- 1School of Fishery, Zhejiang Ocean University, Zhoushan, China
- 2Zhejiang Province Key Laboratory of Mariculture and Enhancement, Zhejiang Marine Fisheries Research Institute, Zhoushan, China
To explore the influence of different densities of Sinonovacula constricta on the composition of easily degradable organic matter and related functional bacteria, four experimental ecosystems were established: three polyculture systems (PMB1, PMB2, and PMB3) of Portunus trituberculatus and Marsupenaeus japonicus with different stocking densities of S. constricta (11.6, 23.1, and 34.7×104 ind./hm2, respectively) and a polyculture system with only P. trituberculatus and M. japonicus (PM). Among the easily degradable organic components in all aquaculture systems, protein content was the highest (0.74%~0.86%), followed by carbohydrates (0.16%~0.21%) and lipids (0.06%~0.13%). In the high-density (34.7×104 ind./hm2) S. constricta mixed culture system, the contents of carbohydrates, lipids, and proteins in the sediment were significantly lower than those of the other polyculture systems. The number of cellulose-decomposing bacteria in PMB3 was 3.79×106 cfu/g, which was significantly higher than that in the other systems. The number of starch-degrading bacteria and glutin-degrading bacteria was the lowest in PMB3, 1.26×104 cfu/g, and 160.00 cfu/g, respectively. The number of lipid-degrading bacteria in PMB3 was 0.77×104 cfu/g, which was significantly lower than that in the other systems. The easily degradable organics content in sediment was significantly positively correlated with the corresponding functional bacteria. The results showed that mixed culture of S. constricta could reduce the content of easily degradable organics in the sediment of mariculture ponds and change the number of functional bacteria in the sediment and the availability of degradable organic sediments may determine the abundance of corresponding degradable bacteria.
Introduction
In aquatic ecosystems, sediments are not only the repository of nutrients and organic particles in water but also the nutrient source of the overlying water and are an important part of the aquatic environment (Søndergaard et al., 1999). Simple organic compounds such as carbohydrates, starches, and proteins in sediments are quickly decomposed by functional bacteria, but lignin and resin are resistant to microbial decomposition and are not easily oxidized (Zaki et al., 2015; Wang et al., 2019). Easily degradable organics are decomposed into small molecular organic matter or inorganic matter through a series of microbial catabolic activities, which participate in the biogeochemical cycle of the polyculture ecosystem, thereby affecting the entire aquaculture environment (Behera et al., 2022). Studies have shown that the degradable components in surface sediments can affect the dynamics and metabolism of bottom fauna (Grant and Hargrave, 1987; Dell'Anno et al., 2000), and the content of degradable parts in organic matter can also be used to evaluate the nutritional status of ecosystems (Dell'Anno et al., 2002; Pusceddu et al., 2003).
Sediment is the habitat of many farmed animals and is very important to the success of farming (Boyd, 1995). During the farming period, residual baits and feces are continuously accumulated in the sediment, which increases the organic matter content of the sediment (Liu et al., 2022). It was reported that approximately 40.44% of sedimentary organic matter in shellfish aquaculture ecosystems can be assimilated by benthic bait animals, but 59.56% of sedimentary organic matter still needs microbial transformation (Ji et al., 1998). The degradation of easily degradable organics in sediments involves various types of functional bacteria, such as proteolytic bacteria, cellulose-decomposing bacteria, and starch-decomposing bacteria (Prijambada et al., 2009; Artha et al., 2019). These functional bacteria can produce proteases, dehydrogenases, lipases, amylases, oxidases, decarboxylases, and other extracellular enzymes acting on organic matter. Through anaerobic or aerobic processes, a series of biochemical reactions occur to degrade organic matter gradually and ultimately convert it into inorganic elements that can be absorbed and utilized by phytoplankton and aquaculture animals (Pisarek and Grata, 2013; Gawas et al., 2019). For example, the amylase secreted by starch-degrading bacteria can decompose the insoluble starch in the sediment into soluble monosaccharides, which are further decomposed into CO2 and participate in the carbon cycle at the water-air interface (Prijambada et al., 2009; Artha et al., 2019). Different types of functional bacteria secrete different extracellular enzymes to promote the degradation of easily degradable organic matter in sediment, which plays an important role in the circulation of biogenic elements in the aquaculture system.
Benthic shellfish change the physical properties of the sediment, such as its spatial heterogeneity, surface composition, particle size distribution, and porosity, by digging holes and via ventilation, excretion, and other biological disturbances (Thayer, 1979) and then affect the easily degradable organics in sediment and its related decomposing bacteria. In addition, benthic shellfish can also transfer particulate organic matter (POM) from water into the sediment in the form of feces or pseudofeces through the filtering effect (Newell, 2004). Sinonovacula constricta is a common zoobenthos species in coastal areas of China. It has a filter-feeding habit and is one of the important cultured shellfish in coastal areas of China (Chang, 2007). Studies have shown that the water transparency was greatly improved during the rapid growth of razor clam from June to October (Ren and Li, 2016), and the sediment organic matter content in the polyculture pond was significantly lower than that in the monoculture pond (He and Li, 2013). This is because S. constricta absorbs suspended organic substances from the upper water surface through its water pipe, improves water transparency, and reduces organic matter content in sediments (Tsuchiya and Kurihara, 1979; Lukwambe et al., 2018). When its distribution density is high, the ability of the population to remove organic matter in sediments is significantly strengthened (Tsuchiya and Kurihara, 1979; Lukwambe et al., 2018). However, there is a lack of research on the effect of filter-feeding shellfish in polyculture ecosystems on easily degradable organics, which thus needs further study. Based on the above understanding, four experimental ecosystems were established in this study: S. constricta was mixed into a Portunus trituberculatus–Marsupenaeus japonicus polyculture ecosystem at different densities to clarify the effects on the distribution and proportion of easily degradable organics in sediment and the changes in the number of easily degradable organics-degrading bacteria in different marine aquaculture ponds.
