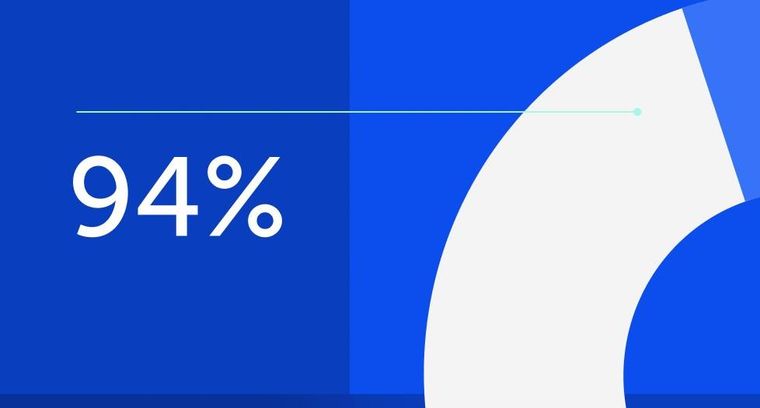
94% of researchers rate our articles as excellent or good
Learn more about the work of our research integrity team to safeguard the quality of each article we publish.
Find out more
ORIGINAL RESEARCH article
Front. Mar. Sci., 22 November 2022
Sec. Marine Biology
Volume 9 - 2022 | https://doi.org/10.3389/fmars.2022.1025615
The seed bank of Halophila ovalis is crucial for resilience to disturbance through re-establishment. Understanding seasonal changes in abundance and quality of seeds in natural seed banks is critical for seed-based restoration. We selected an estuary in southwestern Australia and investigated the seasonal changes of seed distribution and viability in H. ovalis seed banks. We also adapted an X-ray viability test used for terrestrial seeds to test the viability of H. ovalis seeds. We then simulated the effect of low salinity on seed viability through a short-term indoor experiment. Seed density was significantly different between sites and seasons (0 to 43590 seeds·m-2), and the highest seed density in the seed banks was found after the reproductive season (May). The proportion of viable seeds in the seed bank was less than 22%, and was not subjected to substantial seasonal variability. The density of seeds in the seed bank decreased in spring, which indicated winter conditions were not prompt seed loss. We also predicted that extreme rainfall events and the resulting extremely low salinity would significantly reduce seed viability, and could decrease in seed germination; limit population recruitment. As it rapidly colonizes marine sediments from seeds, H. ovalis was considered an ideal seagrass for restoration purposes. Our results provide physiological information for H. ovalis seed banks to support seed-based restoration plans. Such understanding would enable accurate predictions about seagrass population resilience to extreme climate events in estuaries, where variable and extremely low salinity may limit seagrass population recovery from seeds through decreasing their viability.
Seagrasses provide a number of ecosystem services like provision of habitat, food, and a nursery for a variety of marine organisms, and are the key sites for global carbon storage. Seagrasses are also being lost at an alarming rate (Orth et al., 2006a; Barbier et al., 2011; Fourqurean et al., 2012). Seed-based restoration is recognized as a cost-efficient method for large-scale seagrass restoration programs (Marion and Orth, 2010; Tanner and Parham, 2010). The high genetic diversity in seeds promote high genetic diversity in restored meadows with resistance and resilience capacities (Reynolds et al., 2013; Kilminster et al., 2015). Although, seeding has emerged as an effective tool to some seagrass species, the science behind seed-based approaches including collection of seeds, viability of seeds, and conditions for seed storage and germination, requires further research.
The seed bank in surface sediments of seagrass beds for a given species can be transient (turnover in less than 1 year) or persistent (seeds that remain longer than 1 year) and the length of time that seeds are viable in the seed bank is critical for long-term seagrass population stability (Orth et al., 2000). The presence, persistence and spatial dispersion of a sediment seed bank drives recovery and growth of seagrass meadows due to its functional characteristics (Fenner and Thompson, 2005). In some species, seeds produced from sexual reproduction undergo a period of dormancy which provides a buffer against times of reduced reproductive output, thereby increasing the resilience of local populations to disturbance (Smith et al., 2016). However, previous research on recruitment from seed in seagrasses have found high mortalities in seed settlement, with the probability of surviving at a density of 100 seeds·m-2 was expected to be around 0.1% (Orth et al., 2006b; Statton et al., 2017a). That phenomenon might be caused by the failure of seedling establishment or the loss of seed viability before the germination or seedings. There have been numerous studies focusing on the relationships between seeding density and seedling establishment, and the seedling establishment process was considered as the most vulnerable step limiting restoration success (Marion and Orth, 2010; Statton et al., 2017a). However, few studies focused on the changes of seed abundance and viability, which is also a critical process in seed banks.
The abundance of seeds in seed banks of seagrass beds are highly variable, but less is known about their spatial distribution, particularly over an annual reproductive cycle (Harwell and Orth, 2002; Hovey et al., 2015). The density of seeds varied significantly among the seasons, and a large amount of seagrass seeds are lost after reproduction and seed dispersal (Hovey et al., 2015; Máñez-Crespo et al., 2020; Yue et al., 2020; Vercaemer et al., 2021). Comprehensive studies over the life cycle in seagrass species are necessary to collect complete information on that spatial variability of seed abundance. Additionally, previous studies had indicated variability in seeds is driven by several factors such as wave and currents, distribution of female and male flowers, the distribution of large mammals and birds, hence more in situ and ex situ experiments are needed to be conducted on the spatial and temporal dynamics of seagrass seed abundance (Inglis, 2000; Wu et al., 2016).
