- 1Marine Environment Research Division, National Institute of Fisheries Science, Busan, South Korea
- 2Division of Earth Environmental System, Pusan National University, Busan, South Korea
Regenerated nitrogen (N) cycling was studied in a turbid and nutrient-rich estuary located in the southeast region of the Yellow Sea (Yeongsan River Estuary; YRE), in order to elucidate the biogeochemical consequences of coastal development. Ammonium regeneration and potential uptake rates were measured from March 2012 to June 2013 using 15N tracer techniques. Size fractionation suggested that small-sized bacteria (<0.7 μm), rather than zooplankton, were responsible for most of the ammonium regeneration. Intermittent freshwater discharge events might have prevented stable zooplankton community development and caused the insignificant role of zooplankton in ammonium regeneration. Ammonium regeneration and potential uptake rates were relatively high (0.1–1.2 and 0.2–1.5 µmol L−1 h−1, respectively), and were highest during summer. Ammonium turnover times were shorter than water residence times throughout the study period. These results indicate that ammonium is actively recycled and is likely to supply enough N required to sustain the high primary productivity observed in the YRE (50%–450%). Reduced turbidity and increased water residence times caused by the construction of an estuarine dam in the YRE have probably resulted in the formation of optimal conditions for the high ammonium regeneration.
Introduction
The Yellow Sea is a shallow marginal sea situated between mainland China and the Korean Peninsula. Increased anthropogenic nitrogen (N) inputs to coastal regions from rivers in China and the Korean Peninsula stimulate eutrophication, trigger the formation of hypoxic zones, and influence the structure and functioning of these coastal ecosystems (Wei et al., 2007; Zhang et al., 2007; Rabalais et al., 2010; Hyun et al., 2020). However, ecological responses to N inputs in this region have been poorly studied.
The ecological implications of N loading in an estuary depend on the estuary’s physical and biogeochemical characteristics (Heip et al., 1995; An and Gardner, 2002; Middelburg and Herman, 2007; Bianchi, 2007). Dissolved inorganic N (DIN) inputs from land, usually in the form of nitrate () are assimilated into particulate organic N (PON) during autochthonous production; some of the PON is recycled into DIN during microbial regeneration, usually in the form of ammonium (). The degree of → PON → conversion may be important for primary productivity because ammonium is the preferred N source for phytoplankton (McCarthy et al., 1977). Many studies have reported that the uptake and turnover rates of ammonium in estuarine environments are higher than those of nitrate (Middelburg and Nieuwenhuize, 2000a; Yuan et al., 2012).
Ammonium regeneration is mainly mediated by bacteria, protozoa and zooplankton. The contribution of bacteria to water column ammonium regeneration differed according to the coastal characteristics such as the temperature, plankton community structure and supply of microbial substates (Gardner et al., 1997; Jochem et al., 2004). For example, Picoplankton (mainly associated with bacteria) contributed significantly to ammonium regeneration during the summer (20-45%), while nanoplankton (mainly associated with ciliates) accounted for 83-88% of the regeneration in winter in the shallow well-mixed water (Maguer et al., 1999). Maguer et al. (1999) suggested that the contribution of picoplankton versus nanoplankton to total ammonium regeneration is regulated by seasonal planktonic community structure. Regenerated ammonium is consumed by processes such as nitrification and assimilation of phytoplankton and heterotrophic bacteria. Photosynthetic ammonium uptake tends to be light-dependent, whereas heterotrophic uptake is largely regulated by the availability of organic substrates (Gardner et al., 2004). The relative importance of ammonium uptake between phytoplankton and heterotrophic bacteria can be demonstrated by light-dark incubations. (Gardner et al., 2004; McCarthy et al., 2009, 2013). In several aquatic systems including Mississippi River plume, Lake Champlain, Western Lake Erie, the light ammonium uptake rates were higher than dark rates suggesting that predominance of photosynthetic uptake (Jochem et al., 2004; McCarthy et al., 2013; Hoffman et al., 2022).
Nutrients and grazing are generally the most important factors controlling phytoplankton productivity and biomass at the ocean surface (Tilman et al., 1982; Armstrong, 1994). However, physical factors, such as light availability, vertical mixing, and freshwater flushing, are also significant (Alpine and Cloern, 1988; Mallin and Paerl, 1992; Sin et al., 1999). The coastal waters of the western Korean Peninsula adjacent to the Yellow Sea are eutrophic; however, algal blooms are relatively rare in the region despite the high nutrient levels (Kim et al., 2013; Park et al., 2013). The rarity of algal blooms may be due to the high turbidity (and low light levels) caused by the high input of suspended solids from rivers and resuspension by the macrotidal (10 m range) regime (Son et al., 2005; Byun et al., 2007). In estuaries with low, rapidly changing light conditions, the N form (nitrate versus ammonium) may be important because nitrate (as an oxidized chemical species) is less likely to be coupled to primary productivity (Middelburg and Nieuwenhuize, 2000a; Yuan et al., 2012).
The Yeongsan River Estuary (YRE) is located in the southeastern Yellow Sea and receives high nutrient loads from a highly populated area (Gwangju; population 1.5 million) and as a result of agricultural practices. A dam was constructed at the mouth of the YRE in 1981 to secure freshwater for agricultural and industrial use (Yim et al., 2018). A large artificial lake was formed, with poor water quality (Lee et al., 2009). The characteristics of the estuary also changed dramatically after the dam construction, which caused reduced water circulation (Cho et al., 2004). The estuary is a tide-dominated, shallow, semi-enclosed bay with intermittent freshwater discharge regulated by dam sluice gates, which affect the physical and biogeochemical characteristics such as current, turbidity, nutrient concentration, and plankton dynamics (2015; Byun et al., 2004; Cho et al., 2009; Sin et al., 2013; Sin and Jeong, 2020).
Like other estuaries adjacent to the Yellow Sea, primary production in the YRE is controlled by physical factors, including vertical mixing, the concentrations of suspended solids, and temperature (Byun et al., 2007). However, the YRE has a higher primary productivity (36–8900 mg C m−2 d−1; Lee et al., 2011; Sin et al., 2015) than other adjacent estuaries without dams, most likely because turbidity is generally lower due to reduced tidal resuspension near the dam. Summer hypoxia has been reported in the bottom layer of the YRE, which is an unusual finding for a macrotidal estuary (Lim et al., 2006).
This study aims to estimate the ammonium regeneration and potential uptake rates in the YRE by measuring changes in ammonium isotope ratios (Gardner et al., 1995; An et al., 2013) during incubation experiments. An additional goal is to improve the understanding of N recycling in the YRE, where a combination of artificial (high anthropogenic N loads, dam construction) and natural (macrotidal regime, high suspended sediment loads) perturbations co-occur, and to examine how dam construction may alter N cycling in an estuarine system.
Materials and methods
Study area
The Yeongsan River (drainage area 3455 km2, length 129 km) is one of the four major rivers in South Korea that flow into the Yellow Sea. An estuarine dam was constructed, inside the dam, an artificial freshwater lake (the Youngsan Lake) was created. Youngsan Lake has experienced massive sediment accumulation since the dam was constructed (33.6% reduction of the total water storage capacity) (Lee et al., 2009). Restriction of tidal circulation and loading of anthropogenic nutrients and organic compounds from upstream have transformed the area into a eutrophic lake with frequent occurrences of algal blooms and oxygen depletion in the bottom waters (Lee et al., 2009). Outside the dam, a semi-enclosed bay (the YRE) was created. the YRE (2 km wide, 7 km long, average depth 14 m; Figure 1) has a semi-diurnal tidal cycle, with mean spring and neap ranges of approximately 6 and 3 m, respectively. Annual mean precipitation in the region is 1322 mm, and the estuary’s annual mean freshwater discharge is approximately 1.5 billion m3. More than half of this precipitation is concentrated in the summer monsoon season (July and August); therefore, freshwater discharge occurs mostly during summer (>80% of annual discharge; Cho et al., 2004). The estuary’s vertical temperature and salinity profiles are homogeneous until early June, due to vertical mixing. Thermohaline stratification occurs in summer as a result of increased surface temperatures and warmer freshwater discharges (Cho et al., 2004). This summer stratification is disrupted by heavy freshwater discharges, but redevelops quickly (in approximately two days) after cessation of discharge (Cho et al., 2014).
