- 1State Key Joint Laboratory of Environmental Simulation and Pollution Control, School of Environment, Beijing Normal University, Beijing, China
- 2Shandong Yellow River Delta National Nature Reserve Administration Committee, Dongying, China
- 3Department of Water Environment, China Institute of Water Resources and Hydropower Research, Beijing, China
Burrowing crabs are widely distributed and have large populations in estuarine wetlands. Crab excavation can have potentially significant bioturbation effects on the vertical structure of sediments, and the processes of nutrients deposition and mineralization. However, the effects of crab micro activities on the geochemical cycling processes of the whole estuarine ecosystems are not clear, specifically the contributions of burrowing crabs to sediment and nutrients turnover in coastal ecosystems. Due to the lack of knowledge on crab burrowing behavior and borrow morphology, it is difficult to accurately estimate the excavation and turnover volumes of crabs. Therefore, this study examined the bioturbation activity of the crab Helice tientsinensis in western Pacific estuary ecosystems by analyzing their burrow morphology and local sediment properties. The common burrow shapes of Helice tientsinensis were J- and Y-shaped burrows. Burrow morphological characteristics such as total burrow depth, curve burrow length, burrow volume, and opening diameter significantly differed among tidal zones. Crab carapace size, water depth, soil hardness, and bulk density were the main factors driving burrow morphology. Sediment excavation by crabs was ~50 times greater than the deposition of sediment into crab burrows. The net transported amounts of sediment (31.66–33.18 g·d-1·m-2) and nutrients (total nitrogen: 0.075–0.090 g·d-1·m-2, total carbon: 3.96–4.55 g·d-1·m-2, and organic matter: 0.44–0.77 g·d-1·m-2) were mainly from the belowground sediment to the surface. These results highlighted the important role of crabs in sediment and nutrients cycling within coastal estuary ecosystems.
Introduction
Burrowing crabs can act as ecosystem engineers, herbivores, and prey, playing important roles in coastal estuarine ecosystems (Alberti et al., 2008; He et al., 2017). Crabs feed on various food resources including microphytobenthos, plant, leaf litter, organic debris, and fungi, directly affecting the deposition and mineralization of soil organic matter (OM) and nutrients (Pennings et al., 1998; Zhang et al., 2013; He et al., 2015; Chen et al., 2016; Ouyang et al., 2021). Digging is the most basic crab behavior. Crabs support nutrient cycling, promote sediment-water exchange, and affect sediment properties through their burrowing activities (Koo et al., 2007; Xin et al., 2009; Fanjul et al., 2015; Vu et al., 2017). They can redistribute sediments by depositing soils from the bottom of burrows and by building mounds, which in turn promotes CO2 emission due to increase area of the sediment-air interface (Penha-Lopes et al., 2010; Ouyang et al., 2017). In this process, they turn-over the sediment from belowground to the surface, thereafter the sediments are eroded by tides contributing to carbon and nitrogen cycling (Wang et al., 2010). Nutrient redistribution occurs alongside sediment redistribution. Due to the large populations and wide spatial distribution of crabs in estuarine wetlands, the regulation of crab burrowing activities on nutrient cycling processes in estuarine wetlands cannot be ignored (Martinetto et al., 2011). However, the contributions of burrowing crabs to sediment and nutrient turnover in coastal ecosystems are unclear. This has created a gap in the understanding of the links between the micro behavior of small animals and the macroscopic ecological processes of ecosystems (Ren et al., 2022).
Knowledge of crab digging behavior and their burrow morphology is key to accurately calculating crab excavation volume (Bang and Lee, 2019). The daily excavation volume of crabs and burrow morphological parameters, such as maximum length and crab burrow volume, are vital for estimating their ability to turnover sediments. The burrow structures of different crab species vary greatly, even between those built by the same crab species but in different locations in estuarine wetlands. Previous studies have shown that many crab species have burrowing ability (Botto and Iribarne, 1999; Canepuccia et al., 2008; Alberti et al., 2015; de Oliveira et al., 2016). Burrows are essential for intertidal mud crabs for a number of reasons; enabling the avoidance of stressful conditions and protection from temperature extremes (Saher and Qureshi, 2011); protection from aerial and terrestrial predation (Wang et al., 2014); and provision of a molting and mating space (Christy, 1987; McLain et al., 2003). To date, a variety of burrow shapes have been reported, including J-, U-, V-, and Y-shaped burrows (Lim, 2003; Lim et al., 2011; Saher and Qureshi, 2011; Sen and Homechaudhuri, 2018). With the development of methods for detecting the underground structure of crab burrows, more accurate burrow morphology data can be used to estimate crab excavation and bioturbation.
Several crab burrow morphologies have been studied for the species of Uca annulipes (Lim, 2003; Saher and Qureshi, 2011), Sesarma dehaani (Wang et al., 2015), Uca rosea (Sen and Homechaudhuri, 2018), Uca chlorophthalmus, Uca sindensis (Saher and Qureshi, 2011), Uca uruguayensis, Uca leptodactylus (Machado et al., 2013), Uca arcuata (Wang et al., 2015), Uca triangularis (Sen and Homechaudhuri, 2018), and Uca vocans (Lim, 2006). These were revealed using the melted paraffin method (Machado et al., 2013), plaster of Paris (Saher and Qureshi, 2011), fast bonding foam (Ribeiro et al., 2005), and unsaturated polyester resin (Stieglitz et al., 2000; Thongtham and Kristensen, 2003; Katrak et al., 2008), among others. Burrow morphology has commonly been described using burrow diameter, total burrow depth (TBD), total curve burrow length (CBL), and total burrow volume (BV) (Lim, 2003; Saher and Qureshi, 2011; Machado et al., 2013). Previous studies have shown that salinity (Bianchini et al., 2008), soil water content (Reinsel and Rittschof, 1995; Leoville et al., 2021), temperature, and sediment properties (Spivak et al., 1994) affected crab burrow distribution. Elucidating the controlling environmental factors of crab burrow morphology is important for large-scale estimation of crab excavation, and is beneficial for assessing the impact of future environmental changes on crab bioturbation in estuarine wetlands. However, the main factors driving burrow morphology remain unknown.
Helice tientsinensis, a semi-terrestrial burrowing crab, prefers habitats that are soft, wet, and rich in food resources (He et al., 2015). It is known for its burrowing and herbivorous activities (He et al., 2017). In addition, H. tientsinensis shows a characteristic burrow building behavior, particularly chimney building in the vicinity of the burrow opening, known as a burrow mound. Crabs can directly excavate sediments, and their burrows can also trap sediments (Qiu et al., 2019). Whether burrows with and without mounds have different sediment trapping effects remains unclear, consequently, net sediment transported rates in the ecosystem are not known.
Helice tientsinensis is a common burrowing crab species and the dominant crab species in the salt marshes of the Yellow River Delta, China. However, little is known about its burrow morphology characteristics and what drives its burrow morphology. In addition, its contributions to the sediment turnover rate for maintaining ecosystem sustainability are unknown. Therefore, this study aimed to: 1) determine the prevalent shape of H. tientsinensis burrows across different salt marsh zones, 2) identify the main factors driving burrow morphology, and 3) estimate the contributions of its burrowing activities to sediment turnover rate across salt marsh zones in coastal ecosystems.
Materials and methods
Study area
Fieldwork was conducted in salt marshes (119°09’ E; 37°46’ N) of the Yellow River Delta, China. The local climate is warm temperate with irregularly semidiurnal tides (Li et al., 2016a). The lowest average tide, mean high water neap, mean high water spring tide, and highest average tide are -0.74, -0.07, 0.34, and 0.63 m, respectively. The subtidal, low tidal, mid tidal, high tidal, and supratidal zones are divided by these four tidal range lines (Xie et al., 2019).
The Yellow River Delta salt marshes are mainly dominated by Suaeda salsa, with interspersed zones of Salicornia europaea and Tamarix chinensis (Li et al., 2016b). The dominant crab species is H. tientsinensis (Grapsidae), which inhabits almost the entire intertidal salt marshes of the Yellow River Delta (He et al., 2012). To understand the crab burrow morphology across different salt marshes, six study sites were established in different zones of a salt marsh (Figure 1). Two sites were located in the mid intertidal zone (Site 1: 0.10 m, Site 4: 0.22 m), two were established in the high intertidal zone (Site 2: 0.52 m, Site 5: 0.58 m), and the other two were located in the supratidal zone (Site 3: 0.78 m, Site 6: 1.44 m). Site 1 and Site 4 were set up on a bare salt flat lacking vegetation, but adjacent to a Spartina alterniflora community. Site 2 and Site 5 were established in a short S. salsa community. Site 3 and Site 6 were located in a tall S. salsa community with neighboring T. chinensis.
Crab density and size
Crab burrows, rather than crab number, have often been used to estimate their populations (Schlacher et al., 2016; Stelling-Wood et al., 2016). Adult H. tientsinensis live alone in a concentrated burrow; therefore, it’s reasonable to use their burrows to estimate their population (Xie et al., 2020). To determine the relationship between crab size and burrow diameter, 84 crab burrows were randomly marked across the whole intertidal salt marsh. These crabs were caught when they emerged through their burrow, and their carapace length and width were then measured. Their corresponding burrow diameter was also measured using a pair of digital Vernier calipers, and then the crabs were released. To determine the crab density among the six study sites across different salt marsh zones, six sampling plots (1 m × 1 m) were established at each site. The number of crab burrows (diameter > 10 mm) was counted instead of crab number when determining crab density.
Crab burrow morphology
Helice tientsinensis digs underground sediment to close its burrow at flood tide and opens it at ebb tide, resulting in the formation of a mound at the entrance of their burrow (Xie et al., 2020). Its burrows drain well and are always empty. The crab burrow can be divided into two parts: the aboveground mound and the underground cave. To understand the crab burrow aboveground morphology, the diameter, height, length, and width of crab mounds were measured at each site. To document the crab burrow belowground morphology, fast bonding foam was injected into open burrows (Ribeiro et al., 2005), the crab burrow belowground structures were then dug out 12 hours later. In total, 24 crab burrow belowground structures were obtained, four for each site. To compare the differences in burrow morphology, the burrow morphology nomenclature of H. tientsinensis (Figure 2) was analyzed according to previous studies (Lim, 2003; Heng and Lim, 2007).
