- 1Shandong Provincial Key Laboratory of Applied Microbiology, Ecology Institute, Qilu University of Technology (Shandong Academy of Sciences), Ji’nan, China
- 2Guangdong Provincial Key Laboratory of Water Quality Improvement and Ecological Restoration for Watersheds, Institute of Environmental and Ecological Engineering, Guangdong University of Technology, Guangzhou, China
- 3Henan Key Laboratory of Ecological Environment Protection and Restoration of Yellow River Basin, Yellow River Institute of Hydraulic Research, Zhengzhou, China
The soil carbon sequestration largely depends on soil organic carbon (SOC) stability. However, the mechanism of SOC stabilization in coastal wetlands under different hydrologic conditions remains to be clarified. In this study, the effect of mineral protection on SOC stabilization was investigated in freshwater-flooded Phragmites australis wetlands (FPW), tidal P. australis wetlands (TPW), non-flooded P. australis wetlands (NPW) and tidal Suaeda salsa wetlands (TSW). Two stabilized SOC fractions (Na2S2O8 resistant SOC and H2O2 resistant SOC) and Al/Fe/Si oxides extracted by acid oxalate, dithionite–citrate and pyrophosphate, respectively, were determined in soil samples collected to a depth of 25 cm. The contents of soil Na2S2O8 resistant SOC and H2O2 resistant SOC were not significantly different among the four wetlands (p > 0.05), with the highest mean values occurring in FPW (1.44 ± 0.43 g kg-1) and TPW (1.79 ± 0.40 g kg-1), respectively. The contents of Al/Fe/Si oxides (except for Sip) and the values of mineral phase indicators were significantly lower in NPW than in TPW or FPW (p < 0.05). Linear regressions among Al/Fe/Si oxides, mineral phase indicators, and two stabilized SOC fractions showed that Na2S2O8 resistant SOC and H2O2 resistant SOC were positively and significantly affected by mineral contents (p < 0.05). Additionally, edaphic factors such as soil organic matter, total nitrogen, soil water content, clay and silt contents were identified as important factors influencing the two stabilized SOC fractions.
Introduction
As the largest terrestrial carbon pool, SOC stock in soils is three times larger than the size of atmospheric carbon pool and vegetation carbon stock (Gulde et al., 2008; Warren and Ziegler, 2017). The sequestration of carbon as SOC is a major topic of research worldwide because of its great importance in climate change mitigation and inhibition of soil degradation (Vos et al., 2017; Rüegg et al., 2019). The balance between soil carbon inputs (i.e., vegetation and exogenous sources) and outputs (i.e. mineralization, erosion and leaching) determines the SOC stock, and the proportion of carbon input that becomes stabilized in SOC and the maintenance of its stability is vital to ensuring long-term SOC stocks (Rüegg et al., 2019). Moreover, land-atmosphere carbon exchanges can be altered by the changes in SOC stability, leading to dynamic variations in soil carbon release (Tian et al., 2016). Furthermore, SOC stability is critical to soil quality and fertility through the regulation of soil characterization and nutrient level (Yang et al., 2020). The concept of “SOC stability” refers to the resilience or resistance to changes (Doetterl et al., 2016), with the ideal situation that SOC remains constant in its molecular composition and concentration for long periods of time (Berhe and Kleber, 2013). To sum up, the biochemical recalcitrance of molecules, the spatial inaccessibility of SOC for decomposer organisms and enzymes, the stabilization by the interactions of SOC with mineral surfaces and metal ions, and physical protection from aggregates control the spatial heterogeneity of SOC stability (Lützow et al., 2006; Lorenz et al., 2009; Sierra et al., 2016; Tian et al., 2016).
Although there are no unified standards for the assessment of SOC stability, a series of complementary techniques enable the evaluation and interpretation of the SOC stabilization mechanisms in different soils. Various techniques (i.e., thermal analysis techniques and chemical separation methods) have been considered suitable metrics in the evaluation of SOC stability (Lorenz et al., 2009; Sierra et al., 2016; Soucémarianadin et al., 2018). SOC pools can be classified into labile and stable SOC pools (Gulde et al., 2008) or labile (active), intermediate and stable (persistent) SOC pools (Tian et al., 2016; Soucémarianadin et al., 2018; Lyu et al., 2021). The most stable organic carbon components can be stored for hundreds of years or longer as a result of the retardation of the internal physical, chemical and microbial cycles (Gulde et al., 2008). Stable SOC contributes more to the sequestration and storage of SOC than labile SOC (Gulde et al., 2008; Soucémarianadin et al., 2018). Correspondingly, the proportion of stable SOC in soil determines the stability of SOC and the capacity of soil to store SOC long term. The preservation of stable SOC enormously affects atmospheric CO2 levels, soil fertility, plant productivity, and the soil ecosystem (Lyu et al., 2021). Both natural and anthropogenic perturbations can alter SOC stability by affecting the SOC decomposition, which depends on physicochemical properties and carbon availability for microbial and enzyme activities (Yang et al., 2020). Therefore, it is of the utmost importance to investigate how SOC stabilization responds to the changes of environmental conditions so as to better understand the potential for enhancement of global carbon storage.
Strengthening soil carbon sequestration and CO2 fixation has become an urgent issue in the context of global climate change. Coastal wetlands feature with great capacity for carbon accumulation, with the potential to substantially affect the global carbon budget (Baustian et al., 2021). Specifically, it has been estimated that coastal wetlands account for only 0.2% of the ocean surface, but 50% of carbon stock is sequestrated in marine sediments (Macreadie et al., 2017). Furthermore, coastal wetlands are associated with high productivity and high capacity for the regulation of CO2 levels, causing them to be major carbon sinks (Lu et al., 2017). Consequently, coastal wetlands have gained an increasing amount of research and the protection and restoration of coastal wetlands are targets for greenhouse gas emissions offsetting and carbon sequestration projects (Kroeger et al., 2017). The soil carbon sequestration rates in coastal wetlands partly depend on their unique hydrologic conditions, as flooding duration, the oxygen-deficient soil environment and high salinity lead to low decomposition rate of organic matter and tidal oscillations could increase organic matter inputs (Chambers et al., 2013; Ferronato et al., 2019; Baustian et al., 2021). Moreover, variations in wetland hydrologic conditions would induce changes in the vegetation type and soil physiochemical characterization that further cause fluctuations in the soil carbon stock (Zhao et al., 2017; Ma et al., 2017). Notably, coastal wetlands may face increased flooding under future global climate change (Ferronato et al., 2016). Thus, the impact of hydrologic variations on SOC stabilization in coastal wetlands is worthy deeply probing into.
The fluctuations in hydrologic conditions will undoubtedly affect SOC stabilization in many ways, especially by augmenting carbon inputs and outputs. Moreover, it has been reported that waterlogging affects the chemical stability of soil organic matter by altering soil biochemical characteristics (i.e., enzyme activities and microbial activities) and the quality of organic matter (Ferronato et al., 2019). Lyu et al. (2021) showed that the sizes of intermediate and stable SOC pools were predominantly and positively controlled by the contents of nanocrystalline minerals. The initial interaction between minerals and SOM mediated controlled SOC stability by mediating the sorption of chemically diverse organic matter molecules via distinct surface areas and chemical functional group availabilities, and this mineralogic control has been reported by several studies conducted in volcanic soils, dry tropical forest soils, temperate forest soils and grassland soils (Lorenz et al., 2009; Soucémarianadin et al., 2018; Zhao et al., 2020; Lyu et al., 2021). However, few studies have examined their effects on SOC stabilization in coastal wetlands, to our knowledge. The current study aimed to investigate how different hydrologic conditions affect the contents of representative stabilized SOC fractions (Na2S2O8 resistant SOC and H2O2 resistant SOC), and stabilization mechanisms associated with minerals in typical coastal wetlands of the Yellow River Delta, China. The clarification of how SOC stabilization in coastal wetlands responds to hydrologic variations and SOC stabilization protected by minerals will inform the assessment of SOC stock in coastal wetlands, strategic decision making for wetland restoration and guidelines for climate change mitigation.
Materials and methods
Sampling sites, soil collection and analysis
Four typical wetlands in the Nature Reserve of the Yellow River Delta were selected as sampling sites, including freshwater-flooded Phragmites australis wetlands (FPW), tidal P. australis wetlands (TPW), non-flooded P. australis wetlands (NPW) and tidal Suaeda salsa wetlands (TSW). FPW, NPW and TSW are located on the north bank of the Yellow River, and TPW is located on the south bank of the Yellow River (Figure 1). Different hydrologic conditions were observed in these four wetlands. Soil cores were randomly collected to a depth of 25 cm and stratified into five layers at 5 cm interval in each wetland in October 2018. Three soil samples were collected for each soil increment. Samples for the measurement of soil bulk density (BD) were collected by the cutting-ring method with 100 cm3 cores. Methods for the pretreatment of soil samples and determination of BD, soil water content (WC), pH, electric conductivity (EC), total carbon (TC), total nitrogen (TN), soil organic matter (SOM) and soil base cation and anion (e.g., Na+, K+, Mg2+, Ca2+, Cl- and SO42-) contents can be found in Zhao et al. (2020).