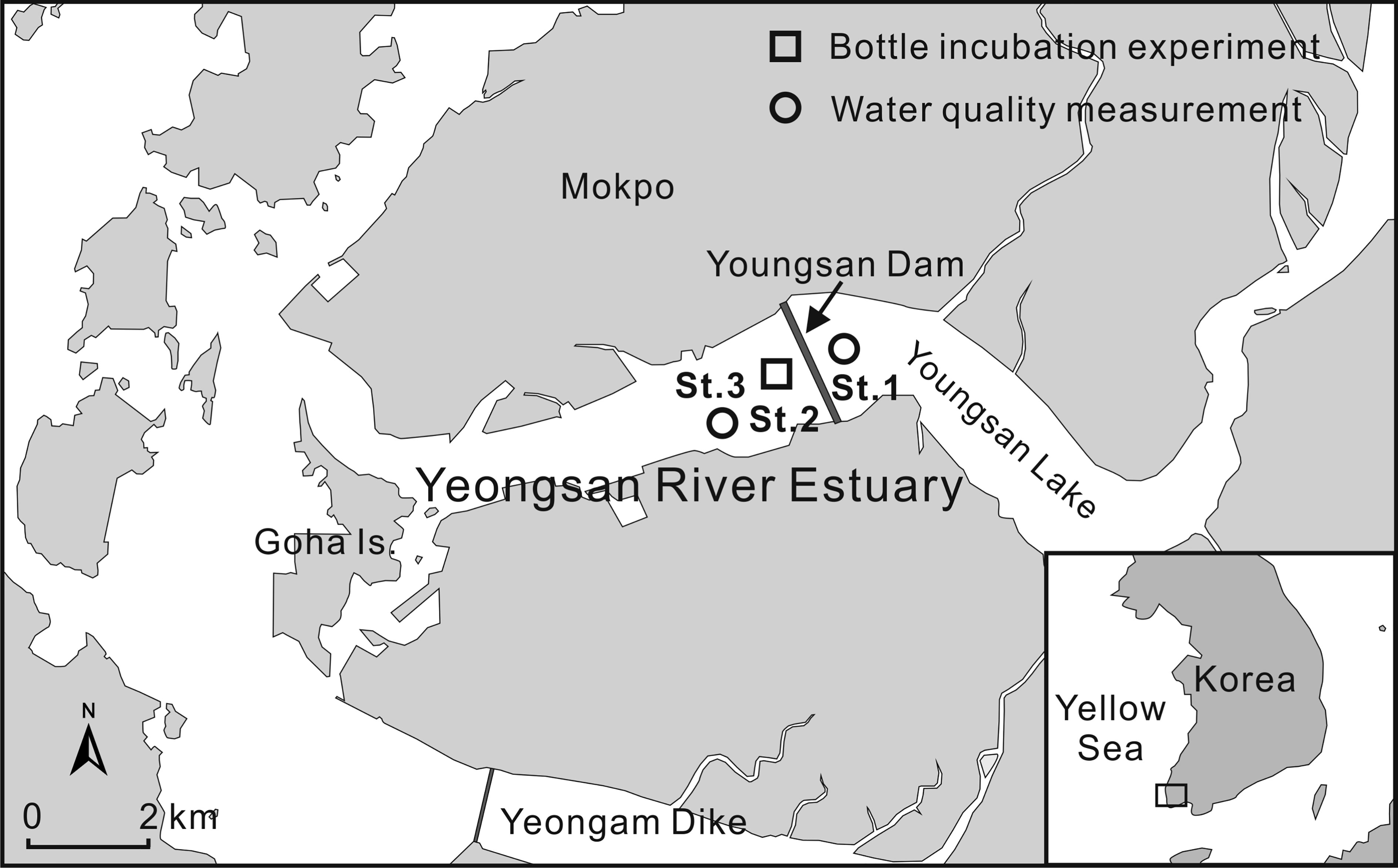
Figure 1 Locations of the study sites in the YRE. Water quality data for Sts. 1 and 3 were archived from the National Institute of Environmental Research and the Marine Environment Information System, respectively.
Experimental and analytical procedures
Isotope dilution experiments were conducted five times between March 2012 and June 2013 at St. 2 in the YRE (34°47’21.86”, 12626’23.06”; Figure 1) to estimate seasonal variations in ammonium recycling rates. Water samples for incubation experiments were collected 4–7 days after a freshwater discharge: salinities were similar to the outer estuarine bay (Table 1). Isotope dilution experiments were conducted as described in previous studies (Gardner et al., 1997; Jochem et al., 2004; McCarthy et al., 2013). Briefly, surface water samples were collected in Niskin bottles (at a sampling depth of 0.5 m) and spiked with 15 to achieve a final concentration of 8 µM (98 atom%; 15NH4Cl, Sigma-Aldrich). Water Samples were divided into six clear polystyrene culture bottles (75 cm2; SPL Lifesciences). Each experiment was performed in triplicate in light and dark (wrapped in aluminum foil) bottles. The bottles were floated on the surface of the sampling station and incubated for 24 h. Subsamples were collected from each sample after 15 addition (0 h) and after the incubation intervals. Subsamples were filtered over a 0.2 µm nylon filter (Whatman puradisc syringe filter); the resulting filtrates were collected in 10 ml glass vials (Wheaton) and frozen immediately. In June 2013, ammonium recycling rates were measured in three size fractions (whole fraction,<200 µm,<0.7 µm) to assess the contribution of phytoplankton and zooplankton to ammonium recycling (Jochem et al., 2004). The collected water samples were filtered using a 200 µm stainless steel sieve or Whatman GFF glass-fiber filters (0.7 µm pore size). Each filtrate (<200 µm and<0.7 µm) and unfiltered water sample was then treated according to the procedure described above.
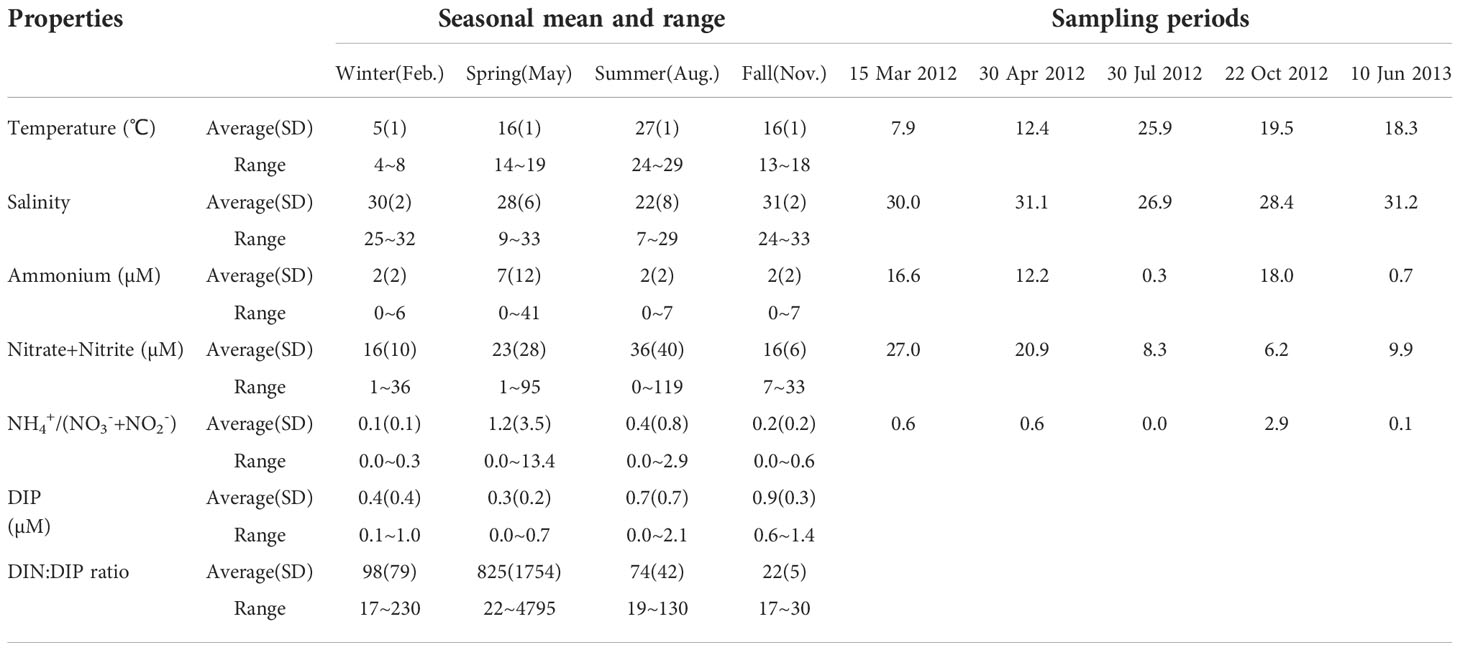
Table 1. Seasonal variations in temperature, salinity, ammonium concentration, nitrate + nitrite, ammonium/nitrate + nitrite ratios, dissolved inorganic phosphate (DIP), and DIN:DIP ratio during 2000–2013 obtained from Marine Environment Information System (St. 3) and the sampling periods in the YRE (St. 2).
Ammonium isotope ratios and concentrations were measured by high-performance liquid chromatography (HPLC) using a cation-exchange column as described in Gardner et al. (1995), but with a modified procedure using updated HPLC pumps as described in An et al. (2013). Ammonium regeneration and potential uptake rates were calculated from changes in ammonium concentration and isotope ratios over time, using the Blackburn–Caperon model (Blackburn, 1979; Caperon et al., 1979).
In the natural-light incubations in July 2012 and June 2013, ammonium was exhausted by the end of the 24 h incubation period; thus, the uptake and regeneration rates could not be obtained for these samples. However, minimum uptake rates were estimated from the initial ammonium concentrations of these samples and the regeneration rates of the dark incubations.
Sufficient amounts of 15 were added to the samples to prevent nutrient exhaustion over the incubation period (Gardner et al., 1997; McCarthy et al., 2013). In March, May, and October 2012, the added 15 was 44%–65% of the ambient levels. In contrast, in July 2012 and June 2013, when ambient ammonium levels were very low, 15 additions were 1100%–2800% of the ambient levels. The regeneration rates obtained in this study are considered to be the actual rates, as high-level nutrient addition should not affect regeneration rates (Blackburn, 1979), whereas uptake rates are regarded as potential rates (Gardner et al., 1997).
The ammonium and nitrate + nitrite concentrations of collected water samples at St. 2 were determined spectrophotometrically (Strickland and Parsons, 1972; Jones, 1984).
In order to find out the biogeochemical properties of Yeongsan Lake surface water that affects the study area, inorganic nutrient concentration (nitrate plus nitrite, ammonium, dissolved inorganic phosphorus [DIP]) data at St. 1 for 2002–2013 were obtained from the National Institute of Environmental Research (http://water.nier.go.kr) (Figure 2). Physico-chemical data at St. 3 near the survey point were also archived on the Marine Environment Information System (MEIS: http://www.meis.go.kr) website (Table 1). DIP data represented in this study were measured as soluble reactive phosphates (Strickland and Parsons, 1972). t test was conducted to explore the difference between light/dark and size-fraction incubation (whole fraction,<200 µm,<0.7 µm) of the regeneration and uptake rates. Regression analysis was also performed to assess the relationship between temperature with the regeneration and uptake rates. All statistical analyses were carried out using SPSS v20.0.