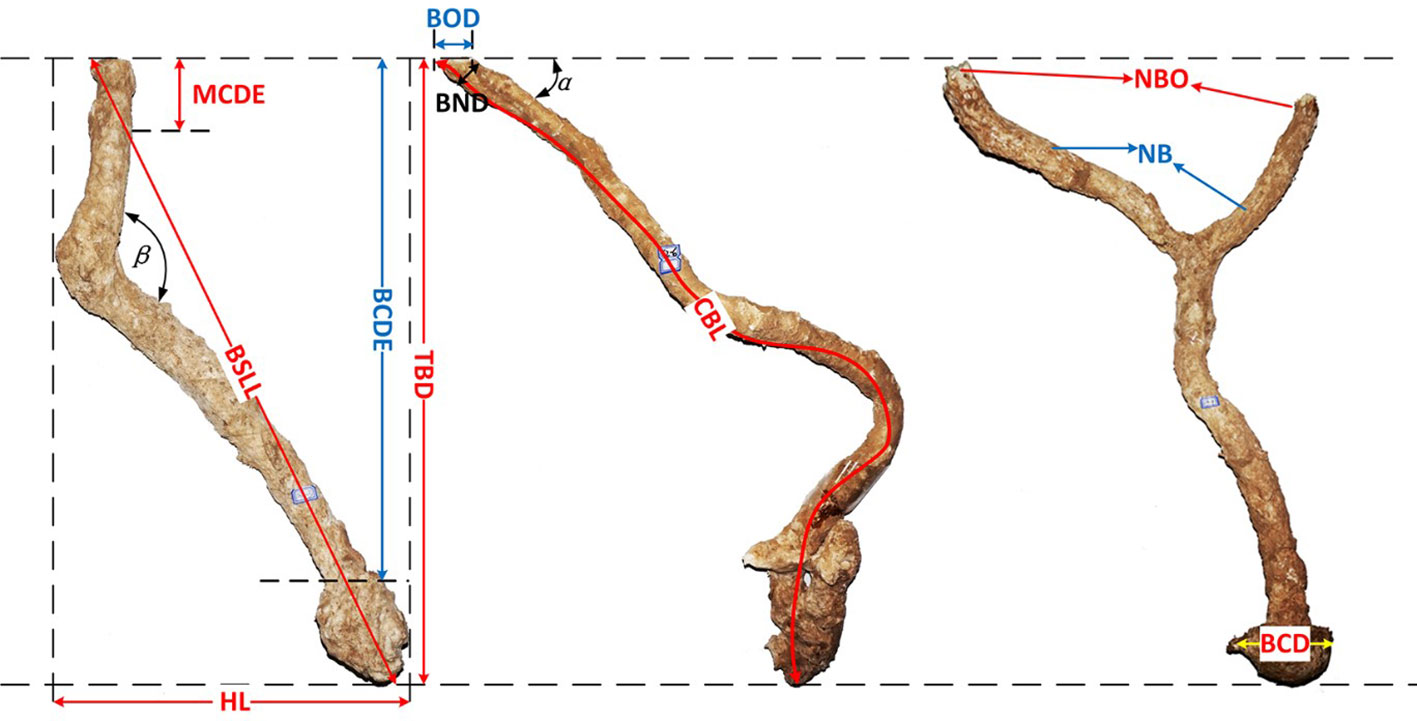
Figure 2 Burrow morphology nomenclature of H. tientsinensis. TBD, total burrow depth; BOD, burrow opening diameter; α, ground angle; CBL, curve burrow length; HL, horizontal length; β, mid angle; BND, burrow neck diameter; MCDE, middle chamber depth; BCDE, bottom chamber depth; BSLL, burrow’s straight-line length; BCD, bottom chamber diameter; NBO, number of burrow openings; NB, number of branches.
Water depth and sediment properties
When the crab burrow belowground morphology was dug out, it revealed that the crab burrows ended at the groundwater level in all marsh zones. Thus, four groundwater levels were measured at each site. To identify the differences in sediment properties at each site, sediment cores from the ground surface to groundwater level were examined at 10-cm intervals, with three replicates at each site. The profile distributions of pH, bulk density, sediment temperature, water content, sediment hardness, OM, total nitrogen (TN), total carbon (TC), and salinity at each soil depth of the six studied sites were determined. The sediment water content and bulk density were determined by weighing soil cores before and after oven-drying at 60°C for 3 days (He et al., 2012). Salinity and pH were measured by mixing one volume of dry soil and five volumes of deionized water, and after 3 hours of standstill, the salinity and pH of the supernatant were recorded (Pennings et al., 2003). Sediment hardness was measured using a soil penetrometer. Sediment temperature was recorded using an ordinary rectangular geothermometer. TN and TC were assessed using a continuous-flow analysis instrument (AA3, Europe). Sediment OM content was measured using the method of Walkley and Black (Bai et al., 2012).
Crab excavation amount
To estimate the total amount of sediment and nutrients excavated by the crabs at each site, the following formulae were used:
The sediment was divided into n layers. In the i layer, SRi is the sediment excavation (g/m2), Di is the crab burrow density (ind./m2), BVi is the burrow volume (cm3), BDi is the soil bulk density (g/cm3), NRi is the nutrient excavation amount (g/m2), and NCi is the nutrient content (%).
Sediment deposition amount and turnover rate
To determine the crab excavation rate, 24 crab burrows, four burrows at each site, were randomly selected and marked. For each burrow, all of the burrow mounds were removed on the first day, then the crab excavation sediment was collected on each day of the following 7 days. Sediment was dried at 60°C for 3 days and then weighed.
To estimate the amount of sediment deposited in crab burrows, six pairs of 3-cm diameter PVC pipes with and without crab burrow mounds were set at each salt marsh zone (Figure 3). The 3 cm diameter was based on the average burrow diameter measured. Two treatments were established because crab burrows with mounds may obstruct sediment deposited into burrows, while the crab burrows without mounds may trap more sediment. The crab burrow mimic was established on the first day, as above. Seven days later, the deposited sediment was collected, dried and weighed, and the daily sediment deposited amount was calculated. The TN, TC, and OM content of the sediment was determined. The net turnover rate is calculated as the difference between the crab excavation rate and sediment deposited rate.
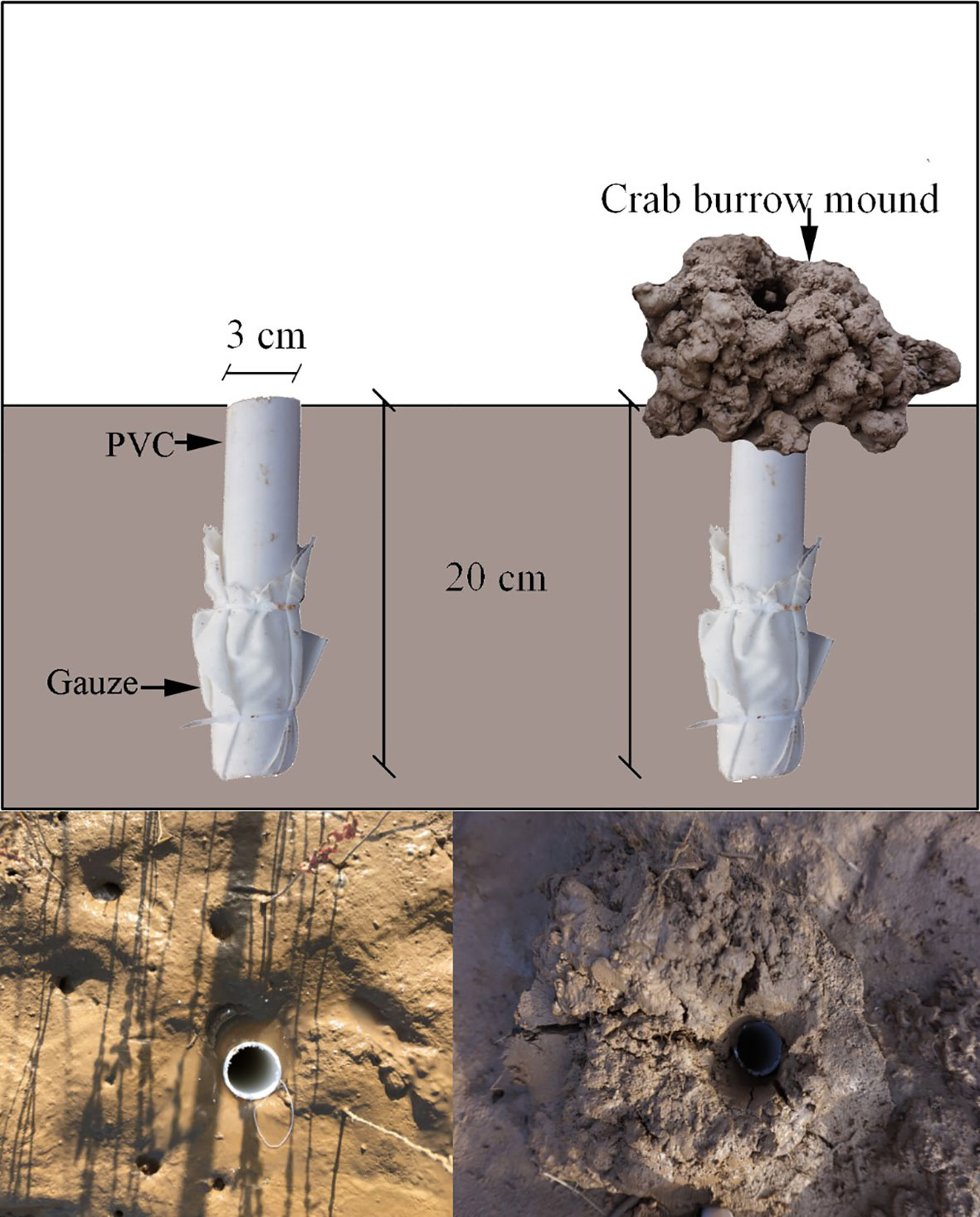
Figure 3 Crab burrow mimic with and without burrow mounds. A 3-cm diameter and 20-cm long PVC pipe was used to simulate crab burrow trapping sediments. The bottoms of the PVC pipes were closed by gauze.
