of megafauna, but they are likely to be large bony fish, sharks, rays, dolphins, turtles, or dugongs.
Sonar imagery of the water column and the bottom (side-scanning sonar) was used to determine habitat features within a segment. From these images we broadly classified bottom habitat features through qualitative visual assessment. The habitat features defined were rubble (unconsolidated, detectable grainsize, Figure 2A), rock (boulder and consolidated rock, hard sonar return, Figure 2B), soft bottom (smooth sonar return - grainsize undetectable, Figure 2C), and the number or absence of pylons in the water column (0, 1-2 or >2 per 20m segment).
2.5 Video sampling and processing
To compliment the interpretation of sonar sampling, we sought to build a list of species found in the areas under investigation. To identify the range of fish species that occurred in each area (marina, harbour walls, ports, estuary), camera arrays were used to record underwater video. We used both bottom-set RUVs and mid-water RUVs, deployed from a boat or from shore. Units were deployed for at least 15 minutes. Video sampling was not comprehensive or stratified, and RUVs were deployed opportunistically during sampling periods of adequate visibility conditions. In many instances, video and sonar were collected concurrently, allowing for confidence that there was true overlap in the fauna detected in both methods. This resulted in 91 video samples. Video surveys were completed in accordance with Ethics Approval (JCU A2615). Videos were viewed and all discernible species identities were recorded. We added to these observations taxa that were observed directly from shore or boat, including dolphins, which are rarely recorded on video and are commonly surveyed by direct observation. This produced a non-exhaustive list of species known to be present in each area (Table S5).
2.6 Data analysis
We used an exploratory approach, aimed at letting the dominant patterns of mobile marine fauna across time and space reveal important factors in the seascapes examined. First, we calculated total counts of fauna per ~2 km sonar run. Second, we examined where fauna was detected vs where no fauna was detected, relative to a range of factors (site, trip, day/night, habitat and depth) by looking at the fraction of segments that contained fauna. Finally, we examined why certain faunal groups occurred in some segments and not others. Rather than testing between a priori categories, we employed a series of multivariate Classification and Regression Tree (CART) analyses to examine dominant patterns. Abundance of each faunal category, grouping behaviour and distribution in the water column were used to produce a multivariate assessment of the assemblage of mobile marine fauna and their spatial arrangement in the water column for each segment, using count data of each faunal category in each segment. To prepare data for multivariate analysis, we removed all segments where no fish or megafauna were present as these data is not appropriate for multivariate analysis, and we ran the tree analysis excluding faunal categories that occurred in <3 samples. The multivariate regression tree analyses were performed with Bray–Curtis dissimilarity as the distance measure, using the mvpart package in R (De’Ath, 2007; Ouellette and Legendre, 2012).
Firstly, to determine the relative importance of different spatial and temporal factors in structuring the assemblage of mobile marine fauna and their arrangement in the water column, we examined a subset of segments from Mackay Harbour that included the full range of habitat features and were replicated at each sampling event, across day and night, and across sampling trips. A similar examination was conducted for the Pioneer River. Secondly, based on the importance of temporal factors (see Results section), temporally consistent subsets of data were then used to examine the influence of spatial factors. For important factors identified in multivariate analysis, we produced violin plots of the abundance of small fish and large fish relative to those factors (including samples where no fauna occurred).
3 Results
Overall, 2,341 sonar segments were performed across Mackay Harbour and the Pioneer River estuary. Between 72 and 3588 large fish were encountered per ~2km transect, with an average of 661. Between 0 and 28 megafauna were encountered per ~2km transect, with an average of 5. 71% of segments contained fauna, 46% contained 10 or more individuals and 9% contained 100 or more individuals. Mobile marine fauna was frequently absent from shallow water segments (<2 m), while most deeper segments (>2 m) contained fauna. A variety of taxa were found in the different habitats sampled by RUV and direct observation (see Table S5). Taxa were all typical reef, coastal and estuarine species found in the region. No exotic species were observed.
3.1 Dominant drivers of assemblage and spatial arrangement
The difference between sonar samples that occurred in the day, and sonar samples that occurred during the night, was the most important factor accounting for the overall variability in the dataset. This overall difference can be seen clearly in the multivariate analysis of harbour samples (Figure 3). Daytime samples were dominated by small fish scattered on the bottom, and in tight schools. Night-time samples were dominated by large fish scattered throughout the water column. The number of large fish in samples was substantially higher at night than during the day, though the number of small fish in samples were generally similar between day and night (Figure 4). These strong differences were mirrored in the multivariate analysis of estuary sonar sampling (Figures 5, 6).
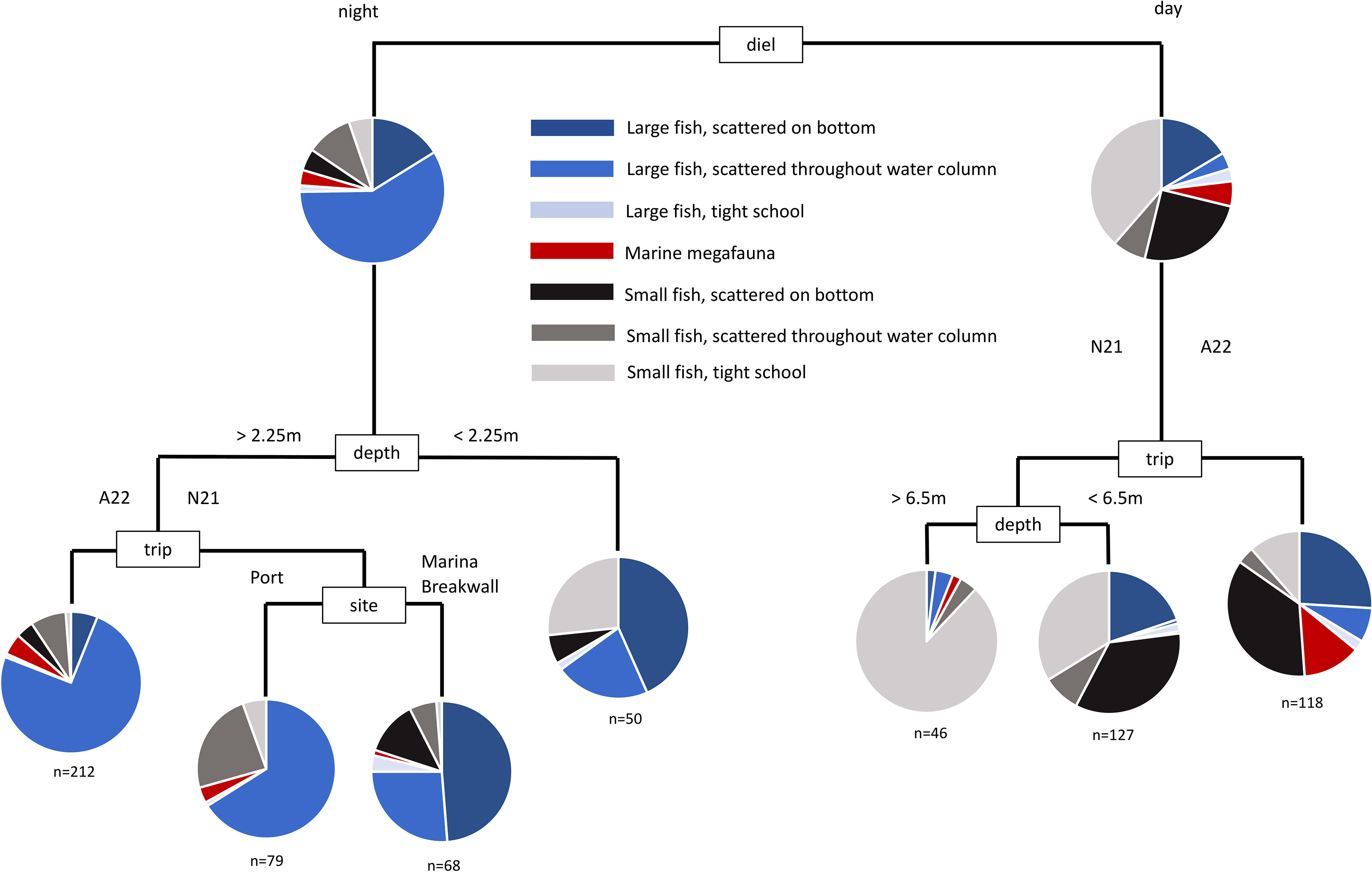
Figure 3 Multivariate classification and regression tree of the assemblage of mobile marine fauna from sonar sampling conducted in Mackay Harbour. Sites were replicated at every sampling event, across day and night, and across two seasons (see Table S1).
