- 1Frontiers Science Center for Deep Ocean Multispheres and Earth System, and Key Laboratory of Marine Chemistry Theory and Technology, Ministry of Education, Ocean University of China, Qingdao, China
- 2College of Chemistry and Chemical Engineering, Ocean University of China, Qingdao, China
- 3North China Sea Environmental Monitoring Center, State Oceanic Administration, Qingdao, China
- 4China Petrochemical Corporation (Sinopec Group), Beijing, China
The migration and transformation of the petroleum hydrocarbons from sediments into water columns is an important basis for assessing the impact on marine environment. In this paper, a static release experiment of crude oils from the Bohai Sea was carried out to evaluate the temporal and spatial variation of petroleum hydrocarbons and microbial communities. The results showed C1 phenanthrene (C1-P) can be used as an evaluation index of different crude oils during static release of oil pollution sediments and their trends of the static release were similar. The crude oil with higher C1-P content released C1-P into the water body significantly higher. After 72 hours, the C1-P release degree of crude oil with a smaller viscosity was more obvious. In the crude oil with the smallest viscosity and the higher viscosity, the bacterial phyla abundance increase was greater. And more importantly, the top 10 abundance of the microbial communities in the water columns and sediments appeared partial (3 species) replacement phenomenon. The research results can deep understanding the migration and transformation of the petroleum hydrocarbons from oil pollution sediments and understanding of the interaction between extreme weather events and human activities by incorporating an eco-evolutionary perspective.
1 Introduction
In the process of offshore oil exploration and development, maritime transportation, and coastal oil refining, marine oil spills happen frequently (Zhang et al., 2019; Wang et al., 2020). After a large amount of oil spills into the ocean, many components in the oil will exist for a long time in the marine environment (Kingston, 2002; Nelson and Grubesic, 2017; Passow and Lee, 2022). These petroleum hydrocarbons produce toxins to shellfish and fish in the sea, and the toxins will eventually endanger human health through the accumulation of the food chain (Farrington, 2014; Okafor-Yarwood, 2018; Zhang et al., 2021). It is worth noting that petroleum hydrocarbons in sediments are continuously released into the water column, playing an important role in determining the transport and destination of oil spills. Gradually, the marine environment may be damaged (Blumer et al., 1971; Gui et al., 2022). Therefore, marine oil spills remain a global concern (Li et al., 2018; Li et al., 2020).
When a marine oil spill occurs, the oil spill will undergo a series of physical, chemical, and biological process changes (Gong et al., 2014; Narimannejad et al., 2019). In this series of changes, evaporation of oil components is frequently the major process when hydrocarbons are loss from spilled oil (Lee, 2002). Subsequently, the fate of petroleum components are related to solubilization, biodegradation, adsorption/desorption of sediments and suspended particulate matter, and dilution in water bodies (Urum and Pekdemir, 2004; Khamehchiyan et al., 2007; Li and Li, 2011; Wu et al., 2013; Yang et al., 2019). It’s worth noting that the dissolved oil components will be incorporated into the bottom sediments for a long time (Yamada et al., 2003; Li et al., 2015; Saracino et al., 2017; Shi et al., 2018). The research on the oil spill in West Falmouth shows that nearly 40 years after the oil spill, there is still plenty of oil at 8-20 cm below the surface of the sediment (Culbertson et al., 2007). The oil presenting in the sediment may be slowly released into the water columns (Chao et al., 2003). In the past few decades, researchers have mainly studied the adsorption-desorption behavior of crude oil or part of polycyclic aromatic hydrocarbons (PAHs) on sediment through batch experiments, Tenax beads, biosurfactants, and oil dispersants (Cao et al., 2011; Cao et al., 2013). However, these studies cannot evaluate the oil release process in the sediment-water system (Morgan and Watkinson, 1989; Dong et al., 2019). Hence, valuate the migration and transformation of the petroleum hydrocarbons from sediments into water columns is important to systematically assess the impact of oil spill on marine environment.
Recently, the oil release process and dynamics was simulated in a sediment-seawater system with reproductive heavy oil-contaminated sediment. The results showed the Dissolved Organic Matter (DOM) increase the oil solubility in the overlying water and compete with oil molecules for adsorption to the sediment surface (Yuan et al., 2017). The static release process is a first-order kinetic process, and the higher the pollution intensity of sediments, the higher the saturated concentration of petroleum hydrocarbons in the water body, and the faster the release rate (Dong et al., 2019). With the increase in temperature, the first-order rate constant increases, but it is not affected by DOM and salinity (Yuan et al., 2017). These results can be used to better understand the fate of heavy oil in the contaminated sediments. At the same time, there are a large number of bacteria and fungi that have the ability to degrade petroleum hydrocarbons’ compounds on the ocean (Kimes et al., 2014). The main species of oil degradable bacteria had been found to be Bacillus, Micrococcus, Pseudomonas and Actinomyces, Nocardia, Golden Streptomyces, Pseudomycetes, Red Ferment, Sporotrichum, Aspergillus, Trichoderma, Fusarium and Penicillium etc (Arvanitis et al., 2008; Vila et al., 2010; Yu et al., 2014). Microbes can degrade petroleum hydrocarbons into carbon dioxide and water (Head and Swannell, 1999; Li et al., 2016; Li et al., 2018; Wang et al., 2018). The biodegradation of petroleum compounds consists of three processes. First, petroleum compounds are adsorbed to the surface of microorganisms; Second, these petroleum compounds are transferred to microbial cell membranes; These compounds are then degraded in microbial cells, eventually breaking down into various small molecules (Das and Chandran, 2011). The comprehensive study of petroleum hydrocarbons and microbial community changes, especially in the petroleum hydrocarbons release process from sediments into water columns is still rare.
In this paper, a static release experiment of oil pollution sediments (crude oils from the Bohai Sea) was carried out, and the release of C1 phenanthrene was used as a representative indicator for the migration and evaluation of petroleum hydrocarbons, and the distribution characteristics of petroleum hydrocarbons released by different crude oils into water bodies were analyzed. At the same time, diversity and composition of microbial communities in water and sediments before and after the experiment were initially explored. The research results can provide a basis for oil spill emergency response and impact assessment and deep understanding of the interaction between extreme weather events and human activities.
2 Materials and methods
2.1 Materials
Three different crude oils from the Bohai Sea (China) were selected to evaluate the temporal and spatial variation of petroleum hydrocarbons and microbial communities over time, depending on the type of pollutant. According to the viscosity at 25°C, the three crude oils were classified into type I oil (R crude oil with a viscosity of 763.3 mPa·s), type II oil (U crude oil with a viscosity of 1147.8 mPa·s) and type III oil (T crude oil with a viscosity of 12985 mPa·s). The more detailed physical and chemical information of the three crude oils was shown in Table S1.