Statistical analyses
The data normality of the crab burrow density, crab burrow morphology, water depth, crab excavation amount, and sediment deposition amount data were assessed by the Kolmogorov–Smirnov test (α = 0.05). Data sets were natural-log transformed when necessary to meet assumptions of normality and homogeneity of variance. Crab burrow density, crab burrow morphology, water depth crab excavation amount, and sediment deposition amount were compared among the six studied sites using one-way analysis of variance (ANOVA) with Tukey post hoc multiple tests. The relationship between crab size and burrow diameter was analyzed by liner regression. These analyses were carried out using SPSS v.22 (IBM Corp., Chicago, IL, USA). A principal component analysis (PCA) was used to correlate sediment properties with crab burrow morphology using Canoco v.5.0 software (Microcomputer Power, New York, USA).
Results
Crab burrow density and water depth along the elevation gradient
Crab burrow density varied significantly (F = 6.043, P< 0.001) among the different salt marsh zones (Figure 4). Crab burrow density was ~1.7 times higher in the supratidal marsh zone than in the high marsh zone, and crab burrow density was ~1.3 times higher in the middle marsh zone than in the high marsh zone. Water depth varied differently along the elevation gradient (F = 191.138, P< 0.001). The underground water level increased from low to high marsh zones (Figure 4).
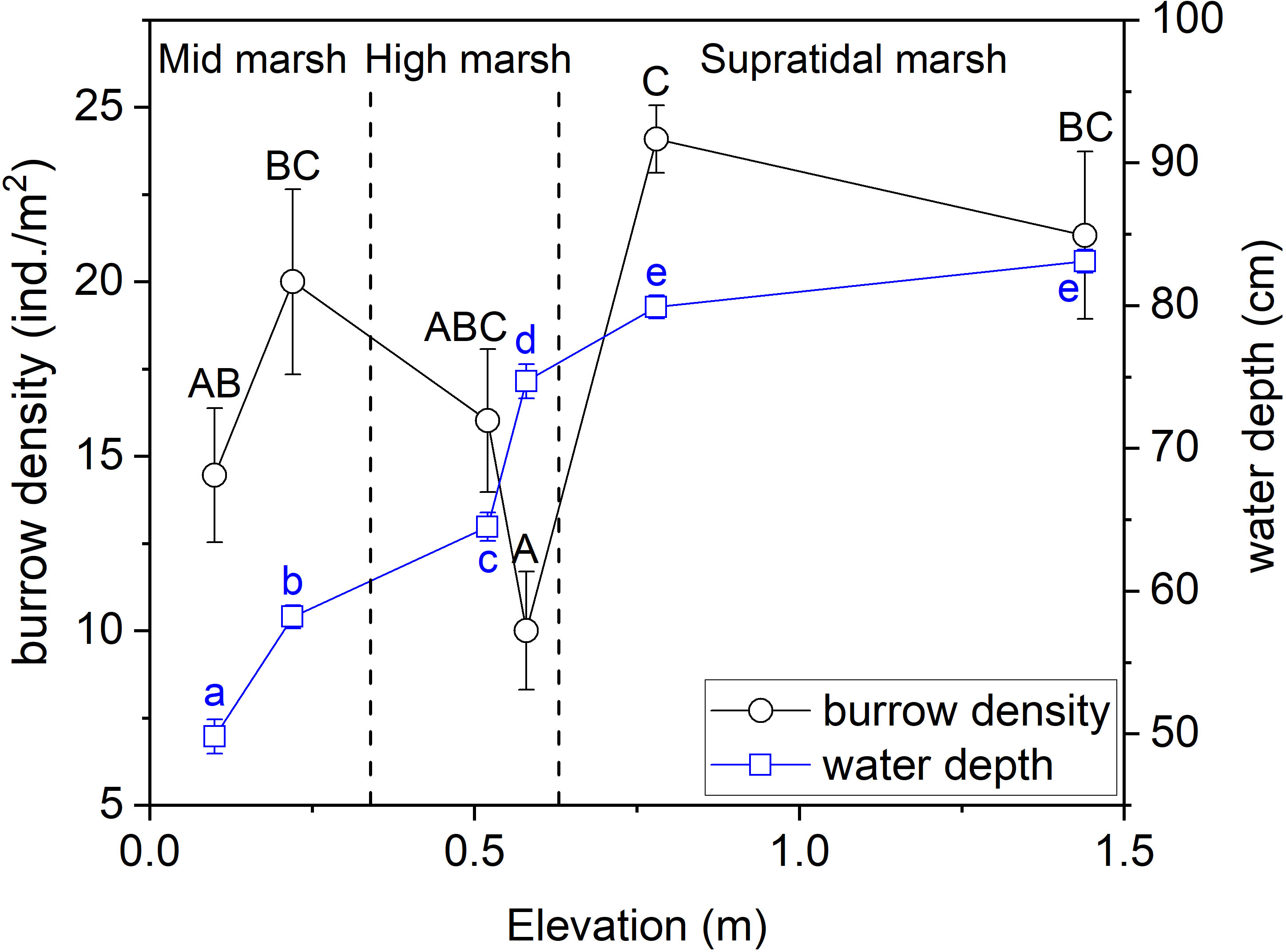
Figure 4 Crab burrow density and water depth across the three tidal salt marshes. Data are shown as means ± SE. Lowercase letters indicate significant differences among different water depth datasets and capital letters indicate significant differences among burrow density datasets, with a Tukey test.
Sediment properties
Soil pH, bulk density, soil temperature, water content, soil hardness, TN, TC, salinity, and OM varied at different soil depths among the six study sites (Figure 5). There were no obvious tendencies for variations in soil pH and bulk density with depth. The temperature of the soil surface was usually higher than that of the underground soil; the soil temperature decreased primarily and then increased with increasing depth. Water content increased with increasing depth. Soil was softer in deeper soil. TN, OM, and TC were lower in deeper soil.
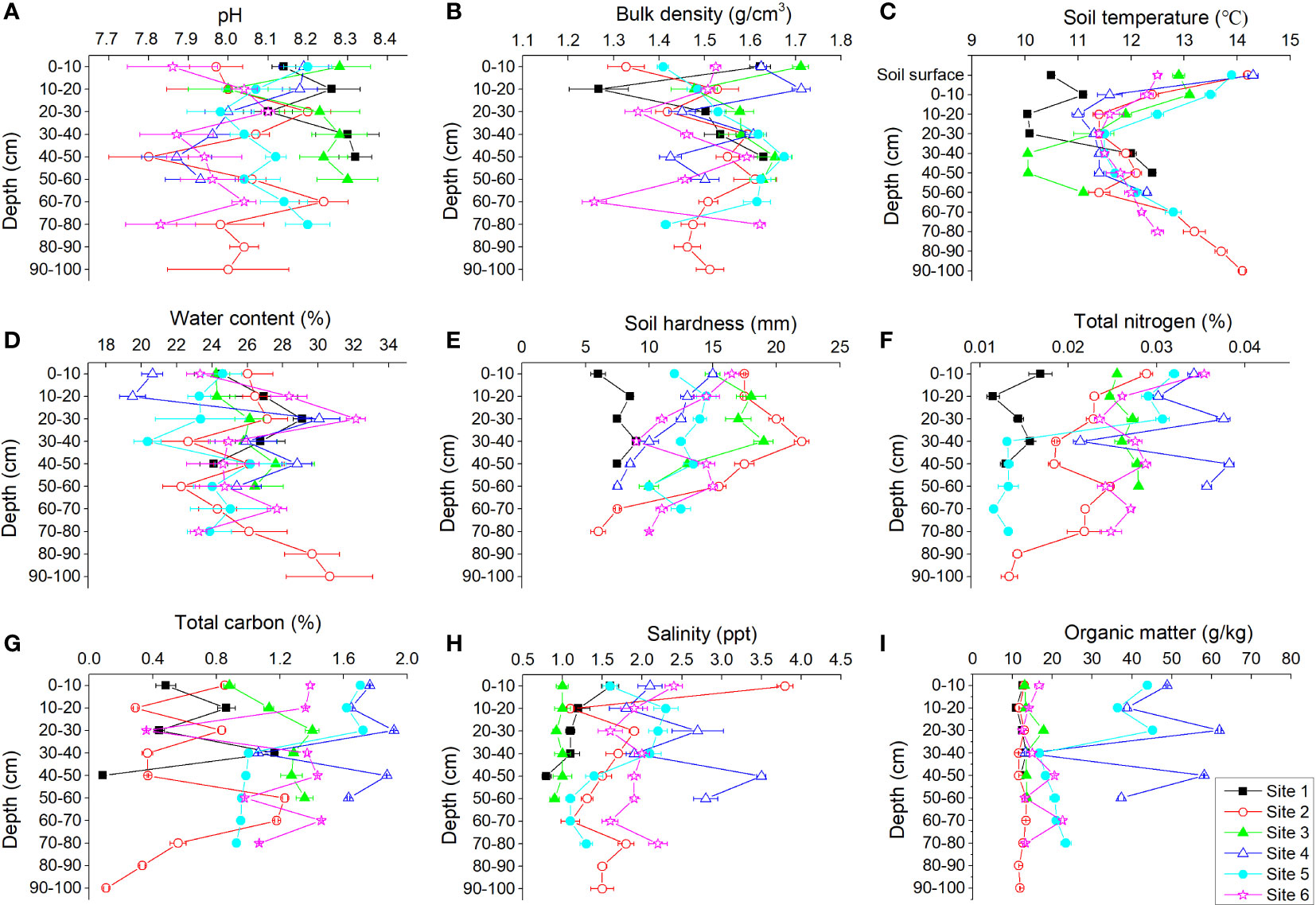
Figure 5 Profile distributions of pH (A), bulk density (B), soil temperature (C), water content (D), soil hardness (E), total nitrogen (F), total carbon (G), salinity (H), and organic matter (I) at each site of the Yellow River Delta.
