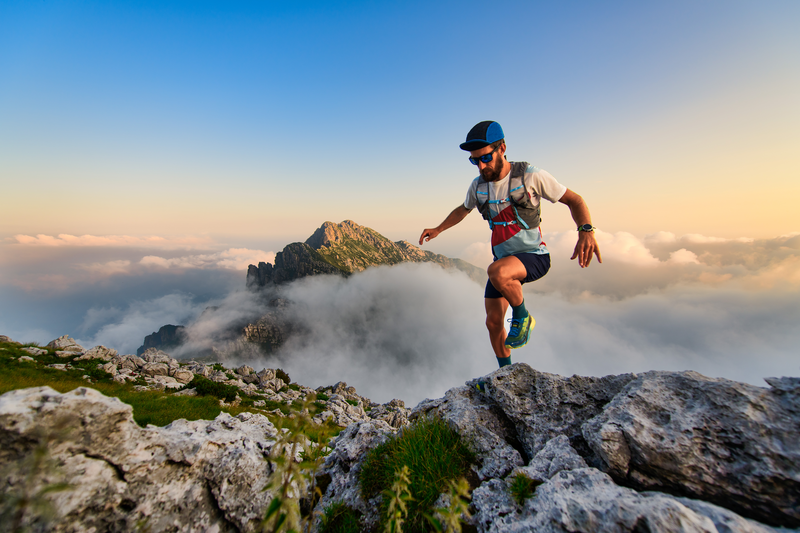
94% of researchers rate our articles as excellent or good
Learn more about the work of our research integrity team to safeguard the quality of each article we publish.
Find out more
ORIGINAL RESEARCH article
Front. Mar. Sci. , 14 November 2022
Sec. Ocean Solutions
Volume 9 - 2022 | https://doi.org/10.3389/fmars.2022.1009834
Ever since the concept of deep-sea mining was first described, the prospect of deep-sea mining testing and monitoring has proved to be a subject of great interest. Despite the considerable research and commercial attention given to developing deep-sea mining strategies, no previous study has looked explicitly at the sustainability of deep-sea mining transport plans. The objective of this paper, therefore, is to evaluate the sustainability of deep-sea mining vertical transport plans, using the methodology of fuzzy analytic network process. Major criteria for evaluating deep-sea mining vertical transport plans can be divided into technological, economic, environmental, and social components, each containing both qualitative and quantitative attributes. Weights for each criterion are determined through a questionnaire survey, completed by experts in the fields of environmental impact, policy making, marine mining, project sustainability consultancy and project economic profitability research. The research presented in this paper could be used directly in the sustainability assessment of upcoming deep-sea mining projects, furtherly contributing to the industrialization of the entire deep-sea mining industry.
Recent advances in deep-sea mining (DSM) strategies have led many international marine mining companies to develop implementation plans for the first seabed mining project. For example, the Canadian company Nautilus Minerals had stated its aim to begin mining minerals from the seabed belonging to Papua New Guinea in 2019 (Mining Weekly, 2018). Nautilus Minerals company had announced that they will be chartering a production support vessel (PSV) from Fujian Mawei Shipbuilding Ltd. for $199,910 per day, for a rental period of at least five years (Nautilus Minerals, 2018). Although the company is now bankrupt and many projects are on hold, its contribution to the exploration of DSM cannot be ignored. The industrial exploitation of DSM seems to be full of thorns, scientific research activities, technological improvement, but deep-sea trial mining, deep-sea exploration and environmental monitoring in this field have never been interrupted (Bauch and Cherniavskaia, 2018; Jungblut et al., 2018; MiningImpact, 2018; Otte et al., 2021). Despite this, no research exists that looks at the sustainability of DSM vertical transport plans and considers the related associated technological, economic, environmental and social factors (ISA, 2022a; ISA, 2022b).
The schematic diagram of one of the typical DSM projects is shown in Figure 1. The majority of the workload involved in DSM takes place at sea. A key research topic – and one that has received considerable attention previously – is the environmental impact of DSM activities, particularly given the low productivity (Pabortsava and Lampitt, 2020; Peng et al., 2021) and vulnerability of deep ocean ecology (Tilot, 2010; Van Dover, 2011; Jones et al., 2017; Tilot et al., 2018). Beside environmental impacts, both the technological and economic considerations of DSM are key components in the development of a sustainable DSM transport plan (Ma et al., 2017a; Ma et al., 2017b). Furthermore, other researchers also have examined the social implications of DSM in influencing sustainability projects (Roche and Bice, 2013; Tilot et al., 2021).
Figure 1 Graphic representation of an example DSM project (Ma et al., 2018a).
At present, most of the researches on sustainable development evaluation of deep-sea mining are qualitative analysis, even the quantitative analysis involved is very superficial (Sharma, 2011; Hallgren and Hansson, 2021). Another research gap in this topic is the incompleteness of the impact parameters. For sustainable development, a part of researchers only study one aspect of environmental pollution, economic benefits or social impact, while ignoring the interconnection relationship between each other (Filer and Gabriel, 2018; Levin et al., 2020a; Carver et al., 2020). Given that the decision-making process for a sustainable DSM transport plan involves the integration of several discrete-related aspects (e.g., technological, environmental, economic and social), a traditional cost-benefit model would not be qualified/suitable to address this problem (Folkersen et al., 2018; Madiraju, 2019; Amon et al., 2022). For instance, due to the large information interconnections, Folkersen et al. (2018) stated that it is ‘very difficult’ (‘if not impossible’) applying cost-benefit model in DSM sustainability assessment. The objective of this paper is to evaluate the sustainability of DSM vertical transport plans through Fuzzy-ANP (analytic network process) analysis. The sustainability considered in this paper is not limited to the sustainable development of environment, ecology and society, but also analyses the topic from a holistic perspective, which also includes technological and economic sustainable development. The paper is arranged as follows: the first (previous) section introduces the principles and aims of our research; the second section explains the MCDM (multiple criteria decision making) methodology and evaluation criteria selection. In the third section, numerical calculation principles and definitions of the evaluation criteria are described in detail. The fourth section focuses on a test case, demonstrating the application procedure for our framework. In the fifth section, conclusions and recommendations for future research are presented.
There are different methods available to address the DSM sustainability problems, such as Multi-criteria Decision Making (MCDM), Marine Spatial Planning (MSP), Integrated Coastal Zone Management (ICZM), etc. Different approaches have their respective focus, advantages and common application areas. However, compared with other methods, the application of MCDM in the direction of sustainable development is earlier, the application method is more mature, and the application field is more extensive. The authors of this paper conducted a systematic literature review to analyze the feasibility, research procedure and evaluation criteria of applying MCDM to the assessment of sustainable development in deep-sea mining (Ma et al., 2022).
