- 1School of Chemical and Biotechnology, Sastra University, Thanjavur, India
- 2Ocean Science and Technology for Islands, National Institute of Ocean Technology, Ministry of Earth Sciences, Government of India, Chennai, India
Introduction
India’s coastal ecosystems have been severely damaged in recent years as a result of increased urban and industrial development (Dheenan et al., 2014; Dheenan et al., 2016; Yuvaraj et al., 2018; Jha et al., 2022). The contaminants that originate from these anthropogenic actions have the potential to negatively impact various ecosystems such as corals (Jha et al., 2011; Jha et al., 2013a), mangroves (Das et al., 2014), and the ecological health and functioning of coastal regions (Pandey et al., 2022) as well as estuaries (Pandey et al., 2021). Previous studies have also shown that the areas subjected to minimal human interference generally contain better seawater quality (Jha et al., 2012; Jha et al., 2013b; Jha et al., 2015). Among the various contaminants such as nutrient eutrophication, microbial pollution, and pesticide, metal contamination plays a significant role in regulating the fisheries dynamics in the coastal regions (Jha et al., 2021). Because of their abundance, persistence, bioaccumulation, and toxicity, trace metals have been proven to influence coastal ecosystems (Ali et al., 2013; Jha et al., 2018). Fish are at the end of the aquatic food chain and thus can accumulate metals and pass them on to humans via the food chain, causing severe health problems (Al-Yousuf et al., 2000). The coastal population is highly dependent on fish and related products for their dietary supplement, and hence, it is important to estimate the metal concentration in the different organs of fish for better health management.
Seabass is protandric and generally grows up to 150 cm in size tolerating a wide range of salinity up to 40 ppt making it euryhaline (Mozanzadeh et al., 2021), and reproduces through catadromous migration (Noakes, 1988). However, these attributes, i.e., the high salinity tolerance and reproductive migration, also make them prone to various contaminations. It is well known that natural processes such as mineral weathering, volcanic eruptions, dust deposition, human-caused activities such as mining, burning fossil fuels, agriculture, industry, marine traffic, urban development, and sewage, can all contribute to metal pollution in the near-shore environment. Some metals are essential, at optimal concentration, for biological functions whereas others have no known biological role and are recognized for their toxic effects on aquatic organisms, even at environmental concentrations (Mason, 2013). The toxicity of non-essential metals such as aluminum (Al), cadmium (Cd), mercury (Hg), and lead (Pb) increases with concentration. These metals are thought to be more bio-available in their dissolved ionic form, causing toxicity. On the other hand, toxic effects of essential metals such as copper (Cu), zinc (Zn), chromium (Cr), nickel (Ni), cobalt (Co), and iron (Fe) occur either at metabolic deficiencies or at high concentrations. Fishes mostly absorb metals through their gills and digestive tract, and little amounts are absorbed through their skin (Sfakianakis et al., 2015). Heavy metals act as neurotoxins and can alter the brain and neurological systems if consumed in higher concentrations through the food chain (Fu and Xi, 2020). Considering the commercial importance and high demand of Lates cacarifer for food, this study aimed to examine the concentration of heavy metals in the different organs such as the 1) muscle, 2) liver, 3) intestine, and 4) gills. Through multivariate statistical analysis, our study estimates heavy metal contamination in the different organs of fish and helps in the assessment of environmental conditions.
Methods
Seawater and sediment were also collected with the fish samples from the Rameswaram coastal region, Tamil Nadu (Figure 1A). The area is a known religious destination in India and thousands of pilgrims visit every day. The sewage, fuel combustion, cosmetics etc., resulting from domestic and industrial discharge increase various contaminants in the nearshore environment.
Sample collection and analysis
Niskin and Van Veen Grab samplers that had been acid-cleaned were used to collect surface seawater (30–50 cm depth) and sediment samples, respectively. The samples were collected during monsoon (MON) (June to September). The collected seawater and sediment samples were transferred into acid-cleaned polypropylene bottles and polyethylene airtight bags, respectively. The seawater samples were promptly brought to pH 2–3 using supra-pure HNO3, and then stored at 4°C until analysis. Following the prescribed procedure, the sediment samples were first dried, crushed in an agate pestle and mortar, and then sieved through a 2 mm sieve (USEPA, 2001). A significant quantity of seawater and sediment in triplicates were taken and kept in an ice box for metal analysis (USEPA, 2001).