Crab burrow morphology
Crab burrow aboveground mound morphology differed among the six study sites (Figure 6). The mound opening diameter was larger in the supratidal marsh (F = 3.280, P< 0.05), but the height (F = 3.477, P< 0.05), length (F = 4.919, P< 0.01) and width (F = 6.610, P< 0.001) of mounds in the high marsh were the largest. Regarding the underground morphology of crab burrows, the common burrow shapes of H. tientsinensis were J- and Y-shaped. Among all analyzed H. tientsinensis burrow morphology nomenclature, TBD, CBL, bottom chamber depth (BCDE), burrow straight line length (BSLL), BV, and per opening volume (POV) were significantly different among the six study sites (Tables 1A, B).
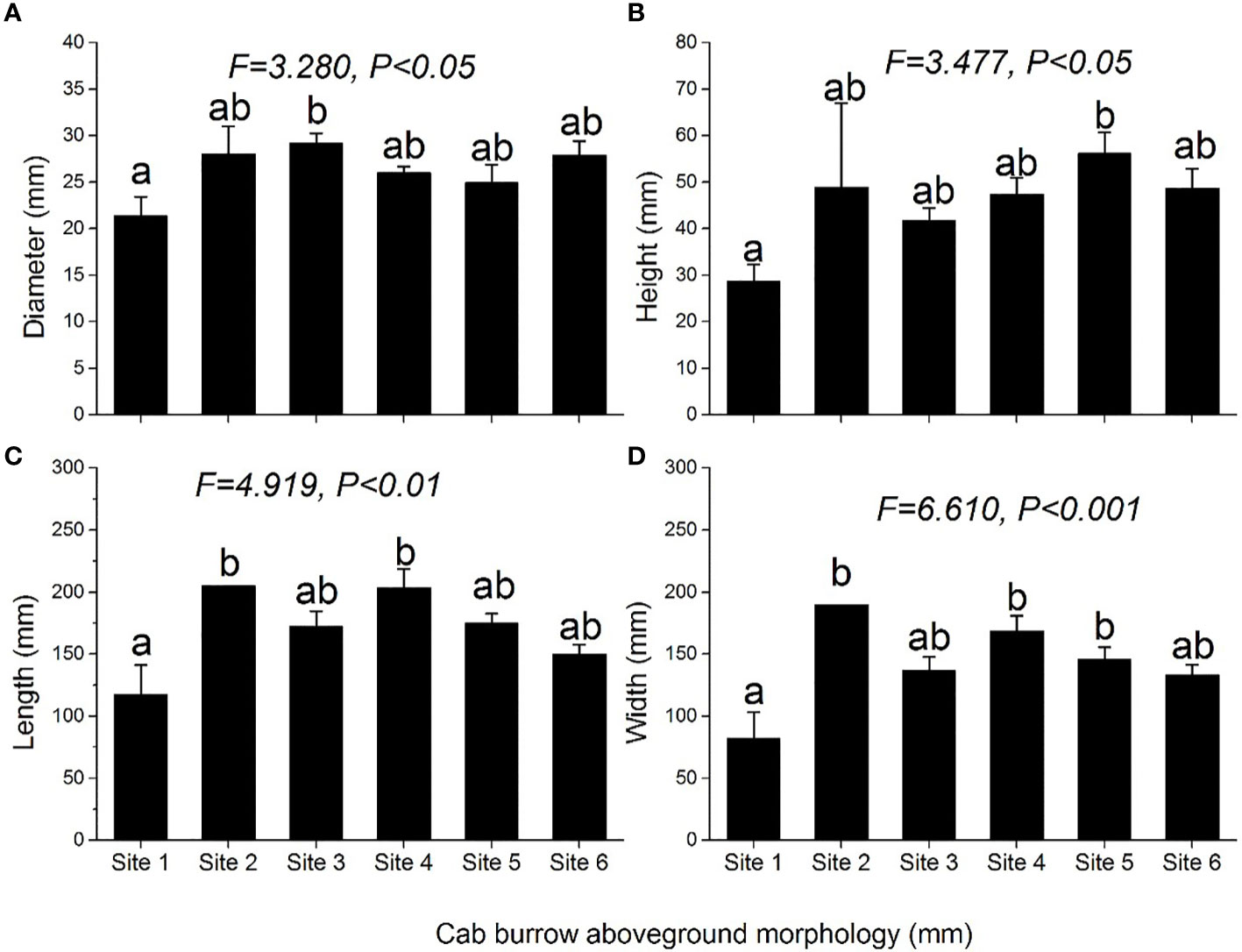
Figure 6 Morphological characteristics: (A) diameter, (B) height, (C) length, and (D) width of crab burrow aboveground mounds at each site. Data are shown as means ± SE. Letters indicate significant differences with a Tukey test.
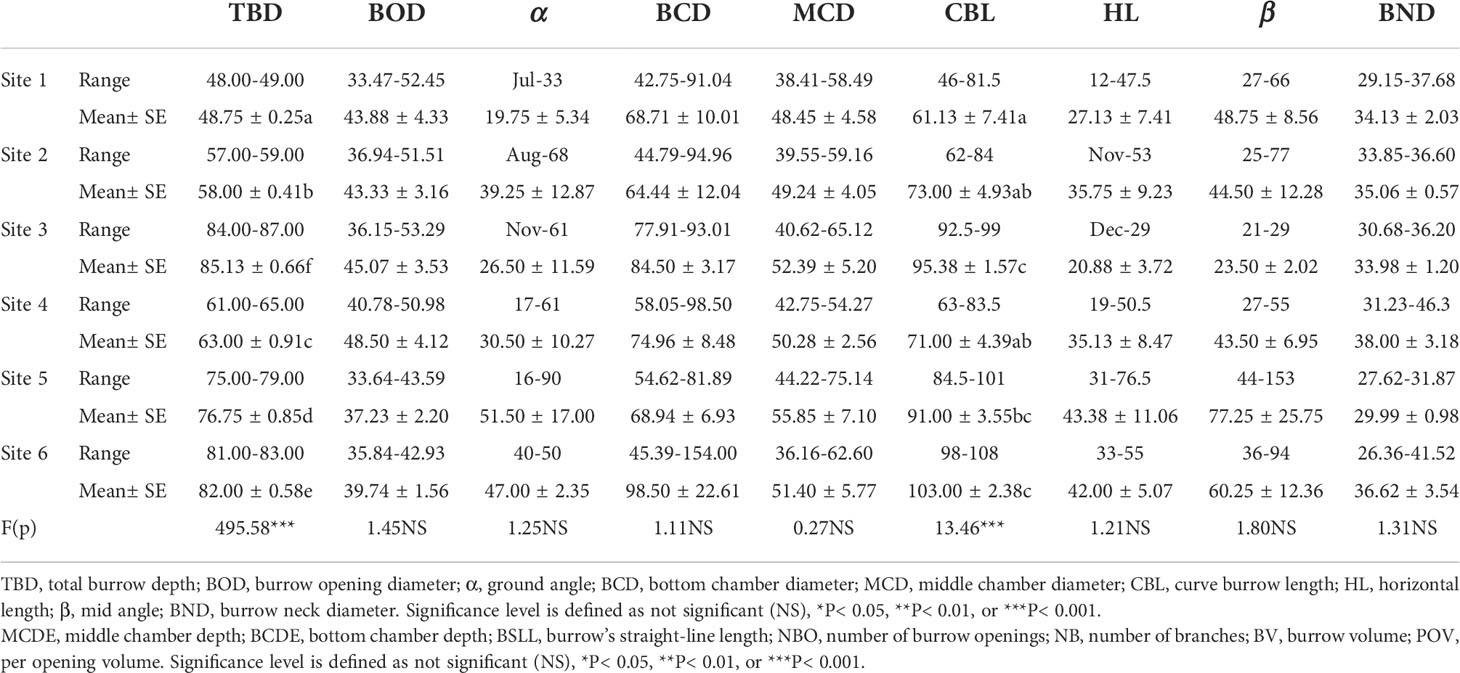
Table 1A. Burrow morphology nomenclature of Helice tientsinensis at each site. Table 1B. Burrow morphology nomenclature of Helice tientsinensis at each site.
Main drivers of burrow morphology
Mound diameter was directly dependent on crab size; larger crabs (large carapace length and width) produced a larger burrow mound diameter (Figure 7). PCA was performed on burrow morphology datasets (black arrow in Figure 8), and the sediment properties involved buck density, salinity, water content, hardness, and water depth (red arrow in Figure 8). The eigenvalues of the first two PCA axes were 0.310 and 0.209, while axes 1 and 2 explained 51.97% of the data. For estimating crab excavation, the TBD, BV, and CBL were the key indicators (purple arrow in Figure 8). Water depth was strongly and positively correlated with TBD, CBL, BV and BSLL. Soil hardness was strongly and negatively correlated with TBD and CBL. Soil bulk density was strongly and negatively correlated with TBD.