Halophila ovalis (R. Br.) Hook.f. is a pioneer species in the Swan-Canning Estuary, Western Australia, providing several important ecological functions such as provision of food for black swan, habitat for invertebrates, and sediment stabilization (Orth et al., 2006c; Kilminster and Forbes, 2014). However, H. ovalis coverage declined by more than one-third over the last 30 years due to anthropogenic activities and climate change (Kilminster et al., 2014). H. ovalis has an annual life cycle with fast vegetative growth, flowering and high seed production during spring and summer months (Kilminster and Forbes, 2014). Previous studies pointed out the persistence and recovery of H. ovalis depended on sexual reproduction, with 900 seeds m-2 potentially entering the sediment seed bank each year in southwest Australia (Kuo and Kirkman, 1992; Kilminster et al., 2014). However, a thorough assessment of the sediment seed bank of H. ovalis in this region has been poorly investigated.
Conditions required for a seed to break dormancy and germinate have not been addressed for every seagrass species (Orth et al., 2000). Also, the methodology of seed viability testing needs to be improved. H. ovalis has dormant seeds like Zostera spp. In Zostera, tetrazolium staining and squish/sink viability methods have been used to test seed viability (Inglis, 2000; Orth et al., 2000). Conventional tetrazolium seed staining has been applied to Zostera and Ruppia to test seed viability, but is typically labor intensive and destructive (Sawma and Mohler, 2002; Cho and Sanders, 2009; Marion and Orth, 2010). The interpretation of viability from tetrazolium staining in H. ovalis seeds has been shown to be equivocal (Waite et al., 2021). Moreover, the above approaches would consume large numbers of seagrass seeds when testing their viability. Meanwhile, the “squish/sink” method which commonly used to test viability in Zostera marina (Orth et al., 2012), does not fit H. ovalis seeds due to their seed’s small size (< 1mm). X-ray analysis is used widely in determining seed viability in terrestrial species and is a quick and non-destructive approach (Al-Turki and Baskin, 2017). Waite et al. (2021) compared this method with the squish/sink test to detect H. ovalis seed viability, and it proved to be substantially more efficient and less destructive.
The present study was aimed at testing the following hypotheses: (1) H. ovalis seeds have seasonal differences in spatial distribution and abundance; (2) viability of H. ovalis seeds from in situ seed banks were variable between sites; (3) spatial and temporal variability in salinity within the Swan-Canning Estuary was the main cause of loss of viability in H. ovalis seeds.
The study was conducted in the Swan-Canning Estuary, Southwestern Australia (Figure 1). The Swan and Canning rivers flow into Swan-Canning estuary and reach the Indian Ocean at Fremantle. This area covers around 40 km2, and salinities vary among sites (Webster et al., 2021). Point Roe site (RCK, 32°01′32″ 115°46′22″) is the site closest to the mouth of the Swan-Canning estuary and therefore more impacted by marine biota than the other sites. Freshwater Bay site (FB, 31°59′59″ 115°46′16″) is located downstream near the inlet of the estuary and therefore experiences near-oceanic salinities. Another site is located near the Pelican Point Marine Park (PPT, 32°02′31″ 115°47′20″) exposed to south westerly winds, and is a high use area for water sports. The Canning River site (CAN, 32°02′29″ 115°51′45″) is subjected to large salinity variability driven by rainfall (Kilminster and Forbes, 2014). All four sites contain H. ovalis, where water depths of H. ovalis meadows are less than 1 m during the low tide period, and were selected because they varied in salinity (Hillman et al., 1995; Kilminster and Forbes, 2014).
In the Swan-Canning estuary, H. ovalis starts flowering from early summer to late autumn. Fruits develop and seeds are released in summer and autumn (January-May, Figure S1). The frequency of reproduction varies between sites (Kilminster and Forbes, 2014). Once released, the seed immediately sinks to the sediment, with minimal capability for long distance dispersal by water movement (Kendrick et al., 2012, 2019). The salinity regime varies across upstream (W1, W2), middle (W3) and downstream (W4) sections of the estuary (Figure 1). The highest salinity occurs in summer (36-40 psu) due to minimal river flow and increased evaporation, while in winter the lowest salinity varies from 0 to10 psu as a result of rainfall, runoff and river flow (Webster et al., 2021).
Figure 1 Study sites (box) and the water quality monitoring (circle) across the Swan-Canning estuary (Webster et al., 2021). FB and RCK located at downstream, and PPT and CAN located at upstream.
Considering the short spatial dispersion of H. ovalis seeds, the Generalised Random Tessellation Stratified (GRTS) sampling design (Stevens and Olsen, 2004) was applied to investigate seed bank abundance at FB, PPT, and CAN within permanent plots. Sampling was carried out at four different times over an annual reproductive cycle of H. ovalis during 2014-2015: (1) May, after seed release at the end of the reproductive period and representing autumn conditions; (2) July, two months after seed release and representing winter conditions; (3) December, seven months after seed release and representing the end of spring condition; (4) January, nine months after seed release or before the next reproductive event, and representing summer conditions. At each of the 3 sites, a permanent plot of 16×16 m was established and divided into 64 sampling units within the plot. Each sampling unit was further subdivided in four sub-units, which were set up to avoid repeated sampling at the same point during the study. At each sampling time, a total of 60 core samples were collected in the sub-unit randomly within each site during the low tide period, and brought back to the laboratory in an incubator (Esky) on the same day (Figure 2). Sediment cores, made from PVC tubes with the size of 10cm diameter, 7 cm length, were then sieved with fresh water using two layers of sieves in the greenhouse. The first layer (1mm) removed the large particle of sediment and fragments of H. ovalis, whereas the second layer (710 μm) extracted H. ovalis seeds, small particles and decayed organic material. Then, the seeds were identified and counted under a microscope, and the numbers of shoots, flowers, fruits in cores were recorded to exhibit the reproductive performance of every site.