Forty-two fish of similar size groups were collected and transported to the laboratory in an ice box for further processing and subsequent analyses. In the laboratory, after recording the length and weight of fishes, they were dissected from their anal aperture using a sterile dissection knife and forceps to collect the organs like 1) muscle, 2) liver, 3) intestine and 4) gills. The organs were stored in labeled pre acid-cleaned falcon tubes at −20°C until analysis. Analytical-grade chemicals and Milli-Q water (Elix UV5 and Milli-Q, Millipore, USA) were used to prepare the solution and reagent. The Teflon container and polypropylene containers were cleaned by soaking in 5% HNO3 for 24 h, followed by a rinse in Milli-Q water and drying. Following the standard digestion protocol, 1 g of dried sediment was digested at 140°C with 5 mL HNO3 (Suprapur®, Merck) in a microwave digestion system (Anton Paar) (APHA, 2005). After digestion, the clear solution was transferred to centrifuge tubes, and 10 mL of Milli-Q water (Millipore, resistivity: 18.2 M/cm) was added to make the volume equal (Cortada and Collin, 2013). Similar procedures were used to add 5 mL of concentrated HNO3 to a 5 mL seawater sample before microwave digestion. Fish organs were digested in 5 mL of pure HNO3 and diluted to 20 mL with Milli-Q water for trace metal analysis. Inductively coupled plasma mass spectrometry (ICP-MS) was used to measure the concentrations of trace elements (Agilent 7500). Samples, certified reference materials (CRM), and duplicate analytical blanks were all examined for any potential contamination as per the procedure followed by Jha et al. (2019). The reference value (seawater and sediment), as well as the analyzed mean and standard deviation of the elemental values obtained for the CRM [NIST CRM048 (sediment) and NIST CRM QC3163 (seawater)], is adopted from Jha et al. (2019). By comparing the analyzed value to the CRM value, the precision and correctness of the analysis were confirmed. The metal concentrations in seawater were represented as ppb, whereas in sediment and fish organs as ppm.
Seawater was tested for pH, salinity, and temperature using the multi-parameter water quality probes (HANNA, HI98194) (APHA, 2005). The data collected for seawater, sediment, and fish were subjected to descriptive statistics to estimate the metal concentration. An analysis of variance (ANOVA, one-way) test was used to examine the significant differences (α = 0.05) among the different organs of fish. Though a detailed analysis was carried out for the seawater, sediment, and fish organs, the major focus was on metal concentration in the fish organs. Box-whisker plots and correlation between metal levels in various organs of the fish were determined. Hierarchical clustering was performed using Ward’s method, through squared Euclidean distances as a measure of the dissimilarity matrix. All multivariate analyses were carried out using the SPSS software (version 18).
Results and discussion
The length and body weight of L. calcarifer ranged from 10.2 to 12.4 cm and 10.93 to 23 g, respectively. Trace metal concentrations and bioavailability in coastal environments are heavily influenced by water quality characteristics such as pH, salinity, and organic matter, since they can precipitate as fresh/estuarine water interacting with seawater (Jha et al., 2019). The seawater temperature variation (29.00°C–30.65°C), pH (7.95–8.15), and salinity (30.5–31.5 PSU) were measured during the study. The trace metal percentage of CRM for seawater and sediment are given in Supplementary Table S1. The trace metal concentration in seawater and sediment are summarized in Table S2. However, trace metal concentrations in different fish organs are summarized in Table S3. Heavy metals like Cd, Hg and Pb were below detection level (BDL) in seawater and sediment samples. However, Fe had comparatively higher concentrations in seawater, sediment, and also in fish samples.
The concentration of Nickel (Ni) in seawater varied from 1.86 to 5.43 ppb, whereas it ranged from 6.9 to 7.62 ppm in sediment. Further, in fish organs, gills showed a higher value (1.31–4.89 ppm) whereas muscle showed BDL. The variation of Ni was significant (p < 0.05, F = 11.915) in various fish organs.
Copper (Cu) and zinc (Zn) enter the coastal ecosystem mostly through anthropogenic wastes such as fertilizers, algaecides, fungicides, and molluscicides, which in turn are deposited in the atmosphere and return to the sea through precipitation (Srichandan et al., 2016). Copper concentration varied from 24.3 to 96.6 ppb in seawater, while the concentration ranged from 1.5 to 10.6 ppm in sediment. This could be attributed to the application of biocides (antifouling paints) in ships and boats to prevent the growth of potential fouling organisms and also due to organic inputs from anthropogenic activities such as agriculture and industry in the coastal regions (Anbuselvan et al., 2018). Cu showed a higher value in the gills (10–48.26 ppm) whereas it was least in the muscle (0.85–4.49 ppm) of the fish. Zinc varied from 16.24 to 24.30 ppb in seawater, while the concentration ranged from 3.91 to 4.86 ppm in sediment. Ships and boats use anticorrosive paint, in which zinc sulphate is one of the chemical component (Yasar et al., 2001). The Zn showed a higher value in gills (16.4–24 ppm) and lower value was observed in the liver (5.44–11.09 ppm).