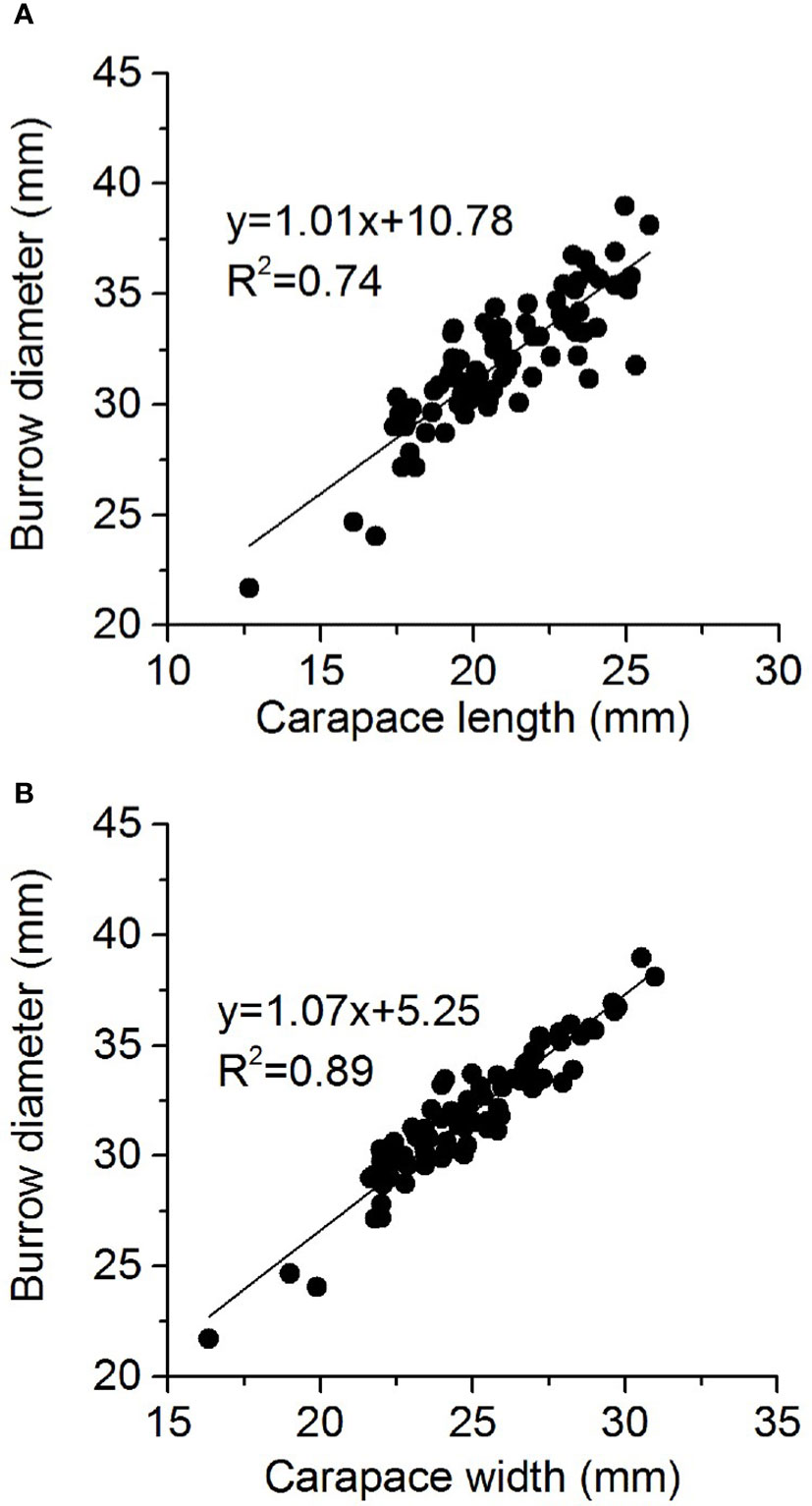
Figure 7 Relationship between Helice tientsinensis size (A): carapace length and (B): carapace width) and burrow diameter.
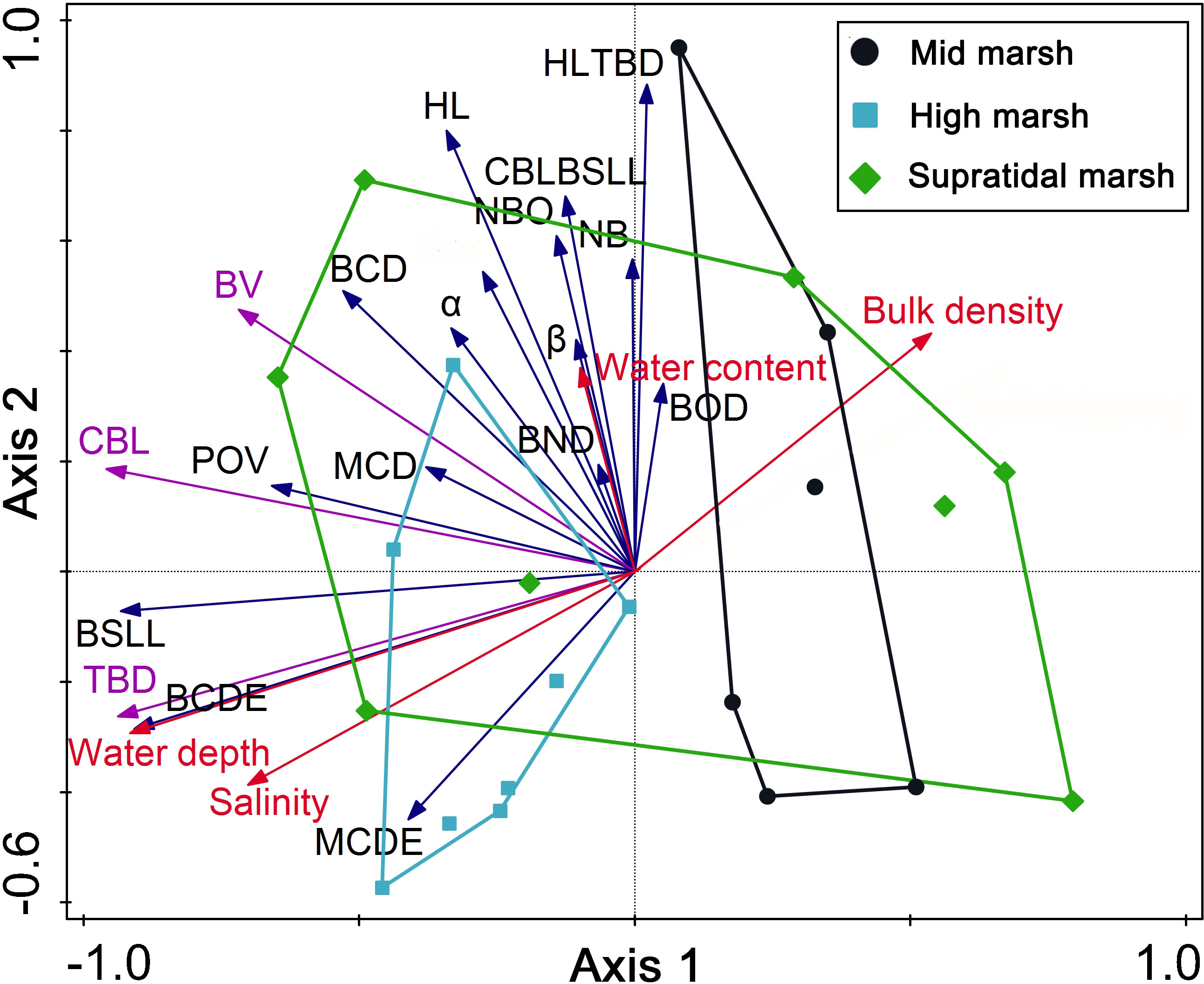
Figure 8 Relationships between abiotic factors and crab burrow morphological characteristics using PCA. Codes for morphological characteristics are shown in Table 1. Abiotic factors are represented by red arrows. The morphological characteristics are represented by black arrows. The key morphology indicators for estimating crab excavation are represented by purple arrows. The region surrounded by colorful lines indicate a cluster of sampling sites into the three salt marsh zones.
Sediment excavation and turnover rate
Sediment and nutrient excavations were estimating from BV and stratified morphological characteristics. The amounts of sediment TN, TC, and OM in excavated soil differed among the three salt marsh zones (Figure 9). The TN and TC of excavated sediment were the highest in the supratidal marsh (sediment: F = 7.178, P< 0.001; TN: F = 11.060, P< 0.001; TC: F = 7.852, P< 0.001). The OM excavated in the middle marsh was higher than in the other two marsh zones (F = 21.161, P< 0.01).