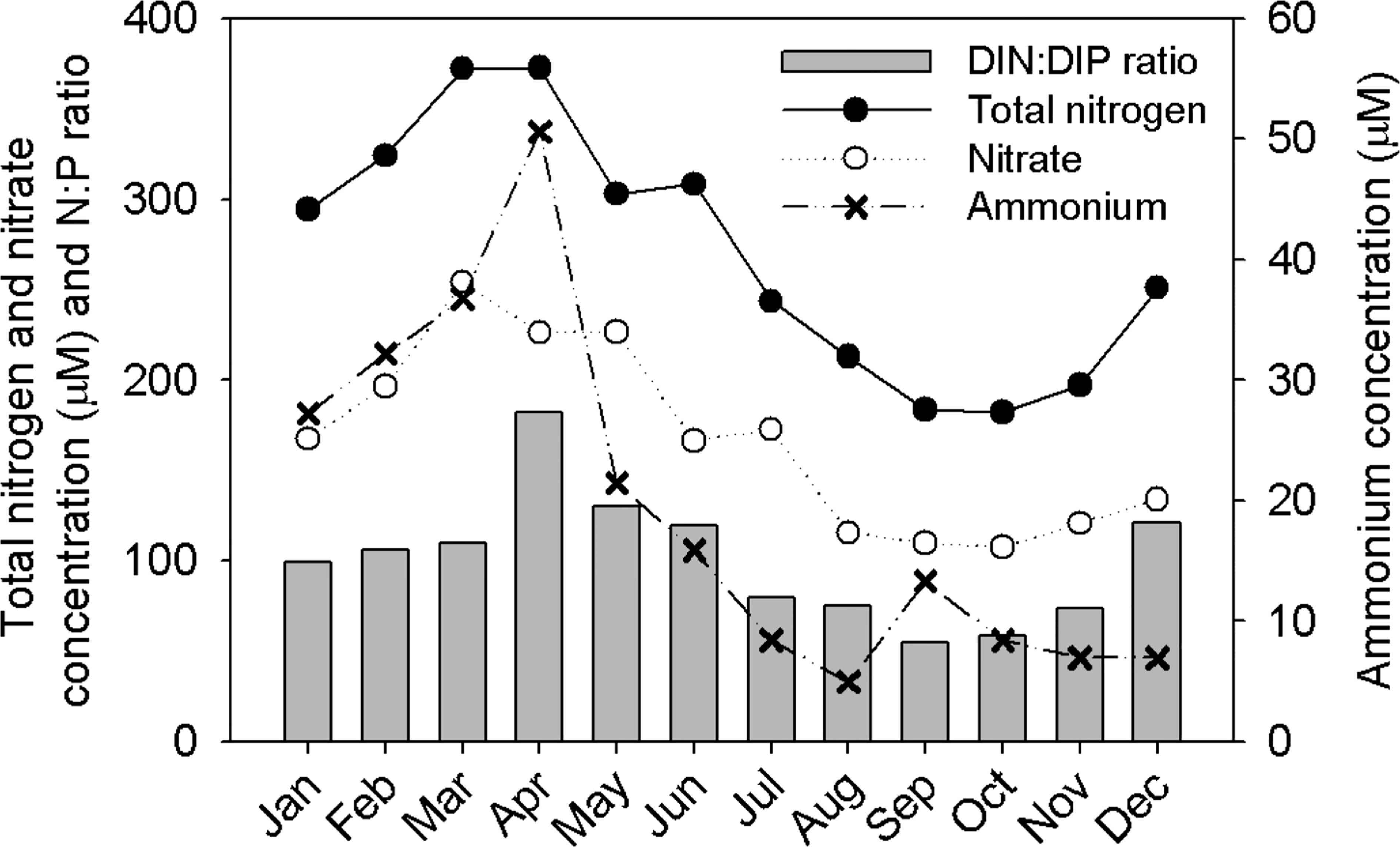
Figure 2 Monthly concentration variations in total nitrogen, inorganic nitrogen, and DIN:DIP ratios near the inside of the dam (Yeongsan Lake; St. 1), obtained from the National Institute of Environmental Research (http://water.nier.go.kr).
Results
During the incubation experiments, DIN concentrations were comparable to seasonal values in the YRE (Table 1). Nitrate + nitrite concentrations were usually high with large fluctuations in surface water throughout the year. On average, seasonal ammonium concentrations were an order of magnitude lower than those of nitrate (Table 1). However, the ammonium concentrations during the study period were usually higher than the seasonal mean value (Table 1). Surface-water nitrate concentrations were generally within the seasonal range obtained by MEIS (St. 3), whereas ammonium concentrations exceeded the seasonal range in spring and fall, and were less than 1 µM in summer (June and July). The average seasonal concentration of DIP was low, not exceeding 1 µM, and nutrient exhaustion was observed in the surface water in spring and summer. The DIN : DIP ratio exceeded the Redfield ratio all year round. The DIN : DIP ratio was highest in spring surface water, averaging 825, and was lowest in the fall, although still higher than the Redfield ratio of 16:1.
To identify the riverine input of nutrients into YRE, the monthly variations in total nitrogen, DIN, and DIN : DIP ratio near the inside of the dam were shown using data from the National Institute of Environmental Research (Figure 2). At St.1, the total and inorganic N concentrations tended to be high in spring (Figure 2). Inorganic N concentrations were high during all seasons, with ammonium and nitrate concentrations of approximately 10 and 100 µM, respectively, even during the summer, when the concentrations were at their lowest. The ratios of nitrate and ammonium to total N were 56%–88% and 2%–14%, respectively. The DIN : DIP ratio (55–182) at St. 1 was higher than the Redfield ratio (Figure 2). We calculated the DIN flux into the estuary through the dam sluice gates based on the DIN concentrations of the Yeongsan Lake and of the freshwater discharge. The DIN flux was 110–682 ton mon−1. DIN loading into the surveyed area was highest in summer.
Ammonium uptake rates varied with seasonal temperature and were highest in July 2012 (light uptake versus temperature, r2 = 0.57; dark uptake versus temperature, r2 = 0.59). Ammonium uptake rates were higher in light incubations than in the dark (Figure 3A), although the difference was only statistically significant in July 2012 (P< 0.001) and June 2013 (P< 0.05). Light enhancement (light uptake − dark uptake)/light uptake) ranged from 47% to 66%. Light uptake was significantly lower in the<0.7 µm fraction than in the whole fraction (P = 0.008) in June 2013 (Figure 3A). Uptake in the<0.7 µm fraction was similar in both light and dark incubations; light enhancement was not observed in this size fraction. Potential uptake rates among the size fractions were not significantly different in the dark incubations (Figure 3B).
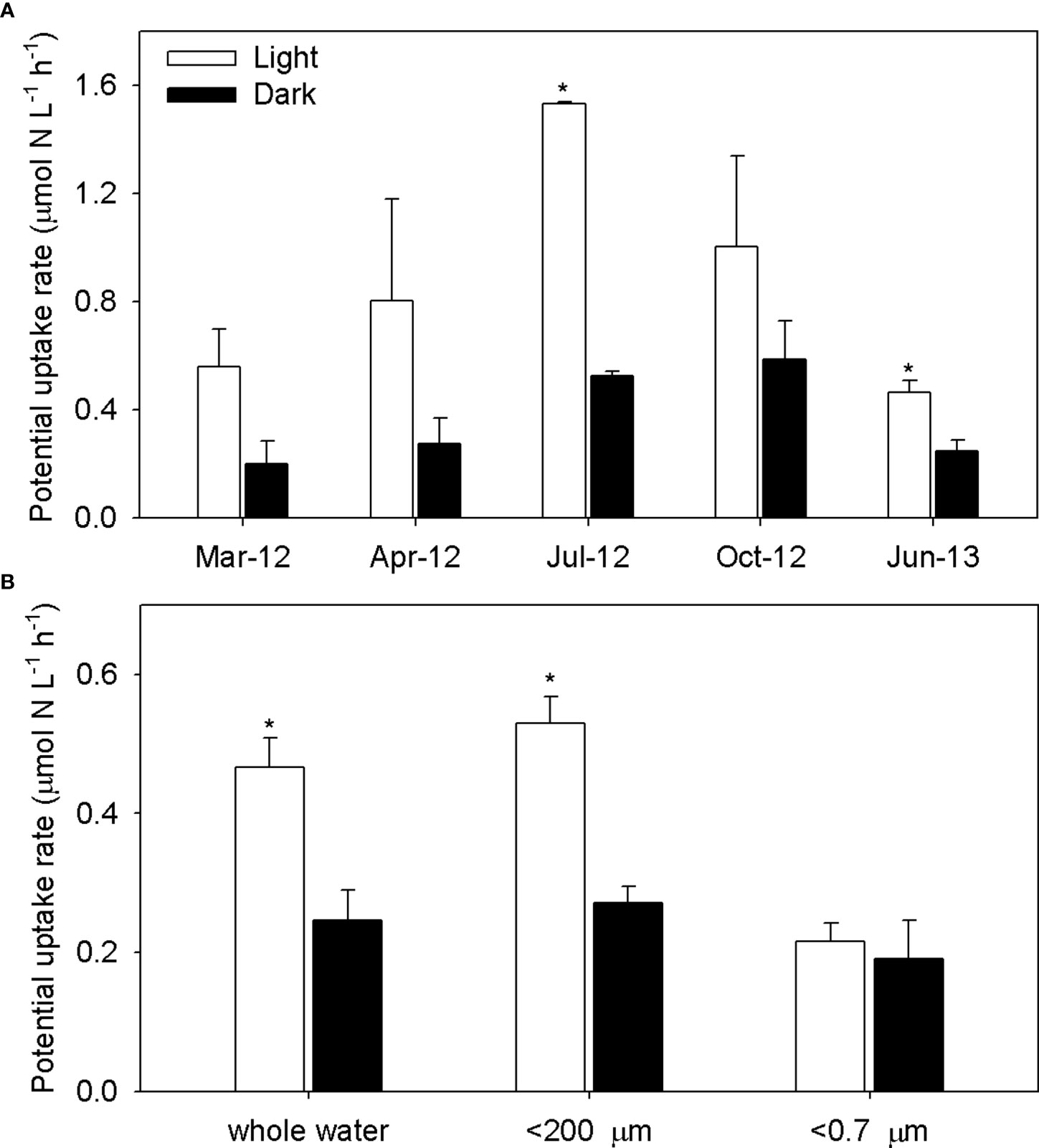
Figure 3 Ammonium potential uptake rates for whole-water samples (A) and size-fractioned (whole, <200 μm, and <0.7 μm) water samples (B) in light and dark treatments in the YRE. The * indicates a minimum value estimated from the initial ammonium concentration and regeneration rates in dark incubations.