The discipline of MCDM was first proposed by Zionts (1979). The MCDM method is used by Pohekar and Ramachandran (2004) to address the sustainable energy management issue with conflicting and multiple objectives. Huang et al. (2011) carried out a literature review on the MCDM application in the environmental impact assessment considering of trade-offs between social, political, environmental and economic aspects. Khalili and Duecker (2013) proposed a series of clean production projects by designing a sustainable environmental management system, which considers the environmental, industrial, economic and social criteria through the whole decision-making process. Rabbani et al. (2014) developed a new approaching by coupling MCDM method with sustainability balanced scorecard method to evaluate the oil producing companies’ performances. Carli et al. (2018) utilized MCDM method analyzing the sustainable development of energy, water and environmental systems in a city. An integrated MCDM method was also used by Nzotcha et al. (2019) to select an optimal hydro-energy storage plant site through a sustainable development perspective. The method of MCDM has been adopted in this research, following its successful application to a number of industrial fields (such as coastal mining (Vaghela et al., 2018), sustainable project planning (Pohekar and Ramachandran, 2004; Abdel-Basset et al., 2021) and manufacturing system scheduling (Erdogan et al., 2019)) to address similar multiple criteria problems (Ho et al., 2010; Rezaei, 2015). Following review of the existing literature, when applying the MCDM theory in DSM system, three key practices are identified: MCDM method selection, evaluation criteria determination and the calculation of criteria weights (Kahraman, 2008).
The major research components of this study are vertical transport plan generation, questionnaire survey, and MCDM application. The relationship of above components is intertwined. The questionnaire can get the weight of the evaluation parameters, while the vertical transport plan is the evaluation object, and the MCDM is the data evaluation methodology.
Fuzzy set theory is a widely used algorithm that allows imprecise and uncertain information to be examined through rigorous mathematical process (Zadeh, 1965). It has previously been used to integrate qualitative information and allow fuzzy date to be expressed as crispy evaluation weights (Boran et al., 2009). In the evaluation and analysis of the sustainable development of DSM, there are not only quantitative data, but also vague opinions suggestions (such as the preference of experts), and uncertain information to be considered. The method of ANP was developed by Saaty in the 1990s taking into consideration the interdependencies between different evaluation criteria (Saaty, 1996). It represents the development of analytic hierarchy process (AHP) method. Both ANP and AHP methods have the ability to divide a complex MCDM problem into several hierarchies with specific affiliation relationships. ANP has a highly systematic procedure of application and is quite easy to understand. Fuzzy & analytic network process (Fuzzy-ANP) is a typical integrated MCDM method, which integrates fuzzy set theory with ANP methodology. Mohanty et al. (2005) applied a Fuzzy-ANP approach to the analysis of the iron and steel industries of Southeast Asia by developing this approach further to describe a structured methodology to evaluate preference ambiguity in decision makers (DMs). Promentilla et al. (2008) redeveloped the Fuzzy-ANP method by redefining the fuzzy scale in terms of the degree of fuzziness, confidence level, and attribute toward fuzziness. They used this redeveloped Fuzzy-ANP method to evaluate contaminated site remedial countermeasures. Pang (2009) analysed supplier selection in order to facilitate optimal order allocation, by combining the Fuzzy-ANP method with fuzzy preference programming. Zhou (2012) proposed a systematic analytic framework using Fuzzy-ANP methodology to address project selection and evaluation problems. Given that the Fuzzy-ANP method has been successfully applied to such a wide variety of industrial research fields and also referring the literature review results of authors’ publication (Ma et al., 2022), it was selected and utilised for evaluation of sustainability in DSM vertical transport plans (Ma, 2019). This method can effectively integrate and process the evaluation data, so as to obtain the weights of different evaluation parameters and the scores of vertical transport plans for different evaluation criteria.
Before the application of MCDM method, a set of DSM vertical transport plans should be generated for evaluation. The formation of the evaluated DSM transport plans is a long vector (see Eq. 26), and the elements in the vector are the values of all evaluation criteria. The variables involved in a DSM transport plan consist of the type of lifting technology, e.g., continuous line bucket technology (CLB), pipe lifting with centrifugal pumps or with airlifting pumps, working period, maximum winch force (for CLB systems), pipe diameter and gas flux rate for hydraulic lifting and airlifting systems respectively. In real-life DSM working conditions, different vertical lifting methods require different compatible technology systems, such as tailings disposal systems and mineral processing systems. These may influence sustainability assessments of DSM vertical transport plans (Hoagland et al., 2010; Sharma, 2011). In these publications, however, the influences of such compatible systems on vertical lifting technologies are considered to be negligible, even though they are important for an assessment of the project. The sustainability evaluation framework described in this paper could be used in whole project assessment in DSM following the initial assessment of the technological, economic, environmental, and social factors at play.
Expert opinion is elicited through the designed questionnaire (see Appendix A) in order to determine the evaluation criteria weights. 27 researchers and engineers – in the fields of environmental impact, policy making, marine mining, project sustainability consultancy, and economic profitability – participated in this questionnaire survey.
Through the application of MCDM methodology, all DSM vertical transport plans can be evaluated and ranked, based on a comprehensive performance index that takes into consideration the technological, economic, environmental, and social aspects of these plans. The detailed explanation of the MCDM application is given in the following section.
Following a literature review of existing MCDM applications to sustainable project planning, manufacture scheduling systems, and other similar, industrial problems, an application procedure for a Fuzzy-ANP MCDM method was derived and is summarised in Figure 2. The procedure consists of 4 steps.
Figure 2 Application procedure of Fuzzy-ANP methodology in sustainable DSM transport plan selection.
Determination of a sustainable DSM vertical transport plan is dependent on technological, economic, environmental, and social factors. These factors are, of course, not completely independent of one another and are likely to exhibit a number of inter factorial relationships with each other.
MCDM has not, until now, been applied to DSM projects. Despite this, a number of useful theories, application frameworks, and evaluation criteria – some of which have potential utility in DSM vertical transport plan sustainability assessment – have been developed in other industries (Titus and Liberatore, 1991; Ho et al., 2010; Padhye and Deb, 2011; Rezaei, 2015). Using these pre-existing evaluation criteria, along with knowledge of the real-life working conditions involved in DSM activities, a set of evaluation criteria for use in the decision making process for sustainable DSM vertical transport plans is proposed in Table 1.
Table 1 Evaluation criteria for selecting a sustainable DSM transport plan (Akash et al., 1999; Mamlook et al., 2001; Yedla and Shrestha, 2003; Begić and Afgan, 2007; McDowall and Eames, 2007; Afgan and Carvalho, 2008; Chatzimouratidis and Pilavachi, 2009; Jovanović et al., 2009; Pilavachi et al., 2009; Pilavachi et al., 2009; Campos-Guzmán et al., 2019; Drazen et al., 2020; Gerber and Grogan, 2020; Ma et al., 2022).
As presented in Table 1, the major criteria in our methodology are the technological, economic, environmental, and social aspects of a sustainable DSM transport plan. These have both quantitative and qualitative sub-criteria. For example, technological sub-criteria include such quantitative parameters as specific energy consumption for lifting and production rate, and qualitative parameters, such as technological reliability. Sub-criteria are not completely independent of one another. For instance, there is a relationship between the proven technology, technology reliability and technology safety, because a well-proven technology tends to be associated with good technology reliability and high levels of safety (Haralambopoulos and Polatidis, 2003; Chatzimouratidis and Pilavachi, 2009; Ligus, 2017). There are also interconnections between environmental sub-criteria and physical-chemical parameters of the water column for example which amplify natural and anthropic impacts, so-called cumulative impacts, to the marine environment (Levin et al., 2016; Tilot, 2019; Levin et al., 2020b).