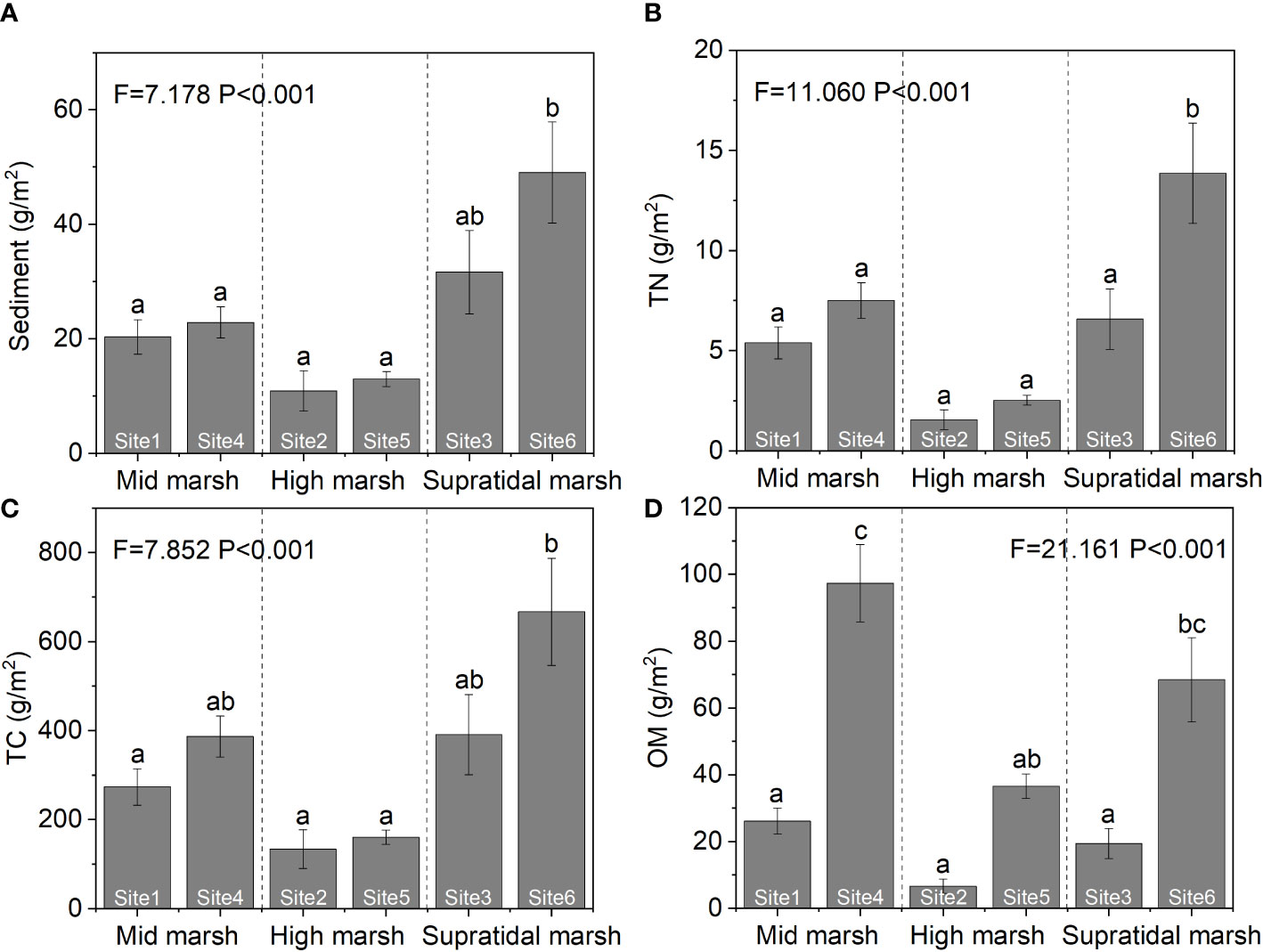
Figure 9 Contributions of the ecosystem engineers, (H) tientsinensis, to sediment (A), TN (B), TC (C), and OM (D) excavation among the three marsh zones in the Yellow River Delta. Data are shown as means ± SE. Letters indicate significant differences with a Tukey test.
There was no significant difference in the daily excavated sediment rate among the three salt marsh zones. In middle and high marsh zones, the amount of sediment deposited into the PVC pipes did not differ between the burrow mimics with and without crab burrow mounds. However, in the supratidal marsh zone, the amount of sediment deposited into the PVC pipes was greater in mimic burrows with a burrow mound than in mimic burrows without a burrow mound (F = 11.92, P< 0.01). In all three salt marsh zones, daily crab excavated sediment amounts (~32.59 g·d-1·m-2) were considerably larger than daily deposited sediment amounts (~0.57 g·d-1·m-2 PVC pipe with crab burrow or 0.34 g·d-1·m-2 PVC pipe without crab burrow). TN, TC, and OM showed similar trends. Thus, sediment and nutrient net transported amounts were mainly from the belowground soil to the soil surface (Figure 10).
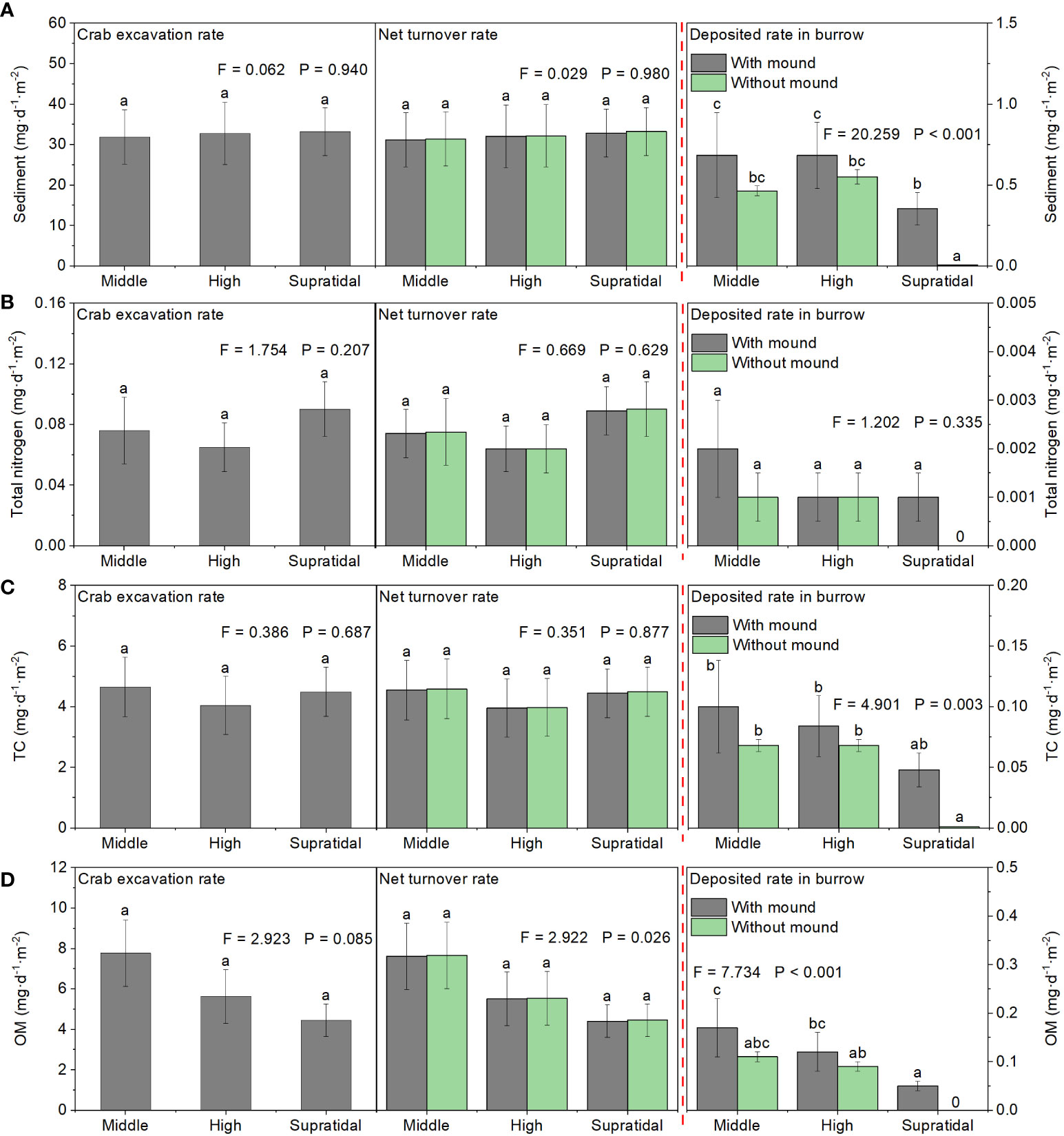
Figure 10 Estimated effects of crab excavation on sediment (A), TN (B), TC (C), and OM (D) daily turnover rates among the different marsh zones. Data are shown as means ± SE. Letters indicate significant differences with a Tukey test.
Discussion
Semi-terrestrial crabs affect the distribution, quality, and composition of sediment through their burrowing activities (Qiu et al., 2019; Ren et al., 2022), consequently affecting the structure and dynamics of other biotic communities. Physical, chemical, and biological processes disturbed by animals are known as the “bioturbation” (Xie et al., 2020). Burrowing crabs are considered key ecosystem engineers in coastal ecosystems. Crab bioturbation activity in coastal ecosystems can be examined through the analysis of burrow morphology and local sediment properties.
Relationship between crab size and burrow diameter
Several previous studies have shown that crab burrow diameter was positively correlated with crab carapace size (Lourenco et al., 2008; de Oliveira et al., 2016), consistent with the present findings. Different crab species have different coefficients of crab burrow diameter and crab carapace width (Saher and Qureshi, 2011; Sen and Homechaudhuri, 2018), which indicates species-specific characteristics. Burrowing crabs create a larger burrow diameter than their carapace width, allowing them to move in and out of the burrow comfortably, and providing them a fast escape route when under threat.
Burrow morphology characteristics and their main drivers
Burrow morphology characteristics not only indicate crab species-specific behavior and preferences, but also indicate local environmental conditions. Burrows have numerous functions and are essential throughout the entire crab life cycle. Crabs start digging burrows from a very young age (Saher and Qureshi, 2011). Burrows can protect them from excessive wave action and enable them to avoid extreme temperatures, as well as provide a refuge against predators during rest and egg incubation (Sen and Homechaudhuri, 2018). The present results showed that burrows of H. tientsinensis in all six study sites were simple, commonly with one or two branches, and ending in a single large bottom chamber. Crab burrows always reach the water table to maintain inside moisture during the whole tidal cycle (Iribarne et al., 1997).
Burrow morphology complexity also has important functions. For example, all burrows have a large bottom chamber, which provides a resting room for their entire life and a safe place for mating. Each burrow usually has a ground angle at the burrow entrance, the burrow neck diameter is usually smaller than the burrow opening diameter, and at ~15 cm depth there is a middle chamber, all of which provide a means of a fast escape from threats.
The present results showed that crab size, water depth, and bulk density are related to burrow diameter, TBD, CBL, BSLL, and BV. The CBL and mid angle indicate the difficulty of burrow construction. Previous studies have shown that plant characteristics affect burrow morphology (Ringold, 1979; Wang et al., 2015), and the number of burrow openings, and branches can be influenced by plant roots. However, in the present study, the influence of plant roots was not considered because the study areas were covered by patches of the annual plant species S. salsa, which commonly has short roots (less than 15 cm).
