- Xiamen Key Laboratory for Feed Quality Testing and Safety Evaluation, Fisheries College of Jimei University, Xiamen, China
An 8-week feeding trial was conducted to investigate whether dietary sodium butyrate (SB) administration alleviates growth reduction and enteritis of orange-spotted grouper (Epinephelus coioides) caused by high soybean meal (SBM) feeding. The control diet (FM diet) was formulated to contain 48% protein and 11% fat. Soybean meal was used to replace 60% FM protein in FM diet to prepare a high SBM diet (HSBM diet). Sodium butyrate (SB) at 0.1%, 0.2%, and 0.3% were added to HSBM diets to prepare three diets. Triplicate groups of 30 groupers (initial weight: 33.0 ± 0.3 g) were fed one of the diets twice daily, to apparent satiety. HSBM diets had lowered growth rate and feed efficiency vs FM diets (P <0.05). Growth rate and feed efficiency were improved by dietary SB administration and were in a dose-dependent manner (P <0.05). A similar pattern to the growth rate was observed for plasma LDL-C and gut digestive activity of lipase, trypsin, and protease, but the opposite trend was observed for intestinal contents of D-lactic acid and endotoxin, in response to dietary SB inclusion levels (P >0.05). The muscular thickness in the middle and distal intestines in SB-treated diets were higher than that in HSBM diets (P <0.05). The mRNA levels of intestinal pro-inflammatory cytokines IL-8, IL-1β, IL-12 and TNF-α had a decreasing trend, and the mRNA level of intestinal anti-inflammatory cytokine TGF-β1 had the opposite trend, with increasing SB inclusion levels (P < 0.05). The above results indicate that dietary SB intervention could enhance growth and feed utilization of groupers with SBM-induced enteritis by promoting intestinal digestive enzyme activities, reducing mucosa permeability, maintaining the integrity of intestinal morphology and attenuating the intestinal inflammatory response.
Introduction
Farmed marine fish generally require a high-quality protein feed, and fish meal (FM) is the major protein source that can fully meet the demand for the high-quality protein due to its high protein level, balanced amino acids profile, and less anti-nutritional factors in comparison with terrestrial animal and plant proteins (Zhao et al., 2021a; Mahamud et al., 2022). This determines the importance of FM as a protein source in marine aquaculture. However, over the past few decades, there has been a huge contrast between the rapid expansion of global aquaculture and the declining production of marine fishery catches (FAO, 2020). This causes a great deficit between FM supply and demand, making it a general consensus in the industry to find alternative protein sources of FM (Hardy, 2010). Soybean meal (SBM) is widely regarded as the most potential plant protein source to replace FM in marine fish feeds due to its relatively balanced amino acids profile, stable supply and low price. However, the majority of fish, especially carnivorous fish, show an obvious intolerance to SBM when given a diet containing a high percentage of SBM (Baeverfjord and Krogdahl, 1996; Zhu et al., 2021). Some anti-nutritional factors in SBM are the direct factors inducing enteritis, the so-called SBM induced enteritis (SBMIE) (Carmona, 2008), which leads to poor growth and other side effects, such as damage to the intestinal mucosal barrier, disturbance of the intestinal flora, an increased presence of inflammatory cells (Gatlin et al., 2007; Booman et al., 2018; Wang et al., 2020; Zhang et al., 2022). Therefore, how to prevent and control the widespread SBMIE is the key to maintaining normal daily fish culture and reducing disease risk. One of the effective ways to mitigate fish intestinal damage and enteritis caused by SBM-based feeds is the dietary use of functional feed additives through the nutrition regulation strategy (Peng et al., 2013; Ferrara et al., 2015; Rimoldi et al., 2016; Zhao et al., 2019).
Butyric acid is a metabolite of intestinal bacterium Clostridium butyricum, and as an important energy substance, provides energy for intestinal epithelial cell metabolism (Topping and Clifton, 2001; Hamer et al., 2007; Robles et al., 2013), promotes cellular function, and maintains intestinal health (Biagi et al., 2007). Butyric acid is a volatile fatty acid, and its stable form is sodium butyrate (SB). In animal feeding practice, SB, as a substitute for butyric acid, is often used as a functional additive in livestock and poultry feeds as well as aquafeeds (Bedford and Gong, 2018; Lin et al., 2020). The positive effects of dietary SB supplementation on growth performance have been achieved in fish such as turbot (Scophthalmus maximus L.) (Liu et al., 2019; Yu et al., 2021), yellow drum (Nibea albiflora) (Wu et al., 2020) and Pengze crucian carp (Carassius auratus Pengze) (Fang et al., 2021). The above study results showed that SB supplementation in high-SBM diets could alleviate SBMIE of fish by promoting digestion and absorption capacity and maintaining the integrity of intestinal morphology and structure, as well as intestinal microbial homeostasis (Liu et al., 2019). In addition, dietary SB was found to exert a regulatory role in the expression of related genes, such as intestinal inflammatory factor genes and growth-related factor genes (Wu et al., 2020; Abdel-Tawwab et al., 2021; Yu et al., 2021).
Grouper is a carnivorous marine fish that has been widely cultivated in Southeast Asia and China due to its fast growth and high quality of fish flesh (Dennis et al., 2020; Qin et al., 2022). The aquaculture output of grouper in China reached 192,045 tons in 2020 (China Fishery Statistics Yearbook, 2021). Great progress regarding its nutrition and feed research and development has been achieved (Ko et al., 2020; Bai et al., 2021; Kuo et al., 2021; Yang et al., 2021). However, there is still a lack of nutritional regulation research on the prevention of SBMIE in fish species. Our latest study also showed that a high SBM diet caused inflammatory reactions and reduced the growth performance of orange-spotted grouper (Epinephelus coioides) (Wang et al., 2017a; Zhao et al., 2021b). So far, the intervention effect of dietary SB on grouper SBMIE has not been reported yet. Therefore, the present study aimed to investigate the effects of SB supplementation (0.1%, 0.2%, and 0.3%) in high SBM diets on the growth performance, plasma components, and intestinal health of orange-spotted grouper. This study provided the basis for the prevention and control of SBMIE in the fish species.
Materials and methods
Experimental diets and rearing management
The ingredients and proximate composition of experimental diets are presented in Table 1. A basal diet (FM diet) was formulated to contain 48% crude protein and 11% crude lipid using fish meal (FM), gelatin and casein as the main protein sources and soybean and fish oil, and soy lecithin as the lipid sources. Soybean meal (SBM) was used to replace 60% FM protein in the FM diets to prepare a high SBM diet (HSBM diet). Sodium butyrate (SB) were added to the HSBM diet at 0.1%, 0.2%, and 0.3% to prepare three experimental diets (SB0.1 diet, SB0.2 diet, SB0.3 diet, respectively). Coarse dry feed ingredients were ground from a hammer mill (GH-20B, Jiangyin Kejia Machinery Manufacturing Co., Ltd., Jiangyin, Jiangsu, China), sieved through a 60-mesh sieve, then weighed and homogenized. Liquid ingredients (fish and soybean oil, soy lecithin and freshwater) were then incorporated into the dry feed ingredients and a mash was prepared. The dough was extruded into strands and made into 2.5 mm and 4 mm pellets through dies using the cold press extrusion method (CD4XITS, South China University of Technology, Guangzhou, Guangdong, China). The pellets were dried in a ventilated oven at 55°C for 24 h until the moisture was reduced to 10%, and then placed at room temperature for 24 h, before being sealed in plastic bags and stored at -20°C.
The juvenile groupers obtained from a commercial fish farm were transported to Dabeinong experimental station (Zhangzhou City, Fujian Province, China). Fish were stocked in a concrete pond and fed a commercial feed for 3-week acclimatization. At the beginning of the experiment, the fish (initial mean weight of 33.0 ± 0.3 g) were allocated to five groups each with triplicate tanks (500 L/tank), at a stocking density of 30 fish/tank in a water temperature-controlled recirculating culture system. Fish were fed one of the experimental diets twice daily (8:00, 17:00) to apparent satiety each meal under a natural photoperiod across a feeding period of 56-day. Excess feed was collected by siphoning 30 min after each meal, then dried at 65°C, and weighed to calculate feed intake (FI). Because daily sewage discharge will cause water loss in the aquaculture system, fresh seawater was refilled until the original water level of tanks was reached. During the feeding period, water temperature was kept at 28.5°C, the dissolved oxygen level was > 5.7 to 8.0 mg/L, and the ammonia nitrogen content was < 0.22 mg/L.
Sample collection
At the end of the growth trial, fish in each tank were caught and anesthetized with a dose of 100 mg/L solutions of MS-222 (tricaine methane sulphonate, Sigma-Aldrich Shanghai Trading Co., Ltd, Shanghai, China). Fish weight and number were then recorded for each tank to measure weight gain (WG), feed efficiency (FE), specific growth rate (SGR), and survival. Three fish from each tank were randomly sampled and pooled in plastic bags, and stored at -20°C for whole-body proximate composition determination. Nine fish per tank (27 fish each group) were weighed individually after an aesthesia with MS-222 (100 mg/L) to calculate the hepatosomatic index (HSI) and condition factor (CF). Blood was drawn from the caudal vein, using a 1-mL heparinized syringe, and centrifuged at 1027 × g, 4°C, 10 min. Plasma was then collected, pooled by tank and stored in 1.5-mL Eppendorf tubes at -80°C for the subsequent biochemical analysis. The intestine of nine fish per tank were aseptically removed and pooled into one tube by tank, stored at -80°C for the analysis of biochemical components, microbiota analysis, and gene expression.
Proximate composition analysis
Prior to component analysis, whole-fish samples were prepared according to the method described by Ye et al. (Ye et al., 2011). The proximate composition of diet and whole-body fish samples were determined according to standard methods (AOAC, 1995). Dry matter was determined by drying the samples in an oven at 105°C to a constant weight. Crude protein was determined by the Kjeldahl method (N × 6.25) using Kjeltec TM 8400 Auto Sample Systems (Foss Teacher AB). The crude lipid content was determined by the Soxtec extraction method by using Soxtec Avanti 2050 (Foss Teacher AB). Ash was measured in the residues of samples burned in a muffle furnace at 550°C for 6 h.
Plasma component determination
The plasma contents of triglycerides (TG), total cholesterol (TC), high-density lipoprotein cholesterol (HDL-C), and low-density lipoprotein cholesterol (LDL-C) were determined using respective kits (Nanjing Jiancheng Bioengineering Institute, Nanjing, China).
Gut digestive enzyme activity and mucosal function analysis
The intestinal activity for lipase and amylase was assayed using commercial assay kits (Nanjing Jiancheng Bioengineering Institute, Nanjing, Jiangsu, China) according to the protocols of the manufacturer. The activities of intestinal protease and trypsin were determined according to the method described by Hu et al. (2014). The contents of diamine oxidase (DAO), D-lactic acid (D-Lac), Endotoxin (ET) and Endothelin-1 (ET-1) in the intestine were determined using ELISA kits (Nanjing Jiancheng Bioengineering Institute, Nanjing, Jiangsu, China) according to the manufacturer’s instructions.
Intestinal histological observation
To perform histological analysis, one fish was caught from each tank and dissected to obtain the whole gut, then divided into proximal, middle, and distal intestines (i. e. PI, MI, and DI, respectively), according to the method (Anguiano et al., 2013). All the segments were washed with normal saline, fixed in Bouin’s solution for 24 h, rinsed with 70% (v/v) ethanol solution, and finally immersed in 70% (v/v) ethanol until histological processing was performed (Niu et al., 2021). The fixed gut segments were embedded in paraffin and 5-µm sections were cut by using a rotary microtome (KD-2258S, China). The serial histological sections were then mounted on glass slides, and stained with hematoxylin and eosin for morphometric analysis. Pictures were examined under a light microscope (Leica DM5500B, Germany), and digital images were taken and processed with a digital camera (Leica DFC450) equipped with the image program LAS AF (Version 4.3.0 Leica). Five slides were prepared for each gut segment sample and 30 measurements were made to determine the number of mucosal folds, muscle layer thickness, and length of the complete mucosal fold.