In a Fuzzy-ANP method, the criteria weights are determined through a pairwise comparison method. The acceptability of a pairwise comparison matrix is based on the consistency index (CI) and consistency ratio (CR). If the CR is larger than 0.10, the pairwise comparison matrices are not acceptable (Yüksel and Dagdeviren, 2007). The CI can then be calculated as follows (Yüksel and Dagdeviren, 2007; Zhang et al., 2015):
The parameter of CR can be calculated as follows:
in which RI means the random index.
When applying AHP methodology to multiple criteria problems, all criteria and sub-criteria are required to be independent of each other. However, in many real-life working conditions, there may be dependencies that exist between the different criteria (Saaty, 2005). A Fuzzy-ANP method was selected for use in this paper to evaluate the sustainability of DSM transport plans. This methodology has the further advantage of allowing consideration of both qualitative evaluation criteria as well as the dependency relationships between different criteria.
Following analyses using Fuzzy-ANP methodology, all DSM vertical transport plans can be evaluated based on a comprehensive performance index, denoted by Eq. (3) (Xu and Yang, 2001). The transport plan with the maximum comprehensive index is regarded to be the most preferable, i.e., the most sustainable DSM vertical transport plan.
In this section, the numerical calculation principles and definitions of these evaluation criteria for a sustainable DSM vertical transport plan are explained in detail. The authors’ previous work (Ma et al., 2017a; Ma et al., 2017b; Ma et al., 2017c; Ma et al., 2018a; Ma et al., 2018b; Ma, 2019; Ma et al., 2022) provided further detail concerning these criteria and their ability to systematically evaluate the technological, economic, and environmental impacts of DSM transport plans. Ma et al. (2017a, Ma et al., 2017b) quantitatively analyses various DSM lifting technologies (e.g., continuous line bucket lifting, hydraulic lifting and airlifting) from the perspective of technical feasibility and economic performance. Ma et al. (2018a, Ma et al., 2018b; Ma et al., 2017c) uses both qualitative and quantitative methods to analyze the environmental pollution (e.g., tailing disposal, sediment plume, species disturbance, physical seabed disturbance) caused by DSM. Ma et al. (2022) analyses the feasibility of using MCDM to solve the sustainable development of DSM through a systematic literature review, and clarifies the application technology route and research process of this method. In a summary, the essence of this paper is to apply the MCDM method to quantitatively analyze the sustainable development of DSM. This article is a step forward on the basis of these documents. It not only quantifies qualitative analysis, but also integrates technical, economic, environmental and social impacts to assess the sustainability of DSM.
* Specific energy for lifting
The specific energy consumption for lifting can be calculated as follows (Ma et al., 2017a):
In this paper, we compare three different methods of mineral vertical transportation technologies, e.g., the continuous line bucket lifting (CLB), hydraulic lifting (HL) using centrifugal pumps, and airlifting (AL) systems. Figure 3 presents a schematic diagram for a CLB and a HL system (Ma et al., 2017a). The arrangement of an AL system is similar to that of a centrifugal lifting system [as illustrated in Figure 3 (b)]. The primary differences are that, in AL systems, air compressors are installed on the production support vessel (PSV), and an auxiliary pipe is required to transport the compressed air to the lifting pipe (Yoshinaga and Sato, 1996). In CLB systems, total energy consumption is closely related to the winch force and the speed supplied by the steel ropes, see Eq. (5) (Schulte, 2013).
For a HL system, the drops in pressures required in lifting the mineral mixtures – in addition to the lifting pipe dimension – are the major determinants of energy consumption, see Eq. (6) (Shook and Bartosik, 1994).
The inlet pressure and gas flux rate are very firmly connected with the energy consumption of airlifting systems, see Eq. (7) (Yoshinaga and Sato, 1996).
* Proven technology
Proven technology, in this context, can be defined as the maturity of the utilised technology. A well-proven technology is one in which the initial operational faults and intrinsic problems have been reduced or eliminated through a long development and application process (Anuar et al., 2015; Chapman, 2016).
* Technology reliability
Technology reliability can be defined as the technical property of continuous, correct functionality according to the particular specifications of that technology. A reliable technology has a high probability of completing its objective under a normal working condition (Johnson and Ettlie, 2001).
* Production rate
For CLB systems, production rate is determined by the cable lifting velocity and bucket dimensions, see Eq. (8) (Schulte, 2013).
For AL systems, the mineral production rate can be calculated using the flux rate of solid particles and cross-sectional area of lifting pipes, see Eq. (9) (Yoshinaga and Sato, 1996).
* Technology availability
Technology availability refers to the possibility and accessibility of utilising the technology in DSM specific working conditions with the correct format (Pick et al., 2013).
* Gross income
Gross income represents the income derived from mineral extraction in DSM activities. This can be calculated as follows (Ma et al., 2017a):
* Capital cost
Capital cost can be explained as the one-time expenditure involved in purchasing equipment and construction infrastructure for production. It is the entire initial expenditure required to bring a DSM project to a commercially operable status (UKessays, 2018).
* Operation and maintenance cost
Operation and maintenance cost is defined as the expenditure required to support the normal running of a DSM project, which covers maintenance costs (in repairing and replacing equipment), labour costs and energy consumption costs (Nyhart et al., 1978; Sharma, 2011). Nyhart et al. (1978) defined the subcategories of both capital cost and operation and maintenance cost as those relating to three sectors: mining, transport and processing, see Eq. (11).
The subscripts of m , t , p represent the mining, transport, and processing sectors, respectively.
* Investment recovery period
The investment recovery period, also known as the ‘payback period’, refers to the period needed to recover the investment in a DSM project. It is an important economic parameter in determining whether a DSM project is profitable or not, and DSM project investors – unsurprisingly – prefer an investment recovery period to be as small as possible (Investopedia, 2018). Investment recovery period is determined by comparing the net cash inflow with net cash outflow. In this paper, it can be simply understood as the period when the gross income is larger than the initial capital cost, and operation and maintenance cost.
* Species disturbance variance
Species disturbance variance is an integrated ecological index, comparing species conditions before and after DSM activities. It can be calculated as follows (Ma et al., 2018a):
in which the subscripts of aft and bef reflect species condition both after and before DSM disturbances, respectively, SD represents the integrated species index, incorporating Margalef’s indices, and both the Shannon and Simpson diversity indexes, see Eq. (13).
* Severity of ill effect
Severity of ill effect is another important ecological parameter, first proposed by Newcombe and Jensen (1996) through a meta-analysis, which is introduced in DSM activities (Ma et al., 2018a). The severity of ill effect is defined in Table 2.
Table 2 Severity of ill effect on marine species (Newcombe and Jensen, 1996).
Severity of ill effect can be calculated using Eq. (14) (Newcombe and Jensen, 1996).
in which the sediment plume mass concentration and residence time can be determined by solving the plume advection-diffusion model (Ma et al., 2018b).
* Turbidity of ocean water
Based on the literature review, most aquatic species have a preferred ocean turbidity range (Dyer, 1972; Cyrus and Blaber, 1987a). To keep the impact on benthic species community within an environmentally acceptable range, the changing rate of the turbidity of ocean water should be constrained, see Eq. (15) (Cyrus and Blaber, 1987a; Cyrus and Blaber, 1987b).