The contents of Na2S2O8 resistant SOC and H2O2 resistant SOC represent the amount of stable SOC (also called chemically recalcitrant SOC and non-oxidizable SOC) and functionally passive SOC, respectively (Lorenz et al., 2009; Sierra et al., 2016). Thus, they are usually selected as representative stabilized SOC fractions. The determination of these two stabilized SOC fractions, acid oxalate-extractable Al/Fe/Si (Alo, Feo and Sio), dithionite-citrate-extractable Al/Fe/Si (Ald, Fed and Sid) and pyrophosphate-extractable Al/Fe/Si (Alp, Fep and Sip) was conducted with reference to modified chemical fractionation methods by Lorenz et al. (2009). Acid oxalate-extractable Al, Fe, and Si represent most organic complexes and irregularly arranged minerals such as allophane, ferrihydrite, and imogolite. Dithionite-citrate extracts Fe from both crystalline and poorly crystalline Fe oxides, and Al substituted into Fe oxides and produced by the partial dissolution of poorly ordered Al (oxy) hydroxides are represented by dithionite-citrate-extractable Al. All of the organically bound Al and an intermediate percentage of the organically bound Fe were extracted using sodium pyrophosphate. The fraction of total Fe oxides with poor crystallinity is represented by the Feo/Fed ratio. As a result, Feo minus Fep reflects the amount of ferrihydrite, while Fed minus Feo represents crystalline Fe oxides. The ratio of organically-bound to total amorphous aluminum, or the proportion of aluminum that is in organic complexes, is represented by the Alp/Alo ratio. Sesquioxide content is denoted by Alo+0.5Feo, which is also indicative of the amount and status of nanocrystalline minerals (Lyu et al., 2021).
Statistical analysis
One-way analysis of variance (ANOVA) was conducted to identify the differences in soil properties, Na2S2O8 resistant SOC, H2O2 resistant SOC, acid oxalate extractable Al/Fe/Si, dithionite-citrate extractable Al/Fe/Si, pyrophosphate-extractable Al/Fe/Si and mineral phase indicators among the four wetlands using SPSS 22.0 software package. Differences were considered to be significant if p < 0.05. Redundancy analysis (RDA), correlation analysis and single variable linear regression were also performed to analyze the relationships between Na2S2O8 resistant SOC, H2O2 resistant SOC, Al/Fe/Si oxides, mineral phase indicators and soil properties with Canoco 4.5 software package and SPSS 22.0 software package, respectively. Figures were created using Origin 2019 software package.
Results
Soil physiochemical characteristics in the four wetlands
The SOM, TC, and TN contents were significantly higher in TPW than in NPW (p < 0.05), but no significant differences were observed among those in TPW, TSW, and FPW (p > 0.05, Table 1). The C/N ratio was not significantly different among the soils of the four wetlands (p > 0.05). Soil BD in TPW (< 1.5 g cm−3) was significantly lower than that of the other three wetlands (>1.5 g cm−3, p < 0.05). In general, soil EC, Na+, K+, Mg2+, Ca2+, Cl−, and SO42− contents in TSW soils were significantly higher than in the other three wetlands (p < 0.05), with the lowest values of EC, Na+, and Cl− occurring in FPW soils and K+, Mg2+, Ca2+, and SO42− appearing in NPW soils, respectively. For pH, significantly higher values (>9) were observed in the soils of NPW than in the soils of the other three wetlands (p < 0.05). The soil texture of the four wetlands was mainly composed of sand (>70%), with much lower proportions of clay and silt. The silt and sand contents in TPW were significantly higher and lower than those of NPW and FPW (p < 0.05). The WC was significantly higher in FPW than that in the other three wetlands (p < 0.05). Overall, flooding caused by tides or freshwater increased and reduced soil EC and salt ions (except for Ca2+), respectively. Compared with NPW which lacks flooding from tides or freshwater, flooding conditions in TSW, TPW, and FPW increased the SOM, TN, silt, and WC contents, and lowered the sand content and pH.
The contents and profile distribution of the two stabilized SOC fractions
The Na2S2O8 resistant SOC and H2O2 resistant SOC contents in the four wetlands are shown in Figure 2. Both Na2S2O8 resistant SOC and H2O2 resistant SOC showed no significant differences among the four wetlands (p > 0.05), and the mean values of Na2S2O8 resistant SOC at 0-25 cm soil depth were lower than H2O2 resistant SOC in all four wetlands. The highest mean values of Na2S2O8 resistant SOC and H2O2 resistant SOC were observed in FPW (1.44 ± 0.43 g kg-−1) and TPW (1.79 ± 0.40 g kg−1), respectively, while the lowest mean values of Na2S2O8 resistant SOC and H2O2 resistant SOC were found in NPW (1.19 ± 0.44 g kg−1) and TSW (1.50 ± 0.52 g kg−1), respectively.
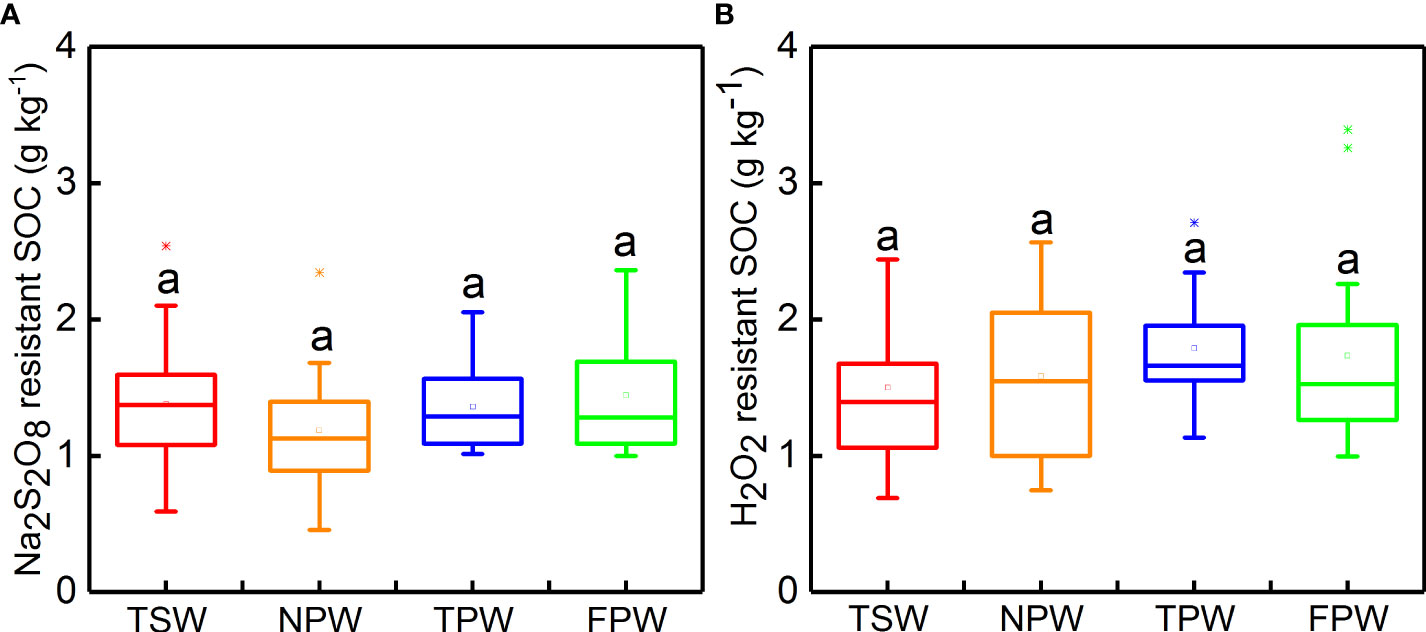
Figure 2 Box chart of Na2S2O8 resistant SOC (A) and H2O2 resistant SOC (B) in 0-25 cm soils in the four wetlands. (Different lowercase letters represent significant differences at p < 0.05 level).
Figure 3A shows the profile distribution of Na2S2O8 resistant SOC in the 0-25 cm soils of the four wetlands. The Na2S2O8 resistant SOC in soils of TSW, NPW and TPW showed an “increasing before decreasing” tendency as depth increased, with higher Na2S2O8 resistant SOC levels occurring in the 10-15 cm soils. And the highest values of Na2S2O8 resistant SOC among five soil layers in TSW, NPW and TPW were 1.70 ± 0.37 g kg-1, 1.53 ± 0.72 g kg-1 and 1.90 ± 0.13 g kg-1, respectively. The Na2S2O8 resistant SOC in 0-5 cm soils was significantly higher in FPW (1.80 ± 0.62 g kg-1) than in NPW (0.82 ± 0.31 g kg-1, p < 0.05), while Na2S2O8 resistant SOC in other soil layers showed no significant difference among the four wetlands (p > 0.05).
The distribution pattern of soil H2O2 resistant SOC was similar to that of Na2S2O8 resistant SOC in TSW, NPW and TPW, showing an initial increasing then decreasing trend (Figure 3B). The H2O2 resistant SOC content in 0-5 cm soils was significantly higher in soils of TPW (1.85 ± 0.37 g kg-1) and FPW (2.35 ± 0.87 g kg-1) than that in soils of NPW (0.90 ± 0.16 g kg-1, p < 0.05), while a significantly higher value of H2O2 resistant SOC in 10-15 cm soils of NPW (2.19 ± 0.30 g kg-1) and TPW (2.10 ± 0.54 g kg-1) than that of FPW (1.42 ± 0.26 g kg-1) was observed (p < 0.05). The H2O2 resistant SOC in 5-10 cm, 15-20 cm and 20-25 cm soils showed no significant differences among the four wetlands (p > 0.05), with higher values occurring in soils of FPW (5-10 cm, 2.17 ± 1.08 g kg-1).