Identification of seasonal trends of ammonium regeneration over the entire survey period was difficult due to the absence of light data for July 2012 and June 2013. A direct light effect may be insignificant for regeneration because ammonium is mainly regenerated by processes such as dissimilatory bacterial respiration and zooplankton excretion. The dark rate is therefore thought to explain ammonium regeneration by the plankton community in the surveyed area. Ammonium regeneration rates in the dark incubation were 0.1–1.2 µmol L−1 h−1, with the maximum rate recorded in July 2012 (Figure 4A). Dark regeneration was positively correlated with temperature (r2 = 0.54). Light regeneration rates tended to be higher than dark rates in spring and fall, although this difference was not statistically significant (Figure 4A). Size-fractionated dark regeneration rates (total plankton community,<200 µm, and<0.7 µm size classes) also exhibited no significant differences (Figure 4B).
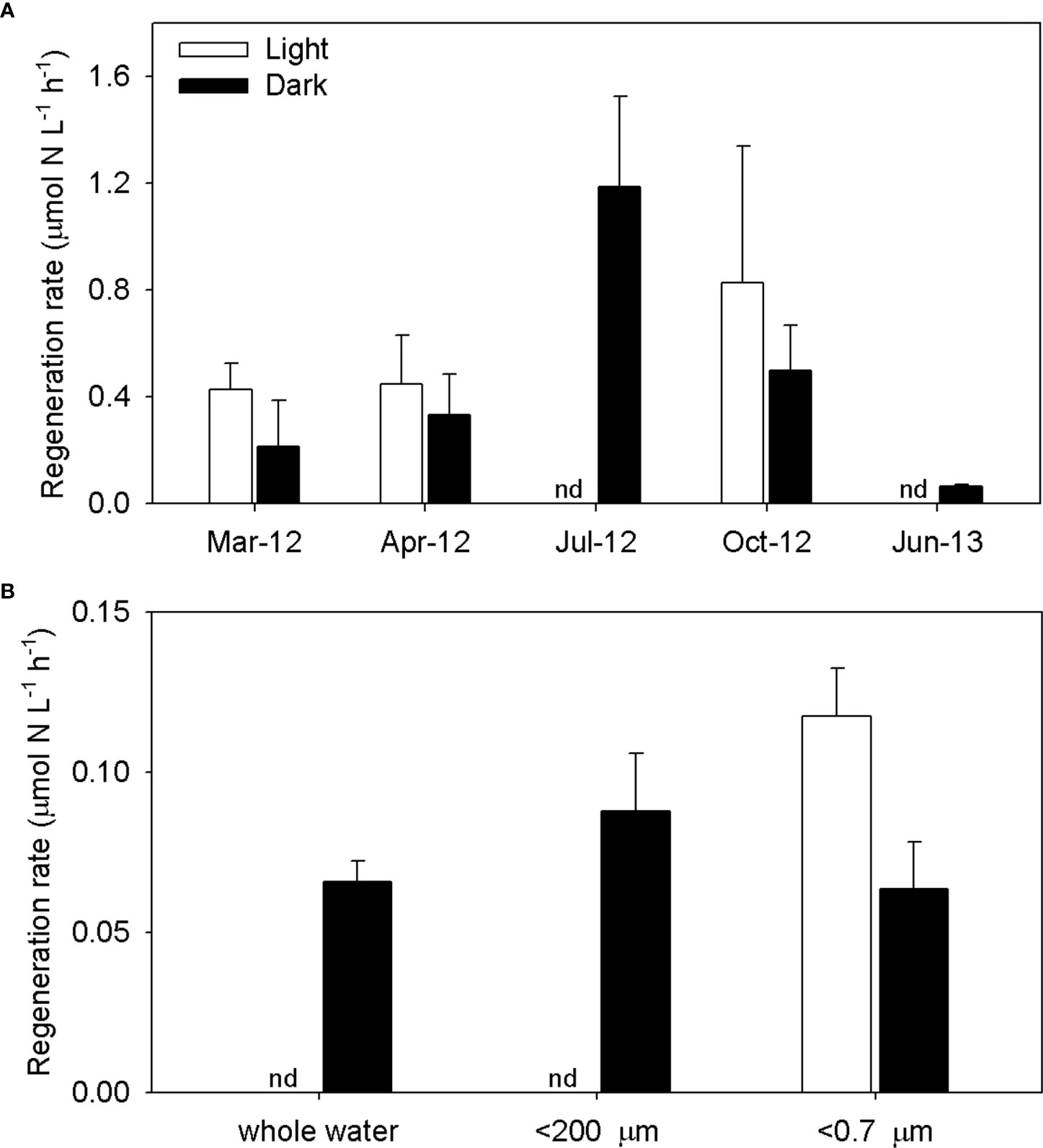
Figure 4 Ammonium regeneration rates for whole-water samples (A) and size-fractioned (whole, <200 μm, and <0.7 μm) water samples (B) in light and dark treatments in the YRE; nd, no data.
To assess changes in the turbidity of the surveyed area caused by dam construction, annual mean values of the suspended sediment concentration were compared for the period prior to construction (one year’s worth of data; 1976), during construction (1979–1981), and after construction of the dam until 2013 (MEIS, 1997–2013; NFRDA, 1983; Moon et al., 1991). The turbidity of the sampled area decreased after dam construction (Figure 5). Before construction, the annual average suspended sediment concentration of the Mokpo coast was 86.9 mg l−1; this decreased by 48% during construction, averaging 45.5 mg l−1 (NFRDA, 1983; Moon et al., 1991). After construction, the annual average turbidity range was greatly reduced to 9.9–32.3 mg l−1, one-quarter (63%–92% decrease) of pre-construction levels (MEIS, 1997–2013). From 1984 until the present, similar levels have persisted. The range of turbidity variation has also decreased compared to the pre-construction values (Figure 5).
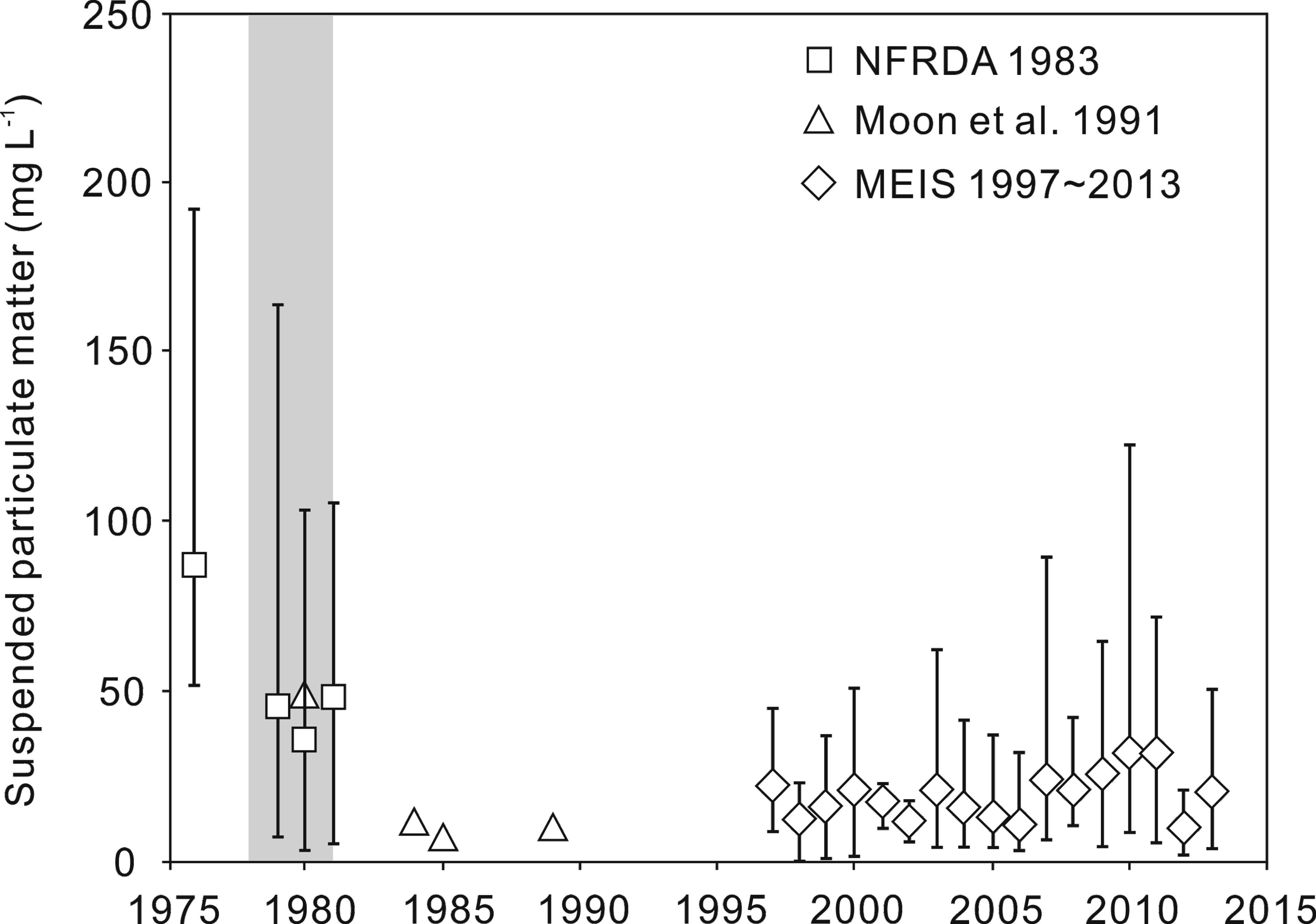
Figure 5 Annual mean values and ranges of suspended sediment concentrations for one year prior to (1976), during (1979–1981, shaded area), and after dam construction, until 2013 (MEIS 1997–2013; NFRDA, 1983; Moon et al., 1991).