Natural seawater (pH 7.50, salinity 29.1 and density 1.01 g•cm-3) from the sea area of Qingdao Zhongyuan Wharf was processed by speedy quantitative filter paper to remove suspended droplets and the majority of planktons and stored under refrigeration (0°C~4°C). The sediments were taken from the coastal waters of Dongying Port in Bohai Sea and then stored under refrigeration (0°C~4°C). The granularity of the sediments belonged to granularity. TOC and TPHs were 0.50×10-6 mg/L and 20.3×10-6 mg/L respectively. The more detailed information can be found in Table S2.
2.2 Experimental simulated device
In order to simulate the process of releasing petroleum hydrocarbons from seabed sediments to water bodies to the maximum extent in the laboratory, we designed a set of simulation devices (Figure S1) independently, and carried out static petroleum hydrocarbons release experiments based on the typical oil products, sediments and natural sea water in Bohai Sea. The main body of the simulation experiment device is 4 columns with a height of 100 cm and a diameter of 19 cm, and each column could hold 23.8L water. Besides, each column is provided with 4 sampling ports with a vertical interval of 20 cm, and the top is equipped with a lid for sealing. In addition, in order to spread the sediment evenly at the bottom of the device, the device is equipped with 100 cm long poles, and the top of which is fixed with 18 cm diameter and 1 cm thickness cylinder (mainly made of quartz).
2.3 Experimental method
2.3.1 Oil pollution sediment aging treatment
Based on the investigation results of the Penglai 19-3 accident in the Bohai Sea of China (Wang et al., 2018), the maximum petroleum hydrocarbons content in the sediment was 7100 mg/kg. In the literature (Yuan et al., 2017; Dong et al., 2019), the petroleum hydrocarbons content of sediment used in similar experiments carried out was 464, 734, 1239, 2317, 4382 and 9196 mg/kg. In order to simulate the static release process of petroleum hydrocarbons more truly in the sediments of Bohai Sea, and considering that the obtained results can play a certain reference role in the study of similar experiments in other areas, with reference to the two, we determined that the petroleum hydrocarbons content of the sediment in this experiment was 8000 mg/kg.
2.65 g three crude oils dissolved respectively in n-hexane were stirred and mixed uniformly with 331.7 g Bohai Sea sediments (dry sediment). They were placed in a fume cupboard to completely volatilize the n-hexane, and then refrigerated at 4°C in the dark. The blank test with the same amount of hexane under the same conditions to make sure the hexane is completely evaporated. All the sediment in each sample were taken on 1 day, 5 days, 9 days, 11 days, and 13 days to simulate aging over time. The blank control samples were taken on 1 day and 13 days. Each sample was added with 50 mL distilled water for 30 minutes static settlement. The water sample was poured into a separatory funnel with 10 mL n-hexane and extracted by shaking twice for 5 minutes each time. The extract was uniformly measured with an ultraviolet spectrophotometer, and the petroleum hydrocarbons concentration released into the water from the oily sediments was calculated from the previous crude oil standard curve (Figure S2). Due to the different viscosity and other properties of the three kinds oil, although the mixing of oil and sediment has reached the maximum possible homogenization, it can be seen from the data that the fluctuation of petroleum hydrocarbons released by the three kinds oils into water was still large. The focus of this paper is to investigate temporal and spatial variation of petroleum hydrocarbons and microbial communities during static release of oil pollution of sediments. Hence, the oil release of T crude oil has reached equilibrium at 9 days, so the best aging time we chose was 9 days.
2.3.2 Static release simulation experiment of oil pollution sediments
Three experimental groups and one blank control group were set up in static release simulation experiment, which were oil spill sediment group prepared by U crude oil, oil spill sediment group prepared by R crude oil, oil spill sediment group prepared by T crude oil and natural sediment release group respectively. The aged greasy sediment (2 cm thick) based on the above settings was evenly spread at the bottom of the four columns of the simulated experimental device, and then natural seawater was slowly added to a height of 100 cm, so as not to disturb the bottom sediment as much as possible. The static release experiment of petroleum hydrocarbons was carried out at 25°C for 240 h (10 days). 50 mL Samples (twice) were taken at 1 h, 3 h, 5 h, 10 h, 24 h (1 day), 48 h (2 days), 72 h (3 days), 120 h (5 days), 192 h (8 days), and 240 h (10 days), respectively. The water sample were extracted with 5 mL n-hexane twice, oscillated for 4-5 minutes each time, and stood for 5 minutes. We ensure maximum accuracy and parallelism of sample extraction by taking the water samples at different times twice. And each sample was extracted twice to reduce the great uncertainty as soon as possible. The extraction solution was placed in a 20 mL brown bottle for cold storage. At the end of the static release experiment, 500~1000 mL of the remaining water samples from the four columns were taken out and filtered with 0.25 μm filter membrane respectively. The above static release process of oil pollution sediment was used to valuate petroleum hydrocarbons from sediment to water columns to reflect partitioning of oil components. Meantime, this also laid the groundwork for the future study during oil spills. The water samples were stored in a centrifuge tube as AW.1, AW.2, UW.1, UW.2, RW.1, RW.2, TW.1 and TW.2. Subsequently, 150 g of sediment was taken from the bottom of the four columns respectively and encapsulated in 50 mL centrifuge tube as AS.1, AS.2, US.1, US.2, RS.1, RS.2, TS.1 and TS.2. The water samples and sediment samples were stored for ultra-low temperature refrigeration at -80°C. In addition, take the same amount of original sediments, original natural seawater and freeze-dried sediment two copies, respectively numbered as OS.1, OS.2S, SW.1, SW.2, FD.1 and S.FD.2. Prepare with the above samples for microbial communities analysis.
2.4 Microbial communities analysis
The microbial communities in all the sediment samples and water samples were analyzed by high throughput sequencing analyses. The total DNA was extracted using a FastDNA SPIN Kit (MP, USA). The primer 515F (5′-GTGCCAGCMGCCGCGGTAA-3′) and 907R (5′-CCGTCAATTCCTTTGAGTTT-3′) were used to amplify the hypervariable fragments of the bacterial 16S rRNA. All samples were analyzed successively by genomic DNA extraction and PCR amplification, PCR product mixed purification, library construction and computer sequencing, information analysis, sequencing data processing, OTU clustering and species annotation, species relative abundance display, data processing and mapping analysis. Based on the above data, microbial communities were comprehensively analyzed (Fuhrman et al., 2015).