Manganese (Mn) is a transition metal that is essential in low concentrations but toxic in high concentrations (Srichandan et al., 2016). Its concentration in seawater ranged from 12.69 to 26.38 ppb and from 231 to 243 ppm in sediment. Further, in fish organs, liver showed a higher concentration (2.44–102.39 ppm) whereas it was least in the intestine (0.001–0.43 ppm), followed by muscle (0.82–1.6 ppm). Mn variation was significant (p < 0.05, F = 8.828) in various fish organs.
The concentration of cobalt (Co) in seawater was below detection level (BDL), whereas it ranged from 3.2 to 3.49 ppm in sediment. Further, fish organs, gills, and liver showed a similar value of 0.72 ppm whereas muscle and intestine showed negligible value. It is reported that (Tovar-Sánchez and Flegal, 2004) Co reaches to the coastal environment through the estuary system. During the current investigation, Co variation was insignificant (p > 0.05, F = 2.774) in various fish organs.
Total aluminum (Al) normally exists in relatively low amounts (5 ppb) in seawater; but in river water, it may reach 400 ppb (Jha et al., 2019). It is toxic to fish at 1500 ppb level in seawater (https://www.lenntech.com/periodic/water/aluminum/aluminum-and-water.htm). Total Al concentrations in seawater varied from 3.30 to 5.47 ppb, whereas in sediment it ranged from 3759 to 4918 ppm. Further, in fish organs, gill showed a higher concentration (16.95–68 ppm) whereas it was least in the muscle (0.96–1.89 ppm). During the current investigation, Al variation was significant (p < 0.05, F = 15.589) in various fish organs. The seawater contained higher concentrations of Iron (Fe) (355–463 ppb). Similarly, in sediment also higher Fe concentration was recorded ranging from 3665 to 4986 ppm. However, in fish, the maximum value of Fe was observed (43–159 ppm) in the gills compared to the other organs and it was least in the muscle (11.74–19.89 ppm). The concentration in the muscle was well within the permissible level as per the guidelines of WHO (1989).
The main sources of Cr are river drainage, dredging sludge, and dumping of industrial wastes (Jha et al., 2019). The concentration of Cr varied from 0.40 to 0.58 ppb in seawater, while it ranged from 15.48 to 16.80 ppm in sediment. Further, in fish organs, gills showed a higher value of 2.6 to 78 ppm whereas it was least in the muscle (0.001 to 0.55 ppm). The concentration of lead (Pb) varied from 2 to 4.6 ppb in seawater, while it ranged from 3.57 to 4.60 ppm in sediment. It enters the coastal ecosystem mainly by atmospheric deposition (Mukai et al., 1993), fly ash (Selvaraj et al., 2003), coal combustion (Anbuselvan et al., 2018), and anthropogenic activities. The Pb concentration in the fish organ was observed as BDL.
The rank order distribution in terms of mean metal concentration in seawater was: Fe (424 ppb) > Cu (56.67 ppb) > Mn (17.89 ppb) > Al (4.32 ppb) > Ni (3.83 ppb) > Pb (3.1 ppb) > Cr (0.46 ppb) > Co (BDL). In comparison, the rank order of metals in sediment was: Al (4226 ppm) > Fe (4117 ppm) > Mn (236 ppm) > Cr (15.95 ppm) > Ni (7.26 ppm) > Cu (5.66 ppm) > Zn (4.52 ppm) > Pb (3.97 ppm) > Co (3.32 ppm). The concentration of each metal varied considerably based on different organs (Table S3); however, the overall rank order of mean concentration of metals in fish was: Fe (56.99 ppm) > Mn (22.27 ppm) > Cr (13.82 ppm) > Zn (12.31 ppm) > Al (11.91 ppm) > Cu (9.02 ppm) > Ni (1.33 ppm) > Co (0.26 ppm) > Pb (BDL).
The box-whisker plots for trace metals in fish organs are given in Figures 1B–H. Ni, Cu, Fe, Cr, Al, Co (Figures 1B–G), and Mn (Figure 2A) show that the underlying distribution is skewed toward high concentration in the fish organs and has long whiskers at the top of the box. Overall, the concentration of metals was found higher in gills compared to other organs. The mild outliers (circles) and extreme outliers (asterisks), which are randomly distributed, suggest the natural variability of metal in different organs of the fish. The one-way ANOVA results showed that apart from Co (p > 0.05), the concentration of all the metals significantly varied (p < 0.05) between different organs of the fish (Table S5).
