- Tianjin Key Laboratory of Aqua-ecology and Aquaculture, College of Fisheries, Tianjin Agricultural University, Tianjin, China
Ocean acidification (OA) can have widespread implications for marine bivalves. While our current understanding of OA effects on the physiological performance is increasing, very little is known about the physiology of digestive gland of marine bivalves in response to OA. Here, we examined how the digestive system of razor clams (Sinonovacula constricta) responded to OA. Following 35-day exposure to CO2-driven seawater acidification, no significant decreases in phenotypic traits, such as dry body weight gain, specific growth rate, condition index and survival rate, as well physiological functions, such as activities of antioxidant and digestive enzymes, were observed, demonstrating the resistance of razor clams under acidified conditions. Histological results showed that some direct damages on the structure of digestive gland was observed, including degradation of digestive tubular, atrophy of epithelial cells, loose cell arrangement, even diffuse. This study provides insights into the digestive performance of marine bivalves in a rapidly acidifying ocean.
Introduction
The stress of marine environmental variation will affect the physiological response, stress tolerance, and adaptation potential of marine organisms (Dong et al., 2019; Liao et al., 2022; Xu et al., 2022), especially the increasingly severe ocean acidification. Ocean acidification (OA), the continuing decline in global ocean pH, is one of the most significant consequences of rapid increases in anthropogenic carbon dioxide (CO2) emissions since the industrial Revolution (Intergovernmental Panel on Climate Change [IPCC], 2014). The pH of surface ocean has dropped 0.1 units over the past 200 years and is projected to decrease 0.3–0.5 units by the end of this century (Intergovernmental Panel on Climate Change [IPCC], 2014). Considering the unprecedented occurrence rate of OA, whether marine organisms can keep up with and adapt to rapid acidifying ocean has attracted widespread attention (Zhao et al., 2020; Zhang et al., 2022). Many acute and short-term exposure experiment have demonstrated that lowered seawater pH can have widespread impacts on the growth, survival, and essential physiological processes, such as shell formation, metamorphosis, cellular acid-base homeostasis, biomineralization, physiological energetic, immunity, development, and reproduction of marine invertebrates (e.g., Kurihara, 2008; Talmage and Gobler, 2009; Zittier et al., 2015; Figueiredo et al., 2016; Xu et al., 2016; Zhao et al., 2019; Liu et al., 2020).
For bivalves, as the important marine calcifiers, their exposures to OA have been widely demonstrated to inhibit shell formation, growth, survival, and metamorphosis (Talmage and Gobler, 2009; Liu et al., 2020). The digestive gland is an important functional tissue in mollusks (Zhang et al., 2022), which plays an important role in nutrient intake, hormone synthesis, energy metabolism and immune defense (Khan et al., 2018; Zhang et al., 2022). However, its response under OA conditions has not been well understood. At present, for bivalves, the exposure to OA has been widely demonstrated to inhibit the antioxidant capacity (Wang et al., 2016; Zhang et al., 2022), damage the tissue structure (Wang et al., 2019), and reduce the activity of digestive enzymes (Kong et al., 2019; Xu et al., 2020). The antioxidant defense system can remove excessive ROS produced by biological organisms due to environmental changes and prevent cells from oxidative damage (He et al., 2021). Digestive enzymes play an important role in nutrient digestion, and their activities can directly reflect the digestive capacity and nutritional status and ultimately translate into the growth performance of marine bivalves (Liang et al., 2021). However, in previous studies, most bivalves were directly exposed to acidified seawater, while most of the bivalves in the natural environment lived in the seabed sediment. Therefore, the impact of OA on the digestive gland of bivalves in the real living environment deserves further attention and evaluation.
Razor clams (Sinonovacula constricta) are infaunal bivalve species that constitute a considerable component of infaunal soft-bottom communities, inhabiting fine sand, silt or sandy-mud ocean floors (Fernández-Tajes et al., 2010). As a traditional seafood, they are widely distributed in global coastal areas and are one of the important mariculture species in China. In 2020, for example, their output exceeded 860,000 tons and breeding area was nearly 4,326 hectares (Fishery Administration Bureau, Ministry of Agriculture and Rural Affairs, National Fisheries Technology Extension Station, 2021). However, as of now, the responses of razor clams to the environmental changes and the accompanied impacts on their fitness remain poorly understood. In the present study, we evaluated the impacts of OA on the growth index and survival of razor clam, as well as the antioxidant capacity and enzyme activity of digestive gland. The results of this study will provide a better understanding of the response and adaptive potential of bivalves in a rapidly acidifying ocean.
Materials and methods
Individual collection and laboratory acclimation
A total of 300 razor clams (50.95 ± 2.45 mm shell length, 0.47 ± 0.10 g dry tissue weight) were collected from a muddy farming pond in Sanmen county, Zhejiang province, China (29.057166N, 121.562862E). Upon arrival in the laboratory by airplane, razor clams were immediately cleaned and acclimated in an aquarium facility equipped with a filtering system and air supply for one week. Environmental conditions of acclimation with seawater temperature, salinity, pH, and dissolved oxygen concentration were kept at 28°C, 18, 8.1 and >7 mg O2 L−1, respectively, which were rather comparable to those recorded at the sampling site and day of collection. Razor clams were fed daily twice (at 8:00 am and 20:00 pm, respectively) with fresh microalgae Isochrysis galbana. Considering that the fresh food supply may offset the effects of ocean acidification on marine bivalves (Thomsen et al., 2012) and fulfill their nutritional requirements for metabolism (Xu et al., 2016), 2ml of concentrated Chlorella vulgaris (2×108 cells ml-1) was also fed every time throughout the course of the experiment.