2.5 Petroleum hydrocarbons analysis
The 10 mL petroleum hydrocarbons extract obtained with n-hexanewas in water samples and sediments during static release simulation experiment concentrated to about 1 mL by nitrogen blowing. Subsequently, 20 μL three internal standards (101 ug/mL C24D50, 101 ug/mL 5α-androstane, and 9.9 ug/mL terphenyl-D14) were added respectively into extract and calibrate them to 1 mL. Petroleum hydrocarbons were analyzed using Gas Chromatograph (DB-5MS fused silica column 30 m x 0.32 mm id 0.25 μm film thickness, Supelco Canada) and Mass Spectrometer (GC-MS) (QP2010, Shimadzu Japan). The GC-MS detailed conditions with reference to Li et al. (2020): Helium was used as carrier gas at a flow rate of 1-2.5 mL/min; The inlet temperature was 290-300°C; The temperature of the detector was 300-310°C; The heating procedure was to keep at 50°C for 2 min, then increase to 300°C at the rate of 6°C/min, and keep at 300°C for 16 min. The injection volume was 1 μL; The injection method was not split; The injection time was 1 min. The main characteristic ions of samples detected were shown in Table S3. The relative peak area of the relative internal standard for each component was calculated for petroleum hydrocarbons analysis.
3 Results and discussion
3.1 Identification and screening of petroleum hydrocarbons components in water during static release process
In order to reduce the amount of water in the columns due to excessive sampling volume, which affects the study of the release process, so this time each sampling volume is 50 mL. The amount of petroleum hydrocarbons released during static release process was not much and the sampling volume was small (the amount of normal petroleum total monitoring is 500 mL, and the amount of organic matter monitoring in water is 1000 mL, 2000 mL) (Pan et al., 1969). The petroleum hydrocarbons component we detected was relatively small (Figure 1), mainly naphthalene series, phenanthrene and C1 phenanthrene, and a small amount of normal alkanes. This supports that partly water-soluble compounds were measured that are still contained in fresh crude oil. The less soluble steroids and terpenoids and other polycyclic aromatic hydrocarbons have almost no other peaks except for individual peaks. In particular, C1-P was not obviously detected in the blank sample in this water sample, while C1-P peaks were detected in different degrees in other experimental samples. Therefore, C1 phenanthrene can be regarded as a characterization index of static release of crude oil at a lower concentration.
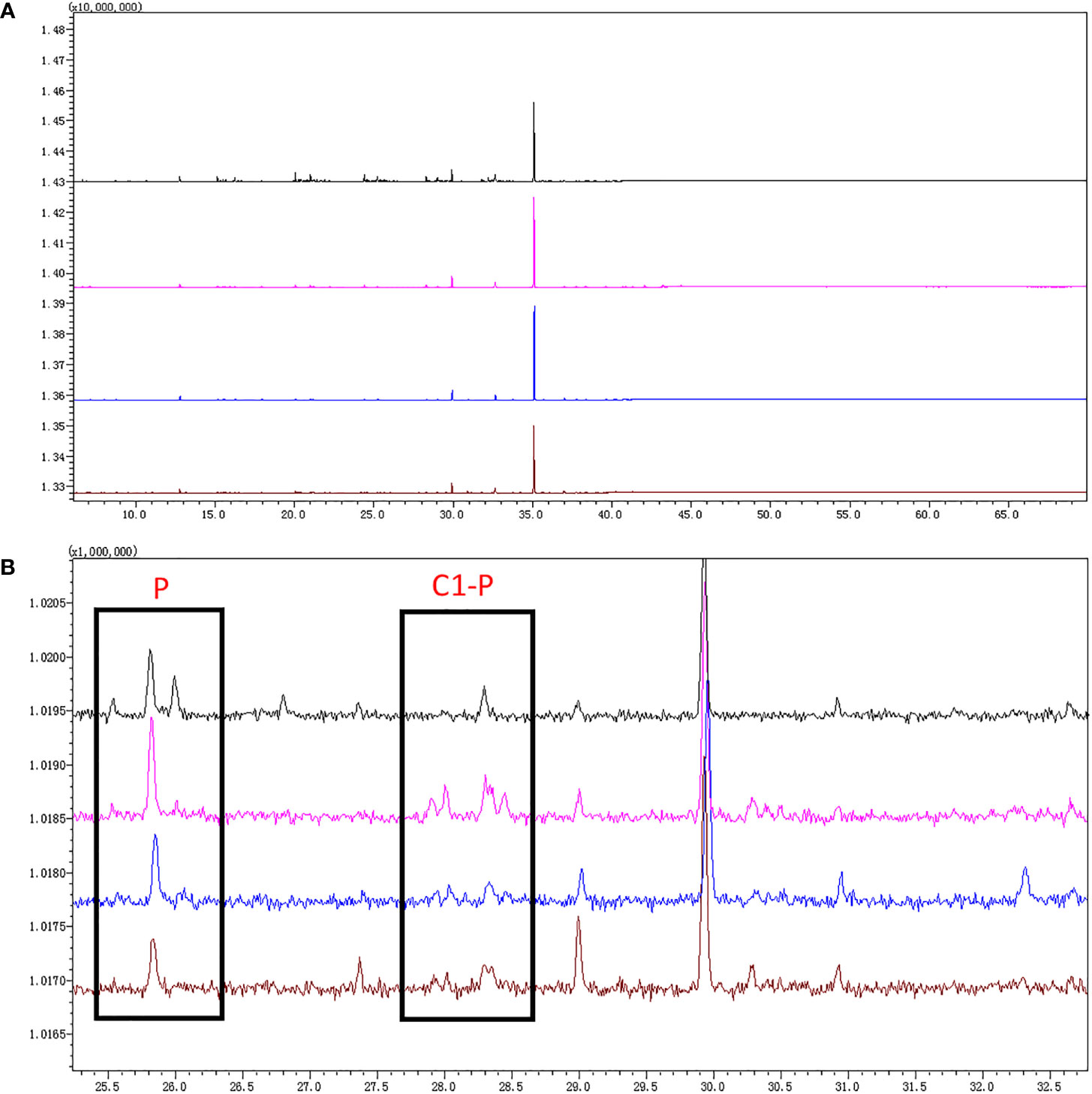
Figure 1 The total ion flow chromatogram (TIC) and mass chromatogram (Philippines series) of petroleum hydrocarbons in original surface water samples (A) and experiment samples (B) (from top to bottom represent blank group, U crude oil group, R crude oil group, T crude oil group).
