- 1Consejo Superior de Investigaciones Científicas, (CSIC), Institut de Ciències del Mar, CSIC, Barcelona, Spain
- 2Unitat de Tecnologia Marina, CSIC, Barcelona, Spain
- 3Instituto de Oceanografía y Cambio Global (IOCAG), Unidad Asociada ULPGC-CSIC, Universidad de Las Palmas de Gran Canaria, Las Palmas de Gran Canaria, Spain
- 4Pelagic Ecology Research Group, Scottish Oceans Institute, School of Biology, Gatty Marine Laboratory, University of St Andrews, St. Andrews, United Kingdom
Mesopelagic fish populations are characterised by high species richness and abundance, and have been identified as important contributors to the active carbon fluxes in the open ocean. We report variability in communities of mesopelagic fish between five zones around the Iberian Peninsula, i.e. Balears and Alboran in the Mediterranean, and Cadiz, Lisboa and Galicia in the Atlantic. Day and night samples were collected from 7 layers of the water column with a midwater trawl fitted with a multisampler. Temperature and salinity regimes were very different on the Mediterranean and Atlantic sides of the peninsula, with much higher values through the entire water column in the Mediterranean, characterized by a strong pycnocline. The highest productivity was observed off Lisboa, where Chlorophyll a concentrations were two orders of magnitude higher than in any other zone. Samples from the western Mediterranean held 22 fish species, while 67 were found in the Atlantic. The lowest diversity and the highest dominance were observed in Balears, and the highest diversity in Cadiz zone. In all zones, but particularly in those in the Mediterranean, mesopelagic populations were dominated by a high number of small fish with low individual biomasses. The species Benthosema glaciale, Cyclothone pygmaea and Ceratoscopelus maderensis were common in the Mediterranean populations, whereas in the Atlantic, Cyclothone microdon/livida, Valenciennellus tripunctulatus, Ceratoscopelus warmingii and Benthosema suborbitale were the most common species. Temperature and salinity (both at surface and in the mesopelagic zone) were the main environmental factors explaining variability in assemblage composition. A persistent (day-night) deep scattering layer was observed using the vessel-based echosounder in all zones, and was comprised primarily of the gonostomatid Cyclothone spp. Night-time echosounder observations of scattering layers near the surface were observed in Balears, Alboran, Cadiz and Lisboa, where night surface net collections indicated that Myctophidae, Stomiidae and Phosichthyidae migration extended to the upper 100 m. Sternoptychids and the gonostomatid Sigmops elongatus seldom reached the upper 100 m in their night vertical migrations. Night stratified hauls of 30 m resolution carried out in the epipelagic zone showed that abundances maxima of migratory fish coincided with the location of the Chlorophyll a maxima.
Introduction
Mesopelagic fish communities are comprised of a large number of species living permanently, or for part of the daily cycle, in the so-called ocean ‘twilight zone’ (200 to 1,000 m depth) (Haedrich, 1997). Mesopelagic fish are generally small, very numerous and have evolved specific morphological and physiological traits that enable them to cope with the dim-light conditions and other challenges of the deep-sea environment (e.g. pressure), and to perform diel vertical migration (DVM) across a substantial pressure gradient (Priede, 2017). Many mesopelagic organisms perform DVM to the upper epipelagic layer at night, following the same displacement of most of their zooplankton prey, before returning to the twilight zone at dawn (Sutton, 2013; Brierley, 2014).
Despite the present uncertainty in global mesopelagic fish biomass, that arises in part from trawl net escapement and avoidance (Koslow et al., 1997; Irigoien et al., 2014; Proud et al., 2019; Pauly et al., 2021), there is a general agreement on their biomass estimations between 1.8 and 16 billion tonnes (Proud et al., 2019). Regardless of their high biomass and extended distribution, they are not commercially exploited yet although they may potentially be fished in the future (Grimaldo et al., 2020). Their likely high biomass and DVM behaviour make them potentially important contributors to the active flux of carbon between the epipelagic and mesopelagic layers (Hopkins et al., 1996; Davison et al., 2013; Hudson et al., 2014; Ariza et al., 2015; Hernández-León et al., 2019).
Mesopelagic fish are widely distributed in all oceans, with vertical ranges varying across basins, resulting in biogeographic regions (Proud et al., 2017; Sutton et al., 2017; Reygondeau et al., 2018) that partially map on to Longhurst’s shallower biogeography (Longhurst, 2007). However, mesopelagic ecoregion boundaries are less pronounced than surface boundaries (Vecchione et al., 2015) and in some cases may be highly dynamic (Ramírez-Llodra et al., 2010; Sutton et al., 2017; Reygondeau et al., 2018). While the number of mesopelagic fish species is large (>400 species reported in the North Atlantic Ocean (Whitehead et al., 1984; Merrett, 1994)), this group is mostly comprised of a few and very specious orders, mainly Stomiiformes and Myctophiformes, but also Argentiniformes and Aulopiformes are common components of mesopelagic populations (Badcock and Merrett, 1976; Whitehead et al., 1984; Ross et al., 2010). Some species of Alepocephaliformes and Beryciphormes, and few Anguilliformes also are among the largest mesopelagic fish (Whitehead et al., 1984; Carpenter and de Angelis, 2016; Sutton et al., 2020).
Research on the mesopelagic region has been typically addressed using trawls through the water column or at particular depth strata (to extract organisms) and ship-based acoustics (e.g., hull-mounted echosounders) to estimate their biomass. Both methods suffer from depth-related biases: fish escapement (Olivar et al., 2017) and net avoidance (Kaartvedt et al., 2012), and the similar acoustic signal from mesopelagic fish and other organisms (e.g., siphonophores), make it difficult to partition the received signal (Proud et al., 2019). This uncertainty coupled with the relatively large effort needed to sample the deep ocean, has resulted in midwater biodiversity drastically under-represented (Webb et al., 2010), which implies a ‘dark hole’ in our understanding of mesopelagic ecosystems (St. John et al., 2016).
Studies from oceanic expeditions during the last century have provided comprehensive reference guides for species identification (Hulley, 1981; Whitehead et al., 1984; Carpenter and de Angelis, 2016; Sutton et al., 2020). This information has been bolstered by more recent phylogenetic analyses based on DNA sequences, which have identified more species and new lineages (Poulsen et al., 2013; Denton and Adams, 2015; Rees et al., 2020). In terms of biogeographic distribution and vertical patterns, there is also a large body of literature, in particular for the Mediterranean and northeast Atlantic (Goodyear et al., 1972; Badcock and Merrett, 1976; Hulley, 1981; Roe and Badcock, 1984; Fock et al., 2004; Sutton et al., 2008; Olivar et al., 2012; Olivar et al., 2017), and a few more from the “Malaspina-2010” circumnavigation expedition (Klevjer et al., 2016; Olivar et al., 2016).
This study is part of the EU H2020-SUMMER project, which is focused on improving our understanding of mesopelagic ecosystems (i.e. studying species diversity, distribution, abundance and regional variability) before any large-scale commercial extraction takes place. The present investigation was carried out in the western Mediterranean and Atlantic waters around the Iberian Peninsula. The two areas have different hydrographic features and productivity regimes. The study encompassed open-sea waters from the oligotrophic western Mediterranean, to the productive western Iberian Peninsula, located on the northern margin of the Canary Upwelling (Sousa et al., 2017). The Mediterranean Sea (MS) is a semi-enclosed sea with the only connection to the ocean through the Gibraltar Strait (width ~13 km, sill depth ~300 m), which plays an important role in the renewal of Mediterranean waters. The MS is a concentration basin (freshwater loss exceeds freshwater inputs) which forces an anti-estuarine circulation, with a deep outflow of salty and nutrient-rich Mediterranean waters towards the Atlantic Ocean and a compensating surface and subsurface entrance of the fresher, well oxygenated and nutrient-poor Atlantic water (AW) through the Gibraltar strait (Armi and Farmer, 1988; Siokou-Frangou et al., 2010). This water exchange results in a net nutrient export from MS. Along its passage through the Mediterranean, the signature of AW is modified transforming into the more saline and less oxygenated modified Atlantic Water. From a dynamic viewpoint, the inflow of this water into the MS basin favours a system of high energy anticyclonic structures in the Alboran Sea, resulting in anticyclonic eddies along the Algerian current path (Millot, 2005).
Previous investigations have shown that mesopelagic fish populations in the western Mediterranean and northeastern Atlantic are composed of a mixture of diel vertical migrant and non-migrant species (Goodyear et al., 1972; Badcock and Merrett, 1976; Hulley, 1981; Roe and Badcock, 1984; Fock et al., 2004; Sutton et al., 2008; Olivar et al., 2012; Olivar et al., 2017) and that active carbon flux funnelled by the migratory micronektonic organisms sharply responds to increases in productivity (Hernández-León et al., 2019). In this study we aim to compare the diversity and diel vertical distribution patterns of mesopelagic fish between contrasting environmental zones in the Mediterranean and Atlantic margins of the Iberian Peninsula and relate them to the different hydrographic structures and productivity levels of both regions.
Materials and methods
Study area and period
The cruise took place from 29 September to 20 October 2020 on board the RV Sarmiento de Gamboa in the Mediterranean and Atlantic waters around the Iberian Peninsula (Figure 1A), where five zones were sampled repeatedly throughout the day and night: Balears (Emile Beaudot Escarpment in the western Mediterranean Sea), Alboran (western Alboran Sea), Cadiz (Gulf of Cadiz), Lisboa (off Lisbon) and Galicia (off the northwest of the Iberian Peninsula) (Figure 1A). Between 48 and 60 hours were spent at each study zone, where repeated stations were performed to sample the community for at least two consecutive days using a variety of instruments both during the day and night. Nevertheless, in the Galicia zone, the rough sea conditions restricted the work to only one day and one night station (Table 1). At each study zone, and according to their specific sunset and sunrise time, the schedule of operations was set beforehand to perform net hauls both in complete darkness and under daylight conditions.
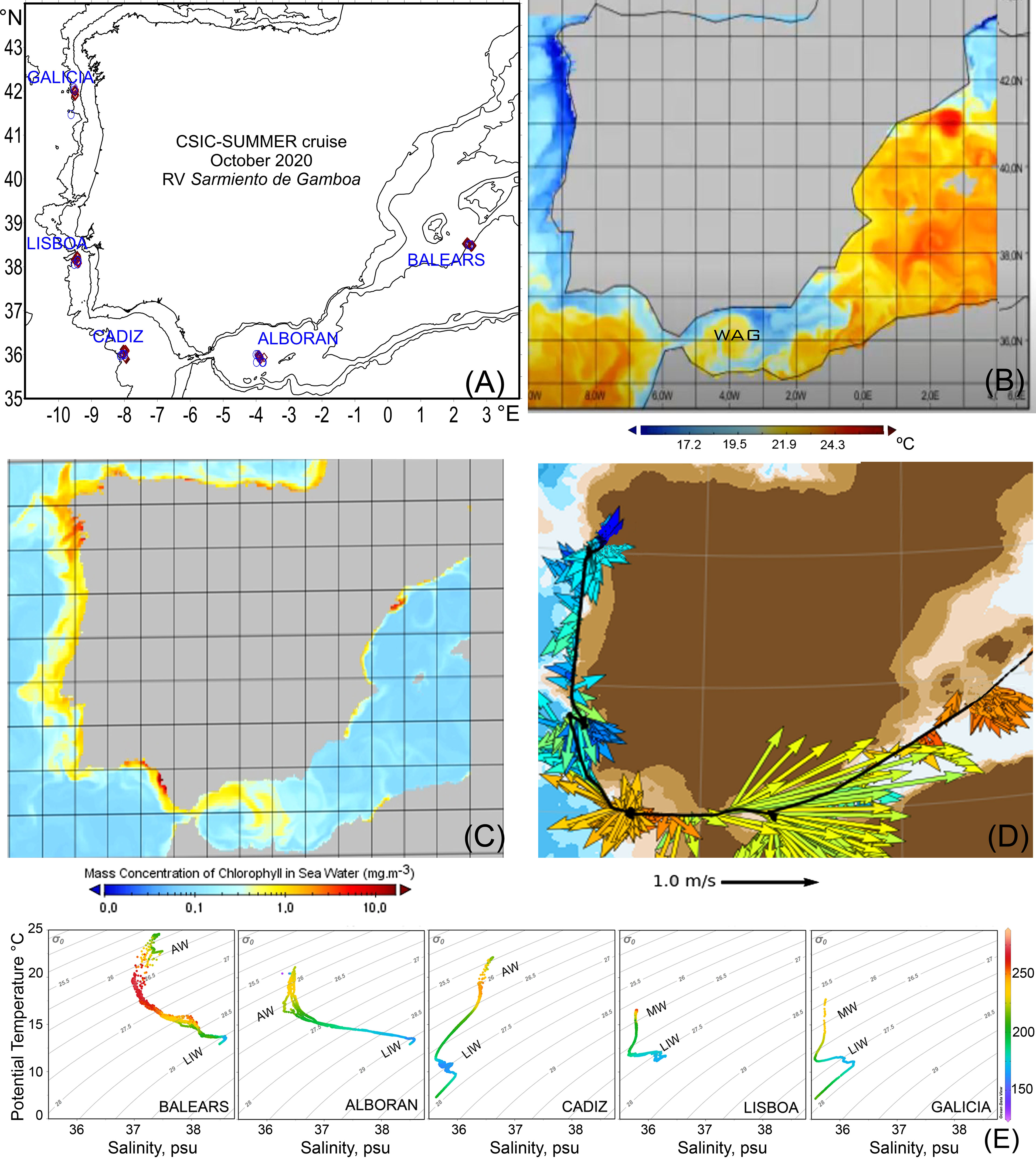
Figure 1 (A) Study region, showing the five studied zones. Red diamonds indicate the repeated sampling stations in each zone. (B) Sea Surface Temperature (°C), (C) Chlorophyll-a (mg/m3) for 10/10/2020 as inferred from the Mercator Global Ocean forecasting system and (D) Surface current from SADCP. (E) Temperature - Salinity diagrams and water masses in the five studied zones along the cruise. AW, Atlantic Water; LIW, Levantine Intermediate Water; MW, Mediterranean Water. Colour scale denotes oxygen concentrations in µmol/kg. WAG, Western anticyclonic gyre. The name of each study zone follows the local naming.