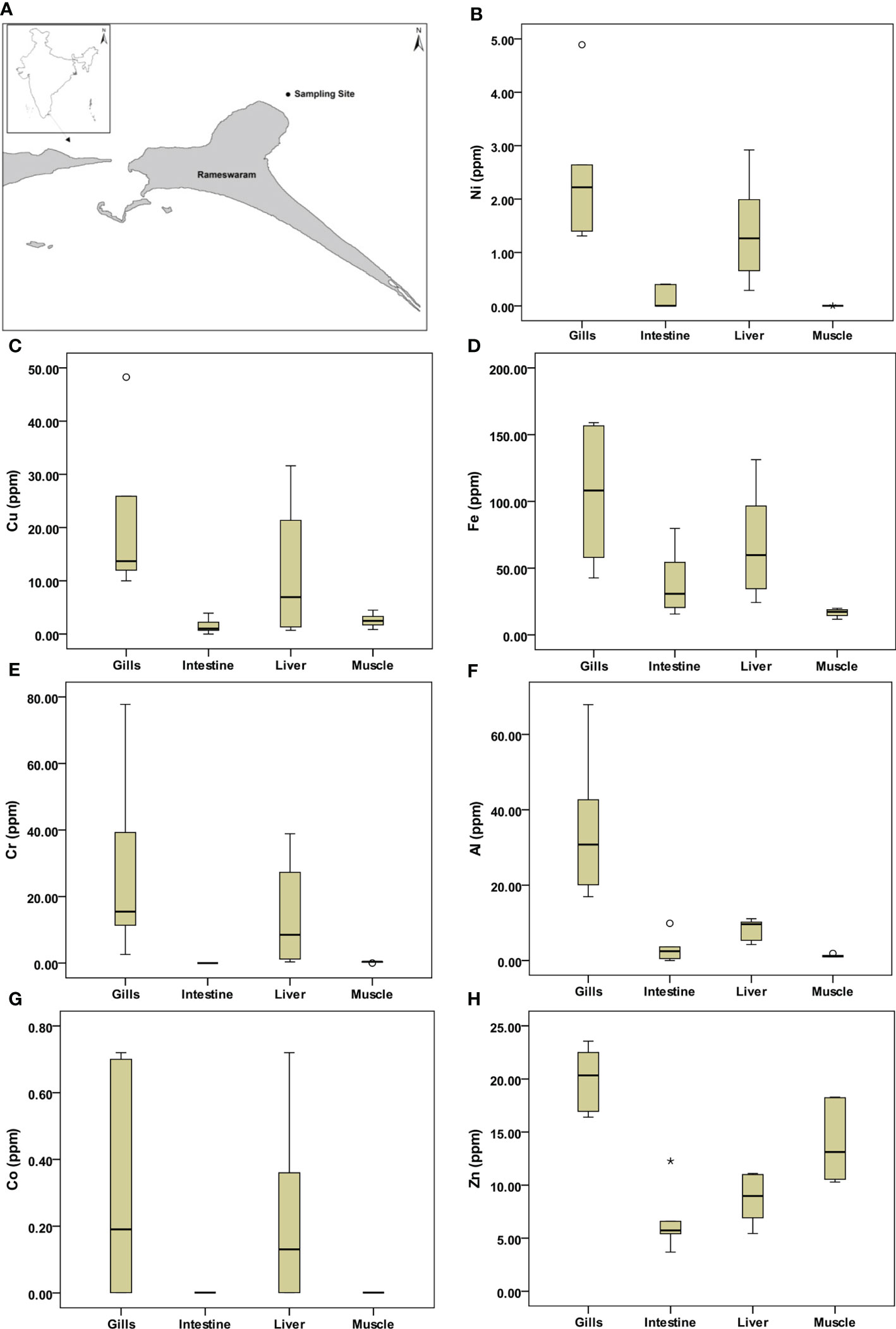
Figure 1 Study area map and box-whisker plots for selected metal variables in different parts of fish. (A) Sampling location, (B) Nickel, (C) Copper, (D) Iron, (E) Chromium, (F) Aluminum, (G) cobalt, and (H) Zinc. In each plot, the median is represented by the central point, the interval is represented by the rectangle (i.e. 25% and 75% percentiles), and the range is indicated by the whisker (*: extreme outlier; ◦: mild outlier).