* Total organic carbon content
Total organic carbon content is an important ecological indicator that can be significantly influenced by DSM activities (Saleem et al., 2016). Similar to the turbidity of ocean water, minimising the impact on benthic ocean species, the changing rate of total organic carbon content should also be taken into consideration. The smaller the changing rate of total organic carbon content is, the smaller the caused environmental impacts are. The total organic carbon content can be calculated as Eq. (16).
* Sedimentation thickness
Benthic ocean species (such as coral, sponges, holothurian, and other macrofauna) have different resistance abilities to sedimentation burial thickness (Lohrer et al., 2006; Thrush and Dayton, 2002; Cummings et al., 2003). The larger the sedimentation thickness is, the more intensive the DSM impacts are on the ecological community. The most significant factor concerning both technological and environmental impact is the mineral production rate (Ma et al., 2018a).
* Social acceptability
Social acceptability is widely regarded as one of the most important parameters, which is closely related to the success of a DSM project (Brunson et al., 1996; Rosenbaum and Grey, 2016; Wakefield and Myers, 2018). In this paper, social acceptability is a qualitative parameter, the value of which is derived from expert opinions.
* Policy (legislation & regulation) support
Policy support refers to the possibility of support through marine policy, mining legislation, and regulation; this is also regarded as one of the most important parameters in the implementation of a DSM project (Lewis and Wiser, 2007; Rosenbaum and Grey, 2016; Wakefield and Myers, 2018; ISA, 2022c).
After collating experts’ opinions provided through the completion of the questionnaire, the data were checked through the functions of consistency index (CI) and consistency ratio (CR), as Eq. (1-2). The weights of the major criteria, including technological (TEC), economic (ECO), environmental (ENV), and social (SOC) aspects, can then be determined (assuming independence of one another).
Following this, the interconnections (or dependencies) between the major criteria can be examined, see Figure 4 (Ma et al., 2017c). Depending on the pairwise comparison matrices derived from the experts’ opinions, dependency weight vectors for technological, economic, environmental, and social factors can be listed subsequently.
Figure 4 Diagram reflecting the interconnection relationships between the major criteria (Ma et al., 2017c).
The interconnection matrix for the major criteria is determined as follows:
Weighs for the major criteria can now be calculated, considering their interconnections.
Comparing the weights of the major criteria through considering the interconnection relationship or not, i.e., w1 and w7 , we found that the order of the major criteria weights was unchanged, i.e., ECO>TEC>ENV>SOC . However, following considering of the interconnection relationships, the evaluation criteria (e.g., social impact consideration, which can be easily overlooked) have been evaluated more objectively and fairly.
Weights determinations for sub-criteria follow the similar principles to those of the major criteria. However, in order to reduce the difficulty of data collection and to reduce the number of calculations, we treated the evaluation sub-criteria as independent of each other. The sub-criteria for each major criterion are listed as follows. Technological: energy consumption (EC), proven technology (PT), technology reliability (TR), production rate (PR), and technology availability (TA). Economic: gross income (GI), capital cost (CC), operation and maintenance cost (OM), and investment recovery period (IRP). Environmental: species disturbance variances (SD), severity of ill effect (SIE), turbidity of ocean water (TOW), total organic carbon content (TOC), and sedimentation thickness (ST). Social: social acceptability (SA) and policy support (PS). The weights for all sub-criteria can be calculated based on the pairwise comparison matrices, as follows:
Global weights for all evaluation sub-criteria (w12 ) can be determined by multiplying the individual weights, i.e., w8−11 , by the major criteria weight, i.e., w7 , see Figure 5.
In this paper, besides the quantitative evaluation criteria, there are also a series of qualitative evaluation criteria. As described in Table 1, the qualitative evaluation criteria consist of proven technology (PT), technology reliability (TR), technology availability (TA), social acceptability (SA), and policy support (PS). Values for each of these qualitative parameters, based on questionnaire date, are derived for each of the vertical lifting technologies in DSM activities (CLB, HL, and AL). As we all known, the most accurate evaluations of these qualitative criteria should be combined with the specific DSM transport plans. Here, in this paper, the scores of these qualitative evaluating parameters for all DSM transport plans are simplified to be directly connected with the vertical lifting technologies. Based on the collected data through questionnaire, the scores of all these DSM transport plans in terms of proven technology can be calculated using the Mean of Maxima (MOM) defuzzification method (Özdemir, 2010).
Following the same principle, the scores for the other qualitative evaluation parameters are listed as follows:
Before the application of MCDM to DSM vertical transport plan evaluation, two assumptions are first made, as follows: (i) the minerals storage richness is sufficient for the whole mining period, and (ii) reduction in marine species numbers (with the same temporal and spatial scales) is simplified to be related to the mineral production rate (see Table 3).
The parameters involved in the MCDM methodology for selecting a sustainable DSM vertical transport plan are presented in Table 3.
The DSM vertical transport plans with different changing variables are listed as Table 4.
Using the numerical calculation theories and equations presented in section 3, the quantitative parameters for DSM vertical transport plans can be calculated. Combining the results of the quantitative evaluation criteria with the qualitative evaluation criteria allows for comprehensive assessment for all DSM vertical transport plans through MCDM. These are listed in Appendix B. The preference value trend for all evaluation criteria is presented in Table 5.
As the evaluation criteria have different preferable trends and desired values, prior to the specific operations of the evaluation process of all DSM vertical transport plans, the evaluation matrix of these DSM transport plans should first be normalised (Mateo, 2012). Vafaei et al. (2016) examined the use of normalization techniques in analytic hierarchy process method (AHP), and here we extend their findings to the Fuzzy-ANP method presented in this paper. Except the unsuitable logarithmic normalisation method, the combined application of Linear-Max normalisation and Linear-Sum normalisation is selected to be used, see Eq. (25) (Vafaei et al., 2016).
After the normalisation process with Eq. (25), the super-matrix of all DSM vertical transport plans is changed into the following format, i.e., MDSM :
Finally, scores for all DSM vertical transport plans can be determined by multiplying the normalisation matrix, i.e., Eq. (26), by the global weights of all evaluation criteria, i.e.,
w12.
The scores for all evaluated DSM vertical transport plans are presented in Figure 6.
Figure 6 Diagrammatic representation of the evaluated DSM transport plan scores. Note: Transport plan (TR) No. 15 represents the maximum score and is marked in the figure with a score of 0.0376. TRs. No (1-10), (11-20) and (21-32) are CLB, HL and AL systems, respectively.