The study started in the western Mediterranean Sea, an oligotrophic basin and continued sampling in the relatively more productive Alboran Sea (Yebra et al., 2018), crossing the Gibraltar strait into the Gulf of Cadiz, and visiting two zones of the more productive upwelling off western Iberian Peninsula, where the main upwelling occurs from spring to early autumn (Sousa et al., 2017).
Hydrographic data
The water masses and vertical structure of the water column were characterised using measurements obtained with a SBE 911 Plus CTD and a carrousel of 24 Niskin bottles of 12 L each. The temperature, conductivity and pressure sensors had an accuracy of 0.001, 0.0003 and 0.0015%, respectively, and were calibrated <8 months prior to the cruise. The most recent manufacturer calibration of the oxygen sensor, with accuracy of 0.89 µMol/kg, took place three months prior to the cruise. Water samples to calibrate the fluorometer were collected at multiple depths layers of the water column (5, 50, 100, 150 and 200 m) in the different studied zones. The current velocity, magnitude and direction in the upper 600 m were obtained with the Shipboard Acoustic Doppler Current Profiler Ocean Surveyor OS75 (SADCP). The software used to process the SADCP was CODAS (Firing and Hummon, 2010). Repeated CTD casts (0 – 1000 m) were performed at each study zone. One of the CTD casts performed at each zone had a maximum depth close to the bottom. The CTD and SADCP data were processed in quasi-real time using the manufacturers’ software and visualized with Ocean Data View Software (Schlitzer, 2016).
Fishing gear and sampling method
The scientific midwater trawl Mesopelagos (Meillat, 2012) equipped with a Multisampler (7 discrete collectors) was used during the cruise. This gear works with a single traction cable and no doors. It uses kites on its wings as well as floats on the headline and 120 kg ballast on the footrope to open the mouth. The total length of the net is 58 m, and it consists of a graded-mesh netting of 30 mm near the mouth and 4 mm in its lower part. A MARPORT system was used to assess the mouth aperture (estimated between 20 and 30 m2), and a Scanmar acoustic communication system was used to control net depth. The Multisampler was equipped with a depth sensor and a motor that turns a carrousel plate containing the cod-ends, which enabled 7 different pre-programmed depths horizons to be sampled. A total of 17 deployments were made (between 0 and 700 m), of which 14 sampled discrete depth layers. On three occasions the Multisampler system failed, and hence it was not possible to determine catch depth (all samples were mixed). Hauls were oblique and the following layers were sampled: 700-600 m (1), 600-500 m (2), 500-400 m (3), 400-300 m (4), 300-200 m (5), 200-100 m (6), 100-0 m (7). Additionally, to more accurately sample the epipelagic layers and to obtain information to control any bias of contamination from lower layers, we performed 5 night hauls restricted to the first 210 m of the water column, where we sampled every 30 m. During the hauls, ship speed was maintained at ca. 2 knots and the ship’s course remained constant. Winch retrieval was generally 20 m/min, except when large quantities of gelatinous plankton were expected, where the speed was 30 m/min to prevent net clogging. Detailed information for each haul location (latitude, longitude and depth), date and time and estimated volume of water filtered is given in Olivar et al. (2022).
Sample processing
Fish identifications, counts and measurements were carried out on board. However, many specimens were stored (frozen at either -80 or -20°C), for later examination in the laboratory, or for additional analyses (e.g. lipids, isotope, gut content, ETS or DNA). Fish standard lengths (SL) were measured to the nearest mm. Wet weights (WW) of groups of fish of the same species were measured using a marine precision balance POLS S-182 P-15 (precision 2 g). Single/small fish were weighed with a PESOLA Lightline dynamometer (precision 0.1 g).
Acoustic sampling
Acoustic data were collected underway and on station with a hull-mounted Simrad EK80 scientific echosounder with 4-sector split-beam transducers operating at 18, 38, 70, 120 and 200 kHz (Yang et al., 2021). The EK80 transducers had a typical beam width of 7˚, except for the 18 kHz, which has a beam width of 11˚. A Simard WBAT (Wideband Autonomous Transceiver) was deployed on station (i.e. when vessel was stationary) down to 500 m to make close up acoustic observations of the scattering layers in the five zones. The WBAT system consisted of 4 or 3-sector split beam wideband transducers with a frequency band ranging from 35 to 445 kHz. Both the EK80 and WBAT acoustic systems were calibrated using a Tungsten sphere with a diameter of 38.1 mm, following a standard approach (Demer et al., 2015). Volume backscattering strength (Sv, mean echo energy per m3) from the surface down to a depth of 1000 m (observed from the hull-mounted EK80) was calculated at each zone and grouped by day and night periods based on local sunrise/sunset times to study DVM. Raw echosounder data were pre-processed using Echoview software v 12.1 (Echoview Software Pty Ltd, 2021), including correcting TVG-amplified background noise (de Robertis and Higginbottom, 2007) and removing surface noise (aeration/near-field), impulse noise and transient noise (Ryan et al., 2015).
Data analyses
The volume of water filtered per net was calculated using the mean mouth area of 30 m2, and the distance travelled during each haul. Comparisons of fish abundances between stations and zones were carried out based on integrated abundances between 700 and 0 m (total number of fish x 700 m divided by the total volume of water filtered, i.e., abundances standardized by unit of surface). Integrated abundance through the water column was not significantly different between day and night, therefore we combined day and night stations by zones. Fish abundances by stratified hauls at different layers of the water column were standardized using the volume of water filtered in each sampled layer.
Patterns of species abundances throughout the water column (0-700 m) were first compared between zones employing abundance k-dominance plots that were constructed as cumulative relative abundances vs species ranked in order of importance in terms of the number of individuals. Then species were ranked in terms of biomass, and both abundance and biomass curves were represented on the same plot (Clarke and Gorley, 2015). Whether k-dominance curves are plotted from the species abundance distribution or from species biomass values, the y-axis is always scaled in the same range (0 to 100). This facilitates community structure comparisons in terms of abundance and biomass.
Taking into account that there were no significant differences (p=0.95) among mean volumes of water filtered through the water column by zones (mean= 160780.3 m3 SD= 71658.9 m3), diversity was then analysed using quantitative indices: number of species, Shannon-Wiener diversity index (H’) and Simpson equitability index (1-λ). We also calculated a measure of taxonomic diversity based on presence/absence: average taxonomic distinctness (AvTD or D+) (Clarke and Warwick, 2001). ANOSIM was applied to test significant differences in abundance and diversity among zones. These analyses were performed using the software package PRIMER 7.
Community patterns through the study region were investigated using non-metric multi-dimensional scaling (nMDS) and cluster analysis within the software package PRIMER 7 (Clarke et al., 2014; Clarke and Gorley, 2015). Fish abundance integrated for the 0-700 m was log-transformed to reduce the effect of dominant species. Species that appeared in less than three stations and represented less than 0.1% of abundance were eliminated from these analyses. A Bray-Curtis similarity matrix was calculated and cluster analysis was carried out using group average similarities. The SIMPROF procedure was used to identify the significant groups of samples (Clarke et al., 2008), and the similarity percentage (SIMPER) routine was used to determine the species contributions within each cluster group.
To enable exploration of fish abundances in relation to environmental conditions a matrix of temperature, salinity, oxygen and Chlorophyll a (at surface and in the strata 400-500 m, and integrated Chlorophyll a in the upper 200 m) was built. The relationships between the fish assemblages and environmental variables were examined using distance-based linear models (DistLM), using the step-wise selection procedure with R2 as the selection criterion. The variables correlated (R2 > 0.8) were removed from the analyses. We finally used SST (correlated to surface salinity and oxygen), the temperature in the 400-500 m (450T) (correlated to salinity and oxygen in this stratum), the surface Chlorophyll a (SC), and the Chlorophyll a integrated in the first 200 m (Int_C).
Diel and depth–dependent variation was examined by grouping taxa abundances by layer and differentiating day and night conditions. A nested PERMANOVA was used to test significant differences between zones, depth layers and light conditions. For all taxa the weighted mean depth was calculated as:
where Zi is the depth of the ith stratified haul (the centre-point of each sampled interval), and Pi is the proportion of fish at that depth (Fortier and Leggett, 1983).
Results
Oceanographic conditions
Sea surface temperature (SST) was higher in the Mediterranean and Gulf of Cádiz than off Lisboa and Galicia (Figure 1B). Chlorophyll-a satellite images showed the lowest values in Balears and Gulf of Cadiz zones and the highest off Lisboa and Galicia (Figure 1C). The Ѳ,S-curves and currents fields showed the entrance of surface Atlantic water into the western Mediterranean through the Gibraltar Strait and below them the outflowing of Levantine Intermediate Water, which becomes transformed into Mediterranean Water mass in the Atlantic (Figures 1D, E). Surface current fields were around 0.2 m/s except in the Alboran Sea where they reached high values of 1 m/s, with an eastward and east-southeast direction that is coherent with the dynamics of the intensive currents that originate an anticyclonic gyre, the Western Alboran Gyre (WAG) (Figure 1D), also evident from SST and Chlorophyll a images (Figures 1B, C).
The vertical structure of the water column (Figure 2) in the Mediterranean was characterized by intense thermal and salinity gradients between surface and 150 m, while mesopelagic layers were almost homogeneous (temperature ca. 13.5°C and salinity ca. 38.5, below 200 m). Chlorophyll a profiles showed a Deep Chlorophyll Maximum (DCM) below the thermocline (at 74-86 m in Balears and 30-48 m in Alboran). Associated with DCM the highest dissolved oxygen was detected. In the Atlantic, the water column was characterized by lower stratification, and temperatures and salinities in the mesopelagic layers reached lower values (<11°C and <36, respectively) than in the Mediterranean. The Cadiz zone still displays certain stratification, with a DCM at 77-90 m, below the thermocline. Temperature and salinity profiles off Lisboa and Galicia were fairly homogeneous, with high Chlorophyll a in the first 17 m in Lisboa and at 40 m in Galicia. Lisboan Chlorophyll a concentrations were by far the highest for the entire survey.
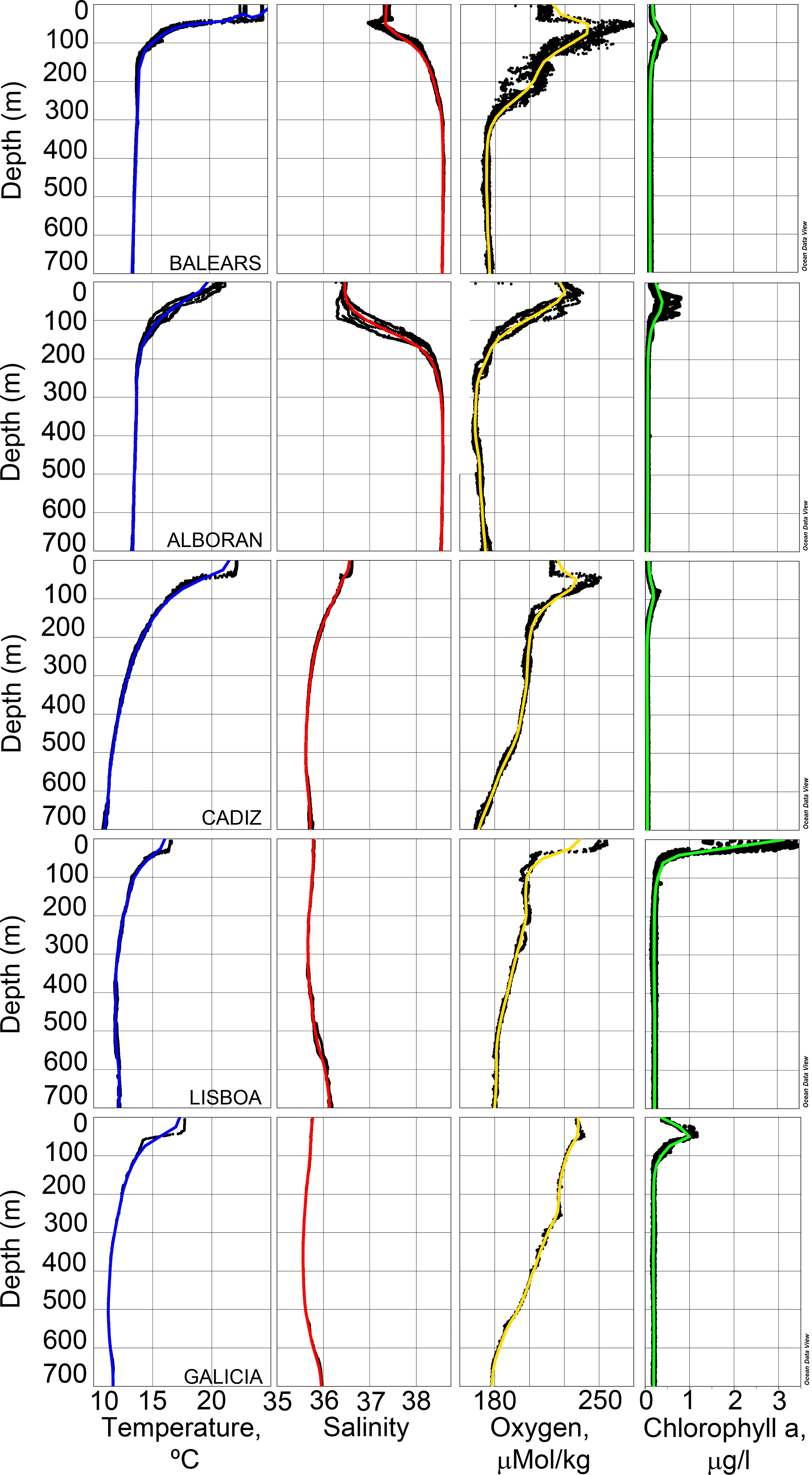
Figure 2 Vertical profiles of temperature, salinity, dissolved oxygen, and Chlorophyll a at the five studied zones (from top to down: Balears, Alboran, Cadiz, Lisboa and Galicia).