Materials and methods
Sampling ponds
The culture ponds were located on Changbai Island, Zhoushan city, Zhejiang Province (30°10′ N, 122°02′ E), and the size of the ponds was 1.33×104 m2. Three ponds were stocked with P. trituberculatus, M. japonicus, and S. constricta, which were termed PMB1, PMB2, and PMB3 according to the stocking density of S. constricta from low to high. The fourth pond was stocked with P. trituberculatus and M. japonicus only and was termed PM. The stocking time of S. constricta was early April, the stocking time of P. trituberculatus was early June, and the stocking time of M. japonicus was early July. The stocking densities of P. trituberculatus and M. japonicus in all ponds were 31.2×104 and 22.5×104 ind./hm2, respectively. The stocking densities of S. constricta in PMB1, PMB2, and PMB3 were 11.6×104, 23.1×104, and 34.7×104 ind./hm2. The stocking densities of P. trituberculatus and M. japonicus were according to practical experience as well as the low stocking density of S. constricta in PMB1, and the middle and high density of S. constricta were set based on the above practical stocking density. The initial average individual weights of P. trituberculatus, M. japonicus, and S. constricta were 0.0240, 0.0087, and 0.3240 g, respectively. During the farming period, frozen trash fish were fed as the main feed once a day in the morning and evening. The protein, lipid, and carbohydrate contents of frozen trash fish were 15.4%, 3.3%, and 0.3%, respectively. Water exchange was carried out through the inlet and outlet of the pond, and the water exchange capacity of the different breeding ponds was consistent.
Sample collection and processing
The sediment samples from each pond were collected from April to December 2020. The initial sampling time was early April, and the sediment samples at the end of each month were collected in the middle ten days. Three sampling points were selected along the diagonal line in each pond, and four sediment samples were collected at each sampling point using a sediment column sampler (diameter: 10 cm), mixed evenly and placed in an incubator. According to Chai et al. (2022), the sediment temperature ranged from 7.47 to 30.13°C during the farming season in all the aquaculture systems, with an average of (21.36 ± 6.76)°C. The mean sediment pH values of PM, PMB1, PMB2, and PMB3 were 7.55 ± 0.10, 7.58 ± 0.10, 7.56 ± 0.11, and 7.65 ± 0.07, respectively. The mean sediment redox potential (ORP) of PM, PMB1, PMB2, and PMB3 were (-153.85 ± 26.87) mV, (-147.15 ± 28.56) mV, (-141.11 ± 23.00) mV and (-141.19 ± 18.90) mV, respectively.
Determination of the organic matter content
After the mud samples were mixed evenly, 40 g of each sample was freeze-dried with a freeze-drying machine. The mud samples were ground into powder by a bowl mill and passed through a 100-mesh standard test sieve. The filtered fine powder was collected and ignited at 450°C for 5 hours. The weight difference before and after ignition was calculated. The ratio of lost mass to dry sample mass was the organic matter content (OM, mg·g-1).
Determination of easily degradable organics
In the analysis of easily degraded organic matter in the sediment, the phenol−sulfuric acid method was used for the determination of carbohydrates (Masuko et al., 2005). According to the Editorial Board of the Water and Wastewater Monitoring and Analysis Methods of the State Environmental Protection Administration (2002), the Lowry method was used for the determination of proteins, and the methanol-chloroform method was used for the determination of lipids (Bligh and Dyer, 1959).
Statistics of functional bacteria
The bacterial counts of different groups in sediment samples were performed by the maximum possible count (MPN) and plate count (CFU) methods (Xu and Zheng, 1986). The MPN method was used for starch-degrading bacteria and glutin-degrading bacteria, and the CFU method was used for cellulose-degrading bacteria and lipid-degrading bacteria. In the MPN counting method, the mud samples were serially diluted 10 times to the final dilution, and when bacterial growth was not observed after inoculation, this was considered the critical stage. Then, 1 mL of each of the last five diluents in the series was placed into the corresponding liquid medium, and three parallel dilutions were performed; this MPN counting method is also called the three-tube method. The plate counting method (CFU) also used sample serial dilution, with three parallel dilutions performed, and 0.1 mL diluents were added to each plate for uniform coating. The culture temperature of all biological samples was 30°C, and the culture time was the same as the previous corresponding index.
Statistical analysis
In the statistical analysis, the mean values of four parallel samples were taken as the measured values of each sampling point. SPSS 22.0 and SigmaPlot 14.0 were used for data analysis. Repeated-measures analysis of variance was used to analyze the differences in the number of microorganisms in the sediment of the different breeding systems and the differences in the number of easily biodegradable organic substances in the sediment of the different breeding systems.
Results
Changes in easily degradable organics in the sediment
During the farming period, the carbohydrate content of the sediment in the different breeding systems generally showed a trend of first increasing and then decreasing (Figure 1A). The carbohydrate content ranges in the sediments of PM, PMB1, PMB2 and PMB3 were 0.04~0.40%, 0.05~0.35%, 0.04~0.43% and 0.04~0.33%, respectively, and the average values were (0.20 ± 0.12) %, (0.21 ± 0.09) %, (0.21 ± 0.12) %, and (0.16 ± 0.11) %, respectively. The results of the difference analysis showed that the carbohydrate content in the sediment of the PMB3 system was the lowest, which was significantly lower than that of the other polyculture ecosystems (P < 0.05), and there was no significant difference among PM, PMB1, and PMB2 (P > 0.05).