Figure 2 The layout of a permanent plot with 64 sampling units (2×2m2) and 256 subsampling units(1×1m2).
From the result of above investigation, the seed density in CAN was the lowest among the three sites, which could not support the following seed viability investigation. Thus, CAN was changed with RCK where H. ovalis seeds could be easier collected from sediment. We randomly sampled every two months from September 2019 to March 2020, and collected 60 sediment cores as above at every site. In the laboratory, the samples were sieved as above but with the use of sea water. The number of seeds, shoots, flowers, and fruits in cores were recorded, and seed viability was measured.
X-ray analysis was applied to examine H. ovalis seed viability following methods from Waite et al. (2021). The seeds from every site were separated into 4 groups. Then, we assessed the viability of these seeds by visualizing seed fill via X-ray analysis (MX-20 digital X-ray, Faxitron, Tucson, AZ, USA; and Leica M205C). The seeds were patted dry and placed onto a plate with plastic wells (one seed per well). According to the X-ray image, the seeds from sediment samples were classified as 7 types (Figure 3). It was reasonably assumed that the soft and dark seeds were non-viable, and the seeds with an intact seed coat were potentially viable seeds. The seed coat (Figure 3G) was ignored to avoid double counting as seed number. After analyzing H. ovalis seeds, the seeds displaying “intact coat” (Figure 3A), “intact endocarp” (Figure 3C) and “intact endosperm” (Figure 3D) from RCK samples were selected to test their viability via a germination experiment. All these seeds were divided into 30 seeds per replicate, four replicates per type, then cultured in one aquaculture tank for 1 month with red light, 30 psu salinity and 30°C. The germination rate, non-viable seeds (soft seed and dark seed, Figures 3E, F) were recorded every week (Waite et al., 2021).
Figure 3 The X-ray images and microscope photos of H. ovalis seeds. (A) intact seed coat; (B) intact endocarp with small-scale broken seed coat and intact endocarp; (C) intact endosperm and endocarp with large-scale broken seed coat; (D) intact endosperm without endocarp; (E) soft seed; (F) dark seed with unhealthy endosperm; (G) seed coat. (A–C) were considered as viable seeds, and (D–F) were considered as non-viable seeds.
To determine the effect of salinity changes on H. ovalis seed viability, the seeds were exposed to three different salinity conditions mimicking natural seasonal variability in the study area (Table S1). The lowest and highest salinity values in the Swan-Canning estuary were 2.04 psu in August 2018 (winter) and 37.07 psu in April 2019 (autumn), respectively. All the viable seeds came from mature fruits of H. ovalis, which were collected from PPT on the 30th Jan 2020 following Statton et al. (2017b). Groups made of 50 seeds was put into a single heat-sealed tulle mesh bag (Harwell and Orth, 1999). A total of 36 replicated bags were placed in fresh water tank (salinity 0.2 psu) and sea water tank (salinity 34.7 psu) for one week to simulated heavy rain fall and the otherwise habitual salinity during that period, respectively. Then, these bags were transported into salinity levels set at 5, 20, 40 psu for another 2 weeks. Each treatment level contained 6 replicate bags. Seed viability was checked in 3 bags with X-ray twice, once per week. All the incubations were under room temperature (22 °C) and red light (Ocean Optics, Largo, FL, USA). We recorded the dark seeds (Figure 3E) and soft seeds (Figure 3F) as non-viable seeds.
The differences in the abundance of seeds in the seed bank across the four sites (Factor 1) and over eight sampling times (Factor 2) were analyzed using a two-way ANOVA. When the interaction was significant, a simple effect test (a one-way ANOVA and Tukey’s multiple comparisons) was conducted (Zar, 1999). The differences of germination rates and non-viable rates among different seed states were arcsine transformed to normalize the distributions and checked for homogeneity of variances using Levene’s test (p > 0.05) with SPSS (version 21) before a one-way ANOVA. To estimate the number of sample and data precision in seed bank, we used the seed density data from FB (May, 2014). Sample size was estimated by assessing the behaviour of the standard error of seed density along a number of replicates varying from 3 to 50. The minimax algorithm revealed that the associated standard error stabilized at 40 replicates. Such value was taken as sample size for testing for potential differences in the abundance of seeds among H. ovalis seed banks. Moreover, to analyze seed spatial distribution, data were interpolated using the Simple Kriging method in Arc GIS version 10.3 software.
The seed distribution varied greatly between FB, PPT and CAN over an annual cycle (Figure 4). The seed density in FB was the highest among the three sites, and varied across the grid from 0 to 43590 seeds·m-2 and was more dense in the southern offshore area in May. The seeds in PPT were more dispersed than the other two sites, while seeds in CAN were mostly located at the offshore area. The maximum seed density in PPT and CAN decreased through time from 15513 seeds·m-2 and 6154 seeds·m-2 to 1026 seeds·m-2 and 513 seeds·m-2, respectively, indicating significate seed loss between mid-winter and summer at these sites.