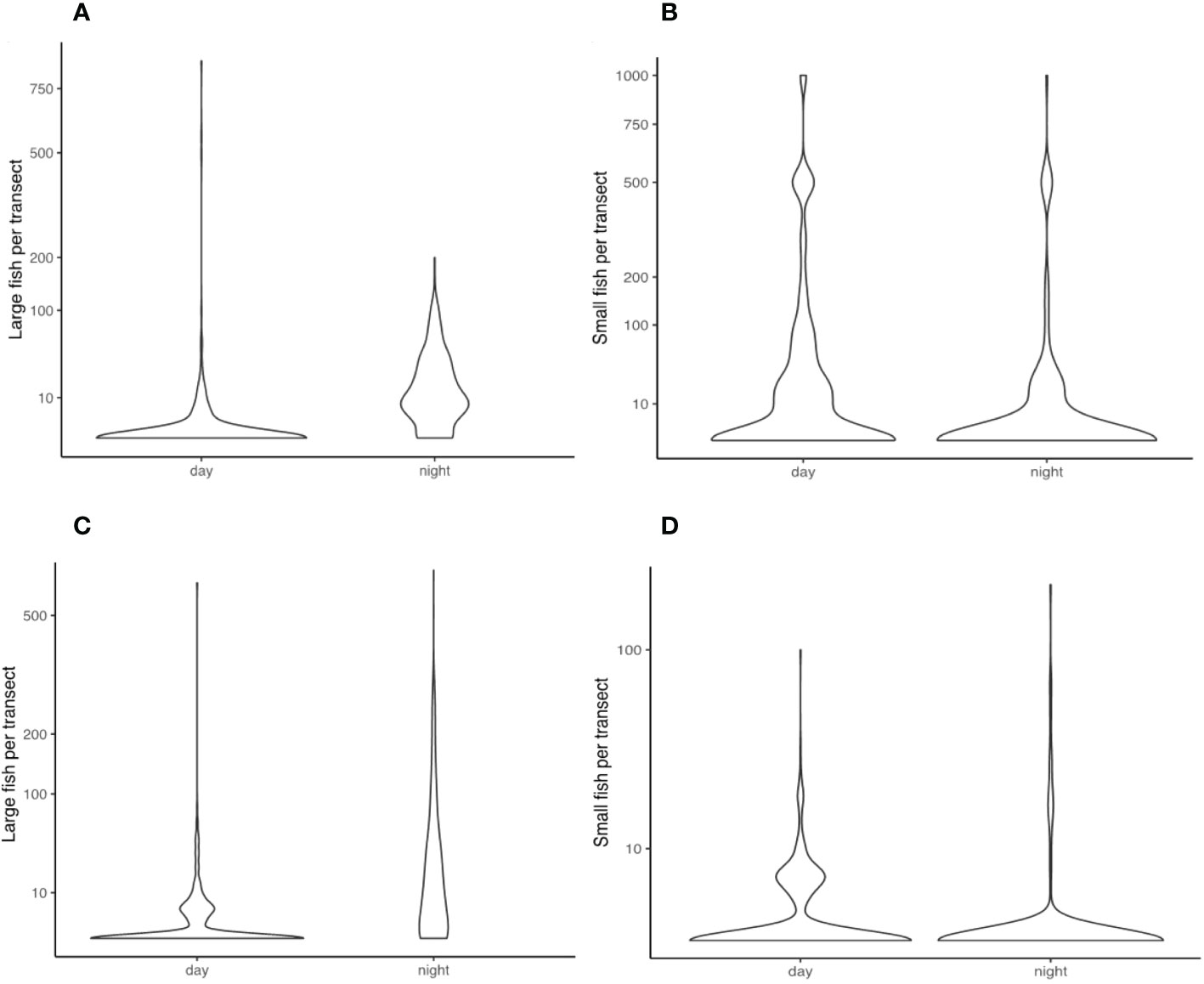
Figure 4 Violin distribution plots comparing the number of large fish (A) and small fish (B) recorded in sonar segments between day and night, fromMackay Harbour, and large fish (C) and small fish (D) recorded in sonar segments between day and night, from Pioneer River.
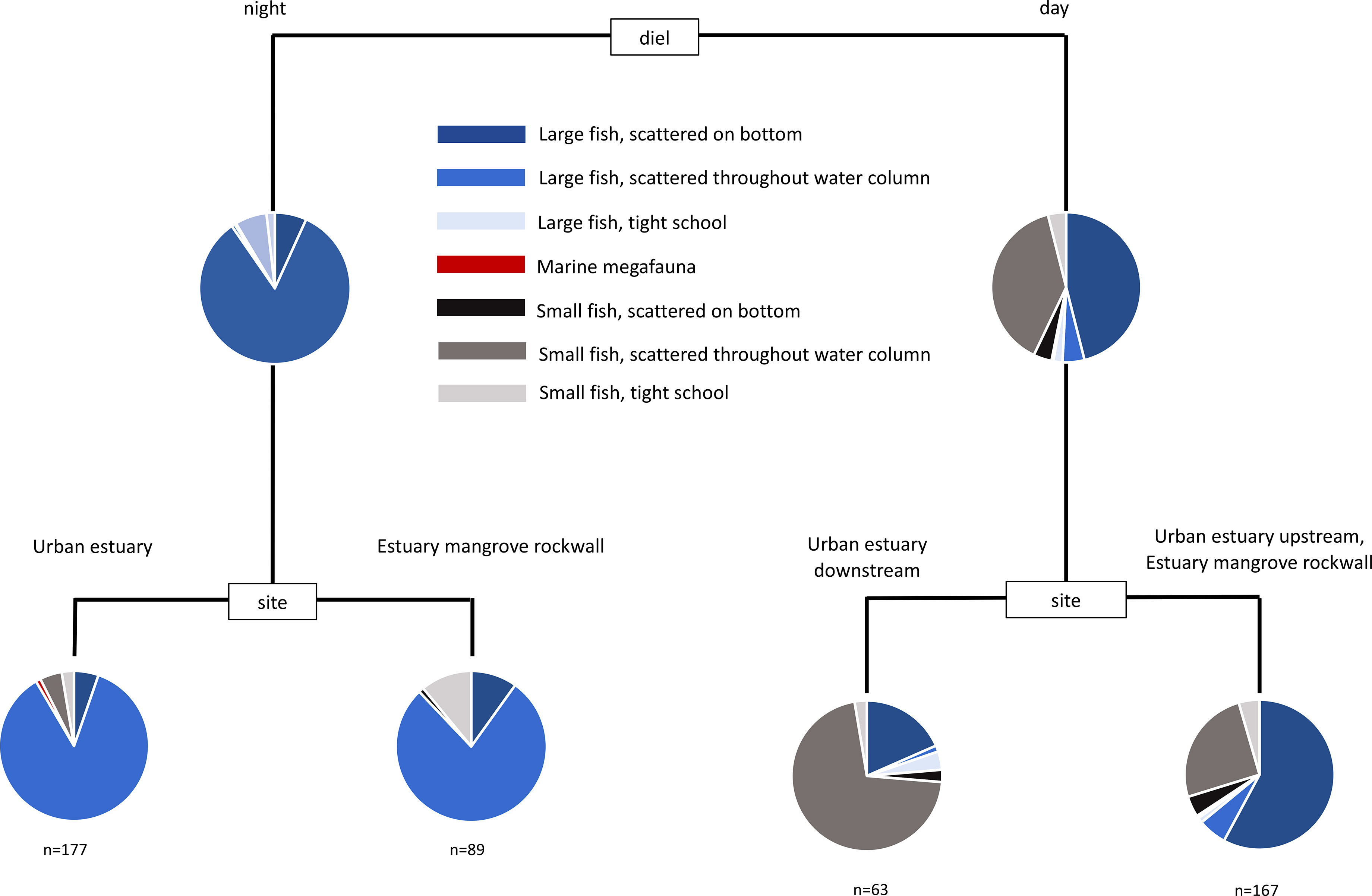
Figure 5 Multivariate classification and regression tree of the assemblage of mobile marine fauna from sonar sampling conducted in the Pioneer River estuary. Sites were replicated at every sampling event and across day and night (see Table S2).