Intestinal microbiota analysis
Total DNA of the distal intestine (DI) of the fish was extracted using a DNA extraction kit (Omega Bio-teK, Norcross, GA, USA) according to the manufacturer’s instructions. The integrity and quality, purity and quantity of DNA samples were assessed by electrophoresis on a 1% (w/v) agarose gel and spectrophotometer method (NanoDrop 2000, Wilmington, DE, U.S. 260nm/280nm optical density ratio), respectively. The V3-V4 region of the 16S rDNA gene of DI bacteria was amplified by polymerase chain reaction (PCR) using the forward primer 338F (5’-ACTCCTACGGGAGGCAGCAG-3’) and the reverse primer 806R (5’-GGACTACNNGGGTATCTAAT-3’). The PCR reaction system included pre-denaturation at 95°C for 5 min; denaturation at 95°C for 45 s, annealing at 55°C for 50 s, and extension at 72°C for 45 s, 32 cycles; extension at 72°C for 10 min. Subsequently, high-throughput sequencing was performed using Illumina Miseq PE300 at Beijing Allwegene Technology Co., Ltd (Beijing, China). The sequencing data of all samples were deposited into Sequence Read Archive (SRA) (Accession number: PRJNA875282). A library of small fragments was constructed using a paired-end for sequencing, and the data was passed through QIIME (v1.8.0) for removal of low-quality sequences and chimeras. Based on 97% sequence similarity, similar sequences were assigned to the same operational taxonomic units (OTU). Species classification information corresponding to each OTU was obtained by comparing with the sliva database, and alpha diversity analysis (Shannon, Ace, and Chao1) was performed using Mothur software (version 1.31.2). Based on the weighted unifrac distance, the pheatmap of the R (v3.1.1) software package was used for clustering analysis. After the UniFrac algorithm, the information on system evolution was used to compare the difference in species communities among samples and beta diversity analysis was performed.
RNA extraction and gene expression analysis
The total RNA was extracted from the intestinal samples using TRIzol® reagent (Takara Co., Ltd, Japan) according to the manufacturer’s instructions. Isolated RNA was quantified using the NanoDrop ND-2000 Spectrophotometer, and its integrity was confirmed by agarose gel electrophoresis. The cDNA was generated from 1 μg DNase-treated RNA and synthesized by a PrimeScript™ RT Reagent Kit with gDNA Eraser (Perfect Real Time) (Takara Co., Ltd, Japan). Real-time PCR was employed to determine mRNA levels based on the TB Green™ Premix Ex Taq™ II (Tli RNaseH Plus) (Takara Co., Ltd, Japan) using a QuantStudio™ Real-Time PCR System (ABI) quantitative thermal cycler. The fluorescent quantitative PCR solution consisted of 10 μL TB Green Premix Ex Taq™ II (Tli RNaseH Plus) (2×), 0.8 μL PCR forward primer (10 μM), 0.8 μL PCR reverse primer (10 μM), 2.0 μL RT reaction (cDNA solution), and 6 μL dH2O. The thermal program included 30 s at 95°C, 40 cycles at 95°C for 5 s, and 60°C for 30 s. The sequences of primers are shown in Table 2. All amplicons were initially separated by agarose gel electrophoresis to ensure that they were of the correct size. β-actin served as the internal reference gene to normalize cDNA loading. The gene expression levels of the target genes were analyzed by the 2-ΔΔCt method (Schmittgen and Livak, 2008) after verifying that the primers were amplified with an efficiency of approximately 100% (Hanaki et al., 2014), and the data for all treatment groups were compared with the data for the control group.
Statistical analysis
All data were presented as mean and standard error of the mean (SEM). The data were analyzed using a one-way analysis of variance (ANOVA) to test for differences between treatments and then the Student-Neuman-Keuls multiple comparison test was performed after confirming the normality and homogeneity of variance using the Kolmogorov-Smirnov test and Levene’s test in SPSS Statistics 25.0 (SPSS, Michigan Avenue, Chicago, IL, USA). The data expressed as percentages or ratios were subjected to data conversion prior to statistical analysis. P-values <.05 was deemed as significant difference.
Results
Growth performance and proximate composition
The results of growth performance and proximate composition of groupers are presented in Table 3. The fish fed HSBM diet had significantly lower WG and SGR compared with those fed FM diet (P < 0.05). The WG and SGR in fish fed diets SB0.1-SB0.3 were higher than that in fish fed diet HSBM, and reached the level of diet FM (P > 0.05). The WG and FE were in a dose-dependent manner with the dietary SB inclusion levels (Figure 1). The maximum values for WG and FE were observed for diet SB0.2 and diet SB0.3 respectively. The FE, HSI, CF and whole-body proximate composition were not affected by dietary treatments (P > 0.05).
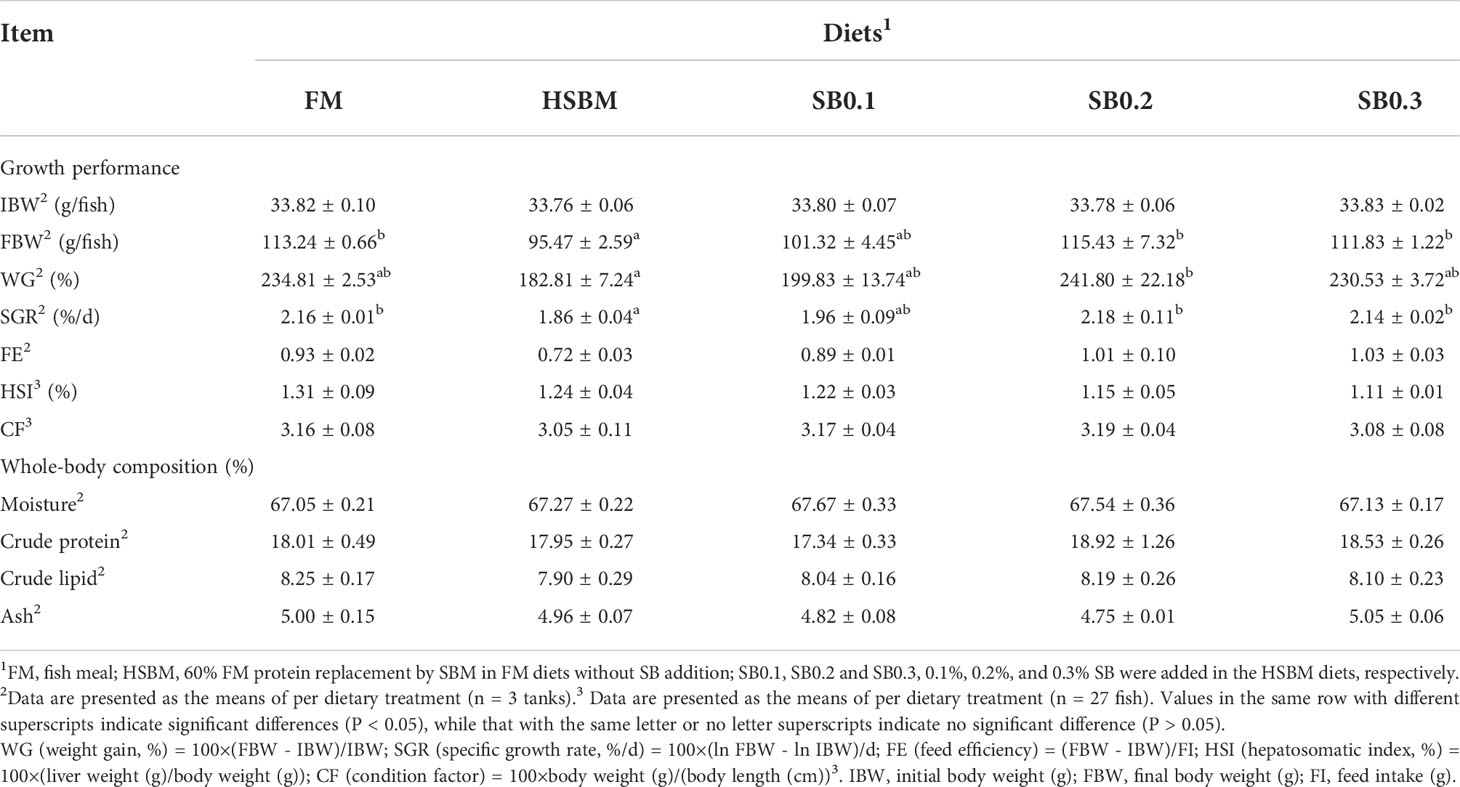
Table 3 Effects of sodium butyrate (SB) addition in high soybean meal (SBM) diets on growth performance and proximate composition of groupers in a 56-d feeding period.
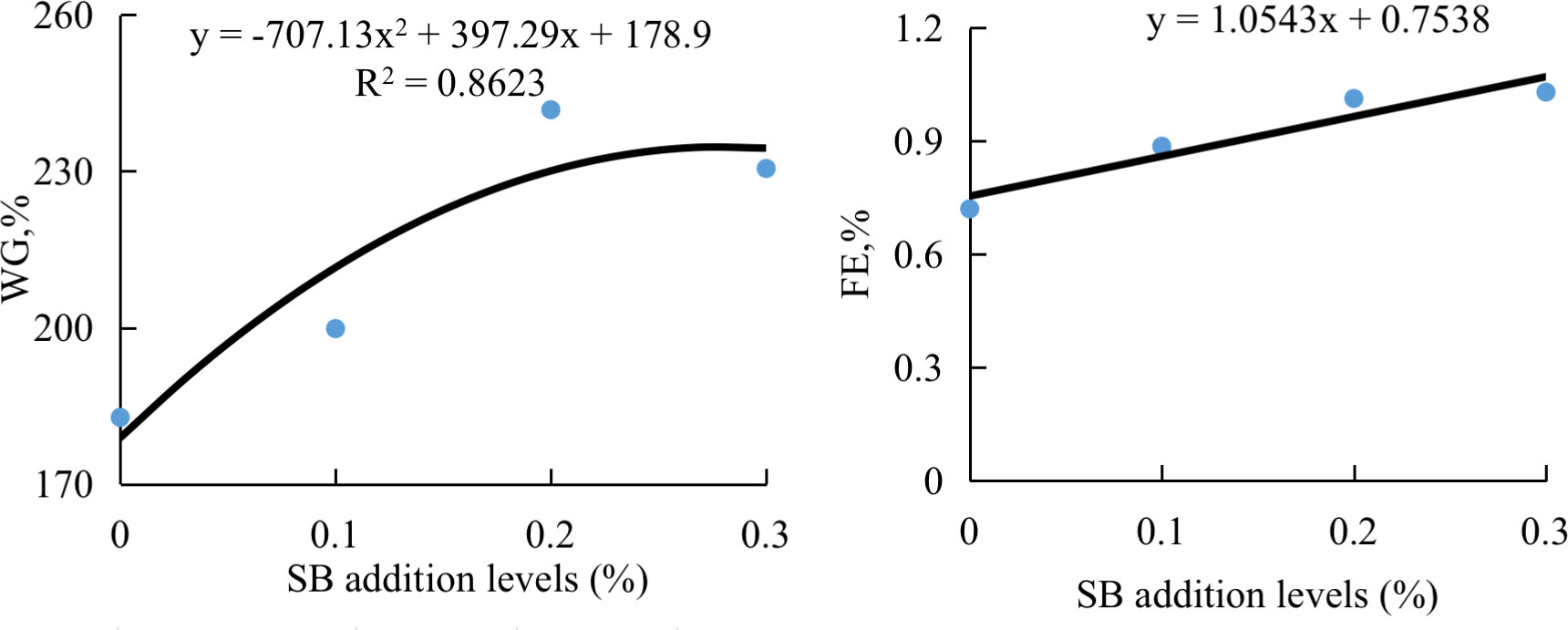
Figure 1 The relationship between weight gain (WG) or feed efficiency (FE) of groupers and sodium butyrate (SB) inclusion levels in HSBM diets in a 56-d feeding period. Data are presented as the means of per dietary treatment (n = 3 tanks). HSBM, 60% FM protein replacement with soybean meal (SBM) in FM diet and without sodium butyrate (SB) addition; SB0.1, SB0.2 and SB0.3, 0.1%, 0.2%, and 0.3% SB were added in HSBM diets,respectively.
Plasma components
As shown in Table 4, plasma LDL-C concentration in HSBM group was significantly lower (P < 0.05). However, plasma LDL-C concentration in dietary SB-treated groups was not different from those of SB0.1 group and SB0.2 groups (P > 0.05), and lower than that in SB0.3 group (P < 0.05). The plasma HDL-C, TC and TG concentrations were not affected by dietary treatments (P>0.05).
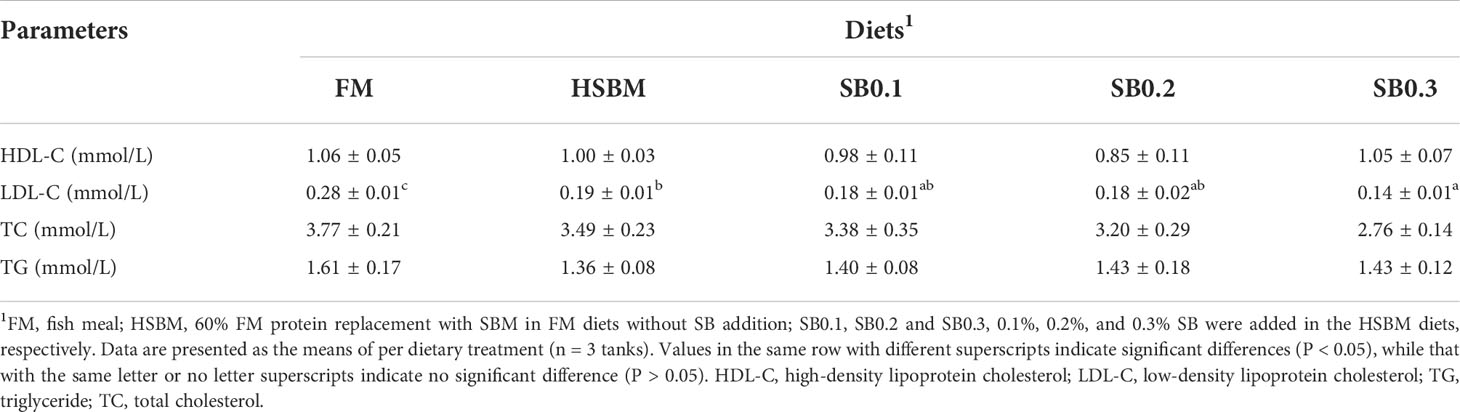
Table 4 Effect of sodium butyrate (SB) addition in high soybean meal (SBM) diets on plasma components of groupers in a 56-d feed period.