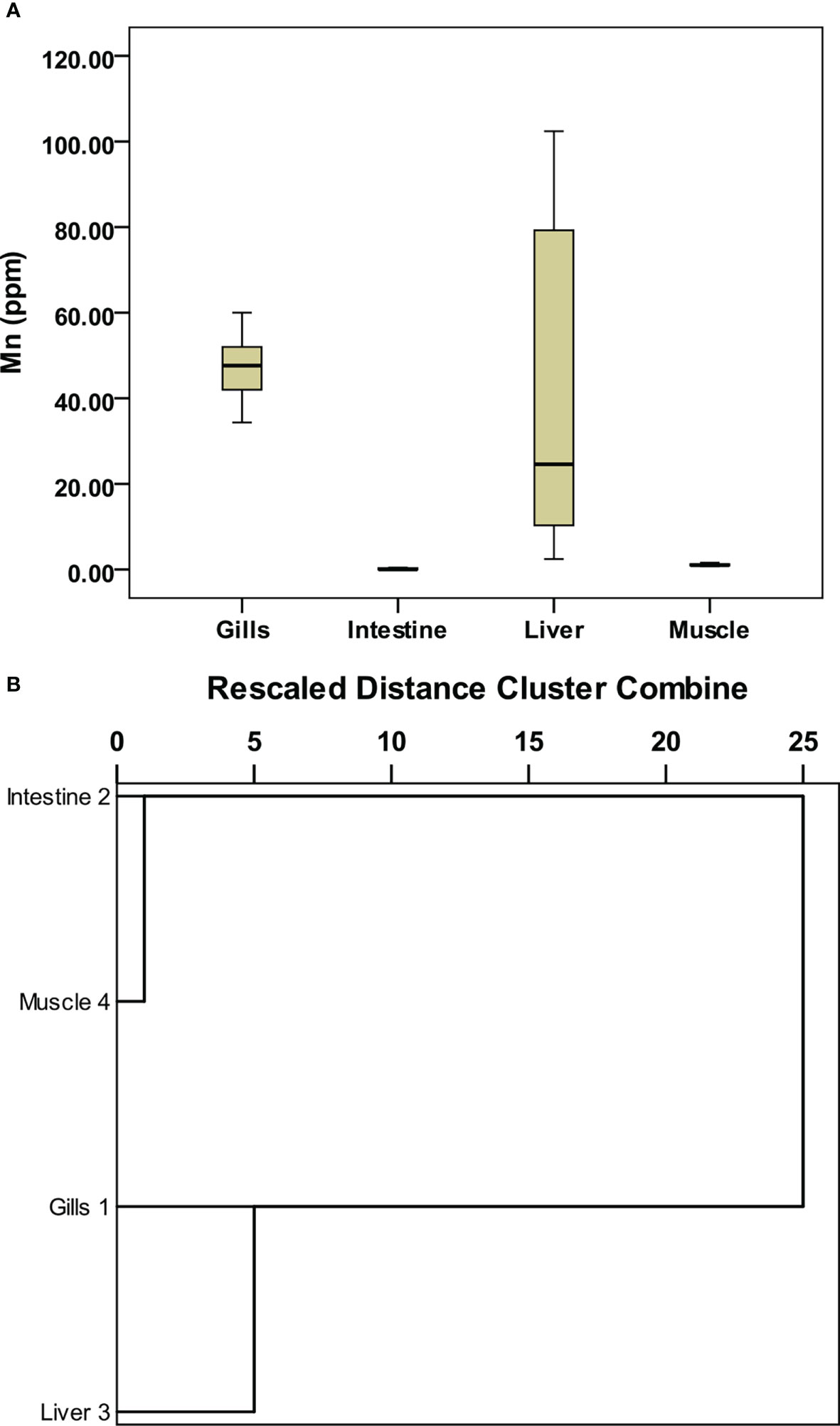
Figure 2 Box-whisker plots for selected metal variables in different parts of fish and Cluster analysis. (A) Manganese, and (B) dendrogram of the metal variables in different organs of the fish.
The hierarchical clustering (Figure 2B) grouped the fish organs in the same or different clusters based on similarity or dissimilarity in the concentration of each metal. The resultant dendrogram produced one cluster keeping the intestine and muscle together whereas the gills and liver corresponded to outliers. The concentration of metals in gills was significantly different from that of other organs; therefore, it formed an outlier in the dendrogram. The metal concentration in the intestine and muscle was quite similar to each other; therefore, they formed a separate cluster. The concentration of metals in the liver showed similarity with the gills and therefore formed a separate cluster associated with the gills.
The concentration of metals in each organ of the fish was analyzed using Pearson’s correlation to establish their relationship, and the results are shown in Table S4. Except for a few, all metals showed a significant positive correlation (p < 0.05) with each other. The correlation of Zn with Mn and Co was not significant (p > 0.05); Al and Cr were also not significantly correlated. This could be attributed to different concentrations of these metals in different organs of the fish.