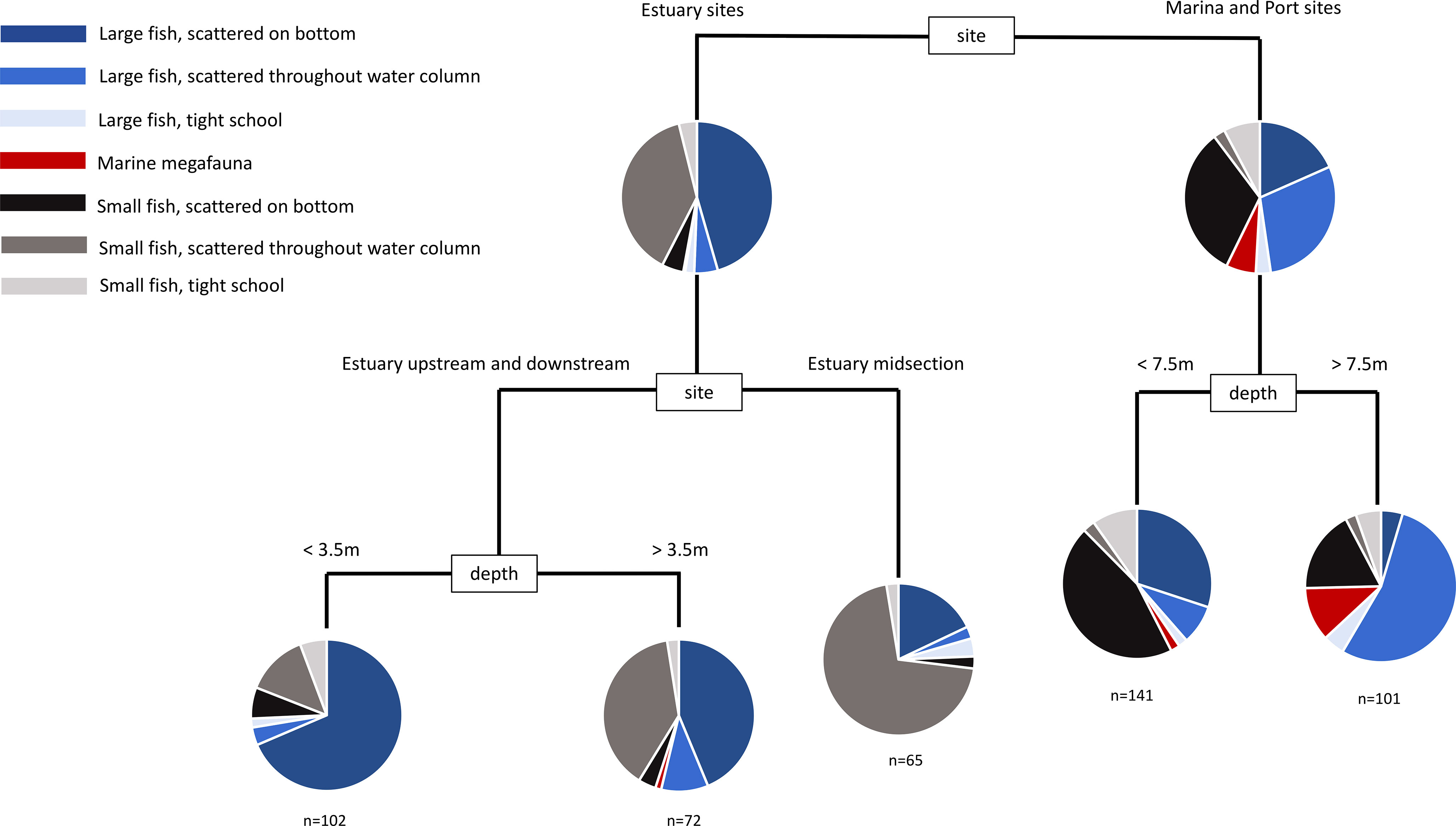
Figure 6 Multivariate classification and regression tree of the assemblage of mobile marine fauna from daytime sonar sampling. Sites were replicated at each sampling event (see Table S3).
3.2 Spatial patterns
When diel and trip variation is removed by sub-setting the data, a complex interaction of location, depth and structural features shape the assemblage of mobile marine fauna and their spatial arrangement in the water column. Within the urban-industrial seascapes examined, this indicates that all of these factors play an important role in determining animal-habitat associations.
During the day, the most important factor accounting for variability between sonar samples was whether the sample was in the harbour or estuary (Figure 7). Harbour samples tended to have more large fish dispersed through the water column and more small fish scattered on the bottom, whereas estuary sites tended to have more large fish scattered on the bottom and more small fish dispersed through the water column. Deeper samples (>7.5m) in the harbour tended to be dominated by large fish scattered through the water column, whereas the estuary lacked deeper water (max depth 6m).
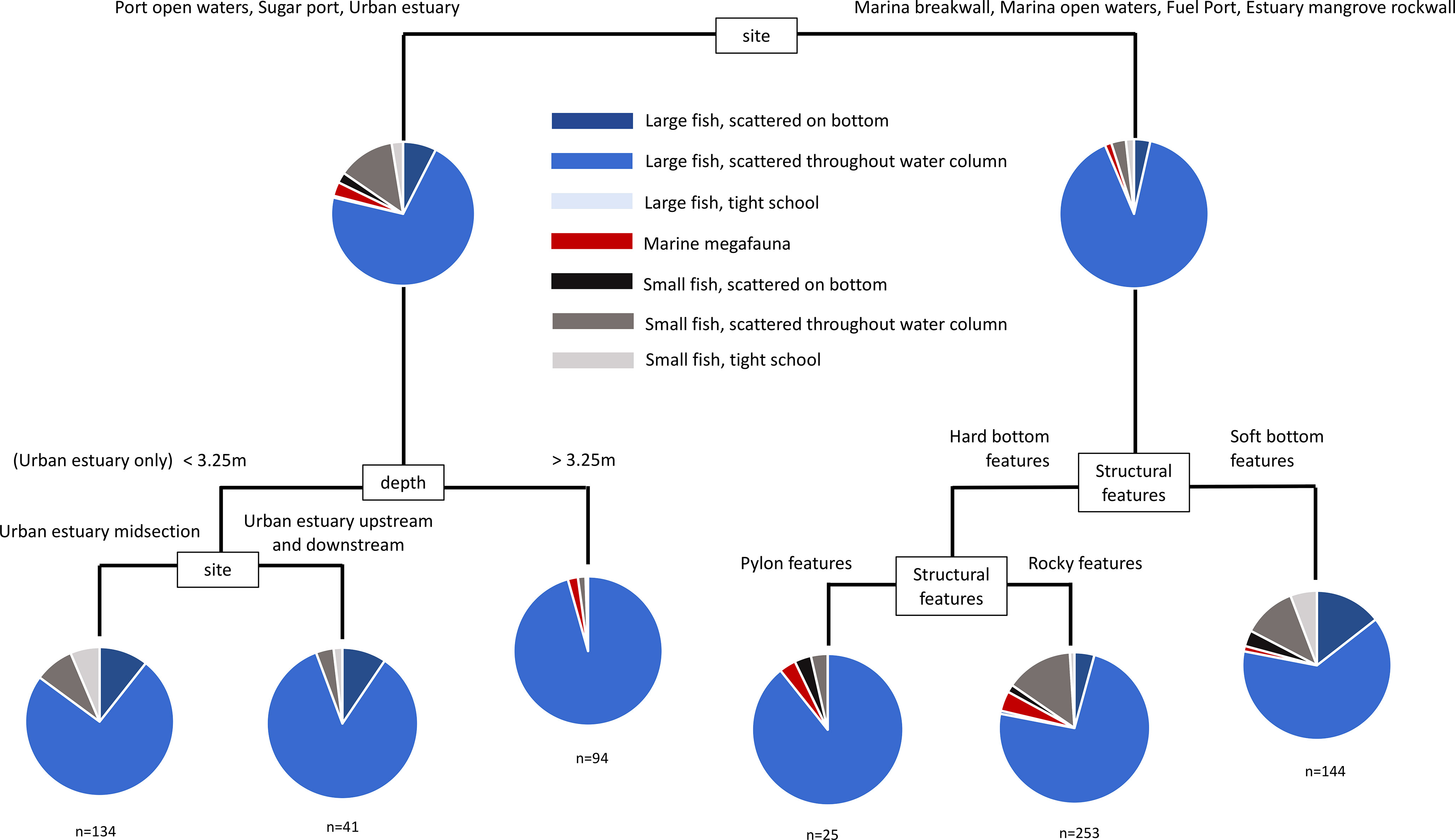
Figure 7 Multivariate classification and regression tree of the assemblage of mobile marine fauna from night time sonar sampling. Sites were replicated at each sampling event (see Table S4).