3.2 Temporal, spatial distribution and comparison of C1 phenanthrene
The molecular marker method is used to analyze the aliphatic hydrocarbon of the sample to determine the source of petroleum hydrocarbons for identifying oil spills (Franco et al., 2006). The petroleum hydrocarbons widely exist for a long time in coastal sediments after the oil spill occurred (Page et al., 1988). Source characteristics and chemical fingerprints of environmental persistence biomarkers can be used to determine the source of oil spills, identify and monitor the degradation and weathering process of crude oil under various conditions (Wang et al., 2016). The C1-P released by U crude oil, R crude oil and T crude oil showed a slow upward trend in the surface, upper and middle layers as a whole. It tended to be flat after 5 h and C1-P release decreased to 0 and remained balanced after 48 h (Figures S3-5). The detailed trend description was described in supporting document. Temporal, spatial distribution and comparison of C1 phenanthrene was showed in Figure 2.
The static release of three kinds crude oil all released a large amount of petroleum hydrocarbons within 1 h. In the crude oil with the lowest viscosity and the highest viscosity, the content of water at different heights was similar in order (surface layer>upper layer≥middle layer>bottom layer). In crude oil with medium viscosity, the order of content of water bodies at different heights was opposite to the other two crude oils (bottom layer>middle layer>surface layer>upper layer). At the same time, the three crude oils were based on the maximum amount of surface release. The crude oil with the smallest viscosity released most, the crude oil with the highest viscosity was the next, and the crude oil with the medium viscosity was the least. In the crude oil with the smallest viscosity and the largest viscosity, the ratio of the time to the peak of the content of the C1-P components at each height was that the time ratio of the middle, bottom, surface, and upper layers was about 1:2. Three kinds crude oils were at different heights, the medium-viscosity crude oil always released more than the two crude oil with low viscosity and high viscosity, which is about 2:1. However, the release of crude oil with low viscosity was equivalent to the crude oil with high viscosity within 48 h. After 48 h, the release of crude oil with high viscosity decreased, and the release of crude oil with low viscosity remained stable (Franco et al., 2006). Figure 3 shows the comparison of C1-P content in fresh oils (three kinds of crude oil). The C1-P content of in fresh oil (U crude oil) with medium viscosity was obviously higher than that of the other fresh oils (R crude oil and T crude oil). This conclusion was consistent with the C1-P content released in different water layers in Figure 2.
3.3 The impact of the release process on the microbial communities
The community structure related to petroleum-degrading bacteria can provide an important basis for exploring the mechanism of petroleum degradation. Therefore, it is necessary to study the changes of microbial communities structure. By studying the changes in the microbial communities structure, the main degrading bacteria of petroleum hydrocarbons at different stages are analyzed (Vila et al., 2010; Shi et al., 2018).
3.3.1 The freeze-drying influence on the microbe in original sediment
The freeze-drying treatment can eliminate the influence of Bohai Sea water contained in the sediment, because the water used in the experiment was from the sea area of Qingdao Zhongyuan Wharf. If the influence brought by this part is not excluded, the credibility of subsequent microbial analysis will be affected. Therefore, the accuracy of the experiment was ensured as much as possible by freeze-drying, especially in the part of biological community. In the process, after freeze-drying, the sediment was evenly ground and then mixed with the oil, which is conducive to the full mixture of oil and sediment. Since the sediment temperature has returned to room temperature at this time, its effect on oil recovery can be ignored. The results showed that the types of microorganisms that have been freeze-dried in the native sediments have not changed, indicating that freeze-drying has a small impact on the microorganisms in the sediments (Figure S6). The 10 dominant phyla in the original sediments were, Proteobacteria 0.55%, Bacteroidetes 0.1%, Chloroflexi 0.07%, Planctomycetes 0.03%, Unidentified_Bacteria 0.03%, Nitrospirae 0.03%, Acidobacteria 0.02%, Cyanobacteria 0.01%, Firmicutes 0.01%, Campylobacterota 0.005%, and others 0.15%. After freeze-drying, the 10 dominant phyla in the microorganisms remained unchanged, but their relative abundances were changed. Among them, the Proteobacteria phylum decreased by 0.09%, the Bacteroides phylum increased by 0.008%, the Chloroflexum phylum increased by 0.02%, Plantomyces phylum increased by 0.04%, unidentified bacteria increased by 0.002%, Nitrospira phylum decreased by 0.005%, Acidobacteria phylum increased by 0.01%, Cyanobacteria increased by 0.01%, Firmicutes decreased by 0.003%, Campylobacterota phyla increased by 0.01%, the other phyla increased by 0.005%.
3.3.2 Diversity and composition of microbial communities in water during static release process
The relative abundance of microorganisms in natural waters in the top 10 phyla were Proteobacteria, Bacteroidetes, Cyanobacteria, Acidobacteria, Planctomycetes, unidentified_Bacteria, Verrucomicrobia, Firmicutes, Chloroflexi, Euryarchaeota, other bacteria Door (Others) (Table S2). From Figure 4, we can know that after the petroleum hydrocarbons static release experiment, among the top 10 bacteria phyla in the relative abundance of microbial communities in natural waters, three types of bacteria (cyanobacteria, verrucomicrobial, and broad archaea) have been not in the top 10 abundance phyla. Therefore, we can think that these three types of bacteria were not suitable for survival and reproduction in an oxygen-deficient environment. After the static experiment, in addition to the 7 types of the original 10 bacteria, three additional dominant bacteria were added, Fusobacteria, Spirochaetes, and Thaumarchaeota. From the perspective of the three pillars, Proteobacteria and Bacteroidetes, which can grow and reproduce regardless of the presence of petroleum hydrocarbons in the water, belong to the dominant bacteria phyla, and their abundance account for more than 95% of the relative abundance of the entire microbial communities. However, the newly added three bacteria phyla were suitable for growth and reproduction in anoxic environment, but whether the newly added three bacteria can grow and reproduce using petroleum hydrocarbons as a carbon source still needs further research.
