- 1Guangdong Provincial Key Laboratory of Marine Biotechnology, Shantou University, Shantou, Guangdong, China
- 2The Key Laboratory of Mariculture, Ministry of Education, Ocean University of China, Qingdao, Shandong, China
- 3Fisheries College, Zhejiang Ocean University, Zhoushan, Zhejiang, China
Growth performance and aggressive behavior are important measures in fish aquaculture. So, from the perspectives of food production and fish welfare, it is always valuable to increase growth rate and reduce fish aggression efficiently. Physical enrichment has been verified to be a promising method to improve fish welfare. But there is very scarce knowledge about social enrichment, which is an important category of environmental enrichment. In the wild, several fish species often inhabit the same habitat, inevitably experiencing social interactions with each other. From the perspective of nature-based welfare, such social interactions are essential for fish ontogenesis, and deprivation of this social environment may damage fish welfare. Here, we focused on two typical territorial fish species, black rockfish (Sebastes schlegelii) and fat greenling (Hexagrammos otakii), which are often found to inhabit the same area in the wild, in contrast, traditional fish farms simultaneously rear them with the monoculture mode. And we explored the effects of social enrichment (through introducing different numbers of two fishes into the same environment) on their growth and aggression. The results showed that introducing rockfish as a stimulus significantly increased the growth performance of greenling (especially the medium and high enrichment amounts), but social enrichment did not present such effects on the growth of rockfish. Proper social enrichment also significantly decreased the intraspecies aggression both among rockfish and among greenling. Moreover, greenling expressed significantly more aggressive behavior toward rockfish (i.e., the interspecies aggression from greenling toward rockfish) than in reverse. Finally, the average distribution proportion in central areas and standard metabolic rate of greenling were significantly higher than those of rockfish. These results suggest that proper social enrichment is an effective method to decrease aggression and increase growth of the fat greenling, but this improvement is accompanied by a compromised rockfish welfare. This study will promote the application of environmental enrichment strategy on improving the welfare of specific fish species and provide fundamental information for reducing fish aggression and improving fish growth in aquaculture. Further research is needed to optimize the combinations of fish species for practical applications in aquaculture.
Introduction
Aquaculture is one of the fastest-growing food-producing systems in the world (Gerber et al., 2015). Growth performance and aggressive behavior have been seen as two of the most important measures of fish welfare in aquaculture (Ashley, 2007; Martins et al., 2012). And aggressive behavior may cause higher physiological stress, severe body damage, lower appetite and food conversion efficiency, lower disease resistance, and finally suppressed growth rate (Wendelaar Bonga, 1997; Mommsen et al., 1999; Ashley, 2007; Galhardo and Oliveira, 2009; Näslund and Johnsson, 2014). Therefore, aggressive behavior is often regarded as a reliable indicator of fish welfare (Martins et al., 2012; Näslund and Johnsson, 2014; Zhang et al., 2020a; Zhang et al., 2020b; Zhang et al., 2021a). In recent years, with the development of the aquaculture industry, fish welfare has gained increasing attention from scientists, animal protection organizations, and the public (Huntingford et al., 2006; Ashley, 2007; Martins et al., 2012; Gerber et al., 2015; Stevens et al., 2017; Kristiansen et al., 2020). The concept of fish welfare has abundant connotation and can be simply defined as the state of fish as it copes with surrounding environment (Broom, 1991). More detailed, fish welfare can be defined by function-based, nature-based, or feelings-based approaches (Huntingford et al., 2006). Among them, function-based welfare may be the most common used concept in aquatic animal welfare field, and it constitutes part of bases of the other two welfare context (Zhang et al., 2022). Function-based welfare concept is prevalent in aquaculture field mainly due to relatively clear indicators for evaluating fish welfare status and its close association with production outputs (Huntingford et al., 2006; Ashley, 2007). Commonly, fish welfare can be assessed by several behavioral, physiological (and maybe psychological) indicators, for example, body damage, aggressive behavior, stereotypy, cortisol and other blood parameters, growth performance and disease resistance (Martins et al., 2012; Kristiansen et al., 2020; Barreto et al., 2022). Considering aquaculture systems are inevitably inundated with various stressors (e.g., handling, social stress), it is essential to further optimize present aquaculture systems and to find practical ways to improve fish growth and decrease aggressive behavior, from the perspectives of fish welfare and food production.
Environmental enrichment, a method to increase the environmental complexity and heterogeneity, has been repeatedly proposed to have great potential to improve fish behavioral and physiological performance (Johnsson et al., 2014; Näslund and Johnsson, 2014; Gerber et al., 2015; Jones et al., 2021). Based on the aims and methods of enrichment, it is generally categorized into five types: physical enrichment, social enrichment, sensory enrichment, occupational enrichment, and dietary enrichment (Bloomsmith et al., 1991; Young et al., 2020). Until now, most enrichment studies have focused on physical enrichment, and few studies attempted to explore the effects of other enrichment types, especially social enrichment (Näslund and Johnsson, 2014; Jones et al., 2021; Arechavala-Lopez et al., 2022). Social enrichment refers to the increase of social interactions among intra- or inter-species. And obviously, such an increase could be seen as an increase in environmental complexity and heterogeneity (Bloomsmith et al., 1991; Näslund and Johnsson, 2014; Young et al., 2020). Considering that general consequences of physical enrichment and social enrichment is somewhat similar (i.e., increase environmental complexity) and that physical enrichment has been verified to have positive effects on fish behaviors and physiology (Näslund and Johnsson, 2014; Gerber et al., 2015; Jones et al., 2021), we predicted that social enrichment maybe also improves fish welfare and growth. In recent years, it has been widely studied how to manipulate the rearing density in fish monoculture to improve the welfare and growth performance. However, the studies focusing on the effects of polyculture (i.e., social enrichment through introducing inter-species stimuli) on fish welfare are relatively few.
Black rockfish Sebastes schlegelii and fat greenling Hexagrammos otakii are two typical territorial fish species, widely distributed in the coastal waters of China, Korea, and Japan (Hu et al., 2017; Zhang et al., 2021b). In recent years, aquaculture of these two fishes in northern China has rapidly developed. But severe aggressive behavior, body damage, and subsequent growth suppression are often observed during the rearing process (Zhang et al., 2019; Zhang et al., 2020a). So, how to efficiently reduce aggression and improve growth are important questions to be solved, from the perspectives of both food production and fish welfare. Typical rearing environments in industrial aquaculture rear fish in single species to optimize the growth rate. This strategy may be efficient for fish growth considering each species has its specific optimal range of environmental factors, but it may be not true for fish behaviors and welfare (Johnsson et al., 2014; Johnsson and Näslund, 2018). In the wild, black rockfish and fat greenling always inhabit the same sea area and interact with each other. This phenomenon indicates that the social stimulus created by social interactions with the other species may be an important factor to guarantee normal individual development. In contrast, a single-species fish rearing environment in aquaculture (i.e., deprivation of interspecies social interaction) may disturb the expression of natural instincts, stimulate harmful behaviors, suppress growth performance, and damage the nature-based welfare (Johnsson et al., 2014; Kristiansen et al., 2020). In addition, in practical production, black rockfish and fat greenling are often reared simultaneously in the same fish farms, with similar daily management procedures (e.g., feeding strategy, fodder characters, seawater change, oxygen supplement, and others). This situation makes the polyculture of the two fish species feasible and economic. The similar ecological habits of the two species allow us to predict that polyculture will, at least, provide additional social stimuli from the other fish and subsequent welfare benefits compared with the monoculture of each species.