Analysing Figure 6, it clearly expresses the following information: (i) Transport plan No. 15 possesses the maximum calculated score, i.e., 0.0376, and thus represents the most sustainable DSM transport plan on the basis of the technological, economic, environmental, and social considerations analysed. Transport plan No. 15 utilizes centrifugal pump lifting minerals, working period 36 weeks per year, lifting pipe diameter 0.30 m. Transport plan No. 15 is not the working plan with the maximum gross income; neither does it have the least environmental impact. However, it is the working plan with the highest integrated evaluating index based on the multiple criteria evaluating results. The transport plan comprehensively considers factors including environmental, economic, technological, and social aspects, and combines qualitative and quantitative analysis and assessment indicators to obtain a comprehensive evaluation score. Therefore, Transport plan No. 15 is suggested to be the most preferable or sustainable vertical transport plan for the selected DSM working condition. (ii) Compared to continuous line bucket lifting and airlifting systems, centrifugal pump lifting system proves to be more preferable, with relative larger evaluation scores. The technology of centrifugal pump lifting is also the most widely used in deep sea mining trials and experimental projects, and the lifting technology is more mature with a higher degree of mechanical automation for continuous ore lifting. It is widely used in land mining and dredging projects, which provides a lot of references for deep sea mining (Hu et al., 2021; Kang et al., 2022). These scores can be explained as follows: (a) centrifugal pump lifting system may achieve a larger solid production rate at a depth of 2000 m than continuous line bucket lifting and airlifting systems. This advantage will be expanded with the increase of mining water depth (Ma et al., 2017a). (b) For airlifting systems, mineral particle velocity may be a problem in achieving a relatively large mineral production rate. In airlifting systems, pressures inside the pipe lifting system decrease with the continuous lifting of minerals. The volumetric concentration of mineral particles can be very small, which will lead to a fast-moving velocity of solid particles (roughly 20 – 80 m/s) (Ma et al., 2017b). (c) Compared to centrifugal pumps – that can be added along the pipe lifting system to improve its mining capacity – steel rope strength is an important limiting factor in a continuous line bucket lifting system, which limits both its maximum lifting depth and maximum solid production rate (Ma et al., 2017a). Different lifting technologies are limited by the most advanced structural design, construction materials, craftsmanship and work plan. Technological innovation in one of the above certain aspects has far-reaching significance for promoting the promotion of deep-sea mining.
In future applications of this evaluating system, a large number of DSM vertical transport plans could be calculated in order to determine an optimal sustainable working plan. In addition, the evaluation method can be improved in combination with some artificial intelligence algorithms (such as artificial neural network methods, etc.) in the future to realize self-learning and self-correction of data, and find the optimal solution more accurately (Ma et al., 2022). However, this would involve an extremely large workload when applying Fuzzy-ANP evaluating methods (Yüksel and Dagdeviren, 2007). In order to improve the multiple criteria evaluating efficiency, a pre-filtering process could be used to reduce the number of evaluated DSM transport plans to be included in the model. For instance, if the DSM working period is due to be 20 years, the investors would like a large profitability. It means the investment recovery period should be smaller than the mining period. On analysis of Figure 7, it becomes apparent that transport plans No. 1-3 could be excluded from the evaluation process because these DSM working conditions are unprofitable in this given timeframe. This operation can greatly reduce the substandard evaluation database, thereby speeding up the acquisition of the optimal transport plan. Additionally, as suggested by Ma et al. (2018a) in a paper examining the environmental impacts of DSM using a numerical calculation method combined with in-situ tests, an environmental impact standard or acceptance threshold should be established in order to allow the greatest degree of ecological recovery possibility. The environmental impact standard considers physical disturbances, species disturbances, turbidity of ocean water change, sedimentation rate, and sedimentation thickness. Outcomes are measured in terms of species mortality, the mass concentration of sediment plume, and its residence time (Van Dover, 2011; Jones et al., 2017). These environmental impact standards facilitate the exclusion of many substandard transport plans, thereby further reducing the potential workload and allowing for more accurate and concise identification of the most preferable working condition.
Figure 7 Investment recovery period (IRP) of all evaluated DSM vertical transport plans. An IRP of these transport plans less than 20 years is represented by the orange bars; the red bar is the most preferable and sustainable DSM vertical transport plan; the IRP of the transport plan No. (1-3) is equal to, or greater than 20 years. Here, they are simplified as 20 years and represented by the blue bars.
Furthermore, in the evaluation of sustainable DSM vertical transport plans, it is notable that economically advantageous evaluation parameters often have conflicting results with environmentally advantageous evaluation parameters. An economically advantageous DSM transport plan may have a relatively poor environmental impact performance and vice versa. One of the characteristics of the MCDM method is that it can deal with conflicting and dependent evaluation parameters. In the case of multiple objectives and constraints, the optimal balance point between the evaluation parameters is clarified by systematic mathematical methods. Figure 8 illustrates this, presenting the normalised scores for gross income and sedimentation thickness (parameters belonging to economic and environmental impact considerations, respectively). Gross income is considered by many businesses to be one of the most important indicators of economic profitability, reflecting the company’s development trends and health. Here, the parameter of sedimentation thickness represents the thickness of the sediment plume redeposited on the seafloor. This parameter can reflect the damage degree of the seafloor disturbance and directly reflect the severity of the sediment plume. The redeposit that exceeds a certain thickness has a fatal threat to the soft-bodied organisms living on the seabed. Therefore, this parameter is also an important indicator in the field of deep-sea mining environmental assessment. The normalised values can be understood as the preferable levels of all DSM transport plans in terms of all these selected evaluating criteria. Figure 8 illustrates that the normalised values for gross income demonstrate a negative correlation with those for sedimentation thickness. That reflects why, in many industrial working conditions, a larger economic profit can be obtained at the expense of the environment (Ghisellini et al., 2016; Panayotou, 2016). Through a systematic use of multi-criteria decision making method, we have tried to find the optimal balance of all included evaluation criteria – encompassing technological, economic, environmental, and social perspectives. Deep sea mining multinational companies can use this method to conduct a systematic and objective evaluation of different mining options. It has shown to environmental protection agencies and organizations around the world that the method has made sufficient research on the bearing capacity of the environment. Its greatest role is to ensure that the development of seabed mineral resources is in line with sustainable development modes and in line with environmental standards set by the International Seabed Authority in the future.
Figure 8 Normalised scores of gross income and sedimentation thickness. The red points represent the most preferable and sustainable DSM transport plan.
In this paper, Fuzzy-ANP method is utilised for sustainability assessment in DSM vertical transport plans. The decision-making criteria constituting the technological, economic, environmental, and social components of these plans possess both quantitative and qualitative attributes. The application of Fuzzy-ANP methodology is demonstrated in section 4. The MCDM results show that transport plans involving centrifugal pump lifting are more competitive compared to bucket lifting and airlifting systems. It can also be concluded that economically advantageous evaluation parameters often demonstrate a conflicting relationship with environmentally advantageous evaluating parameters. Although this paper focuses on the utility of the proposed framework in vertical lifting technologies, the basic model after considering more key components (such as real-time data of deep-sea environment and ecology, complex seabed environmental loads, specific mining spatial and temporal conditions, and specific seabed organisms, etc.) of sustainability evaluation may allow for sustainability assessment of DSM projects as a whole.
Future improvements of the proposed framework could include (i) consideration of the interconnections of evaluation sub-criteria, (ii) sustainability assessment of whole DSM projects, and (iii) global optimisations of DSM working conditions.
The original contributions presented in the study are included in the article/supplementary material. Further inquiries can be directed to the corresponding author.
Ethical review and approval was not required for the study of human participants in accordance with the local legislation and institutional requirements. Written informed consent from the participants was not required to participate in this study in accordance with the national legislation and the institutional requirement.
WM is responsilbe for the paper writing, KZ, YD, YS are responsible for the paper editing and reviewing. All authors contributed to the article and approved the submitted version.