Discussion
N:P stoichiometry in the YRE
The surface waters of the study area were rich in nitrate throughout the year, and ammonium was only exhausted in summer (Table 1). The relatively high levels of nitrate in the YRE surface waters appeared to be related to high N loading from upstream areas, which contain farmland, livestock, and large cities (Lee, 2015; Figure 2). The YRE DIN : DIP ratio was higher than the Redfield ratio throughout the year (Table 1). Studies of nutrient-uptake kinetics have suggested that a dissolved N:P ratio of higher than 20–30 indicates a potential P limitation on phytoplankton growth (Goldman et al., 1979; Justic et al., 1995). Although N is a very important limiting element for primary production in oceanic environments, this is not true for all coastal environments, for example, P limitation (N:P ratio of 200:1) has been reported for the coastal area of the Pearl River Estuary in the South China Sea and North Sea estuaries (Yin et al., 2001; Howarth and Marino, 2006). P limitations in the YRE appear to be the result of enhanced controls on P input from metropolitan areas, coupled with extremely high N input from the agricultural sector (Howarth and Marino, 2006).
However, there is a limit to determining the nutrient limitation only by the N:P ratio in the rapidly changing YRE environment. Which nutrients are limiting or co-limiting factors for phytoplankton growth can change dynamically throughout the year and among years (Fisher et al., 1992; Wu et al., 2017). In a previous study of nutrient limitation in the YRE, although P limitation was predominant estimated through the N:P ratio, bioassay experiments showed that phytoplankton growth was regulated by P inputs from winter to spring, while N played a more dominant controlling role in fall. No nutrient limitation was observed during summer in the YRE (Yoon, 2012; MOF, 2014). In this study, the results of winter with a relatively high N:P ratio and autumn with the lowest values showed similar tendencies to those of Yoon (2012).
The YRE is highly affected by eutrophic freshwater with high N:P ratio discharged from the Youngsan Lake (Figure 2). During the non-discharge period, the N:P ratio and limiting nutrients of phytoplankton can be changed by biogeochemical processes such as recycling of nutrients and sediment-water nutrient exchange within the estuary (Finlay et al., 2013; Wu et al., 2017; Zhou et al., 2022). In this study, active recycling of ammonium in summer can supply sufficient N demand for primary production and is expected to affect the N:P stoichiometry (see the discussion of ‘Implications of estuarine dam on ammonium recycling’ below). In order to more accurately determine the role of N and P on phytoplankton growth in YRE, further studies are needed in the future, including the biogeochemical processes of N and P.
Ammonium regeneration in the water column
Although results for natural-light ammonium regeneration by size‐fractionated plankton were not obtained, the dark regeneration rates from the size-fraction experiments can provide clues as to which plankton communities mediate ammonium regeneration, as heterotrophic organisms do not generally require light energy for such processes (Wheeler et al., 1989; Gardner et al., 1997). The similarity in dark ammonium regeneration rates between the whole-water and<0.7 μm fractions (Figure 4B) suggests that in early summer, most of the ammonium is regenerated by small bacteria, whereas zooplankton excretion is less important. Similar observations have been reported for the Mississippi River plume (Jochem et al., 2004) and the western English Channel (Maguer et al., 1999). In the Mississippi River plume, no differences were observed between ammonium regeneration rates in whole-water samples and the<2 µm size fraction at several sites along a salinity gradient, indicating that bacterial mineralization was important to the ammonium recycling process (Jochem et al., 2004). Maguer et al. (1999) reported that picoplankton contribute significantly to ammonium regeneration during summer in the shallow, well-mixed waters of the English Channel. The less significant role of zooplankton in ammonium regeneration may be the result of abrupt freshwater discharges, as sudden changes in salinity and concentration of suspended solids during discharge events may be accompanied by changes in plankton species composition and substantial decreases in zooplankton biomass (Seo, 2008; Sin et al., 2013). Sin et al. (2013) suggested that phytoplankton and zooplankton that accumulate in an estuary during a non-discharge period can be flushed from the estuary by intermittent freshwater discharge events because the freshwater residence time (0.2–2.03 days; Lee and Jun, 2009) is lower than the phytoplankton doubling time (0.56–5.8 days; Sin et al., 2013). Moreover, although the phytoplankton biomass recovers within several days, the zooplankton respond more slowly (MOF, 2014). These intermittent discharges into the YRE, which create an environment that is unsuitable for stable zooplankton populations, may be one reason why ammonium regeneration by zooplankton excretion is not important in this estuary.
Light effects on heterotrophic nutrient regeneration are less obvious than those on autotrophic nutrient uptake (phytoplankton; Wheeler et al., 1989; Gardner et al., 1997). However, previous studies have shown that ammonium regeneration can be enhanced in light incubations (Gardner et al., 1997; James et al., 2011). James et al. (2011) demonstrated that ammonium regeneration in a turbid lake in south Florida was higher in light conditions than in the dark. Differences between light and dark regeneration rates were also observed in the surface waters of the Mississippi River plume, indicating a close coupling between phytoplanktonic production of DON and microbial N recycling processes (Gardner et al., 1997). Slightly higher regeneration rates under natural light incubation were also observed in the present study during spring and fall and support the idea that labile organic nitrogen from phytoplankton was produced more during the day than at night (Figure 4A).
Ammonium uptake in the water column
The differences between light and dark uptake rates were used to estimate the role of phytoplankton in total ammonium uptake, assuming that the dark ammonium uptake reflects the bacterial contribution to this process. The ammonium uptake in the dark treatment can be considered as the uptake primarily contributed by heterotrophic bacteria, as N uptake by phytoplankton is closely associated with light energy, whereas uptake by heterotrophic bacteria is more correlated with the availability of organic matter (Gardner et al., 2004). Although dark N assimilation by phytoplankton has been reported, this process usually occurs under severe N limitation and is less likely in N-replete systems such as the YRE (Clark et al., 2002; Jochem et al., 2004; Maguer et al., 2011). We also observed that the dark uptake rate for the total plankton community and the light and dark uptake rates for plankton less than 0.7 µm in size were similar (Figure 3B); thus, at least for June 2013, we concluded that the dark uptake was due to small-sized heterotrophic bacteria.
As incubations were performed in surface waters for 24 h, uptake under light conditions reflects the total ammonium uptake for one full day. Therefore, the difference between light and dark (bacterial) uptake can be regarded as the photosynthetic ammonium uptake. The photosynthetic ammonium uptake was usually higher than the bacterial uptake, although this difference was not statistically significant, except during summer, when photosynthetic uptake was twice as high as bacterial uptake (Figure 3A).
The contribution of phytoplankton and bacteria to ammonium uptake derived from the light/dark uptake differences has been reported in previous studies (Jochem et al., 2004; Gardner et al., 2004; McCarthy et al., 2009; James et al., 2011). McCarthy et al. (2009) demonstrated that there were no differences between light and dark potential uptake rates in Florida Bay. From the results of 15N incubations and bacterial abundance, it has been suggested that a substantial fraction of potential ammonium uptake can be ascribed to heterotrophic bacteria. Jochem et al. (2004) have shown that potential ammonium uptake rates in light bottles were higher than those in dark bottles, and the dark uptake as a percentage of total uptake was approximately 40% to 80% in the Mississippi River plume. Gardner et al. (2004) found that the dark uptake rates (attributed mainly to bacteria) during spring in Lake Michigan were ~70% of the natural light uptake rates (bacteria plus phytoplankton) at most lake sites, but only ~30% at river-influenced sites. The results of the present study demonstrated that dark ammonium uptake rates were 34%–53% of natural light uptake rates and were within the range of ratios observed for other estuarine environments, indicating slightly dominant autotrophic consumption of ammonium during most investigation periods (Gardner et al., 2004; Jochem et al., 2004; McCarthy et al., 2009).
Importance of ammonium regeneration for primary production in the YRE
The ammonium regeneration rates in the YRE were higher than the values previously reported for the Delaware River (Lipschultz et al., 1986), Florida Bay (McCarthy et al., 2009), the West Florida Shelf (Bronk et al., 2014), Copano Bay (Bruesewitz et al., 2015), St. Lucie Estuary (Hampel et al., 2019), a wetland at Lake Erie (Lavrentyev et al., 2004), the Thau lagoon, France (Collos et al., 2003), and the Mississippi River plume (Cotner and Gardner, 1993; Gardner et al., 1997). Our results in the dark treatment were also 22 times higher than those in the Peruvian upwelling system (Fernandez et al., 2009). The maximum value in this study (dark regeneration rate of 1.19 μmole N L−1 h−1 in July 2012) was comparable to the rates obtained from a coastal mangrove swamp in India (Dham et al., 2002), Lake Okeechobee (Hampel et al., 2019), Yangtze River (Xue et al., 2021a), and lower than those of the subtropical eutrophic Lake Taihu in China (McCarthy et al., 2007; Hampel et al., 2018; Jiang et al., 2019; Xue et al., 2021b). YRE ammonium uptake rates were higher than those observed in other estuaries, including the nitrate-rich Thames Estuary (Middelburg and Nieuwenhuize, 2000b), turbid European tidal estuaries (the Ems, Douro, and Rhine; Middelburg and Nieuwenhuize, 2000a), the West Florida Shelf (Bronk et al., 2014), Copano Bay (Bruesewitz et al., 2015), and Cochin Estuary (Bhavya et al., 2016). However, ammonium uptake rates were similar to those observed for the Mississippi River plume (Gardner et al., 1997), a coastal mangrove swamp in India (Dham et al., 2002), St. Lucie Estuary (Hampel et al., 2019), Yangtze River (Xue et al., 2021a), a Lake Erie wetland (Lavrentyev et al., 2004; Hoffman et al., 2022), and the mesohaline region of the Loire and Scheldt estuary (Middelburg and Nieuwenhuize, 2000a). The ammonium uptake rates were also lower than the values of the Lake Okeechobee (Hampel et al., 2019) and Lake Taihu (Hampel et al., 2018; Jiang et al., 2019). In this study, ammonium regeneration and uptake rates were in a range similar to the values in eutrophic freshwater rather than coastal waters.The ammonium turnover time (ambient concentration divided by uptake rate) in light incubations ranged from 0.01 (July 2012) to 1.24 days (March 2012), even though ammonium was almost depleted in summer. The average residence time of freshwater reported for the YRE ranged from 0.26 (summer wet season) to 2.03 days (fall dry season; Lee and Jun, 2009). Therefore, the ammonium turnover time was shorter than the freshwater residence time, and we can conclude that ammonium recycling actively occurs within this estuary throughout the year. Similarly, it was suggested that the ammonium turnover times reported along a river–estuary continuum in a coastal catchment of the Texas Coastal Bend were lower than water replacement times, supporting high productivity (Bruesewitz et al., 2015). A high rate of ammonium turnover was also reported in Lake Taihu, indicating that may promote cyanobacterial bloom during N-limited season (Xue et al., 2021b).