During the night, sonar samples were dominated by large fish scattered through the water column. The most important factor accounting for variability between individual samples was again location (Figure 8), which was distinguished further by structural features, depth and site. In sites where structural features distinguished between samples, areas with hard structure were clearly different from soft bottom areas, and areas with large piling features were clearly different from areas with rocky features. This is a meaningful result, given that sub-types of these features were able to cluster freely, but were partitioned according to these broad attributes.
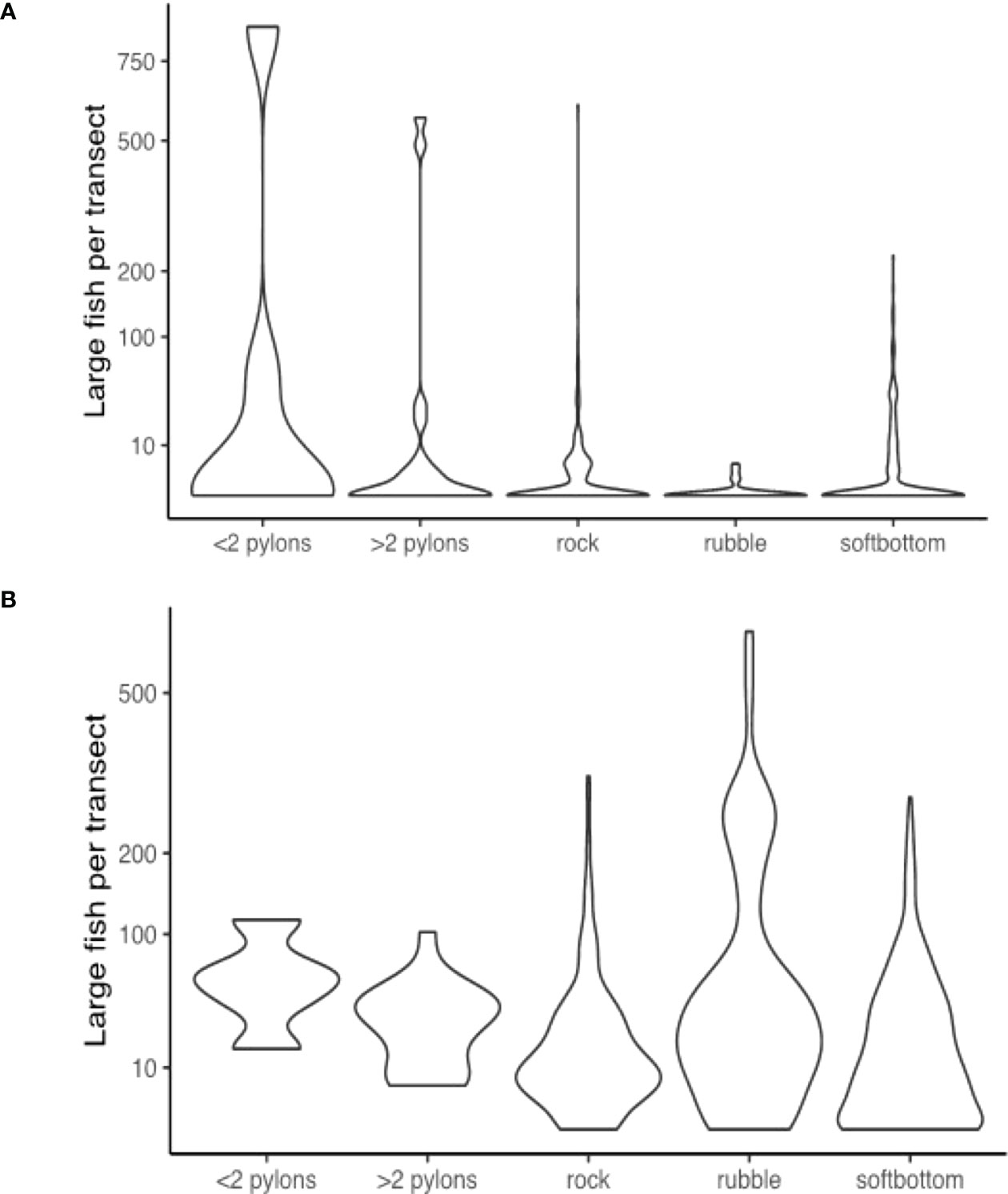
Figure 8 Violin distribution plots during the (A) day; and (B) night surveys comparing the number of large fish recorded in sonar segments from areas with each structural feature.
3.3 Deeper industrial seascapes
The interplay of two factors appears to be important in distinguishing deeper industrial locations from other areas. Firstly, large fish appear to be particularly associated with the presence of large pylon structures, and these structures only occurred in deeper industrial areas. Unique from other habitat types, there were always large fish present when pylons featured in a segment during night-time sampling, and generally larger numbers in these segments in daytime sampling (Figure 9). Secondly, deeper waters often contained larger numbers of large fish, regardless of structural features present (Figure 10). Large fish, megafauna and prey were often found in the same segments at night-time, and there was some preliminary indications of direct predator-prey interaction from deeper industrial areas (Figure 10).
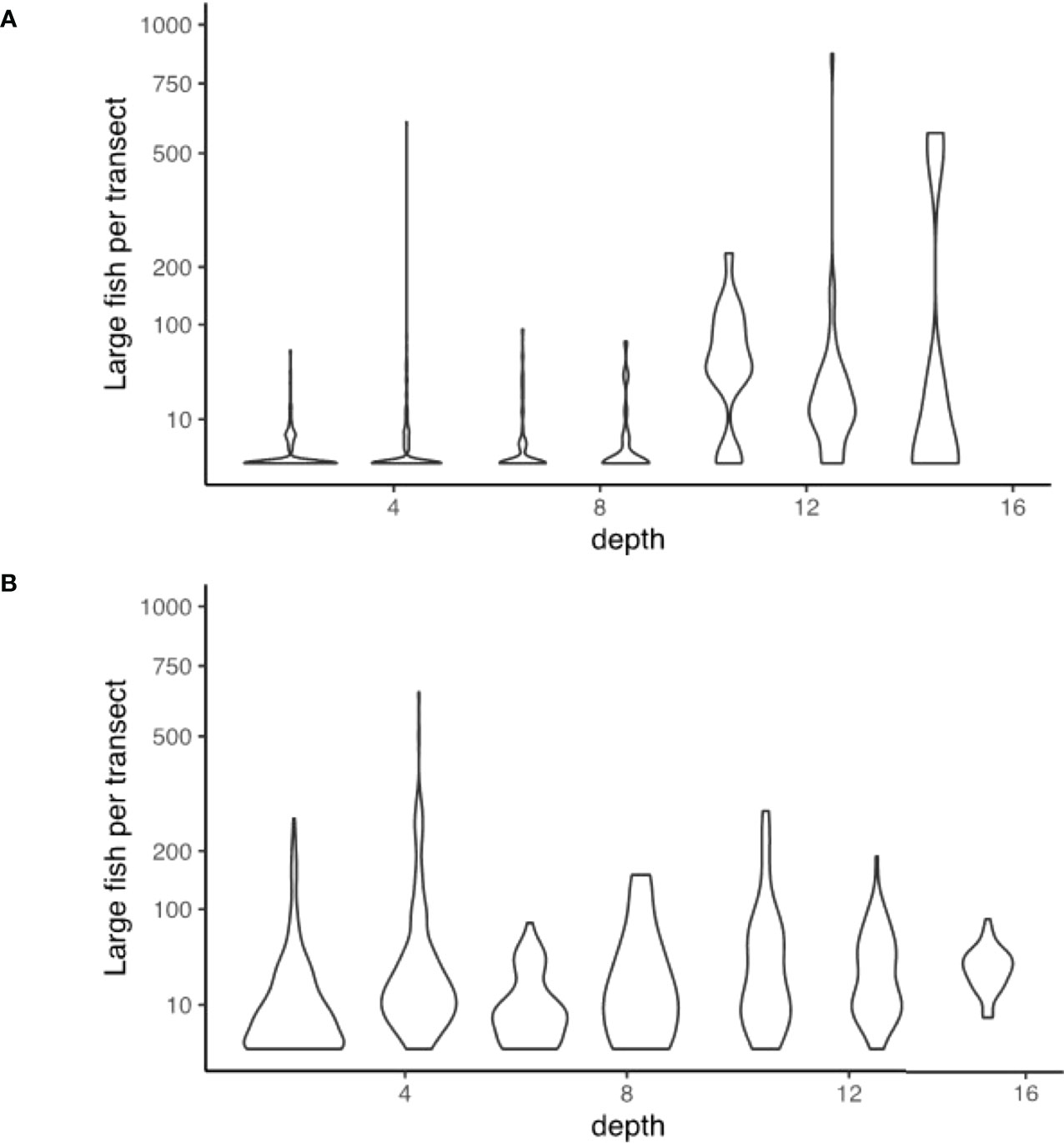
Figure 9 Violin distribution plots during the (A) day; and (B) night surveys comparing the numbers of large fish recorded in sonar segments at different depths.