Based on the above backgrounds, we questioned that in an aquaculture scenario, whether black rockfish can be regarded as a social stimulus to modulate the welfare of fat greenling, and vice versa. The aim of this study was to explore whether social enrichment is an effective modulator for the growth and aggression of black rockfish and fat greenling and to compare the metabolic difference between the two fish species to give a potential explanation for the phenotypic difference. Different amounts of social enrichment were created by introducing different numbers of two fishes into the same environment. According to the ecological habits of the target fish, we predicted that social enrichment would provide additional social stimuli, distract fish attention, modulate aggression and distribution, and consequently improve growth performance.
Materials and methods
Animals and general descriptions for the serial experiments
We designed three serial experiments to explore the effects of social enrichment on the welfare of two fishes, black rockfish S. schlegelii and fat greenling H. otakii. The three experiments, including a rearing experiment (last for one month), a short-term behavior-observational experiment (last for seven days), and a metabolism-determined experiment, were sequentially conducted. The logical chain of this study was first explore the enrichment effects on fish growth, then give explanations for the growth phenotype at the behavioral level, and finally, elucidate possible reasons for behavioral differences between the two fish species from the perspective of metabolism.
In the three experiments, rockfish and greenling juveniles were all obtained from a commercial hatchery (Weihai City, Shandong Province, China) and acclimated to the experimental conditions for one week before the formal experiments. Rearing glass aquaria (60 cm × 50 cm × 50 cm) were part of an indoor flow-through seawater system equipped with mechanical filters and were provided with air stones to ensure sufficient dissolved oxygen (6.9 ± 0.1 mg/L, 98.5 ± 0.3% saturation). The water flow rate was 2 L/min, and the water depth was maintained at 40 cm. The water used in all experiments was natural seawater kept at 19°C. And the photoperiod followed the autumnal natural day-night cycle (12 h light/12 h dark). The experiment I, II, and III were conducted in sequence. And a cohort of fresh fish were used in each experiment. No fish died during the three experiments.
Experiment I: Effects of social enrichment on fish growth performance
As mentioned above, in this study we constructed social enrichment by introducing two fish species into the same rearing environment. The two territorial fish species that we focused on have similar behavioral performance, which makes it possible to rear them in the same tanks on real fish farms. In brief, 45 rockfish juveniles (total length 15.1 ± 0.1 cm, body weight 58.6 ± 1.6 g, mean ± S.E.) and 45 greenling juveniles (total length 16.8 ± 0.1 cm, body weight 52.5 ± 1.1 g, mean ± S.E.) were divided into 15 aquaria, in groups of 6, in 5 triplicated groups (average rearing density ~ 2.78 kg/m3). The 5 experimental groups were as follows: 6 rockfish per aquarium, 5 rockfish and 1 greenling per aquarium, 3 rockfish and 3 greenling per aquarium, 1 rockfish and 5 greenling per aquarium, and 6 greenling per aquarium (hereafter, we simply referred to the groups as R6, R5:G1, R3:G3, R1:G5, and G6; Figure 1). Considering that the practical rearing density of these two fish species in fish farms (~ 2.5-3 kg/m3) and that the number of fish allowing us to accurately quantify fish behaviors, we introduced 6 fish individuals into each aquarium. To create a medium-level physical enrichment scenario, we introduced six identical physical structures (material: PVC, color: grey, shape: pyramid, height: approximately 10 cm, basal area: approximately 72 cm2) into each aquarium. From the practical point of view, the structures were put in the center of the aquarium bottom, and this layout can increase the utilization efficiency of the water area and meanwhile benefit daily management. Considering that each aquarium included 6 fish and each fish approximately occupied 2 structures, introducing 6 physical structures (equivalently medium-level enrichment) was deemed to be sufficient for fish to occupy the structures as well as to support free swimming in the aquarium.
Fish were maintained in experimental environments for one month. During this period, fish were fed with commercial floating dry pellets (Kaido Brand, Santong Bioengineering Co. Ltd., Anqiu City, China) to satiation by hand thrice daily (09:00 a.m., 2:00 p.m., and 5:00 p.m.). Feces and residual fodders were siphoned once daily before the first feeding. The adjacent sides of the aquaria were masked with white plastic boards to ensure visual separation among fish. All the aquaria and physical structures were gently cleaned once weekly. No fish died during the whole experimental period. After the rearing period, all fish were euthanized with an overdose of anesthetic (Tricaine methanesulfonate, MS-222, 200 mg/L), and then their body weights (precision 0.01 g) and total lengths (precision 0.01 cm) were recorded.
Experiment II: Effects of social enrichment on fish aggression and group distribution
The second experiment was conducted to explore the enrichment effects on the individual and group behaviors of fish (Figure 1). Forty-five rockfish juveniles (total length 14.4 ± 0.6 cm, body weight 52.1 ± 1.1 g, mean ± S.E.) and 45 greenling juveniles (total length 17.0 ± 0.5 cm, body weight 53.1 ± 1.2 g, mean ± S.E.) were used in this experiment. The average rearing density was approximately 2.63 kg/m3. The treatment groups and the other experimental conditions were all identical to Experiment I. The fish were maintained under experimental conditions for one week.
In this study, we mainly focused on fish aggressive behavior and group distribution. During the one-week rearing period, fish behaviors were recorded on days 1, 3, 5, and 7 for each aquarium using a digital camera (HDR-AS100V, Sony Corporation, Tokyo, Japan). For each day, a 10-min video was randomly filmed between 11:00 a.m. and 2:00 p.m. from the top side of each aquarium. And the distance between the aquarium and camera was approximately 50 cm. Aggressive behavior was further classified into intraspecies aggression and interspecies aggression and was quantified by counting the total amounts of chasing, nipping, and biting per fish during 10 mins (Zhang et al., 2020a; Zhang et al., 2020b; Zhang et al., 2021a; Zhang et al., 2021c). To quantify the group distribution of fish, we divided the aquarium bottom surface into three areas by two circles whose diameters were 30 cm and 40 cm, respectively. From the interior to the exterior, the three areas were defined as central area, transition area, and boundary area, respectively. The six physical structures were all located in the central area. As mentioned above, we filmed one 10-min video (during 11:00 a.m. ~ 2:00 p.m. for all aquaria) per aquarium per recording day. As we filmed four days during the experimental week and set up three aquaria per treatment group, we totally got 12 10-min video clips per treatment. To calculate group distribution, we took one screenshot every minute to get 120 pictures for each treatment. Considering fish’s somatic length, we regarded the location of the fish head as the area of the whole fish. The group distribution was calculated as the number of fish distributed in each area. The behavior data were analyzed by one person who was blind to the experimental design (i.e., Yiqiu Fu).