This work was supported by Key Project of National Natural Science Foundation of China [research project number 52231012], Hainan University High-level Talents Research Start-up Funding [grant number KYQD(ZR)-22060], International Association of Maritime Universities [research project number 20220304]. We would also like to thank for the contributions from Prof. Cees van Rhee, Prof. Dingena Schott and Prof. Xiangwei Liu.
The authors declare that the research was conducted in the absence of any commercial or financial relationships that could be construed as a potential conflict of interest.
All claims expressed in this article are solely those of the authors and do not necessarily represent those of their affiliated organizations, or those of the publisher, the editors and the reviewers. Any product that may be evaluated in this article, or claim that may be made by its manufacturer, is not guaranteed or endorsed by the publisher.
The Supplementary Material for this article can be found online at: https://www.frontiersin.org/articles/10.3389/fmars.2022.1009834/full#supplementary-material
Abdel-Basset M., Gamal A., Chakrabortty R. K., Ryan M. (2021). A new hybrid multi-criteria decision-making approach for location selection of sustainable offshore wind energy stations: A case study. J. Cleaner Production 280, 124462. doi: 10.1016/j.jclepro.2020.124462
Afgan N. H., Carvalho M. G. (2008). Sustainability assessment of a hybrid energy system. Energy Policy 36 (8), 2903–2910. doi: 10.1016/j.enpol.2008.03.040
Akash B. A., Mamlook R., Mohsen M. S. (1999). Multi-criteria selection of electric power plants using analytical hierarchy process. Electric Power Syst. Res. 52 (1), 29–35. doi: 10.1016/S0378-7796(99)00004-8
Amon D. J., Gollner S., Morato T., Smith C. R., Chen C., Christiansen S., et al. (2022). Assessment of scientific gaps related to the effective environmental management of deep-seabed mining. Mar. Policy 138, 105006. doi: 10.1016/j.marpol.2022.105006
Anuar N., Kahar W. S. W. A., Manan J. A. N. A. (2015). “Defining the “proven technology” technical criterion in the reactor technology assessment for malaysia’s nuclear power program,” Advancing of nuclear science and energy for national development, AIP Conference Proceedings, Vol. 1659. (Skudai, Johor, Malaysia: AIP Publishing LLC).
Bauch D., Cherniavskaia E. (2018). GEOMAR Helmholtz centre for ocean research Kiel, wischhofstr (38 Bering str., St. Petersburg 199397, Russia: 24148 Kiel, Germany 2 Arctic and Antarctic Research Institute), 1–3.
Begić F., Afgan N. H. (2007). Sustainability assessment tool for the decision making in selection of energy system–Bosnian case. Energy 32 (10), 1979–1985. doi: 10.1016/j.energy.2007.02.006
Boran F. E., Genç S., Kurt M., Akay D. (2009). A multi-criteria intuitionistic fuzzy group decision making for supplier selection with TOPSIS method. Expert Syst. Appl. 36 (8), 11363–11368. doi: 10.1016/j.eswa.2009.03.039
Brunson M. W., Kruger L. E., Tyler C. B., Schroeder S. A. (1996). Defining social acceptability in ecosystem management: workshop proceedings (Portland: United States Department of Agriculture. Forest Service. Pacific Northwest Research Station).
Campos-Guzmán V., García-Cáscales M. S., Espinosa N., Urbina A. (2019). Life cycle analysis with multi-criteria decision making: A review of approaches for the sustainability evaluation of renewable energy technologies. Renewable Sustain. Energy Rev. 104, 343–366. doi: 10.1016/j.rser.2019.01.031
Carli R., Dotoli M., Pellegrino R. (2018). Multi-criteria decision-making for sustainable metropolitan cities assessment. J. Environ. Manage. 226, 46–61. doi: 10.1016/j.jenvman.2018.07.075
Carver R., Childs J., Steinberg P., Mabon L., Matsuda H., Squire R., et al. (2020). A critical social perspective on deep sea mining: Lessons from the emergent industry in Japan. Ocean Coast. Manage. 193, 105242. doi: 10.1016/j.ocecoaman.2020.105242
Chapman R. J. (2016). The rules of project risk management: Implementation guidelines for major projects (London: Routledge).
Chatzimouratidis A. I., Pilavachi P. A. (2009). Technological, economic and sustainability evaluation of power plants using the analytic hierarchy process. Energy Policy 37 (3), 778–787. doi: 10.1016/j.enpol.2008.10.009
Cummings V., Thrush S., Hewitt J., Norkko A., Pickmere S. (2003). Terrestrial deposits on intertidal sandflats: sediment characteristics as indicators of habitat suitability for recolonising macrofauna. Mar. Ecol. Prog. Ser. 253, 39–54. doi: 10.3354/meps253039
Cyrus D. P., Blaber S. J. M. (1987a). The influence of turbidity on juvenile marine fish in the estuaries of natal, south Africa. Continental Shelf Res. 7 (11-12), 1411–1416. doi: 10.1016/0278-4343(87)90046-X
Cyrus D. P., Blaber S. J. M. (1987b). The influence of turbidity on juvenile marine fishes in estuaries. part 1. field studies at lake st. Lucia on the southeastern coast of Africa. J. Exp. Mar. Biol. Ecol. 109 (1), 53–70. doi: 10.1016/0022-0981(87)90185-7
Drazen J. C., Smith C. R., Gjerde K. M., Haddock S. H., Carter G. S., Choy C. A., et al. (2020). Midwater ecosystems must be considered when evaluating environmental risks of deep-sea mining. Proc. Natl. Acad. Sci. 117 (30), 17455–17460. doi: 10.1073/pnas.2011914117
Dyer K. R. (1972). Sedimentation in estuaries. Estuar. Environ. 12, 32. doi: 10.1016/S0070-4571(05)80034-2
Erdogan S. A., Šaparauskas J., Turskis Z. (2019). A multi-criteria decision-making model to choose the best option for sustainable construction management. Sustainability 11 (8), 2239. doi: 10.3390/su11082239
Filer C., Gabriel J. (2018). How could nautilus minerals get a social licence to operate the world’s first deep sea mine? Mar. Policy 95, 394–400. doi: 10.1016/j.marpol.2016.12.001
Folkersen M. V., Fleming C. M., Hasan S. (2018). The economic value of the deep sea: A systematic review and meta-analysis. Mar. Policy 94, 71–80. doi: 10.1016/j.marpol.2018.05.003
Gerber L. J., Grogan R. L. (2020). Challenges of operationalising good industry practice and best environmental practice in deep seabed mining regulation. Mar. Policy, 114. doi: 10.1016/j.marpol.2018.09.002
Ghisellini P., Cialani C., Ulgiati S. (2016). A review on circular economy: The expected transition to a balanced interplay of environmental and economic systems. J. Cleaner Prod. 114, 11–32. doi: 10.1016/j.jclepro.2015.09.007
Hallgren A., Hansson A. (2021). Conflicting narratives of deep sea mining. Sustainability 13 (9), 5261. doi: 10.3390/su13095261
Haralambopoulos D. A., Polatidis H. (2003). Renewable energy projects: structuring a multi-criteria group decision-making framework. Renewable Energy 28 (6), 961–973. doi: 10.1016/S0960-1481(02)00072-1
Hoagland P., Beaulieu S., Tivey M. A., Eggert R. G., German C., Glowka L., et al. (2010). Deep-sea mining of seafloor massive sulfides. Mar. Policy 34 (3), 728–732. doi: 10.1016/j.marpol.2009.12.001
Ho W., Xu X., Dey P. K. (2010). Multi-criteria decision making approaches for supplier evaluation and selection: A literature review. Eur. J. operational Res. 202 (1), 16–24. doi: 10.1016/j.ejor.2009.05.009
Huang I. B., Keisler J., Linkov I. (2011). Multi-criteria decision analysis in environmental sciences: Ten years of applications and trends. Sci. total Environ. 409 (19), 3578–3594. doi: 10.1016/j.scitotenv.2011.06.022
Hu Q., Chen J., Deng L., Kang Y., Liu S. (2021). CFD-DEM simulation of backflow blockage of deep-sea multistage pump. J. Mar. Sci. Eng. 9 (9), 987. doi: 10.3390/jmse9090987
Investopedia (2018) Payback period. what is the ‘Payback period’. retrieved on Oct 1st. Available at: https://www.investopedia.com/terms/p/paybackperiod.asp.