Integrated abundance and taxa account
Most of the fish specimens collected with the Mesopelagos net were juveniles and adults, and a few were transformation stages. The size range varied from fish in transformation stages of 10-20 mm to a couple of long mesopelagic anguilliforms of ca. 600 mm, and the modal size class was 20-30 mm. Individual biomass ranged from 2 mg to 60 g, although only a few individuals weighed more than 5 g, and the modal size class was ca. 1 g.
In general, the stations carried out in the Mediterranean zones had higher numerical abundance than those from the Atlantic, but biomasses (wet weight) were similar or lower in the Mediterranean (Figure 3). Nevertheless, the mean abundance of the station by zone was only significantly different between Balears (higher) and Cadiz zones (ANOSIM R=0.82, p<0.03), and no significant differences in biomass were observed among zones, although Lisboa showed higher mean biomass. The two stations sampled off Galicia presented the lowest abundances. Finally, the six night stations covering only the epipelagic layers (not used in statistical comparisons) rendered overall lower catches in number and biomass, although the total number of taxa was fairly similar to that of hauls covering the 700 m (Figure 3).
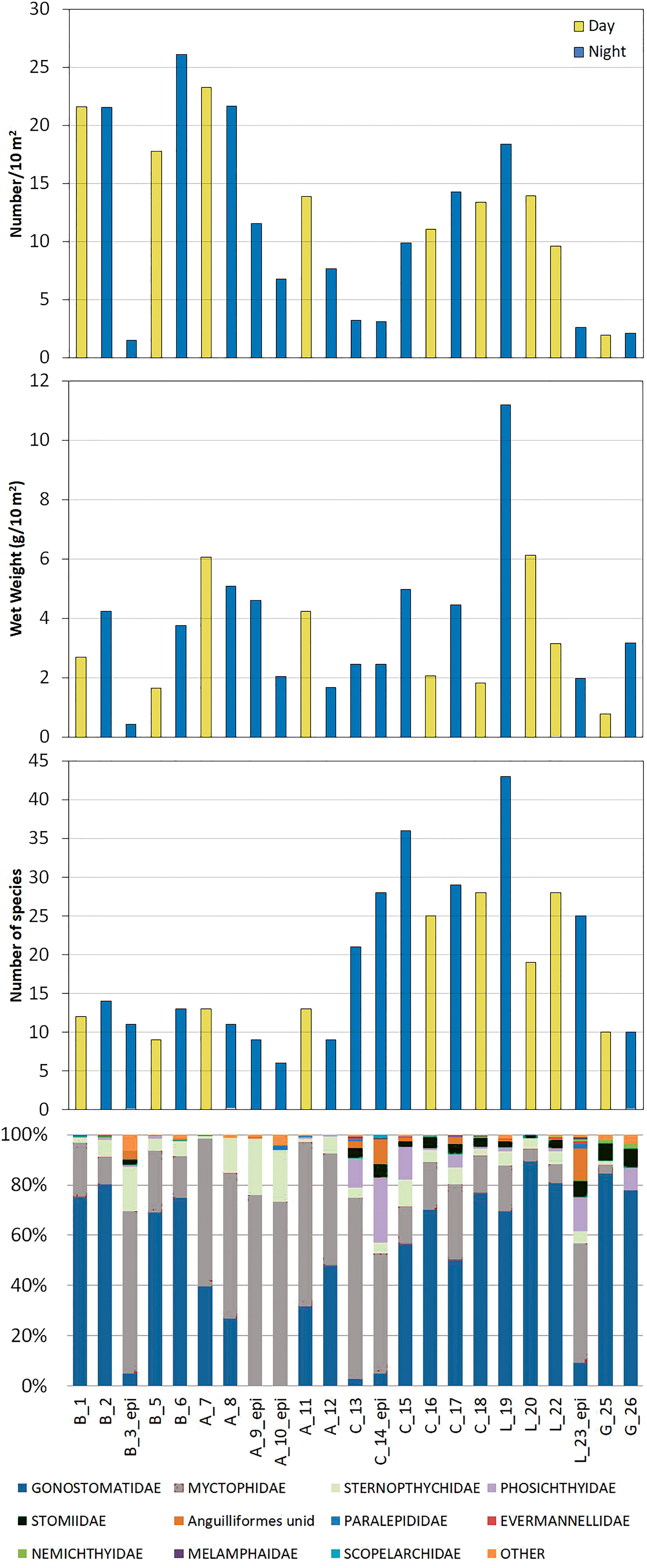
Figure 3 Abundance (number/10 m2), biomass (wet weight g/10 m2), number of taxa and relative contribution of different families in the different sampled stations from 0-700 m, or from 0-210 m in case of epipelagic night stations (epi). The first letter of each station label indicates the zone of study (B: Balears, A: Alboran, C: Cadiz, L: Lisboa and G: Galicia), followed by station number and the light condition (D: day and N: night).
Fish of the orders Stomiiformes (bristlemouths, lightfishes and dragonfishes) and Myctophiformes (lanternfishes) dominated the samples (66.9 and 32.3%, respectively). In the Mediterranean 10 species of myctophiforms and 6 stomiiforms were identified, these two orders constituted >90% of hauls composition. In the Atlantic myctophiforms and stomiiforms were represented by 29 and 30 species, respectively, while other orders such as Alepocephaliformes, Argentiniformes, Aulopiformes, Beryciformes and Perciformes also showed a moderate contribution (Figure 3).
Among Stomiiformes, the family Gonostomatidae was dominant, mainly due to several species of the genus Cyclothone (C. braueri and C. pygmaea in the Mediterranean and C. acclinidens, C. braueri, C. livida, C. microdon and C. pseudopallida in the Atlantic), but also by the frequent occurrence of Sigmops elongatus (in 75% of the Atlantic stations) (Table 2). Family Stomiidae was represented by species of the subfamilies Astronesthiinae, Chauliodontinae, Idiacanthinae, Malacosteinae, Melanostomiinae and Stomiinae, with the Chauliodontinae, Chauliodus sloane and C. danae the most abundant and frequent. Sternoptychidae were represented by Argyropelecus hemigymnus and Maurolicus muelleri, in the Mediterranean, as well as by A. sladeni, A. aculeatus, A. gigas and Sternoptyx diaphana, Sternoptyx sp. and Valenciennellus tripunctulatus in the Atlantic. Finally, Phosichthydae were represented by Vinciguerria atenuatta in the Mediterranean and V. attenuata, V. nimbaria and V. poweriae in the Atlantic (Table 2).
Diversity patterns
A total of 70 different species was recorded during the whole cruise. The total number of species caught in the Mediterranean Sea (22) was lower than in the Atlantic (67). The species accumulation curve was flatter for the Mediterranean than for the Atlantic samples (Supplementary Figure 1), indicating that more species inhabit the Atlantic than those recorded. Both, the observed and the modelled number of taxa approached an asymptote (although still rising) at the last stations. There were no differences in species richness between the two Mediterranean zones or between Cadiz and Lisboa, which had significantly higher species richness than the Mediterranean zones. The highest numbers of species were found at night at Cadiz and off Lisboa in the surface layer. However, in the Galicia zone the number of species found was significantly lower. Interestingly, the number of species collected at night stations covering just the epipelagic layers was fairly similar to those covering the entire 700 m of the water column (Figure 3).
Cumulative ranked species abundances showed the most elevated curve, indicating the lowest diversity and the highest dominance, for Balears and Galicia, with 2 species accumulating >75% of abundance (Figure 4A). The lowest curve was for Cadiz, revealing the highest diversity. The steepest curve corresponds to Alboran, with the first abundant species contributing ca.40%, of total numbers, but reaching the 75% with 4 species. Cadiz and Lisboa showed the flattest curves. They have a single dominant species, and a more even contribution of the next rank species. For the combined dominance abundance and biomass plots (AB) (Figures 4B–F) it was observed that the abundance curves tended to be depicted on top of those of biomass, although they were closer to each other for the Alboran and Galician samples.
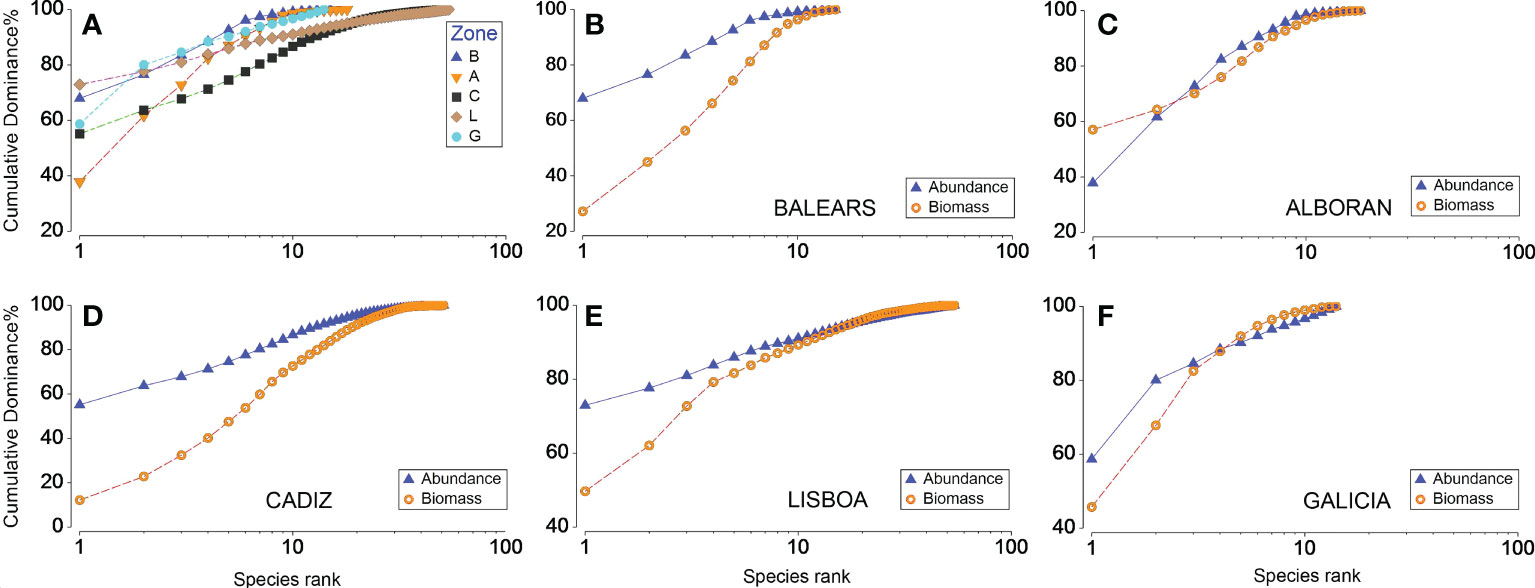
Figure 4 (A) Abundance k-dominance plots by zone (B: Balears, A: Alboran, C: Cadiz, L: Lisboa, G: Galicia), and (B–F) combined abundance and biomass dominance plots for the five zones. The species are ranked in order of importance in terms of abundance, or biomass, on the x-axis. The y-axes indicate cumulative percentage dominance.
In the five zones small fish (25-40 mm) dominated. Both for Myctophiformes and the Stomiiformes the larger size classes were better represented in the Atlantic than in the Mediterranean (Supplementary Figure 2).
In terms of diversity indices (Figure 5), H’ Shannon index showed a progressive increase from Balears to Cadiz, followed by a decrease in Lisboa and Galicia. Despite of the same number of species and similar abundance between Balears and Alboran samples, H’ diversity and Simpson Equitability were significantly higher in Alboran (ANOSIM R=0.9, p<0.03). Although abundance and number of species were not significantly different between Cadiz and Lisboa, H’ and Simpson were lower in Lisboa (although only significant at 17 and 11%, respectively). The Average Taxonomic Distinctness (AvTD) also reflected the pattern of lower diversity in Balears and Alboran than in the Atlantic zones, but differences were only significant between Balears and Cadiz (ANOSIM R=0.93, p<0.03).