Crab excavation and sediment turnover
Crab burrowing activities include building new burrows, maintaining or reconstructing burrows through pushing mud out of the burrow and retreating back into burrow, opening the burrow entrance by pushing aside the mud at the entrance, and closing the burrow entrance with mud from inside the burrow (Nordhaus et al., 2009). Thus, burrows receiving crab maintenance and reconstruction will not trap much sediment, as simulated in the present study. The burrow mimic can be used to simulate abandoned burrows, for example when the burrows owner has been preyed on by water birds or other large predators. The amount of adult crab excavation through their burrow cast can be estimated, but it is not clear how long it takes an adult crab to complete their burrow construction. Many previous studies have examined crab burrow morphology (Lim, 2003; Saher and Qureshi, 2011; Machado et al., 2013), but few have estimated the amount of crab excavation. Wang et al. (2010) estimated the amount of crab excavation through 5-days of sediment collection, while in the present study, daily crab excavation and deposited amounts were estimated, in addition to the total amount of adult crab excavation through their burrow cast and local sediment bulk density.
Because crab burrowing activities not only include the burrow construction period, but also the burrow maintenance period (Nordhaus et al., 2009), the 7-day sediment collection period in the present study could not reflect the crab excavation rate accurately. However, the field survey was conducted in spring, which is probably the right time period for new burrow building. To improve the accuracy of crab excavation amount estimation, further studies are needed.
The present results also showed that sediment excavation by crabs was ~50 times greater than that of sediment deposition within crab burrow mimics, similar to previous studies (Gutierrez et al., 2006; Wang et al., 2010). Meanwhile, crab burrowing activities promote underground sediment and nutrient cycling vertically, and enhance the sediment and nutrient transfer on the soil surface horizontally (Wang et al., 2010; Alberti et al., 2015). However, there are some biases in the estimation of nutrient turnover rate based on the survey for sediment cores in this study, because excavated sediments are exposed to the air and the nutrients are easily mineralised under aerated conditions compared with nutrients in sediments.
In summary, crab burrowing activities are known to increase sediment-water exchange, promote substrate drainage and oxidation, accelerate plant debris decomposition, and enhance the growth of substrate micro-organisms (Lim, 2003). Crabs can directly and indirectly influence many ecological processes through their bioturbation and herbivory (Alberti et al., 2015). Although it is currently unclear how long it takes an adult crab to build a complete burrow, the present results still highlighted the important functions of crabs in sediment and nutrient cycling. Thus, this study has begun to fill the knowledge gap in understanding the contributions of crabs to sediment and nutrient turnover, and will facilitate managers in understanding the roles of crabs in coastal ecosystems, especially for salt marsh protection and restoration.
Data availability statement
The original contributions presented in the study are included in the article/supplementary material. Further inquiries can be directed to the corresponding author.
Author contributions
TX, AW, and SL conceived the ideas and designed methodology. TX and SL collected the data. TX, AW, and SL conducted all the experiments. BC, JB and DS analyzed the data. TX, BC, JB and DS led the writing of the manuscript. All authors contributed critically to the drafts and gave final approval for publication.
Funding
We acknowledge the financial support from the National Research Program of China (2019YFE0121500) and the National Science Foundation for Young Scientists of China (51909006).
Conflict of interest
The authors declare that the research was conducted in the absence of any commercial or financial relationships that could be construed as a potential conflict of interest.
Publisher’s note
All claims expressed in this article are solely those of the authors and do not necessarily represent those of their affiliated organizations, or those of the publisher, the editors and the reviewers. Any product that may be evaluated in this article, or claim that may be made by its manufacturer, is not guaranteed or endorsed by the publisher.
References
Alberti J., Daleo P., Fanjul E., Escapa M., Botto F., Iribarne O. (2015). Can a single species challenge paradigms of salt marsh functioning? Estuaries coasts 38 (4), 1178–1188. doi: 10.1007/s12237-014-9836-z
Alberti J., Escapa M., Iribarne O., Silliman B., Bertness M. (2008). Crab herbivory regulates plant facilitative and competitive processes in argentinean marshes. Ecology 89 (1), 155–164. doi: 10.1890/07-0045.1
Bai J., Wang J., Yan D., Gao H., Xiao R., Shao H., et al. (2012). Spatial and temporal distributions of soil organic carbon and total nitrogen in two marsh wetlands with different flooding frequencies of the yellow river delta, China. CLEAN–Soil Air Water 40 (10), 1137–1144. doi: 10.1002/clen.201200059
Bang J. H., Lee E. J. (2019). Differences in crab burrowing and halophyte growth by habitat types in a Korean salt marsh. Ecol. Indic. 98, 599–607. doi: 10.1016/j.ecolind.2018.11.029
Bianchini A., Lauer M. M., Nery L. E. M., Colares E. P., Monserrat J. M., dos Santos Filho E. A. (2008). Biochemical and physiological adaptations in the estuarine crab Neohelice granulata during salinity acclimation. Comp. Biochem. Physiol. Part A: Mol. Integr. Physiol. 151 (3), 423–436. doi: 10.1016/j.cbpa.2007.12.001
Botto F., Iribarne O. (1999). Effect of the burrowing crab Chasmagnathus granulata (Dana) on the benthic community of a SW Atlantic coastal lagoon. J. Exp. Mar. Biol. Ecol. 241 (2), 263–284. doi: 10.1016/S0022-0981(99)00089-1
Canepuccia A. D., Fanjul M. S., Fanjul E., Botto F., Iribarne O. O. (2008). The intertidal burrowing crab Neohelice (= chasmagnathus) granulata positively affects foraging of rodents in south western Atlantic salt marshes. Estuaries coasts 31 (5), 920–930. doi: 10.1007/s12237-008-9076-1
Chen H., Hagerty S., Crotty S. M., Bertness M. D. (2016). Direct and indirect trophic effects of predator depletion on basal trophic levels. Ecology 97 (2), 338–346. doi: 10.1890/15-0900.1
Christy J. H. (1987). Female choice and the breeding behavior of the fiddler crab Uca beebei. J. Crustacean Biol. 7 (4), 624–635. doi: 10.2307/1548648
de Oliveira C. A., Souza G. N., Soares-Gomes A. (2016). Measuring burrows as a feasible non-destructive method for studying the population dynamics of ghost crabs. Mar. biodiversity 46 (4), 809–817. doi: 10.1007/s12526-015-0436-3
Fanjul E., Escapa M., Montemayor D., Addino M., Alvarez M. F., Grela M. A., et al. (2015). Effect of crab bioturbation on organic matter processing in south West Atlantic intertidal sediments. J. Sea Res. 95, 206–216. doi: 10.1016/j.seares.2014.05.005
Gutiérrez J. L., Jones C. G., Groffman P. M., Findlay S. E., Iribarne O. O., Ribeiro P. D., et al. (2006). The contribution of crab burrow excavation to carbon availability in surficial salt-marsh sediments. Ecosystems 9 (4), 647–658. doi: 10.1007/s10021-006-0135-9
He Q., Altieri A. H., Cui B. (2015). Herbivory drives zonation of stress-tolerant marsh plants. Ecology 96 (5), 1318–1328. doi: 10.1890/14-0937.1
He Q., Cui B., An Y. (2012). Physical stress, not biotic interactions, preclude an invasive grass from establishing in forb-dominated salt marshes. PLoS One 7 (3), e33164. doi: 10.1371/journal.pone.0033164
Heng M., Lim S. (2007). Mangrove micro-habitat influence on bioturbative activities and burrow morphology of the fiddler crab, Uca annulipes (H. Milne Edward) (Decapoda, ocypodidae). Crustaceana 80 (1), 31–45. doi: 10.1163/156854007779696488
He Q., Silliman B. R., Liu Z., Cui B. (2017). Natural enemies govern ecosystem resilience in the face of extreme droughts. Ecol. Lett. 20 (2), 194–201. doi: 10.1111/ele.12721
Iribarne O., Bortolus A., Botto F. (1997). Between-habitat differences in burrow characteristics and trophic modes in the southwestern Atlantic burrowing crab Chasmagnathus granulata. Mar. Ecol. Prog. Ser. 155, 137–145. doi: 10.3354/meps155137
Katrak G., Dittmann S., Seuront L. (2008). Spatial variation in burrow morphology of the mud shore crab Helograpsus haswellianus (Brachyura, grapsidae) in south Australian saltmarshes. Mar. Freshw. Res. 59 (10), 902–911. doi: 10.1071/MF08044
Koo B. J., Kwon K. K., Hyun J. H. (2007). Effect of environmental conditions on variation in the sediment-water interface created by complex macrofaunal burrows on a tidal flat. J. Sea Res. 58 (4), 302–312. doi: 10.1016/j.seares.2007.07.002
Leoville A., Lagarde R., Grondin H., Faivre L., Rasoanirina E., Teichert N. (2021). Influence of environmental conditions on the distribution of burrows of the mud crab, Scylla serrata, in a fringing mangrove ecosystem. Regional Stud. Mar. Sci. 43, 101684. doi: 10.1016/j.rsma.2021.101684