Gut digestive enzymes activity
There was a general reduction in lipase, trypsin, and protease activity for HSBM diet vs for FM diet (Table 5). A general enhancement in lipase, trypsin, and protease activity by dietary SB addition, and showed positive quadratic (P < 0.05) responses to increasing dietary SB inclusion levels, and the maximum value both were observed for diet SB0.1 (Figure 2). Dietary treatment did not affect intestinal amylase activity (P >0.05).
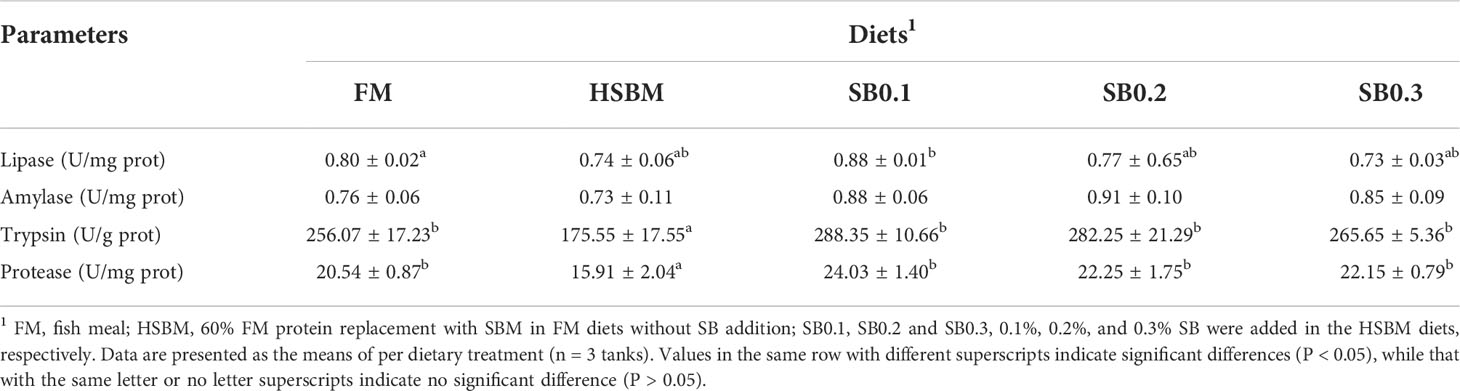
Table 5 Effects of sodium butyrate (SB) addition in high soybean meal (SBM) diets on activities of gut digestive enzymes of groupers in a 56-d feed period.
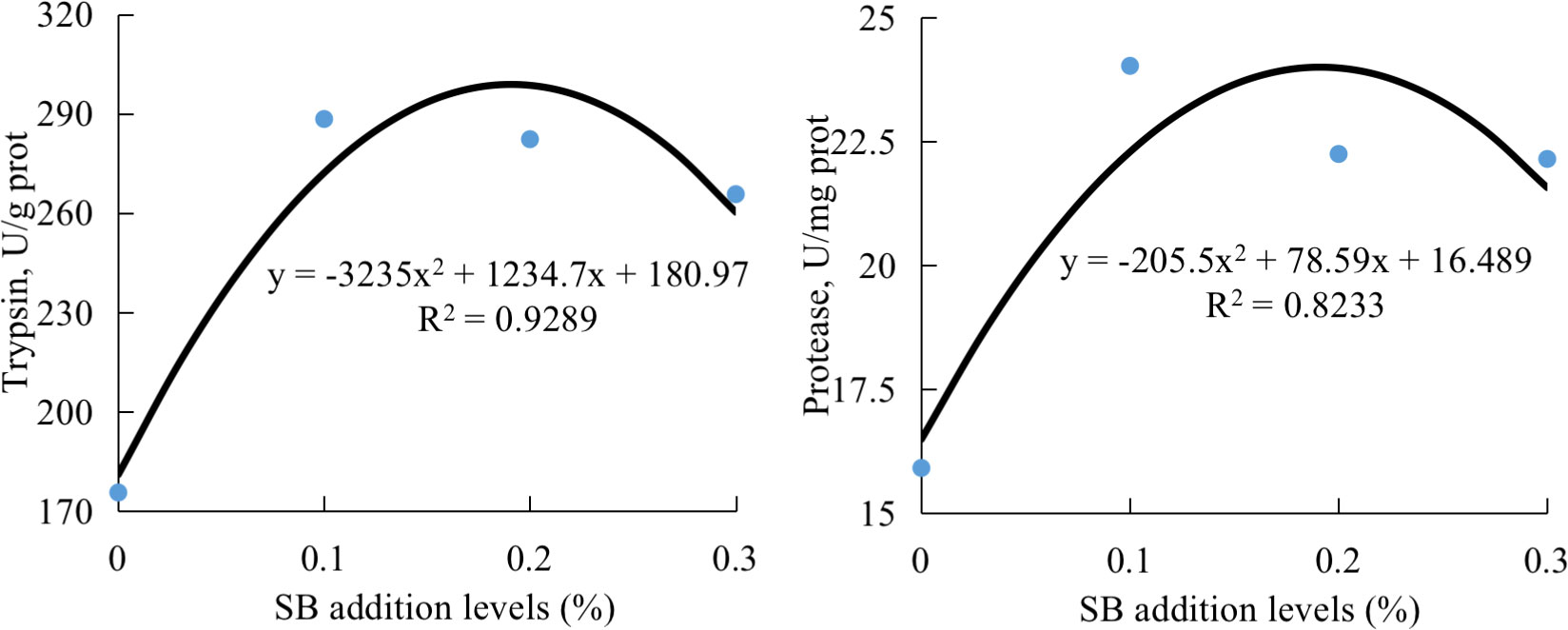
Figure 2 The relationship between intestinal activities of trypsin or protease of groupers and sodium butyrate (SB) inclusion levels in HSBM diets in a 56-d feeding period. Data are presented as the means of per dietary treatment. (n = 3 tanks). HSBM, 60% FM protein replacement with soybean meal (SBM) in FM diet and without SB addition; SB0.1, SB0.2 and SB0.3, 0.1%, 0.2%, and 0.3% SB were added in HSBM diets, respectively.
Intestinal permeability
As shown in Table 6, intestinal D-Lac and ET concentrations were higher in HSBM group than that in FM group (P < 0.05). However, dietary SB addition lowered intestinal DAO activity, D-Lac and ET concentrations, and intestinal D-Lac concentration showed a declining trend with dietary SB inclusion levels. Furthermore, intestinal D-Lac and ET concentrations were reduced to the level of FM group (P >0.05). The intestinal ET-1 content was not affected by dietary treatments (P >0.05).
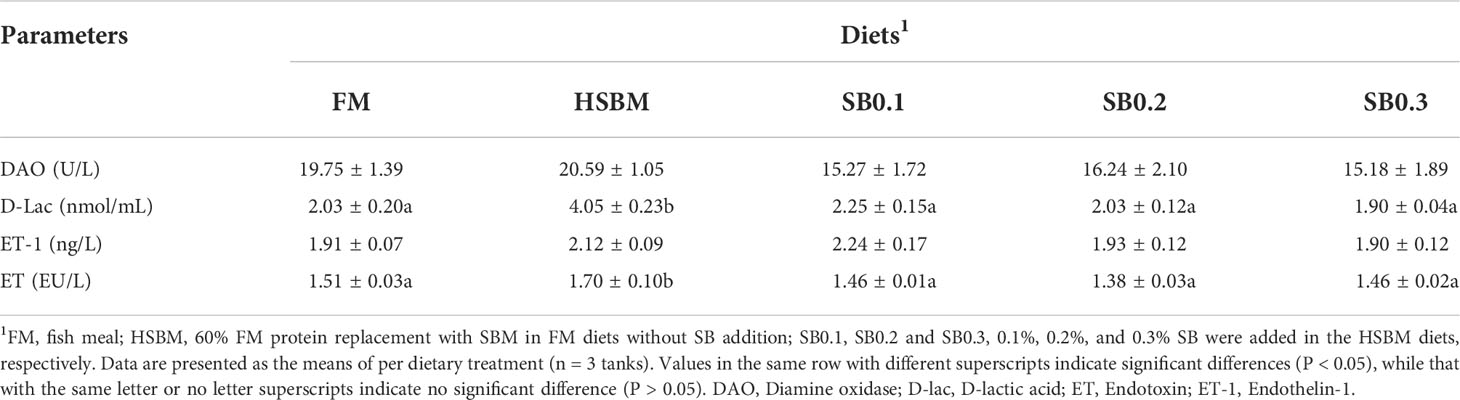
Table 6 Effects of sodium butyrate (SB) addition in high soybean meal (SBM) diets on intestinal permeability of groupers in a 56-d feeding period.
Intestinal morphology
As shown in Figure 3, the sizes of intestinal lumen and MFN were ordered as PI >MI >DI. The thickness of lamina propria and submucosa of MI in the HSBM group was increased compared to others. There was a reduction in the number of goblet cells with lymphocyte infiltration of DI for HSBM diet vs for FM diet, but an increase with increasing SB inclusion levels.
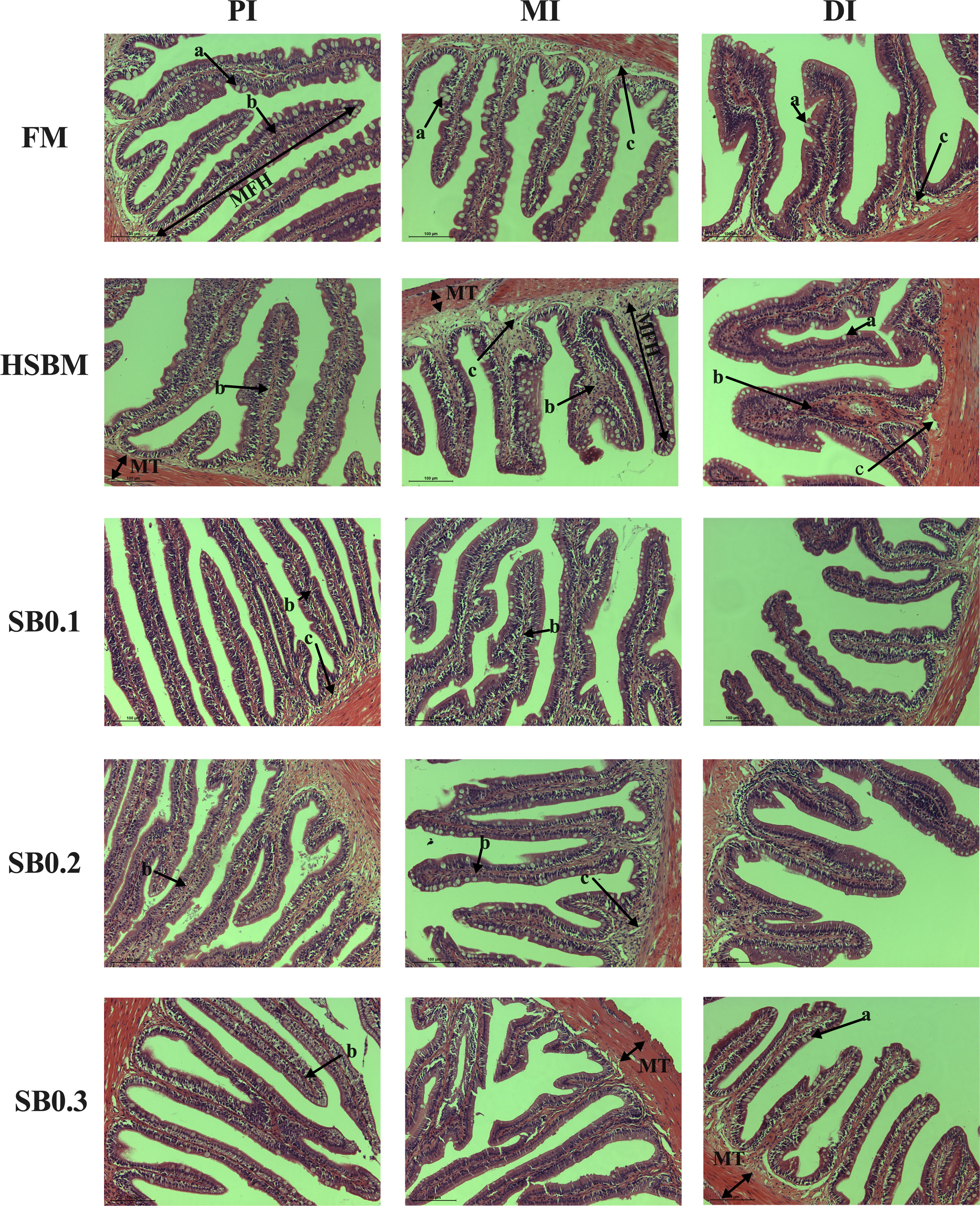
Figure 3 Intestinal histological examinations of sodium butyrate (SB) addition in high soybean meal (SBM) diets on the proximal intestine (PI), mid intestine (MI), and distal intestine (DI) in groupers in a 56-d feeding period (magnification 200 X, scale bar = 100 mm). FM, fish meal; HSBM, 60% FM protein replacement with SBM in FM diets without SB addition; SB0.1, SB0.2 and SB0.3, 0.1%, 0.2%, and 0.3% SB were added in the HSBM diets, respectively. MFH, mucosal fold height; MT, muscular thickness; a, goblet cell; b, lamina propria; c, submucosa.