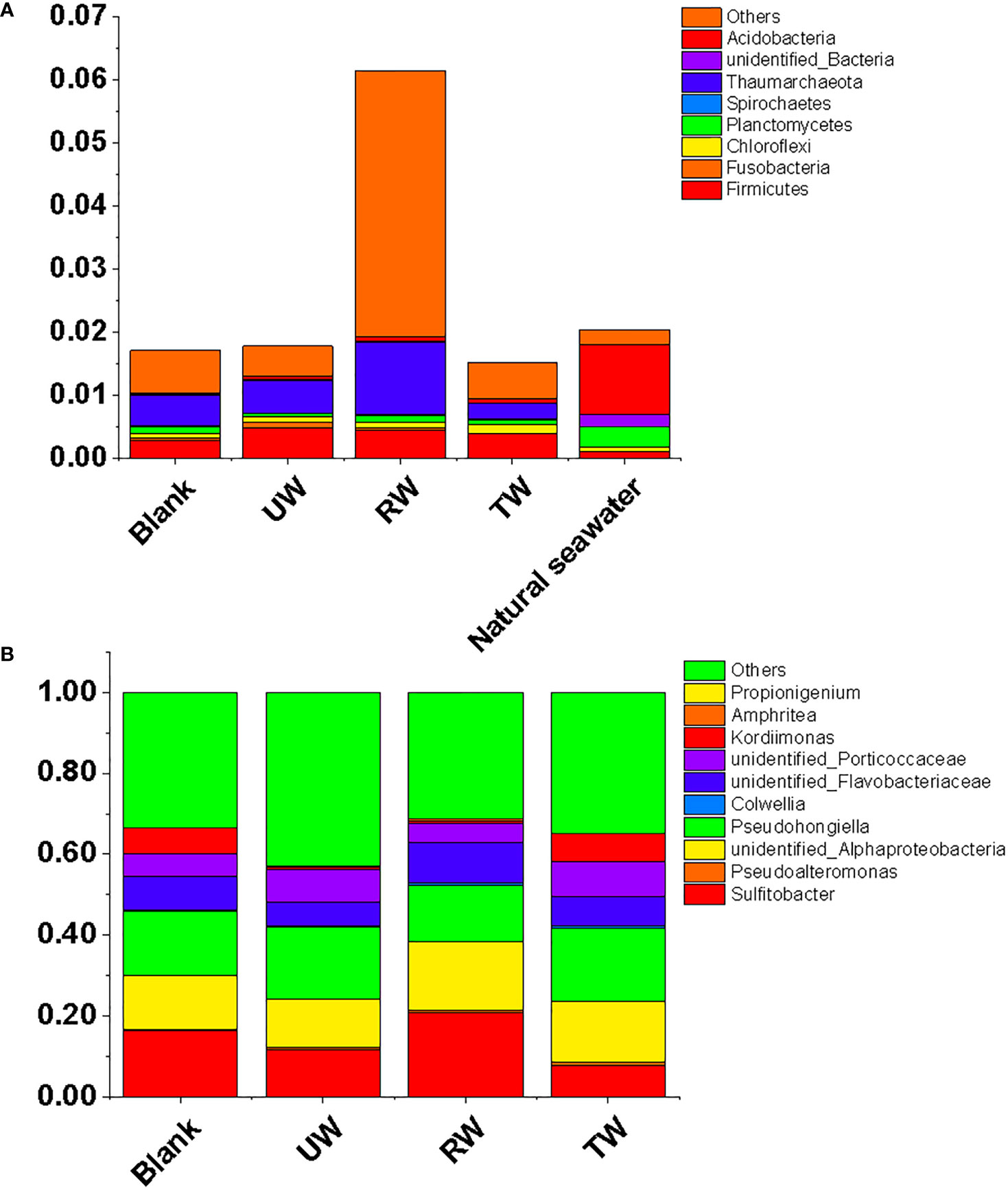
Figure 4 Comparison of microbial communities in water body (A) and sediments (B) for the four pillars at phyla and genus level.
The relative abundance of microorganisms in original sediments at the beginning of static release in the top 10 genus were Propionigenium, Amphritea, Kordiimonas, unidentified_Flavobacteriaceae, Colwellia, Pseudohongiella, unidentified_Alphaproteobacteria, Pseudoalteromonas, Sulfitobacter. During static release process, most of the genus remained unchanged from the original sediment. The relative abundance of Proteobacteria in the water body and Amphritea in the sediment changes was most affected by U crude oil with higher viscosity, followed by R crude oil with the smallest viscosity, and finally T crude with the highest viscosity. However, the relative abundance of Bacteroidetes in the water body was consistent with that of Proteobacteria, which is most affected by U crude oil with higher viscosity, followed by R crude oil with the smallest viscosity, and finally T crude oil with the highest viscosity.
3.3.3 Diversity and composition of microbial communities in sediments during static release process
The top 10 phyla of microorganisms after freeze-drying, Nitrospira, Cyanobacteria, Campylobacterota phyla was replaced by the dominant bacteria phyla [fusobacterium (a variant of fusobacterium), Spirochaetes, Thaumarchaeota)] in the sediments after the static release experiment. Although the other 7 species of mycota have no changes, their abundances have changed (Figure S7). Among them, the blank column AS did not mix with crude oil, but the microbial species changes in the sediments were consistent with the changes in the microbial species of the other three sediments mixed with crude oil. Therefore, it was speculated that the disappeared three bacteria phyla may not be suitable for survival in anoxic environment, and the carbon source of crude oil cannot be absorbed and utilized by the three bacteria phyla. However, the three alternative phyla (fusobacterium (a variant of fusobacterium), Spirochaetes, and Thaumarchaeota) were suitable for survival in anoxic environment and may use crude oil as a carbon source for absorption and utilization.
The relative abundance of the Proteobacteria in the 7 types of bacteria phyla was higher than the abundance in the original sediment regardless of the presence or absence of crude oil (Figure 5A). However, in the R crude oil with the smallest viscosity and the U crude oil with the higher viscosity, the bacterial phyla abundance increase was greater, and in the T crude oil with the highest viscosity, the bacterial phyla abundance increase was lower than that of the A column without crude oil. Therefore, we can get that the Proteus phylum is suitable for growth in a mixture of crude oil and sediment with a small viscosity, and was inhibited in a mixture of crude oil with a high viscosity. Compared with oils with low viscosity, the relative abundance of Bacteroidetes in crude oils with high viscosity had increased. Therefore, the increase in oil viscosity promoted the growth of Bacteroides oleifera, but the opposite was true for Firmicutes. Chloroflexi, Planctomycetes, unidentified_Bacteria, Acidobacteria is not suitable for the environment where crude oil exists, and even the components of crude oil can inhibit the growth and reproduction of it.