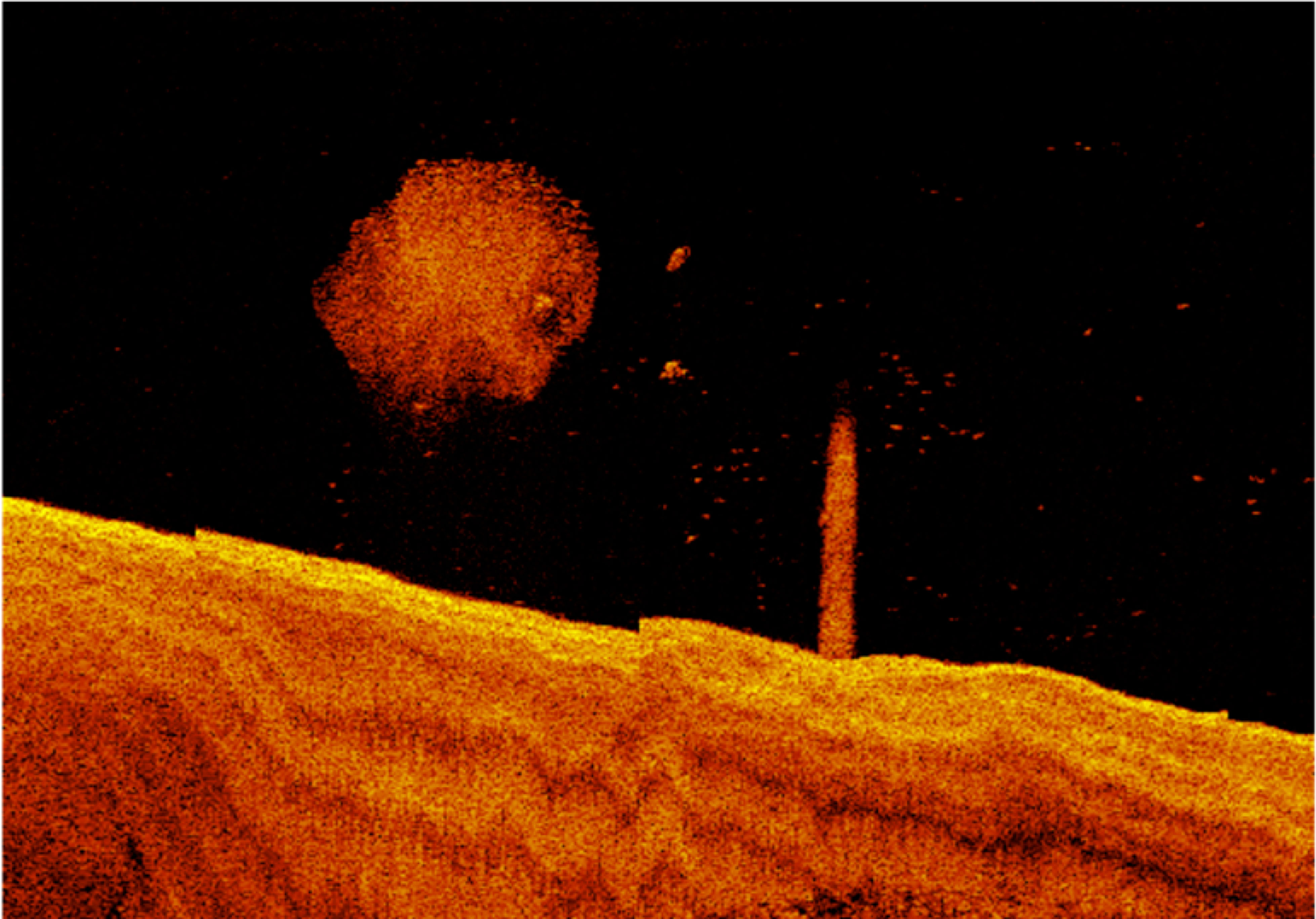
Figure 10 Example of potential predator-prey interaction from deeper industrial port areas recorded in sonar sampling at night time.
4 Discussion
The urban-industrial seascapes of Mackay contained an abundant and species rich community of mobile marine fauna. Most sonar segments contained fish, and roughly half contained 10 or more individuals. Some parts of these seascapes regularly contained more than 100 individuals per 20 m segment. There were a range of faunal types (small fish, large fish and megafauna) present in different spatial arrangements (scattered or schooling) in different parts of the water column. This is generally comparable to sonar surveys of natural areas of the GBR coast. In a sheltered bay in the Whitsunday Islands, Barnett et al. (2022) encountered between 1 and 89 large fish per ~2km transect, with an average of 13, and encountered between 1 and 11 marine megafauna per ~2km transect, with an average of 6. These data are not directly comparable, as the extent, type, and scale of structurally complex habitat differ greatly between study areas. However, this gives an indication that the urban-industrial seascapes in our study were far from depauperate when compared with a natural rocky bay of similar size. Based on underwater video and visual census, the mobile marine fauna in these areas were likely a range of locally typical species including coastal planktivores, demersal coastal, estuarine and reef fish and nearshore pelagic piscivores, and dolphins. These urban-industrial seascapes are clearly used extensively by fauna. Rather than being depauperate or low-diversity locations (e.g. dominated by a few invasive or synanthropic species), they are used by species from at least 24 different families of typical local fauna. Contrary to patterns described elsewhere (Brook et al., 2018), the most urbanised seascape contained the most abundant fauna. Thus, these areas should be viewed not just as marine infrastructure but also as marine habitat that provides opportunities for local fauna, alters local biodiversity and influences broader seascape function (Sheaves et al., 2021a).
Diel rhythms dominated the assemblage of mobile marine fauna in the urban-industrial seascapes. In most areas of the seascape, night samples were dominated by large fish, while day samples were dominated by small fish. This represents a massive shift in the assemblage of mobile marine fauna and their spatial arrangement in the water column, observed in both harbour and estuary environments. The details of this shift are unclear, but two possible explanations are presented here – vertical and horizontal migration. Being tightly bottom or structure associated can ‘hide’ fish from sonar imaging due to confusion of the acoustic signal with the bottom or structure, and a reduced gas in the swim bladder can lead to low acoustic reflectivity (Horne, 2000). Migrating vertically into the water column makes fish easily detectable on sonar imaging by providing a clearly defined signal and improved acoustic reflectivity with an inflated swim bladder (Horne, 2000). Secondly, fauna may be migrating from other parts of the seascape, either entering urban-industrial seascapes from outside (e.g. the open coast) or dispersing from high-density locations within the urban-industrial seascape. Whatever the reason, a major rearrangement of fauna is taking place. Fish communities are often temporally dynamic, as fish rearrange themselves within the seascape as the risks and rewards associated with different habitats change between day and night (Verweij et al., 2006; Krumme, 2009; Midwood et al., 2016). Any realistic understanding of fish-habitat relationships in these seascapes must therefore be made up of an understanding of habitat use during the day and during the night.