Table 7 shows the results of intestinal morphometrical parameters (MFH, mucosal fold height; MT, muscular thickness; MFN, mucosal fold number) in three intestinal segments (PI, MI, and DI) of groupers. There was a significant reduction (P < 0.05) in MT of MI and DI in fish fed the HSBM diet compared with those fed with FM diet. The MT of MI was increased with increasing SB inclusion levels in HSBM diets, the maximum value was observed for SB0.3 diet (P < 0.05), and MT value for SB0.2 diet returned to that of FM diet (P > 0.05). The MT of DI was also promoted by dietary SB inclusion levels, but the values in SB diets were lower than that in the FM diet (P < 0.05). The MFH of PI, MI, and DI, the MT of PI, as well as MFN of PI, MI, and DI remained unaffected across dietary treatments (P > 0.05).
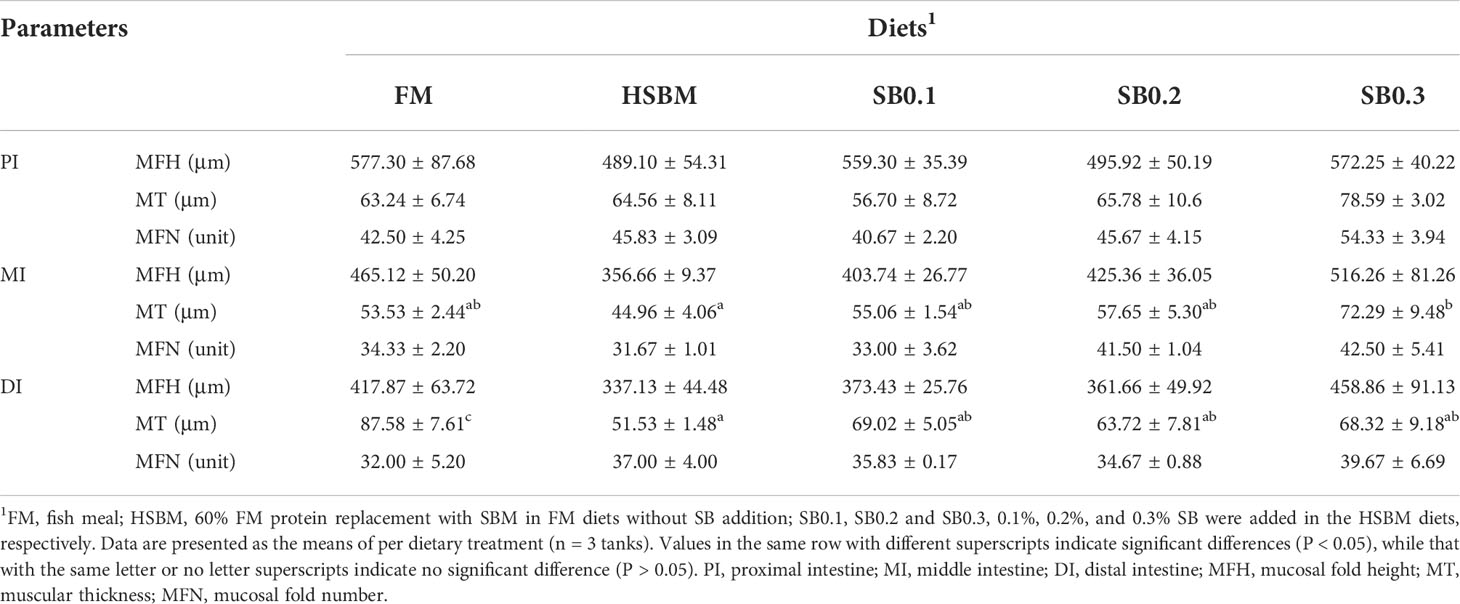
Table 7 Effects of sodium butyrate (SB) addition in high soybean meal (SBM) diets on the intestinal morphology of groupers in a 56-d feeding period.
Intestinal microbial abundance
The species abundance and diversity of DI samples are shown in Table 8. Although OTU and Ace showed a declining trend and Simpson and Shannon showed an upward trend with increasing dietary SB levels, the species abundance and diversity remained unaffected across dietary treatments (P > 0.05). At the phylum level, the average bacteria in the DI flora map of grouper were dominated by Firmicutes (relative abundance of 43.13%), Bacteroidetes (26.82%) and Proteobacteria (22.53%). Spirochaetae (1.53%) and Fusobacteria (1.08%) were subdominants (Figure 4A). However, these phyla bacterial abundance among all groups did not show significant differences (Figure 4B, P > 0.05). At the genus level, the bacteria in the DI flora map of grouper mainly included Photobacterium (FM: 18.9%; HSBM: 11.25%; SB0.1: 5.43%; SB0.2: 25.47%; SB0.3: 0.42%), Selenomonas_1 (FM: 13.70%; HSBM: 13.04%; SB0.1: 11.76%; SB0.2: 7.90%; SB0.3: 12.64%), Prevotella_1 (FM: 8.34%; HSBM: 7.88%; SB0.1: 7.61%; SB0.2: 6.08%; SB0.3: 9.16%), Rikenellaceae_RC9_gut_group (FM: 3.24%; HSBM: 3.81%; SB0.1: 4.88%; SB0.2: 5.75%; SB0.3: 6.37%) and Curvibacter (FM: 2.97%; HSBM: 2.81%; SB0.1: 2.76%; SB0.2: 1.50%; SB0.3: 2.08%) (Figure 5A) and marked difference in the abundance of these genus bacteria between groups was not observed (Figure 5B, P > 0.05). There was no variation in the relative abundance of Anaerovibrio among dietary treatments except for the SB0.3 diet, whose value was significantly higher than in FM and SB0.2 diets (P < 0.05). The relative abundance of Vibrio in SB diets was generally lower (P > 0.05) than that in HSBM diet and was similar to that of FM diet.
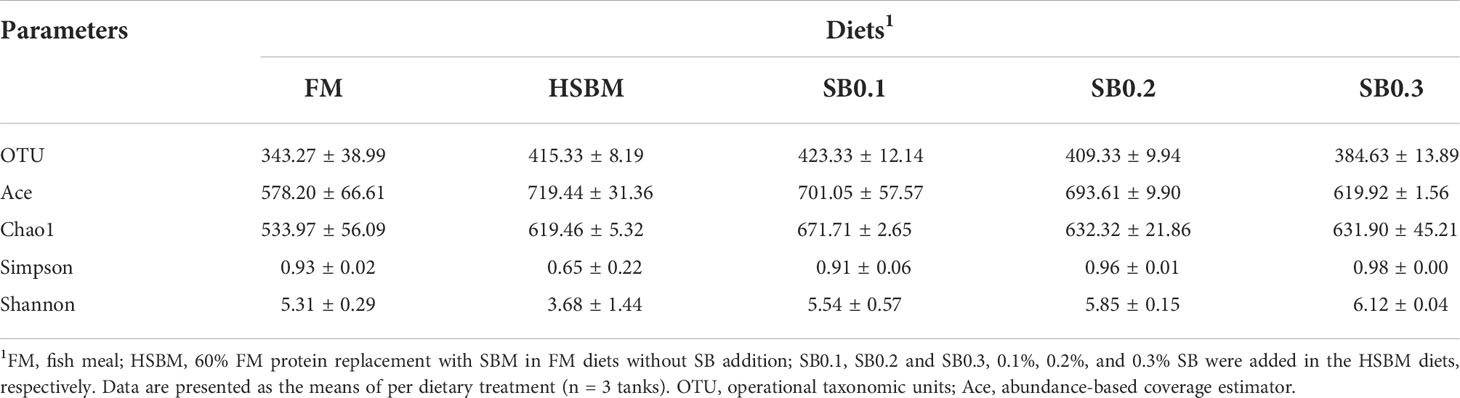
Table 8 Richness and diversity index of bacterial community for different samples in distal intestine of groupers.
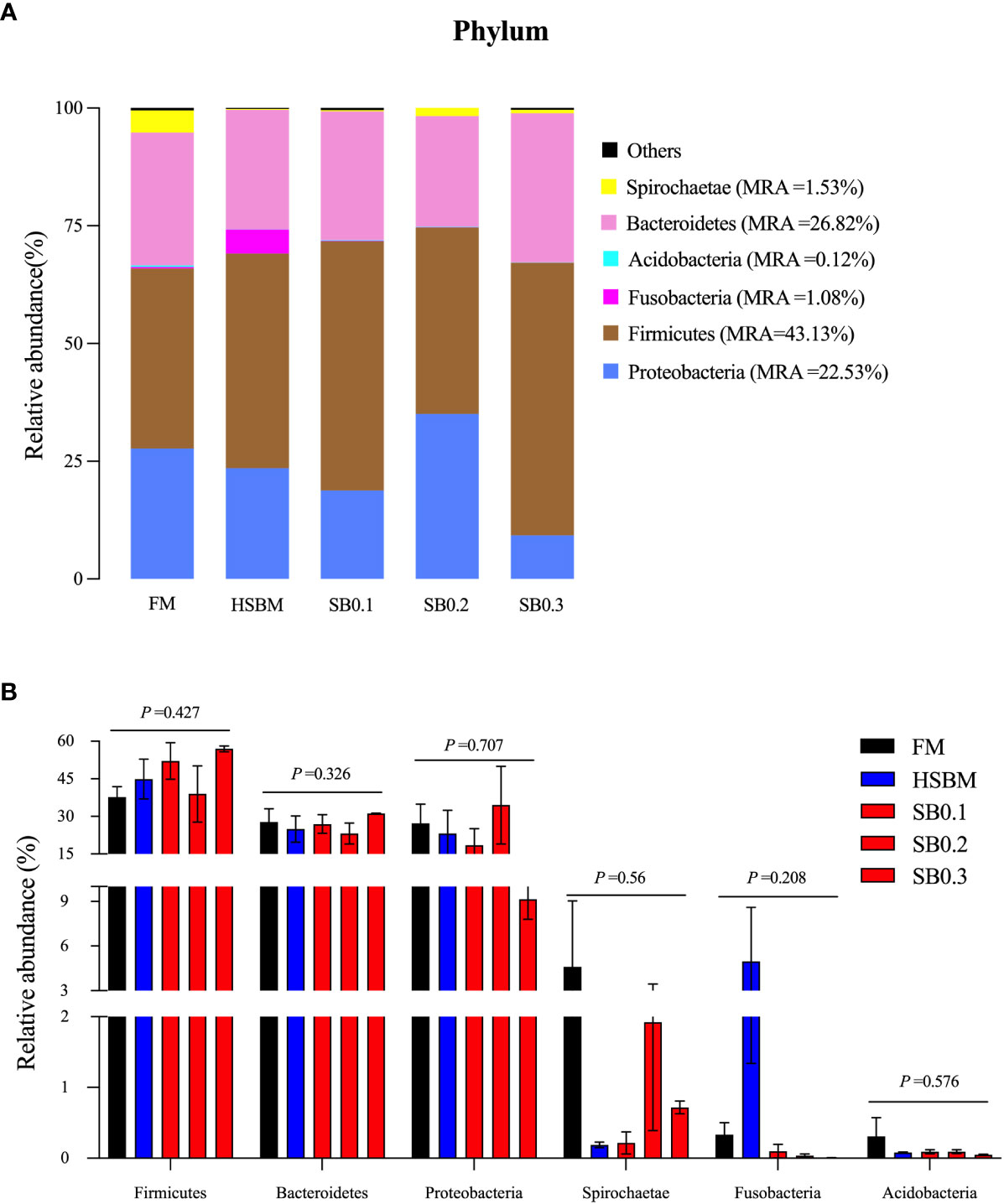
Figure 4 Relative abundance of the dominant bacterial phylum in different samples in the distal intestine of E. coioides (A) Intestine microbial composition at phylum level, (B) Differential analysis at phylum level. Bars bearing the different letters indicate significant differences (P < 0.05), while that with the same letters indicate no significant difference (P > 0.05). Data are presented as means ± SEM (n = 3 tanks). Statistical analysis was performed by one-way ANOVA, followed by S-N-K test. FM, fish meal; HSBM, 60% FM protein replacement with SBM in FM diets without SB addition; SB0.1, SB0.2 and SB0.3, 0.1%, 0.2%, and 0.3% SB were added in the HSBM diets, respectively. MRA, mean relative abundance.