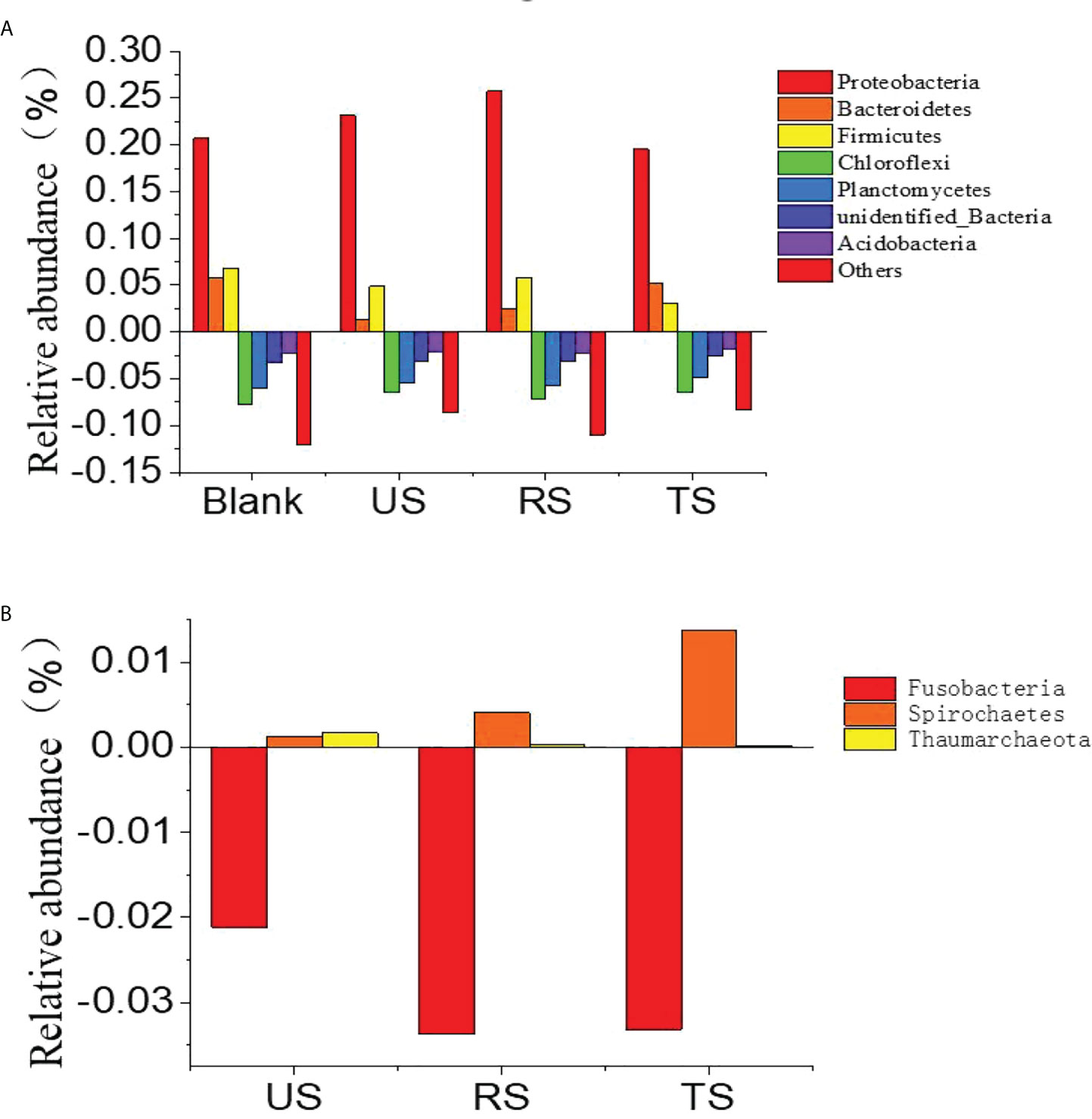
Figure 5 (A) The relative abundance of the Proteobacteria in the seven types of bacteria phyla. (B) Changes in abundance of changes in three microbial communities that are not in the top 10 relative abundance in the original sediments, but appear after the experiment.
As shown in Figure 5B, the three bacteria are those that are not in the top 10 relative abundance in the original sediments, but appear after the experiment. The relative abundance of the three bacteria minus the relative abundance of the blank column, what we get is the degree of influence of crude oil with different viscosities on these three kinds bacteria. Among them, for Spirochaetes and Thaumarchaeota, crude oils of different viscosities can promote them. For Spirochaetes, T crude oil with the highest viscosity promotes it the most; for Thaumarchaeota, U crude oil with medium viscosity promotes it the most. For the Fusobacterium (fusobacterium deformation), three crude oils of different viscosity have an inhibitory effect on it, the R crude oil with the smallest viscosity has the greatest impact, and the U crude oil with the greater viscosity has the least impact. The results can deep understanding the relationship between migration and transformation of petroleum hydrocarbons and microbial communities succession from oil pollution sediments to the water body. At the same time, they can provide a basis for oil spill emergency response and impact assessment.
4 Conclusion
In this paper, the distribution of petroleum hydrocarbons during the static release of crude oil was studied and analyzed, and the influence of them on the microbial communities was discussed. The results showed C1-P can be used as a representative indicator of different crude oils for petroleum hydrocarbons migration and evaluation indicators and their trends of the static release were similar. The crude oil with higher C1-P content releases C1-P into the water body significantly higher. After 72 hours, the release change of C1-P of R crude oil with a smaller viscosity is quite different from that of the other two crude oils. During the static release process, the top 10 abundance of the microbial communities in the water columns and sediments appeared partial (3 species) replacement phenomenon. The results can enrich understanding of the interaction between extreme weather events and human activities by incorporating an eco-evolutionary perspective.
Data availability statement
The datasets presented in this study can be found in online repositories. The names of the repository/repositories and accession number(s) can be found below: BioProject, accession number PRJNA873280.
Author contributions
HL: Methodology, Investigation, Data curation, Conceptualization, Writing-original draft, Writing-review & editing. HS: Methodology, Investigation, Validation. XW: Data curation, Investigation, Validation. FL: Data curation, Investigation. LC: Data curation, Investigation. YL: Resources, Supervision. RD: Data curation, Writing-review. YS: Writing-review. PS: Resources, Investigation, Writing-review. MB: Resources, Supervision, Writing-review. All authors contributed to the article and approved the submitted version.
Funding
This work was funded by National Natural Science Funds of China (Grant No. 42106156); Guangdong Provincial Key Laboratory of Marine Resources and Coastal Engineering; the Applied Research Project of Postdoctoral Staff in Qingdao; the Natural Science Foundation of Shandong Province (ZR2018MD017). This is MCTL Contribution No.284. A special thanks to three anonymous reviewers for their constructive comments to this work.
Conflict of interest
Author YL was employed by company China Petrochemical Corporation (Sinopec Group).
The remaining authors declare that the research was conducted in the absence of any commercial or financial relationships that could be construed as a potential conflict of interest.
Publisher’s note
All claims expressed in this article are solely those of the authors and do not necessarily represent those of their affiliated organizations, or those of the publisher, the editors and the reviewers. Any product that may be evaluated in this article, or claim that may be made by its manufacturer, is not guaranteed or endorsed by the publisher.