Experimental setup
To examine the influence of CO2-induced seawater acidification on razor clams, two seawater recirculating systems were established. Each system consists of three 45-L exposure aquariums (housing 50 clams per aquarium), a 120-L filtering aquarium (containing filter material), and a 70-L gas mixing aquarium (containing bubble and temperature regulator). By bubbling air or pure CO2 gas into the gas mixing aquarium, two scenarios of seawater pH (i.e., 8.1 and 7.7) were manipulated, the latter mimicking the future ocean condition by the end of this century (Intergovernmental Panel on Climate Change [IPCC], 2014). Seawater pH was monitored using a digital pH controller (PB-10, sartorius, Germany), and CO2 gas flow regulated by mass flow controller (D08-8CM, SEVENSTAR, China). The temperature of each system was maintained by a digital temperature regulator (CN606, CHANNG, China). In the gas mixing aquarium of each system, seawater was continuously aerated by an air pump (ACO-012, SunSun, China) to keep the water dissolved oxygen levels. To make the simulation environmentally realistic, about 15cm of sandy-mud was laid in each exposure aquarium. When all razor clams buried into the sediment, seawater pH of the system was gradually adjusted to the setting level, and after 24 h of equilibration, the system reached a steady state. The experiment lasted for 35d, during which seawater was renewed by a quarter every three days, and constant lowered pH condition was maintained. Razor clams were fed daily in the same manner as during the acclimation period. Temperature, salinity, pH, and mortality were recorded on a daily basis.
Sample preparation
Prior to sample collection, all razor clams were starved for 12h to empty their intestine. A total of 30 razor clams from each system (i.e., 10 samples from each replicate) were randomly selected to measure the growth performance. A total of 18 specimens (i.e., six samples from each replicate) were sampled from each scenario and then carefully dissected on an ice tray to obtain the digestive gland and stored at -80°C pending analyses of enzyme activity. Besides, the visceral mass of 6 additional sample (i.e., two samples from each replicate) was fixed with 10% formalin solution for histological assay.
Growth performance analysis
The growth performance of razor clams from each system was measured at the end of the experiment. The final dry body weight (Wf), dry body weight gain (DWG) (Liu et al., 2019), specific growth rate (SGR) (Liu et al., 2019), condition index (CI) (Xu et al., 2016), and survival rate (SR) were chosen and then calculated using the following equations.
where Wi (g) and Wf (g) are the initial and final dry body weight of razor clams, respectively. D (d) is the duration of experiment days. Wshell (g) is the finial dry shell weight. Ni and Nf are the initial and final number of surviving individuals, respectively.
Enzyme activity analysis
The digestive gland of three razor clams from each replicate was mixed as one sample to determine activities of antioxidant enzymes, including superoxide dismutase (SOD), catalase (CAT), malondialdehyde (MDA), and glutathione (GSH), and digestive enzymes, including amylase (AMS) and lipase (LPS). The preparation method was based on our previous study (Liang et al., 2021). In brief, digestive gland samples were homogenized three times with physiological salt solution (1:9W/V) in ice water for 30s, using an electric mixer at 6,000 rpm. The homogenate was centrifuged at 9000g at 4°C for 30m to remove tissue fragments and lipids. The supernatant (enzyme extract) was then distributed into 1.5 mL Eppendorf tubes and stored at −20°C until enzyme detection. Activities of SOD, CAT, MDA, GSH, AMS, and LPS were measured using kits (Nanjing Jiancheng Bioengineering Institute, Nanjing, China), according to the manufacturer’s instructions.
Histological assay
The visceral mass fixed in the formalin solution were firstly dehydrated through an increasing ethanol concentration series, and then transparent and embedded in paraffin following the standard procedures. The sections were 7-μm thick and placed on a glass slide. Histological strain was performed with hematoxylin and eosin solution (Dridi et al., 2007). Images of stained sections were collected by Motic BA600 digital scanning microscope. There were 5 sections per slide and 2 slides for each razor clam. That meant there were 60 sections for each group, which met the requirement for statistical analysis (n=5×2×6).
Statistical analysis
All data obtained were presented as means ± SEM, and analyzed using software SPSS 22.0 (Chicago, IL, USA). The variance equality of experimental results was firstly analyzed using Lenene test, and then independent sample T test method was used to determine the difference between the two groups. The statistically significant difference was set as p< 0.05.
Results
Environmental conditions during the experiment
Continuous monitoring of the recirculating systems showed that scenarios of seawater acidification were successfully achieved (Figure 1). Seawater pH targets were consistently maintained throughout the experiment, with dissolved oxygen concentrations above 6 mg L-1, temperature varying from 22.3 to 26.1°C, and salinity maintained at 16-18 PSU.
Growth performance
The effects of seawater acidification on the growth performance of razor clams were demonstrated in Figure 2. Exposure of razor clams to reduced seawater pH did not elicit statistically significant decreases in Wf, DWG, SGR, and CI (p = 0.161, 0.161, 0.177, and 0.957, respectively). Under acidified conditions, no mass mortality event occurred, as exemplified by survival rate reaching 86% and its simultaneously gradual decrease compared with individuals grown under ambient conditions (Figure 3).