Experiment III: The metabolic difference between the two territorial fishes
This experiment was conducted to compare the difference in standard metabolic rate between rockfish and greenling (Figure 1). Twenty fish per species (rockfish: total length 12.0 ± 0.3 cm, body weight 26.5 ± 0.6 g; greenling: total length 14.1 ± 0.3 cm, body weight 27.3 ± 0.8 g; mean ± S.E.) were used in this experiment and all fish fasted for one day before the formal experiment. Then, each fish was introduced into a continuous-flow respirometer to measure the standard metabolic rate. The experimental apparatus and procedure followed the classical method which was repeatedly evidenced to be valid in several studies (Xie and Sun, 1990; Fu et al., 2005; Fu et al., 2021; Shen et al., 2021). Briefly, the main components of the apparatus included a big glass aquarium (60 cm × 50 cm × 50 cm), 11 parallel respiratory chambers (length 15 cm, diameter 7 cm), 3 heating rods, 2 air stones, and an oxygen meter. The aquarium was part of an indoor flow-through natural seawater system equipped with mechanical filters. The water flow rate was 3 L/min and the water depth was maintained at 40 cm. The heating rods were used to control the temperature (19°C). And the air stones were used to maintain high dissolved oxygen content (~ 7 mg/L). Each respiratory chamber was equipped with a water pump (2.5W, MY-018, Foshan Nanhai Mingyi Aquarium Equipment Factory, Foshan City, China) to maintain proper velocity of water flow. The apparatus consisted of 11 parallel respiratory chambers, one of which did not contain fish and was used as a control chamber. The whole apparatus was covered by opaque sheets to eliminate the disturbances from the surrounding environment and ensure visual separation among fish. The fish were introduced into the respiratory chambers at 20:00 on the last day and were given one night to recover from possible handling stress. Then, the dissolved oxygen contents of water outlets (S9-Field kit, Mettler-Toledo, Zurich, Switzerland) were measured at 8:00, 10:00, 12:00, 14:00, 16:00, 18:00, and 20:00 on the next day, and the average value of the seven measurements was used to calculate the standard metabolic rate of the experimental fish. The water flow rate was obtained by measuring the flow volume at the outlet of the respiration chamber for one minute each time. To avoid the potential physiological stress, the flow rate in each chamber was adjusted to ensure that the outlet water had a minimum saturation of 70% dissolved oxygen (Fu et al., 2005).
Calculations and data analyses
The following parameters were adopted to evaluate fish growth performance:
weight gain (g)=BWf−BWi
length gain (cm)=TLf−TLi
where BWi (g) = mean initial body weight, BWf (g) = mean final body weight, TLi (cm) = mean initial total length, TLf (cm) = mean final total length, and T (d) = days of captive period.
For group distribution, we calculated the distribution proportion to assess the group distribution of fish:
where NT = number of fish distributed in the target area (i.e., central area, transition area, or boundary area), and NF = total number of fish introduced into the aquarium.
For standard metabolic rate, we adopted the formula proposed by Xie and Sun (1990) and Fu et al. (2005):
where △O2 (mg/L) = dissolved oxygen content difference between respiration chamber and control respiration chamber, V (L/h) = flow velocity in the respiration chamber, and M (kg) = body weight of the experimental fish.
For growth performance, we were primarily interested in the differences among treatments within the same species. Therefore, a general linear model was applied to compare treatment differences in each growth parameter, and then Tukey’s honestly significant difference post hoc test for multiple comparisons of means from these ANOVA model fits was further applied (Hothorn et al., 2008). The normality and homogeneity of the data were tested before ANOVA (Bartlett, 1937; Royston, 1982). Because the aggressive behavior is count data, generalized linear models with Poisson error structure and log link function were adopted to evaluate the overall differences in inter- and intra-species aggression, then Tukey-corrected post hoc multiple comparisons were conducted (Fox, 2015; Lenth et al., 2018). The homogeneity of data was tested by fitting the residuals and fitted values using a general linear model. The overdispersion of data was also tested (Lüdecke et al., 2021). Because the distribution proportion ranges from 0 to 1, we performed a generalized linear model with binomial error structure and logit link function to test the distribution difference between the two fish species (Fox, 2015). By changing the model structure to a quasi-binomial family and comparing the model results between the two models above (i.e., binomial and quasi-binomial), we could determine whether data presented overdispersion. For standard metabolic rate, we performed a general linear model with fish species and the body weight of each fish as fixed factors to compare the metabolic difference between the two fishes. Similarly, the normality and homogeneity of data were detected before constructing the ANOVA models. All statistical analyses were done in R software (version 4.0.2, R Core Team, 2020). Differences were considered significant at P < 0.05.
Results and discussion
Experiment I: Effects of social enrichment on fish growth performance
For rockfish, introducing greenling as a social stimulus significantly decreased the growth performance of rockfish, regardless of the enrichment amount (i.e., the number of greenling) (Figure 2). In sharp contrast, introducing rockfish as a stimulus significantly increased the growth performance of greenling, especially the medium (i.e., R3:G3 group) and high (i.e., R5:G1) enrichment amounts (Figure 2).