Figure 4 Halophila ovalis seed bank spatial distribution of FB, PPT, and CAN over an annual reproductive cycle. The legend on the right side explains the number of seeds at each of the sites. The permanent plot has a size of 16×16 m2.
Based on the seed density in FB on May 2014, sample size as 40 was likely to have a stable standard error (SE) over replication across five times. Therefore, this sample size could set at 40 in the further H. ovalis seed bank investigation (Figure S2).
Based on the X-ray imagery, we classified 7 types of H. ovalis seeds from the sediment (Figure 3). After 1 month incubation and culture, the germination rates among intact coat seed, intact endocarp seed and intact endosperm seed were similar (Figure 5). However, the seeds without intact coat deteriorated easily, and the nonviable rate in the seed without seed coat and endocarp (Figure 3D) was the highest. Seeds with intact coat, or lacking coat but presenting intact endocarp were identified as viable seeds (healthy seeds, Figures 3A–C), while, soft seeds, or seeds with dark coat, or lacking endocarp but displaying intact endosperm were all considered as non-viable seeds (unhealthy seeds, Figures 3D–F).
Figure 5 The germination rate and non-viable rate of three types H. ovalis seeds after culturing in red light, 30 psu salinity and 30°C for 1 month (n=12). The black column represents the germination rate and the gray column represents the non-viable rate (mean ± SE). Different letters a, b, and c indicate significant differences among H. ovalis seed types in non-viable rate. And x represents no significant differences among these seed types in germination rate. The data were analyzed using one-way ANOVA.
There were significant differences in seed density in H. ovalis seed banks among RCK, FB, and PPT (p<0.001) in the late 2019 to early 2020 period. Seed densities in RCK and FB showed significant differences among seasons (pRCK<0.001, pFB<0.001), while the seed bank in PPT was relatively constant (p=0.072, Figure 6A). Moreover, the seed density in RCK was generally higher than in FB and PPT, and the seed density in PPT was the lowest among the three sites, indicating that a relatively higher salinity with less seasonal variation may contribute to higher seed survival rates (Figures 4, 6A and Table S1).
Figure 6 The seed density (n=720) and non-viable seed rate (n=48)in RCK, FB, and PPT during 2019-2020 (mean ± SE). (A) the seed density (seeds·m-2). (B) the non-viable seed rate (%). Different letters x, y, and z represent significant difference among the sites. Different letters a, b, and c indicate significantly different among the survey time in one site. And * indicates the significant difference between March and the other three investigations at PPT.
The density of non-viable seeds among the three sites was lower than 22%, and the differences among seasons were not statistically significant in RCK and FB (Figure 6B; pRCK=0.107, pFB=0.721) but it was in March for PPT. The density of non-viable seeds in March was significantly lower than the other three investigation times (p=0.01), because of the newborn seeds input (Figure 6B). Moreover, seeds with intact endosperm without endocarp contributed to the highest proportion of non-viable seeds in all three sites (Figure 3D).
Halophila ovalis shoots were recorded during all seasons in all four sites with varying densities across the 2014-2015 and the 2019-2020 seed bank assessments (Figure 7). The shoot density would change with the season conversion, the overall shoot density trend is autumn > summer > spring > winter. Moreover, the shoot density at CAN was also impacted by salinity change: the highest shoot density (3529 ± 167 shoots·m-2) was recorded in May with higher salinity, and decreased significantly in July and December (1212 ± 103 and 1245 ± 128 shoots·m-2, respectively) then increased in January (1871 ± 118 shoots·m-2) with the salinity increasing (Figure 7A).
Figure 7 The density of H. ovalis shoots and reproductive performance including flower and fruit density in the sites (mean ± SE, n=720). (A–C) showed the investigation results in FB, PPT, and CAN during 2014-2015; (D–F) showed the investigation results in RCK, FB, and PPT during 2019-2020.
Sexual reproduction started from November, and the number of flowers were highest in January in the Swan-Canning Estuary (Figures 7B, E). In general, higher reproductive performances were founded in CAN and PPT than FB and RCK, with less shoot density and more flower or fruits produced. However, the overwinter seed banks were larger in FB and RCK than the other two sites, which indicated low salinity exposure would hurt the H. ovalis seeds and resulted in reduction in seed banks over winter (Figure 6 and Table S1).
Treatments exposing the seeds to fresh water were applied to estimate the impacts of extremely low salinity (salinity 0.4 psu) on seed viability and seeds exposed to seawater was the control (Figure 8). Non-viable seeds were found in every treatment and were either dark seeds or soft seeds (Figures 3E, F). Moreover, the non-viable H. ovalis seeds when exposed to fresh water for one week were significantly greater than those in seawater, and more than half of the seeds (29 ± 7 seeds from 50 seeds) were non-viable in the treatment “freshwater to 5 psu salinity” after culturing for 14 days. Whereas cultured at a relatively high salinity (≧20 psu) the non-viable numbers were reduced within 7 days (p=0.02). The seeds in these treatments showed no statistically significant differences in viability after 14 days (p= 0.6, Figure 8). The non-viable seeds in the freshwater pre-treatment and the low salinity recovery treatment were significantly greater than other salinities indicating that low salinity which is commonly found in winter across Swan-Canning Estuary would result in loss of seeds in the H. ovalis seed banks.
Figure 8 Non-viable seed rate after exposing to different salinity (mean ± SE). (A) non-viable seeds after culturing for 7days (n=18); (B) non-viable seeds after culturing for 14 days(n=18). Different letters a and b indicate significant differences among different exposure salinities. * represents significant between fresh water and sea water treatment. The data were analyzed using one-way ANOVA.