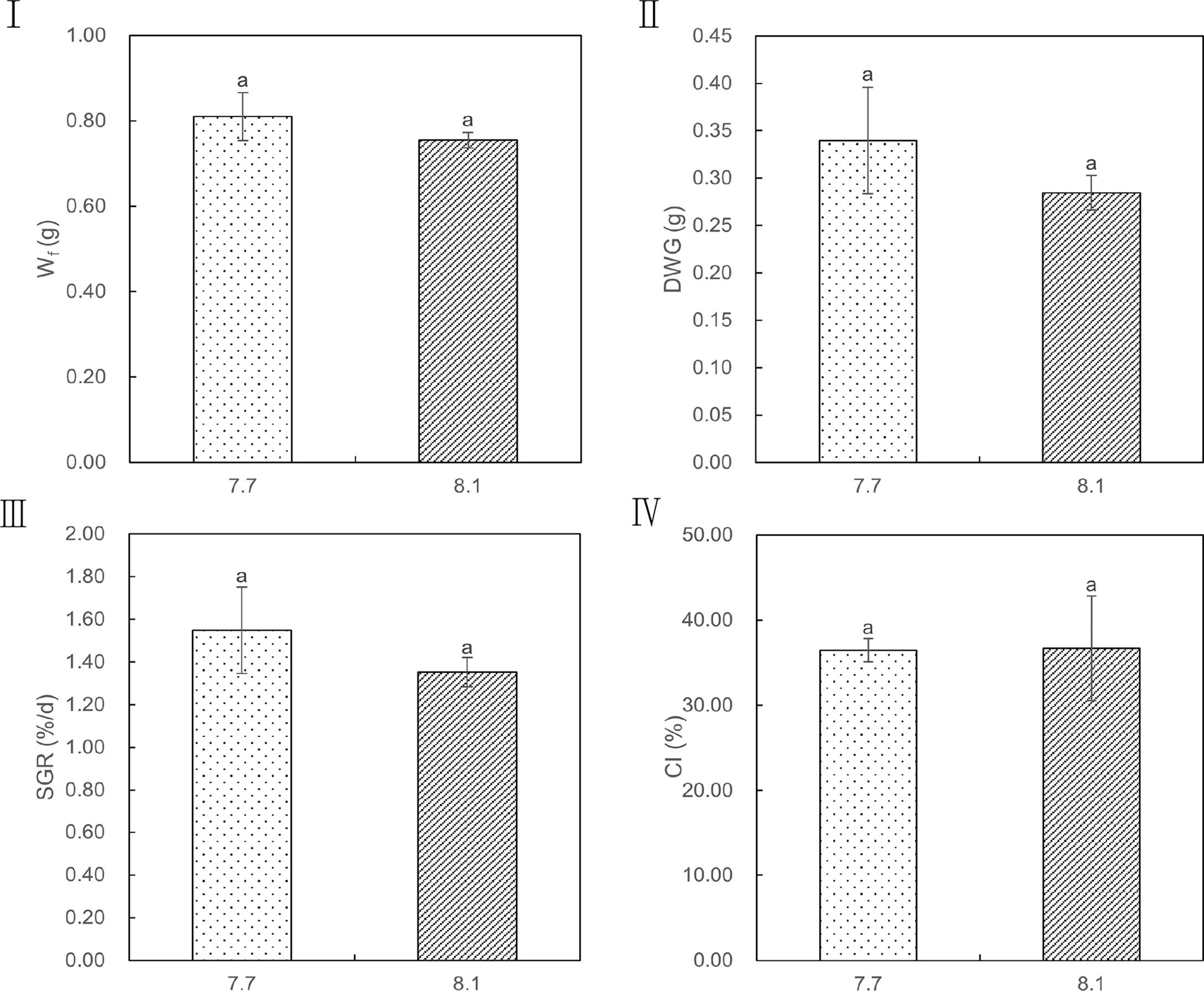
Figure 2 Wf, DWG, SGR, and CI values of razor clam cultured under pH 7.7 and pH 8.1 exposure over the 35-day experiment. Wf (I): finial dry body weight, DWG (II): finial dry body weight gain, SGR (III): specific growth rate, CI (IV): condition index. Different lowercase letters indicate significant differences (P<0.05) between the two groups.
Activities of digestive and antioxidant enzymes
In comparison to razor clams reared at pH 8.1, exposure to lowered pH of 7.7 significantly depress activities of LPS (p = 0.02; Figure 4I) in the 14-day exposure periods, but no significant difference was observed in the 35-day exposure periods (p = 0.56; Figure 4I). The activities of AMS had no significant difference between the two groups until the end of the experiment (p = 0.45 on 14-day and p = 0.23 on 35-day; Figure 4II). In addition, the LPS and AMS activities of the two groups at 35 days were significantly higher than those at 14 days (p = 0.00 for all; Figure 4I and II). These results suggest that acidification did not significantly reduce the level of digestive enzymes in razor clam.