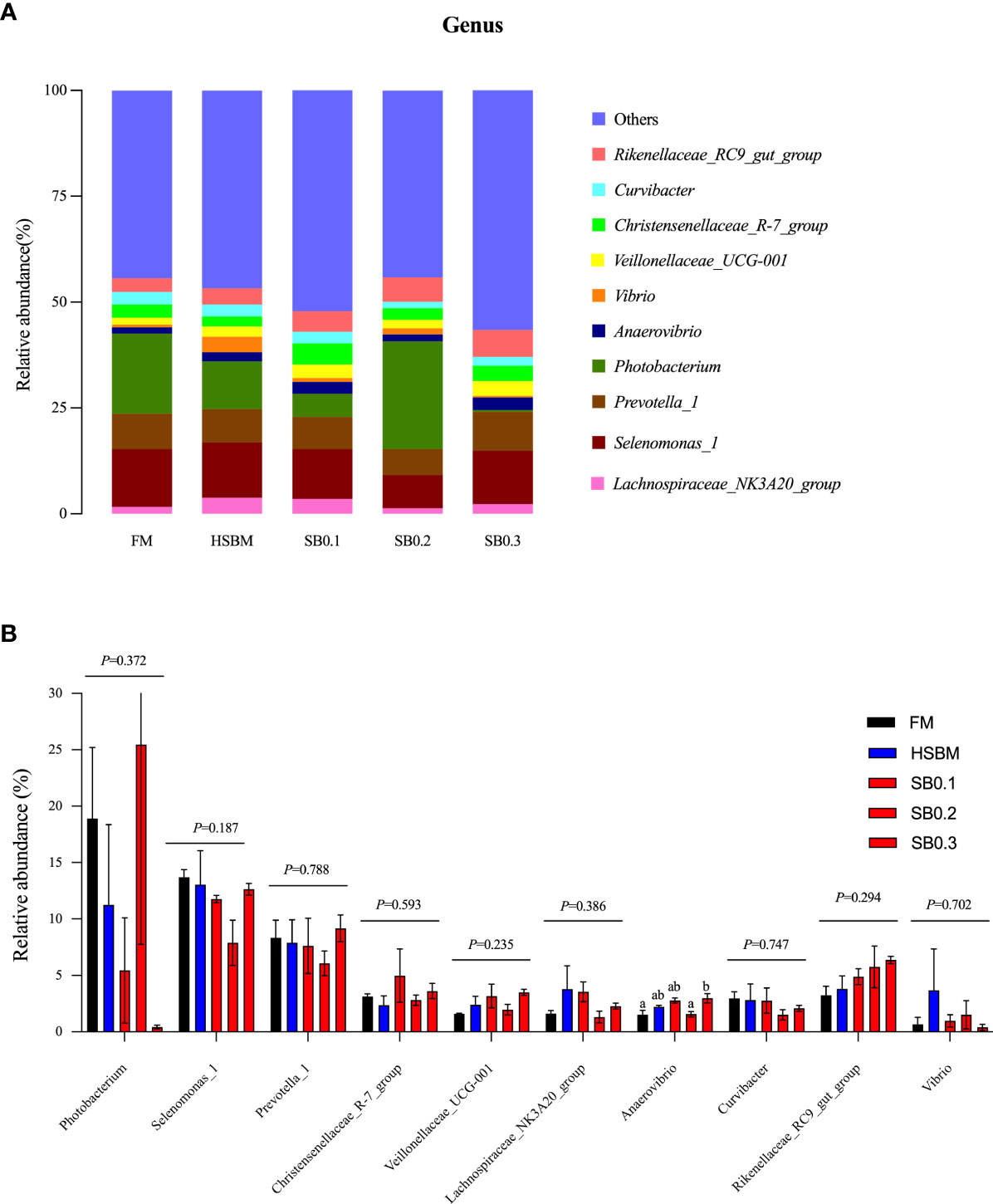
Figure 5 Relative abundance of the dominant bacterial genus in different samples in distal intestine of E. coioides. (A) Intestine microbial composition at genus level, (B) Differential analysis at genus level. Bars bearing the different letters indicate significant differences (P < 0.05), while that with the same letters indicate no significant difference (P > 0.05). Data are presented as the means of per dietary treatment (n = 3 tanks). FM, fish meal; HSBM, 60% FM protein replacement with SBM in FM diets without SB addition; SB0.1, SB0.2 and SB0.3, 0.1%, 0.2%, and 0.3% SB were added in the HSBM diets, respectively.
Expression of intestinal inflammatory factor genes
The relative mRNA levels of intestinal inflammatory factor genes are presented in Figure 6. HSBM group had higher mRNA levels for IL-8, IL-1β, IL-12 and TNF-α genes vs FM group (P < 0.05), but the opposite was true for IL-10 gene. The mRNA level of TGF-β1 for HSBM diet was not different from that for FM diet (P > 0.05). The mRNA levels of IL-8, IL-1β, IL-12 and TNF-α showed a decreasing trend with increasing SB inclusion levels in HSBM diets, and the values of these parameters reduced to the similar level of FM diet, or even lower (P > 0.05). The mRNA level of TGF-β1 in SB0.3 group was the highest among dietary treatments and higher than any other group (P < 0.05). The mRNA level of IL-10 was not affected by dietary treatments (P > 0.05).
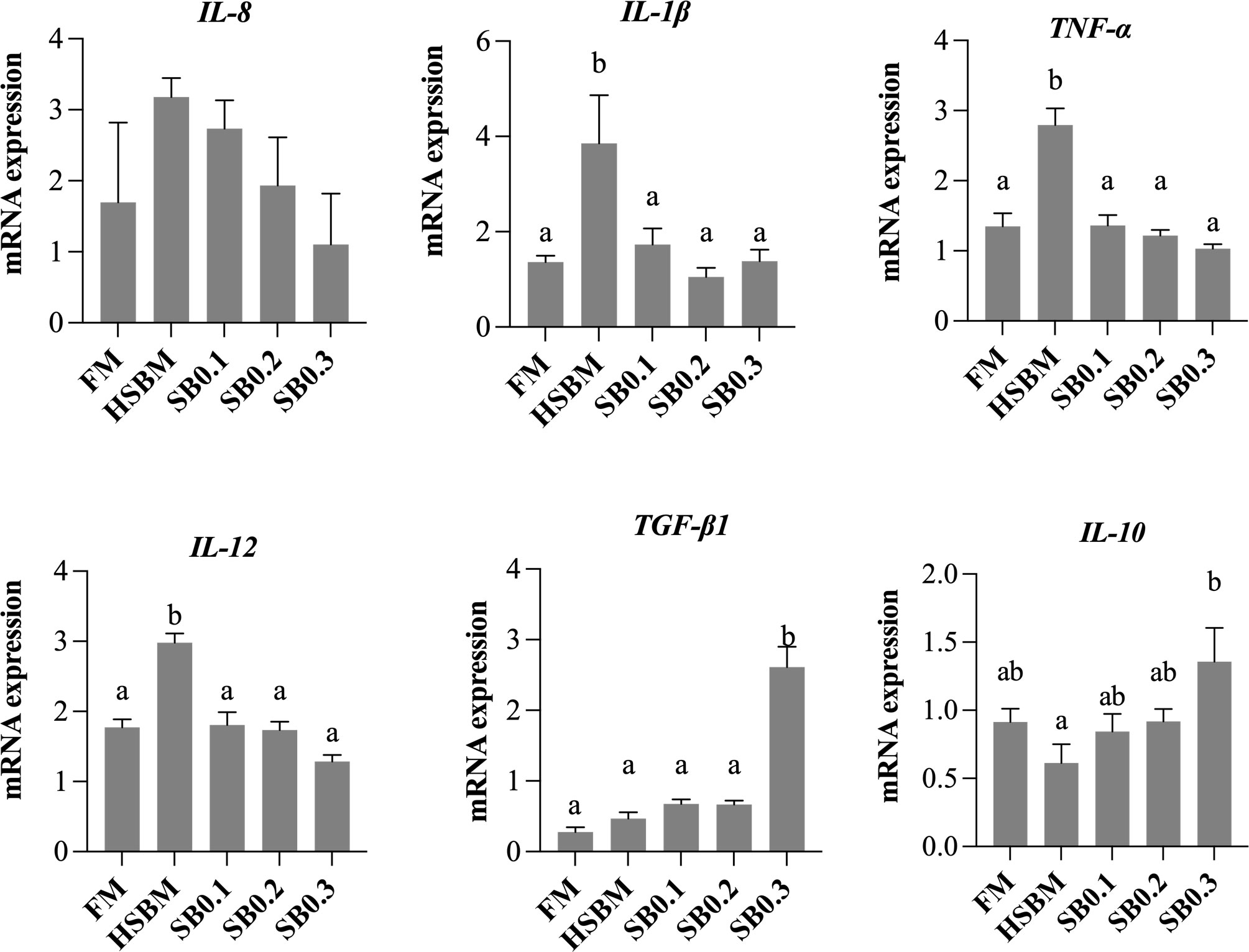
Figure 6 Effects of sodium butyrate (SB) addition in high soybean meal (SBM) diets on mRNA levels of intestinal inflammatory factor genes of groupers in a 56-d feeding period. Bars bearing the different letters indicate significant differences (P < 0.05), while that with the same letters indicate no significant difference (P > 0.05). Data are presented as means ± SEM (n = 3 tanks). Statistical analysis was performed by one-way ANOVA, followed by S-N-K test. FM, fish meal; HSBM, 60% FM protein replacement with SBM in FM diets without SB addition; SB0.1, SB0.2 and SB0.3, 0.1%, 0.2%, and 0.3% SB were added in the HSBM diets, respectively. IL-8, interleukin-8; IL-1β, interleukin-1β; TNF-α, tumor necrosis factor-alpha; IL-12, interleukin-12; TGF-β1, transforming growth factor-beta 1; IL-10, interleukin-10.
Discussion
Growth performance and proximate composition
Results of the present study showed that in HSBM diet decreased significantly WG and SGR vs FM diet, which was observed in previous studies on FM replacement with high SBM (Wang et al., 2017b; Zhu et al., 2021; Zhang et al., 2022). The growth-limiting effect is attributed to the presence of antinutritional factors (ANFs), poor palatability, and lack of some nutrients (Gatlin et al., 2007), of which the most typical side effect is considered as SBMIE (Sahlmann et al., 2013). The SB supplementation in HSBM diets significantly promoted growth performance in a dose-dependent manner and had results comparable to the FM diet in this study. Our current study supported what has been reported in sea bream (Robles et al., 2013), and common carp (Liu et al., 2014a), turbot (Liu et al., 2019), yellow drum (Wu et al., 2020), and Nile tilapia (Abdel-Tawwab et al., 2021).
The whole-body composition was not affected by dietary treatments in this study. This finding was supported by the results observed in similar experiments on grass carp (Liu et al., 2017), black seabream (Ullah et al., 2020), and yellow drum (Wu et al., 2020). In contrast, several studies reported an inconsistent result that dietary supplementation of SB significantly promoted whole-body crude protein contents of Nile tilapia (Ahmed and Sadek, 2015) and European seabass (Abdel-Mohsen et al., 2018), as a result of the promotion of proliferation and differentiation of intestinal epithelial cells (Canani et al., 2012) and upregulated expression of the intestinal small peptide transporter PepT1 (Liu et al., 2014b) in the case of dietary SB administration.
Plasma components
Plasma biochemical indicators reflect the metabolic function and nutritional status of fish (Ren et al., 2021). Plasma HDL-C and LDL-C are a family of lipoproteins involved in cholesterol transport. HDL-C is mainly responsible for TG clearance and TC removal from peripheral tissues, while LDL-C transports cholesterol from the liver to peripheral tissues (Eisenberg, 1984; Jiang et al., 2015). In the current study, except for LDL-C, plasma HDL-C, TC and TG contents were not affected by dietary treatments. The decreased plasma LDL-C content caused by dietary high SBM was also observed in previous studies on FM replacement with SBM in groupers (Ye et al., 2019; Zhao et al., 2021b). As observed in our current study, high SBM diets could decrease plasma TC, TG, LDL-C, and/or HDL-C contents in several previous studies with other fishes (Yamamoto et al., 2010; Dossou et al., 2018; Rahimnejad et al., 2021). However, the values of the index were not improved after dietary SB administration in HSBM diets in this study. Therefore, it seems that groupers were still in a certain degree of malnutrition caused by HSBM diets, though growth improvement by the dietary intervention of SB.