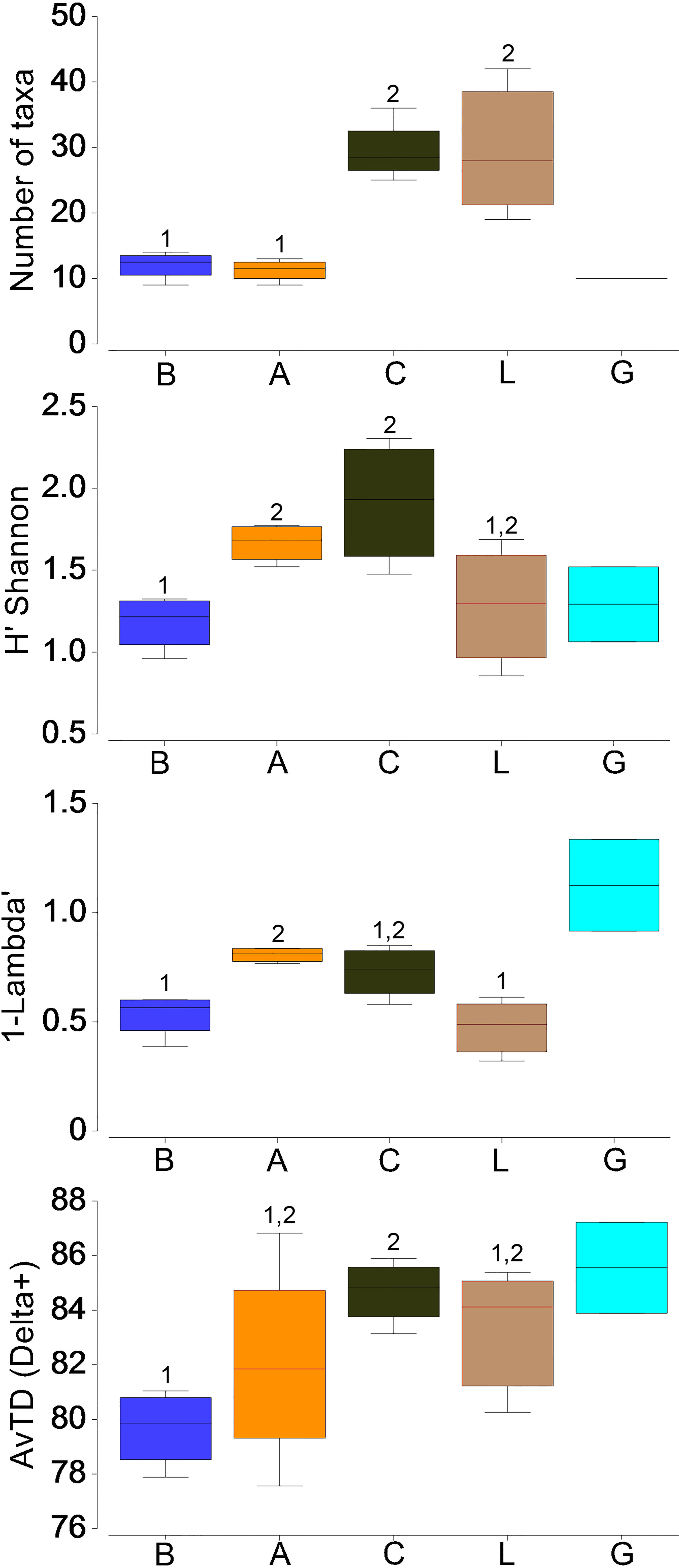
Figure 5 Diversity patterns by zones from stations covering from 0 to 700 m with the Mesopelagos net. Number of taxa, H’ Shannon-Wiener diversity index, 1-Lambda: Simpson Equitability index and AvTD (Delta+) index (Average Taxonomic Distinctness). (B: Balears, A: Alboran, C: Cadiz, L: Lisboa, G: Galicia). Different numbers indicate significant differences in mean values means (ANOSIM, p < 0.05).
Community differences by zones
Both the MDS plots and the cluster analysis revealed a strong and consistent pattern, differentiating the stations from the Mediterranean and Atlantic (Figures 6A, B). SIMPROF test proved significant differences between Mediterranean and Atlantic, and also within the three Atlantic zones, but not between Balears and Alboran. SIMPER test showed that the main difference in the fish species composition between Mediterranean and Atlantic samples was due to B. glaciale, C. pygmaea and C. maderensis, contributing up to 77.63% in the Mediterranean samples, but absent in the Atlantic. Conversely C. microdon/livida, V. tripunctulatus, C. warmingii and B. suborbitale were absent in the Mediterranean, but common in the Atlantic. The two stations from Galicia were separated due to their low species contribution and their lower abundances, with Cyclothone spp constituting up to 86.64% of all species caught. Lisboa and Cadiz zones had similar species composition, but with different contributions: B. suborbital, V. nimbaria, L. gaussi, and C. danae composed 15.3% of the species composition in Cadiz and were rare in Lisboa, and M. punctatum and S. elongatus were more abundant and frequent in Lisboa.
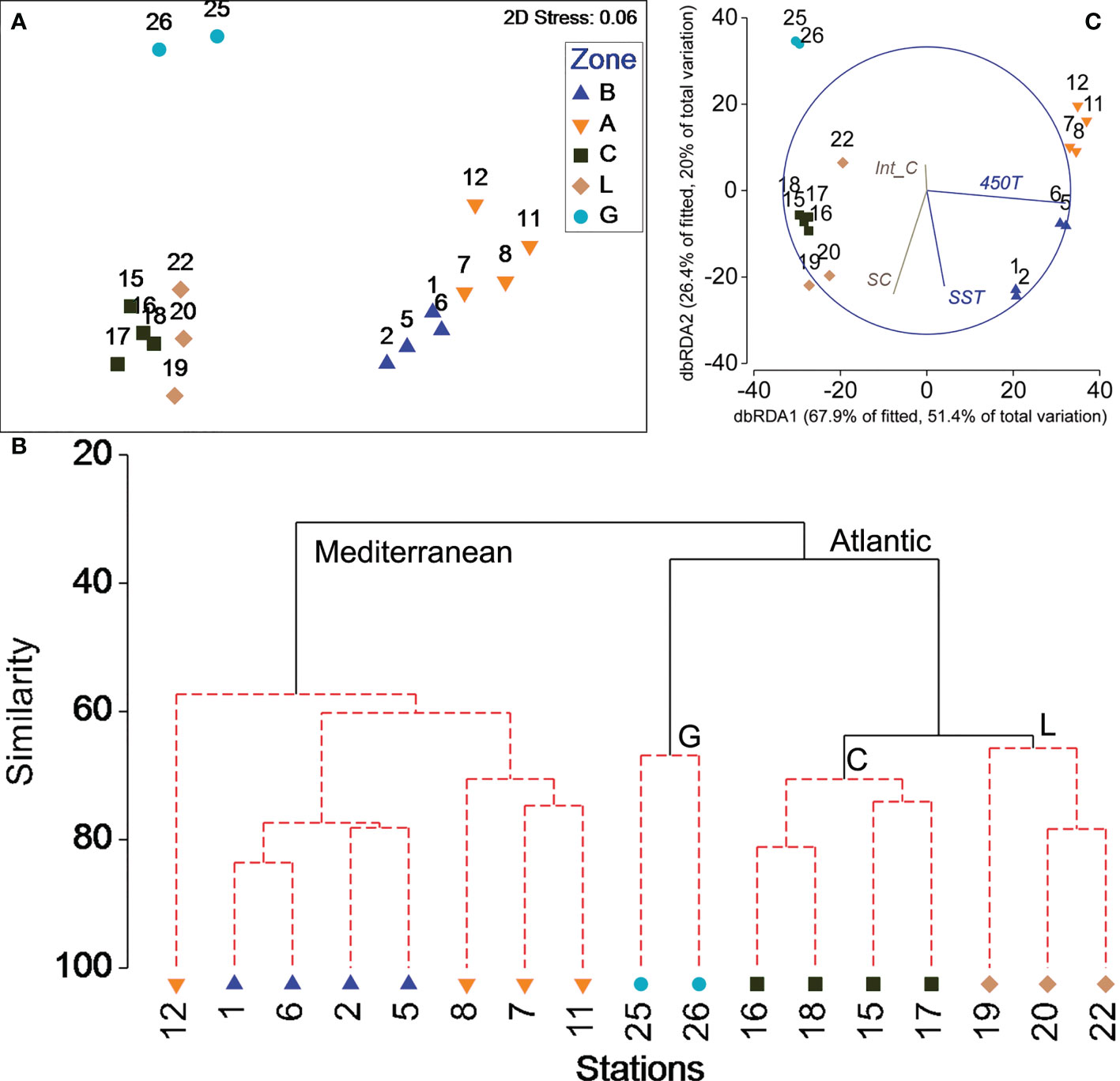
Figure 6 Assemblage structure for the 17 stations sampled through the 0-700 m of the water column around the Iberian Peninsula. (A) MDS multi-dimensional scaling, (B) Distance-based redundancy bi-plot showing the relationships between mesopelagic fish assemblages and environmental variables (C) Dendrogram of station similarities (continuous black lines denote significant groups of samples defined by the SIMPROF test). Zone labels: B: Balears, A: Alboran, C: Cadiz, L: Lisboa, G: Galicia). 450T: Temperature at 450 m. SC, Surface Chlorophyll a. SST, Sea Surface Temperature. Int_C, Integrated Chlorophyll a in the upper 200 m.
The distance based linear model relating integrated species abundance and environmental factors, sea surface Chlorophyll a and 200 m-integrated Chlorophyll a showed that SST and 450T (correlated to the corresponding salinity variables) were significant in structuring the community, but not the Chlorophyll a ones (Supplementary Table 1). The first axis explained 67.9% of the fitted variation and was positively correlated with temperature, separating the stations from the Balears and Alboran zones (ordered in the positive side of dbRDA graph) from those in the Atlantic zones (Figure 6C).
Acoustic scattering layers
A permanent (day and night) deep scattering layer (DSL) was observed using the 38 kHz echosounder between 400 and 500 m depth in all the zones except for Lisboa, where the DSL was 100 m shallower (Figure 7). Backscattering intensity in the layer was relatively high in the Balears, Alboran and Lisboa zones, and generally increased at the surface at night-time due to DVM. DVM was more prominent in the 18 kHz echosounder observations (Supplementary Figure 3).
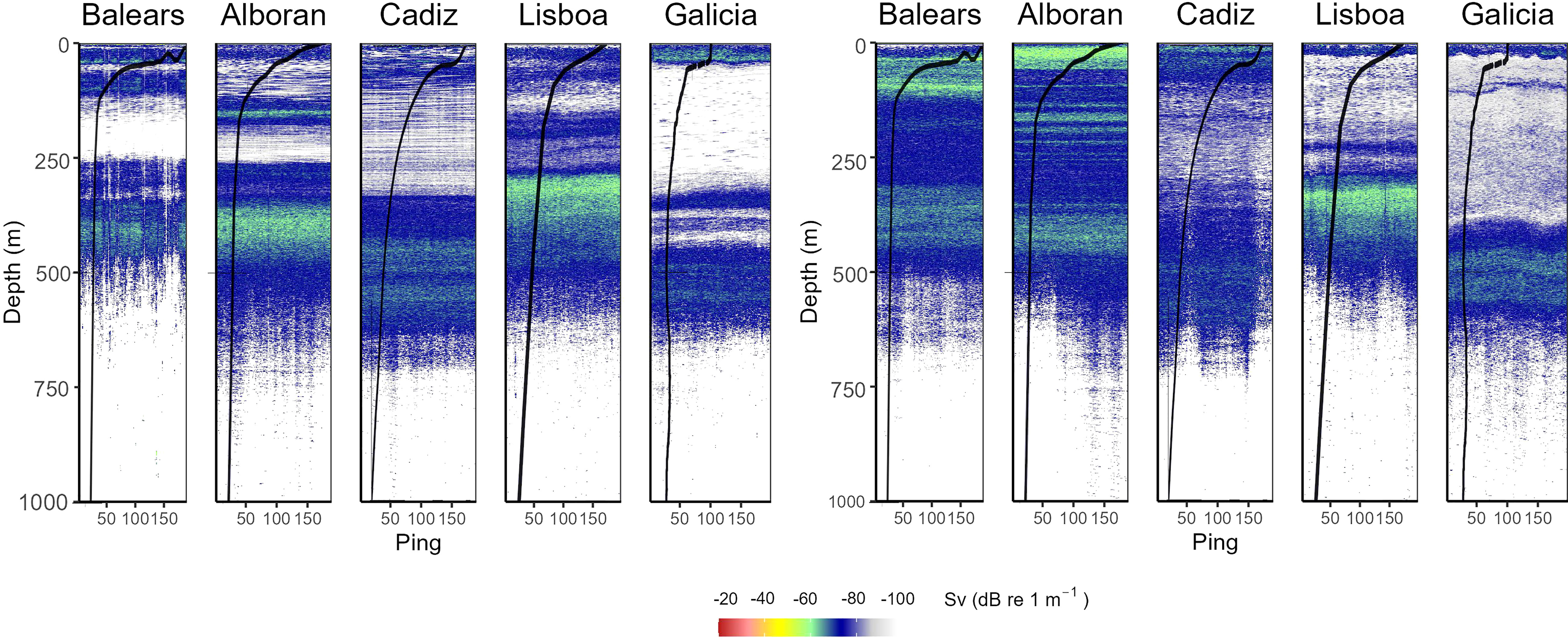
Figure 7 Typical zonal scattering layer distributions observed using the hull-mounted 38 kHz echosounder. The colour scale is echo intensity (mean volume backscattering strength, Sv, dB re 1 m-1). Left panels are daytime observations, and right panels are night-time. Ping repetition interval was 2 seconds. At the c. 2.5 knots vessel speed that these observations were made (between net hauls), the 200 pings shown equate to about 500 m distance travelled. The overlaid black curves represent the temperature profiles for simultaneous CTD casts (as shown in Figure 2).
Diel depth distribution by taxa
The analyses of community structure considering the seven vertical net sampled layers (700-600 m, 600-500 m, 500-400 m, 400-300 m, 300-200 m, 200-100 m and 100-0 m) showed differences in the fish diversity/composition depending on the zone, layer of the water column, and light (day and night conditions) linked to the level in the water column (Supplementary Table 2). In all the geographical zones the overall fish collections were higher (both in number and in biomass) in the mesopelagic layers (particularly below 400 m) than near the surface (Supplementary Figure 4).