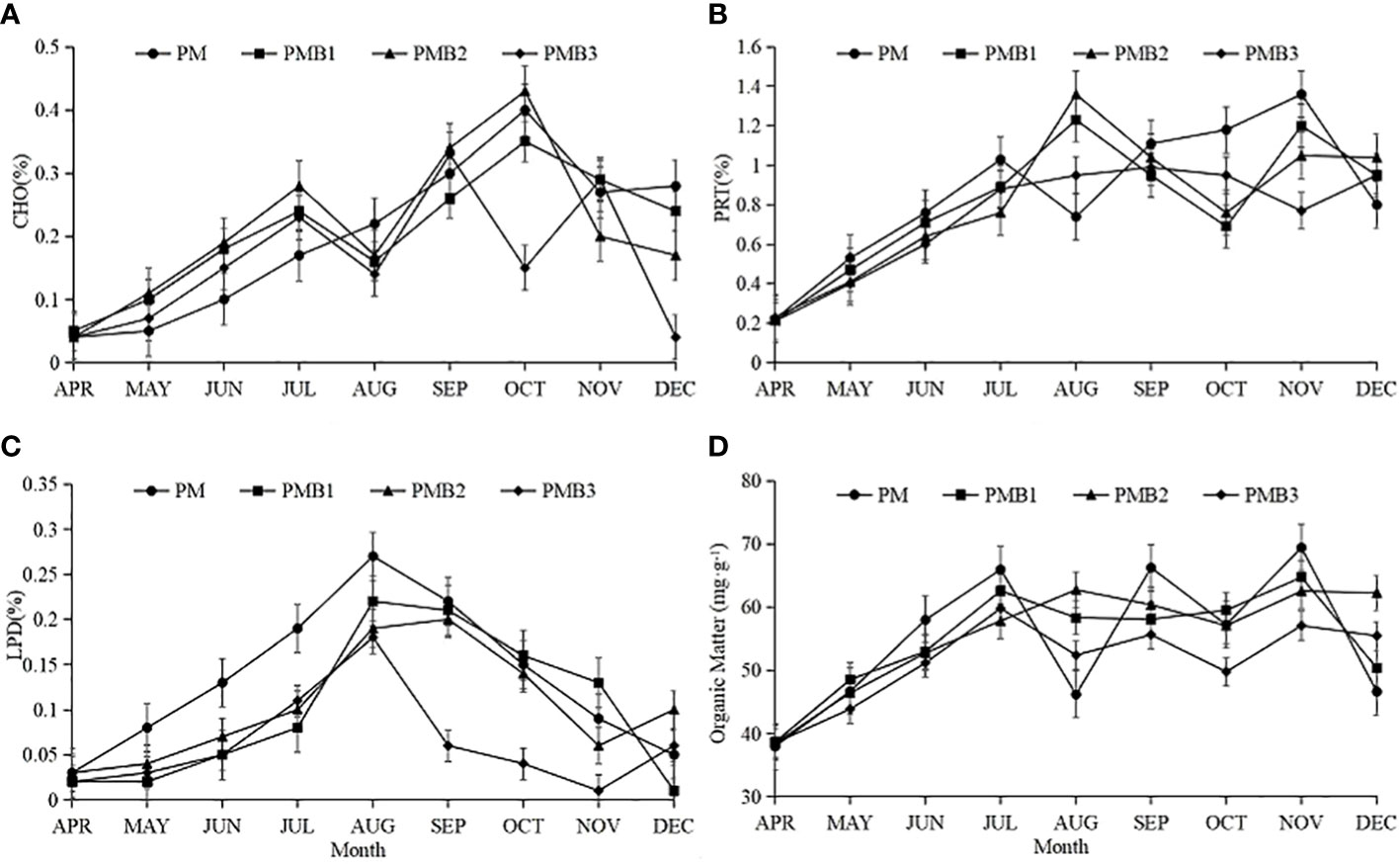
Figure 1 Changes in sediment easily degradable organics during the farming period. The data are shown as the means±SDs. (A), change of CHO; (B), change of PRT; (C), change of LPD; (D), change of Orgnic matters. CHO, carbohydrate in the sediment; PRT, protein in the sediment; LPD, lipid in the sediment.
As shown in Figure 1B, during the farming period, the protein content ranges in the sediments of PM, PMB1, PMB2 and PMB3 were 0.22~1.36%, 0.21~1.23%, 0.23~1.36% and 0.21~0.99%, respectively, and the average values were (0.86 ± 0.35) %, (0.81 ± 0.33) %, (0.81 ± 0.35) % and (0.74 ± 0.28) %, respectively. The results of the difference analysis showed that the sediment protein content of the PMB3 system was the lowest, which was significantly lower than that of the other polyculture ecosystems (P < 0.05). The protein content in the sediment of the PM system was the highest, followed by PMB1 and PMB2, and there was no significant difference between PMB1 and PMB2 (P > 0.05).
During the farming period, the trend of lipid content in the four breeding systems increased first and then decreased, with the highest content found in August (Figure 1C). The lipid content ranges in the sediments of PM, PMB1, PMB2, and PMB3 were 0.03~0.27%, 0.01~0.22%, 0.03~0.19%, and 0.01~0.18%, respectively, and the average values were (0.13 ± 0.08) %, (0.10 ± 0.08) %, (0.10 ± 0.06) %, and (0.06 ± 0.05) %, respectively. The results of the difference analysis showed that the lipid content in the sediment of PMB3 was the lowest, which was significantly lower than that of the other polyculture ecosystems (P < 0.05). The lipid content of the sediment in the PM system was the highest, followed by PMB1 and PMB2, and there was no significant difference between PMB1 and PMB2 (P > 0.05).
As shown in Figure 1D, during the farming period, the organic matter contents in PM, PMB1, PMB2, and PMB3 sediments ranged from 37.91 to 69.41 mg·g-1, from 38.62 to 64.72 mg·g-1, from 38.45 to 62.72 mg·g-1 and from 38.47 to 59.78 mg·g-1, with average values of (54.91 ± 11.05) mg·g-1, (54.86 ± 8.12) mg·g-1, (55.59 ± 8.36) mg·g-1 and (51.51 ± 6.74) mg·g-1, respectively. The difference analysis showed that the organic matter content of the PMB3 sediment was significantly lower than that of the other treatment groups (P < 0.05) and that there was no significant difference among PM, PMB1, and PMB2 (P > 0.05).
Changes in the number of functional bacteria in the sediment
The number of starch-degrading bacteria in the PM and PMB1 sediments increased first and then decreased but remained low (Figure 2A). The number of starch-degrading bacteria in PMB2 and PMB3 decreased continuously from the beginning of the culture (Figure 2A). The number of starch-degrading bacteria in the PM, PMB1, PMB2, and PMB3 sediments ranged from 4.5×102 to 2.5×104 cfu/g, 4.5×102 to 2.5×104 cfu/g, 1.5×104 to 9.8×104 cfu/g and 9×102 to 4.5×104 cfu/g, respectively. The average values were (2.47 ± 1.03) ×104 cfu/g, (1.82 ± 0.86) ×104 cfu/g, (1.87 ± 0.97) ×104 cfu/g and (1.26 ± 0.90) ×104 cfu/g, respectively. The number of starch-degrading bacteria in the PMB3 sediment was the lowest, which was significantly lower than that in the other treatment groups (P < 0.05). Systems PM and PMB1 were significantly higher than PMB3 (P < 0.05), and there was no significant difference between PMB1 and PMB2 (P > 0.05), PM was the highest (P < 0.05).