Gut digestive enzymes activity
The activity of digestive enzymes is directly associated with the digestion of nutrients (Jesus et al., 2019). In this study, the high SBM diet resulted in reduced trypsin and protease activities vs FM diet, which was consistent with other studies with other fishes such as hybrid tilapia (Lin and Luo, 2011), Japanese seabass (Zhang et al., 2018), Totoaba macdonaldi (Fuentes-Quesada et al., 2018). The reduction of intestinal trypsin and the protease activities were attributed to the presence of trypsin inhibitors or other ANFs in SBM, resulting in poor growth and feed utilization in fish (Dias et al., 2005; Santigosa et al., 2008; Lin and Luo, 2011; Yaghoubi et al., 2016). After dietary SB intervention, the intestinal activities of trypsin and protease were enhanced in comparison with HSBM group and showed an increasing trend with increasing dietary SB inclusion levels. As evidenced by previous studies of SB intervention on poor growth caused by high SBM feeding, on account of the ability of SB to activate digestive enzyme activity (Tian et al., 2017; Fang et al., 2021; Xie et al., 2021).
Intestinal mucosal barrier
The intestine is not only the site of nutrient digestion and absorption, but also provides an important barrier against exogenous pathogens (Tang et al., 2022). Butyric acid is generally considered as an important substance for the energy supply and proliferation of intestinal epithelial cells (Biagi et al., 2007). Many studies showed that the increased intestinal DAO activity, D-Lac and ET concentrations reflect impaired mucosal permeability, indicating the intestinal mucosal capillary endothelial damage and intestinal flora disorder (Fukudome et al., 2014; Long et al., 2022). Feeding high-SBM diets resulted in increased intestinal D-Lac and ET concentrations vs feeding low-SBM diets or FM diets without SBM (Zhang et al., 2018; Rahimnejad et al., 2021) and in our current study. In the present study, we observed reduced intestinal DAO activity, D-Lac and ET concentrations in fish administrated with dietary SB vs those of fish fed HSBM diets, and reduced to the level of FM diets. Similar results were observed in broilers (Zou et al., 2019) and weaning piglets (Lin et al., 2020) with dietary SB administration. This indicated that dietary SB administration could reduce the intestinal mucosal permeability of farmed animals including fish.
The intestinal histomorphology also acts as a physical barrier to intestinal mucosa (Escaffre et al., 2007). It is clear that the typical signs of SBMIE can cause thickening of the lamina propria and submucosa, and disappearance of supranuclear vacuoles in the enterocytes of DI, accompanied by an infiltration of inflammatory cells (Baeverfjord and Krogdahl, 1996; Urán et al., 2009). Consistent with the previously reported results, we observed a reduction in the number of goblet cells with lymphocyte infiltration, and an increase in the thickness of lamina propria and submucosa caused by high SBM in this study. Higher intestinal MT means a larger surface area for absorbing nutrients (Caspary, 1992). The intestinal MT of fish fed high-SBM diets was lower than that of fish fed FM diets in this study and previous studies with Japanese seabass (Zhang et al., 2018), hybrid grouper (Zhou et al., 2020), and spotted seabass (Rahimnejad et al., 2021). After dietary SB intervention, the intestinal MT was increased with increasing SB inclusion levels, which supported the similar results on European Seabass (Abdel-Mohsen et al., 2018), yellow drum (Wu et al., 2020), and yellow catfish (Zhao et al., 2021a). The number of goblet cells of DI was increased with increasing SB inclusion levels in this study, facilitating the expulsion of pathogens and maintaining the integrity of mucus protective layers (Kim and Ho, 2010). As a result, the intervention effect of dietary SB administration on the SBMIE of groupers can also be achieved by improving histomorphology.
Intestinal microbiota
Dysbacteriosis of the intestinal flora increases the susceptibility to intestinal pathogens, and in severe cases, it will further develop into intestinal infection and reduce immune function (Fu et al., 2021; Vargas-Albores et al., 2021). In the current study, no alterations in intestinal microbial abundance and diversity were observed at both phylum and genus levels among dietary treatments. Previous research reveals that Proteobacteria, Firmicutes, and Bacteroidetes are the dominant phyla of intestine of marine carnivorous fish (Nayak, 2010; Wang et al., 2018), which was consistent with our current results. The change of intestinal bacterial abundance of fish fed high-SBM diets vs FM diets conflicted with what has been reported in phyla and genus abundance of turbot (Li et al., 2020), and in genus abundance of large yellow croaker (Wang et al., 2019) when fed a fermented SBM diet. This inconsistency may be that the latter used fermented SBM instead of SBM as an alternative protein source for FM. Although the changes in phylum and genus intestinal bacterial abundance in fish fed the diets administrated with SB were consistent with a study with common carp (Liu et al., 2014a), our results still deviated from many other experimental results on gilthead sea bream (Piazzon et al., 2017) and turbot (Liu et al., 2019), and in genus abundance changes of young grass carp (Tian et al., 2017) and European seabass (Abdel-Mohsen et al., 2018). The inconsistency regarding the effect of dietary SB on the intestinal flora of fish may be due to complex rearing environmental factors such as fish species, growth stage, and feed composition. Further study is required in this regard.
Expression of intestinal inflammatory factor genes
The presence of an inflammatory response is a complex pathophysiological process, which is mediated by the activation of a variety of cytokines and complement factors secreted by macrophages and leukocytes (Ruhee et al., 2019). Previous studies showed that the SBMIE mediated the expression of up-regulated pro-inflammatory cytokine genes (IL-8, IL-1β, TNF-α and IL-12) and the expression of down-regulated anti-inflammatory factor genes (TGF-β1 and IL-10) in fish (Urán et al., 2008; Wang and Secombes, 2013). Similarly, we observed an up-regulation expression of intestinal IL-8, IL-1β, TNF-α and IL-12 and the down-regulation of intestinal IL-10 in SBM-fed fish, which was in accordance with previous observations (Wang et al., 2017a; Li et al., 2020; Zhao et al., 2021b). Intestinal TGF-β1 is involved in the inactivation of the NF-κB signaling pathway, playing a role in the regulation of the inflammatory response and controlling the expression of genes encoding pro-inflammatory cytokines (Inan et al., 2000; Pedersen et al., 2022). Butyrate and SB are shown to function as an energy source for intestinal epithelial cells to exhibit anti-inflammatory as well as immune modulatory effects on mammals (Nancey et al., 2002; Weber and Kerr, 2006; Meijer et al., 2010; Ali et al., 2022). In this study, the SB intervention achieved a reduction in the expression of intestinal IL-8, IL-1β, TNF-α and IL-12 and an increase in the expression of intestinal TGF-β1. This finding indicates dietary SB intervention could attenuate SBMIE of fish by promoting the production of anti-inflammatory factors and preventing the production of pro-inflammatory factors (Tian et al., 2017; Liu et al., 2019; Yu et al., 2021).
Conclusions
The present study shows that supplementation of SB in high-SBM diets not only improves growth and feed utilization, but also reduces the permeability of intestinal mucosal cells and attenuates the intestinal inflammatory response in juvenile orange-spotted groupers. The optimal inclusion level was 0.2% SB in a high-SBM diet according to the regression analysis of percent weight gain against dietary SB inclusion levels. This is the first report on the intervention effect of dietary SB on grouper enteritis induced by high-SBM diet feeding. Our current study will provide a basis for dietary SB use as a functional feed additive to alleviate SBMIE of fish.
Data availability statement
The datasets presented in this study can be found in online repositories. The names of the repository/repositories and accession number(s) can be found below: BioProject, accession number PRJNA875282.
Ethics statement
The animal study was reviewed and approved by Jimei University, Xiamen.
Author contributions
LK: Investigation, Methodology, and Writing-original draft. YQ: Data curation, Formal analysis, and Investigation. TS: Investigation and data curation. KW: Project Administration and Supervision. JY: Conceptualization, Supervision, and Writing-review. All authors contributed to the article and approved the submitted version.
Funding
This study was supported by funding from the National Natural Science Foundation of China (Grant Nos. 31772861 and 31372546), and the Science and Technology Project of Fujian Province, China (No. 2020N0012).
Acknowledgments
The author sincerely thanks TS for the assistance in the Method section (Song et al., 2022).
Conflict of interest
The authors declare that the research was conducted in the absence of any commercial or financial relationships that could be construed as a potential conflict of interest.
Publisher’s note
All claims expressed in this article are solely those of the authors and do not necessarily represent those of their affiliated organizations, or those of the publisher, the editors and the reviewers. Any product that may be evaluated in this article, or claim that may be made by its manufacturer, is not guaranteed or endorsed by the publisher.
References
Abdel-Mohsen H. H., Wassef E. A., El-Bermawy N. M., Abdel-Meguid N. E., Saleh N. E., Barakat K. M., et al. (2018). Advantageous effects of dietary butyrate on growth, immunity response, intestinal microbiota and histomorphology of European seabass (Dicentrarchus labrax) fry. Egypt. J. Aquat. Biol. Fish. 22, 93–110. doi: 10.21608/ejabf.2018.12055
Abdel-Tawwab M., Shukry M., Farrag F. A., El-Shafai N. M., Dawood M. A. O. (2021). And abdel-latif, h Dietary sodium butyrate nanoparticles enhanced growth, digestive enzyme activities, intestinal histomorphometry, and transcription of growth-related genes in Nile tilapia juveniles. M. R. Aquaculture 536, 736467. doi: 10.1016/j.aquaculture.2021.736467
Ahmed H. A., Sadek K. M. (2015). Impact of dietary supplementation of sodium butyrate and/or protexin on the growth performance, some blood parameters, and immune response of Oreochromis niloticus. Int. J. Agric. Innov. Res. 3, 985–991. doi: 10.1016/0003-9969(77)90069-3
Ali I., Li C., Kuang M., Shah A. U., Shafiq M., Ahmad M. A., et al. (2022). Nrf2 activation and NF-kb and caspase/bax signaling inhibition by sodium butyrate alleviates LPS-induced cell injury in bovine mammary epithelial cells. Mol. Immunol. 148, 54–67. doi: 10.1016/j.molimm.2022.05.121
Anguiano M., Pohlenz C., Buentello A., Gatlin D. M. (2013). The effects of prebiotics on the digestive enzymes and gut histomorphology of red drum (Sciaenops ocellatus) and hybrid striped bass (Morone chrysops × m. saxatilis). Br. J. Nutr. 109, 623–629. doi: 10.1017/S0007114512001754
AOAC (1995). Official methods of analysis. 16th Edn (Washington: Association of Official Analytical Chemists).
Baeverfjord G., Krogdahl A. (1996). Development and regression of soybean meal induced enteritis in Atlantic salmon, Salmo salar l., distal intestine: a comparison with the intestines of fasted fish. J. Fish Dis. 19, 375–387. doi: 10.1111/j.1365-2761.1996.tb00376.x
Bai F., Niu X., Wang X., Ye J. (2021). Growth performance, biochemical composition and expression of lipid metabolism related genes in groupers (Epinephelus coioides) are altered by dietary taurine. Aquacult. Nutr. 27, 2690–2702. doi: 10.1111/anu.13395
Bedford A., Gong J. (2018). Implications of butyrate and its derivatives for gut health and animal production. Anim. Nutr. 4, 151–159. doi: 10.1016/j.aninu.2017.08.010
Biagi G., Piva A., Moschini M., Vezzali E., Roth F. X. (2007). Performance, intestinal microflora, and wall morphology of weanling pigs fed sodium butyrate. J. Anim. Sci. 85, 1184–1191. doi: 10.2527/jas.2006-378
Booman M., Forster I., Vederas J. C., Groman D. B., Jones S. R. M. (2018). Soybean meal-induced enteritis in Atlantic salmon (Salmo salar) and Chinook salmon (Oncorhynchus tshawytscha) but not in pink salmon (O. gorbuscha). Aquaculture 483, 238–243. doi: 10.1016/j.aquaculture.2017.10.025
Canani R. B., Costanzo M. D., Leone L. (2012). The epigenetic effects of butyrate: potential therapeutic implications for clinical practice. Clin. Epigenet. 4, 4–0. doi: 10.1186/1868-7083-4-4
Carmona P. A. U. (2008). Etiology of soybean-induced enteritis in fish [PhD thesis]. [Netherlands]: Wageningen University of Netherlands.
Caspary W. F. (1992). Physiology and pathophysiology of intestinal absorption. Am. J. Clin. Nutr. 55, 299S–308S. doi: 10.1093/ajcn/55.1.299s
China Fishery Statistics Yearbook (2021). Bureau of fisheries, ministry of agriculture (Beijing, China: China Agriculture Press).