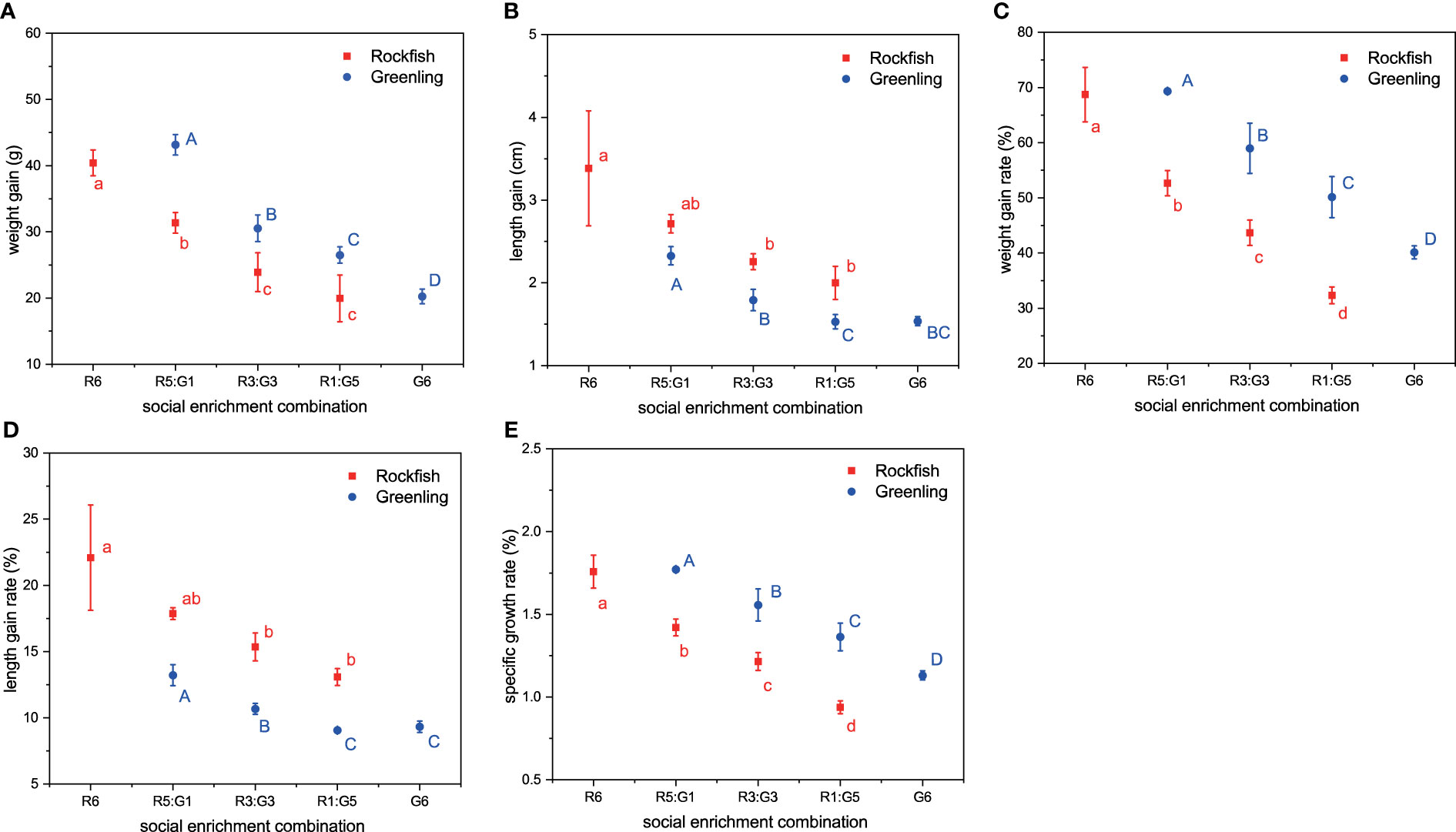
Figure 2 The growth performance of black rockfish and fat greenling from different social enrichment combinations after one-month rearing. (A) weight gain, (B) length gain, (C) weight gain rate, (D) length gain rate, (E) specific growth rate. Different red, small letters indicate significant differences among social enrichment combinations within black rockfish, and different blue, capital letters indicate significant differences among social enrichment combinations within fat greenling. R6 means 6 rockfish per aquarium, R5:G1 means 5 rockfish and 1 greenling per aquarium, R3:G3 means 3 rockfish and 3 greenling per aquarium, R1:G5 means 1 rockfish and 5 greenling per aquarium, and G6 means 6 greenling per aquarium. n = 3. Data are presented as means ± S.D.
Although there are many existing studies about polyculture of different species in ponds (Milstein, 1992; Martínez Porchas et al., 2010; Wang and Lu, 2016), it has not been discussed under the concept of social enrichment. As mentioned above, the two species have relatively similar ecological habits, inhabit similar habitats which often include some rocks and sea grasses, and are often observed to coexist in the wild (Zhang et al., 2021a). Before conducting this experiment, we predicted that polyculture of the two species might benefit both of their growth because this social enrichment meets well with their instinct (Johnsson et al., 2014). Contrary to our prediction, only greenling showed improved growth performance when they were exposed to social enrichment. Even though we did not get positive growth results for rockfish, from the perspective of greenling, our results still have important meaning for practical applications in aquaculture. If fish farmers can tolerate a certain degree of growth depression of rockfish, it is a promising way to improve greenling growth by introducing a small proportion of rockfish into greenling aquaria as a stimulus. Furthermore, the fish species that serve as a stimulus may be an important moderator for enrichment effects. Although we failed to find an ideal combination that benefits the growth of both species, our results showed valuable potential for increasing fish growth by social enrichment, and it is interesting for future studies to further explore optimal fish species combinations. For potential fish combinations, from the perspective of practical aquaculture, we propose following four aspects for consideration in further studies. Firstly, the target fish should inhabit similar areas in the wild. This reflects the long-term adaptation of fish to surrounding environments and is one of the fundamentals of high nature-based welfare. Secondly, the cultured conditions (e.g., feeding strategy, photoperiod, water temperature) of the target fish should be similar, or at least close to each other. Thirdly, the target fish can be produced on a commercial scale. Finally, physical enrichment (i.e., introducing physical structures and other objects to increase environmental complexity and heterogeneity of rearing water) can be considered to combine with social enrichment to get ideal results.
Experiment II: Effects of social enrichment on fish aggression and group distribution
Why did greenling show improved growth while rockfish showed depressed growth when they were exposed to social enrichment? To solve this question, we monitored the aggressive behaviors and group distributions of the two fishes for one week. Our results showed that introducing 3 greenling as a stimulus significantly decreased the intraspecies aggression among rockfish (Z = -2.439, P = 0.0391; Figure 3A), however introducing 1 greenling did not significantly affect rockfish intraspecies aggression (Z = -1.578, P = 0.2552; Figure 3A). Similarly, introducing 3 rockfish as a stimulus significantly decreased the intraspecies aggression among greenling (Z = -3.667, P = 0.0007; Figure 3A), but introducing 1 rockfish did not significantly affect greenling intraspecies aggression (Z = -1.019, P = 0.5648; Figure 3A).