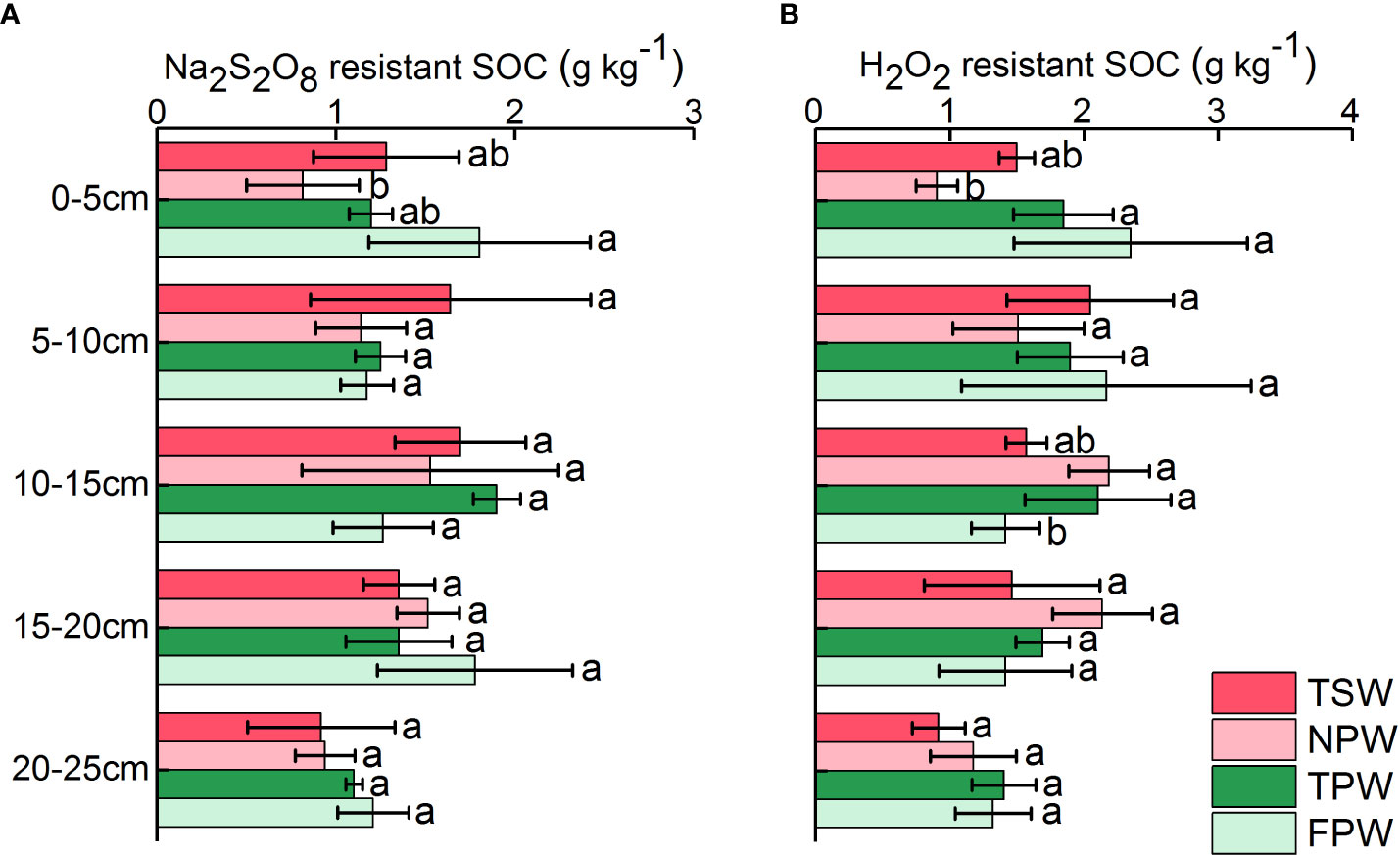
Figure 3 Profile distribution of soil Na2S2O8 resistant SOC (A) and H2O2 resistant SOC (B) in the four wetlands. (Different lowercase letters represent significant differences between the same layers of the four wetlands at p < 0.05 level).
Comparison of Al, Fe and Si oxides in soils of the four wetlands
The contents of Al extracted by acid-oxalate, dithionite-citrate and pyrophosphate in the soils of the four wetlands are shown in Figures 4A-C, respectively. The content of soil Alo in NPW was significantly lower than that in the other three wetlands (p < 0.05), with a mean value of 0.22 ± 0.04 g kg-1. However, the content of soil Alo showed no significant difference among TSW, TPW and FPW (p > 0.05), with the highest mean soil Alo content observed in TPW (0.39 ± 0.10 g kg−1). TSW (0.38 ± 0.09 g kg-1) and TPW (0.40 ± 0.06 g kg-1) had significantly higher soil Ald contents than NPW (0.28 ± 0.03 g kg-1, p <0.05). However, Ald in FPW (0.40 ± 0.12 g kg-1) did not differ significantly from NPW and TSW (p > 0.05), but was significantly lower than TPW (p < 0.05). As for Alp, a significantly lower value in NPW (0.033 ± 0.01 g kg-1) was found than in FPW (0.054 ± 0.04 g kg-1), but no significant differences were observed among TSW, NPW and TPW, or among TSW, NPW and FPW (p > 0.05).
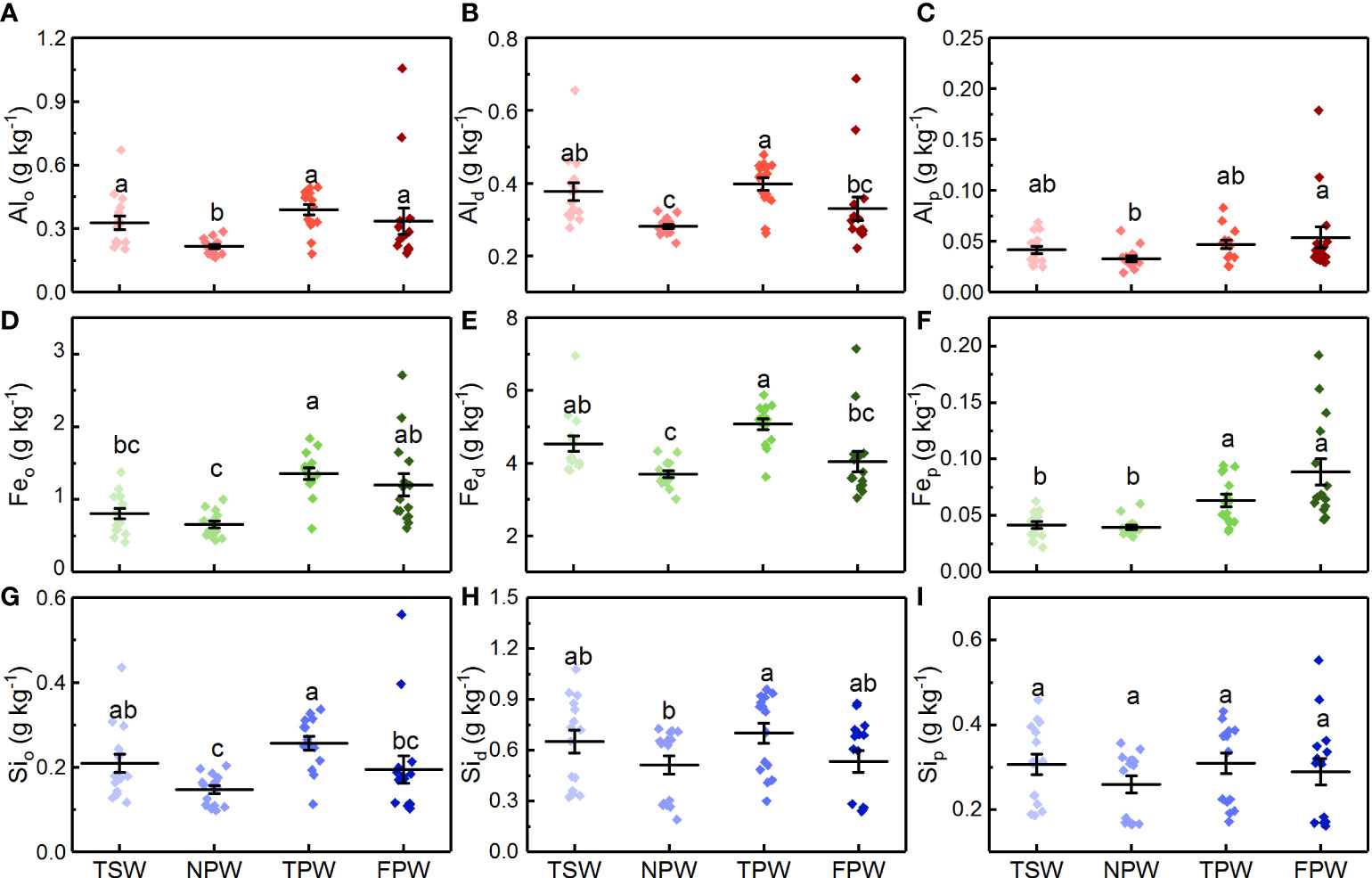
Figure 4 Comparison of Alo (A), Ald (B), Alp (C), Feo (D), Fed (E), Fep (F), Sio (G), Sid (H) and Sip (I) contents in the four wetlands. (Different lowercase letters represent significant differences between the same layers of the four wetlands at p < 0.05 level).
The contents of acid-oxalate, dithionite-citrate and pyrophosphate extractable Fe are shown in Figures 4D-F, respectively. The content of soil Feo in NPW (0.65 ± 0.17 g kg-1) was significantly lower than that in TPW (1.36 ± 0.30 g kg-1) and FPW (1.20 ± 0.59 g kg-1, p < 0.05), but showed no significant difference with TSW (0.80 ± 0.28 g kg-1, p > 0.05). TSW had a significantly lower soil Feo content than TPW (p < 0.05), but did not differ from FPW (p > 0.05). Although the content of soil Fed in NPW (3.70 ± 0.36 g kg-1) was significantly lower than that in TSW (4.53 ± 0.82 g kg-1) and TPW (5.07 ± 0.57 g kg-1, p < 0.05), no significant difference was observed between NPW and FPW (p > 0.05). Moreover, no significant difference was observed between TSW and TPW or between TSW and FPW (p > 0.05). Both TSW and NPW had significantly lower soil Fep contents than TPW (0.063 ± 0.02 g kg-1) and FPW (0.088 ± 0.05 g kg-1, p < 0.05), but no significant differences were found between TSW (0.042 ± 0.01 g kg-1) and NPW (0.039 ± 0.01 g kg-1), or between TPW and FPW (p > 0.05).