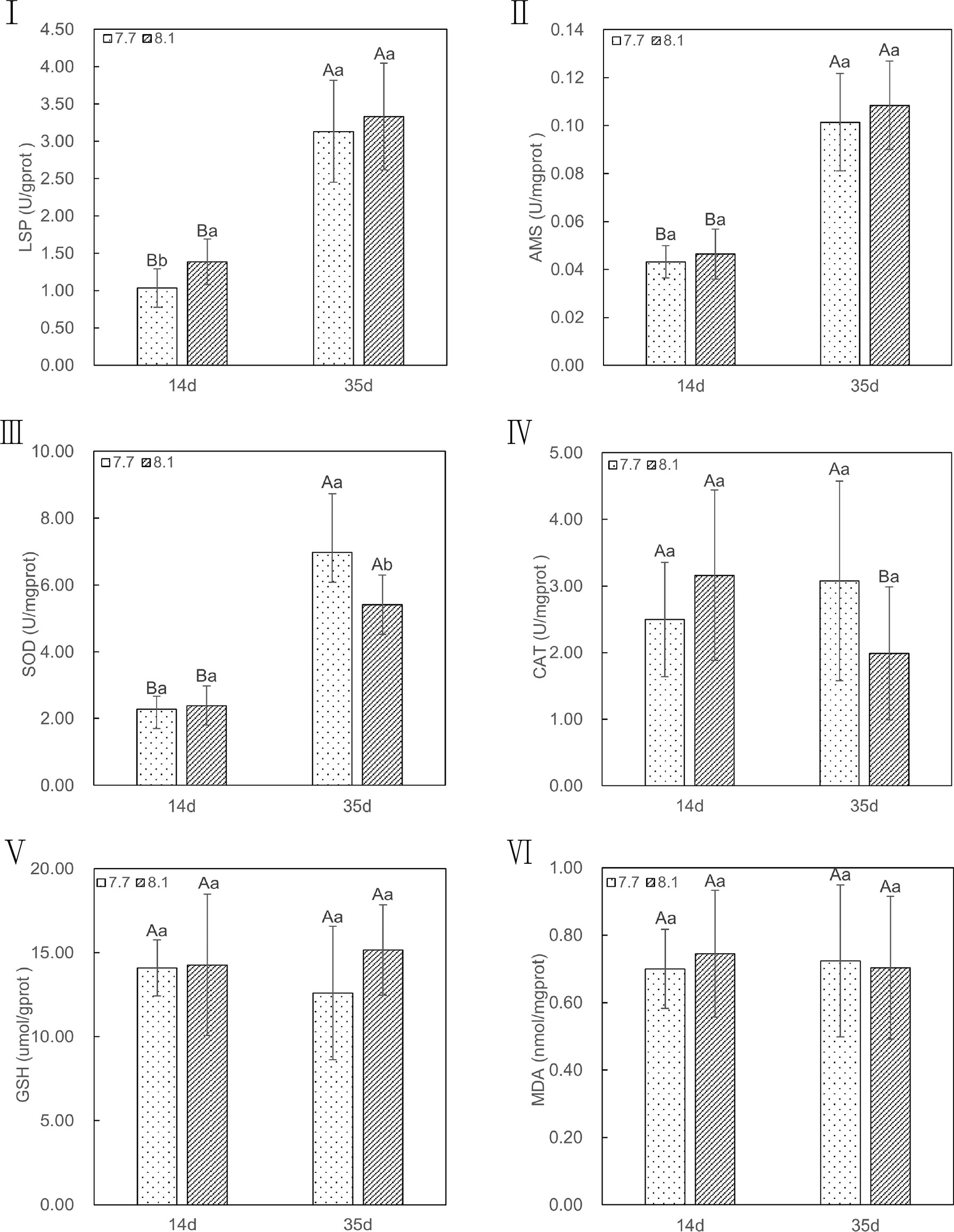
Figure 4 LSP, AMS, SOD, CAT, MDA, and GSH content of razor clam cultured under pH 7.7 and pH 8.1 exposure at the 14-day and 35-day experiment. LPS (I): lipase, AMS (II): amylase, SOD (III): Superoxide dismutase, CAT (IV): catalase, GSH (V): glutathione, MDA (VI): malondialdehyde. Note: different capital letters indicate significant differences (P<0.05) between the same groups at different time points; different lowercase letters indicate significant differences (P<0.05) between groups at the same point in time.
Likewise, when exposed to seawater acidification, activities of antioxidant enzymes, such as CAT (p = 0.21 on 14-day and p = 0.09 on 35-day; Figure 4IV), GSH (p = 0.91 on 14-day and p = 0.70 on 35-day; Figure 4V), and the level of MDA (p = 0.26 on 14-day and p = 0.37 on 35-day; Figure 4VI) were not significantly higher than those assayed in razor clams grown under ambient conditions in whole periods of acidification exposure, except for SOD, in which the pH 7.7 group showed significantly (p =0.03; Figure 4III) increase activity compare with pH 8.1 group after 35-day of acidification exposure. In addition, at the end of acidification exposure, the GSH and MDA in both two groups displayed no significant difference (p =0.94 and 0.78 for pH 7.7 group, p =0.61 and 0.70 for pH 8.1 group; Figure 4V and VI) compared to those of the group in 14-day of experiment periods, just except for CAT, in which significant lower was observed in pH 8.1 group (p =0.04; Figure 4IV). Meanwhile, the level of SOD activities in both two groups after 35-day exposure were all significantly higher (p =0.00 for all; Figure 4III) than those of group in 14-day. In conclusion, the antioxidant capacity of razor clam could not be significantly affected by acidification seawater.
Histological observation
The normal structure of digestive tubules (dt) in the digestive gland is shown in Figure 5I. The tubules consist of a single layer of full rounded ciliated epithelial cells (ec) that narrow or even block the lumen. The interstitial tissue (it) was composed of fibroblasts. Exposure to acidified sea water apparently damaged the structure of the digestive tubes (Figure 5II). Some severe lesions were observed in the pH 7.7 group, including degradation of digestive tubular, atrophy of epithelial cells, and loose cell arrangement, manifesting as the lumen enlarged abnormally and basement membrane of epithelial cells became thin and discontinuous. At the same time, the digestive glands in the pH 7.7 group showed extensive local lesions, where diffuse digestive tubules and cellular fibrosis (shown by arrows) appeared, which may be a precursor to necrosis. In addition, a higher proportion of haemocyte infiltrations were observed in the pH 7.7 group.