Dennis L. P., Ashford G., Thai T. Q., Van In V., Ninh N. H., Elizur A. (2020). Hybrid grouper in Vietnamese aquaculture: Production approaches and profitability of a promising new crop. Aquaculture 522, 735108. doi: 10.1016/j.aquaculture.2020.735108
Dias J., Alvarez M. J., Arzel J., Corraze G., Diez A., Bautista J. M., et al. (2005). Dietary protein source affects lipid metabolism in the European seabass (Dicentrarchus labrax). Comp. Biochem. Phys. A. 142, 19–31. doi: 10.1016/j.cbpb.2005.07.005
Dossou S., Koshio S., Ishikawa M., Yokoyama S., Dawood M. A. O., El Basuini M. F., et al. (2018). Effect of partial replacement of fish meal by fermented rapeseed meal on growth, immune response and oxidative condition of red sea bream juvenile, Pagrus major. Aquaculture 490, 228–235. doi: 10.1016/j.aquaculture.2018.02.010
Eisenberg S. (1984). High density lipoprotein metabolism. J. Lipid. Res. 25, 1017–1058. doi: 10.1016/S0022-2275(20)37713-0
Escaffre A.-M., Kaushik S., Mambrini M. (2007). Morphometric evaluation of changes in the digestive tract of rainbow trout (Oncorhynchus mykiss) due to fish meal replacement with soy protein concentrate. Aquaculture 273, 127–138. doi: 10.1016/j.aquaculture.2007.09.028
Fang L., Wang Q., Guo X., Pan X., Li X. (2021). Effects of dietary sodium butyrate on growth performance, antioxidant capacity, intestinal histomorphology and immune response in juvenile pengze crucian carp (Carassius auratus pengze). Aquacult. Rep. 21, 100828. doi: 10.1016/j.aqrep.2021.100828
FAO (2020). The state of world fisheries and aquaculture 2020. Food Agric. Organ. United Nations (FAO) 0-206. doi: 10.4060/ca9229en
Ferrara E., Gustinelli A., Fioravanti M. L., Restucci B., Quaglio F., Marono S., et al. (2015). Histological and micro-/macro-morphological evaluation of intestine in sharpsnout seabream (Diplodus puntazzo) fed soybean meal-based diets added with MOS and inulin as prebiotics. Aquacult. Int. 23, 1525–1537. doi: 10.1007/s10499-015-9902-y
Fuentes-Quesada J. P., Viana M. T., Rombenso A. N., Guerrero-Rentería Y., Nomura-Solís M., Gomez-Calle V., et al. (2018). Enteritis induction by soybean meal in Totoaba macdonaldi diets: Effects on growth performance, digestive capacity, immune response and distal intestine integrity. Aquaculture 495, 78–89. doi: 10.1016/j.aquaculture.2018.05.025
Fukudome I., Kobayashi M., Dabanaka K., Maeda H., Okamoto K., Okabayashi T., et al. (2014). Diamine oxidase as a marker of intestinal mucosal injury and the effect of soluble dietary fiber on gastrointestinal tract toxicity after intravenous 5-fluorouracil treatment in rats. Med. Mol. Morphol. 47, 100–107. doi: 10.1007/s00795-013-0055-7
Fu R., Li Z., Zhou R., Li C., Shao S., Li J. (2021). The mechanism of intestinal flora dysregulation mediated by intestinal bacterial biofilm to induce constipation. Bioengineered 12, 6484–6498. doi: 10.1080/21655979.2021.1973356
Gatlin D. M., Barrows F. T., Brown P., Dabrowski K., Gaylord T. G., Hardy R. W., et al. (2007). Expanding the utilization of sustainable plant products in aquafeeds: a review. Aquacult. Res. 38, 551–579. doi: 10.1111/j.1365-2109.2007.01704.x
Hamer H. M., Jonkers D., Venema K., Vanhoutvin S., Troost F. J., Brummer R.-J. (2007). Review article: the role of butyrate on colonic function. Aliment. Pharm. Ther. 27, 104–119. doi: 10.1111/j.1365-2036.2007.03562.x
Hanaki K.-I., Ike F., Kajita A., Yasuno W., Yanagiba M., Goto M. (2014). A broadly reactive One-Step SYBR Green I Real-Time RT-PCR assay for rapid detection of murine norovirus. PLoS ONE 9, E98108. doi: 10.1371/journal.pone.0098108
Hardy R. W. (2010). Utilization of plant proteins in fish diets: effects of global demand and supplies of fishmeal. Aquacult. Res. 41, 770–776. doi: 10.1111/j.1365-2109.2009.02349.x
Hu H., Liu J., Li Y., Zhang Y., Mai K., Ai Q., et al. (2014). Effects of dietary daidzein on growth performance, activities of digestive enzymes, anti-oxidative ability and intestinal morphology in juvenile turbot (Scophthalmus maximus l.). J. Fisheries China 38, 1503–1513. doi: 10.3724/SP.J.1231.2014.49253.
Inan M. S., Rasoulpour R. J., Yin L., Hubbard A. K., Rosenberg D. W., Giardina C. (2000). The luminal short-chain fatty acid butyrate modulates NF-κB activity in a human colonic epithelial cell line. Gastroenterology 118, 724–734. doi: 10.1016/S0016-5085(00)70142-9
Jesus G. F. A., Pereira S. A., Owatari M. S., Addam K., Silva B. C., Sterzelecki F. C., et al. (2019). Use of protected forms of sodium butyrate benefit the development and intestinal health of Nile tilapia during the sexual reversion period. Aquaculture 504, 326–333. doi: 10.1016/j.aquaculture.2019.02.018
Jiang S., Wu X., Li W., Wu M., Luo Y., Lu S., et al. (2015). Effects of dietary protein and lipid levels on growth, feed utilization, body and plasma biochemical compositions of hybrid grouper (Epinephelus lanceolatus ♂ × Epinephelus fuscoguttatus ♀) juveniles. Aquaculture 446, 148–155. doi: 10.1016/j.aquaculture.2015.04.034
Kim Y. S., Ho S. B. (2010). Intestinal goblet cells and mucins in health and disease: recent insights and progress. Curr. Gastroenterol. Rep. 12, 319–330. doi: 10.1007/s11894-010-0131-2
Ko Y.-T., Hsu S.-C., Kazłowski B., Huang F.-H., Liou C.-H. (2020). White muscle proteome analysis showing insights into the protein expression in orange-spotted grouper (Epinephelus coioides) muscle. Food Biosci. 36, 100655. doi: 10.1016/j.fbio.2020.100655
Kuo J., Chen C.-Y., Han C.-C., Ju Y.-M., Tew K. S. (2021). Analyses of diet preference of larval orange-spotted grouper (Epinephelus coioides) grown under inorganic fertilization method using next-generation sequencing. Aquaculture 531, 735916. doi: 10.1016/j.aquaculture.2020.735916
Lin F., Li X., Wen J., Wang C., Peng Y., Feng J., et al. (2020). Effects of coated sodium butyrate on performance, diarrhea, intestinal microflora and barrier function of pigs during the first 2-week post-weaning. Anim. Feed Sci. Tech. 263, 114464. doi: 10.1016/j.anifeedsci.2020.114464
Lin S., Luo L. (2011). Effects of different levels of soybean meal inclusion in replacement for fish meal on growth, digestive enzymes and transaminase activities in practical diets for juvenile tilapia, Oreochromis niloticus × O. aureus. Anim. Feed Sci. Tech. 168, 80–87. doi: 10.1016/j.anifeedsci.2011.03.012
Liu Y., Chen Z., Dai J., Yang P., Xu W., Ai Q., et al. (2019). Sodium butyrate supplementation in high-soybean meal diets for turbot (Scophthalmus maximus l.): Effects on inflammatory status, mucosal barriers and microbiota in the intestine. Fish Shellfish. Immunol. 88, 65–75. doi: 10.1016/j.fsi.2019.02.064
Liu M., Guo W., Wu F., Qu Q., Tan Q., Gong W. (2017). Dietary supplementation of sodium butyrate may benefit growth performance and intestinal function in juvenile grass carp (Ctenopharyngodon idellus). Aquac. Res. 48, 4102–4111. doi: 10.1111/are.13230
Liu W., Yang Y., Zhang J., Gatlin D. M., Ringø E., Zhou Z. (2014a). Effects of dietary microencapsulated sodium butyrate on growth, intestinal mucosal morphology, immune response and adhesive bacteria in juvenile common carp (Cyprinus carpio) pre-fed with or without oxidised oil. Br. J. Nutr. 112, 15–29. doi: 10.1017/S0007114514000610
Liu Z., Zhou Y., Liu S., Zhao Q., Feng J., Lu S., et al. (2014b). Characterization and dietary regulation of oligopeptide transporter (PepT1) in different ploidy fishes. Peptides 52, 149–156. doi: 10.1016/j.peptides.2013.12.017
Li C., Zhang B., Liu C., Zhou H., Wang X., Mai K., et al. (2020). Effects of dietary raw or Enterococcus faecium fermented soybean meal on growth, antioxidant status, intestinal microbiota, morphology, and inflammatory responses in turbot (Scophthalmus maximus l.). Fish Shellfish. Immunol. 100, 261–271. doi: 10.1016/j.fsi.2020.02.070
Long S., You Y., Dong X., Tan B., Zhang S., Chi S., et al. (2022). Effect of dietary oxidized fish oil on growth performance, physiological homeostasis and intestinal microbiome in hybrid grouper (♀ Epinephelus fuscoguttatus × ♂ Epinephelus lanceolatus). Aquacult. Rep. 24, 101130. doi: 10.1016/j.aqrep.2022.101130
Mahamud A. G. M. S. U., Anu M. S., Baroi A., Datta A., Khan M. S. U., Rahman M., et al. (2022). Microplastics in fishmeal: A threatening issue for sustainable aquaculture and human health. Aquacult. Res. 25, 101205. doi: 10.1016/j.aqrep.2022.101205
Meijer K., de Vos P. (2010). And priebe, m Butyrate and other short-chain fatty acids as modulators of immunity: what relevance for health? G. Curr. Opin. Clin. Nutr. 13, 715–721. doi: 10.1097/MCO.0b013e32833eebe5
Nancey S., Bienvenu J., Coffin B., Andre F. O., Descos L., Flourie B. (2002). Butyrate strongly inhibits in vitro stimulated release of cytokines in blood. Digest. Dis. Sci. 47, 8. doi: 10.1023/A:1014781109498
Nayak S. K. (2010). Role of gastrointestinal microbiota in fish: Role of gastrointestinal microbiota in fish. Aquacult. Res. 41, 1553–1573. doi: 10.1111/j.1365-2109.2010.02546.x
Niu X., Qian X., Feng H., Yi K., Li D., Chen W., et al. (2021). Growth and metabolic responses of grouper juveniles (Epinephelus coioides) fed diets containing varying levels of leucine. Aquaculture 534, 736281. doi: 10.1016/j.aquaculture.2020.736281
Pedersen S. S., Prause M., Williams K., Barrès R., Billestrup N. (2022). Butyrate inhibits IL-1β-induced inflammatory gene expression by suppression of NF-κB activity in pancreatic beta cells. J. Biol. Chem. 102312, 298. doi: 10.1016/j.jbc.2022.102312
Peng M., Xu W., Ai Q., Mai K., Liufu Z., Zhang K. (2013). Effects of nucleotide supplementation on growth, immune responses and intestinal morphology in juvenile turbot fed diets with graded levels of soybean meal (Scophthalmus maximus l.). Aquaculture 392–395, 51–58. doi: 10.1016/j.aquaculture.2013.02.002
Piazzon M. C., Calduch-Giner J. A., Fouz B., Estensoro I., Simó-Mirabet P., Puyalto M., et al. (2017). Under control: how a dietary additive can restore the gut microbiome and proteomic profile, and improve disease resilience in a marine teleostean fish fed vegetable diets. Microbiome 5, 164. doi: 10.1186/s40168-017-0390-3
Qin Y., He L., Wang Y., Li D., Chen W., Ye J. (2022). Growth performance, fatty acid composition, and lipid metabolism are altered in groupers (Epinephelus coioides) by dietary fish oil replacement with palm oil. Anim. Nutr. 8, 102–113. doi: 10.1016/j.aninu.2021.04.007
Rahimnejad S., Zhang J.-J., Wang L., Sun Y., Zhang C. (2021). Evaluation of Bacillus pumillus SE5 fermented soybean meal as a fish meal replacer in spotted seabass (Lateolabrax maculatus) feed. Aquaculture 531, 735975. doi: 10.1016/j.aquaculture.2020.735975
Ren M., Liang H., Ge X., Chen X., Mi H., Ji K. (2021). Effects of dietary carbohydrate-to-lipid ratio on growth performance, plasma parameters and hepatic antioxidant status in ide (Leuciscus idus, linnaeu). Aquacult. Rep. 19, 100618. doi: 10.1016/j.aqrep.2021.100618
Rimoldi S., Finzi G., Ceccotti C., Girardello R., Grimaldi A., Ascione C., et al. (2016). Butyrate and taurine exert a mitigating effect on the inflamed distal intestine of European sea bass fed with a high percentage of soybean meal. Fish Aquat. Sci. 19, 40. doi: 10.1186/s41240-016-0041-9
Robles R., Lozano A. B., Sevilla A., Márquez L., Nuez-Ortín W., Moyano F. J. (2013). Effect of partially protected butyrate used as feed additive on growth and intestinal metabolism in sea bream (Sparus aurata). Fish Physiol. Biochem. 39, 1567–1580. doi: 10.1007/s10695-013-9809-3
Ruhee R. T., Ma S., Suzuki K. (2019). Sulforaphane protects cells against lipopolysaccharide-stimulated inflammation in murine macrophages. Antioxidants 8, 577. doi: 10.3390/antiox8120577
Sahlmann C., Sutherland B. J. G., Kortner T. M., Koop B. F., Krogdahl Å., Bakke A. M. (2013). Early response of gene expression in the distal intestine of Atlantic salmon (Salmo salar l.) during the development of soybean meal induced enteritis. Fish Shellfish. Immunol. 34, 599–609. doi: 10.1016/j.fsi.2012.11.031
Santigosa E., Sánchez J., Médale F., Kaushik S., Pérez-Sánchez J., Gallardo M. A. (2008). Modifications of digestive enzymes in trout (Oncorhynchus mykiss) and sea bream (Sparus aurata) in response to dietary fish meal replacement by plant protein sources. Aquaculture 282, 68–74. doi: 10.1016/j.aquaculture.2008.06.007
Schmittgen T. D., Livak K. J. (2008). Analyzing real-time PCR data by the comparative C(T) method. Nat. Protoc. 3, 1101–1108. doi: 10.1038/nprot.2008.73
Song T., Qin Y., Ke L., Wang X., Wang K., Sun Y., et al. (2022) Dietary lactoferrin supplementation improves growth performance and intestinal health of juvenile orange-spotted groupers (Epinephelus coioides). Available at: https://www.researchsquare.com/article/rs-1723165/v1.