We now have sufficient information to broadly characterise the urban-industrial seascapes examined. During the day, most of the seascape contained bottom associated large fish. These are likely a range of species including lutjanids, sparids, haemulids, mugilids, carangids and siganids. In the estuary, small fish scattered throughout the water column were common, likely clupeiforms and ambassids. In the constructed harbour environment, small fish scattered throughout the water column were likely clupeids, but small fish were more often found scattered on the bottom. These were likely small structure associated reef fish, such as Pomacentrids, which were not present in similar habitat in the estuary. The marine conditions of the harbour can be used by the nearshore reef fish community, whereas the estuary was dominated by typically estuarine planktivores. Within the urban-industrial seascape, deeper industrial areas stood out as unique, often containing many large fish scattered throughout the water column, including barramundi (Lates calcarifer), adult haemulids, ephippids and large schools of carangids, as well as megafauna such as dolphins. It was the only habitat in the seascape where adult black jewfish (Protonibea diacanthus) and golden snapper (Lutjanus johnii) were found, both important and heavily targeted commercial fisheries species. The greatest abundances of large fish (>500 in a single 20m segment) were recorded in the deep pylon habitats of the industrial harbour seascape. During the night, fauna were organised primarily by habitat and depth. Large fish scattered through the water column were found throughout the seascape, however they were most common along rock walls, near pylons and in deeper areas. Again, deeper industrial areas appear unique. The deep pylon habitats of the industrial harbour seascape always contained large fish, often in large numbers, and there was preliminary evidence of predator-prey interaction between megafauna and small fish (Figure 10). In both the day and the night, deep industrial areas appear to function differently as habitat than other parts of the seascape.
The urban-industrial seascapes we studied contained typical coastal fauna in novel situations. The harbour represents an entirely constructed environment, replacing a wave dominated open coast beach with a sheltered and structurally complex environment. As with other marine urban developments (Jones and Nithyanandan, 2013), the harbour probably contains persistently higher abundance of mobile marine fauna than the low complexity habitat it has replaced. Whether it is actually more productive is unclear, though possible, given the mechanisms of productivity found for other artificial structures (Paxton et al., 2020). However, given the inevitability of human-induced change it seems sensible to understand the faunal assemblages of these constructed environments as adaptive outcomes of change and understand their values (Sheaves et al., 2021b), rather than to categorise them as ‘good’ or ‘bad’. Deeper industrial areas of the seascape do appear to function as sites of major aggregation and possibly trophic transfer. How these unique locations, with their novel habitat features, interact with predator-prey relationships is important to understand. These areas may represent a challenge to naturally selected adaptive behaviours of both predator and prey (Hobbs et al., 2009). For each species present, the direction in which the balance of favour is tipped will determine whether these areas function as genuine hotspots of productivity or as ecological traps. These areas are clearly attractive to many fish species, so if they confer lower fitness than other, less attractive habitats, this has the possibility to cause population declines (Battin, 2004; Hale and Swearer, 2016). Given how prevalent these seascapes are (Feary et al., 2011; Waltham and Sheaves, 2015; Burt and Bartholomew, 2019), going beyond patterns of distribution to determine ecological consequences, especially at larger scales (McLean et al., 2022) will be vital.
4.1 Consequences for the management of urban-industrial seascapes
In the context of marine ecosystem conservation and protection, managers challenged with approving more coastal development require access to data and information that is fine-scaled enough and focused on basic questions around marine ecosystems in urban-industrial seascapes. The data here adds to the growing evidence that urban-industrial infrastructure may provide functional habitat for coastal fish and fisheries. The addition of pylons and rock seawalls to the seascape appear to provide habitat for large fish, particularly at night when pylons consistently harboured between 10 and 100 individuals, in contrast with soft bottom areas that usually harboured no or low numbers of individuals. This raises interesting movement ecology questions, such as the possibility that large fish move from elsewhere into these urban-industrial waters at night to forage. In an urbanised seascape in North America, the nocturnal distribution of juvenile pacific salmon (Oncorhynchus spp.) was twice that of daytime along a waterfront of Seattle harbour (Accola et al., 2022). The diel foraging movements of fish has been well documented on coral reefs, where some species migrate away from reefs at night to feeding areas (Francis and Côté, 2018). One possible cause of this higher nocturnal abundance is the attraction of fish to these structures because of the proliferation of artificial light at night, which can alter fish community behaviour (Marangoni et al., 2022) including providing optimal conditions for predators (Becker et al., 2013). These emerging patterns highlight the importance of undertaking diel fish distribution and abundance surveys, so that we can begin to tackle some of the most basic questions about how these areas are used by mobile marine fauna, which has important implications for the optimal design and construction of coastal urban-industrial structures.
Our study indicates that, unique within the urban-industrial seascape, deeper industrial infrastructure such as ports could, in effect, function as no-take marine sanctuaries for commercially important fisheries species, with the potential to positively contribute to fished populations. We found that large numbers of commercially and recreationally fished species may be regularly inhabiting large industrial infrastructure. These areas are effectively marine reserves, as only authorised vessels may legally enter the area, and activities such as fishing are prohibited, because of interference with port operations. They are also effectively some of the best enforced marine reserves, with 24hr surveillance and active security. This is in stark contrast to enforcement and compliance within official marine protected areas around the world, including in the neighbouring Great Barrier Reef Marine Park (Bergseth et al., 2018), where surveillance is challenging owing to the scale of the operation. A similar situation has been found for oil platforms in the Arabian Gulf (Burt and Bartholomew, 2019), which function as no-take reserves for commercially important species, due to security concerns around petroleum operations (Vaughan et al., 2019). In our study, abundances of fish associated with port infrastructure as habitat are confounded with the potential effects of port infrastructure in eliminating fishing pressure. More research is needed to understand the effects of large industrial infrastructure as habitat vs as marine reserves. If target species using ports are sufficiently site-attached, and these areas confer a sufficient fitness advantage, it is possible that these areas may positively contribute to broader fished populations through the supply of larvae or adults (Gell and Roberts, 2003; Russ et al., 2004).
5 Conclusions
As marine urbanisation changes our coastlines, there is a critical need to understand the ecological outcomes of this change. With a sound understanding of these novel ecosystems, as a society we will have the opportunity to ensure that their value is optimised and avoid perverse outcomes. Advances in fish sampling approaches have allowed us here to advance basic ecological knowledge relating to highly modified seascapes, revealing the importance and potential benefits of these hybrid coastal ecosystems. The data here provides the first indication that urban-industrial seascapes can contain abundances of mobile marine fauna similar to natural areas, and that these seascapes are characterised by dynamic diel shifts in faunal arrangement, where large fish become prevalent in the water column at night. This study provides a proof of concept for the use of affordable consumer grade, ‘trailer-boat’ technology to provide an effective assessment of mobile marine fauna, opening the doorway for regular, large-scale monitoring of coastal environments, particularly to detect broad changes in faunal abundance. The study brings new information to the nascent field of urban marine ecology, but important research questions remain relating to the functional implications of these marine urban habitats. As part of broader coastal habitat mosaics, these novel habitats likely represent an enormous shift in the nature of local ecosystems, and may have important flow-on effects at various scales.
Data availability statement
The datasets presented in this study can be found in online repositories. The names of the repository/repositories and accession number(s) can be found below: James Cook University Tropical Data Hub (link will be provided once data has been lodged after article acceptance). Further queries should be directed to the corresponding author.
Ethics statement
The animal study was reviewed and approved by James Cook University Animal Ethics Committee.
Author contributions
MB, MS, and NW conceived the ideas and design; MB and NW collected the data; MB analysed the data; MB led the writing of the manuscript. All authors contributed to drafts and gave approval for publication.
Funding
North Queensland Bulk Ports provided funding for this project, with support by James Cook University’s Marine Data Technology Hub.
Acknowledgments
We acknowledge the Traditional Owners of the lands on which this research was completed and pay respect to their leader’s past, current and emerging. We thank Ben Anderson, Manager Mackay Marina, for access to the port facility.
Conflict of interest
The authors declare that the research was conducted in the absence of any commercial or financial relationships that could be construed as a potential conflict of interest.
Publisher’s note
All claims expressed in this article are solely those of the authors and do not necessarily represent those of their affiliated organizations, or those of the publisher, the editors and the reviewers. Any product that may be evaluated in this article, or claim that may be made by its manufacturer, is not guaranteed or endorsed by the publisher.