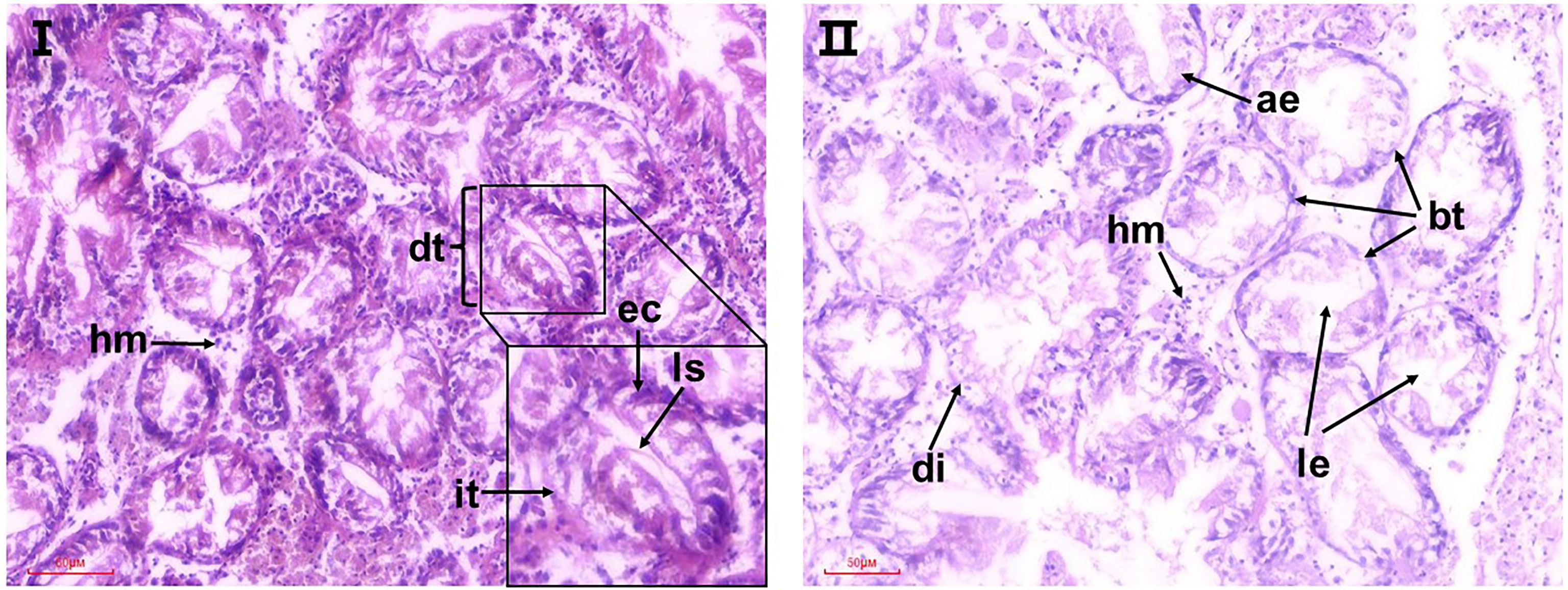
Figure 5 Representative micrographs of observed histopathological alterations in the digestive glands of razor clams after 35 days of exposure to seawater acidification. I: the pH 8.1 group; II: pH 7.7 group; dt, digestive tubules; it, interstitial tissue; ec, epithelial cell; ls, luminal stenosis; le, luminal enlarged; hm, hemocyte. ae, atrophy of epithelial cells; bt: basement membrane of epithelial cells became thin; di, diffuse digestive tubules.
Discussion
Rapidly increasing anthropogenic CO2 emissions are causing ocean acidification and global warming. Elevated pCO2 in seawater, and associated reduction in pH, are expected to affect basic physiological processes, ultimately affecting growth and reproduction, and even survival in extreme cases (Wang et al., 2015; Xu et al., 2016). These environmental changes could have serious consequences for all kinds of marine organisms.
Many studies have shown that OA negatively affected on the survival, calcification, growth, development, and abundance of marine bivalves at different levels of biological organization (Kroeker et al., 2013). The effects of ocean acidification on the growth and development of shellfish larvae are particularly evident. Mercenaria mercenaria, Argopecten irradians, and Crassostrea virginica are all negatively affected in terms of their metamorphosis, growth, and survival (Talmage and Gobler, 2009), which can most likely be attributed to impairments in their calcification physiologies. Presently, some studies have demonstrated that acidification can adversely affect the digestive performance of shellfish and hinder the absorption of nutrients, which in turn reduced growth rate, fecundity, and survival rate of shellfish (Wang et al., 2019; Kong et al., 2019; Xu et al., 2020; Zhang et al., 2022). However, in this study, the detrimental effects of OA on the growth performance were not significant. There were no significant differences in Wf, DWG, SGR, and CI between pH 7.7 group and pH 8.1 group. In particular, CI is an indicator that can track gonad development and spawning time in bivalve mollusks (Gosling, 2003). For example, the CI of the Ruditapes philippinarum was increased during gonad maturation and decreased during spawning (Dang et al., 2010). A higher CI value was observed at the end of the experiment, indicating the gonad maturation. In addition, our results showed that the adverse effects of reduced pH on CI were not significant at the end of the experiment, suggesting that ocean acidification may not affect the reproductive capacity of razor clam. In this study, we also found that the acidified seawater did not significantly reduce the survival rate of razor clams.