As shown in Figures 4G-I, the contents of soil Sio, Sid and Sip in the four wetlands decreased in the order TPW > TSW > FPW > NPW. The content of Sio in NPW (0.15 ± 0.04 g kg-1) was significantly lower than that in TSW (0.21 ± 0.09 g kg-1) and TPW (0.26 ± 0.06 g kg-1, p < 0.05), but did not differ from that of FPW (0.21 ± 0.13 g kg-1, p > 0.05). TPW had a significantly higher content of Sid (0.70 ± 0.23 g kg-1) than NPW (0.51 ± 0.21 g kg-1, p < 0.05). However, no significant differences were found among TSW, NPW and FPW, or among TSW, TPW and FPW (p > 0.05). Unlike the Sio and Sid, the contents of soil Sip in the four wetlands were not statistically different (p > 0.05).
Mineral phase indicators of Al, Fe and Si in the four wetlands
Table 2 shows the comparison of mineral phase indicators (Feo/Fed ratio, Fed-Feo, Feo-Fep, Alp/Alo ratio and Alo+0.5Feo) among the four wetlands. There were significantly higher poorly crystalline Fe oxide contents (based on Feo/Fed ratio) in TPW and FPW than in TSW and NPW (p < 0.05), with mean values > 0.2. As shown in Table 2, TSW and TPW had significantly higher crystalline Fe oxides contents (Fed-Feo, 3.73 ± 0.62 g kg-1 and 3.72 ± 0.36 g kg-1, respectively) than NPW and FPW (p < 0.05), but no significant difference was found between TSW and TPW or between NPW and FPW (p > 0.05). FPW has the lowest Fed-Feo content of the four wetlands (< 3 g kg-1). The ferrihydrite content represented by Feo-Fep was significantly higher in TPW and FPW than those in TSW and NPW (p < 0.05), which were higher than 1 g kg-1 in TPW and FPW. As the indicator for the relative amount of Al in organic complexes, the Alp/Alo ratio in TPW (0.12) was significantly lower than that in NPW and FPW (p < 0.05), but showed no significant difference with TSW (p > 0.05). The Alp/Alo ratio in FPW was significantly higher than that in TSW (p < 0.05), but did not significantly differ from NPW (p > 0.05). The content of sesquioxides represented as Alo+0.5Feo in TPW (> 1 g kg-1) was significantly higher than that in TSW and NPW (p < 0.05), but showed no significant difference with that in FPW (p > 0.05).
Relationships between the two stabilized SOC fractions, Al/Fe/Si oxides, mineral phase indicators and soil properties
The RDA between the two stabilized SOC fractions, Al/Fe/Si oxides, mineral phase indicators, and soil properties is shown in Figure 5. The cumulative percentage of variance of two stabilized SOC fractions was 79.1% of Axis 1 (62.1%) and Axis 2 (17.0%). The two stabilized SOC fractions, SOM, TC, TN, C/N ratio, WC, clay, silt, Al/Fe/Si oxides (except for Sid and Sip), and mineral phase indicators were mainly distributed on the right part of Axis 1, and the two stabilized SOC fractions were highly and positively affected by SOM, TC, TN, WC, clay, silt, Feo, Fep and Alp. The sand content, BD, and EC were located on the left part of Axis 1, and these soil edaphic factors negatively influenced the two stabilized SOC fractions. The correlation analysis also showed that Na2S2O8 resistant SOC was significantly and positively correlated with SOM, TC, TN, clay, WC, Al/Fe/Si oxides (except for Fep, Sid and Sip), Fed-Feo, Feo-Fep and Alo+0.5Feo (Figure 6, p < 0.05), and significantly and negatively correlated with sand (p < 0.05, Figure 6). A significantly positive relationship was observed between H2O2 resistant SOC and SOM, TC, TN, WC, Fe/Al/Si oxides (except for Sid and Sip), as well as Fed-Feo, Feo-Fep and Alo+0.5Feo (p < 0.05).
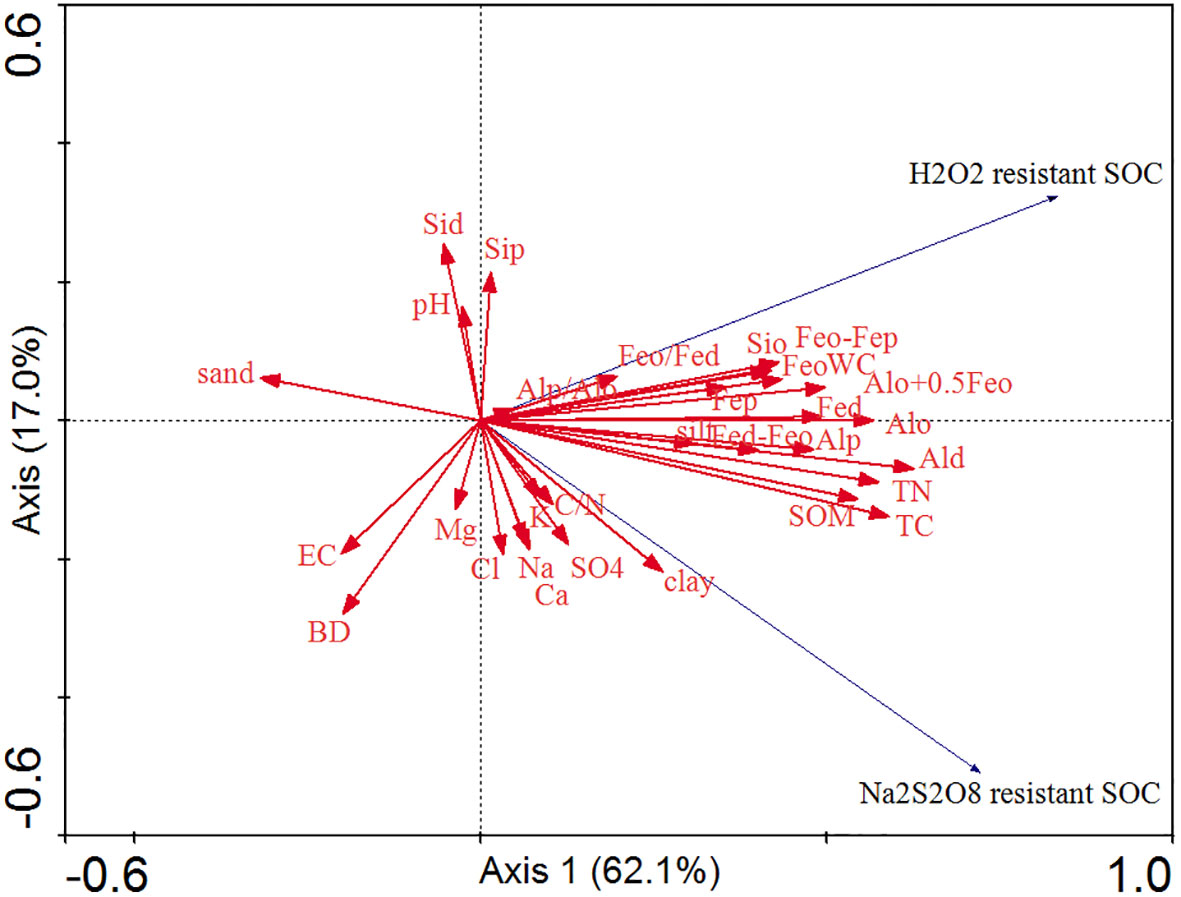
Figure 5 Redundancy analysis between the two stabilized SOC fractions, Fe/Al/Si oxides, mineral phase indicators, and soil properties.