The major differences between the day and night vertical location of the mesopelagic fish were observed for species of the families Myctophidae, Stomiidae and Phosichthyidae (Figure 8) that migrate from the mesopelagic habitat at daytime to the epipelagic layers at night. Nevertheless, individuals of the same species were also collected in the mesopelagic zone during the night. Sternoptychidae did not show a clear difference in vertical location, although they were slightly shallower at night than during the day, and presented an overall shallower location in the Mediterranean than in the Atlantic. A common Gonostomatidae found only in the Atlantic was Sigmops elongatus that was caught mostly at night, but never reached the upper 100 m. The gonostomatids of the genus Cyclothone usually occurred below 300 m both day and night, with the dark species found deeper than the light coloured ones.
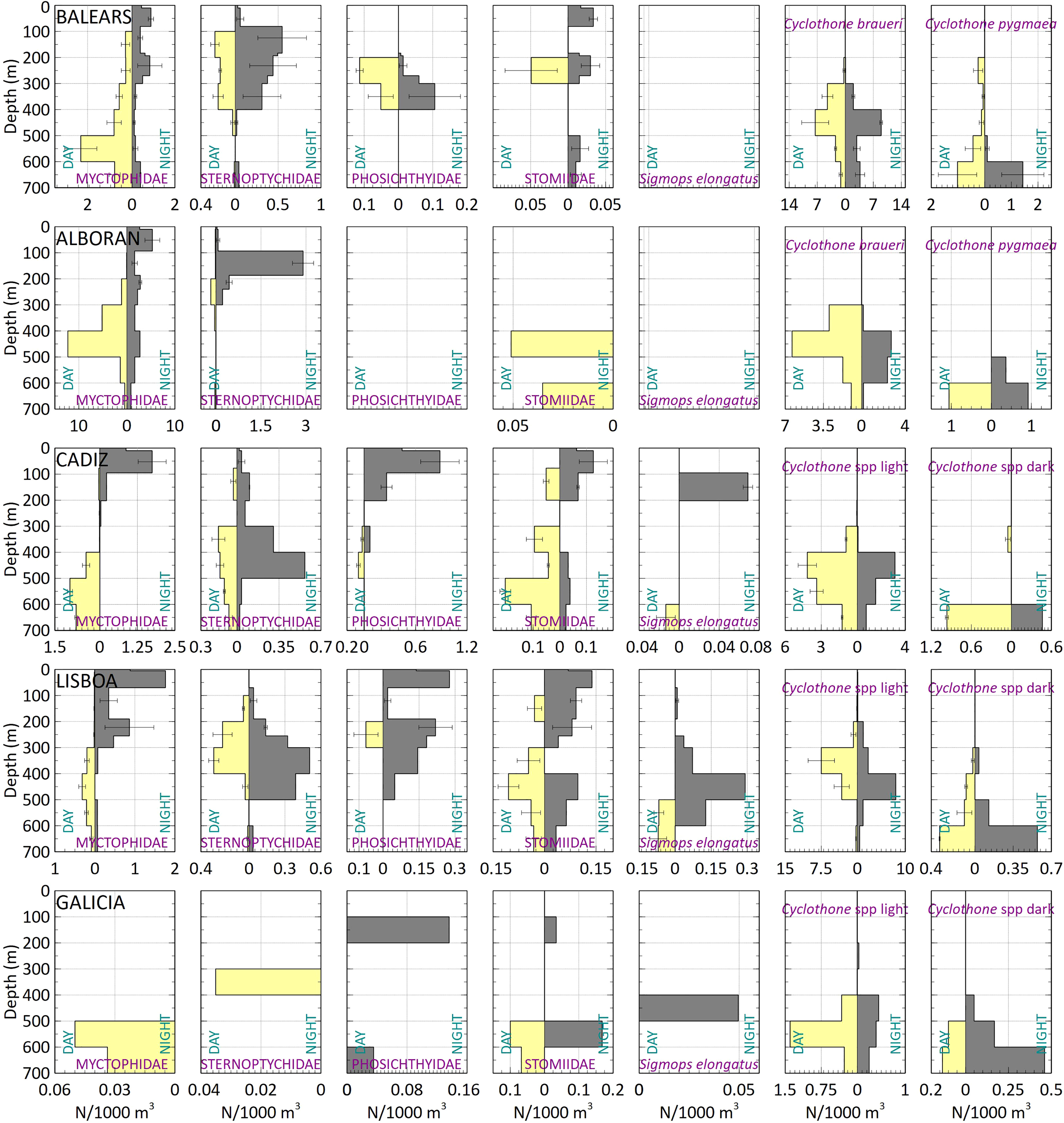
Figure 8 Mean vertical distribution patterns for the most abundant fish taxa in the different studied zones. From top to bottom: Balears, Alboran, Cadiz, Lisboa and Galicia.
Night samples restricted to the epipelagic layers (every 30 m in the upper 210 m) showed a close relationship between the depths of maximum Chlorophyll a and the main concentration of migrating fish. In Balears, Alboran and Cadiz, where a DCM was detected between 74-86 m, 30-48 m and 77-90 m, respectively the highest concentrations of fish were located between 60-90, 30-60 and 30-90 m, respectively. In Lisboa, where maximum Chlorophyll a was detected nearer to the surface, 0-17 m, the highest fish concentrations were found in the upper 30 m (Figure 9).
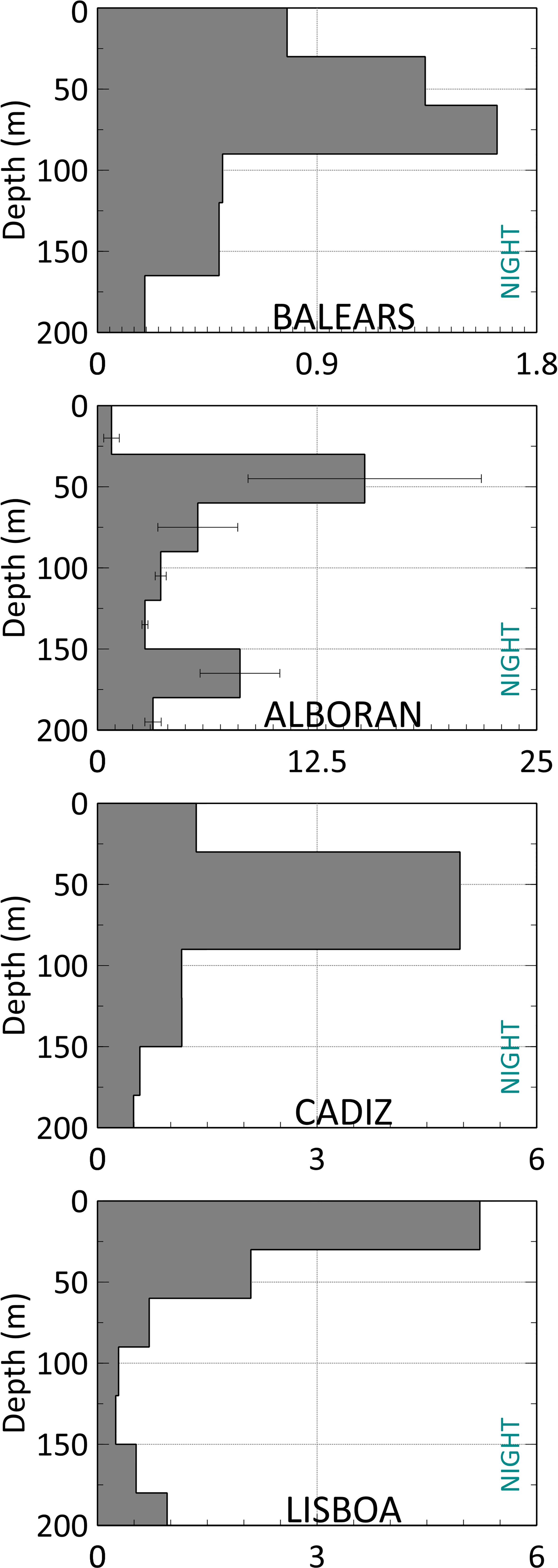
Figure 9 Vertical distribution from epipelagic samples performed with the Mesopelagos net every 30 m at night. From top to bottom: St#3 (Balears), St #9 and #10 (Alboran), St#14 (Cadiz) and St#23 (Lisboa).
Discussion
The present investigation encompasses a large geographical region, with contrasting hydrographic and productivity conditions. The sampling strategy, a survey conducted in a relatively short period (<1 month), using the same sampling methodology and equipment throughout the survey, has allowed comparison of mesopelagic fish diversity, the relative species abundance and their vertical migratory patterns, between the western Mediterranean and the northeast Atlantic.
Oceanography
The study was carried out under contrasting environmental conditions, i.e., during the most oligotrophic period of the western Mediterranean when surface nutrients are depleted after the summer stratification (Salat et al., 2002; Sabatés et al., 2007), and at the end of the Western Iberian Peninsula upwelling period (Fraga, 1981; Álvarez et al., 2012; Sousa et al., 2017). The most productive zone was Lisboa, which was in agreement with the expected upwelling in this region. Although the Mediterranean is an oligotrophic sea, mesoscale structures such as eddies and fronts, which are sites of enhanced nutrient inputs, contributed to increasing biological productivity (Estrada, 1996; Sabatés et al., 2007). In the case of the Alboran Sea, the intense jet from the Gilbraltar Strait (also observed during this cruise) is associated with the formation of the Western Alboran Gyre (WAG) (Millot, 2005), with consequences to the overall productivity in the zone. Our sampling roughly corresponds to the core of the WAG, which tends to concentrate higher zooplankton biomass than at their edges (Yebra et al., 2018). The maximum Chlorophyll a concentrations from the CTDs in the Alboran zone were two orders of magnitude higher those of the Balears.
Sampling constraints
The cumulative species richness curves, for the stations of the Mediterranean and the Atlantic, indicated that with the sampling protocol used (gear and sampled depths) it is unlikely that additional stations will render many more species. Trawl avoidance (for large fish) and mesh extrusions (for thin species) are important shortcomings to assess the biomass of mesopelagic fish populations (Heino et al., 2011; Kaartvedt et al., 2012; Olivar et al., 2017). Our net (with a small mouth and trawled at low speed) underestimates larger fish. However the sampling strategy was the same throughout the entire cruise, therefore diversity patterns can be consistently compared, while absolute values may be different in case different nets were used. One possible problem with trawling systems such as the present one (a single net and the individual cod-ends placed at the end) is contamination of samples from successive layers. Species with large jaws, long teeth or large fins are often entangled in the meshes, and may eventually be detached and fall into a cod-end of a layer that is not the one where they came from during the sampling. The night hauls restricted to the epipelagic layers were used to test for possible contamination of deeper samples from mesopelagic layers.
Whenever possible, identifications were carried out to the species level. When specimens were too damaged or when they were too small to confidently perform species identification using adult dichotomous keys, we used higher taxonomic levels. This was generally the case for Cyclothone spp. The two Mediterranean species, C. braueri and C. pygmaea, could be easily identified (Badcock, 1984). However, a larger number of Cyclothone species are reported for the Atlantic and their species identification relies on several characteristics difficult to examine (Badcock, 1984) in the easily damaged specimens. For the present study, the Atlantic Cyclothone species were grouped into two groups: C. braueri and C. pseudopallida as Cyclothone spp-light, and C. acclinidens, C. livida, C. microdon and C. pallida as Cyclothone spp-dark.
Data of the Galician zone came from a single day and night station, carried out under very rough sea conditions, therefore they are less representative of the fish community than those from the other zones.
Integrated abundance and biomass comparison among zones
The overall abundances or biomass of mesopelagic fish through the water column were not significantly different among zones, despite the conspicuous differences in Chlorophyll a values. In Lisboa, with Chlolorphyl-a values two orders of magnitude higher than in the other zones, integrated biomass was higher (but not significantly) than in the other zones. Comparison between the Balears zone, the most oligotrophic, and the relatively productive Alboran sea, also show a tendency for higher biomass in the productive zone, but not significant.
The fact that in the combined AB plots the abundance curves were above those of biomass points to a community numerically dominated by a few species with low biomass or at the juvenile stages. The gear we used (small mouth opening and slow speed) may in part explain the dominance of small fish vs larger ones. Larger double-warp midwater otter trawls tend to collect a higher proportion of larger fishes (Judkins et al., 2017). However, the small mesh sizes of our net allowed for a better representation of small mesopelagic fishes such as bristlemouth of the genus Cyclothone. In all zones, except Alboran, the numerically dominant taxa were the small gonostomatid Cyclothone spp., and most species of the rest of the families were represented by juveniles. The Alboran and Galicia samples had more overlapping AB curves, indicating the contribution of other less abundant taxa with higher individual biomass. In Alboran, the biomass curve started in a higher position than that of abundance, because the dominant species (both in number and biomass) was the myctophid B. glaciale. Although Galician samples were numerically dominated by the small Cyclothone spp., the community was also composed of some larger fish such as Chauliodus spp. and Sigmops elongatus.
An interesting comparison was the overall shorter body size within Myctophiformes and Stomiiformes in the Mediterranean than in the Atlantic, which is a common pattern for other groups of species (Tortonese, 1960; Stefanescu and Cartes, 1992; Massutí et al., 2004). Shorter body sizes together with the absence of large-bodied taxa, such as S. elongatus, explains that despite numerical abundance being higher in the Mediterranean samples, there were no significant differences in biomass. The comparatively lower food resources and higher temperatures at depth in the Mediterranean than in the Atlantic have been suggested as the main factors responsible for Mediterranean species attaining adulthood at smaller sizes (Massutí et al., 2004).