The growth of aquatic animals depends on nutrient utilization to a great extent, and digestive enzymes play an important role in nutrient digestion (Liang et al., 2021). Their activity directly reflects the digestive capacity and nutritional status of aquatic animals (Furne et al., 2005). The digestive glands have a specific pH environment and are susceptible to changes in the pH of the microenvironment (Zhang et al., 2022). An optimal pH will help ensure the activity of the digestive enzyme (Krogdahl and Sell, 1989). It was reported that with the decrease of pH in seawater, the pH in the stomach of sea urchin larvae significantly decreased and resulting in inhibition of digestive enzyme activity (Stumpp et al., 2011). We found similar results in the present study, 2 kinds of digestive enzymes including lipase and amylase, were inhibited to varying degrees by OA. At the same time, it also shows that there was certain difference in dietary carbohydrate and lipid between pH 7.7 group and pH 8.1 group, but the differences were not significant. This further demonstrated that razor clam has the capacity to adapt their digestive physiology in response to the acidification of living environment.
It is well known that the antioxidant defense system of digestive gland is one of the important physiological functions of bivalves, which can remove excessive ROS induced by environmental changes and prevent cellular dysfunction (Wang et al., 2019; He et al., 2021).The antioxidant defense system of organisms can be divided into enzyme antioxidants, such as SOD and CAT, and non-enzyme antioxidants, such as GSH and ascorbic acid (De Zwart et al., 1999). In the present study, when acutely exposed to acidification at the early stages, SOD, CAT, MDA, and GSH were certainly decreased, indicating that short-term acidification did not produce enough ROS in razor clam to activates its antioxidant defense system. However, after long-term exposure to acidified environment, CAT, and MDA were enhanced, especially SOD was significantly higher than the pH 8.1 group, suggesting that razor clam is either able to withstand acidification tress and/or can implement positive physiological response to cope with acidification stress. These results demonstrate the promise of antioxidant related enzymes as indicators of acidification influence on essential physiological processes. In addition, prolonged acidification may cause damage to cells. Evidence supporting this hypothesis comes from lower GSH than the control group after 35 days of acidification exposure.
Impairment from ocean acidification at the cellular level can extend to higher levels of biological tissues (He et al., 2021). This kind of tissue impairment caused by environmental variation can be basically divided into two types: one is the impairment caused by defense reaction, including epithelial cell hypertrophy, hyperplasia, edema (Cengiz and Unlu, 2006). The second is the direct damage, including epithelial cell necrosis and shedding (Wong and Wong, 2000). In this study, we observed some direct damages on the structure of digestive gland, including degradation of digestive tubular, atrophy of epithelial cells, loose cell arrangement, even diffuse. Atrophy and death of the epithelium may disrupt the integrity of the tubule wall, resulting in leakage of digestive juices and threatening surrounding tissue cells. This may be related to the diffusion of the tubules and activation of antioxidant capacity of the present study. In addition, excessive abnormal death of the digestive epithelium may affect the secretion and flow of digestive juices, thereby reducing enzymes and reducing digestive enzyme activity (Zhang et al., 2022). This also explains why the activity of digestive enzymes in pH 7.7 group was inhibited in this study.
Conclusions
Based on the above results, we speculated that the exposure to acidifying seawater changes the inner pH of digestive gland, which is not conducive to the activation of digestive enzymes. Moreover, the decrease inner pH of the digestive gland damages the structure of the digestive gland. Although the inactivation of digestive enzymes is aggravated, the activity of antioxidant enzymes is activated. However, these adverse reactions did not cause significant effect on razor clams, in line with the results of growth performance. These results indicate that razor clams may have evolved to cope with lowered seawater pH, especially considering that they are used to live in muddy flats where the pH of pore water is much lower than that of overlying water.
Data availability statement
The original contributions presented in the study are included in the article/supplementary material. Further inquiries can be directed to the corresponding author.
Author contributions
JL and YG contributed to the concept and design of the study. YL and FZ carried out the experiments respectively. YRL and SL made the statistical analysis. JL, YL, and FZ wrote the first draft. YRL, SL and YG wrote part of the manuscript. All authors contributed to the article and approved the submitted version.
Funding
This work was supported by the National Natural Science Foundation of China under Grant No. 31972814 and 42076121, the Project of Tianjin Science and Technology under Grant No. 20YDTPJC01800, the Earmarked Fund for Modern Agro-Industry Technology Research System under Grant No. CARS-49, and the Fundamental Research Funds of Tianjin Universities under Grant No. 2019KJ041.
Conflict of interest
The authors declare that the research was conducted in the absence of any commercial or financial relationships that could be construed as a potential conflict of interest.
Publisher’s note
All claims expressed in this article are solely those of the authors and do not necessarily represent those of their affiliated organizations, or those of the publisher, the editors and the reviewers. Any product that may be evaluated in this article, or claim that may be made by its manufacturer, is not guaranteed or endorsed by the publisher.