ISA (2022a) Deep-ocean stewards initiative (DOSI): Jeff ardron, institute for advanced sustainability studies, Kristina gjerde, wycliffe management and IUCN, Malcolm Clark, NIWA, new Zealand Lisa a. Levin, Scripps institution of oceanography kathryn mengerink, enviro. Available at: https://www.isa.org.jm/deep-ocean-stewards-initiative-dosi-jeff-ardron-institute-advanced-sustainability-studies-kristina.
ISA (2022b) ISA-NOC expert meeting defines pathways to advance innovation and technology development for sustainable exploitation of deep-sea minerals in the area. Available at: https://www.isa.org.jm/news/isa-noc-expert-meeting-defines-pathways-advance-innovation-and-technology-development.
ISA (2022c) High-level event at HLPF 2021 | ensuring the sustainable management of deep-seabed resources for the benefit of humankind. Available at: https://www.isa.org.jm/event/side-event-hlpf2021-ensuring-sustainable-management-and-stewardship-deep-seabed.
Johnson M. D., Ettlie J. E. (2001). Technology, customization, and reliability. J. Qual. Manage. 6 (2), 193–210. doi: 10.1016/S1084-8568(01)00037-2
Jones D. O., Kaiser S., Sweetman A. K., Smith C. R., Menot L., Vink A., et al. (2017). Biological responses to disturbance from simulated deep-sea polymetallic nodule mining. PloS One 12 (2), e0171750. doi: 10.1371/journal.pone.0171750
Jovanović M., Afgan N., Radovanović P., & Stevanović V. (2009). Sustainable development of the Belgrade energy system. Energy 34 (5), 532–539. doi: 10.1016/j.energy.2008.01.013
Jungblut S., Liebich V., Bode M. (2018). YOUMARES 8–oceans across boundaries: Learning from each other: Proceedings of the 2017 conference for YOUng MARine RESearchers in Kiel, Germany (Cham: Springer Nature), 251.
Kahraman C. (2008). Fuzzy multi-criteria decision making: theory and applications with recent developments. Vol. 16 (Berlin: Springer Science & Business Media).
Kang Y., Su Q., Liu S. (2022). On the axial thrust and hydraulic performance of a multistage lifting pump for deep-sea mining. Ocean Eng. 265, 112534. doi: 10.1016/j.oceaneng.2022.112534
Khalili N. R., Duecker S. (2013). Application of multi-criteria decision analysis in design of sustainable environmental management system framework. J. Cleaner Production 47, 188–198. doi: 10.1016/j.jclepro.2012.10.044
Levin L. A., Amon D. J., Lily H. (2020a). Challenges to the sustainability of deep-seabed mining. Nat. Sustainability 3 (10), 784–794. doi: 10.1038/s41893-020-0558-x
Levin L., Mengerink K., Gjerde K., Rowden A., Van Dover C. L., Clark M. R., et al. (2016). Defining « serious harm » to the marine environment in the context of deep-seabed mining.Mar. Policy 74, 245–259. doi: 10.1016/j.marpol.2016.09.032
Levin L., Wei C.-L., Dunn D., Amon D., Ashford O., Cheung W., et al. (2020b). Climate change considerations are fundamental to management of deep-sea resource extraction. Glob. Change Biol. 26, 4664–4678. doi: 10.1111/gcb.15223
Lewis J. I., Wiser R. H. (2007). Fostering a renewable energy technology industry: An international comparison of wind industry policy support mechanisms. Energy Policy 35 (3), 1844–1857. doi: 10.1016/j.enpol.2006.06.005
Ligus M. (2017). Evaluation of economic, social and environmental effects of low-emission energy technologies development in Poland: A multi-criteria analysis with application of a fuzzy analytic hierarchy process (FAHP). Energies 10 (10), 1550. doi: 10.3390/en10101550
Lohrer A. M., Thrush S. F., Lundquist C. J., Vopel K., Hewitt J. E., Nicholls P. E. (2006). Deposition of terrigenous sediment on subtidal marine macrobenthos: response of two contrasting community types. Mar. Ecol. Prog. Ser. 307, 115–125. doi: 10.3354/meps307115
Madiraju K. S. (2019). Contemplating a domestic regulatory and enforcement framework for deep seabed mining. Natural Resour. Environ. 34 (2), 17–21. https://www.proquest.com/openview/91d0b7afcc1af82f3531806bde0f9506/1?pq-origsite=gscholar&cbl=46452
Ma W., Du Y., Liu X., Shen Y. (2022). Literature review: Multi-criteria decision-making method application for sustainable deep-sea mining transport plans. Ecol. Indic. 140, 109049. doi: 10.1016/j.ecolind.2022.109049
Mamlook R., Akash B. A., Nijmeh S. (2001). Fuzzy sets programming to perform evaluation of solar systems in Jordan. Energy Conversion Manage. 42 (14), 1717–1726. doi: 10.1016/S0196-8904(00)00152-7
Ma W., Schott D., Lodewijks G. (2017a). Continuous line bucket lifting versus pipe lifting. J. Offshore Mechanics Arctic Eng. 139 (5), 051704. doi: 10.1115/1.4036375
Ma W., Schott D., Lodewijks G. (2017c). A new procedure for deep-sea mining tailings disposal. Minerals 7 (4), 47. doi: 10.3390/min7040047
Ma W., Schott D., van Rhee C. (2018a). Numerical calculations of environmental impacts for deep-sea mining activities. Sci. Total Environ. 652, 996–1012. doi: 10.1016/j.scitotenv.2018.10.267
Ma W., van Rhee C., Schott D. (2017b). Technological and profitable analysis of airlifting in deep-sea mining systems. Minerals 7 (8), 143. doi: 10.3390/min7080143
Ma W., van Rhee C., Schott D. (2018b). A numerical calculation method of environmental impacts for the deep-sea mining industry–a review. Environ. Science: Processes Impacts 20 (3), 454–468. doi: 10.1039/C7EM00592J
Mateo J. R. S. C. (2012). ‘Vikor’. in Multi criteria analysis in the renewable energy industry (London: Springer), 49–53.