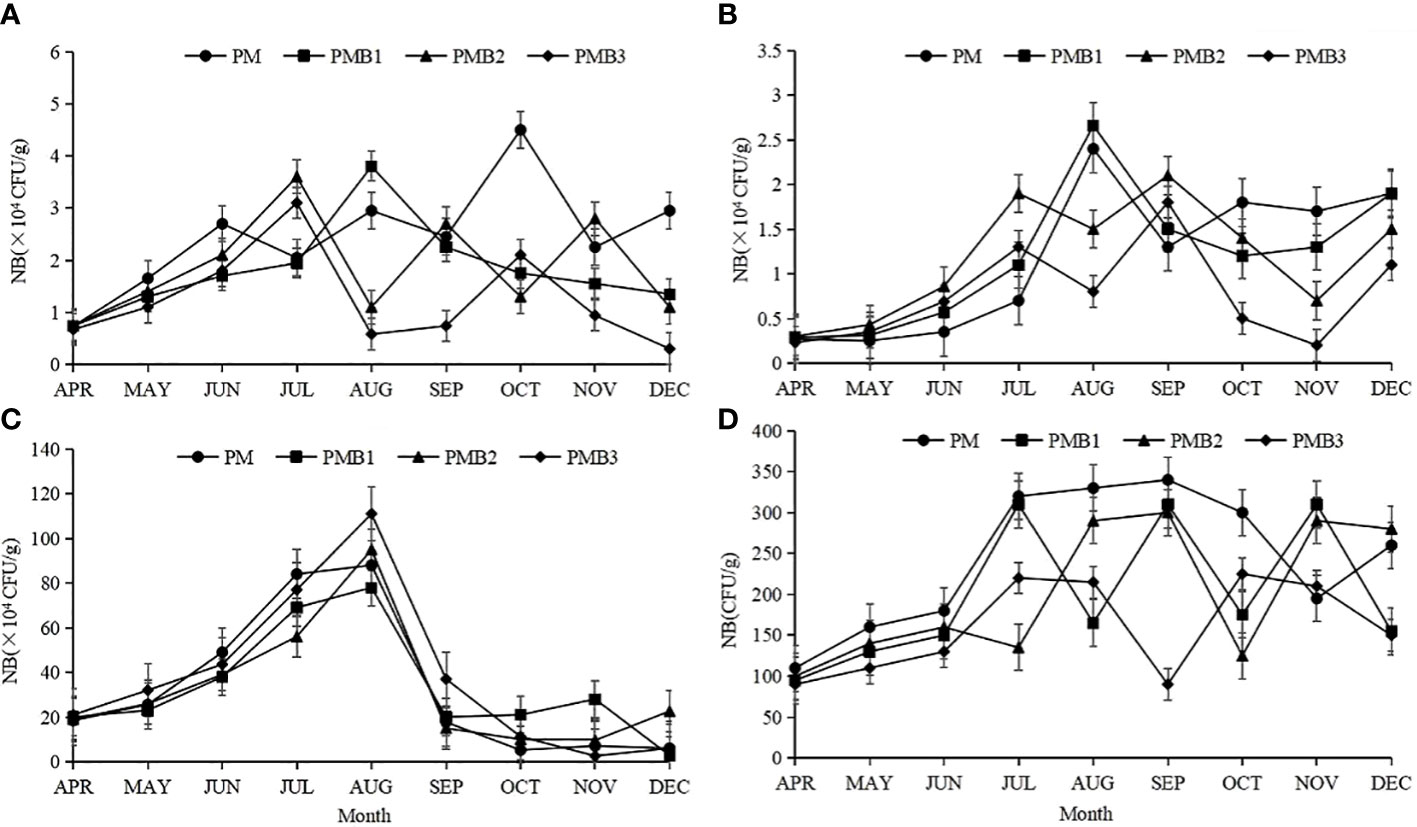
Figure 2 Changes in the number of functional bacteria in sediment during the farming period. The data are shown as the means ± SDs. (A), the number of starch-degrading bacteria; (B), the number of lipid-degrading bacteria; (C), the number of cellulose-decomposing bacteria; (D), the number of glutin-degrading bacteria.
The number of lipid-degrading bacteria in the PM and PMB1 sediments showed a trend of first increasing and then decreasing (Figure 2B). The number of bacteria in the PMB2 and PMB3 polyculture ecosystems showed the same trend, showing a trend of first increasing and then decreasing and then increasing and then decreasing (Figure 2B). The number of lipid-degrading bacteria in PM, PMB1, PMB2, and PMB3 ranged from 2.5×103 to 2.4×104 cfu/g, 2.9×103 to 2.7×104 cfu/g, 3.0×103 to 2.1×104 cfu/g and 2×103 to 1.8×104 cfu/g, with average values of (1.19 ± 0.81) ×104 cfu/g, (1.20 ± 0.77) ×104 cfu/g, (1.19 ± 0.64) ×104 cfu/g and (0.77 ± 0.54) ×104 cfu/g, respectively. The number of lipid-degrading bacteria in the PMB3 sediment was the lowest, which was significantly lower than that in the other systems (P < 0.05). There was no significant difference among the other systems (P > 0.05).
The number of cellulose-decomposing bacteria in the sediment of the four polyculture ecosystems first increased and then decreased, reaching a maximum in August, but the number in PMB2 and PMB3 increased sharply in August, and the growth rate was faster than that of PM and PMB1 (Figure 2C). The number of cellulose-degrading bacteria in the PM, PMB1, PMB2, and PMB3 sediments varied from 5.2×104 to 8.8×105 cfu/g, 2.8 ×104 g to 8.3×105 cfu/g, 9.57×104 to 9.5×105 cfu/g, and 2.6×104 to 1.11×106 cfu/g, with averages of (3.34 ± 3.27) ×105 cfu/g, (3.33 ± 2.47) ×105 cfu/g, (3.25 ± 2.78) ×106 cfu/g and (3.79 ± 3.57) ×106 cfu/g, respectively. In PMB3 and PMB1, the number of cellulose-degrading bacteria in the sediment was the highest, which was significantly higher than that in the other treatment groups (P < 0.05). The numbers in the PM and PMB2 systems were the lowest, and there was no significant difference between the two groups (P > 0.05).