Seed density in seed banks of the Swan-Canning Estuary dramatically varied annually and among sites within the estuary. High seed densities always occurred towards the end of reproduction in austral autumn and through to mid-winter. By the spring and summer months, after reestablishment of meadows from seed in spring, seed bank numbers are significantly lower. Similar seasonal patterns in seed densities in seed banks have been described from tropical H. decipiens in Australia (Hovey et al., 2015) and seems to be a strategy for persistence of colonizing seagrasses under strong seasonal environmental forcing, worldwide (Kilminster et al., 2015; Kendrick et al., 2017).
Most of Halophila seed bank studies applied a random or stratified random survey to collect samples, but a random survey design is likely to generate clumped sampling (Jongman et al., 2006). Stratified random sampling is more reliable to increase the precision of the samples in a diverse community (Benoit et al., 1989; Jongman et al., 2006). However, this method may not be appropriate if the seagrass community is distributed in an environmental gradient such as salinity. Differing from the methods above, we used GRTS method introduced by Stevens and Olsen (2004), which benefit to collect a random distribution of sample points and provides more advantages such as preserving the neighbouring distances between sampling points, and reducing sample variance by using a local variance estimator such as local neighborhood-based. According to the results of FB, PPT, and CAN over an annual reproductive cycle, the H. ovalis seeds in the seed bank always shows high spatial variability, thus, the sample collection along and across transects was more representative than random collections. Moreover, the minimum efficient sample number was 40, which was enough to describe the seed bank distribution of H. ovalis seed bank in Swan-Canning Estuary.
High seed viability is essential for rapid and uniform plant establishment (Orth et al., 2000). Physiological characteristics of seeds such as those active to break dormancy and for germination are generally closely linked to specific environmental conditions and are different among seagrass species (Xu et al., 2016; Statton et al., 2017b; Waite et al., 2021). A number of methods applied in seagrass seed viability testing have been based on visual inspection using squash and sink tests and tetrazolium chloride staining, that are destructive and time consuming (Gu et al., 2018; Jarvis et al., 2021). X-ray analysis which was widely used in the terrestrial seed industry was applied for seagrass seed inspection recently (Kotwaliwale et al., 2014; Huang et al., 2015; Waite et al., 2021). The main advantage of this technique is its ability to non-destructively obtain information on the internal seed morphology (de Medeiros et al., 2021). This method could only be generalized in desiccation tolerance seed of seagrass species such as H. ovalis and Ruppia as seeds need to be dry because water disturbs the X-ray transmission. (Waite et al., 2021; Gu et al., 2018). Moreover, the interpretation of X-ray images is a subjective and tedious task by seed analysts. Hence, we propose development of a deep learning-based, semi-automated or automated image analysis method.
To develop the seed viability method with X-ray analysis, a detail learning of seed X-ray images was conducted. In general, the H. ovalis seed consists of endosperm, endocarp and seed coat. X-ray images of newly produced H. ovalis seeds (Waite et al., 2021) differ from the seeds in the seed bank, and seeds collected from the sediment are more variable. The result of the indoor germination experiment showed all three seed types had similar seed germination rates. However, the non-viable rate in these three seed types indicated seeds without any cover (only intact endosperm) were the most vulnerable seed type. Based on the X-ray images, the viability of newly produced H. ovalis seeds were over 97.46% (Waite et al., 2021) while, the viability of seed bank seeds was lower, approximately 77.95%.
The seasonal cycle of seed germination of H. ovalis in the Swan-River Estuary typically occurs over a period of 4-6 months after seed release, during austral spring, when the rivers flood the estuary resulting in a light shift toward yellow and red wavelengths, and as spring temperatures gradually increase, the seeds start to germinate (Statton et al., 2017b). The precipitation can exceptionally reach up to 114 mm/day in this region, resulting in the estuary salinity sharply decreasing and reaching minimum values in a week or less (Webster et al., 2021). Low salinity and relative high temperature could promote seagrass seed germination (Orth et al., 2000; Xu et al., 2016; Gu et al., 2017; Yue et al., 2019). In such conditions, H. ovalis seeds in the sediment quickly germinate, while the environmental conditions are not suitable for seedling establishment, resulting in losses to the seed bank and less effective recruitment the following spring (Statton et al., 2017a; Waite et al., 2021; Webster et al., 2021). Also, it was here demonstrated in an experiment that freshwater inputs over short-time periods contribute to significant declines in seed viability, and over 20% of seeds lose viability following a pulse of freshwater of a week.
H. ovalis is the most widely distributed species of seagrass in the Swan-Canning Estuary. Its fruit production occurs in the summer months, between January and March, and seed germination occurs in spring, around October (Webster et al., 2021). Other researchers investigated the optimal environmental conditions for H. ovalis seed germination, and found the relative low temperature (15 °C) and high salinity (over 20 psu) limited seed germination, prompting seed storage (Figure 8, Statton et al., 2017a). Red light (peak λ=673 mm) enhanced H. ovalis seed germination, growth and survival (Strydom et al., 2017). Combining this information, we suggest a management and restoration strategy where fruit would be harvested from a site with high seed production followed by storage in the dark in ocean salinities and temperature <15°C then break dormancy through culturing in a gradient changing temperature (such as 15°C-20°C-25°C) in red light (Statton et al., 2017b; Waite et al., 2021). These germinated H. ovalis seeds could be then used to replenish the seed bank after heavy rainfall periods to avoid seed loss caused by extremely low salinity exposure, and increase the potential of seasonal bed recovery.