Implications of estuarine dam on ammonium recycling
High concentrations of suspended solids in a tidally dominated estuary reduce the euphotic depth and limit primary production (Cloern, 1987; Mallin and Paerl, 1992). Moreover, if the estuarine water residence time is shorter than the cell doubling time of phytoplankton, phytoplankton biomass accumulation is low (Sin et al., 1999; Thomas et al., 2005; Sin et al., 2013). Estuaries and coastal waters located in the southeastern part of the Yellow Sea (including the YRE, Han River, and Geum River) are well-mixed and turbid due to sediment resuspension enhanced by strong macro-tidal currents, with abundant sediment input from land (Lee and Chu, 2001; Byun et al., 2007). Light limits primary production in these systems, but phytoplankton blooms can occur in summer when light conditions temporarily improve, even in turbid estuaries (Wafar et al., 1983; L’Helguen et al., 1996). In the southeastern Yellow Sea, however, because annual precipitation is concentrated during summer, which decreases water residence time (in turn reducing phytoplankton biomass accumulation), phytoplankton blooms are relatively rare, despite the high nutrient levels (Kim et al., 2013; Park et al., 2013). The building of estuarine dams causes physical and biogeochemical changes to the estuary environment by restricting seawater circulation (Cho et al., 2004; Byun et al., 2007; Gedan et al., 2009). Dam construction weakens tidal currents and generates stagnant areas (Cho et al., 2004; Cho et al., 2014). Mean turbidity in the YRE has decreased to 63%–92% of pre-dam levels (Figure 5), resulting in improved light conditions.
Vigorous ammonium recycling by bacteria and phytoplankton in the YRE may be a combined result of reduced turbidity and increased water residence times due to construction of the estuarine dam. When stratification intensifies during non-discharge periods, ammonium utilization can be enhanced because of favorable light conditions, increased water residence times. This phenomenon—combined with an ammonium turnover time that is shorter than the freshwater residence time, together with high nutrient inputs from inside the dam—can result in phytoplankton blooms (Thomas et al., 2005; Figure 2). In summer, the primary productivity in the YRE was higher (up to 8900 mg C m−2 d−1, Lee et al., 2011) than that in adjacent estuaries and other estuarine systems (Douro Estuary, Portugal and Westerschelde Estuary, the Netherlands), with similar ranges of suspended solids, nutrients, and flushing times (Kromkamp and Peene, 1995; Middelburg and Nieuwenhuize, 2000a; Azevedo et al., 2006; Middelburg and Herman, 2007). The ammonium demand for primary production, estimated from primary productivity measurements (2,000–8,927 mg C m−2 d−1 from spring to summer, Lee et al., 2011) and C/N ratios (Redfield ratio), was 302–1,347 mg N m−2 d−1 in the YRE. In the current study, the estimated ammonium uptake by phytoplankton (assuming a mean euphotic depth of 4 m; Sin et al., 2013) was 710–1350 mg N m−2 d−1 during the same period. The regeneration of ammonium through active ammonium recycling is likely to supports high phytoplankton production during the spring–summer season in the YRE. Previous studies have suggested that water column N recycling contributes significantly to phytoplankton N demand in aquatic environments (Bronk et al., 2014; Hampel et al., 2018; Jiang et al., 2019; Xue et al., 2021a; Xue et al., 2021b; Xu et al., 2021; Hoffman et al., 2022). In the West Florida Shelf, regenerated ammonium was sufficient to supply the ammonium demand, and supported 85% of the ammonium uptake even during blooms of Karenia brevis (Bronk et al., 2014). Ammonium regeneration in Lake Taihu supported about 60% of potential ammonium demand during summer Microcystis-dominated blooms suggesting the importance of regenerated N cycling for cyanobacterial blooms (Hampel et al., 2018). In studies conducted on Lake Taihu and Lake Erie, extrapolated whole-lake regeneration of ammonium can supply N up to 200% of the external load to the lake, suggesting that internal N cycling plays an important role in supporting high productivity (Hampel et al., 2018; Hoffman et al., 2022). Even though active ammonium recycling was observed within the YRE system, further research is needed on how the altered estuarine structure and function affect the N cycle and primary production such as YRE, including the relative importance of nitrate and ammonium as a source of inorganic N for phytoplankton and primary production.
Conclusions
This study investigated ammonium regeneration and potential uptake rates an anthropogenically altered YRE using stable-isotope techniques. To the best of our knowledge, this study is the first to examine water column nitrogen recycling in an estuary that has been significantly deformed by dam construction, and has improved our understanding of the impact of man-made structures on the nitrogen cycle. This study showed that high ammonium recycling provides a significant proportion of N to sustain phytoplankton production, suggesting that internal nutrient cycling are important in YRE. The higher contribution of bacteria than zooplankton to ammonium regeneration in this study is likely to be associated with artificial freshwater discharge. In addition, as the environment is changed by dam construction from a highly turbid estuary to semi enclosed bay, decrease in turbidity and increase in residence time were assumed to promote ammonium recycling.
Data availability statement
The raw data supporting the conclusions of this article will be made available by the authors, without undue reservation.
Author contributions
JL and SA developed the idea and wrote the manuscript. JL conducted the literature search and analysis. SA provided interpretation of data. Both authors contributed to the article and approved the submitted version.
Funding
This research was supported by Korea Institute of Marine Science & Technology Promotion(KIMST) funded by the Ministry of Oceans and Fisheries, Korea(20220023). This research was also supported by BK21 School of Earth and Environmental and Environmental systems. This research was also supported by the National Institute of Fisheries Science, Ministry of Oceans and Fisheries, Korea (R2021055).
Conflict of interest
The authors declare that the research was conducted in the absence of any commercial or financial relationships that could be construed as a potential conflict of interest.
Publisher’s note
All claims expressed in this article are solely those of the authors and do not necessarily represent those of their affiliated organizations, or those of the publisher, the editors and the reviewers. Any product that may be evaluated in this article, or claim that may be made by its manufacturer, is not guaranteed or endorsed by the publisher.
References
Alpine A. E., Cloern J. E. (1988). Phytoplankton growth rates in a light-limited environment, San Francisco bay. Mar. Ecol. Prog. Ser. 44, 167–173. doi: 10.3354/meps044167
An S., Gardner W. S. (2002). Dissimilatory nitrate reduction to ammonium (DNRA) as a nitrogen link, versus denitrification as a sink in a shallow estuary (Laguna Madre/Baffin bay, Texas). Mar. Ecol. Prog. Ser. 237, 41–50. doi: 10.3354/meps237041
An S., Lee J. Y., Gardner W. S. (2013). Stable isotope measurement of ammonium using HPLC-RTS (high performance liquid chromatography-retention time shift). J. Korean Soc Oceanogr. 18, 47–52. doi: 10.7850/jkso.2013.18.1.47
Armstrong R. A. (1994). Grazing limitation and nutrient limitation in marine ecosystems: steady state solutions of an ecosystem model with multiple food chains. Limnol. Oceanogr. 39, 597–608. doi: 10.4319/lo.1994.39.3.0597
Azevedo I. C., Duarte P. M., Bordalo A. A. (2006). Pelagic metabolism of the douro estuary (Portugal) - factors controlling primary production. Estuar. Coast. Shelf S. 69, 133–146. doi: 10.1016/j.ecss.2006.04.002
Bhavya P. S., Kumar S., Gupta G. V. M., Sudheesh V., Sudharma K. V., Varrier D. S., et al. (2016). Nitrogen uptake dynamics in a tropical eutrophic estuary (Cochin, India) and adjacent coastal waters. Estuaries Coast. 39 (1), 54–67. doi: 10.1007/s12237-015-9982-y
Bianchi T. S. (2007). Biogeochemistry of estuaries (New York: Oxford University Press on Demand) 688 pp.