The heavy metal concentration in fish muscle was found in trace quantities for essential metals whereas toxic metals such as Pb, Hg, and Cd were BDL. The mean concentration of trace metals in fish organs, seawater, and sediment was well within the permissible level as per the guidelines (WHO, 1989). However, considering that this location is a tourist hotspot, regular monitoring is required to estimate the metal concentration, develop a mitigation plan for promoting healthy habitats for flora and fauna, and contribute to coastal conservation and fisheries management (Murugan et al., 2005; Vijayakumaran et al., 2005; Kumar et al., 2009; Jha et al., 2017; Pandey et al., 2021; Pandey et al., 2022).
Conclusion
The study reveals that heavy metal concentrations of cadmium, lead, and mercury were below detection level, whereas other metal concentrations especially for the fish muscle were well within the permissible level. The highest heavy metal concentrations were found in gills and the lowest concentration occurred in the muscle. Since fish muscle is a consumable part, metal contamination may be harmful to human beings. Results indicate that the level of metals in the fish muscle is well within the standard levels and safe for human consumption. Although the concentration of heavy metals is negligible, routine metal toxicology studies must be conducted to evaluate the health of the coastal ecosystem and fish stock assessment in the region.
Data availability statement
The original contributions presented in the study are included in the article/Supplementary Material. Further inquiries can be directed to the corresponding author.
Ethics statement
Commercial fish does not require ethical committee approval.
Author contributions
SV and MK: Collection of samples, analysis of samples, processing of data, and writing-original draft. JS: Sample collection and dissection; VP: Statistical analysis and reviewing the manuscript; DJ: Conceptualization, sampling design, method validation, reviewing the manuscript and guidance; GD: Administration and project management. All authors contributed to the article and approved the submitted version.
Acknowledgments
The authors are thankful to Dr. G. A. Ramadass, Director, NIOT, Chennai, for allowing the student internship project at NIOT. We also like to thank the scientific and supporting staffs of MBT Groups in Chennai, India, for their timely help.
Conflict of interest
The authors declare that the research was conducted in the absence of any commercial or financial relationships that could be construed as a potential conflict of interest.
Publisher’s note
All claims expressed in this article are solely those of the authors and do not necessarily represent those of their affiliated organizations, or those of the publisher, the editors and the reviewers. Any product that may be evaluated in this article, or claim that may be made by its manufacturer, is not guaranteed or endorsed by the publisher.
Supplementary material
The Supplementary Material for this article can be found online at: https://www.frontiersin.org/articles/10.3389/fmars.2022.1012541/full#supplementary-material
References
APHA (American Public Health Association) (2005). Standard Methods for the Examination of Water and Wastewater. 21th edition (Washigton, D.C: American Works Association and Water Environmental Federation).
Ali A., Dahanukar. N., Raghavan R. (2013). Length-weight and length-length relationship of three species of snakehead fish, channa diplogramma, C. marulius and C. striata from the riverine reaches of lake vembanad, Kerala, India, CEPF Western ghats special series. J. Threatened Taxa 5 (13), 4769–4773. doi: 10.11609/JoTT.o3353.4769-73
Al-Yousuf M. H., El-Shahawi M. S., Al-Ghais S. M. (2000). Trace metals in liver, skin and muscle of lethrinus lentjan fish species in relation to body length and sex. Sci. Total Environ. 256, 87–94. doi: 10.1016/S0048-9697(99)00363-0
Anbuselvan N., D. S. N., Sridharan M. (2018). Heavy metal assessment in surface sediments off coromandel coast of India: Implication on marine pollution. Mar. pollut. Bull. 131, 712–726. doi: 10.1016/J.MARPOLBUL.2018.04.074
Cortada U., Collin M. (2013). The nature and contamination of sediments in the Plentzia estuary (Biscay province, Spain). Geogaceta 54, 147–150.
Das A. K., Jha D. K., Devi M. P., Sahu B.K., Vinithkumar N.V., Kirubagaran R., et al. (2014). Post tsunami mangrove evaluation in coastal vicinity of Andaman Islands, India. J. Coast. Conserv. 18, 249–255. doi: 10.1007/s11852-014-0312-5
Dheenan P. S., Jha D. K., Das A. K., Vinithkumar N. V., Prashanthi Devi M., Kirubagaran R., et al. (2016). Geographic information systems and multivariate analysis to evaluate fecal bacterial pollution in coastal waters of Andaman, India. Environ. pollut. 214 (7), 45–53. doi: 10.1016/j.envpol.03.065
Dheenan P. S., Jha D. K., Vinithkumar N. V., Ponmalar A. A., Venkateshwaran P., Kirubagaran R. (2014). Spatial variation of physicochemical and bacteriological parameters elucidation with GIS in rangat bay, middle andaman. India. J. Sea Res. 85, 534–541. doi: 10.1016/j.seares.2013.09.001
Fu Z., Xi S. (2020). The effects of heavy metals on human metabolism. Toxicol. Mech. Methods 30 (3), 167–176. doi: 10.1080/15376516.2019.1701594
Jha D. K., Begum M., Das A. K., Sahu B. K., Kirubagaran. R. (2012). Environmental quality assessment of treis island, nicobar, india. J. Mater. Environ. Sci. 3, 800–807. Available at: http://www.jmaterenvironsci.com/Document/vol3/vol3_N5/81-JMES-211-2012-Jha.pdf.