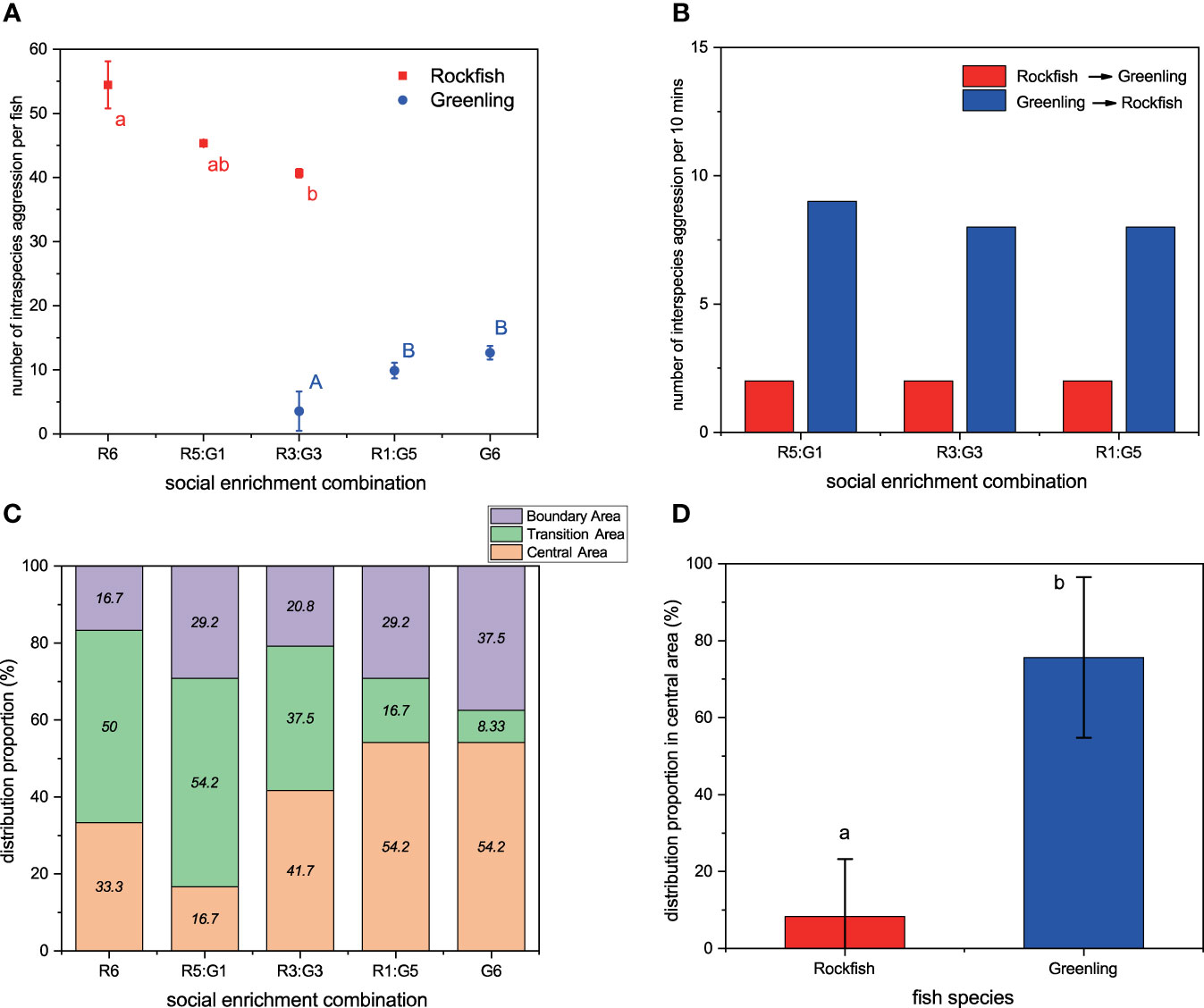
Figure 3 The aggressive behavior and group distribution of black rockfish and fat greenling from different social enrichment combinations during one-week rearing. (A) intraspecies aggression (n = 3), (B) interspecies aggression (n = 3), (C) distribution proportion in three areas (n = 120), (D) distribution proportion in the central area (n = 120). For plot (A), different red, small letters indicate significant differences among social enrichment combinations within black rockfish, and different blue, capital letters indicate significant differences among social enrichment combinations within fat greenling. For plots (A), (B) and (C), R6 means 6 rockfish per aquarium, R5:G1 means 5 rockfish and 1 greenling per aquarium, R3:G3 means 3 rockfish and 3 greenling per aquarium, R1:G5 means 1 rockfish and 5 greenling per aquarium, and G6 means 6 greenling per aquarium. For plot (B), ‘Rockfish points to Greenling’ means the frequency of aggression from black rockfish to fat greenling, and ‘Greenling points to Rockfish’ means opposite context. For plot (D), different letters indicate significant differences between the two fish species. Data are presented as means ± S.D.
Considering aggressive behavior is often related to physiological stress, body damage, and disease resistance (Wendelaar Bonga, 1997; Näslund and Johnsson, 2014), and is an important analog to fish welfare (Martins et al., 2012; Kristiansen et al., 2020), these results suggest improved effects of the specific amount of social enrichment (i.e., R3:G3) on fish welfare. From the perspectives of practical production and fish welfare, we suggested fish farmers conduct social enrichment, considering enrichment could improve growth and decrease aggression of greenling and could decrease aggression of rockfish. Previous studies showed that aggression is negatively associated with fish growth (Gilmour et al., 2005; Batzina and Karakatsouli, 2012; Näslund and Johnsson, 2014; Gerber et al., 2015), therefore it is reasonable to observe that social enrichment enhanced greenling growth in Experiment I.
Although the decreased intraspecies aggression among rockfish was observed in Experiment II, this behavioral superiority did not transfer into growth improvement. This phenomenon is somewhat strange. Therefore, we further counted the frequency of interspecies aggression. The result showed that during the experimental period, although the severity was lower compared with intraspecies aggression, greenling expressed significantly more aggressive behavior for rockfish than the aggression launched by rockfish (χ2 = 12.513, df = 1, P = 0.0004; Figure 3B), and this trend was highly consistent among three social enrichment combinations. This result powerfully indicates that the depressed growth effects of social enrichment on rockfish may be caused by higher interspecies aggression launched by greenling, rather than intraspecies aggression among rockfish.
Then we considered why greenling expressed higher interspecies aggression for rockfish than reversing. So, we monitored the group distribution of the two species. The results showed that with the increase in the proportion of greenling, the distribution proportion in the central area overall increased steadily (Figure 3C). Furthermore, the average distribution proportion in the central area of greenling was significantly higher than that of rockfish (χ2 = 18.779, df = 1, P < 0.0001; Figure 3D). These results indicate that the greenling has stronger taxis for reefs (i.e., physical structures) compared with rockfish, so they spend much time and energy competing for the limited resource (here, physical structures) and express more interspecies aggression toward rockfish. This inference was in accordance with previous results and our personal observations (Zhang et al., 2021a).
For the behavior data collecting method, we should particularly note one point here. In this study, we monitored fish behavior only on days 1, 3, 5, and 7 for each aquarium. For each day, only one 10-min video was randomly filmed between 11:00 a.m. and 2:00 p.m. Although it is commonly adopted by related studies (Lee and Berejikian, 2009; Barley and Coleman, 2010; Batzina and Karakatsouli, 2012; Bilhete and Grant, 2016; Zhang et al., 2020a), this method may still cause some bias in the intensity of fish behaviors because fish activity has a specific rhythm and is meanwhile affected by environmental factors (e.g., feeding). To weaken the effects of diel rhythm and environmental disturbance, we collected behavior data in a completely random order for aquaria during intervals of feeding. This protocol permits to evaluate fish behavior with less labor and time, yet the doubt about its accuracy and representation is kept. To get more robust results, future studies may monitor fish behaviors continuously, at least select several representative periods per day.