Supplementary material
The Supplementary Material for this article can be found online at: https://www.frontiersin.org/articles/10.3389/fmars.2022.1025612/full#supplementary-material
References
Arvanitis N., Katsifas E. A., Chalkou K. I., Meintanis C., Karagouni A. D. (2008). A refinery sludge deposition site: presence of nahH and alkJ genes and crude oil biodegradation ability of bacterial isolates. Biotechnol. Lett. 30, 12, 2105–2110. doi: 10.1007/s10529-008-9816-0
Blumer M., Guillard R. R. L., Chase T. (1971). Hydrocarbons of marine phytoplankton. Mar. Biol. 8 (3), 183–189. doi: 10.1007/bf00355214
Cao X.-Y., Han H., Yang G.-P., Ding H.-B., Zhang H.-H. (2013). A study on the release of oil from oil-contaminated sediment through laboratory experiments. Water Air Soil pollut. 224 (7), 1611. doi: 10.1007/s11270-013-1611-4
Cao X., Yang G., Wei S., Han H. (2011). Sorption of heavy oil onto jiaozhou bay sediment. Mar. pollut. Bull. 62 (4), 741–746. doi: 10.1016/j.marpolbul.2011.01.016
Chao X., Shankar N. J., Wang S. S. Y. (2003). Development and application of oil spill model for Singapore coastal waters. J. Hydraulic Eng. 129 (7), 495–503. doi: 10.1061/(asce)0733-9429(2003)129:7(495
Culbertson J. B., Valiela I., Peacock E. E., Reddy C. M., Carter A., VanderKruik R. (2007). Long-term biological effects of petroleum residues on fiddler crabs in salt marshes. Mar. pollut. Bull. 54 (7), 955–962. doi: 10.1016/j.marpolbul.2007.02.015
Das N., Chandran P. (2011). Microbial degradation of petroleum hydrocarbon contaminants: an overview. Biotechnol. Res. Int. 10, 1–13. doi: 10.4061/2011/941810
Dong Y., Meng L., Li H., Song T., Sun P., Bao M., et al. (2019). Petroleum hydrocarbon release behavior study in oil-sediment aggregates: turbulence intensity and chemical dispersion effect. R. Soc. Chemisty Adv. 9, 7922–7931. doi: 10.1039/C8RA08871C
Farrington J. W. (2014). Oil pollution in the marine environment II: Fates and effects of oil spills. Environ.: Sci. Policy Sustain. Develop. 56 (4), 16–31. doi: 10.1080/00139157.2014.922382
Franco M. A., Viñas L., Soriano J. A., de Armas D., González J. J., Beiras R., et al. (2006). Spatial distribution and ecotoxicity of petroleum hydrocarbons in sediments from the Galicia continental shelf (NW Spain) after the prestige oil spill. Mar. pollut. Bull. 53 (5-7), 260–271. doi: 10.1016/j.marpolbul.2005.10.004
Fuhrman J., Cram J., Needham D. (2015). Marine microbial community dynamics and their ecological interpretation. Nat. Rev. Microbiol. 13, 133–146. doi: 10.1038/nrmicro3417
Gong Y., Zhao X., Cai Z., O’Reilly S. E., Hao X., Zhao D. (2014). A review of oil, dispersed oil and sediment interactions in the aquatic environment: Influence on the fate, transport and remediation of oil spills. Mar. pollut. Bull. 79 (1-2), 16–33. doi: 10.1016/j.marpolbul.2013.12.024
Gui X., Ren Z., Xu X., Chen X., Zhao L., Qiu H., et al. (2022). Oil spills enhanced dispersion and transport of microplastics in sea water and sand at coastal beachheads. J. Hazard. Mater. 436, 129312. doi: 10.1016/j.jhazmat.2022.129312
Head I. M., Swannell R. P. (1999). Bioremediation of petroleum hydrocarbon contaminants in marine habitats. Curr. Opin. Biotechnol. 10 (3), 234–239. doi: 10.1016/s0958-1669(99)80041-x
Khamehchiyan M., Hossein Charkhabi A., Tajik M. (2007). Effects of crude oil contamination on geotechnical properties of clayey and sandy soils. Eng. Geol. 89 (3-4), 220–229. doi: 10.1016/j.enggeo.2006.10.009
Kimes N. E., Callaghan A. V., Suflita J. M., Morris P. J. (2014). Microbial transformation of the deepwater horizon oil spill past, present, and future perspectives. Front. Microbiol. 5 (603). doi: 10.3389/fmicb.2014.00603
Kingston P. F. (2002). Long-term environmental impact of oil spills. Spill Sci. Technol. Bull. 7 (1-2), 53–61. doi: 10.1016/s1353-2561(02)00051-8
Lee K. (2002). Oil-particle interactions in aquatic environments: Influence on the transport, fate, effect and remediation of oil spills. Spill Sci. Technol. Bull. 8 (1), 3–8. doi: 10.1016/s1353-2561(03)00006-9
Li H., Bao M., Li Y., Zhao L., King T., Xie Y. (2020). Effects of suspended particulate matter, surface oil layer thickness and surfactants on the formation and transport of oil-sediment aggregates (OSA). Int. Biodeterioration Biodegradation 149, 104925. doi: 10.1016/j.ibiod.2020.104925
Li Y., Li B. (2011). Study on fungi-bacteria consortium bioremediation of petroleum contaminated mangrove sediments amended with mixed biosurfactants. Advanced Mater. Res. 183-185, 1163–1167. doi: 10.4028/www.scientific.net/amr.183-185.1163
Li H., Shen T., Song T., Bao M., Sun P. (2018). Dominant species succession and oil behavior change under LSH-7’petroleum hydrocarbon degradation bacteria and chemical dispersant in open water columns. J. Taiwan Institute Chem. Eng. 93, 519–527. doi: 10.1016/j.jtice.2018.08.031
Li S., Zhang S., Dong H., Zhao Q., Cao C. (2015). Presence of aliphatic and polycyclic aromatic hydrocarbons in near-surface sediments of an oil spill area in bohai Sea. Mar. pollut. Bull. 100 (1), 169–175. doi: 10.1016/j.marpolbul.2015.09.009
Li X., Zhao L., Adam M. (2016). Biodegradation of marine crude oil pollution using a salt-tolerant bacterial consortium isolated from bohai bay. Mar. pollut. Bull. 105 (1), 43–50. doi: 10.1016/j.marpolbul.2016.02.073
Morgan P., Watkinson R. J. (1989). The use of gel-stabilized model systems for the study of microbial processes in polluted sediments. Microbiology 135 (3), 549–555. doi: 10.1099/00221287-135-3-549
Narimannejad S., Zhang B., Lye L. (2019). Adsorption behavior of cobalt onto saline soil with/without a biosurfactant: Kinetic and isotherm studies. Water Air Soil pollut. 230 (2), 47. doi: 10.1007/s11270-019-4097-x
Nelson J. R., Grubesic T. H. (2017). Oil spill modeling: Risk, spatial vulnerability, and impact assessment. Prog. Phys. Geography: Earth Environ. 42 (1), 112–127. doi: 10.1177/0309133317744737
Okafor-Yarwood I. (2018). The effects of oil pollution on the marine environment in the gulf of Guinea-the bonga oil field example. Transnational Legal Theory 9 (3-4), 254–271. doi: 10.1080/20414005.2018.1562287
Page D. S., Foster J. C., Fickett P. M., Gilfillan E. S. (1988). Identification of petroleum sources in an area impacted by the Amoco cadiz oil spill. Mar. pollut. Bull. 19 (3), 107–115. doi: 10.1016/0025-326x(88)90705-9
Pan Y. S., Donegan S. M., Hayman R. H. (1969). Sweating rate at different body regions in cattle and its correlation with some quantitative components of sweat gland volume for a given area of skin. Aust. J. Agric. Res. 20 (2), 395–403. doi: 10.1071/AR9690395
Passow U., Lee K. (2022). Future oil spill response plans require integrated analysis of factors that influence the fate of oil in the ocean. Curr. Opin. Chem. Eng. 36, 100769. doi: 10.1016/j.coche.2021.100769
Saracino A., Martina C., Bonvicini S., Cozzani V. (2017). Quantitative assessment of environmental impact of offshore oil spills: Influence of remediation strategies. Offshore Mediterr. Conf. Exhibition, 807.