This study contributed to our knowledge of H. ovalis seed bank dynamics through insights into its seasonal seed abundance, spatial distribution, and seed viability. And we provided evidence to support our hypothesis that extremely low salinity would result in seed loss from the seed bank through promoting seed germination or decreasing seed viability. We also demonstrated a potential restoration plan based on H. ovalis seeds, while, this plan still needs further practice to estimate its feasibility. In addition, we used the X-ray analysis to test seed viability of the H. ovalis seed bank, added more X-ray images of H. ovalis seed, and have taken a step further with more evidence of the utility of this technique, which is however labour intensive. Thus, future studies are needed to improve the efficiency of the technique for seagrass seeds, including automatic image recognition.
The original contributions presented in the study are included in the article/Supplementary Material. Further inquiries can be directed to the corresponding author.
RG: Conceptualization, investigation, methodology, writing original draft. JS: Conceptualization, investigation, methodology. SR: Investigation, methodology. RH: Methodology. YZ: Funding acquisition. JT: Funding acquisition. SY: Writing - review & editing. GK: Funding acquisition, writing - review & editing. All authors contributed to the article and approved the submitted version.
This research was supported by National Natural Science Foundation of China (42141016), Guangxi Natural Science Foundation (2019GXNSFBA185033) and Australian Research Council Linage grant (LP160101011) to JS and GK.
We would like to thank Rachel Austin, Brenna Waite and Shannon Duffy for the help in the field survey, and Michael Just for providing X-ray technology.
The authors declare that the research was conducted in the absence of any commercial or financial relationships that could be construed as a potential conflict of interest.
All claims expressed in this article are solely those of the authors and do not necessarily represent those of their affiliated organizations, or those of the publisher, the editors and the reviewers. Any product that may be evaluated in this article, or claim that may be made by its manufacturer, is not guaranteed or endorsed by the publisher.
The Supplementary Material for this article can be found online at: https://www.frontiersin.org/articles/10.3389/fmars.2022.1025615/full#supplementary-material
Al-Turki T. A., Baskin C. C. (2017). Determination of seed viability of eight wild Saudi arabian species by germination and X-ray tests. Saudi J. Biol. Sci. 24 (4), 822–829. doi: 10.1016/j.sjbs.2016.06.009
Barbier E. B., Hacker S. D., Kennedy C., Koch E. W., Stier A. C., Silliman B. R. (2011). The value of estuarine and coastal ecosystem services. Ecol. Monogr. 81, 169–193. doi: 10.1890/10-1510.1
Benoit D. L., Kenkel N. C., Cavers P. B. (1989). Factors influencing the precision of soil seed bank estimated. Can. J. Bot. 67, 2833–2840. doi: 10.1139/b89-364
Cho H. J., Sanders Y. L. (2009). Note on organic dormancy of estuarine Ruppia maritima l. seeds. Hydrobiologia 617, 197–201. doi: 10.1007/s10750-008-9541-7
de Medeiros A. D., Bernardes R. C., da Silva L. J., de Freitas B. A. L., dos Santos Dias D. C. F., da Silva C. B. (2021). Deep learning-based approach using X-ray images for classifying Crambe abyssinica seed quality. Ind. Crops Products 164, 113378. doi: 10.1016/j.indcrop.2021.113378
Fenner M., Thompson K. (2005). Spatial analysis: A guide for ecologists. (Cambridge:Cambridge University Press).
Fourqurean J. W., Duarte C. M., Kennedy H., Marbà N., Holmer M., Mateo M. A., et al. (2012). Seagrass ecosystems as a globally significant carbon stock. Nat. Geosci. 5, 505–509. doi: 10.1038/ngeo1477
Gu R. T., Zhou Y., Song X. Y., Xu S. C., Zhang X. M., Lin H. Y., et al. (2018). Tolerance of Ruppia sinensis seeds to desiccation, low temperature, and high salinity with special reference to long-term seed storage. Front. Plant Sci. 9. doi: 10.3389/fpls.2018.00221
Gu R. T., Zhou Y., Song X. Y., Xu S. C., Zhang X. M., Lin H. Y., et al. (2017). Effects of temperature and salinity on Ruppia sinensis seed germination, seedling establishment, and seedling growth. Mar. pollut. Bull. 134, 177–185. doi: 10.1016/j.marpolbul.2017.08.013
Harwell M. C., Orth R. J. (1999). Eelgrass (Zostera marina l.) seed protection for field experiments and implications for large-scale restoration. Aquat. Bot. 64 (1), 51–61. doi: 10.1016/S0304-3770(99)00008-X
Harwell M. C., Orth R. J. (2002). Seed bank patterns in Chesapeake bay eelgrass (Zostera marina l.): A bay-wide perspective. Estuaries 25, 1196–1204. doi: 10.1007/BF02692216
Hillman K., McComb A. J., Walker D. I. (1995). The distribution, biomass and primary production of seagrass Halophila ovalis in the Swan/Canning estuary, Western Australia. Aquat. Bot. 51, 1–54. doi: 10.1016/0304-3770(95)00466-D