Experiment III: The metabolic difference between the two territorial fishes
Why did greenling express higher aggressiveness and stronger taxis for reefs than rockfish? We answered this question at the metabolism level. Our results showed that greenling had significantly higher standard metabolic rate than rockfish (F1,36 = 1090.835, P < 0.0001; Figure 4), although the significant effect of body weight (F1,36 = 20.8055, P < 0.0001) and interaction effect (F1,36 = 6.3684, P < 0.0162) were also detected. It is well recognized that a higher standard metabolic rate is related to higher aggressive frequency, stronger competitive ability, and higher energy consumption (Sloman et al., 2000; McCarthy, 2001; Sloman and Armstrong, 2002; Millidine et al., 2009). Therefore, it is reasonable that greenling showed higher interspecies aggression toward rockfish and occupied more shelters compared with rockfish. Based on our careful personal observations, in the practical production, the greenling should be fed at least twice daily, otherwise they would express severe aggression and body damage. But this phenomenon is not obvious in rockfish. We think that higher aggressiveness, quicker energy consumption, and higher food requirement may well be the results of a higher standard metabolic rate, and these behavioral-physiological processes further cause more severe aggression and body damage. For the same reason, we could partly explain the growth difference between rockfish and greenling from the ecological and evolutional points of view. Because greenling had higher competitive ability and stronger taxis for reefs, they nearly inevitably occupied more shelters, and consequently, rockfish have to inhabit the open area (Näslund and Johnsson, 2014; Zhang et al., 2021a). Although rockfish have lower taxis for reefs, they still need some shelters inherently (Zhang et al., 2019), and this discrepant situation may cause higher physiological stress therefore suppress their growth when they were exposed to social enrichment. The above interpretations seem logical and reasonable, but our experiments cannot confirm these relations. The biggest problem is that the fish individuals used in the three experiments were not the same, so we cannot infer the correlation or causality by statistical methods. Even so, the similar trends between growth, aggression, and metabolism in this study still suggest that a close regulatory relation between metabolism and behavior may exist. Further studies should design experiments to verify this point.
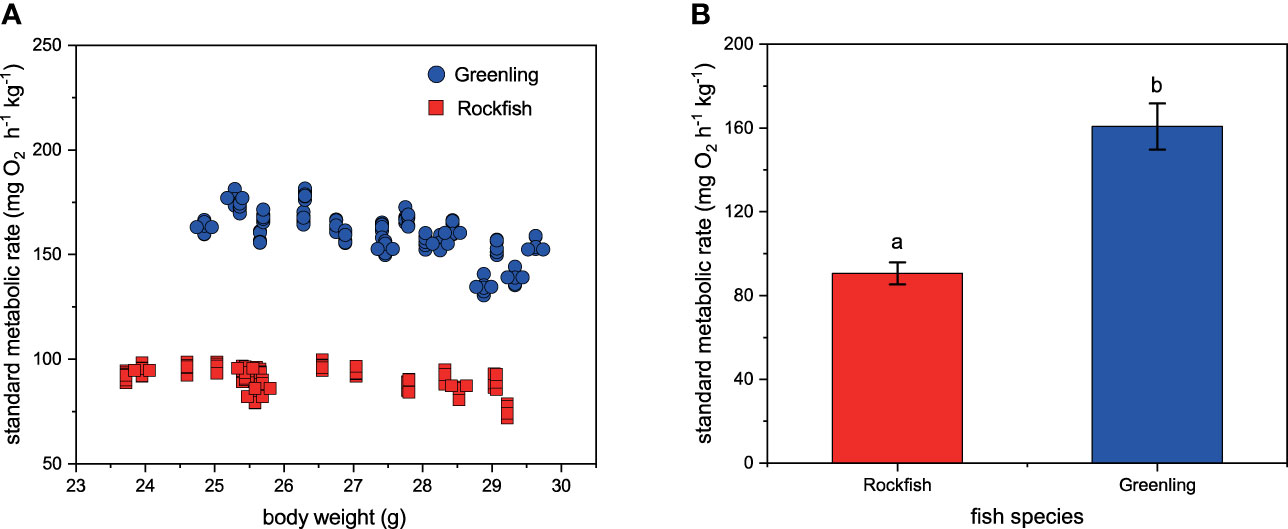
Figure 4 The standard metabolic rate (mg O2 h-1 kg-1) of black rockfish and fat greenling. Plot (A) presented the changes in standard metabolic rate along with the changes in fish body weight. Plot (B) presented the metabolic difference between the two fish species, and different letters indicate significant differences. n = 20. Data are presented as means ± S.D.
Moreover, we recorded two interesting behavioral differences between rockfish and greenling, based on our personal observation. Firstly, although both rockfish and greenling in typical farms often inhibit the bottom of the tank and are habituated to wait for food pellets sinking to the bottom, the moving speed of greenling is significantly faster than rockfish. This overlap of feeding space may cause a certain suppression of the feeding activity of rockfish. Secondly, greenling is more neurotic than rockfish. A large movement range of greenling especially when they are startled or competing for foods will cause a chaos of rockfish population (and maybe individual psychology). Based on these observations, we speculate that overhead shelter may be an efficient method to minimize the compromised effects of social enrichment on rockfish growth, considering shelters can reduce light intensity and surrounding disturbance. In fact, the improved effects of shelter on the growth and physiology have been demonstrated on many fish species (e.g., Hossain et al., 1998; Walker et al., 2016), therefore future research should explore the application potential of shelter on the rockfish and greenling aquaculture.
There is one point we should particularly note, that is, the possibility of fish size as a factor to affect the growth, behavioral and metabolic outcomes in this study. Before conducting the formal experiment, we tried to control fish size and used similar-size fish to conduct the three experiments. However, the size variation between fish species (and individuals) is inevitable. In experiment I, although rockfish had lower total length, they had higher body weight than greenling. The trade-off between length and weight may weaken the effect of body size on the growth outcomes. In experiment II, although rockfish seem to have lower total length, they were very close in body weight between the two species. Therefore, the difference in aggression between species may be mainly attributed to their natural instincts rather than body size. In experiment III, although we detected significant main effects of body weight and interaction effects of body weight and fish species on the standard metabolic rate, we do not think that body weight effect changes our main conclusions. Figure 4A clearly showed that within the scope of body weight, the metabolisms of all greenling individuals were higher than those of rockfish. The statistical result of significant interaction effects has very limited biological meanings in this study (i.e., in the context of juveniles). In this sense, although we detected significant body size (main and interaction) effects on the metabolism, we can still conclude that within the scope of body weight in this study, greenling had significantly higher standard metabolic rate than rockfish.
Conclusions
In conclusion, our present results showed that introducing rockfish as a stimulus significantly increased the growth performance of greenling, especially for medium and high enrichment amounts. Proper social enrichment significantly decreased the intraspecies aggression both among rockfish and among greenling. Moreover, greenling expressed significantly more aggressive behavior toward rockfish than the reverse. Finally, the average distribution proportion in central areas and standard metabolic rate of greenling were significantly higher than those of rockfish. These results clearly indicate that (1) greenling have a higher metabolic requirement and stronger taxis for reefs, so they express more interspecies aggression toward rockfish, consequently suppress the growth of rockfish; and (2) proper social enrichment is an effective method to decrease fish aggression and increase greenling growth, in other words, improve greenling’s welfare, but this improvement is accompanied by compromised rockfish’s welfare. This study will promote the application of environmental enrichment strategy on improving fish welfare and provide fundamental information for reducing fish aggression and improving fish growth in aquaculture. Based on the present results, we recommend that future research design their experiments focusing on the following three aspects: (1) the optimal fish combinations for social enrichment, (2) the interaction effects of physical and social enrichment, and (3) the application potential for weakening adverse effects of social enrichment on specific fish species with overwater shelters.