The number of glutin-degrading bacteria in the PM sediment increased first and then showed a relatively stable trend, while the number in the other systems increased first and then showed a fluctuating trend and remained low (Figure 2D). The number of gelatinolytic bacteria in the PM, PMB2, PMB1, and PMB3 sediments varied from 110 to 340 cfu/g, 95 to 310 cfu/g, 100 to 300 cfu/g and 90 to 225 cfu/g, with average values of (243.89 ± 84.62) cfu/g, (200.00 ± 85.59) cfu/g, (202.22 ± 84.86) cfu/g, and (160.00 ± 57.72) cfu/g, respectively. The number of glutin-degrading bacteria in the PMB3 sediment was the lowest (P < 0.05) and that in PM was the highest (P < 0.05). The numbers in PMB1 and PMB2 were significantly higher than that in PMB3, and there was no significant difference between the former two groups (P > 0.05).
Correlation between the number of different groups of bacteria and easily degradable organics in sediment
Correlation analysis showed that the carbohydrate content in sediment was positively correlated with the number of starch-degrading bacteria (P < 0.05) and negatively correlated with the number of cellulose-decomposing bacteria (P < 0.05) (Table 1). The protein content was positively correlated with the number of gelatin-decomposing bacteria (P < 0.01). The lipid content was negatively correlated with the number of lipid-decomposing bacteria (P < 0.05). There was no significant correlation between the number of functional bacteria and the content of easily degradable organics in the sediment.
Discussion
The results showed that protein was the main easily degradable organics in the sediment of the polyculture system, with an average content of 0.8%, followed by carbohydrates (0.2%) and lipids (0.1%). In the Chaohu Lake ecosystem, the composition of easily degradable organics in surface sediments is mainly carbohydrates, followed by proteins and lipids (He et al., 2016). There are significant differences between polyculture ecosystems and lakes, which may be related to sediment sources. In lake ecosystems, degradable organic compounds such as carbohydrates, proteins, and lipids mainly come from higher-plant residues, zooplankton, plant excreta, and corpses (Baldi et al., 2010; Zhang et al., 2017). Feeding feed is an important difference between polyculture ecosystems and other aquatic ecosystems. Feed is rich in protein, and feed residue settles into the bottom mud, increasing the content of protein in sediment. Therefore, the protein content of easily degraded organic matter is the highest in the polyculture system. In addition, the contents of various substances in sediments in the algae-type lake area of the Taihu Lake were higher than those in the pond aquaculture ecosystem, and their compositions were lipids (0.77%), carbohydrates (0.45%), and proteins (0.08%) (Qi et al., 2019). This may be related to the influence of breeding management measures in the artificial breeding system. Lake ecosystems usually have existed for several decades. A large amount of organic matter is deposited in the sediment environment, while mariculture ponds are dried and dredged every year. This management method leads to the exposure of a large amount of organic matter to the air and to oxidation, which results in the degradation of sediments.
Previous studies have shown that cultured shellfish can accelerate the sedimentation of various organic substances in water (Hatcher et al., 1994). At the same time, shellfish exert the filter-feeding effect of continuous organic matter assimilation, significantly reducing sediment organic matter accumulation. In this study, the order of organic matter content in sediments of all aquaculture systems was PM > PMB1 > PMB2 > PMB3, showing that the organic matter content in the sediment of PMB3, which had the highest S. constricta density, was the lowest, and was significantly lower than that in the other polyculture ecosystems. This shows that mixed culture of S. constricta, especially a high-density mixed culture, will affect the content of organic matter in the sediment. In the pond polyculture ecosystem, suspended particles such as residual bait, excrement of cultured animals, and plankton in water are the main sources of sediment organic matter. The higher the density of S. constricta is, the stronger the sediment purification effect of this filter-feeding shellfish on the environment will be, and thus the stronger the feeding ability of filter-feeding benthic animals on bacteria, particulate organic matter and attached animals and plants in water will be, resulting in a lower content of sediment organic matter in the high-density S. constricta mixed pond than that in the shrimp and crab mixed pond. In this study, the content of easily degraded organic matter was also affected by the mixed culture of S. constricta. The results showed that the content of carbohydrates, lipids, and proteins in the PMB3 sediment was the lowest, which was significantly lower than that in the other polyculture ecosystems. This may be related to the content of organic matter in the sediment. The higher the density of S. constricta is, the stronger the sediment purification effect of this filter-feeding shellfish on the environment and the lower the organic matter content in the sediment will be, thus reducing the total content of carbohydrates, lipids, and proteins in sediment. In addition, the nutrients required for the survival of filter-feeding shellfish are mainly proteins, lipids, and carbohydrates. Therefore, the higher the density of S. constricta in mixed polyculture, the greater the demand for nutrients and sediment protein.
Bacterial communities in sediments could affect the transformation processes of organic matter, and the changes in organic matter composition in sediments could also in turn change the abundance and composition of bacterial communities (Judd et al., 2006). The results of the correlation analysis showed that different types of easily degradable organics were significantly positively correlated with their corresponding functional bacteria (Table 1). Previous studies also found that the total number of bacteria in the sediment ecosystem was closely related to the concentration of organic matter, and the number was positively correlated with the change in organic matter content (Albertelli et al., 1999; Hjelm et al., 2004), which is consistent with the conclusion of this study. In the aquaculture ecosystem, with the extension of breeding time, organic matter is enriched at the bottom of the pond, creating a good living environment for bacteria and promoting their mass reproduction. Therefore, the availability of readily biodegradable organic sediments may determine the abundance of corresponding decomposing bacteria.
According to previous studies, the change in bacterial number in sediment was controlled by temperature (Garland and Mills, 1991), organic matter content in sediment (Classen et al., 2003; Xu et al., 2020), redox conditions in sediment (Muyzer et al., 1993), and feeding effects of small and large benthic animals (Sherysheva, 2021). In this study, the numbers of starch-degrading bacteria, glutin-degrading bacteria, and lipid-degrading bacteria were the smallest in PMB3, followed by PMB2 and PMB1, indicating that the mixed culture of different densities of S.constricta in the breeding system had an effect on the number of functional bacteria in the sediment. With the increase in breeding density, the number of starch-decomposing bacteria, gelatin-decomposing bacteria, and lipid-decomposing bacteria in the sediment decreased. The higher density of S. constricta can reduce the accumulation of residual bait in the sediment through the filter-feeding effect so that the organic matter content in the sediment is reduced, thus reducing the energy supply of functional bacteria. In addition, the number of cellulose-degrading bacteria was the largest in all breeding systems, which may be related to the environment of the breeding ponds. During the farming period, Suaeda salsa and other cellulose-rich plants grew in the pond dam and the surrounding shallow water area. Their dead bodies were deposited on the surface of the sediment, which may have promoted the growth and reproduction of cellulose-decomposing bacteria. The number of cellulose-decomposing bacteria in sediment was the highest in the polyculture ecosystem, which may be related to the higher biomass of S. salsa in the shallow water around PMB3.