References
Cengiz E. I., Unlu E. (2006). Sublethal effects of commercial deltamethrin on the structure of the gill, liver and gut tissues of mosquitofish, Gambusia affinis: A microscopic study. Environ. Toxicol. Pharmacol. 21 (3), 246–253. doi: 10.1016/j.etap.2005.08.005
Dang C., De Montaudouin X., Gam M., Paroissin C., Bru N., Caill-Milly N. (2010). The Manila clam population in arcachon bay (SW france): can it be kept sustainable? J. Sea. Res. 63, 108–118. doi: 10.1016/j.seares.2009.11.003
De Zwart L. L., Meerman J. H., Commandeur J. N. M., Vermeulen N. P. E. (1999). Biomarkers of free radical damage: Applications in experimental animals and in humans. Free Radical Biol. Med. 26, 202–226. doi: 10.1016/S0891-5849(98)00196-8
Dong Z., Mao S., Chen Y., Ge H., Li X., Wu X., et al. (2019). Effects of air-exposure stress on the survival rate and physiology of the swimming crab Portunus trituberculatus. Aquaculture 500 (2019) 429–434. doi: 10.1016/j.aquaculture.2018.10.049
Dridi S., Romdhane M. S., Elcafsi M. H. (2007). Seasonal variation in weight and biochemical composition of the pacific oyster, Crassostrea gigas in relation to the gametogenic cycle and environmental conditions of the bizert lagoon, Tunisia. Aquaculture 263 (2007), 238–248. doi: 10.1016/j.aquaculture.2006.10.028
Fernández-Tajes J., Freire R., Méndez J. (2010). A simple one-step PCR method for the identification between European and American razor clams species. Food Chem. 118, 995–998. doi: 10.1016/j.foodchem.2008.10.043
Figueiredo D., Branco P. C., Santos D., Emerenciano A. K., Iunes R. S., Borges J., et al. (2016). Ocean acidification affects parameters of immune response and extracellular pH in tropical sea urchins Lytechinus variegatus and Echinometra luccunter. Aquat. Toxicol. 180, 84–94. doi: 10.1016/j.aquatox.2016.09.010
Fishery Administration Bureau, Ministry of Agriculture and Rural Affairs, National Fisheries Technology Extension Station (2021). China Fishery statistics yearbook 2021 (Beijing, China: China Agriculture Press).
Furne M., Hidalgo M. C., Lopez A., Garcia-Gallego M., Morales A. E., Domezain A., et al. (2005). Digestive enzyme activities in Adriatic sturgeon acipenser naccarii and rainbow trout Oncorhynchus mykiss. a comparative study. Aquaculture 250 (1-2), 391–398. doi: 10.1016/j.aquaculture.2005.05.017
He G., Liu X., Xu Y., Liang J., Deng Y., Zhao L. (2021). Repeated exposure to simulated marine heatwaves enhances the thermal tolerance in pearl oysters. Aquat. Toxicol. 239 (2021), 105959. doi: 10.1016/j.aquatox.2021.105959
Intergovernmental Panel on Climate Change [IPCC] (2014). “Climate change,” in Climate change 2014: Synthesis report. contribution of working groups I, II and III to the fifth assessment report of the intergovernmental panel on climate change. Eds. Pachauri R. K., Barros A. M. R., Barros V. R., et al (Cambridge, United Kingdom and New York, NY, USA: Cambridge University Press), 151.
Khan B., Clinton S. M., Hamp T. J., Oliver J. D., Ringwood A. H. (2018). Potential impacts of hypoxia and a warming ocean on oyster microbiomes. Mar. Environ. Res. 139, 27–34. doi: 10.1016/j.marenvres.2018.04.018
Kong H., Wu F., Jiang X., Wang T., Hu M., Chen J., et al. (2019). Nano-TiO2 impairs digestive enzyme activities of marine mussels under ocean acidification. Chemosphere 237, 124561. doi: 10.1016/j.chemosphere.2019.124561
Kroeker K. J., Kordas R. L., Crim R., Hendriks I. S., Ramajo L., Singh G. S., et al. (2013). Impacts of ocean acidification on marine organisms: quantifying sensitivities and interaction with warming. Global Change Biol. 19 (6), 1884–1896. doi: 10.1111/gcb.12179
Krogdahl A., Sell J. L. (1989). Influence of age on lipase, amylase, and protease activities in pancreatic tissue and intestinal contents of young turkeys. Poultry. Sci. 68, 1561e1568. doi: 10.3382/ps.0681561
Kurihara H. (2008). Effects of CO2-driven ocean acidification on the early developmental stages of invertebrates. Mar. Ecol. Prog. Ser. 373, 275–284. doi: 10.3354/meps07802
Liang J., Bi X., Dai W., Deng Y. (2021). Particulated microalgae composite (PMC) as substitute for live microalgae in culture of juvenile Manila clam Ruditapes philippinarum (A. adams and reeve 1850): A feasibility analysis. Indian J. Fisheries. 68 (3), 53–63. doi: 10.21077/ijf.2021.68.3.114197-07
Liao X., Sun Z., Cui Z., Yan S., Fan S., Xia Q., et al. (2022). Effects of different sources of diet on the growth, survival, biochemical composition and physiological metabolism of clam (Cyclina sinensis). Aquacult. Res. 00, 1–10. doi: 10.1111/are.15886
Liu G., Deng Y., Verdegem M., Ye Z., Zhu S. (2019). Using poly(β-hydroxybutyrate-β-hydroxyvalerate) as carbon source in biofloc-systems: Nitrogen dynamics and shift of Oreochromis niloticus gut microbiota. Sci. Total. Environ. 694, 133664. doi: 10.1016/j.scitotenv.2019.133664