Date availability statement
The raw data supporting the conclusions of this article will be made available by the authors, without undue reservation.
Ethics statement
This study was reviewed and approved by the Institutional Animal Care and Use Committee of the Ocean University of China (20180101).
Author contributions
ZZ wrote the first draft of the manuscript and performed the statistical analysis. ZZ and YF conducted the experiments and contributed to the conception and design of the study. HZ contributed to the revisions of discussion, figures, and language. XZ contributed to the figures, discussed the results, revised the paper and acquired the research funding. All authors contributed to the article and approved the submitted version.
Funding
This work was supported by funds from the National Natural Science Foundation of China (32072966) and the Province Key Research and Development Program of Zhejiang (2021C02047).
Conflict of interest
The authors declare that the research was conducted in the absence of any commercial or financial relationships that could be construed as a potential conflict of interest.
Publisher’s note
All claims expressed in this article are solely those of the authors and do not necessarily represent those of their affiliated organizations, or those of the publisher, the editors and the reviewers. Any product that may be evaluated in this article, or claim that may be made by its manufacturer, is not guaranteed or endorsed by the publisher.
References
Arechavala-Lopez P., Cabrera-Álvarez M. J., Maia C. M., Saraiva J. L. (2022). Environmental enrichment in fish aquaculture: A review of fundamental and practical aspects. Rev. Aquacult. 14, 704–728. doi: 10.1111/raq.12620
Ashley P. J. (2007). Fish welfare: current issues in aquaculture. Appl. Anim. Behav. Sci. 104, 199–235. doi: 10.1016/j.applanim.2006.09.001
Barley A. J., Coleman R. M. (2010). Habitat structure directly affects aggression in convict cichlids Archocentrus nigrofasciatus. Curr. Zool. 56 (1), 52–56. doi: 10.1093/czoolo/56.1.52
Barreto M. O., Rey Planellas S., Yang Y., Phillips C., Descovich K. (2022). Emerging indicators of fish welfare in aquaculture. Rev. Aquacult. 14, 343–361. doi: 10.1111/raq.12601
Bartlett M. S. (1937). Properties of sufficiency and statistical tests. P. R. Soc A. 160, 268–282. doi: 10.1098/rspa.1937.0109
Batzina A., Karakatsouli N. (2012). The presence of substrate as a means of environmental enrichment in intensively reared gilthead seabream Sparus aurata: growth and behavioral effects. Aquaculture. 370–371. doi: 10.1016/j.aquaculture.2012.10.005
Bilhete C., Grant J. W. A. (2016). Short-term costs and benefits of habitat complexity for a territorial fish. Ethology 122 (2), 151–157. doi: 10.1111/eth.12456
Bloomsmith M. A., Brent L. Y., Schapiro S. J. (1991). Guidelines for developing and managing an environmental enrichment program for nonhuman primates. Lab. Anim. Sci. 41, 372–377.
Broom D. M. (1991). Animal welfare: concepts and measurement. J. Anim. Sci. 69, 4167–4175. doi: 10.2527/1991.69104167x
Fox J. (2015). Applied regression analysis and generalized linear models (Thousand Oaks: Sage Publications).
Fu S., Xie X., Cao Z. (2005). Effect of fasting and repeat feeding on metabolic rate in southern catfish, Silurus meridionalis Chen. Mar. Freshw. Behav. Phy. 38, 191–198. doi: 10.1080/10236240500231532
Fu Y., Zhang Z., Zhang Z., Shen F., Xu X., Li Z., et al. (2021). Boldness predicts aggressiveness, metabolism and activity in black rockfish Sebastes schlegelii. Front. Mar. Sci. 8. doi: 10.3389/fmars.2021.770180
Galhardo L., Oliveira R. F. (2009). Psychological stress and welfare in fish. Annu. Rev. Biomed. Sci. 11, 1–20. doi: 10.5016/1806-8774.2009v11p1
Gerber B., Stamer A., Stadtlander T. (2015). Environmental enrichment and its effects on welfare in fish (Frick: FiBL).
Gilmour K. M., Dibattista J. D., Thomas J. B. (2005). Physiological causes and consequences of social status in salmonid fish. Integr. Comp. Biol. 45, 263–273. doi: 10.1093/icb/45.2.263
Hossain M. A., Beveridge M. C., Haylor G. S. (1998). The effects of density, light and shelter on the growth and survival of African catfish (Clarias gariepinus burchell 1822) fingerlings. Aquaculture 160, 251–258. doi: 10.1016/S0044-8486(97)00250-0
Hothorn T., Bretz F., Westfall P. (2008). Simultaneous inference in general parametric models. Biometrical J. 50, 346–363. doi: 10.1002/bimj.200810425
Huntingford F. A., Adams C., Braithwaite V. A., Kadri S., Pottinger T. G., Sandøe P., et al. (2006). Current issues in fish welfare. J. Fish Biol. 68, 332–372. doi: 10.1111/j.0022-1112.2006.001046.x
Hu F., Pan L., Gao F., Jian Y., Wang X., Li L., et al. (2017). Effect of temperature on incubation period and hatching success of fat greenling (Hexagrammos otakii, Jordan & starks) eggs. Aquacult. Res. 48, 361–365. doi: 10.1111/are.12853
Johnsson J. I., Brockmark S., Näslund J. (2014). Environmental effects on behavioural development consequences for fitness of captive-reared fishes in the wild. J. Fish Biol. 85, 1946–1971. doi: 10.1111/jfb.12547
Johnsson J. I., Näslund J. (2018). Studying behavioural variation in salmonids from an ecological perspective: Observations questions methodological considerations. Rev. Fish. Biol. Fish. 28, 795–823. doi: 10.1007/s11160-018-9532-3
Jones N. A., Webster M. M., Salvanes A. G. V. (2021). Physical enrichment research for captive fish: Time to focus on the DETAILS. J. Fish Biol. 99, 704–725. doi: 10.1111/jfb.14773
Kristiansen T. S., Fernö A., Pavlidis M. A., van de Vis H. (2020). The welfare of fish (Cham: Springer).
Lee J. S., Berejikian B. A. (2009). Structural complexity in relation to the habitat preferences, territoriality, and hatchery rearing of juvenile China rockfish (Sebastes nebulosus). Environ. Biol. Fish. 84 (4), 411–419. doi: 10.1007/s10641-009-9450-2
Lenth R., Singmann H., Love J., Buerkner P., Herve M. (2018). Emmeans: Estimated marginal means, aka least-squares means. R Package version 1, 3.