Based on the data on the physical and chemical factors of sediment from Chai et al. (2022), it was found that temperature had no significant effect on the change in organic matter content in sediment, while Ladd et al. (1981) found that increasing temperature would accelerate the decomposition rate of soil organic matter, which is inconsistent with the conclusions of this study. This may be because the increase in sediment temperature affects the appetite of benthic organisms, making the residual bait accumulate, and the sedimentation rate of sediment is much larger than the decomposition rate of organic matter, resulting in the non-significant effect of temperature on the change in organic matter content. In addition, based on the sediment physical and chemical factor data of Chai et al. (2022), it was found that the pH value and redox potential of sediment were negatively correlated with the content of easily degraded organic matter in sediment and the number of starch-degrading bacteria, glutin-degrading bacteria, and lipid-degrading bacteria (see Table 2). The reason for this result may be that the accumulation of easily degradable organics in sediment leads to an increase in the number of corresponding functional bacteria, thus accelerating the decomposition rate of organic matter. During the decomposition process, oxidized inorganic substances act as electron acceptors and are continuously reduced, resulting in a decrease in the redox potential in the sediment (Lv et al., 2018), while the decomposition of organic matter also releases HCO3- and CO2, resulting in a decrease in pH in the sediment (Shakir et al., 2016). Previous studies have found that the oxidation–reduction potential (ORP) value can be affected by the number or activity of bacteria (Senga et al., 2010; Bollag and Stotzky, 2021).
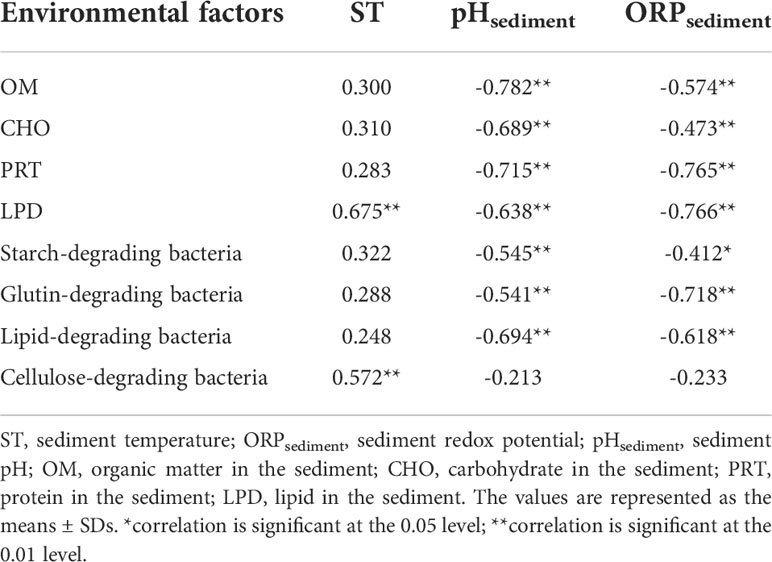
Table 2 Correlation analysis between environmental factors and sediment easily degradable organics and functional bacteria.
Conclusion
In this study, through the analysis and comparison of easily degradable organics and related functional bacteria in the sediments of different polyculture ecosystems, it was found that the composition of easily degradable organics in the sediments was different from that in other aquatic ecosystems. Protein was the dominant factor, followed by carbohydrates and lipids. Affected by the biological disturbance of Sinonovacula constricta, the content of organic matter and the number of bacteria in the sediment changed, thereby affecting pH and ORP in the sediment. The pH and ORP are important factors that reflect the changes in the number of easily degradable organics and related functional bacteria in the sediment. During the farming period, the contents of carbohydrates, lipids, and proteins in the sediment of polyculture system PMB3 were the lowest, and the easily degradable organics in the sediment were significantly correlated with its corresponding functional bacteria. High-density S. constricta coculture could significantly reduce the contents of carbohydrates, lipids, and proteins in sediment and the number of starch-degrading bacteria, glutin-degrading bacteria, and lipid-degrading bacteria. This study confirmed that the mixed culture of S. constricta can affect the content and proportion of easily degradable organics in the sediment and provide a basis for understanding the influence of S. constricta mixed culture on the sediment environment of marine aquaculture ponds.
Data availability statement
The original contributions presented in the study are included in the article/supplementary material. Further inquiries can be directed to the corresponding author.
Author contributions
HL and XC: Investigation, Formal analysis, Writing-original draft. DZ: Conceptualization, Data curation, Formal analysis. WX: Visualization, Writing-reviewing, and editing. JH: Supervision, Funding acquisition, Writing-reviewing, and editing. All authors contributed to manuscript revision, read, and approved the submitted version.
Funding
This work was supported by the National Science Foundation of China (Grant No. 32002395, 32172993); the Basic public welfare program of Zhejiang Province (Grant No. LGN22C190005); the Major Agricultural Technology Cooperation Plan of Zhejiang Province (Grant No. 2020XTTGSC03); the science and technology project of Zhoushan City and Putuo District (Grant No. 2018C31078, 2020JH131); the planned project of Zhejiang Marine Fisheries Research Institute (HYS-CZ-202105, HYS-CZ-202209).
Conflict of interest
The authors declare that the research was conducted in the absence of any commercial or financial relationships that could be construed as a potential conflict of interest.
Publisher’s note
All claims expressed in this article are solely those of the authors and do not necessarily represent those of their affiliated organizations, or those of the publisher, the editors and the reviewers. Any product that may be evaluated in this article, or claim that may be made by its manufacturer, is not guaranteed or endorsed by the publisher.