Supplementary material
The Supplementary Material for this article can be found online at: https://www.frontiersin.org/articles/10.3389/fmars.2022.1034039/full#supplementary-material
References
Accola K. L., Horne J. K., Cordell J. R., Toft J. D. (2022). Nocturnal distributions of juvenile pacific salmon along an eco-engineered marine shoreline. Mar. Ecol. Prog. Ser. 687, 113–123. doi: 10.3354/meps14006
Adams L., Morris R., Hull R., Dempster T., Strain E. (2021). Making marinas bivalve friendly for enhanced biodiversity outcomes. Mar. pollut. Bull. 169, 112464. doi: 10.1016/j.marpolbul.2021.112464
Airoldi L., Beck M. W., Firth L. B., Bugnot A. B., Steinberg P. D., Dafforn K. A. (2020). Emerging solutions to return nature to the urban ocean. Annu. Rev. Mar. Sci. 13, 445–477. doi: 10.1146/annurev-marine-032020-020015
Alongi D., McKinnon A. (2005). The cycling and fate of terrestrially-derived sediments and nutrients in the coastal zone of the great barrier reef shelf. Mar. pollut. Bull. 51 (1), 239–252. doi: 10.1016/j.marpolbul.2004.10.033
Barnett A., Fitzpatrick R., Bradley M., Miller I., Sheaves M., Chin A., et al (2022) Scientific response to a cluster of shark bites People Nature, 963–982. doi: 10.1002/pan3.10337
Battin J. (2004). When good animals love bad habitats: ecological traps and the conservation of animal populations. Conserv. Biol. 18 (6), 1482–1491. doi: 10.1111/j.1523-1739.2004.00417.x
Becker A., Whitfield A. K., Cowley P. D., Järnegren J., Næsje T. F. (2013). Potential effects of artificial light associated with anthropogenic infrastructure on the abundance and foraging behaviour of estuary-associated fishes. J. Appl. Ecol. 50 (1), 43–50. doi: 10.1111/1365-2664.12024
Bergseth B. J., Gurney G. G., Barnes M. L., Arias A., Cinner J. E. (2018). Addressing poaching in marine protected areas through voluntary surveillance and enforcement. Nat. Sustainability. 1 (8), 421–426. doi: 10.1038/s41893-018-0117-x
Bishop M. J., Mayer-Pinto M., Airoldi L., Firth L. B., Morris R. L., Loke L. H., et al. (2017). Effects of ocean sprawl on ecological connectivity: impacts and solutions. J. Exp. Mar. Biol. Ecol. 492, 7–30. doi: 10.1016/j.jembe.2017.01.021
Bradley M., Baker R., Nagelkerken I., Sheaves M. (2019). Context is more important than habitat type in determining use by juvenile fish. Landscape Ecol. 34 (2), 427–442. doi: 10.1007/s10980-019-00781-3
Bradley M., Nagelkerken I., Baker R., Sheaves M. (2020). Context dependence: A conceptual approach for understanding the habitat relationships of coastal marine fauna. BioScience 70 (11), 986–1004. doi: 10.1093/biosci/biaa100
Brook T. W., Gilby B. L., Olds A. D., Connolly R. M., Henderson C. J., Schlacher T. A. (2018). The effects of shoreline armouring on estuarine fish are contingent upon the broader urbanisation context. Mar. Ecol. Prog. Ser. 605, 195–206. doi: 10.3354/meps12756
Bulleri F., Chapman M. G. (2010). The introduction of coastal infrastructure as a driver of change in marine environments. J. Appl. Ecol. 47 (1), 26–35. doi: 10.1111/j.1365-2664.2009.01751.x
Burt J. A., Bartholomew A. (2019). Towards more sustainable coastal development in the Arabian gulf: Opportunities for ecological engineering in an urbanized seascape. Mar. pollut. Bull. 142, 93–102. doi: 10.1016/j.marpolbul.2019.03.024
Claassens L. (2016). An artificial water body provides habitat for an endangered estuarine seahorse species. Estuarine. Coast. shelf. Sci. 180, 1–10. doi: 10.1016/j.ecss.2016.06.011
Claassens L., de Villiers N., Waltham N. (2022). How developed is the south African coast? baseline extent of south africa’s coastal and estuarine infrastructure. Ocean. Coast. Manage. 222, 106112. doi: 10.1016/j.ocecoaman.2022.106112
Claisse J. T., Pondella D. J., Love M., Zahn L. A., Williams C. M., Williams J. P., et al. (2014). Oil platforms off California are among the most productive marine fish habitats globally. Proc. Natl. Acad. Sci. 111 (43), 15462–15467. doi: 10.1073/pnas.1411477111
Clynick B. (2008). Characteristics of an urban fish assemblage: distribution of fish associated with coastal marinas. Mar. Environ. Res. 65 (1), 18–33. doi: 10.1016/j.marenvres.2007.07.005
Daniel S., Le Léannec F., Roux C., Soliman B., Maillard E. P. (1998). Side-scan sonar image matching. IEEE J. Oceanic. Eng. 23 (3), 245–259. doi: 10.1109/48.701197
De’Ath G. (2007) Mvpart: Multivariate partitioning r package. version 1.2-6. Available at: http://cran.r-project.org/package=mvpart.
Durá E., Bell J., Lane D. (2004). Reconstruction of textured seafloors from side-scan sonar images. IEE. Proceedings-Radar. Sonar. Navigation. 151 (2), 114–126. doi: 10.1049/ip-rsn:20040262
Feary D. A., Burt J. A., Bartholomew A. (2011). Artificial marine habitats in the Arabian gulf: Review of current use, benefits and management implications. Ocean. Coast. Manage. 54 (10), 742–749. doi: 10.1016/j.ocecoaman.2011.07.008
Fleming P., Daugherty D., Smith N., Betsill R. (2018). Efficacy of low-cost, side-scan sonar for surveying alligator gars. Trans. Am. Fisheries. Soc. 147 (4), 696–703. doi: 10.1002/tafs.10047
Francis F. T., Côté I. M. (2018). Fish movement drives spatial and temporal patterns of nutrient provisioning on coral reef patches. Ecosphere 9 (5), e02225. doi: 10.1002/ecs2.2225
Francis M. P., Morrison M. A., Leathwick J., Walsh C., Middleton C. (2005). Predictive models of small fish presence and abundance in northern new Zealand harbours. Estuarine. Coast. Shelf. Sci. 64, 419–435. doi: 10.1016/j.ecss.2005.03.007
Gell F. R., Roberts C. M. (2003). Benefits beyond boundaries: the fishery effects of marine reserves. Trends Ecol. Evol. 18 (9), 448–455. doi: 10.1016/S0169-5347(03)00189-7
Gerlotto F., Georgakarakos S., Eriksen P. K. (2000). The application of multibeam sonar technology for quantitative estimates of fish density in shallow water acoustic surveys. Aquat. Living. Resour. 13 (5), 385–393. doi: 10.1016/S0990-7440(00)01055-X
Gill A. B. (2005). Offshore renewable energy: ecological implications of generating electricity in the coastal zone. J. Appl. Ecol. 42, 605–615. doi: 10.1111/j.1365-2664.2005.01060.x
Gonzalez-Socoloske D., Olivera-Gomez L. D., Ford R. E. (2009). Detection of free-ranging West Indian manatees trichechus manatus using side-scan sonar. Endangered. species. Res. 8 (3), 249–257. doi: 10.3354/esr00232
Hale R., Swearer S. E. (2016). Ecological traps: current evidence and future directions. Proc. R. Soc. B.: Biol. Sci. 283 (1824), 20152647. doi: 10.1098/rspb.2015.2647
Hobbs R. J., Higgs E., Harris J. A. (2009). Novel ecosystems: implications for conservation and restoration. Trends Ecol. Evol. 24 (11), 599–605. doi: 10.1016/j.tree.2009.05.012
Horne J. K. (2000). Acoustic approaches to remote species identification: a review. Fisheries. oceanography. 9 (4), 356–371. doi: 10.1046/j.1365-2419.2000.00143.x
Jones D. A., Nithyanandan M. (2013). Recruitment of marine biota onto hard and soft artificially created subtidal habitats in sabah Al-ahmad Sea city, Kuwait. Mar. pollut. Bull. 72 (2), 351–356. doi: 10.1016/j.marpolbul.2012.11.001
Krumme U. (2009). Diel and tidal movements by fish and decapods linking tropical coastal ecosystems. In Ecological connectivity among tropical coastal ecosystems (pp. 271–324). (Dordrecht: Springer).