Hovey R. K., Statton J., Fraser M. W., Ruiz-Montoya L., Zavala-Perez A., Rees M., et al. (2015). Strategy for assessing impacts in ephemeral tropical seagrasses. Mar. pollut. Bull. 101, 594–599. doi: 10.1016/j.marpolbul.2015.10.054
Huang M., Wang Q. G., Zhu Q. B., Qin J. W., Huang G. (2015). Review of seed quality and safety tests using optical sensing technologies. Seed Sci. Technol. 43, 337–366. doi: 10.15258/sst.2015.43.3.16
Inglis G. J. (2000). Disturbance-related heterogeneity in the seed banks of a marine angiosperm. J. Ecol. 88, 88–99. doi: 10.1046/j.1365-2745.2000.00433.x
Jarvis J. C., McKenna S. A., Rasheed M. A. (2021). Seagrass seed bank spatial structure and function following a large-scale decline. Mar. Ecol. Prog. Ser. 665, 75–87. doi: 10.3354/meps13668
Jongman R. H. G., Bunce R. G. H., Metzger M. J., Mücher C. A., Howard D. C., Mateus V. L. (2006). Objectives and applications of a statistical environmental stratification of Europe. Landscape Ecol. 21, 409–419. doi: 10.1007/s10980-005-6428-0
Kendrick G. A., Orth R. J., Statton J., Hovey R., Ruiz Montoya L., Lowe R. J., et al. (2017). Demographic and genetic connectivity: The role and consequences of reproduction, dispersal and recruitment in seagrasses. Biol. Rev. 92, 921–938. doi: 10.1111/brv.12261
Kendrick G. A., Pomeroy A. W., Orth R. J., Marion L. C., Jeremy S., Lukasz K., et al. (2019). A novel adaptation facilitates seed establishment under marine turbulent flows. Sci. Rep. 9, 19693. doi: 10.1038/s41598-019-56202-7
Kendrick G. A., Waycott M., Carruthers T. J. B., Cambridge M. L., Hovey R., Krauss S. L., et al. (2012). The central role of dispersal in the maintenance and persistence of seagrass populations. BioScience 62, 56–65. doi: 10.1525/bio.2012.62.1.10
Kilminster and Forbes (2014). Seagrass as an indicator of estuary condition in the Swan-Canning estuary, Water Science Technical Series, report no. 62, Department of Water, Western Australia
Kilminster K., Forbes V., Holmer M. (2014). Development of a “sediment-stress” functional-level indicator for the seagrass halophila ovalis. Ecol. Indic. 36, 280–289. doi: 10.1016/j.ecolind.2013.07.026
Kilminster K., McMahon K., Waycott M., Kendrick G. A., Scanes P., Mckenzie L., et al. (2015). Unravelling complexity in seagrass systems for management: Australia as a microcosm. Sci. Total Environ. 534, 97–109. doi: 10.1016/j.scitotenv.2015.04.061
Kotwaliwale N., Singh K., Kalne A., Jha S. N., Seth N., Kar A. (2014). X-Ray imaging methods for internal quality evaluation of agricultural produce. J. Food Sci. Technol. 51, 1–15. doi: 10.1007/s13197-011-0485-y
Kuo J., Kirkman H. (1992). Fruits, seeds and germination in the seagrass Halophila ovalis (Hydrocharitaceae). Botanica Marina 35, 197–204. doi: 10.1515/botm.1992.35.3.197
Máñez-Crespo J., Tuya F., Fernández-Torquemada Y., Royo L., Pilar-Ruso Y. D., Espino F., et al. (2020). Seagrass Cymodocea nodosa across biogeographical regions and times: Differences in abundance, meadow structure and sexual reproduction. Mar. Environ. Res. 162, 105159. doi: 10.1016/j.marenvres.2020.105159
Marion S. R., Orth R. J. (2010). Innovative techniques for large-scale seagrass restoration using Zostera marina (eelgrass) seeds. Restor. Ecol. 18, 514–526. doi: 10.1111/j.1526-100X.2010.00692.x
Orth R. J., Carruthers T. J. B., Dennison W. C., Duarte C. M., Fourqurean J. W., Heck K. L., et al. (2006a). A global crisis for seagrass ecosystems. Bioscience 56, 987–996. doi: 10.1641/0006-3568(2006)56[987:AGCFSE]2.0.CO;2
Orth R. J., Harwell M. C., Bailey E. M., Bartholomew A., Jawad J. T., Lombana A. V., et al. (2000). A review of issues in seagrass seed dormancy and germination: implications for conservation and restoration. Mar. Ecol. Prog. Ser. 200, 277–288. doi: 10.3354/meps200277
Orth R. J., Kendrick G. A., Marion S. R. (2006c). Predation on Posidonia australis seeds in seagrass habitats of rottnest island, Western Australia: patterns and predators. Mar. Ecol. Prog. Ser. 313, 105–114. doi: 10.3354/meps313105
Orth R. J., Luckenbach M. L., Marion S. R., Moore K. A., Wilcox D. J.. (2006b). Seagrass recovery of in the Delmarva coastal bays, USA. Aquat. Bot. 84, 26–36. doi: 10.3354/meps09522
Orth R. J., Moore S. R., Marion D. J. (2012). Seed addition facilitates eelgrass recovery in a coastal bay system. Mar. Ecol. Prog. Ser. 448, 177–195. doi: 10.3354/meps09522
Reynolds L. K., Waycott M., McGlathery K. J. (2013). Restoration recovers population structure and landscape genetic connectivity in a dispersal-limited ecosystem. J. Ecol. 101, 1288–1297. doi: 10.1111/1365-2745.12116
Sawma J., Mohler C. (2002). Evaluating seed viability by an unimbibed seed crush test in comparison with the tetrazolium test. Weed Technol. 16, 781–786. doi: 10.1614/0890-037X(2002)016[0781:ESVBAU]2.0.CO;2