References
Albertelli G., Covazzi-Harriague A., Danovaro R., Fabiano M., Fraschetti S., Pusceddu A. (1999). Differential responses of bacteria, meiofauna and macrofauna in a shelf area (Ligurian Sea, NW mediterranean): role of food availability. J. Sea Res. 42 (1), 11–26. doi: 10.1016/S1385-1101(99)00012-X
Artha O. A., Pramono H., Sari L. A. (2019). Identification of extracellular enzyme-producing bacteria (proteolytic, cellulolytic, and amylolytic) in the sediment of extensive ponds in Tanggulrejo, Gresik, IOP Conf. Ser.: Earth Environ. Sci. 236 (1), 012003. doi: 10.1088/1755-1315/236/1/012003
Baldi F., Marchetto D., Pini F., Fani R., Michaud L., Giudice A. L., et al. (2010). Biochemical and microbial features of shallow marine sediments along the Terra Nova bay (Ross Sea, Antarctica). Cont. Shelf Res. 30 (15), 1614–1625. doi: 10.1016/j.csr.2010.06.009
Behera P. K., Das S. K., Ghosh D., Mani D., Kalpana M. S., Ikehara M., et al. (2022). Organic biogeochemical study of deeper southeastern Bengal basin sediments in West Bengal, India. Org. Geochem. 170, 104451. doi: 10.1016/j.orggeochem.2022.104451
Bligh E. G., Dyer W. J. (1959). A rapid method of total lipid extraction and purification. Can. J. Biochem. Physiol. 37 (8), 911–917. doi: 10.1139/o59-099
Chai X. R., Xu W. J., Zhang D. X., He J., Liu H. L. (2022). Effects of sinonovacula constricta polyculture on sedimentary environment in the integrated culture system of portunus trituberculatus and marsupenaeus japonicus. Oceanol. Limnol. Sin 53(3), 718–25. doi: 10.11693/hyhz20211100276
Classen A. T., Boyle S. I., Haskins K. E., Overby S. T., Hart S. C. (2003). Community-level physiological profiles of bacteria and fungi: plate type and incubation temperature influences on contrasting soils. FEMS Microbiol. Ecol. 44 (3), 319–328. doi: 10.1016/s0168-6496(03)00068-0
Dell'Anno A., Mei M. L., Pusceddu A., Danovaro R. (2002). Assessing the trophic state and eutrophication of coastal marine systems: a new approach based on the biochemical composition of sediment organic matter. Mar. Pollut. Bull. 44 (7), 611–622. doi: 10.1016/S0025-326X(01)00302-2
Dell¹Anno A., Fabiano M., Mei M. L., Danovaro R. (2000). Enzymatically hydrolyzed protein and carbohydrate pools in deep-sea sediments: Estimates of the potentially bioavailable fraction and methodological considerations. Mar. Ecol.: Prog. Ser. 196, 15–23. doi: 10.3354/meps196015
Editorial board of the Water and Wastewater Monitoring and Analysis Methods of the State Environmental Protection Administration (2002). Water and wastewater monitoring and analysis methods. 4th ed. (China: China Environmental Science Press).
Garland J. L., Mills A. L. (1991). Classification and characterization of heterotrophic microbial communities on the basis of patterns of community-level sole-carbon-source utilization. Appl. Environ. Microbiol. 57 (8), 2351–2359. doi: 10.1002/bit.260380413
Gawas V. S., Shivaramu M. S., Damare S. R., Pujitha D., Meena R. M., Shenoy B. D. (2019). Diversity and extracellular enzyme activities of heterotrophic bacteria from sediments of the central Indian ocean basin. Sci. Rep. 9 (1), 1–9. doi: 10.1038/s41598-019-45792-x
Grant J., Hargrave B. T. (1987). Benthic metabolism and the quality of sediment organic carbon. Biol. Oceanogr. 4 (3), 243–264. doi: 10.1080/01965581.1987.10749493
Hatcher A. J. G. B., Grant J., Schofield B. (1994). Effects of suspended mussel culture (Mytilus spp.) on sedimentation, benthic respiration and sediment nutrient dynamics in a coastal bay. Mar. Ecol. Prog. Ser. 115, 219–219. doi: 10.3354/meps115219
He Y. Z., Ke F., Feng M. H., Li W. C., Chen X. C., Zhou F. (2016). Characteristics and distribution of biodegradable compounds of surface sediments in lake chaohu. J. Lake Sci. 28 (1), 40–49. doi: 10.18307/2016.0105
He L. H., Li J. S. (2013). The sediment oxygen demand in the integrated prawn and solenidae ponds. Chin. Agric. Sci. Bull. 29 (35), 80–83. doi: 10.11924/j.issn.1000-6850.2013-2275
Hjelm M., Riaza A., Formoso F., Melchiorsen J., Gram L. (2004). Seasonal incidence of autochthonous antagonistic roseobacter spp. and vibrionaceae strains in a turbot larva (Scophthalmus maximus) rearing system. Appl. Environ. Microbiol. 70 (12), 7288–7294. doi: 10.1128/AEM.70.12.7288-7294.2004
Ji R. B., Mao X. H., Zhu M. Y. (1998). Impacts of coastal shellfish aquaculture on bay ecosystem. Adv. Mar. Sci. 16 (1), 21–27. https://kns.cnki.net/kcms/detail/detail.aspx?dbcode=CJFD&dbname=CJFD9899&filename=HBHH801.003&uniplatform=NZKPT&v=8UL9Zv6kaz3UTYWMwxf22XelzdVeMqAdoAlJRutddk4chkqN8RsYS5UN33OONsSl
Judd K. E., Crump B. C., Kling G. W. (2006). Variation in dissolved organic matter controls bacterial production and community composition. Ecology 87 (8), 2068–2079. doi: 10.1890/0012-9658(2006)87[2068:VIDOMC]2.0.CO;2
Ladd J. N., Oades J. M., Amato M. (1981). Microbial biomass formed from 14C, 15N-labelled plant material decomposing in soils in the field. Soil Biol. Biochem. 13 (2), 119–126. doi: 10.1016/0038-0717(81)90007-9
Liu Q., Li J., Shan H., Xie Y., Zhang D. (2022). Specific patterns and drivers of the bacterial communities in the sediment of two typical integrated multitrophic aquaculture systems. Aquacult. Int. 30 (3), 1–20. doi: 10.1007/S10499-022-00862-2
Lukwambe B., Yang W., Zheng Y., Nicholaus R., Zhu J., Zheng Z. (2018). Bioturbation by the razor clam (Sinonovacula constricta) on the microbial community and enzymatic activities in the sediment of an ecological aquaculture wastewater treatment system. Sci. Total Environ. 643, 1098–1107. doi: 10.1016/j.scitotenv.2018.06.251