Lüdecke D., Ben-Shachar M. S., Patil I., Waggoner P., Makowski D. (2021). Performance: An r package for assessment, comparison and testing of statistical models. J. Open Source Software 16, 3139. doi: 10.21105/joss.03139
Martínez Porchas M., Martínez Córdova L. R., Porchas Cornejo M. A., López Elías J. A. (2010). Shrimp polyculture: a potentially profitable, sustainable, but uncommon aquacultural practice. Rev. Aquacult. 2, 73–85. doi: 10.1111/j.1753-5131.2010.01023.x
Martins C. I., Galhardo L., Noble C., Damsgård B., Spedicato M. T., Zupa W., et al. (2012). Behavioural indicators of welfare in farmed fish. Fish Physiol. Biochem. 38, 17–41. doi: 10.1007/s10695-011-9518-8
McCarthy I. D. (2001). Competitive ability is related to metabolic asymmetry in juvenile rainbow trout. J. Fish Biol. 59, 1002–1014. doi: 10.1111/j.1095-8649.2001.tb00167.x
Millidine K. J., Armstrong J. D., Metcalfe N. B. (2009). Juvenile salmon with high standard metabolic rates have higher energy costs but can process meals faster. P. R. Soc B. 276, 2103–2108. doi: 10.1098/rspb.2009.0080
Milstein A. (1992). Ecological aspects of fish species interactions in polyculture ponds. Hydrobiologia 231, 177–186. doi: 10.1007/BF00018201
Mommsen T. P., Vijayan M. M., Moon T. W. (1999). Cortisol in teleosts: dynamics, mechanisms of action, and metabolic regulation. Rev. Fish. Biol. Fish. 9, 211–268. doi: 10.1023/A:100892441872
Näslund J., Johnsson J. I. (2014). Environmental enrichment for fish in captive environments: effects of physical structures and substrates. Fish. Fish. 17, 1–30. doi: 10.1111/faf.12088
R Core Team (2020). R: A language and environment for statistical computing (Windsor, Wisconsin, USA: R Foundation for Statistical Computing).
Royston J. P. (1982). An extension of Shapiro and wilk's W test for normality to large samples. J. R. Stat. Soc C. 31, 115–124. doi: 10.2307/2347973
Shen F., Zhang Z., Fu Y., Zhang Z., Sun X., Dong J., et al. (2021). Effects of food deprivation duration on the behavior and metabolism of black rockfish (Sebastes schlegelii). Fishes 6, 58. doi: 10.3390/fishes6040058
Sloman K. A., Armstrong J. D. (2002). Physiological effects of dominance hierarchies: laboratory artefacts or natural phenomena? J. Fish Biol. 61, 1–23. doi: 10.1111/j.1095-8649.2002.tb01733.x
Sloman K. A., Motherwell G., O'Connor K., Taylor A. C. (2000). The effect of social stress on the standard metabolic rate (SMR) of brown trout, Salmo trutta. Fish Physiol. Biochem. 23, 49–53. doi: 10.1023/A:1007855100185
Stevens C. H., Croft D. P., Paull G. C., Tyler C. R. (2017). Stress and welfare in ornamental fishes: what can be learned from aquaculture? J. Fish Biol. 91, 409–428. doi: 10.1111/jfb.13377
Walker L. M., Parker T. M., Barnes M. E. (2016). Full and partial overhead tank cover improves rainbow trout rearing performance. N. Am. J. Aquacult. 78, 20–24. doi: 10.1080/15222055.2015.1090504
Wang M., Lu M. (2016). Tilapia polyculture: a global review. Aquac. Res. 47, 2363–2374. doi: 10.1111/are.12708
Wendelaar Bonga S. E. (1997). The stress response in fish. Physiol. Rev. 77, 591–625. doi: 10.1152/physrev.1997.77.3.591
Xie X., Sun R. (1990). The bioenergetics of the southern catfish (Silurus meridionalis chen). i. resting metabolic rate as a function of body weight and temperature. Physiol. Zool. 63, 1181–1195. doi: 10.1086/physzool.63.6.30152639
Young R. J., de Azevedo C. S., Cipreste C. F. (2020). “Environmental enrichment: the creation of opportunities for informal learning,” in Zoo animal learning and training. Eds. Melfi V. A., Dorey N. R., Ward S. J. (Hoboken, New Jersey, USA: Wiley Online Library), 101–118.
Zhang Z., Bai Q., Xu X., Guo H., Zhang X. (2020a). Effects of environmental enrichment on the welfare of juvenile black rockfish Sebastes schlegelii: Growth, behavior and physiology. Aquaculture 518, 734782. doi: 10.1016/j.aquaculture.2019.734782
Zhang Z., Fu Y., Guo H., Zhang X. (2021b). Effect of environmental enrichment on the stress response of juvenile black rockfish Sebastes schlegelii. Aquaculture 533, 736088. doi: 10.1016/j.aquaculture.2020.736088
Zhang Z., Fu Y., Shen F., Zhang Z., Guo H., Zhang X. (2021c). Barren environment damages cognitive abilities in fish: Behavioral and transcriptome mechanisms. Sci. Total Environ. 794, 148805. doi: 10.1016/j.scitotenv.2021.148805
Zhang Z., Fu Y., Zhang Z., Zhang X., Chen S. (2021a). A comparative study on two territorial fishes: the influence of physical enrichment on aggressive behavior. Animals. 11, 1868. doi: 10.3390/ani11071868
Zhang Z., Gao L., Zhang X. (2022). Environmental enrichment increases aquatic animal welfare: A systematic review and meta-analysis. Rev. Aquacult. 14, 1120–1135. doi: 10.1111/raq.12641
Zhang Z., Xu X., Wang Y., Zhang X. (2020b). Effects of environmental enrichment on growth performance, aggressive behavior and stress-induced changes in cortisol release and neurogenesis of black rockfish Sebastes schlegelii. Aquaculture 528, 735483. doi: 10.1016/j.aquaculture.2020.735483
Keywords: environmental enrichment, growth, aggression, Sebastes schlegelii, Hexagrammos otakii
Citation: Zhang Z, Fu Y, Zhao H and Zhang X (2022) Social enrichment affects fish growth and aggression depending on fish species: Applications for aquaculture. Front. Mar. Sci. 9:1011780. doi: 10.3389/fmars.2022.1011780
Received: 04 August 2022; Accepted: 10 October 2022;
Published: 25 October 2022.
Edited by:
Manuel Gesto, Technical University of Denmark, DenmarkReviewed by:
Sébastien Alfonso, COISPA Tecnologia and Ricerca, ItalyAnssi Vainikka, University of Eastern Finland, Finland
Copyright © 2022 Zhang, Fu, Zhao and Zhang. This is an open-access article distributed under the terms of the Creative Commons Attribution License (CC BY). The use, distribution or reproduction in other forums is permitted, provided the original author(s) and the copyright owner(s) are credited and that the original publication in this journal is cited, in accordance with accepted academic practice. No use, distribution or reproduction is permitted which does not comply with these terms.
*Correspondence: Xiumei Zhang, xiumei1227@163.com