Smith T. M., York P. H., Macreadie P. I., Keough M. J., Ross D. J., Sherman C. D. H. (2016). Spatial variation in reproductive effort of a southern Australian seagrass. Mar. Environ. Res. 120, 214–224. doi: 10.1016/j.marenvres.2016.08.010
Statton J., Montoya L. R., Orth R. J., Dixon K. W., Kendrick G. A. (2017a). Identifying critical recruitment bottlenecks limiting seedling establishment in a degraded seagrass ecosystem. Sci. Rep. 7 (1), 1–12. doi: 10.1038/s41598-017-13833-y
Statton J., Sellers R., Dixon K. W., Kilminster K., Merritt D. J., Kendrick G. A. (2017b). Seed dormancy and germination of Halophila ovalis mediated by simulated seasonal temperature changes. Estuarine Coast. Shelf Sci. 198, 156–162. doi: 10.1016/j.ecss.2017.08.045
Stevens D. L., Olsen A. R. (2004). Spatially balanced sampling of natural resources. J. Am. Stat. Assoc. 99, 262–278. doi: 10.1198/016214504000000250
Strydom S., McMahon K., Kendrick G. A., Stratton J., Lavery P. (2017). Seagrass Halophila ovalis is affected by light quality across different life history stages. Mar. Ecol. Prog. Ser. 572, 103–116. doi: 10.3354/meps12105
Tanner C. E., Parham T. (2010). Growing Zostera marina (eelgrass) from seeds in landbased culture systems for use in restoration projects. Restor. Ecol. 18, 527–537. doi: 10.1111/j.1526-100X.2010.00693.x
Vercaemer B. M., Scarrow M. A., Roethlisberger B., Krumansl K. A., Wong M. C. (2021). Reproductive ecology of Zostera marina l. (eelgrass) across varying environmental conditions. Aquat. Bot. 175, 103444. doi: 10.1016/j.aquabot.2021.103444
Waite B., Statton J., Kendrick G. A. (2021). Temperature stratification and monochromatic light break dormancy and facilitate on-demand in situ germination in the seagrass Halophila ovalis, with seed viability determined by a novel X-ray analysis. Estuaries Coasts 44, 412–421. doi: 10.1007/s12237-020-00842-w
Webster C. L., Kilminster K. L., Alarcón S. M., Bennett K., Strydom S., McNamara S., et al. (2021). Population-specific resilience of Halophila ovalis seagrass habitat to unseasonal rainfall, an extreme climate event in estuaries. J. Ecol. 00, 1–20. doi: 10.1111/1365-2745.13648
Wu K., Chen C. N., Soong K. (2016). Long distance dispersal potential of two seagrasses Thalassia hemprichii and Halophila ovalis. PloS One 11 (6), e0156585. doi: 10.1371/journal.pone.0156585
Xu S. C., Zhou Y., Wang P. M., Wang F., Zhang X. M., Gu R. T. (2016). Salinity and temperature significantly influence seed germination, seedling establishment, and seedling growth of eelgrass Zostera marina l. PeerJ 4, e2697. doi: 10.7717/peerj.2697
Yue S. D., Zhang X. M., Xu S. C., Zhang Y., Zhao P., Wang X. D., et al. (2020). Reproductive strategies of the seagrass Zostera japonica under different geographic conditions in northern China. Front. Mar. Sci. 07. doi: 10.3389/fmars.2020.574790
Yue S. D., Zhou Y., Zhang Y., Xu S. C., Gu R. T., Xu S., et al. (2019). Effects of salinity and temperature on seed germination and seedling establishment in the endangered seagrass Zostera japonica asch. & graebn. in northern China. Mar. pollut. Bull. 146, 848–856. doi: 10.1016/j.marpolbul.2019.07.037
Keywords: seed viability, salinity, seasonal variation, seed spatial dispersion, X-ray
Citation: Gu R, Statton J, Rahmawati S, Hovey R, Zhou Y, Tang J, Yu S and Kendrick GA (2022) Seed bank dynamics and quality in the seagrass Halophila ovalis along estuarine salinity gradients—a case in the Swan-Canning Estuary. Front. Mar. Sci. 9:1025615. doi: 10.3389/fmars.2022.1025615
Received: 23 August 2022; Accepted: 24 October 2022;
Published: 22 November 2022.
Edited by:
Renato Crespo Pereira, Fluminense Federal University, BrazilReviewed by:
Sasi Nayar, South Australian Research and Development Institute, AustraliaCopyright © 2022 Gu, Statton, Rahmawati, Hovey, Zhou, Tang, Yu and Kendrick. This is an open-access article distributed under the terms of the Creative Commons Attribution License (CC BY). The use, distribution or reproduction in other forums is permitted, provided the original author(s) and the copyright owner(s) are credited and that the original publication in this journal is cited, in accordance with accepted academic practice. No use, distribution or reproduction is permitted which does not comply with these terms.
*Correspondence: Jianwu Tang, and0YW5nQHNrbGVjLmVjbnUuZWR1LmNu
Disclaimer: All claims expressed in this article are solely those of the authors and do not necessarily represent those of their affiliated organizations, or those of the publisher, the editors and the reviewers. Any product that may be evaluated in this article or claim that may be made by its manufacturer is not guaranteed or endorsed by the publisher.
Research integrity at Frontiers
Learn more about the work of our research integrity team to safeguard the quality of each article we publish.