Blackburn T. H. (1979). Method for measuring rates of turnover in anoxic marine sediments, using a 15N- dilution technique. Appl. Environ. Microbiol. 37, 760–765. doi: 10.1128/aem.37.4.760-765.1979
Bronk D. A., Killberg-Thoreson L., Sipler R. E., Mulholland M. R., Roberts Q. N., Bernhardt P. W., et al. (2014). Nitrogen uptake and regeneration (ammonium regeneration, nitrification and photoproduction) in waters of the West Florida shelf prone to blooms of karenia brevis. Harmful Algae 38, 50–62. doi: 10.1016/j.hal.2014.04.007
Bruesewitz D. A., Gardner W. S., Mooney R. F., Buskey E. J. (2015). Seasonal water column NH4+ cycling along a semi-arid sub-tropical river–estuary continuum: responses to episodic events and drought conditions. Ecosystems 18 (5), 792–812. doi: 10.1007/s10021-015-9863-z
Byun D. S., Wang X. H., Holloway P. E. (2004). Tidal characteristic adjustment due to dyke and seawall construction in the mokpo coastal zone, Korea. Estuar. Coast. Shelf S. 59, 185–196. doi: 10.1016/j.ecss.2003.08.007
Byun D. S., Wang X. H., Zavatarelli M., Cho Y. K. (2007). Effects of resuspended sediments and vertical mixing on phytoplankton spring bloom dynamics in a tidal estuarine embayment. J. Mar. Syst. 67, 102–118. doi: 10.1016/j.jmarsys.2006.10.003
Caperon J., Schell D., Hirota J., Laws E. (1979). Ammonium excretion rates in kaneohe bay, Hawaii, measured by a 15N isotope dilution technique. Mar. Biol. 54, 33–40. doi: 10.1007/BF00387049
Cho Y. K., Lee K. S., Park K. Y. (2009). Year-to-year variability of the vertical temperature structure in the youngsan estuary. Ocean Polar Res. 31, 239–246. doi: 10.4217/OPR.2009.31.3.239
Cho Y. K., Park L. H., Cho C., Lee I. T., Park K. Y., Oh C. W. (2004). Multi-layer structure in the youngsan estuary, Korea. Estuar. Coast. Shelf S. 61, 325–329. doi: 10.1016/j.ecss.2004.06.003
Cho C. W., Song Y. S., Kim C. K., Kim T. I., Han J. S., Woo S. B., et al. (2014). A modeling study on hypoxia formation in the bottom water of the youngsan river estuary, Korea. J. Coast. Res. 31, 920–929. doi: 10.2112/JCOASTRES-D-13-00099.1
Clark K. R., Flynn K., Owens N. (2002). The large capacity for dark nitrate assimilation in diatoms may overcome nitrate limitation of growth. New Phytol. 155, 101–108. doi: 10.1046/j.1469-8137.2002.00435.x
Cloern J. E. (1987). Turbidity as a control on phytoplankton biomass and productivity in estuaries. Continent. Shelf Res. 7 (11-12), 1367–1381. doi: 10.1016/0278-4343(87)90042-2
Collos Y., Vaquer A., Bibent B. (2003). Response of coastal phytoplankton to ammonium and nitrate pulses: seasonal variations of nitrogen uptake and regeneration. Aquat. Ecol. 37, 227–236. doi: 10.1023/A:1025881323812
Cotner J. B. Jr, Gardner W. S. (1993). Heterotrophic bacterial mediation of ammonium and dissolved free amino acid fluxes in the Mississippi river plume. Mar. Ecol. Prog. Ser. 93, 75–87. doi: 10.3354/meps093075
Dham V. V., Heredia A. M., Wafar S., Wafar M. (2002). Seasonal variations in uptake and in situ regeneration of nitrogen in mangrove waters. Limnol. Oceanogr. 47, 241–254. doi: 10.4319/lo.2002.47.1.0241
Finlay J. C., Small G. E., Sterner R. W. (2013). Human influences on nitrogen removal in lakes. Science 342 (6155), 247–250. doi: 10.1126/science.1242575
Fernández C., Farías L., Alcaman M. E. (2009). Primary production and nitrogen regeneration processes in surface waters of the Peruvian upwelling system. Prog. Oceanogr. 83, 159–168.
Fisher T. R., Peele E. R., Ammerman J. W., Harding L. W. Jr (1992). Nutrient limitation of phytoplankton in Chesapeake bay. Mar. Ecol. Prog. Ser. 82, 51–63. doi: 10.3354/meps082051
Gardner W. S., Bootsma H. A., Evans C., St John P. A. (1995). Improved chromatographic analysis of 15N:14N ratios in ammonium or nitrate for isotope addition experiments. Mar. Chem. 48, 271–382. doi: 10.1016/0304-4203(94)00060-Q
Gardner W. S., Cavaletto J. F., Cotner J. B., Johnson J. M. (1997). Effects of natural light on nitrogen cycling rates in the Mississippi river plume. Limnol. Oceanogr. 42, 273–281. doi: 10.4319/lo.1997.42.2.0273
Gardner W. S., Lavrentyev P. J., Cavaletto J. F., McCarthy M. J., Eadie B. J., Johengen T. H., et al. (2004). The distribution and dynamics of nitrogen and microbial plankton in southern lake Michigan during spring transition 1999–2000. J. Geophys. Res. 109, 1–16. doi: 10.1029/2002JC001588
Gedan K. B., Silliman B. R., Bertness M. D. (2009). Centuries of human-driven change in salt marsh ecosystems. Ann. Rev. Mar. Sci. 1, 117–141. doi: 10.1146/annurev.marine.010908.163930
Goldman J. C., McCarthy J. J., Peavey D. G. (1979). Growth rate influence on the chemical composition of phytoplankton in oceanic waters. Nature 279, 210–215. doi: 10.1038/279210a0
Hampel J. J., McCarthy M. J., Gardner W. S., Zhang L., Xu H., Zhu G., et al. (2018). Nitrification and ammonium dynamics in taihu lake, China: seasonal competition for ammonium between nitrifiers and cyanobacteria. Biogeosciences 15 (3), 733–748. doi: 10.5194/bg-15-733-2018
Hampel J. J., McCarthy M. J., Reed M. H., Newell S. E. (2019). Short term effects of hurricane Irma and cyanobacterial blooms on ammonium cycling along a freshwater–estuarine continuum in south Florida. Front. Mar. Sci. 6, 640. doi: 10.3389/fmars.2019.00640
Heip C. H. R., Goosen N. K., Herman P. M. J., Kromkamp J., Middelburg J. J., Soetaert K. (1995). Production and consumption of biological particles in temperate tidal estuaries. Oceanogr. Mar. Biol. Annu. Rev. 33, 1–149.
Hoffman D. K., McCarthy M. J., Boedecker A. R., Myers J. A., Newell S. E. (2022). The role of internal nitrogen loading in supporting non-n-fixing harmful cyanobacterial blooms in the water column of a large eutrophic lake. Limnol. Oceanogr. 67, 2028–2041. doi: 10.1002/lno.12185
Howarth R. W., Marino R. (2006). Nitrogen as the limiting nutrient for eutrophication in coastal marine ecosystems: Evolving views over three decades. Limnol. Oceanogr. 51, 364–376. doi: 10.4319/lo.2006.51.1_part_2.0364
Hyun J. H., Choi K. S., Lee K. S., Lee S. H., Kim Y. K., Kang C. K. (2020). Climate change and anthropogenic impact around the Korean coastal ecosystems: Korean long-term marine ecological research (K-LTMER). Estuar. Coast. 43, 441–448. doi: 10.1007/s12237-020-00711-6
James R. T., Gardner W. S., McCarthy M. J., Carini S. A. (2011). Nitrogen dynamics in lake Okeechobee, forms, functions and changes. Hydrobiologia 669, 199–212. doi: 10.1007/s10750-011-0683-7
Jiang X., Zhang L., Gao G., Yao X., Zhao Z., Shen Q. (2019). High rates of ammonium recycling in northwestern lake taihu and adjacent rivers: An important pathway of nutrient supply in a water column. Environ. pollut. 252, 1325–1334. doi: 10.1016/j.envpol.2019.06.026
Jochem F. J., McCarthy M. J., Gardner W. S. (2004). Microbial ammonium cycling in the Mississippi river plume during the drought spring of 2000. J. Plankton Res. 26, 1265–1275. doi: 10.1093/plankt/fbh118
Jones M. N. (1984). Nitrate reduction by shaking with cadmium: alternative to cadmium columns. Water Res. 18, 643–646. doi: 10.1016/0043-1354(84)90215-X
Justic N., Rabalais N. N., Turner R. E., Dortch Q. (1995). Changes in nutrient structure of river-dominated coastal waters: stoichiometric nutrient balance and its consequences. Estuar. Coast. Shelf S. 40, 339–356. doi: 10.1016/S0272-7714(05)80014-9
Kim T. W., Lee K. T., Lee C. K., Jeong H. D., Suh Y. S., Lim W. A., et al. (2013). Interannual nutrient dynamics in Korean coastal waters. Harmful Algae 30, S15–S27. doi: 10.1016/j.hal.2013.10.003
Kromkamp J., Peene J. (1995). Possibility of net primary production in the turbid schelde estuary (SW Netherlands). Mar. Ecol. Prog. Ser. 121, 249–259. doi: 10.3354/meps121249
Lavrentyev P. J., McCarthy M. J., Klarer D. M., Jochem F., Gardner W. S. (2004). Estuarine microbial food web patterns in a lake Erie coastal wetland. Microbial. Ecol. 48, 567–577. doi: 10.1007/s00248-004-0250-0
Lee J. (2015). The impact of man-made structures on estuarine ecosystems: Nitrogen cycling and halophyte functioning (Busan, Republic of Korea: Pusan National University), 191p.
Lee Y. G., An K. G., Ha P. T., Lee K. Y., Kang J. H., Cha S. M., et al. (2009). Decadal and seasonal scale changes of an artificial lake environment after blocking tidal flows in the yeongsan estuary region, Korea. Sci. Total Environ. 407, 6063–6072. doi: 10.1016/j.scitotenv.2009.08.031
Lee H. J., Chu Y. S. (2001). Origin of inner-shelf mud deposit in the southeastern yellow Sea: Huksan mud belt. J. Sediment Res. 71, 144–154. doi: 10.1306/040700710144
Lee K. S., Jun S. K. (2009). Material budgets in youngsan river estuary with simple box model. J. Korean Soc Mar. Environ. Engineer. 12, 44–53.
Lee Y. J., Sin Y. S., Kim S. H., Shin K. H. (2011). Temporal and spatial variations of primary productivity in youngsan river and mokpo coastal areas. Korean J. Limnol. 44, 327–336.
L’Helguen S., Madec C., Le Corre P. (1996). Nitrogen uptake in permanently well-mixed temperate coastal waters. Estuar. Coast. Shelf S. 42, 803–818. doi: 10.1006/ecss.1996.0051
Lim H. S., Diaz R. J., Hong J., Schaffner L. C. (2006). Hypoxia and benthic community recovery in Korean coastal waters. Mar. pollut. Bull. 52, 1517–1526. doi: 10.1016/j.marpolbul.2006.05.013
Lipschultz F., Wofsey S. C., Fox L. E. (1986). Nitrogen metabolism of the eutrophic Delaware river ecosystem. Limnol. Oceanogr. 31, 701–716. doi: 10.4319/lo.1986.31.4.0701
Maguer J. F., L'Helguen S., Caradec J., Klein C. (2011). Size-dependent uptake of nitrate and ammonium as a function of light in well-mixed temperate coastal waters. Cont. Shelf Res. 31, 1620–1631. doi: 10.1016/j.csr.2011.07.005
Maguer J. F., L’Helguen S., Madec C., Le Corre P. (1999). Seasonal patterns of ammonium regeneration from sizefractionated microheterotrophs. Cont. Shelf Res. 19, 1755–1770. doi: 10.1016/S0278-4343(99)00037-0
Mallin M. A., Paerl H. W. (1992). Effects of variable irradiance on phytoplankton productivity in shallow estuaries. Limnol. Oceanogr. 37, 54–62. doi: 10.4319/lo.1992.37.1.0054
McCarthy M. J., Gardner W. S., Lavrentyev P. J., Jochem F. J., Williams C. J. (2009). Water column nitrogen cycling and microbial plankton in Florida bay. Contrib. Mar. Sci. 38, 49–62.