Jha D. K., Kirubagaran R., Vinithkumar N. V., Dharani G., Madeswaran P. (2018). "The Andaman and Nicobar Islands" in World seas: An environmental evaluation, ed (UK and USA: Charles Sheppard (Academic press), 185–209.
Jha D. K., Dharani G., Verma. P., Ratnam K., Senthil Kumar. R., Rajaguru S. (2021). Evaluation of factors influencing the trace metals in puducherry and diu coasts of India through multivariate techniques. Mar. pollut. Bull. 167 (6), 2021. doi: 10.1016/j.marpolbul.2021.112342
Jha D. K., Pandey V., Muthukumar C., Sathish Kumar P., Venkatnarayanan S., Jebakumar J. P. P., et al. (2022). Investigation of coastal water characteristics along the southeast coast of India: A multivariate approach. Front. Mar. Sci. 9, 945495. doi: 10.3389/fmars.2022.945495
Jha D. K., Prashanthi Devi M., Vinithkumar N. V., Das A. K., Kumar A., Dheenan P. S., et al. (2013b). Comparative investigation of water quality parameters of aerial & rangat bay, Andaman islands using In-situ measurements and spatial modelling techniques. Water Qual. Expo. Health 5, 57–67. doi: 10.1007/s12403-013-0086-2
Jha D. K., Ratnam K., Rajaguru S., Dharani G., Devi M. P., Kirubagaran R. (2019). Evaluation of trace metals in seawater, sediments, and bivalves of nellore, southeast coast of India, by using the multivariate and ecological tool. Mar. pollut. Bull. 146, 1–10. doi: 10.1016/J.MARPOLBUL.2019.05.044
Jha D. K., Vinithkumar N. V., Marimuthu N., Baskar B., Sahu B., Das A. K., et al. (2013a). Field and GIS based post-tsunami assessment of scleractinian coral cover in the aerial bay group of islands, north Andaman, India. J. Coast. Conserv. 17, 671–677. doi: 10.1007/s11852-013-0266-z
Jha D. K., Vinithkumar N. V., Sahu B. K., Dheenan P. S., Das A. K., Begum M., et al. (2015). Multivariate and geo-spatial approach for seawater quality of chidiyatappu bay, south Andaman islands, India. Mar. pollut. Bull. 96, 1–2. doi: 10.1016/j.marpolbul.2015.05.004
Jha D. K., Vinithkumar N. V., Santhnakumar J., Nazar A. K. A., Kirubagaran R. (2011). Assessment of post tsunami coral reef resource in pongi balu coast, south Andaman islands. Curr. Sci. 100 (4), 530–534. Available at: https://www.currentscience.ac.in/Volumes/100/04/0530.pdf.
Jha D. K., Rajaprabhu G, Kirubagaran, R., Sendhil Kumar R., Dharani G., Das A. K. (2017). Estimation of potential zones for offshore mariculture in the Indian Sea using geographical information system as a management tool. J. Coast. Conserv. 21, 893–902. doi: 10.1007/s11852-017-0556-y
Kumar T. S., Vijayakumaran M., Murugan T. S., Jha D. K., Sreeraj G., Muthukumar S. (2009). Captive breeding and larval development of the scyllarine lobster petrarctusrugosus, New Zealand. J. Mar. Freshw. Res. 43 (1), 101–112. doi: 10.1080/00288330909509985
Mukai H., Furuta N., Fujii T., Ambe Y., Sakamoto K., Hashimoto Y. (1993). Characterization of sources of lead in the urban air of Asia using ratios of stable lead isotopes. Environ. Sci. Technol. 27, 1347–1356. doi: 10.1021/es00044a009
Mozanzadeh M. T., Safari O., Oosooli R., Mehrjooyan S., Najafabadi M. Z., Hoseini S. J., et al. (2021). The effect of salinity on growth performance, digestive and antioxidant enzymes, humoral immunity, and stress indices in two euryhaline fish species: Yellowfin seabream (Acanthopagrus latus) and Asian seabass (Lates calcarifer). Aquaculture 534, 736329. doi: 10.1016/J.AQUACULTURE.2020.736329
Murugan T. S., Remany M. C., Mary Leema T., Jha D. K., Santhanakumar J., Vijayakumaran M., et al. (2005). Growth, repetitive breeding, and aquaculture potential of the spiny lobster, panulirus ornatus, new Zealand. J. Mar. Freshw. 39 (2), 311–315. doi: 10.1080/00288330.2005.9517311