Li S., Cui B., Xie T., Shao X., Zhang M. (2016a). Consequences and implications of anthropogenic desalination of salt marshes on macrobenthos. CLEAN–Soil Air Water 44 (1), 8–15. doi: 10.1002/clen.201400787
Li S., Cui B., Xie T., Zhang K. (2016b). Diversity pattern of macrobenthos associated with different stages of wetland restoration in the yellow river delta. Wetlands 36 (1), 57–67. doi: 10.1007/s13157-015-0641-7
Lim S. (2003). Burrow-morphological characters of the fiddler crab, Uca annulipes (H. Milne edwards 1837) and ecological correlates in a lagoonal beach on pulau hantu, Singapore. Crustaceana 76 (9), 1055–1069. doi: 10.1163/156854003322753411
Lim S. (2006). Fiddler crab burrow morphology: how do burrow dimensions and bioturbative activities compare in sympatric populations of Uca vocans (Linnaeus 1758) and u. annulipes (H. Milne edwards 1837)? Crustaceana 79 (5), 525–540. doi: 10.1163/156854006777584241
Lim S., Yong A. Y., Tantichodok P. (2011). Comparison of burrow morphology of juvenile and young adult Ocypode ceratophthalmus from sai kaew, Thailand. J. Crustacean Biol. 31 (1), 59–65. doi: 10.1651/10-3314.1
Lourenço R., Paula J. R., Henriques M. (2008). Estimating the size of" uca tanger"(Crustacea: Ocypodidae) without massive crab capture. Scientia Marina 64 (4), 437–439. doi: 10.3989/scimar.2000.64n4437
Machado G. B., Gusmão-Junior J. B., Costa T. M. (2013). Burrow morphology of Uca uruguayensis and Uca leptodactylus (Decapoda: Ocypodidae) from a subtropical mangrove forest in the western Atlantic. Integr. Zoology 8 (3), 307–314. doi: 10.1111/j.1749-4877.2012.00297.x
Martinetto P., Palomo G., Bruschetti M., Iribarne O. (2011). Similar effects on sediment structure and infaunal community of two competitive intertidal soft-bottom burrowing crab species. J. Mar. Biol. Assoc. United Kingdom 91 (7), 1385–1393. doi: 10.1017/S0025315411000075
McLain D. K., Pratt A. E., Berry A. S. (2003). Predation by red-jointed fiddler crabs on congeners: interaction between body size and positive allometry of the sexually selected claw. Behav. Ecol. 14 (5), 741–747. doi: 10.1093/beheco/arg065
Nordhaus I., Diele K., Wolff M. (2009). Activity patterns, feeding and burrowing behaviour of the crab Ucides cordatus (Ucididae) in a high intertidal mangrove forest in north Brazil. J. Exp. Mar. Biol. Ecol. 374 (2), 104–112. doi: 10.1016/j.jembe.2009.04.002
Ouyang X., Lee S. Y., Connolly R. M. (2017). Structural equation modelling reveals factors regulating surface sediment organic carbon content and CO2 efflux in a subtropical mangrove. Sci. Total Environ. 578, 513–522. doi: 10.1016/j.scitotenv.2016.10.218
Ouyang X., Lee C. Y., Lee S. Y. (2021). Effects of food and feeding regime on CO2 fluxes from mangrove consumers–do marine benthos breathe what they eat? Mar. Environ. Res. 169, 105352. doi: 10.1016/j.marenvres.2021.105352
Penha-Lopes G., Kristensen E., Flindt M., Mangion P., Bouillon S., Paula J. (2010). The role of biogenic structures on the biogeochemical functioning of mangrove constructed wetlands sediments–a mesocosm approach. Mar. pollut. Bull. 60 (4), 560–572. doi: 10.1016/j.marpolbul.2009.11.008
Pennings S. C., Carefoot T. H., Siska E. L., Chase M. E., Page T. A. (1998). Feeding preferences of a generalist salt-marsh crab: relative importance of multiple plant traits. Ecology 79 (6), 1968–1979. doi: 10.1890/0012-9658(1998)079[1968:FPOAGS]2.0.CO;2
Pennings S. C., Selig E. R., Houser L. T., Bertness M. D. (2003). Geographic variation in positive and negative interactions among salt marsh plants. Ecology 84 (6), 1527–1538. doi: 10.1890/0012-9658(2003)084[1527:GVIPAN]2.0.CO;2
Qiu D., Cui B., Yan J., Ma X., Ning Z., Wang F., et al. (2019). Effect of burrowing crabs on retention and accumulation of soil carbon and nitrogen in an intertidal salt marsh. J. Sea Res. 154, 101808. doi: 10.1016/j.seares.2019.101808
Reinsel K. A., Rittschof D. (1995). Environmental regulation of foraging in the sand fiddler crab Uca pugilator (Bosc 1802). J. Exp. Mar. Biol. Ecol. 187 (2), 269–287. doi: 10.1016/0022-0981(94)00192-G
Ren L., Jensen K., Porada P., Mueller P. (2022). Biota-mediated carbon cycling–a synthesis of biotic-interaction controls on blue carbon. Ecol. Lett. 25 (2), 521–540. doi: 10.1111/ele.13940
Ribeiro P. D., Iribarne O. O., Daleo P. (2005). The relative importance of substratum characteristics and recruitment in determining the spatial distribution of the fiddler crab Uca uruguayensis nobili. J. Exp. Mar. Biol. Ecol. 314 (1), 99–111. doi: 10.1016/j.jembe.2004.09.014
Ringold P. (1979). Burrowing, root mat density, and the distribution of fiddler crabs in the eastern united states. J. Exp. Mar. Biol. Ecol. 36 (1), 11–21. doi: 10.1016/0022-0981(79)90097-2
Saher U. S., Qureshi N. A. (2011). Burrow morphology of three species of fiddler crab (Uca) along the coast of Pakistan. Belgian J. Zoology 142 (2), 114–126.
Schlacher T. A., Lucrezi S., Peterson C. H., Connolly R. M., Olds A. D., Althaus F., et al. (2016). Estimating animal populations and body sizes from burrows: Marine ecologists have their heads buried in the sand. J. Sea Res. 112, 55–64. doi: 10.1016/j.seares.2016.04.001
Sen S., Homechaudhuri S. (2018). Comparative burrow architectures of resident fiddler crabs (Ocypodidae) in Indian sundarban mangroves to assess their suitability as bioturbating agents. Proc. Zoological Soc. 71 (1), 17–24. doi: 10.1007/s12595-016-0178-7
Spivak E., Anger K., Luppi T., Bas C., Ismael D. (1994). Distribution and habitat preferences of two grapsid crab species in mar chiquita lagoon (Province of Buenos Aires, Argentina). Helgoländer Meeresuntersuchungen 48 (1), 59–78. doi: 10.1007/BF02366202
Stelling-Wood T. P., Clark G. F., Poore A. G. (2016). Responses of ghost crabs to habitat modification of urban sandy beaches. Mar. Environ. Res. 116, 32–40. doi: 10.1016/j.marenvres.2016.02.009
Stieglitz T., Ridd P., Müller P. (2000). Passive irrigation and functional morphology of crustacean burrows in a tropical mangrove swamp. Hydrobiologia 421 (1), 69–76. doi: 10.1023/A:1003925502665
Thongtham N., Kristensen E. (2003). Physical and chemical characteristics of mangrove crab (Neoepisesarma versicolor) burrows in the bangrong mangrove forest, phuket, thailand; with emphasis on behavioural response to changing environmental conditions. Vie Milieu/Life Environ. 53 (4), 141–151.
Vu H. D., Wie Ski K., Pennings S. C. (2017). Ecosystem engineers drive creek formation in salt marshes. Ecology 98 (1), 162–174. doi: 10.1002/ecy.1628
Wang J. Q., Bertness M. D., Li B., Chen J. K., Lü W. G. (2015). Plant effects on burrowing crab morphology in a Chinese salt marsh: Native vs. exotic plants. Ecol. Eng. 74, 376–384. doi: 10.1016/j.ecoleng.2014.11.019
Wang M., Gao X., Wang W. (2014). Differences in burrow morphology of crabs between spartina alterniflora marsh and mangrove habitats. Ecol. Eng. 69, 213–219. doi: 10.1016/j.ecoleng.2014.03.096
Wang J. Q., Zhang X. D., Jiang L. F., Bertness M. D., Fang C. M., Chen J. K., et al. (2010). Bioturbation of burrowing crabs promotes sediment turnover and carbon and nitrogen movements in an estuarine salt marsh. Ecosystems 13 (4), 586–599. doi: 10.1007/s10021-010-9342-5
Xie T., Dou P., Li S., Cui B., Bai J., Wang Q., et al. (2020). Potential effect of bioturbation by burrowing crabs on sediment parameters in coastal salt marshes. Wetlands 40 (6), 2775–2784. doi: 10.1007/s13157-020-01341-1
Xie T., Li S., Cui B., Bai J., Wang Q., Shi W. (2019). Rainfall variation shifts habitat suitability for seedling establishment associated with tidal inundation in salt marshes. Ecol. Indic. 98, 694–703. doi: 10.1016/j.ecolind.2018.11.056
Xin P., Jin G., Li L., Barry D. A. (2009). Effects of crab burrows on pore water flows in salt marshes. Adv. Water Resour. 32 (3), 439–449. doi: 10.1016/j.advwatres.2008.12.008
Keywords: bioturbation, burrowing crab, nutrients, sediment, yellow river delta
Citation: Xie T, Wang A, Li S, Cui B, Bai J and Shao D (2022) Crab contributions as an ecosystem engineer to sediment turnover in the Yellow River Delta. Front. Mar. Sci. 9:1019176. doi: 10.3389/fmars.2022.1019176
Received: 14 August 2022; Accepted: 20 September 2022;
Published: 04 October 2022.
Edited by:
Nathan John Waltham, James Cook University, AustraliaReviewed by:
Xiaoguang Ouyang, Southern Marine Science and Engineering Guangdong Laboratory, ChinaJeffrey Cornwell, University of Maryland, United States
Xianbiao Lin, Ocean University of China, China
Copyright © 2022 Xie, Wang, Li, Cui, Bai and Shao. This is an open-access article distributed under the terms of the Creative Commons Attribution License (CC BY). The use, distribution or reproduction in other forums is permitted, provided the original author(s) and the copyright owner(s) are credited and that the original publication in this journal is cited, in accordance with accepted academic practice. No use, distribution or reproduction is permitted which does not comply with these terms.
*Correspondence: Andong Wang, bhqkyz@126.com