McCarthy M. J., Gardner W. S., Lehmann M. F., Bird D. F. (2013). Implications of water column ammonium uptake and regeneration for the nitrogen budget in temperate, eutrophic missisquoi bay, lake Champlain (Canada/USA). Hydrobiologia 718, 173–188. doi: 10.1007/s10750-013-1614-6
McCarthy M. J., Lavrentyev P. J., Yang L., Zhang L., Chen Y., Qin B., et al. (2007). Nitrogen dynamics and microbial food web structure during a summer cyanobacterial bloom in a subtropical, shallow, well-mixed, eutrophic lake (Lake taihu, China). Hydrobiologia 581, 195–207. doi: 10.1007/s10750-006-0496-2
McCarthy J. J., Taylor W. R., Taft J. L. (1977). Nitrogenous nutrition of the plankton in the Chesapeake bay. 1. nutrient availability and phytoplankton preferences. Limnol. Oceanogr. 22, 996–1011. doi: 10.4319/lo.1977.22.6.0996
Middelburg J. J., Herman P. M. J. (2007). Organic matter processing in tidal estuaries. Mar. Chem. 106, 127–147. doi: 10.1016/j.marchem.2006.02.007
Middelburg J. J., Nieuwenhuize J. (2000a). Uptake of dissolved inorganic nitrogen in turbid, tidal estuaries. Mar. Ecol. Prog. Ser. 192, 79–88. doi: 10.3354/meps192079
Middelburg J. J., Nieuwenhuize J. (2000b). Nitrogen uptake by heterotrophic bacteria and phytoplankton in the nitrate-rich Thames estuary. Mar. Ecol. Prog. Ser. 203, 13–21. doi: 10.3354/meps203013
MOF (2014). Development of integrated estuarine management system (Sejong: Ministry of Oceans and Fisheries), 629 p.
Moon M. H., Cho J. W., Lee M. C. (1991). A variation on the marine environments in the yeongsan river estuary. Natur. Sci. Res. 14, 101–115.
NFRDA (1983). A comprehensive study on marine pollution for the conservation of the Korean coastal ecosystem with respect to culture areas and fishing grounds (Busan: National fisheries research and development agency), 624. Technical report 58.
Park J. Y., Jeong H. J., Yoo Y. D., Yoon E. Y. (2013). Mixotrophic dinoflagellate red tides in Korean waters: Distribution and ecophysiology. Harmful Algae 30, S28–S40. doi: 10.1016/j.hal.2013.10.004
Rabalais N. N., Diaz R. J., Levin L. A., Turner R. E., Gilbert D., Zhang J. (2010). Dynamics and distribution of natural and human-caused hypoxia. Biogeosciences 7, 585–619. doi: 10.5194/bg-7-585-2010
Seo M. H. (2008). Variation of zooplankton community by Open/Close of yangsan lake dike, Korea (Gwang Ju: Chonnam National University), 63p.
Sin Y. S., Hyun B. K., Jeong B. K., Soh H. Y. (2013). Impacts of eutrophic freshwater inputs on water quality and phytoplankton size structure in a temperate estuary altered by a sea dike. Mar. Environ. Res. 85, 54–63. doi: 10.1016/j.marenvres.2013.01.001
Sin Y., Jeong B. (2020). Anthropogenic disturbance of tidal variation in the water properties and phytoplankton community of an estuarine system. Estuar. Coast. 43, 547–559. doi: 10.1007/s12237-019-00557-7
Sin Y., Lee E., Lee Y., Shin K. H. (2015). The river–estuarine continuum of nutrients and phytoplankton communities in an estuary physically divided by a sea dike. Estuar. Coast. Shelf S 163, 279–289. doi: 10.1016/j.ecss.2014.12.028
Sin Y., Wetzel R. L., Anderson I. C. (1999). Spatial and temporal characteristics of nutrient and phytoplankton dynamics in the York river estuary, Virginia: analyses of long-term data. Estuaries 22, 260–275. doi: 10.2307/1352982
Son S. H., Campbell J., Dowell M., Yoo S., Noh J. (2005). Primary production in the yellow Sea determined by ocean color remote sensing. Mar. Ecol. Prog. Ser. 303, 91–103. doi: 10.3354/meps303091
Strickland J. D. H., Parsons T. R. (1972). A practical handbook of seawater analysis. Bull. Fish. Res. Board Canada 167, 1–310.
Thomas C. M., Perissinotto R., Kibirige I. (2005). Phytoplankton biomass and size structure in two south African eutrophic, temporarily open / closed estuaries. Estuar. Coast. Shelf S. 65, 223–238. doi: 10.1016/j.ecss.2005.05.015
Tilman D., Kilham S. S., Kilman P. (1982). Phytoplankton community ecology: the role of limiting nutrients. Annu. Rev. Ecol. Syst. 13, 349–372. doi: 10.1146/annurev.es.13.110182.002025
Wafar M. V. M., Le Corre P., Birrien J. L. (1983). Nutrients and primary production in permanently well-mixed temperate coastal waters. Estuar. Coast. Shelf S. 17, 431–446. doi: 10.1016/0272-7714(83)90128-2
Wei H., He Y., Li Q., Liu Z., Wang H. (2007). Summer hypoxia adjacent to the changjiang estuary. J. Mar. Syst. 67, 292–303. doi: 10.1016/j.jmarsys.2006.04.014
Wheeler P., Kirchman D., Landry M., Kokkinakis S. (1989). Diel periodicity in ammonium uptake and regeneration in the oceanic subarctic: implications for interactions in microbial food webs. Limnol. Oceanogr. 34, 1025–1033. doi: 10.4319/lo.1989.34.6.1025
Wu Z., Liu Y., Liang Z., Wu S., Guo H. (2017). Internal cycling, not external loading, decides the nutrient limitation in eutrophic lake: A dynamic model with temporal Bayesian hierarchical inference. Water Res. 116, 231–240. doi: 10.1016/j.watres.2017.03.039
Xue J., Yao X., Zhao Z., He C., Shi Q., Zhang L. (2021b). Internal loop sustains cyanobacterial blooms in eutrophic lakes: Evidence from organic nitrogen and ammonium regeneration. Water Res. 206, 117724. doi: 10.1016/j.watres.2021.117724
Xue J., Zhao Z., Yao X., Liu W., Zhang L. (2021a). Suspended solids induce increasing microbial ammonium recycling along the river-estuary continuum of the Yangtze river. Hydrol. Process. 35 (8), e14345. doi: 10.1002/hyp.14345
Xu H., McCarthy M. J., Paerl H. W., Brookes J. D., Zhu G., Hall N. S., et al. (2021). Contributions of external nutrient loading and internal cycling to cyanobacterial bloom dynamics in lake taihu, China: Implications for nutrient management. Limnol. Oceanogr. 66 (4), 1492–1509. doi: 10.1002/lno.11700
Yim J., Kwon B.-O., Nam J., Hwang J. H., Choi K., Khim J. S. (2018). Analysis of forty years long changes in coastal land use and land cover of the yellow Sea: The gains or losses in ecosystem services. Environ. pollut. 241, 74–84. doi: 10.1016/j.envpol.2018.05.058
Yin K., Qian D. P. Y., Wu M. C. S., Chen J. C., Huang L. M., Song X. Y., et al. (2001). Shift from p to n limitation of phytoplankton growth across the pearl river estuarine plume during summer. Mar. Ecol. Prog. Ser. 221, 17–28. doi: 10.3354/meps221017
Yoon B. B. (2012). A study of nutrient limitation in estuary using bioassay experiment mokpo, republic of Korea (Mokpo: Mokpo Maritime University), 51p.
Yuan X., Glibert P. M., Xu J., Liu H., Chen M., Liu H., et al. (2012). Inorganic and organic nitrogen uptake by phytoplankton and bacteria in Hong Kong waters. Estuar. Coast. 35, 325–334. doi: 10.1007/s12237-011-9433-3
Zhang J., Liu S. M., Ren J. L., Wu Y., Zhang G. L. (2007). Nutrient gradients from the eutrophic changjiang (Yangtze river) estuary to the oligotrophic kuroshio waters and re-evaluation of budgets for the East China Sea shelf. Prog. Oceanogr. 74, 449–478. doi: 10.1016/j.pocean.2007.04.019
Keywords: Yeongsan River estuary, estuarine dam, nitrogen cycling, ammonium regeneration, isotope dilution experiments, primary productivity
Citation: Lee J and An S (2022) High ammonium recycling in an anthropogenically altered Yeongsan River Estuary, South Korea. Front. Mar. Sci. 9:1017434. doi: 10.3389/fmars.2022.1017434
Received: 12 August 2022; Accepted: 17 November 2022;
Published: 08 December 2022.
Edited by:
Daidu Fan, Tongji University, ChinaReviewed by:
Silvia Newell, Wright State University, United StatesLijun Hou, East China Normal University, China
Copyright © 2022 Lee and An. This is an open-access article distributed under the terms of the Creative Commons Attribution License (CC BY). The use, distribution or reproduction in other forums is permitted, provided the original author(s) and the copyright owner(s) are credited and that the original publication in this journal is cited, in accordance with accepted academic practice. No use, distribution or reproduction is permitted which does not comply with these terms.
*Correspondence: Soonmo An, c21hbkBwdXNhbi5hYy5rcg==