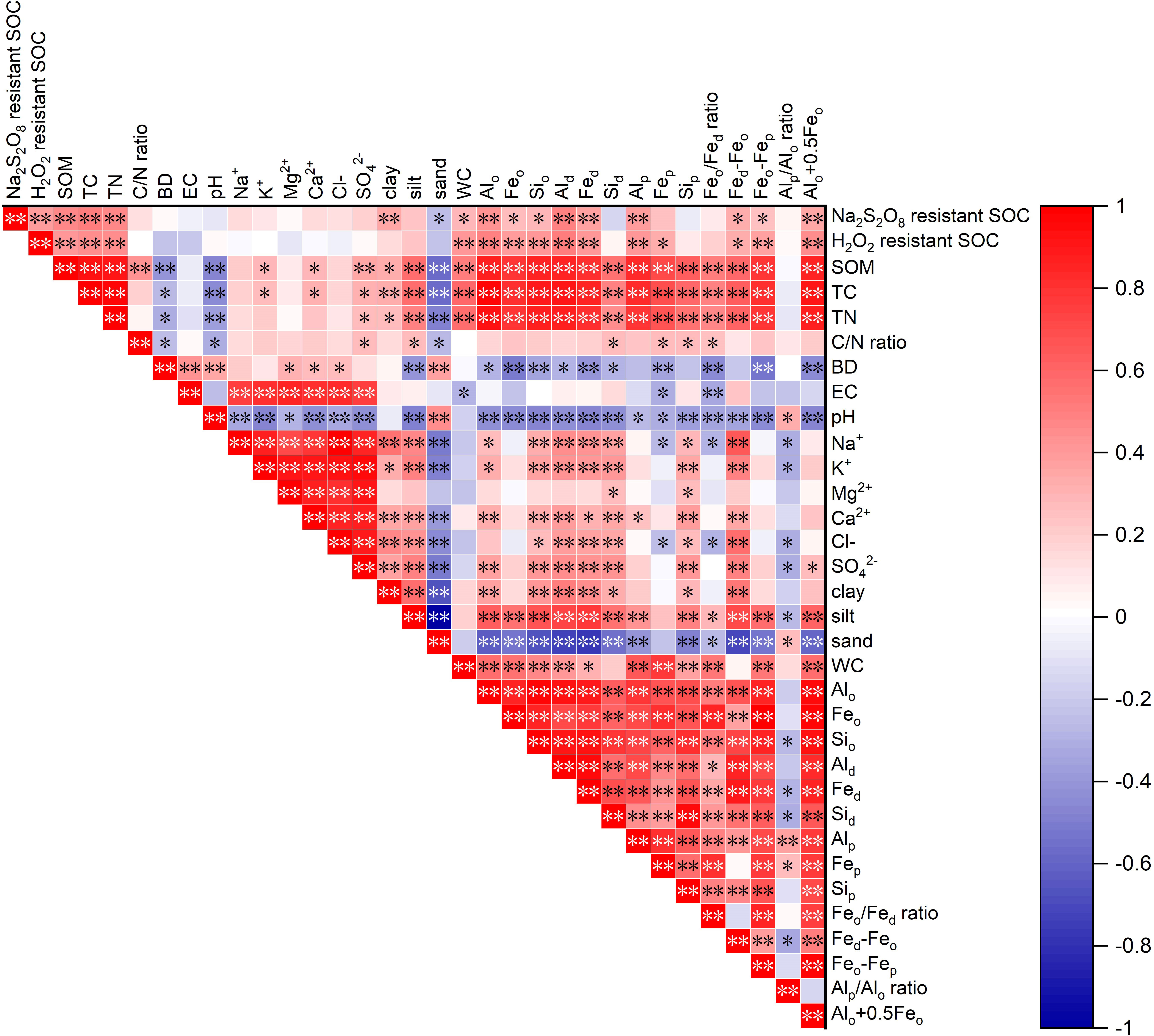
Figure 6 Correlation matrix of the two stabilized SOC fractions with Al/Fe/Si oxides, mineral phase indicators and soil properties. [*Correlation is significant at p < 0.05 level (2-tailed); **Correlation is significant at p < 0.01 level (2-tailed)].
As shown in Table 3, Feo, Sio, Ald, Fed, Alp, Fep, Sip, Feo/Fed ratio, Feo-Fep and Alo+0.5Feo all significantly contributed to the variability of Na2S2O8 resistant SOC and H2O2 resistant SOC (p < 0.01) in 0-5 cm soils. Moreover, Sid and Fed-Feo explained 41% and 46% of the variation of H2O2 resistant SOC (p < 0.05). In particular, the amounts of Feo, Sio, Ald, Alp, Fep, Sip, Feo-Fep and the indicator for amorphous mineral (Alo+0.5Feo) were related to more than 60% of the variability of Na2S2O8 resistant SOC (p < 0.01), with Feo, Sio, Ald, Feo-Fep and Alo+0.5Feo even accounting for more than 80% of the variability of H2O2 resistant SOC (p < 0.001). However, both the variability of Na2S2O8 resistant SOC and H2O2 resistant SOC were independent of the content of Alo and the Alp/Alo ratio (p > 0.05). Additionally, the amount of Sid and Fed-Feo were not related to Na2S2O8 resistant SOC (p > 0.05). In 10-15 cm soils, Alo, Sio, and dithionite extractable Al/Fe/Si oxides each attributed more than 30% of the variability of Na2S2O8 resistant SOC (p < 0.05), whereas the content of H2O2 resistant SOC was independent of Al/Fe/Si oxides and mineral phase indicators.
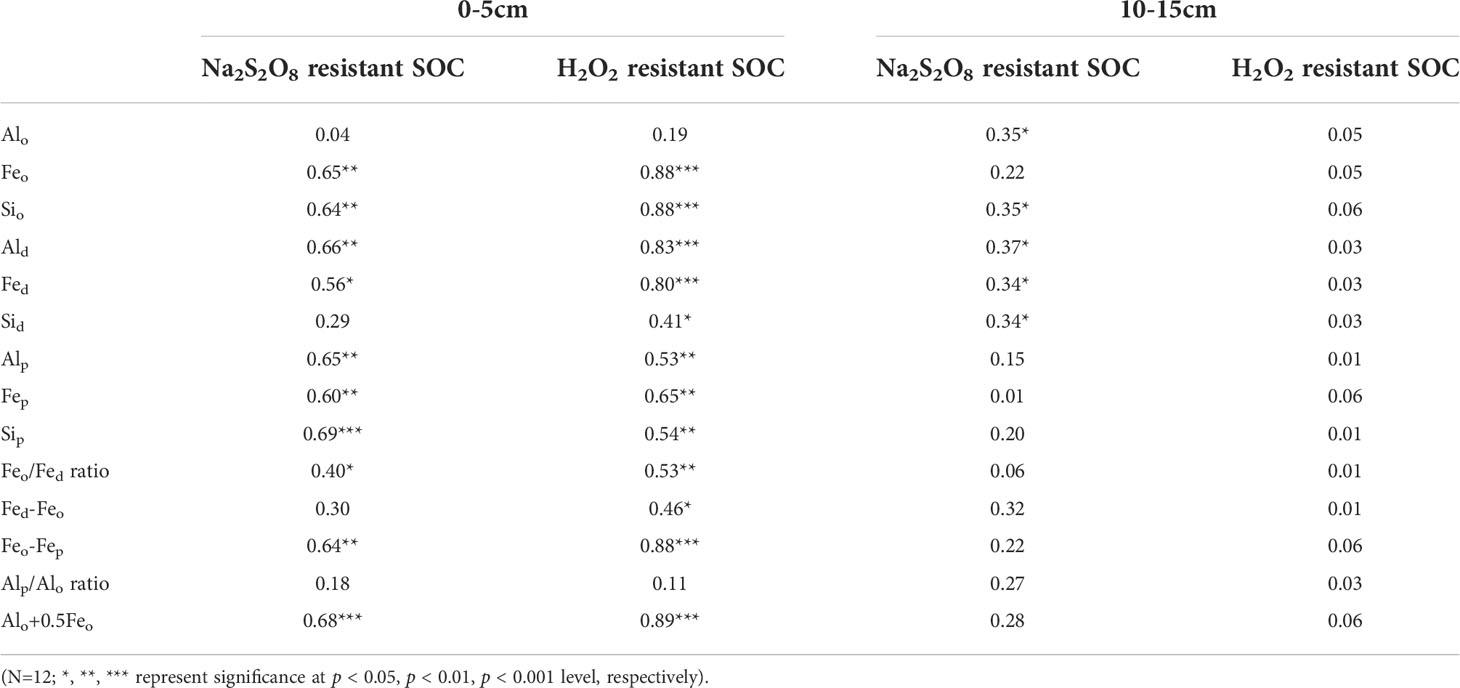
Table 3 Coefficients of determination (R2 values) for single linear regressions of Na2S2O8 resistant SOC and H2O2 resistant SOC as a function of variables (g kg-1) in 0-5 and 10-15cm soils in four wetlands (Regressions for 5-10, 15-20 and 20-25 cm soils were not shown as the two stabilized SOC fractions were not statistically different among four wetlands).
Discussion
Mineral contents and mineral phase indicators in the four wetlands
The contents of Al/Fe/Si oxides extracted by acid oxalate, dithionite–citrate and pyrophosphate were much lower than previously measured in both dry tropical forest and tropical rain forest, which is mainly caused by the difference in soil parent material and weathering process (Lorenz et al., 2009). The significantly lower contents of Alo, Feo, Sio, Ald, Fed and Sid in NPW than in TPW indicate that non-crystalline clay minerals and total “free” oxides were more enriched in TPW than in NPW. The significantly higher contents of Alp and Fep in FPW than in NPW indicate that organically-bound Al and the intermediate fraction of organically-bound Fe oxides might play a more important role in SOC stabilization in FPW than in NPW.
The contents of poorly crystalline Fe oxides as indicated by the Feo/Fed ratio in 0-25 cm soils were significantly lower in NPW than in TPW and FPW, indicating that these two wetlands affected by tidal/freshwater flooding had more active Fe oxides. Higher Fed-Feo contents generally represent frequent wet and dry cycles and high weathering intensity (Lorenz et al., 2009), which was confirmed by the current study. In our study, TSW and TPW were affected by tidal oscillations and contained significantly higher poorly crystalline Fe oxides represented as Fed-Feo than NPW and FPW. Additionally, the complexion of Al and organic acids existed in soils of the four wetlands as the content of soil Sio was lower than 0.6 g kg-1 and the Alp/Alo ratio was no less than 0.5 (Lorenz et al., 2009).
SOC stabilization in the four wetlands
The SOC stabilization in the four wetlands affected by different hydrologic conditions was assessed by determining the resistance of SOC against Na2S2O8 and H2O2, which represent stable SOC and functionally passive SOC, respectively. A significant difference in the content of Na2S2O8 resistant SOC was only observed between NPW and FPW or TPW in 0-5 cm soils, and the mean contents of Na2S2O8 resistant SOC and H2O2 resistant SOC in NPW was lower than that in other three wetlands. Therefore, the three wetlands with the intermittent or long term flooding play a more important role in long-term carbon sequestration than NPW.