Diversity and community structure
Here we compare diversity as a measure of community structure resulting from the interactions between species composition in each of the studied zones. To this aim, we first analysed the overall composition within the integrated 0-700 m water column, which includes all mesopelagic species that may coincide at any given level at any given time.
In all the studied zones the mesopelagic community was dominated by a few species, particularly in the Balears zone. Biodiversity measures of mesopelagic fish obtained from the present study were higher for the Atlantic than for the Mediterranean, not only in terms of number of species by family but also in the number of families and orders, with the AvTD, a measure of the taxonomic breadth, being significantly higher even from the first Atlantic zone (Cadiz), just west of Gibraltar. This is an expected result according to earlier investigations that report the number of mesopelagic species for both regions (Whitehead et al., 1984; Carpenter and de Angelis, 2016). Differences in the H’ Shannon diversity index between Mediterranean and Atlantic zones were less marked than when considering just the species richness. H’ index, which includes both richness and evenness, was highly influenced by the high numbers of the dominant Cyclothone spp (both in Balears and Lisboa); the relatively lower abundances of these species in Alboran and Cadiz are also reflected by the higher evenness in these two zones.
The Mediterranean is generally viewed as a biodiversity hot spot due to the variety of marine fauna that inhabits it (Bianchi and Morri, 2000; Macpherson, 2002; Coll et al., 2010). However, this is not the case for meso and bathypelagic fish. The present ichthyofauna of the Mediterranean Sea is the outcome of its geological history (Bianchi and Morri, 2000). The isolation and evaporation of a large part of the Mediterranean during the Messinian crisis (5.97–5.33 Ma), the cold glacial and warm interglacial periods that this sea underwent are reflected in its inhabitant fauna it nowadays (Pérès, 1985; Bianchi and Morri, 2000; Coll et al., 2010; Roveri et al., 2014), which could explain the low diversity of meso and bathypelagic fish. In general, species that live between the meso- and bathypelagic zones, such as Bathylagidae, Melamphaidae, or many species within Stomiidae are absent from the Mediterranean. Although the depth of the Messinian Mediterranean is still under debate it is generally considered <1000-1500 m (Roveri et al., 2014), and this may explain the lack of such a group of species. During the cooling and warming periods and the inversions in water circulation through Gilbraltar Strait, temperate fauna from the North Atlantic waters below 300 m could enter the Mediterranean Sea, where some of them evolved into vicariant species of the original Atlantic stock (Pérès, 1985). Among mesopelagic fish this is probably the case of some endemic species such as C. pygmaea (Badcock, 1984), or N. elongatus (Hulley and Paxton, 2016a; Hulley and Paxton, 2016b). Additionally, some cold water species living nowadays in the Mediterranean Sea are considered glacial relicts of the fauna that inhabit the region during the colder periods such as B. glaciale (Angel, 1993).
Apart from the species present in the Atlantic but absent in the Mediterranean, the main species differentiating communities between these two regions were the myctophids B. glaciale and C. maderensis and the gonostomatid C. pygmaea present in the Mediterranean and absent from our Atlantic samples. These two myctophids are among the most abundantly reported in midwater trawls from the Mediterranean (Goodyear et al., 1972; Olivar et al., 2012). Although B. glaciale is common in the northeast Atlantic, it has a disjointed distribution, being an abundant species from boreal zones to 35°N but occurring also in southern zones associated with upwelled water of northwest Africa (Hulley, 1981; Hulley, 1984; Hulley and Paxton, 2016b). The absence of C. maderensis in our Atlantic samples is more difficult to explain, because the species has been reported to have a continuous distribution between 50°N and 28°N (Hulley and Paxton, 2016a; Hulley and Paxton, 2016b). However, in previous samplings conducted in a nearby zone (35° and 40°N - 20°W) in June 2018 we did not find this species either (Olivar, pers. observation). Cyclothone pygmaea is endemic to the Mediterranean and was a key species differentiating the Mediterranean and Atlantic mesopelagic communities. Interestingly, in case multivariate analysis was performed to genus level (not shown), differences between Atlantic and Mediterranean samples, although still evident, decrease because all genera present in the Mediterranean are also present in the Atlantic. Many genera are represented by a single or a couple of species in the Mediterranean but several in the Atlantic e.g. Argyropelecus, Benthosema, Chauliodus, Lampanyctus, Hygophum and Vinciguerria.
Vertical patterns
Persistent day and night DSLs were observed in all zones. In Peña et al. (2014), the non-migrating DSLs were attributed to Cyclothone braueri, which is consistent with the haul samples collected in this study. The shallower DSL observed at Lisboa (Figure 7) coincided with the shallower vertical distribution of Cyclothone (mostly C. braueri) observed using the Mesopelagos hauls (Figure 8). During the night, Balears and Alboran zones showed higher abundances above 300 m than the other 3 zones, which was consistent with the relatively high levels of backscattering intensity observed. Whilst DVM was evident from 38 kHz acoustic data at Baleras, Alboran and Cadiz, the increase in surface backscattering intensity, which is characteristic of DVM, was not found to be substantial at Lisboa and Galicia (Figure 7). This finding seems contradictory to the haul samples that showed similar patterns of DVM across all zones. Nevertheless, 18 kHz observations (Supplementary Figure 3) did also show night surface scattering layers in all zones, but again the signal was weaker at Lisboa and Galicia. This suggests that the surface mean target strength of the migrating community in these zones is relatively small. This may be due to a difference in size distribution, swimbladder morphology (i.e., gas volume at surface), presence of other micronekton components (see Proud et al., 2019), or the very patchy distribution of the species. The relatively low levels of backscattering intensity observed at the surface during the night at Galicia could be explained by the absence of swimbladdered myctophids (Figure 8) (relatively high target strength). However, myctophids were abundant in Lisboa hauls, which points to a patchy distribution of these species there.
In general, the largest differences between day and night vertical locations were observed for families Myctophidae and Stomiidae (always >200 m shallower at night). Sternoptychidae and species of genus Cyclothone exemplified the non-migrators and the gonostomatid S. elongatus partial vertical migrators. These results are in line with the feeding migrations patterns previously reported in the Atlantic and Mediterranean (Goodyear et al., 1972; Badcock and Merrett, 1976; Bernal et al., 2015; Drazen and Sutton, 2017; Contreras et al., 2020; Czudaj et al., 2021; Marohn et al., 2021).
Even for the vertical migrator taxa our results show that not all individuals of the population ascend to the surface, with a certain number remaining at depth. This is especially evident in stomiids, for which have been documented that vertical migration does not occur on a regular daily basis, and some individuals remain at depth while digesting prey for 24 to 38 h (Sutton and Hopkins, 1996). In any case, it is interesting to report that our sampling from just the epipelagic layers confirms that these species move to these layers at night (and their presence is not result of cross-contamination from lower layers). Our study also includes evidence of the close link between the levels of DCM (proxy of maximum productivity) and maximum fish concentrations from the Balears to Lisboa zones, the maximum night concentrations of migratory species were found at the level of the DCM, where high night concentrations of zooplankton are usually found (Saiz et al., 2014; Olivar et al., 2014).
Biogeographic and vertical distribution ranges of mesopelagic fish must be influenced by the physiological limits tolerable for each species. At present, the water mass exchanges between the Atlantic and the Mediterranean take place through the surface layer from the Atlantic into the Mediterranean and at depth from the denser Mediterranean waters to the Atlantic. For other marine organisms the Alboran Sea has stronger Atlantic species affinities than other Mediterranean regions (Harmelin and d’Hont, 1993), which has not been observed for the mesopelagic species in our study. As a consequence of the Gibraltar Strait depth (300-900 m), the transport from the Atlantic of fish that live both day and night in the mesopelagic layers (Sternoptychidae or Cyclotohone spp) is limited. However, the night near-surface migrants, and particularly larval stages (always located in the upper layers (Olivar et al., 2018)) should be susceptible to being transported from the Atlantic to the Mediterranean. Despite this, as already discussed, mesopelagic fish fauna composition from both sides of the Strait show marked differences, which could be likely due to the different thermal and salinity regimes. Migrant species must be adapted to cope with the high temperature gradients that they encounter in the daily vertical migration, which requires a high tolerance to changes in this environmental factor. However, the day mesopelagic habitat of Atlantic species is characterized by both lower temperature and lower salinity than the Mediterranean. Therefore, in the event of transport of the night surface fish from the Atlantic to the Mediterranean, they will encounter very different conditions when migrating to mesopelagic layers during the daytime, particularly higher salinity. Osmoregulation to deal with changes in salinity implies high metabolic expenses (Taylor, 1988; Pérès and Mantelatto, 2020) and has been advocated as the reason for differences in populations of crustaceans from both sides of Gibraltar Strait (Ojeda et al., 2022). It is difficult to hypothesize the fate of larvae that could have been displaced from the Atlantic to the Alboran Sea. The surface waters are not so different in temperature and salinity, but they are in terms of the current dynamics of the upper layers, much more intense in Alboran, which may compromise larval survival.
In summary, the results of the present study showed that diversity patterns were not related to the productivity levels of the different zones, but are a combination of species biogeography, temperature and salinity regimes. Mesopelagic communities in the five studied zones were dominated by Cyclothone species, and both the lowest and the highest diversities corresponded to the two more oligotrophic zones, Balears and Cadiz, respectively. However, in terms of the number of species the two Mediterranean zones have a similarly low number of species. Mesopelagic ichthyofauna of the Western Mediterranean was represented by a few species belonging to a small number of orders, i.e, Myctophiformes and Stomiiformes, and with the occurrence of some endemic species. These same taxa dominate in the Atlantic but were composed of a higher number of species, and in addition fish from families (or orders) with deeper distributions were also found. Comparisons of catch collections showed a higher number of fish, but of smaller body sizes in the Mediterranean than in the Atlantic. The combination of net collections and acoustic detections indicated that in all zones there is a persistent day-night DSL that can be associated with Cyclothone species (mainly C. braueri). According to net collections, in all studied zones (except Galicia) fish night migration extends to the upper 100 m layers and their maximum abundances coincide with the location of the Chlorophyll a maxima (much closer to the surface in Lisboa and at the DCM in the other zones). Although differences were not significant, net catches showed a tendency for higher biomass, and a higher relative contribution of larger sizes in the more productive Lisboa zone. Taking into account the positive allometric growth of mesopelagic fish (Eduardo et al., 2019; López-Pérez et al., 2020; Czudaj et al., 2022) and that our trawl system is biased towards small fish, our results suggest that the differences would be even higher if larger size classes had been collected, and therefore the most productive zone would have a higher overall biomass.
Conclusion
The use of the same sampling strategy in a large geographical region, from the Mediterranean Sea to the Atlantic, under contrasting hydrographic and productivity conditions, has provided valuable and comparable information on mesopelagic fish diversity, community composition and vertical migratory patterns. Analyses of diversity patterns indicated that they were not related to the productivity levels of the different zones, but resulted from a combination of species biogeography, temperature and salinity regimes. However, in terms of biomass the results from net collections point to a higher biomass in the more productive zones. Results on vertical migration showed that the layers of maximum night abundances for vertical migrator species coincide with the location of the maximum chlorophyll concentrations. We also found that in all zones there was a persistent, non-migrating DSL that comprised mainly Cyclothone species.
Data availability statement
The raw data supporting the conclusions of this article are publicly available through PANGEA platform: Olivar et al. (2022) https://doi.org/10.1594/PANGAEA.947631, and Yang et al. (2021) https://doi.pangaea.de/10.1594/PANGAEA.938688.
Ethics statement
The netting and acoustic aspects of this study were approved from an ethical point of view by the University of St Andrews’ School of Biology Ethics Committee (Ref. SEC20018).
Author contributions
MPO conceive and promoted this study, acted as cruise leader, and together with AS and AS-L identified the specimens. AC designed the multi-sampler and was responsible for the deployment of the Mesopelagos midwater trawl and the control of fishing operations. AB contributed to sample revision. ME was responsible for CTD data. YY was responsible for acoustic data collection and, together with RP and AB, for acoustic data curation and analyses. All authors contributed to the article and approved the submitted version.
Funding
This work was a component of the European Union Project ‘Sustainable Management of Mesopelagic Resources’ (SUMMER, Grant Agreement 817806) as funded by the Horizon 2020 Research and Innovation Programme.
Acknowledgments
We are very grateful to the crew and scientists on board the research vessel “Sarmiento de Gamboa” and the technicians of the “Unidad de Tecnología Marina” (UTM) for their support and help during the cruise. AS-L was supported by a postgraduate grant (BES-2017-082540) from the Spanish Ministry of Science and Innovation. We acknowledge the Severo Ochoa Centre of Excellence accreditation (CEX2019-000928-S).
Conflict of interest
The authors declare that the research was conducted in the absence of any commercial or financial relationships that could be construed as a potential conflict of interest.
Publisher’s note
All claims expressed in this article are solely those of the authors and do not necessarily represent those of their affiliated organizations, or those of the publisher, the editors and the reviewers. Any product that may be evaluated in this article, or claim that may be made by its manufacturer, is not guaranteed or endorsed by the publisher.
Supplementary material
The Supplementary Material for this article can be found online at: https://www.frontiersin.org/articles/10.3389/fmars.2022.1028717/full#supplementary-material
Supplementary Figure 1 | Number of taxa per station (blue) and modelled (UGE index in grey) species accumulated plot. Stations 1 to 12 from the Mediterranean.
Supplementary Figure 2 | Size structure by zones of Myctophiformes and Stomiiformes collected at stations covering from 0 to 700 m with the Mesopelagos net. Stomiiformes were represented up to 210 mm, although a few specimens of size classes up to 300 mm were also collected. SL: Standard length.