Tang X., Xiong K., Wassie T., Wu X. (2022). Curcumin and intestinal oxidative stress of pigs with intrauterine growth retardation: a review. Front. Nutr. 9. doi: 10.3389/fnut.2022.847673
Tian L., Zhou X.-Q., Jiang W.-D., Liu Y., Wu P., Jiang J., et al. (2017). Sodium butyrate improved intestinal immune function associated with NF-κB and p38MAPK signalling pathways in young grass carp (Ctenopharyngodon idella). Fish Shellfish. Immunol. 66, 548–563. doi: 10.1016/j.fsi.2017.05.049
Topping D. L., Clifton P. M. (2001). Short-chain fatty acids and human colonic function: roles of resistant starch and nonstarch polysaccharides. Physiol. Rev. 81, 1031–1064. doi: 10.1152/physrev.2001.81.3.1031
Ullah S., Zhang G., Zhang J., Shengli T., Wang L., Kalhoro H., et al. (2020). Effects of microencapsulated sodium butyrate supplementation on growth performance, intestinal development and antioxidative capacity of juvenile black sea bream (Acanthopagrus schlegelii). Aquacult. Res. 51, 4893–4904. doi: 10.1111/are.14824
Urán P. A., Gonçalves A. A., Taverne-Thiele J. J., Schrama J. W., Verreth J. A. J., Rombout J. H. W. M. (2008). Soybean meal induces intestinal inflammation in common carp (Cyprinus carpio l.). Fish Shellfish. Immunol. 25, 751–760. doi: 10.1016/j.fsi.2008.02.013
Urán P. A., Schrama J. W., Rombout J. H. W. M., Taverne-Thiele J. J., Obach A., Koppe W., et al. (2009). Time-related changes of the intestinal morphology of Atlantic salmon, Salmo salar l., at two different soybean meal inclusion levels. J. Fish Dis. 32, 733–744. doi: 10.1111/j.1365-2761.2009.01049.x
Vargas-Albores F., Martínez-Córdova L. R., Hernández-Mendoza A., Cicala F., Lago-Lestón A., Martínez-Porchas M. (2021). Therapeutic modulation of fish gut microbiota, a feasible strategy for aquaculture? Aquaculture 544, 737050. doi: 10.1016/j.aquaculture.2021.737050
Wang J., Liang D., Yang Q., Tan B., Dong X., Chi S., et al. (2020). The effect of partial replacement of fish meal by soy protein concentrate on growth performance, immune responses, gut morphology and intestinal inflammation for juvenile hybrid grouper (Epinephelus fuscoguttatus ♀ × Epinephelus lanceolatus ♂). Fish Shellfish. Immunol. 98, 619–631. doi: 10.1016/j.fsi.2019.10.025
Wang A. R., Ran C., Ringø E., Zhou Z. G. (2018). Progress in fish gastrointestinal microbiota research. Rev. Aquacult. 10, 626–640. doi: 10.1111/raq.12191
Wang T., Secombes C. J. (2013). The cytokine networks of adaptive immunity in fish. Fish Shellfish. Immunol. 35, 1703–1718. doi: 10.1016/j.fsi.2013.08.030
Wang J., Tao Q., Wang Z., Mai K., Xu W., Zhang Y., et al. (2017b). Effects of fish meal replacement by soybean meal with supplementation of functional compound additives on intestinal morphology and microbiome of Japanese seabass (Lateolabrax japonicus). Aquacult. Res. 48, 2186–2197. doi: 10.1111/are.13055
Wang Y., Wang L., Zhang C., Song K. (2017a). Effects of substituting fishmeal with soybean meal on growth performance and intestinal morphology in orange-spotted grouper (Epinephelus coioides). Aquacult. Rep. 5, 52–57. doi: 10.1016/j.aqrep.2016.12.005
Wang P., Zhou Q., Feng J., He J., Lou Y., Zhu J. (2019). Effect of dietary fermented soybean meal on growth, intestinal morphology and microbiota in juvenile large yellow croaker, Larimichthys crocea. Aquacult. Res. 50, 748–757. doi: 10.1111/are.13929
Weber T. E., Kerr B. J. (2006). Butyrate differentially regulates cytokines and proliferation in porcine peripheral blood mononuclear cells. Vet. Immunol. Immunop. 113, 139–147. doi: 10.1016/j.vetimm.2006.04.006
Wu X., Wang L., Xie Q., Tan P. (2020). Effects of dietary sodium butyrate on growth, diet conversion, body chemical compositions and distal intestinal health in yellow drum (Nibea albiflora , Richardson). Aquacult. Res. 51, 69–79. doi: 10.1111/are.14348
Xie D., Dai Q., Xu C., Li Y. (2021). Dietary tributyrin modifies intestinal function by altering morphology, gene expression and microbiota profile in common carp (Cyprinus carpio) fed all-plant diets. Aquacult. Nutr. 27, 439–453. doi: 10.1111/anu.13197
Yaghoubi M., Mozanzadeh M. T., Marammazi J. G., Safari O., Gisbert E. (2016). Dietary replacement of fish meal by soy products (soybean meal and isolated soy protein) in silvery-black porgy juveniles (Sparidentex hasta). Aquaculture 464, 50–59. doi: 10.1016/j.aquaculture.2016.06.002
Yamamoto T., Iwashita Y., Matsunari H., Sugita T., Furuita H., Akimoto A., et al. (2010). Influence of fermentation conditions for soybean meal in a non-fish meal diet on the growth performance and physiological condition of rainbow trout Oncorhynchus mykiss. Aquaculture 309, 173–180. doi: 10.1016/j.aquaculture.2010.09.021
Yang X., He Y., Lin S., Dong X., Yang Q., Liu H., et al. (2021). Saccharomyces cerevisiae extracts improved the effects of a low fishmeal, complex plant protein diet in the orange-spotted grouper, Epinephelus coioides. Aquacult. Rep. 19, 100574. doi: 10.1016/j.aqrep.2020.100574
Ye J., Liu X., Wang Z., Wang K. (2011). Effect of partial fish meal replacement by soybean meal on the growth performance and biochemical indices of juvenile Japanese flounder Paralichthys olivaceus. Aquacult. Int. 19, 143–153. doi: 10.1007/s10499-010-9348-1
Ye H., Xu M., Chen L., Tan X., Chen S., Zou C., et al. (2019). Effects of dietary plant protein sources influencing hepatic lipid metabolism and hepatocyte apoptosis in hybrid grouper (Epinephelus lanceolatus ♂ × Epinephelus fuscoguttatus ♀). Aquaculture 506, 437–444. doi: 10.1016/j.aquaculture.2019.03.075
Yu G., Ou W., Ai Q., Zhang W., Mai K., Zhang Y. (2021). In vitro study of sodium butyrate on soyasaponin challenged intestinal epithelial cells of turbot (Scophthalmus maximus l.) refer to inflammation, apoptosis and antioxidant enzymes. Fish Shellfish. Immunol. Rep. 2, 100031. doi: 10.1016/j.fsirep.2021.100031
Zhang C., Rahimnejad S., Wang Y., Lu K., Song K., Wang L., et al. (2018). Substituting fish meal with soybean meal in diets for Japanese seabass (Lateolabrax japonicus): Effects on growth, digestive enzymes activity, gut histology, and expression of gut inflammatory and transporter genes. Aquaculture 483, 173–182. doi: 10.1016/j.aquaculture.2017.10.029
Zhang B., Zhang Y., Cui M., Zhang M., Xu J., Zhang Z., et al. (2022). Comparison of the performance of raw and Lactobacillus paracasei fermented soybean meal in diets for turbot (Scophthalmus maximus l.): Growth, intestinal morphology, apoptosis, tight junction, and microbiota. Aquacult. Rep. 24, 101184. doi: 10.1016/j.aqrep.2022.101184
Zhao S., Chen Z., Zheng J., Dai J., Ou W., Xu W., et al. (2019). Citric acid mitigates soybean meal induced inflammatory response and tight junction disruption by altering TLR signal transduction in the intestine of turbot, Scophthalmus maximus l. Fish Shellfish. Immunol. 92, 181–187. doi: 10.1016/j.fsi.2019.06.004
Zhao H., Wang G., Wang H., Mo W., Huang Y., Cao J., et al. (2021a). Effects of dietary sodium butyrate on growth, digestive enzymes, body composition and nutrient retention-related gene expression of juvenile yellow catfish (Pelteobagrus fulvidraco). Anim. Nutr. 7, 539–547. doi: 10.1016/j.aninu.2020.12.007
Zhao X., Wang Y., Wang X., Ye J. (2021b). Growth performance, plasma components, and intestinal barrier in grouper (Epinephelus coioides) are altered by dietary fish meal replacement with extruded soybean meal. Aquacult. Rep. 21, 100863. doi: 10.1016/j.aqrep.2021.100863
Zhou Z., Yao W., Ye B., Wu X., Li X., Dong Y. (2020). Effects of replacing fishmeal protein with poultry by-product meal protein and soybean meal protein on growth, feed intake, feed utilization, gut and liver histology of hybrid grouper (Epinephelus fuscoguttatus ♀ × Epinephelus lanceolatus ♂) juveniles. Aquaculture 516, 734503. doi: 10.1016/j.aquaculture.2019.734503
Zhu W.-Q., Yuan X.-Q., Luo H.-J., Shao J.-C., Chen X.-H. (2021). High percentage of dietary soybean meal inhibited growth, impaired intestine healthy and induced inflammation by TLR-MAPK/NF-κB signaling pathway in large yellow croaker (Larimichthys crocea). Aquacult. Rep. 20, 100735. doi: 10.1016/j.aqrep.2021.100735
Keywords: sodium butyrate, growth performance, intestinal injury, Epinephelus coioides, inflammatory response
Citation: Ke L, Qin Y, Song T, Wang K and Ye J (2022) Dietary sodium butyrate administration alleviates high soybean meal-induced growth retardation and enteritis of orange-spotted groupers (Epinephelus coioides). Front. Mar. Sci. 9:1029397. doi: 10.3389/fmars.2022.1029397
Received: 27 August 2022; Accepted: 12 September 2022;
Published: 28 September 2022.
Edited by:
Jianchun Shao, Fujian Agriculture and Forestry University, ChinaCopyright © 2022 Ke, Qin, Song, Wang and Ye. This is an open-access article distributed under the terms of the Creative Commons Attribution License (CC BY). The use, distribution or reproduction in other forums is permitted, provided the original author(s) and the copyright owner(s) are credited and that the original publication in this journal is cited, in accordance with accepted academic practice. No use, distribution or reproduction is permitted which does not comply with these terms.
*Correspondence: Jidan Ye, yjyjd@jmu.edu.cn