Long-term accumulation of soil carbon depends on the “net balance” between inputs and outputs in wetland soils (Kirwan et al., 2013). In general, both the aboveground and belowground biomass of P. australis wetlands are higher than those of S. salsa wetlands (Zhang et al., 2020), indicating that the carbon inputs tend to be higher in P. australis wetlands. However, SOM, Na2S2O8 resistant SOC and H2O2 resistant SOC in the soils of TSW were not significantly different from the other three P. australis wetlands in 0-25 cm soils (Table 1 and Figure 2). Moreover, the contents of Na2S2O8 resistant SOC and H2O2 resistant SOC in soils of NPW were significantly lower than those in soils of TPW (H2O2 resistant SOC) and FPW in 0-5 cm soil layer (Na2S2O8 resistant SOC and H2O2 resistant SOC). This shows that decomposition rates and other factors besides litter input caused by different vegetation type importantly controlled the carbon-storage potential more in these four wetlands. For example, the priming effect could lead to a decrease in SOC stock, which accelerates decomposition rates through the stimulation of fresh organic matter inputs (Rüegg et al., 2019). The effects of oxygen availability on SOC stock should also be taken into account as oxygen availability affects microbial activity and ultimately impacts SOC decomposition. Li et al. (2021) found that oxygen availability regulated key determinants of SOC mineralization rate in paddy soils. Undoubtedly, the flooded soil status and the anaerobic soil environment caused by tidal oscillations (in TSW and TPW) and freshwater flooding (in FPW), flooding duration and soil salinity all contribute to the slow decomposition of SOM, and finally result in the accumulation of SOM over time (Chanlabut et al., 2020). This was also shown in our previous study, which confirmed that tidal oscillations benefit soil carbon sequestration (Zhao et al., 2020).
Mineral protection on SOC stabilization and its controlling factors
The protection of nanocrystalline minerals (active Al and Fe) on SOC stabilization has been reported in various soil types such as volcanic soils, dry tropical forest soils and grassland soils (Lorenz et al., 2009; Zhao et al., 2020; Lyu et al., 2021). Soil Al/Fe cations can combine with SOC through electrostatic adsorption, ligand exchange, surface complexation, hydrophobic interaction, hydrogen bonding, and cation bridging, thus forming stable organic carbon components (Catoni et al., 2016). Furthermore, high specific surface area and low crystallinity of Al/Fe oxides affect the adsorption process of organic matter, and the solubility change of redox active metals drives the coprecipitation process of organic matter and metals (Eusterhues et al., 2005; Wiesmeier et al., 2019). Organic particles in soil can be electrostatically adsorbed with Ald and Fed, which reduces the turnover rate of organic matter and promotes the preservation of organic compounds in sediments (Kögel-Knabner et al., 2008; Xue et al., 2020). The significantly positive relationship between Na2S2O8 resistant SOC, H2O2 resistant SOC and Al/Fe/Si oxides (except for Sid and Sip), Fed-Feo, Feo-Fep and Alo+0.5Feo (Figure 5 and Figure 6) indicates that minerals played an important role in the stabilization of SOC. The significantly lower values of Alo, Feo, Sio, Ald, Fed, Fed-Feo, Feo-Fep and Alo+0.5Feo in NPW than in TPW or FPW may explain why lower stabilized SOC fractions were observed in NPW. Amorphous and low-crystalline minerals have a stronger ability to stabilize organic carbon than crystalline minerals by providing a broad surface area and complexing ability, organic biomolecules form metastable and intermediate complexes (Wiseman and Püttmann, 2006; Basile-Doelsch et al., 2007; Paul et al., 2008; Berhe et al., 2012). Thus, the amount of amorphous minerals and crystalline Fe oxides as indicated by the amount of Alo+0.5Feo and the Fed/Feo ratio, respectively, are more important to the protection of two stabilized SOC fractions in 0-5 cm soils (Table 3).
The significantly lower mean contents of Al/Fe/Si oxides (except for Sip) in NPW than in TPW or FPW (Figure 4), and the significantly lower values of mineral phase indicators in NPW than those in TPW indicate that the hydrologic conditions might affect the formation and amount of Al/Fe/Si oxides. Saleh et al. (2013) reported that waterlogging significantly increased the concentrations of acetic acid-extractable Si and Fe in a laboratory experiment. Compared with dry land, flooded paddy soils contained higher contents of Al/Fe oxides, and higher soil moisture in flooded soils can accelerate the association of Fe and SOC (Zhou et al., 2009; Balint et al., 2013). In addition, pH determines the binding site of Al/Fe/Si oxides with SOM, consequently controlling the transformations in the type of soil Al/Fe/Si oxides (Kögel-Knabner et al., 2008; Merino et al., 2017; Gartzia-Bengoetxea et al., 2020). Lyu et al. (2021) found that high soil moisture accompanying low soil pH promotes the formation of nanocrystalline minerals. In the current study, there was a significantly positive correlation between soil WC and Al/Fe/Si oxides and mineral phases (except for Sid, Fed-Feo and Alp/Alo ratio), while pH negatively affected the contents of Al/Fe/Si oxides (Figure 6). Higher soil WC and lower pH in TSW, TPW and FPW than NPW may have promoted the precipitation of Al/Fe/Si oxides and mineral phases to some extent, partly explain the lower Al/Fe/Si oxides contents in NPW than in the other three wetlands.
The interaction between Al/Fe oxides characterized by high activity and clay particles featured with low surface area adsorption can form crystalline or poor crystalline minerals and physically stabilize SOC (Wagai and Mayer et al., 2007; Sarkar et al., 2018). The correlation analysis showed that, except for Alp/Alo ratio, soil Al/Fe/Si oxides and mineral phase indicators had positive correlations with soil clay and silt contents, and had a very significant negative correlation with soil sand (except for Fep, p < 0.05). Additionally, through functioning as binders, Al/Fe oxides can form stable aggregates with clay and silt particles so as to protect SOC (Six et al., 2004). Conversely, a high sand content inhibits the enrichment of Al/Fe oxides, and reduces their protection effects on SOC (Cai et al., 2016). Thus, the lower silt content and higher sand content in NPW are responsible for lower Al/Fe oxides than the other three wetlands to some degree.
Furthermore, the quality of the carbon (specifically, the C/N ratio) and the availability of nutrients influence the reduction and dissolution of Al/Fe/Si oxides in soils (Pallud et al., 2020). Petruzzelli et al. (2005) found that the relatively low C/N ratio (e.g., 9.7) and organic matter content promotes the reduction degree of ferrihydrite and goethite. Thus, it can be concluded that the lower C/N ratio and SOM content might be another reason for the lower contents of Feo, Fed and Fep in NPW than in the other three wetlands.
Martí-Roura et al. (2019) found that some base ions in soil can aggregate with Al and Fe cations and form minerals, a process that is conducive to the enrichment of Al ions and their mineral phases. Our correlation analysis showed that the soil base cations were significantly positively correlated with Al/Fe/Si oxides and mineral phases (except for Feo, Alp, Feo/Fed ratio, Feo-Fep, Alp/Alo ratio and Alo + 0.5Feo). This corresponds to the higher Al/Fe/Si oxides contents in TSW, TPW and FPW (Table 1). Moreover, the correlation analysis also showed that Na+ and Cl- were significantly negatively correlated with Fep, Feo/Fed ratio and Alp/Alo ratio, which may further reduce Al/Fe/Si oxides contents and mineral phases in the NPW. Overall, the deficiency of flooding in NPW might be the reason for lower acid oxalate, dithionite-citrate and pyrophosphate extractable Al/Fe/Si oxides and lower contents of Na2S2O8 resistant SOC and H2O2 resistant SOC.
In brief, soil edaphic factors can indirectly alter SOC stabilization through affecting the formation of Al/Fe/Si oxides and minerals phase. Thereby, it can be concluded that hydrologic conditions posed both direct and indirect effects on the mineral protection aspect of SOC stabilization.
Direct influence of some edaphic factors on SOC stabilization
Numerous studies have shown that environmental variables (i.e., soil depth, moisture, vegetation type, and climate) affected SOC stability (Sierra et al., 2016; Soucémarianadin et al., 2018; Lyu et al., 2021). The quantity and quality of SOM affect soil carbon content by influencing the functional groups and quantity of soil organisms (Chen et al., 2020), while nitrogen input can affect the wetland carbon transformation by improving the productivity and organic matter content of the wetland ecosystem (Bragazza et al., 2006). Neff et al. (2002) reported that long - term nitrogen input through nitrogen fertilization can stabilize the heavier and mineral-associated SOC fractions. This means that high nitrogen content can increase the input of C, and store carbon for a longer period. In this study, Na2S2O8 resistant SOC and H2O2 resistant SOC were significantly and positively correlated with SOM, TC and TN (Figure 6). The contents of TC and TN in NPW were lower than in TSW, TPW and FPW, which may partly explain the generally lower Na2S2O8 resistant SOC and H2O2 resistant SOC contents in NPW than in the other three wetlands.
Among all the edaphic factors, soil texture is particularly important in carbon sequestration. Clay and silt particles combine with soil carbon to form stable clay-organic carbon complexes in soils, which play an important role in preventing microbial oxidation (Six et al., 2002). Cai et al. (2016) reported that fine soil particles (i.e., silt and clay) are capable of stabilizing mineral-related organic carbon and sequestrating persistent SOC. Moreover, Wiesmeier et al. (2014) showed that clay play an important role in maintaining the stability of soil organic carbon. These results support the conclusion that finer soil particles stabilize more SOC than coarser sand particles. The significant relationships between Na2S2O8 resistant SOC and clay (positive), silt (positive), and sand (negative) (Figure 6, p < 0.05) may explain the higher Na2S2O8 resistant SOC accumulation in TSW, TPW, and FPW compared with NPW (Figure 2).
Soil WC could affect soil carbon stability by altering the mineralization rate of SOC (Yin et al., 2019). Ferronato et al. (2019) showed that the decreased oxygen content under long-term flooding and saturated soils reduced the decomposition of SOM. Moreover, soil WC controls the transport of nutrients, the diffusion of enzymes, the movement of microorganisms and the efficiency of microbial utilization of nutrients, further modulating soil carbon stock (Qu et al., 2021). In the current study, Na2S2O8 resistant SOC and H2O2 resistant SOC were significantly correlated with soil WC in a positive way (Figure 6, p < 0.05). The significantly higher soil WC in FPW than in TSW, TPW and NPW may be another reason for higher Na2S2O8 resistant SOC and H2O2 resistant SOC in FPW.