Supplementary Figure 3 | Typical zonal scattering layer distributions observed using the hull-mounted 18 kHz echosounder. The colour scale is echo intensity (mean volume backscattering strength, Sv, dB re 1 m-1). Left panels correspond to daytime observations, and right panels to night-time. Ping repetition interval was 2 seconds. At the c. 2.5 knots vessel speed that these observations were made (between net hauls), the 200 pings shown equate to about 500 m distance. The overlaid black curves represent the temperature profiles for simultaneous CTD casts (as shown in Figure 2).
Supplementary Figure 4 | Day and night vertical distribution of all fish collected through the first 700 m of the water column a) numbers b) mass.
References
Álvarez I., Prego R., de Castro M., Varela M. (2012). Revisión de los eventos de afloramiento en Galicia: eventos fuera de temporada en las rías (1967–2009). Cienc. Mar. 38 (1B), 143–159. doi: 10.7773/cm.v38i1B.17762012
Angel M. V. (1993). Biodiversity of the pelagic ocean. Conserv. Biol. 7 (4), 760–772. doi: 10.1046/j.1523-1739.1993.740760.x
Ariza A., Garijo J. C., Landeira J. M., Bordes F., Hernández-León S. (2015). Migrant biomass and respiratory carbon flux by zooplankton and micronekton in the subtropical northeast Atlantic ocean (Canary islands). Prog. Oceanogr. 134, 330–342. doi: 10.1016/j.pocean.2015.03.003
Armi L., Farmer D. M. (1988). The flow of Mediterranean water through the strait of Gibraltar. Prog. Oceanogr. 21 (1), 1–105. doi: 10.1016/0079-6611(88)90055-9
Badcock J. (1984). “Gonostomatidae,” in Fishes of the north-eastern Atlantic and the Mediterranean, vol. I . Eds. Whitehead P. J. P., Bauchot M.-L., Hureau J. C., Nielsen J., Tortonese E. (Paris: Unesco), 284–300.
Badcock J., Merrett N. R. (1976). Midwater fishes in the eastern north atlantic. i. vertical distribution and associated biology in 30°N, 23°W, with developmental notes on certain myctophids. Prog. Oceanogr. 7 (1), 3–58. doi: 10.1016/0079-6611(76)90003-3
Bernal A., Olivar M. P., Maynou F., Fernández de Puelles M. L. (2015). Diet and feeding strategies of mesopelagic fishes in the western Mediterranean. Prog. Oceanogr. 135, 1–17. doi: 10.1016/j.pocean.2015.03.005
Bianchi C. N., Morri C. (2000). Marine biodiversity of the Mediterranean Sea: Situation, problems and prospects for future research. Mar. pollut. Bull. 40 (5), 367–376. doi: 10.1016/S0025-326X(00)00027-8
Brierley A. S. (2014). Diel vertical migration. Curr. Biol. 24 (22), 1074–1076. doi: 10.1016/j.cub.2014.08.054
Carpenter K. E., de Angelis N. (2016). “The living marine resources of the Eastern central Atlantic,” in Volume 3: Bony fishes, part 1 (Elopiformes-scorpaeniformes) (Rome: FAO Species Identification Guide for Fishery Purposes), 1511–2350.
Clarke K. R., Gorley R. N., Somerfield P. J., Warwick R. M. (2014). Change in marine communities: an approach to statistical analysis and interpretation. 3rd edition (Plymouth: PRIMER-E), 262.
Clarke K. R., Somerfield P. J., Gorley R. N. (2008). Testing of null hypotheses in exploratory community analyses: similarity profiles and biota-environment linkage. J. Exp. Mar. Biol. Ecol. 366 (1), 56–69. doi: 10.1016/j.jembe.2008.07.009
Clarke K. R., Warwick R. M. (2001). A further biodiversity index applicable to species lists: Variation in taxonomic distinctness. Mar. Ecol. Progr. Ser. 216, 265–278. doi: 10.3354/meps216265
Coll M., Piroddi C., Steenbeek J., Kaschner K., ben Rais Lasram F., Aguzzi J., et al. (2010). The biodiversity of the Mediterranean Sea: Estimates, patterns, and threats. PloS One 5 (8), e11842. doi: 10.1371/journal.pone.0011842
Contreras T., Olivar M. P., González-Gordillo J., Hulley P. (2020). Feeding patterns of transforming and juvenile myctophids that migrate into neustonic layers. ar. Ecol. Prog. Ser. 650, 239–252. doi: 10.3354/meps13234
Czudaj S., Koppelmann R., Möllmann C., Schaber M., Fock H. O. (2021). Community structure of mesopelagic fishes constituting sound scattering layers in the eastern tropical north Atlantic. J. Mar. Syst. 224, 103635. doi: 10.1016/j.jmarsys.2021.103635
Czudaj S., Möllmann C., Fock H. O. (2022). Length-weight relationships of 55 mesopelagic fishes from the eastern tropical north Atlantic: across- and within-species variation (body shape, growth stanza, condition factor). J. Fish Biol. 101 (1), 26–41. doi: 10.1111/jfb.15068
Davison P. C., Checkley D. M., Koslow J. A., Barlow J. (2013). Carbon export mediated by mesopelagic fishes in the northeast pacific ocean. Prog. Oceanogr. 116, 14–30. doi: 10.1016/j.pocean.2013.05.013
Demer D. A., Berger L., Bernasconi M., Bethke E., Boswell K., Chu D., et al. (2015). Calibration of acoustic instruments. ICES Cooperative Res. Rep. 326, 133. doi: 10.25607/OBP-185
Denton J. S. S., Adams D. C. (2015). A new phylogenetic test for comparing multiple high-dimensional evolutionary rates suggests interplay of evolutionary rates and modularity in lanternfishes (Myctophiformes; myctophidae). Evol. 69, 2425–2440. doi: 10.1111/evo.12743
de Robertis A., Higginbottom I. (2007). A post-processing technique to estimate the signal-to-noise ratio and remove echosounder background noise. ICES J. Mar. Sci. 64 (6), 1282–1291. doi: 10.1093/icesjms/fsm112
Drazen J. C., Sutton T. T. (2017). Dining in the deep: The feeding ecology of deep-sea fishes. Annu. Rev. Mar. Sci. 9 (1), 337–366. doi: 10.1146/annurev-marine-010816-060543
Echoview Software Pty Ltd (2021). Echoview software pty Ltd (Echoview® version 12.0.304) (Tasmania: Myriax, Hobart).
Eduardo L. N., Mincarone M. M., Villarins B. T., Frédou T., Lira A. S., Bertrand A., et al. (2019). Length–weight relationships of eleven mesopelagic fishes from oceanic islands of the southwestern tropical Atlantic. J. Appl. Ichthyol. 35 (2), 605–607. doi: 10.1111/jai.13840
Firing E., Hummon J. M. (2010). Ship-mounted acoustic Doppler current profilers. in the GO-SHIP repeat hydrography manual. In: A collection of expert reports and guidelines. Available at: http://www.go-ship.org/HydroMan.html (Accessed March 19, 2012). IOCCP Report Number 14, ICPO Publication Series Number 134.
Fortier L., Leggett W. C. (1983). Vertical migrations and transport of larval fish in a partially mixed estuary. Can. J. Fish. Aquat. Sci. 40 (10), 1543–1555. doi: 10.1139/f83-179
Fock H. O., Pusch C., Ehrich S. (2004). Structure of deep-sea pelagic fish assemblages in relation to the mid-Atlantic ridge (45°N to 50°N). Deep-Sea Res. 51, 953–978. doi: 10.1016/j.dsr.2004.03.004
Fraga F. (1981). “Upwelling off the Galician coast, northwest Spain,” in Coastal upwelling. Ed. Richardson F. A. (Washington: American Geophysical Union), 176–182.
Goodyear R. H., Gibbs R. H. Jr., Roper C. F. E., Kleckner R. C., Sweeney M. J. (1972). Mediterranean Biological studies Vol. 2 (Washington DC: Smithsonian Institution), 355.
Grimaldo E., Grimsmo L., Alvarez P., Herrmann B., Møen Tveit G., Tiller R., et al. (2020). Investigating the potential for a commercial fishery in the northeast Atlantic utilizing mesopelagic species. ICES J. Mar. Sci. 77 (7–8), 2541–2556. doi: 10.1093/icesjms/fsaa114
Haedrich R. L. (1997). “Distribution and population ecology,” in Fish physiology, vol. 16 . Eds. Randall D. J., Farrell A. P. (San Diego: Academic Press), 79–114. doi: 10.1016/S1546-5098(08)60228-9
Harmelin, d’Hondt (1993). Transfers of bryozoan species between the Atlantic ocean and the Mediterranean Sea via the strait of Gibraltar. Oceanol. Acta 16 (1), 63–72.
Heino M., Porteiro F. M., Sutton T. T., Falkenhaug T., Godø O. R., Piatkowskiet O. R. (2011). Catchability of pelagic trawls for sampling deep-living nekton in the mid- north Atlantic. ICES J. Mar. Sci. 68 (2), 377–389. doi: 10.1093/icesjms/fsq089
Hernández-León S., Olivar M. P., Fernández de Puelles M. L., Bode A., Castellón A., López-Pérez C., et al. (2019). Zooplankton and micronekton active flux across the tropical and subtropical Atlantic ocean. Front. Mar. Sci. 6. doi: 10.3389/fmars.2019.00535
Hopkins T. L., Sutton T. T., Lancraft T. M. (1996). The trophic structure and predation impact of a low latitude midwater fish assemblage. Prog. Oceanogr. 38 (3), 205–239. doi: 10.1016/S0079-6611(97)00003-7
Hudson J. M., Steinberg D. K., Sutton T. T., Graves J. E., Latour R. J. (2014). Myctophid feeding ecology and carbon transport along the northern mid-Atlantic ridge. Deep Sea Res. I: Oceanogr. Res. Pap. 93, 104–116. doi: 10.1016/j.dsr.2014.07.002
Hulley P. A. (1981). Results of the research cruises of FRV ‘“Walther herwig”’ to south america. 58. family myctophidae (Osteichthyes, myctophiformes). Archiv Für Fischereiwissenschaft 31 (1), 1–300.
Hulley P. A. (1984). “Myctophidae,” in Fishes of the north-eastern Atlantic and the Mediterranean, vol. 1 . Eds. Whitehead P. J. P., Bauchot M.-L., Hureau J.-C., Nielsen J., Tortonese E. (Paris: Unesco), 429–483.
Hulley P. A., Paxton J. R. (2016a). “Neoscopelidae,” in The living marine resources of the eastern central Atlantic, vol. 3 . Eds. Carpenter, Angelis N. (Roma: FAO), 1855–1859. Bony Fishes, Part 1 (Elopiformes-Scorpaeniformes).
Hulley P. A., Paxton J. R. (2016b). “Myctophidae,” in The living marine resources of the eastern central Atlantic, vol. 3 . Eds. Carpenter, Angelis N. (Roma: FAO), 1860–1928. Bony Fishes, Part 1 (Elopiformes-Scorpaeniformes).
Irigoien X., Klevjer T. A., Røstad A., Martinez U., Boyra G., Acuña J. L., et al. (2014). Large Mesopelagic fishes biomass and trophic efficiency in the open ocean. Nat. Commun. 5 (1), 3271. doi: 10.1038/ncomms4271
Judkins H., Vecchione M., Cook A., Sutton T. (2017). Diversity of midwater cephalopods in the northern gulf of Mexico: comparison of two collecting methods. Mar. Biodiv. 47, 647–657. doi: 10.1007/s12526-016-0597-8
Kaartvedt S., Staby A., Aksnes D. L. (2012). Efficient trawl avoidance by mesopelagic fishes causes large underestimation of their biomass. Mar. Ecol. Prog. Ser. 456, 1–6. doi: 10.3354/meps09785
Klevjer T. A., Irigoien X., Røstad A., Fraile-Nuez E., Benítez-Barrios V. M. (2016). Large Scale patterns in vertical distribution and behaviour of mesopelagic scattering layers. Sci. Rep. 6 (1), 1–11. doi: 10.1038/srep19873
Koslow J. A., Kloser R. J., Williams A. (1997). Pelagic biomass and community structure over the mid-continental slope off southeastern Australia based upon acoustic and midwater trawl sampling. Mar. Ecol. Prog. Ser. 146, 21–35. doi: 10.3354/meps146021
Longhurst A. R. (2007). Ecological geography of the sea (London: Academic Press). doi: 10.1016/B978-0-12-455521-1.X5000-1
López-Pérez C., Olivar M. P., Hulley P. A., Tuset V. M. (2020). Length–weight relationships of mesopelagic fishes from the equatorial and tropical Atlantic waters: influence of environment and body shape. J. Fish Biol. 96 (6), 1388–1398. doi: 10.1111/jfb.14307
Macpherson E. (2002). Large–Scale species–richness gradients in the Atlantic ocean. Proc. R. Soc B: Biol. Sci. 269 (1501), 1715–1720. doi: 10.1098/rspb.2002.2091
Marohn L., Schaber M., Freese M., Pohlmann J. D., Wysujack K., Czudaj S., et al. (2021). Distribution and diel vertical migration of mesopelagic fishes in the southern Sargasso Sea — observations through hydroacoustics and stratified catches. Mar. Bio. 51 (6), 87. doi: 10.1007/s12526-021-01216-6
Massutí E., Gordon J. D. M., Morata J., Swan S. C., Stefanescu C., Merrett N. R. (2004). Mediterranean And Atlantic deep-sea fish assemblages: differences in biomass composition and size-related structure. Sci. Mar. 68 (S3), 101–115. doi: 10.3989/scimar.2004.68s3101
Meillat M. (2012). Essais du chalut mésopélagos pour le programme MYCTO 3D – MAP de l’IRD, à bord du Marion ufresne. du 10 au 21 Août 2012. IFREMER Rapport mission Marion Dufresne RBE/STH/LTH 2012-05.