Lai S., Loke L. H., Hilton M. J., Bouma T. J., Todd P. A. (2015). The effects of urbanisation on coastal habitats and the potential for ecological engineering: A Singapore case study. Ocean. Coast. Manage. 103, 78–85. doi: 10.1016/j.ocecoaman.2014.11.006
Lewis S. E., Bartley R., Wilkinson S. N., Bainbridge Z. T., Henderson A. E., James C. S., et al. (2021). Land use change in the river basins of the great barrier reef 1860 to 2019: A foundation for understanding environmental history across the catchment to reef continuum. Mar. pollut. Bull. 166, 112193. doi: 10.1016/j.marpolbul.2021.112193
Lindegarth M. (2001). Assemblages of animals around urban structures: testing hypotheses of patterns in sediments under boat-mooring pontoons. Mar. Environ. Res. 51, 289–300. doi: 10.1016/S0141-1136(00)00030-1
Maclagan S. J., Coates T., Ritchie E. G. (2018). Don’t judge habitat on its novelty: Assessing the value of novel habitats for an endangered mammal in a peri-urban landscape. Biol. Conserv. 223, 11–18. doi: 10.1016/j.biocon.2018.04.022
Marangoni L. F. B., Davies T., Smyth T., Rodríguez A., Hamann M., Duarte C., et al. (2022). Impacts of artificial light at night in marine ecosystems–a review. Global Change Biol. 28, 5346–5367. doi: 10.1111/gcb.16264
McLean D. L., Ferreira L. C., Benthuysen J. A., Miller K. J., Schläppy M., Ajemian M. J., et al. (2022). Influence of offshore oil and gas structures on seascape ecological connectivity. Global Change Biol. 28 (11), 3515–3536. doi: 10.1111/gcb.16134
Midwood J. D., Chapman J. M., Cvetkovic M., King G. D., Ward T. D., Suski C. D., et al. (2016). Diel variability in fish assemblages in coastal wetlands and tributaries of the st. Lawrence river: A cautionary tale for fisheries monitoring. Aquat. Sci. 78 (2), 267–277. doi: 10.1007/s00027-015-0422-7
Ouellette M., Legendre P. (2012) MVPARTwrap: additional functionalities for package mvpart. r package version 0.1-9. Available at: https://cran.r-project.org/package=MVPARTwrap.
Papastamatiou Y. P., Britton C., Burgess G. H. (2020). Using side-scan sonar to survey critically endangered smalltooth sawfish. Fisheries. Res. 228, 105577. doi: 10.1016/j.fishres.2020.105577
Pastor J., Koeck B., Astruch P., Lenfant P. (2013). Coastal man-made habitats: Potential nurseries for an exploited fish species, diplodus sargus (Linnaeus 1758). Fisheries. Res. 148, 74–80. doi: 10.1016/j.fishres.2013.08.014
Paxton A. B., Shertzer K. W., Bacheler N. M., Kellison G. T., Riley K. L., Taylor J. C. (2020). Meta-analysis reveals artificial reefs can be effective tools for fish community enhancement but are not one-size-fits-all. Front. Mar. Sci. 7, 282. doi: 10.3389/fmars.2020.00282
Plaza P. I., Lambertucci S. A. (2017). How are garbage dumps impacting vertebrate demography, health, and conservation? Global Ecol. Conserv. 12, 9–20. doi: 10.1016/j.gecco.2017.08.002
Russ G. R., Alcala A. C., Maypa A. P., Calumpong H. P., White A. T. (2004). Marine reserve benefits local fisheries. Ecol. Appl. 14 (2), 597–606. doi: 10.1890/03-5076
Selfati M., El Ouamari N., Lenfant P., Fontcuberta A., Lecaillon G., Mesfioui A., et al. (2018). Promoting restoration of fish communities using artificial habitats in coastal marinas. Biol. Conserv. 219, 89–95. doi: 10.1016/j.biocon.2018.01.013
Sheaves M., Mattone C., Connolly R. M., Hernandez S., Nagelkerken I., Murray N., et al. (2021a). Ecological constraint mapping: Understanding outcome-limiting bottlenecks for improved environmental decision-making in marine and coastal environments. Front. Mar. Sci. 8, 1133. doi: 10.3389/fmars.2021.717448
Sheaves M., Molony B. (2000). Short-circuit in the mangrove food chain. Mar. Ecol. Prog. Ser. 199, 97–109. doi: 10.3354/meps199097
Sheaves M., Waltham N., Benham C., Bradley M., Mattone C., Diedrich A., et al. (2021b). Restoration of marine ecosystems: Understanding possible futures for optimal outcomes. Sci. Total. Environ. 796, 148845. doi: 10.1016/j.scitotenv.2021.148845
Sheppard C., Al-Husiani M., Al-Jamali F., Al-Yamani F., Baldwin R., Bishop J., et al. (2010). The gulf: a young sea in decline. Mar. pollut. Bull. 60 (1), 13–38. doi: 10.1016/j.marpolbul.2009.10.017
Smith J. A., Lowry M. B., Champion C., Suthers I. M. (2016). A designed artificial reef is among the most productive marine fish habitats: new metrics to address ‘production versus attraction’. Mar. Biol. 163 (9), 1–8. doi: 10.1007/s00227-016-2967-y
Todd P. A., Heery E. C., Loke L. H., Thurstan R. H., Kotze D. J., Swan C. (2019). Towards an urban marine ecology: Characterizing the drivers, patterns and processes of marine ecosystems in coastal cities. Oikos 128 (9), 1215–1242. doi: 10.1111/oik.05946
Torquato F., Jensen H., Range P., Bach S., Ben-Hamadou R., Sigsgaard E., et al. (2017). Vertical zonation and functional diversity of fish assemblages revealed by ROV videos at oil platforms in the gulf. J. Fish. Biol. 91 (3), 947–967. doi: 10.1111/jfb.13394
van Lavieren H., Burt J., Feary D., Cavalcante G., Marquis E., Benedetti L., et al. (2011). Managing the Growing Impacts of Development on Fragile Coastal and Marine Systems: Lessons from the Gulf. (Hamilton, ON, Canada: A Policy Report. United Nations University - Institute for Water, Environment, and Health).
Vaughan G. O., Al-Mansoori N., Burt J. A. (2019). The Arabian Gulf. In: Sheppard C. (Ed.), World Seas: An Environmental Evaluation, second edition. (Amsterdam, NL: Elsevier Science), pp. 1–23.
Verweij M. C., Nagelkerken I., Wartenbergh S. L., Pen I. R., van der Velde G. (2006). Caribbean Mangroves and seagrass beds as daytime feeding habitats for juvenile French grunts, haemulon flavolineatum. Mar. Biol. 149 (6), 1291–1299. doi: 10.1007/s00227-006-0305-5
Vine J. R., Kanno Y., Holbrook S. C., Post W. C., Peoples B. K. (2019). Using side-scan sonar and n-mixture modeling to estimate Atlantic sturgeon spawning migration abundance. North Am. J. Fisheries. Manage. 39 (5), 939–950. doi: 10.1002/nafm.10326
Waltham N. J., Bradley M., Wilson S., Kane K., Langham O., Sheaves M. (2022). Remotely operated vehichle reveals fish orientate to the substrate underneath marina floating pontoons. Estuarine Coastal Shelf Sci. 108184. doi: 10.1016/j.ecss.2022.108184
Waltham N. J., Sheaves M. (2015). Expanding coastal urban and industrial seascape in the great barrier reef world heritage area: Critical need for coordinated planning and policy. Mar. Policy 57, 78–84. doi: 10.1016/j.marpol.2015.03.030
Keywords: sonar, estuaries, ports, ecological engineering, blue economy, ocean sprawl, urban ecology
Citation: Bradley M, Sheaves M and Waltham NJ (2023) Urban-industrial seascapes can be abundant and dynamic fish habitat. Front. Mar. Sci. 9:1034039. doi: 10.3389/fmars.2022.1034039
Received: 01 September 2022; Accepted: 09 December 2022;
Published: 11 January 2023.
Edited by:
Dianne McLean, Australian Institute of Marine Science (AIMS), AustraliaReviewed by:
Iain M. Suthers, University of New South Wales, AustraliaJoel Williams, University of Tasmania, Australia
Copyright © 2023 Bradley, Sheaves and Waltham. This is an open-access article distributed under the terms of the Creative Commons Attribution License (CC BY). The use, distribution or reproduction in other forums is permitted, provided the original author(s) and the copyright owner(s) are credited and that the original publication in this journal is cited, in accordance with accepted academic practice. No use, distribution or reproduction is permitted which does not comply with these terms.
*Correspondence: Michael Bradley, michael.bradley@jcu.edu.au