Conclusions
This study explored the changes in the content of chemically separated stable SOC (Na2S2O8 resistant SOC) and functionally passive SOC (H2O2 resistant SOC) and associated mineral protection provide by Al/Fe/Si oxides extracted by acid oxalate, dithionite-citrate and pyrophosphate, respectively, in four typical coastal wetlands under different hydrologic conditions. Compared with NPW, flooding caused by tides in TPW or freshwater in FPW significantly enhanced the contents of both Na2S2O8 resistant SOC and H2O2 resistant SOC in 0-5 cm soil layer. Multiple statistical analyses showed that Feo, Sio, Ald, Fed, Sid, Alp, Fep, Sip, Feo/Fed ratio, Fed-Feo, Feo-Fep and Alo+0.5Feo provided mineral protection on the two stabilized SOC fractions, and some edaphic factors also attributed to the variability of Na2S2O8 resistant SOC and H2O2 resistant SOC. Thereby, the effects of hydrologic conditions on the amount of Al/Fe/Si oxides, the values of mineral phase indicators and edaphic factors (i.e., SOM, TN, pH, WC and soil texture) caused the variations in Na2S2O8 resistant SOC and H2O2 resistant SOC among the four wetlands. Overall, more attention should be paid to the effects of hydrologic conditions on SOC stabilization and their possible impacts on carbon sequestration in coastal wetlands. Furthermore, from the perspective of carbon sequestration capacity, the strategic decision on wetland type selection when conducting a degraded wetland restoration program may refer to the results of this study.
Data availability statement
The original contributions presented in the study are included in the article/supplementary material. Further inquiries can be directed to the corresponding author.
Author contributions
HZ: investigation, methodology, visualization, writing-original draft. JJ: formal analysis, writing - original draft. QZ: conceptualization, investigation, writing–review and editing, funding acquisition. JW: funding acquisition, supervision. YG: writing-review and editing, funding acquisition, supervision. YH: investigation, methodology. GC: supervision. All authors contributed to the article and approved the submitted version.
Acknowledgments
This study was financially supported by Major Scientific and Technological Innovation Projects in Shandong Province (2021CXGC011201), Joint Funds of the National Natural Science Foundation of China (U1806217), Shandong Provincial Natural Science Foundation (ZR2019BD007, ZR2021MD124 and ZR2021MD126), and Science and Technology Development Fund of the Yellow River Institute of Hydraulic Research (202211).
Conflict of interest
The authors declare that the research was conducted in the absence of any commercial or financial relationships that could be construed as a potential conflict of interest.
Publisher’s note
All claims expressed in this article are solely those of the authors and do not necessarily represent those of their affiliated organizations, or those of the publisher, the editors and the reviewers. Any product that may be evaluated in this article, or claim that may be made by its manufacturer, is not guaranteed or endorsed by the publisher.
References
Balint R., Orbeci C., Nechifor G., Plesca M., Ajmone-Marsan F. (2013). Effect of redox conditions on the crystallinity of fe oxides in soil. Rev. Chimie 64, 1218–1223.
Basile-Doelsch I., Amundson R., Stone W. E. E., Borschneck D., Bottero J. Y., Moustier S., et al. (2007). Mineral control of carbon pools in a volcanic soil horizon. Geoderma 137, 477–489. doi: 10.1016/j.geoderma.2006.10.006
Baustian M. M., Stagg C. L., Perry C. L., Moss L. C., Carruthers T. J. B. (2021). Long-term carbon sinks in marsh soils of coastal louisiana are at risk to wetland loss. J. Geophysical Research: Biogeosci. 126, 1–17. doi: 10.1029/2020JG005832
Berhe A. A., Kleber M. (2013). Erosion, deposition, and the persistence of soil organic matter: Mechanistic considerations and problems with terminology. Earth Surface Processes Landforms 38, 908–912. doi: 10.1002/esp.3408
Berhe A. A., Suttle K. B., Burton S. D., Banfield J. F. (2012). Contingency in the direction and mechanics of soil organic matter responses to increased rainfall. Plant Soil 358, 371–383. doi: 10.1007/s11104-012-1156-0
Bragazza L., Freeman C., Jones T., Rydin H., Limpens J., Fenner N., et al. (2006). Atmospheric nitrogen deposition promotes carbon loss from peat bogs. Proc. Natl. Acad. Sci. - PNAS 103, 19386–19389. doi: 10.1073/pnas.0606629104
Cai A., Feng W., Zhang W., Xu M. (2016). Climate, soil texture, and soil types affect the contributions of fine-fraction-stabilized carbon to total soil organic carbon in different land uses across China. J. Environ. Manage. 172, 2–9. doi: 10.1016/j.jenvman.2016.02.009
Catoni M., D'Amico M. E., Zanini E., Bonifacio E. (2016). Effect of pedogenic processes and formation factors on organic matter stabilization in alpine forest soils. Geoderma 263, 151–160. doi: 10.1016/j.geoderma.2015.09.005
Chambers L. G., Osborne T. Z., Reddy K. R. (2013). Effect of salinity-altering pulsing events on soil organic carbon loss along an intertidal wetland gradient: A laboratory experiment. Biogeochemistry 115, 363–383. doi: 10.1007/s10533-013-9841-5
Chanlabut U., Gomontean B., Srifa A. (2020). Soil organic carbon stocks across hydrologic schemes in freshwater wetlands of the chi river basin, northeast thailand. Wetlands 40, 377–389. doi: 10.1007/s13157-019-01181-8
Chen J., Xiao W., Zheng C., Zhu B. (2020). Nitrogen addition has contrasting effects on particulate and mineral-associated soil organic carbon in a subtropical forest. Soil Biol. Biochem. 142, 107708. doi: 10.1016/j.soilbio.2020.107708
Doetterl S., Berhe A. A., Nadeu E., Wang Z., Sommer M., Fiener P. (2016). Erosion, deposition and soil carbon: A review of process-level controls, experimental tools and models to address c cycling in dynamic landscapes. Earth-Sci. Rev. 154, 102–122. doi: 10.1016/j.earscirev.2015.12.005
Eusterhues K., Rumpel C., Kögel-Knabner I. (2005). Stabilization of soil organic matter isolated via oxidative degradation. Organic Geochem. 36, 1567–1575. doi: 10.1016/j.orggeochem.2005.06.010
Ferronato C., Falsone G., Natale M., Zannoni D., Buscaroli A., Vianello G., et al. (2016). Chemical and pedological features of subaqueous and hydromorphic soils along a hydrosequence within a coastal system (San vitale park, northern Italy). Geoderma 265, 141–151. doi: 10.1016/j.geoderma.2015.11.018
Ferronato C., Marinari S., Francioso O., Bello D., Trasar-Cepeda C., Antisari L. V. (2019). Effect of waterlogging on soil biochemical properties and organic matter quality in different salt marsh systems. Geoderma 338, 302–312. doi: 10.1016/j.geoderma.2018.12.019
Gartzia-Bengoetxea N., Virto I., Arias-González A., Enrique A., Fernández-Ugalde O., Barré P. (2020). Mineral control of organic carbon storage in acid temperate forest soils in the Basque country. Geoderma 358, 113998. doi: 10.1016/j.geoderma.2019.113998
Gulde S., Chung H., Amelung W., Chang C., Six J. (2008). Soil carbon saturation controls labile and stable carbon pool dynamics. Soil Sci. Soc. America J. 72, 605–612. doi: 10.2136/sssaj2007.0251
Kirwan M. L., Langley J. A., Guntenspergen G. R., Megonigal J. P. (2013). The impact of sea-level rise on organic matter decay rates in Chesapeake bay brackish tidal marshes. Biogeosciences 10, 1869–1876. doi: 10.5194/bg-10-1869-2013
Kögel Knabner I., Guggenberger G., Kleber M., Kandeler E., Kalbitz K., Scheu S., et al. (2008). Organo-mineral associations in temperate soils: Integrating biology, mineralogy, and organic matter chemistry. J. Plant Nutr. Soil Sci. 171, 61–82. doi: 10.1002/jpln.200700048
Kroeger K. D., Crooks S., Moseman-Valtierra S., Tang J. (2017). Restoring tides to reduce methane emissions in impounded wetlands: A new and potent blue carbon climate change intervention. Sci. Rep. 7, 11914. doi: 10.1038/s41598-017-12138-4
Li Y., Shahbaz M., Zhu Z., Deng Y., Tong Y., Chen L., et al. (2021). Oxygen availability determines key regulators in soil organic carbon mineralisation in paddy soils. Soil Biol. Biochem. 153, 108106. doi: 10.1016/j.soilbio.2020.108106
Lorenz K., Lal R., Jiménez J. J. (2009). Soil organic carbon stabilization in dry tropical forests of Costa Rica. Geoderma 152, 95–103. doi: 10.1016/j.geoderma.2009.05.025
Lützow M. V., Kögel-Knabner I., Ekschmitt K., Matzner E., Guggenberger G., Marschner B., et al. (2006). Stabilization of organic matter in temperate soils: Mechanisms and their relevance under different soil conditions - a review. Eur. J. Soil Sci. 57, 426–445. doi: 10.1111/j.1365-2389.2006.00809.x