Lv X., Yu P., Mao W., Li Y. (2018). Vertical variations in bacterial community composition and environmental factors in the culture pond sediment of sea cucumber apostichopus japonicus. J. Coast. Res. 84 (10084), 69–76. doi: 10.2112/SI84-010.1
Masuko T., Minami A., Iwasaki N., Majima T., Nishimura S. I., Lee Y. C. (2005). Carbohydrate analysis by a phenol–sulfuric acid method in microplate format. Anal. Biochem. 339 (1), 69–72. doi: 10.1016/j.ab.2004.12.001
Muyzer G., De Waal E. C., Uitterlinden A. (1993). Profiling of complex microbial populations by denaturing gradient gel electrophoresis analysis of polymerase chain reaction-amplified genes coding for 16S rRNA. Appl. Environ. Microbiol. 59 (3), 695–700. doi: 10.1080/15389588.2015.1013535
Newell R. I. (2004). Ecosystem influences of natural and cultivated populations of suspension-feeding bivalve molluscs: a review. J. Shellfish Res. 23 (1), 51–62. https://schlr.cnki.net/Detail/index/GARJ0010_1/NSTL0A423DF5658765D12D60CD88A385A9C5
Pisarek I., Grata K. (2013). The influence of the organic matter of sewage sediments on biological activity of microorganisms which carry out the transformations of carbon and nitrogen compounds. Pol. J. Microbiol. 62 (4), 445. doi: 10.1016/B978-0-12-417029-2.00007-8
Prijambada I. D., Widada J., Kabirun S., Widianto D. (2009). Secretion of organic acids by phosphate solubilizing bacteria isolated from oxisols. J. Trop. Soils 14 (3), 245–251. doi: 10.5400/JTS.2009.V14I3.%P
Pusceddu A., Dell'Anno A., Danovaro R., Manini E., Sara G., Fabiano M. (2003). Enzymatically hydrolyzable protein and carbohydrate sedimentary pools as indicators of the trophic state of detritus sink systems: a case study in a Mediterranean coastal lagoon. Estuaries 26 (3), 641–650. doi: 10.1007/bf02711976
Qi C., Fang J. q., Zhang L. m., Lei B., Tang X. c., Wang g. x. (2019). Composition and distribution of biodegradable compounds in phytoplankton-dominated zone of lake taihu. J. Lake Sci. 31 (4), 941–949. doi: 10.18307/2019.0423
Ren Y. J., Li Y. R. (2016). Pond farming experiment of sinonvacula constricta in tianjin. Tianjin Agric. Sci. 22 (9), 67–70. doi: 10.3969/j.issn.1006-6500.2016.09.016
Søndergaard M., Jensen J. P., Jeppesen E. (1999). Internal phosphorus loading in shallow Danish lakes. Hydrobiologia 408-409, 145–152. doi: 10.1023/A:1017063431437
Senga Y., Okumura M., Seike Y. (2010). Seasonal and spatial variation in the denitrifying activity in estuarine and lagoonal sediments. J. Oceanogr. 66 (1), 155–160. doi: 10.1007/s10872-010-0013-0
Shakir E. M. A. N., Al-Mashhady A. A., Al-Janabi Z. Z., Al-Obaidy A. H. M. (2016). Geochemical and geo-statistical assessment of heavy metals concentration in sediment of shatt Al-basrah, Iraq. J. Basic Appl. Res. Int. 18 (4), 233–239. doi: 10.1016/j.ecss.2009.12.019
Sherysheva N. G. (2021). Ecological trends of spatial distribution and of size-morphological structure of bacteriobenthos in the kuibyshev reservoir, the Volga river, Russia. IOP Conf. Ser.: Earth Environ. Sci 818 (1), 012049. doi: 10.1088/1755-1315/818/1/012049
Thayer C. W. (1979). Biological bulldozers and the evolution of marine benthic communities. Science 203 (4379), 458–461. doi: 10.1126/science.203.4379.458
Tsuchiya M., Kurihara Y. (1979). The feeding habits and food sources of the deposit-feeding polychaete, neanthes japonica (Izuka). J. Exp. Mar. Biol. Ecol. 36 (1), 79–89. doi: 10.1016/0022-0981(79)90101-1
Wang S., Xu J., Zhang X., Wang Y., Fan J., Liu L., et al. (2019). Structural characteristics of humic-like acid from microbial utilization of lignin involving different mineral types. Environ. Sci. Pollut. Res. 26 (23), 23923–23936. doi: 10.1007/s11356-019-05664-3
Xu J., Roley S. S., Tfaily M. M., Chu R. K., Tiedje J. M. (2020). Organic amendments change soil organic c structure and microbial community but not total organic matter on sub-decadal scales. Soil Biol. Biochem. 150, 107986. doi: 10.1016/j.soilbio.2020.107986
Xu G. H., Zheng H. Y. (1986). Manual of soil microbial analysis methods (China: China Agriculture Press).
Zaki M. S., Authman M. M., Abbas H. H. (2015). Bioremediation of petroleum contaminants in aquatic environments. Life Sci. J. 12 (5), 127–139. doi: 10.1007/978-94-017-2986-4_15
Keywords: Sinonovacula constricta, easily degradable organics, functional bacteria, different densities, sediment
Citation: Liu H, Chai X, Zhang D, Xu W and He J (2022) Effect of Sinonovacula constricta on sediment microbial numbers and easily degradable organics in shrimp-crab polyculture systems. Front. Mar. Sci. 9:1012893. doi: 10.3389/fmars.2022.1012893
Received: 06 August 2022; Accepted: 29 August 2022;
Published: 15 September 2022.
Edited by:
Ce Shi, Ningbo University, ChinaReviewed by:
Chengyue Liu, South China Sea Institute of Oceanology, (CAS), ChinaZhe Pan, Hebei Agricultural University, China
Copyright © 2022 Liu, Chai, Zhang, Xu and He. This is an open-access article distributed under the terms of the Creative Commons Attribution License (CC BY). The use, distribution or reproduction in other forums is permitted, provided the original author(s) and the copyright owner(s) are credited and that the original publication in this journal is cited, in accordance with accepted academic practice. No use, distribution or reproduction is permitted which does not comply with these terms.
*Correspondence: Jie He, he_0902@126.com
†These authors have contributed equally to this work and share first authorship