Merrett N. R. (1994). Reproduction in the north Atlantic oceanic ichthyofauna and the relationship between fecundity and species’ sizes. Envir. Biol. Fis. 41, 207–245. doi: 10.1007/bf02197846
Millot C. (2005). Circulation in the Mediterranean Sea: evidences, debates and unanswered questions. Sci. Mar. 69 (S1), 5–21. doi: 10.3989/scimar.2005.69s15
Ojeda V., Serra B., Lagares C., Rojo-Francàs E., Sellés M., Marco-Herrero E., et al. (2022). Interannual fluctuations in connectivity among crab populations (Liocarcinus depurator) along the Atlantic-Mediterranean transition. Sci. Rep. 12 (1), 9797. doi: 10.1038/s41598-022-13941-4
Olivar M. P., Bernal A., Molí B., Peña M., Balbín R., Castellón A., et al. (2012). Vertical distribution, diversity and assemblages of mesopelagic fishes in the western Mediterranean. Deep Sea Res. I: Oceanogr. Res. Pap. 62, 53–69. doi: 10.1016/j.dsr.2011.12.014
Olivar M. P., Castellón A., Sabatés A., Sarmiento-Lezcano A., Bernal A. (2022). Abundance of mesopelagic fish collected at different layers of the water column around the Iberian peninsula. PANGAEA. doi: 10.1594/PANGAEA.947631
Olivar M. P., Contreras T., Hulley P. A., Emelianov M., López-Pérez C., Tuset V., et al. (2018). Variation in the diel vertical distributions of larvae and transforming stages of oceanic fishes across the tropical and equatorial Atlantic. Prog. Oceanogr. 160, 83–100. doi: 10.1016/j.pocean.2017.12.005
Olivar M. P., González-Gordillo J. I., Salat J., Chust G., Cózar A., Hernández-León S., et al. (2016). The contribution of migratory mesopelagic fishes to neuston fish assemblages across the Atlantic, Indian and pacific oceans. Mar. Freshw. Res. 67 (8), 1114–1127. doi: 10.1071/MF14391
Olivar M. P., Hulley P. A., Castellón A., Emelianov M., López C., Tuset V. M., et al. (2017). Mesopelagic fishes across the tropical and equatorial Atlantic: Biogeographical and vertical patterns. Prog. Oceanogr. 151, 116–137. doi: 10.1016/j.pocean.2016.12.001
Olivar M. P., Sabatés A., Alemany F., Balbín R., Fernández de Puelles M. L., Torres A. P. (2014). Diel-depth distributions of fish larvae off the Balearic islands (western Mediterranean) under two environmental scenarios. J. Mar. Sys. 138, 127–138. doi: 10.1016/j.jmarsys.2013.10.009
Pauly D., Piroddi C., Hood L., Bailly N., Chu E., Lam V., et al. (2021). The biology of mesopelagic fishes and their catches (1950–2018) by commercial and experimental fisheries. J. Mar. Sci. Eng. 9 (10), 1057. doi: 10.3390/jmse9101057
Peña M., Olivar M. P., Balbín R., Lopez-Jurado J. L., Iglesias M., Miquel J. (2014). Acoustic detection of mesopelagic fishes in scattering layers of the Balearic Sea (western Mediterranean). Can. J. Fish. Aquat. Sci. 71 (8), 1186–1197. doi: 10.1139/cjfas-2013-0331
Pérès J. M. (1985). “History of the Mediterranean biota and the colonization of the depths,” in Western Mediterranean. Ed. Margalef R. (Oxford: Pergamon Press), 198–232.
Pérès P. A., Mantelatto F. L. (2020). Salinity tolerance explains the contrasting phylogeographic patterns of two swimming crabs species along the tropical western Atlantic. Evol. Ecol. 34 (4), 589–609. doi: 10.1007/s10682-020-10057-x
Poulsen J. Y., Byrkjedal I., Willassen E., Rees D., Takeshima H., Satoh T. P., et al. (2013). Mitogenomic sequences and evidence from unique gene rearrangements corroborate evolutionary relationships of myctophiformes (Neoteleostei). BMC Evol. Biol. 13 (1), 111. doi: 10.1186/1471-2148-13-111
Priede I. G. (2017). Deep-Sea fishes: Biology, diversity, ecology and fisheries (United Kingdom: Cambridge University Press). doi: 10.1017/9781316018330
Proud R., Cox M. J., Brierley A. S. (2017). Biogeography of the global ocean’s mesopelagic zone. Curr. Biol. 27 (1), 113–119. doi: 10.1016/j.cub.2016.11.003
Proud R., Handegard N. O., Kloser R. J., Cox M. J., Brierley A. S. (2019). From siphonophores to deep scattering layers: uncertainty ranges for the estimation of global mesopelagic fish biomass. ICES J. Mar. Sci. 76 (3), 718–733. doi: 10.1093/icesjms/fsy037
Ramírez-Llodra E., Brandt A., Danovaro R., De Mol B., Escobar E., German C. R., et al. (2010). Deep, diverse and definitely different: unique attributes of the world’s largest ecosystem. Biogeosciences 7, 2851–2899. doi: 10.5194/bg-7-2851-2010
Rees D. J., Poulsen J. Y., Sutton T. T., Costa P. A. S., Landaeta M. F. (2020). Global phylogeography suggests extensive eucosmopolitanism in mesopelagic fishes (Maurolicus: Sternoptychidae). Sci. Rep. 10 (1), 20544. doi: 10.1038/s41598-020-77528-7
Reygondeau G., Guidi L., Beaugrand G., Henson S. A., Koubbi P., MacKenzie B. R., et al. (2018). ). global biogeochemical provinces of the mesopelagic zone. J. Biog. 45 (2), 500–514. doi: 10.1111/jbi.13149
Roe H. S. J., Badcock J. (1984). The diel migrations and distributions within a mesopelagic community in the north East atlantic. 5. vertical migrations and feeding of fish. Prog. Oceanogr. 13 (3), 389–424. doi: 10.1016/0079-6611(84)90014-4
Ross S. W., Quattrini A. M., Roa-Varón A. Y., McClain J. P. (2010). Species composition and distributions of mesopelagic fishes over the slope of the north-central gulf of Mexico. Deep Sea Res. II: Top. Stud. Oceanogr 57 (21), 1926–1956. doi: 10.1016/j.dsr2.2010.05.008
Roveri M., Lugli S., Manzi V., Gennari R., Schreiber B. C. (2014). High-resolution strontium isotope stratigraphy of the messinian deep Mediterranean basins: implications for marginal to central basins correlation. Mar. Geol. 349, 113–125. doi: 10.1016/j.margeo.2014.01.002
Ryan T. E., Downie R. A., Kloser R. J., Keith G. (2015). Reducing bias due to noise and attenuation in open-ocean echo integration data. ICES J. Mar. Sci. 72 (8), 2482–2493. doi: 10.1093/icesjms/fsv121
Sabatés A., Olivar M. P., Salat J., Palomera I., Alemany F. (2007). Physical and biological processes controlling the distribution of fish larvae in the NW Mediterranean. Progr. Oceanogr. 74 (2), 355–376. doi: 10.1016/j.pocean.2007.04.017
Saiz E., Sabatés A., Gili J. M. (2014). “The zooplankton,” in The Mediterranean Sea (Dordrecht: Springer), 183–211.
Salat J., Garcia M. A., Cruzado A., Palanques A., Arıín L., Gomis D., et al. (2002). Seasonal changes of water mass structure and shelf slope exchanges at the ebro shelf (NW Mediterranean). Cont. Shelf Res. 22 (2), 327–348. doi: 10.1016/S0278-4343(01)00031-0
Schlitzer R. (2016) Ocean data view. Available at: https://odv.awi.de.
Siokou-Frangou I., Christaki U., Mazzocchi M. G., Montresor M., Ribera d’Alcalá M., Vaqué D., et al. (2010). Plankton in the open Mediterranean Sea: a review. Biogeosciences 7 (5), 1543–1586. doi: 10.5194/bg-7-1543-2010
Sousa M. C., Alvarez I., de Castro M., Gomez-Gesteira M., Dias J. M. (2017). Seasonality of coastal upwelling trends under future warming scenarios along the southern limit of the canary upwelling system. Prog. Oceanogr. 153, 16–23. doi: 10.1016/j.pocean.2017.04.002
Stefanescu C., Cartes J. E. (1992). Benthopelagic habits of adult specimens of Lampanyctus crocodilus (Risso, 1810) (Osteichthyes, Myctophidae) in the western Mediterranean deep slope. Sci. Mar. 56 (1), 69–74.
St. John M. A., Borja A., Chust G., Heath M., Grigorov I., Mariani P., et al. (2016). A dark hole in the understanding of marine ecosystems and their services: perspectives from the mesopelagic community. Front. Mar. Sci. 3. doi: 10.3389/fmars.2016.00031
Sutton T. T. (2013). Vertical ecology of the pelagic ocean: classical patterns and new perspectives. J. Fish Biol. 83 (6), 1508–1527. doi: 10.1111/jfb.12263
Sutton T. T., Clark M. R., Dunn D. C., Halpin P. N., Rogers A. D., Guinotte J., et al. (2017). A global biogeographic classification of the mesopelagic zone. Deep Sea Res. I. 126, 85–102. doi: 10.1016/j.dsr.2017.05.006
Sutton T. T., Hopkins T. L. (1996). Trophic ecology of the stomiid (Pisces: Stomiidae) fish assemblage of the eastern gulf of Mexico: strategies, selectivity and impact of a top mesopelagic predator group. Mar. Biol. 127 (2), 179–192. doi: 10.1007/BF00942102
Sutton T. T., Hulley P. A., Wienerroither R., Zaera-Perez D., Paxton J. R. (2020). “Identification guide to the mesopelagic fishes of the central and southeast Atlantic ocean,” in FAO species identification guide for fishery purposes (Rome: FAO).
Sutton T. T., Porteiro F. M., Heino M., Byrkjedal I., Langhelle G., Anderson C. I. H., et al. (2008). Vertical structure, biomass and topographic association of deep-pelagic fishes in relation to a mid-ocean ridge system. Deep-Sea Res. II. 55 (1–2), 161–184. doi: 10.1016/j.dsr2.2007.09.013
Taylor A. C. (1988). The ecophysiology of decapods in the rock pool environment,” in aspects of decapod crustacean biology: Symposium of the zoological society of London Vol. 59. Eds. Finchamand A. A., Rainbow P. S. (Oxford: Oxford University Press), 227–261.
Tortonese E. (1960). General remarks on the Mediterranean deep-sea fishes. Bull. Inst. Oceanogr. 1167, 1–14.
Vecchione M., Falkenhaug T., Sutton T., Cook A., Gislason A., Hansen H.Ø., et al. (2015). The effect of the north Atlantic subpolar front as a boundary in pelagic biogeography decreases with increasing depth and organism size. Prog. Oceanogr. 138 (A), 105–115. doi: 10.1016/j.pocean.2015.08.006
Webb T. J., Vanden Berghe E., O’Dor R. (2010). Biodiversity’s big wet secret: The global distribution of marine biological records reveals chronic under-exploration of the deep pelagic ocean. PloS One 5 (8), e10223. doi: 10.1371/journal.pone.0010223
Whitehead P. J. P., Bauchot M. L., Hureau J. C., Nielsen J., Tortonese E. (1984). Fishes of the northeastern Atlantic and the Mediterranean Vol. I–II (Paris: UNESCO). Available at: https://books.google.es/books?id=kGYWAQAAIAAJ.
Yang Y., Proud R., Castellón A., Sabatés A., Olivar M. P., Brierley A. S. (2021). EK80 raw multi-frequency (18, 38, 70, 120, 200 kHz) acoustic data collected during RV sarmiento de gamboa cruise ‘Sustainable management of mesopelagic resources’ (SUMMER) in 2020 to the Mediterranean. PANGAEA. doi: 10.1594/PANGAEA.938688
Keywords: diversity, bristlemouths, lanternfishes, vertical migration, deep scattering layers.
Citation: Olivar MP, Castellón A, Sabatés A, Sarmiento-Lezcano A, Emelianov M, Bernal A, Yang Y, Proud R and Brierley AS (2022) Variation in mesopelagic fish community composition and structure between Mediterranean and Atlantic waters around the Iberian Peninsula. Front. Mar. Sci. 9:1028717. doi: 10.3389/fmars.2022.1028717
Received: 26 August 2022; Accepted: 08 November 2022;
Published: 25 November 2022.
Edited by:
Chiara Romano, University of Gastronomic Sciences, ItalyReviewed by:
Eugenio Fraile-Nuez, Consejo Superior de Investigaciones Científicas (CSIC), SpainMichael Vecchione, National Oceanic and Atmospheric Administration (NOAA), United States
Copyright © 2022 Olivar, Castellón, Sabatés, Sarmiento-Lezcano, Emelianov, Bernal, Yang, Proud and Brierley. This is an open-access article distributed under the terms of the Creative Commons Attribution License (CC BY). The use, distribution or reproduction in other forums is permitted, provided the original author(s) and the copyright owner(s) are credited and that the original publication in this journal is cited, in accordance with accepted academic practice. No use, distribution or reproduction is permitted which does not comply with these terms.
*Correspondence: M. Pilar Olivar, cG9saXZhckBpY20uY3NpYy5lcw==
†ORCID: M. Pilar Olivar, orcid.org/0000-0002-8887-9181