Noakes D. (1988). Common strategies of anadromous and catadromous fishes. American fisheries society symposium 1. Trans. Am. Fish. Soc. 117 (5), 515–518. doi: 10.1577/1548-8659-117.5.515
Pandey V., Jha D. K., Sathish Kumar P., Santhanakumar J., Venkatnarayanan S., Jebakumar J., et al. (2022). “Effect of multiple stressors on the functional traits of sub-tidal macrobenthic fauna: A case study of the southeast coast of India”. Mar. pollut. Bull. 175, 113355. doi: 10.1016/j.marpolbul.2022.113355
Pandey V., Venkatnarayanan S., Sathish Kumar P., Ratnam K., Jha D. K., Rajaguru S., et al. (2021). Assessment of ecological health of swarnamukhi river estuary, southeast coast of India, through AMBI indices and multivariate tools. Mar. pollut. Bull. 164, 112031. doi: 10.1016/j.marpolbul.2021.112031
Selvaraj K., Ram Mohan V., Jonathan M. P., Siddartha R., Srinivasalu S. (2003). Distribution of nondetrital trace metals in sediment cores from Ennore Creek, Southeast coast of India. J. Geol. Soc India 62, 191–204.
Sfakianakis D. G., Renieri E., Kentouri M., Tsatsakis A. M. (2015). Effect of heavy metals on fish larvae deformities: A review. Environ. Res. 137, 246–255. doi: 10.1016/J.ENVRES.2014.12.014
Srichandan S., Panigrahy R. C., Baliarsingh S. K., Rao B. S., Pati P., Sahu B. K., et al. (2016). Distribution of trace metals in surface seawater and zooplankton of the bay of Bengal, off rushikulya estuary, East coast of India. Mar. pollut. Bull. 111 (1–2), 468–475. doi: 10.1016/J.MARPOLBUL.2016.06.099
Tovar-Sánchez A., Sergio A., Russell A. (2004). Temporal and spatial variations in the biogeochemical cycling of cobalt in two urban estuaries: Hudson River estuary and San Francisco Bay. Estuar. Coast. Shelf Sci. 60, 717–728. doi: 10.1016/j.ecss.2004.03.010
USEPA (2001). Methods for collection, storage and manipulation of sediments for chemical and toxicological analyses: Technical manual (Office of Water, Washington, DC: USEPA).
Vijayakumaran M., Murugan T. S., Remany M. C., Mary Leema T., Jha D. K., Santhanakumar J., et al. (2005). Captive breeding of the spiny lobster, panulirus homarus, New Zealand. J. Mar. Freshw. Res. 39 (2), 325–334. doi: 10.1080/00288330.2005.9517313
WHO (World Health Organization) (1989). Heavy Metals Environmental Aspects. Environ. Health Criteria (Geneva, Switzerland) 85.
Yasar D., Aksu A. E., Uslu O. (2001). Anthropogenic pollution in Izmit Bay: heavy metals in surface sediments. Turk. J. Eng. Environ. Sci. 25, 299–313.
Keywords: fish, metal toxicity, Asian seabass, multivariate approach, Tamil Nadu coast
Citation: Koduvayur MV, Vasudevan S, Pandey V, Santhanakumar J, Jha DK and Dharani G (2022) Comparative evaluation of heavy metal concentration in different organs of the asian seabass: A multivariate approach. Front. Mar. Sci. 9:1012541. doi: 10.3389/fmars.2022.1012541
Received: 05 August 2022; Accepted: 05 September 2022;
Published: 04 October 2022.
Edited by:
Chee Kong Yap, Putra Malaysia University, MalaysiaReviewed by:
Thilagam Harikrishnan, Pachaiyappa’s College for Men, IndiaRajamanickam Krishnamurthy, Arignar Anna Government Arts and Science College Chennai, India
Copyright © 2022 Koduvayur, Vasudevan, Pandey, Santhanakumar, Jha and Dharani. This is an open-access article distributed under the terms of the Creative Commons Attribution License (CC BY). The use, distribution or reproduction in other forums is permitted, provided the original author(s) and the copyright owner(s) are credited and that the original publication in this journal is cited, in accordance with accepted academic practice. No use, distribution or reproduction is permitted which does not comply with these terms.
*Correspondence: Shrivarshini Vasudevan, shrivarshiniv17@gmail.com