Shi K., Liu Z., Xu H., Xue J., Liu Y., Wu Y., et al. (2018). Degradation characteristics and microbial community change of marine petroleum-degrading bacteria in different degradation environments. Petroleum Sci. Technol. 36, 1–7. doi: 10.1080/10916466.2018.1465969
Urum K., Pekdemir T. (2004). Evaluation of biosurfactants for crude oil contaminated soil washing. Chemosphere 57 (9), 1139–1150. doi: 10.1016/j.chemosphere.2004.07.04
Vila J., Nieto J. M., Mertens J., Springael D., Grifoll M. (2010). Microbial community structure of a heavy fuel oil-degrading marine consortium: linking microbial dynamics with polycyclic aromatic hydrocarbon utilization. FEMS Microbiol. Ecol. 73 (2). doi: 10.1111/j.1574-6941.2010.00902.x
Wang Z., An C., Lee K., Owens E., Chen Z., Boufadel M., et al. (2020). Factors influencing the fate of oil spilled on shorelines: a review. Environ. Chem. Lett. 19, 1611–1628. doi: 10.1007/s10311-020-01097-4
Wang C., Liu X., Guo J., Lv Y., Li Y. (2018). Biodegradation of marine oil spill residues using aboriginal bacterial consortium based on penglai 19-3 oil spill accident, China. Ecotoxicol. Environ. Saf. 159, 20–27. doi: 10.1016/j.ecoenv.2018.04.059
Wang Z., Yang C., Yang Z., Brown C. E., Hollebone B. P., Stout S. A. (2016). Petroleum biomarker fingerprinting for oil spill characterization and source identification. Standard Handb. Oil Spill Environ. Forensics, 73–146. doi: 10.1016/b978-0-12-803832-1.00004-0
Wu G., He L., Chen D. (2013). Sorption and distribution of asphaltene, resin, aromatic and saturate fractions of heavy crude oil on quartz surface: Molecular dynamic simulation. Chemosphere 92 (11), 1465–1471. doi: 10.1016/j.chemosphere.2013.03.05
Yamada M., Takada H., Toyoda K., Yoshida A., Shibata A., Nomura H., et al. (2003). Study on the fate of petroleum-derived polycyclic aromatic hydrocarbons (PAHs) and the effect of chemical dispersant using an enclosed ecosystem, mesocosm. Mar. pollut. Bull. 47 (1-6), 105–113. doi: 10.1016/s0025-326x(03)00102-4
Yang Z., Fang S., Duan M., Xiong Y., Wang X. (2019). Chemisorption mechanism of crude oil on soil surface. J. Hazard. Mater. 386 (15), 121991. doi: 10.1016/j.jhazmat.2019.121991
Yuan L., Han L., Bo W., Chen H., Gao W., Chen B. (2017). Simulated oil release from oil-contaminated marine sediment in the bohai Sea, China. Mar. pollut. Bull. 118 (1-2), 79–84. doi: 10.1016/j.marpolbul.2017.01.065
Yu C., Yao J., Cai M., Yuan H., Chen H., Ceccanti B. (2014). Polycyclic aromatic hydrocarbons degrading microflora in a tropical oil-production well. Bull. Environ. Contamination Toxicol. 93 (5), 632–636. doi: 10.1007/s00128-014-1371-x
Zhang W., Li C., Chen J., Wan Z., Shu Y., Song L., et al. (2021). Governance of global vessel-source marine oil spills: Characteristics and refreshed strategies. Ocean Coast. Manage. 213, 105874. doi: 10.1016/j.ocecoaman.2021.105874
Keywords: oil pollution sediments, petroleum hydrocarbons, vertical variation, C1 phenanthrene, microbial communities succession, marine oil spill
Citation: Li H, Sun H, Wang X, Li F, Cao L, Li Y, Dong R, Sun Y, Sun P and Bao M (2022) Temporal and spatial variation of petroleum hydrocarbons and microbial communities during static release of oil pollution sediments. Front. Mar. Sci. 9:1025612. doi: 10.3389/fmars.2022.1025612
Received: 23 August 2022; Accepted: 09 September 2022;
Published: 23 September 2022.
Edited by:
Ya Ping Wang, East China Normal University, ChinaReviewed by:
Lijuan Feng, Zhejiang Ocean University, ChinaFan Hongyong, Qingdao University of Technology, China
Hui Kong, Beijing Institute of Technology, China
Copyright © 2022 Li, Sun, Wang, Li, Cao, Li, Dong, Sun, Sun and Bao. This is an open-access article distributed under the terms of the Creative Commons Attribution License (CC BY). The use, distribution or reproduction in other forums is permitted, provided the original author(s) and the copyright owner(s) are credited and that the original publication in this journal is cited, in accordance with accepted academic practice. No use, distribution or reproduction is permitted which does not comply with these terms.
*Correspondence: Mutai Bao, mtbao@ouc.edu.cn; Peiyan Sun, sunpeiyan@ncs.mnr.gov.cn