Liu Z., Zhou Z., Zhang Y., Wang L., Song X., Wang W., et al. (2020). Ocean acidification inhibits initial shell formation of oyster larvae by suppressing the biosynthesis of serotonin and dopamine. Sci. Total. Environ. 735, 139469. doi: 10.1016/j.scitotenv.2020.139469
Stumpp M., Wren J., Melzner F., Thorndyke M., Dupont S. (2011). CO2 induced seawater acidification impacts sea urchin larval development I: elevated metabolic rates decrease scope for growth and induce developmental delay. Comp. Biochem. Physiol. A-Mol. Integr. Physiol. 160 (3), 331–340. doi: 10.1016/j.cbpa.2011.06.022
Talmage S. C., Gobler C. J. (2009). The effects of elevated carbon dioxide concentrations on the metamorphosis, size, and survival of larval hard clams (Mercenaria mercenaria), bay scallops (Argopecten irradians), and Eastern oysters (Crassostrea virginica). Limnol. Oceanogr. 54 (6), 2072–2080. doi: 10.4319/lo.2009.54.6.2072
Thomsen J., Casties I., Pansch C., Körtzinger A., Melzner F. (2012). Food availability outweights ocean acidification effects in juvenile Mytilus edulis: laboratory and field experiments. Global Change Biol. 19, 1017–1027. doi: 10.1111/gcb.12109
Wang Q., Cao R., Ning X., You L., Mu C., Wang C., et al. (2016). Effects of ocean acidification on immune responses of the pacific oyster Crassostrea gigas. Fish. Shellfish. Immunol. 49, 24–33. doi: 10.1016/j.fsi.2015.12.025
Wang X., Huang W., Wei S., Shang Y. Y., Gu H., Wu F., et al. (2019). Microplastics impair digestive performance but show little effects on antioxidant activity in mussels under low pH conditions. Environ. pollut. 258, 113691. doi: 10.1016/j.envpol.2019.113691
Wang Y., Li L., Hu M., Lu W. (2015). Physiological energetics of the thick shell mussel Mytilus coruscus exposed to seawater acidification and thermal stress. Sci. Total. Environ. 514 (2015), 261–272. doi: 10.1016/j.scitotenv.2015.01.092
Wong C. K. C., Wong M. H. (2000). Morphological and biochemical changes in the gills of tilapia (Oreochromis mossambicus) to ambient cadmium exposure. Aquat. Toxicol. 48 (4), 517–527. doi: 10.1016/S0166-445X(99)00060-0
Xu Y., Liang J., He G., Liu X., Yang K., Masanja F., et al. (2022). Responses of pearl oysters to marine heatwaves as indicated by HSP70. Front. Mar. Sci. 9, 847585. doi: 10.3389/fmars.2022.847585
Xu M., Sun T., Tang X., Lu K., Jiang Y., Cao S., et al. (2020). CO2 and HCl-induced seawater acidification impair the ingestion and digestion of blue mussel Mytilus edulis. Chemosphere 240, 124821. doi: 10.1016/j.chemosphere.2019.124821
Xu X., Yang F., Zhao L., Yan X. (2016). Seawater acidification affects the physiological energetics and spawning capacity of the manila clam Ruditapes philippinarum during gonadal maturation. Comp. Biochem. Physiol. A-Mol. Integr. Physiol. 196, 20–29. doi: 10.1016/j.cbpa.2016.02.014
Zhang L., Wang X., Zhang W., Yin X., Liu Q., Qiu L. (2022). Impact of ocean acidification on physiology and microbiota in hepatopancreas of pacific oyster Crassostrea gigas. J. Oceanol Limnol. 40, 620–633. doi: 10.1007/s00343-021-0462-x
Zhao L., Liu L., Liu B., Liang J., Lu Y., Yang F. (2019). Antioxidant responses to seawater acidification in an invasive fouling mussel are alleviated by transgenerational acclimation. Aquat. Toxicol. 217, 105331. doi: 10.1016/j.aquatox.2019.105331
Zhao L., Shirai K., Tanaka K., Milano S., Higuchi T., Murakami-Sugihara N., et al. (2020). A review of transgenerational effects of ocean acidification on marine bivalves and their implications for sclerochronology. Estuarine. Coast. Shelf. Sci. 235, 106620. doi: 10.1016/j.ecss.2020.106620
Keywords: ocean acidification, growth, digestive gland, physiology, Sinonovacula constricta
Citation: Liang J, Liu Y, Zhu F, Li Y, Liang S and Guo Y (2022) Impact of ocean acidification on the physiology of digestive gland of razor clams Sinonovacula constricta. Front. Mar. Sci. 9:1010350. doi: 10.3389/fmars.2022.1010350
Received: 03 August 2022; Accepted: 12 September 2022;
Published: 23 September 2022.
Edited by:
Zhongming Huo, Dalian Ocean University, ChinaReviewed by:
Kai Liao, Ningbo University, ChinaZhihua Lin, Zhejiang Wanli University, China
Zhiguo Dong, Jiangsu Ocean University, China
Chenghua Li, Ningbo University, China
Copyright © 2022 Liang, Liu, Zhu, Li, Liang and Guo. This is an open-access article distributed under the terms of the Creative Commons Attribution License (CC BY). The use, distribution or reproduction in other forums is permitted, provided the original author(s) and the copyright owner(s) are credited and that the original publication in this journal is cited, in accordance with accepted academic practice. No use, distribution or reproduction is permitted which does not comply with these terms.
*Correspondence: Yongjun Guo, Z3VveW9uZ2p1bkB0amF1LmVkdS5jbg==