Lu W., Xiao J., Liu F., Zhang Y., Liu C., Lin G. (2017). Contrasting ecosystemCO2 fluxes of inland and coastal wetlands: A meta-analysis of eddy covariance data. Global Change Biol. 23, 1180–1198. doi: 10.1111/gcb.13424
Lyu H., Watanabe T., Kilasara M., Hartono A., Funakawa S. (2021). Soil organic carbon pools controlled by climate and geochemistry in tropical volcanic regions. Sci. Total Environ. 761, 143277. doi: 10.1016/j.scitotenv.2020.143277
Macreadie P. I., Nielsen D. A., Kelleway J. J., Atwood T. B., Seymour J. R., Petrou K., et al. (2017). Can we manage coastal ecosystems to sequester more blue carbon? Front. Ecol. Environ. 15, 206–213. doi: 10.1002/fee.1484
Martí-Roura M., Hagedorn F., Rovira P., Romanyà J. (2019). Effect of land use and carbonates on organic matter stabilization and microbial communities in Mediterranean soils. Geoderma 351, 103–115. doi: 10.1016/j.geoderma.2019.05.021
Ma Z., Zhang M., Xiao R., Cui Y., Yu F. (2017). Changes in soil microbial biomass and community composition in coastal wetlands affected by restoration projects in a Chinese delta. Geoderma 289, 124–134. doi: 10.1016/j.geoderma.2016.11.037
Merino C., Fontaine S., Palma G., Matus F. (2017). Effect of aluminium on mineralization of water extractable organic matter and microbial respiration in southern temperate rainforest soils. Eur. J. Soil Biol. 82, 56–65. doi: 10.1016/j.ejsobi.2017.08.003
Neff J. C., Townsend A. R., Gleixner G., Lehman S. J., Turnbull J. (2002). Variable effects of nitrogen additions on the stability and turnover of soil carbon. Nature 419, 915–917. doi: 10.1038/nature01136
Pallud C., Rhoades C. C., Schneider L., Dwivedi P., Borch T. (2020). Temperature-induced iron (III) reduction results in decreased dissolved organic carbon export in subalpine wetland soils, Colorado, USA. Geochimica Et Cosmochimica Acta 280, 148–160. doi: 10.1016/j.gca.2020.03.023
Paul S., Veldkamp E., Flessa H. (2008). Differential response of mineral-associated organic matter in tropical soils formed in volcanic ashes and marine tertiary sediment to treatment with HCl, NaOCl, and Na4P2O7. Soil Biol. Biochem. 40, 1846–1855. doi: 10.1016/j.soilbio.2008.03.008
Petruzzelli L., Celi L., Ajmone-Marsan F. (2005). Effects of soil organic fractions on iron oxide biodissolution under anaerobic conditions. Soil Sci. 170, 102–109. doi: 10.1097/01.ss.0000155491.40452.94
Qu W., Han G., Wang J., Li J., Zhao M., He W., et al. (2021). Short-term effects of soil moisture on soil organic carbon decomposition in a coastal wetland of the yellow river delta. Hydrobiologia 848, 3259–3271. doi: 10.1007/s10750-020-04422-8
Rüegg J., Quezada J. C., Santonja M., Ghazoul J., Kuzyakov Y., Buttler A., et al. (2019). Drivers of soil carbon stabilization in oil palm plantations. Land Degradation Dev. 30, 1904–1915. doi: 10.1002/ldr.3380
Saleh J., Najafi N., Oustan S., Aliasgharzad N., Ghassemi-Golezani K. (2013). Changes in extractable si, fe, and mn as affected by silicon, salinity, and waterlogging in a sandy loam soil. Commun. Soil Sci. Plant Anal. 44, 1588–1598. doi: 10.1080/00103624.2013.768261
Sarkar B., Singh M., Mandal S., Churchman G. J., Bolan N. S. (2018). “Clay minerals–organic matter interactions in relation to carbon stabilization in soils” in: Garcia C., Nannipieri P., Hernandez T. (Eds.), The Future of Soil Carbon (Academic Press), 71–86. doi: 10.1016/B978-0-12-811687-6.00003-1
Sierra M., Martinez F. J., Braojos V., Romero-Freire A., Ortiz-Bernad I., Martín F. J., et al. (2016). Chemical stabilization of organic carbon in agricultural soils in a semi-arid region (SE Spain). J. Agric. Sci. 154, 87–97. doi: 10.1017/S002185961500012X
Six J., Conant R. T., Paul E. A., Paustian K. (2002). Stabilization mechanisms of soil organic matter: Implications for C-saturation of soils. Plant. Soil 241, 155–176. doi: 10.1023/A:1016125726789
Six J., Bossuyt H., Degryze S., Denef K. (2004). A history of research on the link between (micro)aggregates, soil biota, and soil organic matter dynamics. Soil Tillage Res. 79, 7–31. doi: 10.1016/j.still.2004.03.008
Soucémarianadin L. N., Cécillon L., Guenet B., Chenu C., Baudin F., Nicolas M., et al. (2018). Environmental factors controlling soil organic carbon stability in French forest soils. Plant Soil 426, 267–286. doi: 10.1007/s11104-018-3613-x
Tian Q., He H., Cheng W., Bai Z., Wang Y., Zhang X. (2016). Factors controlling soil organic carbon stability along a temperate forest altitudinal gradient. Sci. Rep. 6, 18783. doi: 10.1038/srep18783
Vos C., Don A., Hobley E., Prietz R., Heidkamp A., Freibauer A. (2017). Drivers for spatial variability in agricultural soil organic carbon stocks in Germany Geophysical Research Abstracts. 19, EGU 2017–4939. Abstract retrieved from Abstracts in EGU General Assembly 2017.
Wagai R., Mayer L. M. (2007). Sorptive stabilization of organic matter in soils by hydrous iron oxides. Geochim. Cosmochim. Ac. 71, 25–35. doi: 10.1016/j.gca.2006.08.047
Warren J., Ziegler S. E. (2017). A solid phase extraction method for isolating HF-extractable soil organic matter for NMR analysis. Organic Geochem. 111, 1–8. doi: 10.1016/j.orggeochem.2017.04.012
Wiesmeier M., Hübner R., Spörlein P., Geuß U., Hangen E., Reischl A., et al. (2014). Carbon sequestration potential of soils in southeast Germany derived from stable soil organic carbon saturation. Global Change Biol. 20, 653–665. doi: 10.1111/gcb.12384
Wiesmeier M., Urbanski L., Hobley E., Lang B., von Lützow M., Marin-Spiotta E., et al. (2019). Soil organic carbon storage as a key function of soils - a review of drivers and indicators at various scales. Geoderma 333, 149–162. doi: 10.1016/j.geoderma.2018.07.026
Wiseman C. L. S., Püttmann W. (2006). Interactions between mineral phases in the preservation of soil organic matter. Geoderma 134, 109–118. doi: 10.1016/j.geoderma.2005.09.001
Xue B., Huang L., Huang Y., Ali Kubar K., Li X., Lu J. (2020). Straw management influences the stabilization of organic carbon by fe (oxyhydr)oxides in soil aggregates. Geoderma 358, 113987. doi: 10.1016/j.geoderma.2019.113987
Yang J., Li A., Yang Y., Li G., Zhang F. (2020). Soil organic carbon stability under natural and anthropogenic-induced perturbations. Earth-Sci. Rev. 205, 103199. doi: 10.1016/j.earscirev.2020.103199
Yin S., Bai J., Wang W., Zhang G., Jia J., Cui B., et al. (2019). Effects of soil moisture on carbon mineralization in floodplain wetlands with different flooding frequencies. J. Hydrol. 574, 1074–1084. doi: 10.1016/j.jhydrol.2019.05.007
Zhang G., Bai J., Zhao Q., Jia J., Wang W., Wang X. (2020). Bacterial succession in salt marsh soils along a short-term invasion chronosequence of spartina alterniflora in the yellow river estuary, china. Microbial. Ecol. 79, 644–661. doi: 10.1007/s00248-019-01430-7
Zhao Q., Bai J., Lu Q., Zhang G. (2017). Effects of salinity on dynamics of soil carbon in degraded coastal wetlands: Implications on wetland restoration. Phys. Chem. Earth, Parts A/B/C 97, 12–18. doi: 10.1016/j.pce.2016.08.008
Zhao Q., Bai J., Wang X., Zhang W., Huang Y., Wang L., et al. (2020). Soil organic carbon content and stock in wetlands with different hydrologic conditions in the yellow river delta, China. Ecohydrol. Hydrobiol. 20, 537–547. doi: 10.1016/j.ecohyd.2019.10.008
Zhao Q., Callister S. J., Thompson A. M., Kukkadapu R. K., Tfaily M. M., Bramer L. M., et al. (2020). Strong mineralogic control of soil organic matter composition in response to nutrient addition across diverse grassland sites. Sci. Total Environ. 736, 137839. doi: 10.1016/j.scitotenv.2020.137839
Keywords: SOC stabilization, Al/Fe/Si oxides, mineral phase indicators, hydrologic conditions, coastal wetlands
Citation: Zhao H, Jia J, Zhao Q, Wang J, Gao Y, Huang Y and Chen G (2022) Soil organic carbon stabilization and associated mineral protection in typical coastal wetlands under different hydrologic conditions. Front. Mar. Sci. 9:1031561. doi: 10.3389/fmars.2022.1031561
Received: 30 August 2022; Accepted: 03 October 2022;
Published: 25 October 2022.
Edited by:
Tian Xie, Beijing Normal University, ChinaCopyright © 2022 Zhao, Jia, Zhao, Wang, Gao, Huang and Chen. This is an open-access article distributed under the terms of the Creative Commons Attribution License (CC BY). The use, distribution or reproduction in other forums is permitted, provided the original author(s) and the copyright owner(s) are credited and that the original publication in this journal is cited, in accordance with accepted academic practice. No use, distribution or reproduction is permitted which does not comply with these terms.
*Correspondence: Qingqing Zhao, cWluZ3Fpbmd6aGFvQHFsdS5lZHUuY24=; Yongchao Gao, Z2FveWNAc2Rhcy5vcmc=
†The authors have contributed equally to this work