McDowall W., Eames M. (2007). Towards a sustainable hydrogen economy: A multi-criteria sustainability appraisal of competing hydrogen futures. Int. J. Hydrogen Energy 32 (18), 4611–4626. doi: 10.1016/j.ijhydene.2007.06.020
MiningImpact, W.P (2018). WP 5–communiaction with stakeholders, policymakers, off-shore mining industry. JPI-O: EcoMining-DEU–Ecological Aspects Deep-Sea Min. 53. Available at: https://oceanrep.geomar.de/id/eprint/46570/1/JPI-O%20EcoMining-DEU_AB.pdf#page=55.
Mining Weekly (2018) Retrieved on July 9th. Available at: http://www.miningweekly.com/article/deep-sea-mining-struggling-to-forge-ahead-amid-investor-and-environmental-concerns-2018-05-24/rep_id:3650.
Mohanty R. P., Agarwal R., Choudhury A. K., Tiwari M. K. (2005). A fuzzy ANP-based approach to R&D project selection: a case study. Int. J. Production Res. 43 (24), 5199–5216. doi: 10.1080/00207540500219031
(2018) Nautilus minerals. Available at: http://www.nautilusminerals.com/irm/content/production-support-vessel.aspx?RID=264.
Newcombe C. P., Jensen J. O. (1996). Channel suspended sediment and fisheries: a synthesis for quantitative assessment of risk and impact. North Am. J. Fisheries Manage. 16 (4), 693–727. doi: 10.1577/1548-8675(1996)016<0693:CSSAFA>2.3.CO;2
Nyhart J. D., Antrim L., Capstaff A. E., Kohler A. D., Leshaw D. (1978) A cost model of deep ocean mining and associated regulatory issues. nautilus minerals. Available at: http://www.nautilusminerals.com/irm/content/production-support-vessel.aspx?RID=264.
Nzotcha U., Kenfack J., Manjia M. B. (2019). Integrated multi-criteria decision making methodology for pumped hydro-energy storage plant site selection from a sustainable development perspective with an application. Renewable Sustain. Energy Rev. 112, 930–947. doi: 10.1016/j.rser.2019.06.035
Otte J. M., Wietz M., Volz J., Molari M., Janssen F., Wenzhöfer F. (2021). Spatial distribution of metal-cycling microbial communities along geochemical gradients in sediments of polymetallic nodule fields of the Eastern Pacific Ocean. Goldschmidt 2021, Virtual; July 4-9.
Özdemir M. (2010). A probabilistic schedule delay analysis in construction projects by using fuzzy logic incorporated with relative importance index (RII) method. Master's thesis. (Ankara, Turke: Middle East Technical University).
Pabortsava K., Lampitt R. S. (2020). High concentrations of plastic hidden beneath the surface of the Atlantic ocean. Nat. Commun. 11 (1), 1–11. doi: 10.1038/s41467-020-17932-9
Padhye N., Deb K. (2011). Multi-objective optimisation and multi-criteria decision making in SLS using evolutionary approaches. Rapid Prototyping J. 17 (6), 458–478. doi: 10.1108/13552541111184198
Panayotou T. (2016). Economic growth and the environment. Environ. Anthropol. 24, 140–148. doi: 10.18574/nyu/9781479862689.003.0018
Pang B. (2009). “A fuzzy ANP approach to supplier selection based on fuzzy preference programming,” in 2009 International Conference on Management and Service Science. International Conference on Beijing. (Beijing: IEEE), 1–4
Peng W., Duan T., Hou J., Guo W., Ding K., Cheng W., et al. (2021). Long-term corrosion behaviour of 1060 aluminium in deep-sea environment of south China Sea. Corrosion Engineering Sci. Technol. 56 (4), 327–340. doi: 10.1080/1478422X.2020.1861732
Pick J., Nishida T., Zhang X. (2013). Determinants of china’s technology availability and utilization 2006–2009: A spatial analysis. Inf. Soc. 29 (1), 26–48. doi: 10.1080/01972243.2012.739266
Pilavachi P. A., Stephanidis S. D., Pappas V. A., Afgan N. H. (2009). Multi-criteria evaluation of hydrogen and natural gas fuelled power plant technologies. Appl. Thermal Eng. 29 (11-12), 2228–2234. doi: 10.1016/j.applthermaleng.2008.11.014
Pohekar S. D., Ramachandran M. (2004). Application of multi-criteria decision making to sustainable energy planning–a review. Renewable Sustain. Energy Rev. 8 (4), 365–381. doi: 10.1016/j.rser.2003.12.007
Promentilla M. A. B., Furuichi T., Ishii K., Tanikawa N. (2008). A fuzzy analytic network process for multi-criteria evaluation of contaminated site remedial countermeasures. J. Environ. Manage. 88 (3), 479–495. doi: 10.1016/j.jenvman.2007.03.013
Rabbani A., Zamani M., Yazdani-Chamzini A., Zavadskas E. K. (2014). Proposing a new integrated model based on sustainability balanced scorecard (SBSC) and MCDM approaches by using linguistic variables for the performance evaluation of oil producing companies. Expert Syst. Appl. 41 (16), 7316–7327. doi: 10.1016/j.eswa.2014.05.023
Rezaei J. (2015). Best-worst multi-criteria decision-making method. Omega 53, 49–57. doi: 10.1016/j.omega.2014.11.009
Roche C., Bice S. (2013). “Anticipating social and community impacts of deep-sea mining.” in Deep sea minerals green economy secretariat pacific community suva, 59–80. Available at: https://www.researchgate.net/profile/Charles-Roche/publication/333608904_Anticipating_Social_and_Community_Impacts_of_Deep_Sea_Mining/links/5cf668d3299bf1fb18567573/Anticipating-Social-and-Community-Impacts-of-Deep-Sea-Mining.pdf.
Rosenbaum H., Grey F. (2016) Accountability zero; a critique of the nautilus mineral environmental and social benchmarking analysis of the solwara 1 project. Available at: http://www.deepseaminingoutofdepth.org/wp-content/uploads/accountabilityZERO_web.pdf.
Saaty T. L. (1996). Decision making with dependence and feedback: The analytic network process Vol. 4922 (Pittsburgh: RWS publications).
Saaty T. L. (2005). Theory and applications of the analytic network process: decision making with benefits, opportunities, costs, and risks (Pittsburgh: RWS publications).
Saleem M., Fetzer I., Harms H., Chatzinotas A. (2016). Trophic complexity in aqueous systems: bacterial species richness and protistan predation regulate dissolved organic carbon and dissolved total nitrogen removal. Proc. R. Soc. B: Biol. Sci. 283 (1825), 20152724. doi: 10.1098/rspb.2015.2724
Sharma R. (2011). Deep-sea mining: Economic, technical, technological, and environmental considerations for sustainable development. Mar. Technol. Soc. J. 45 (5), 28–41. doi: 10.4031/MTSJ.45.5.2
Shook C. A., Bartosik A. S. (1994). Particle–wall stresses in vertical slurry flows. Powder Technol. 81 (2), 117–124. doi: 10.1016/0032-5910(94)02877-X
Tilot V. (2010). Biodiversity and distribution of faunal assemblages. Vol1, Vol2, vol 3 options for the management and conservation of the nodule ecosystem in the clarion clipperton fracture zone (Switzerland: Project Unesco COI/Min Vlanderen). Available at: http://unesdoc.unesco.org/images/0014/001495/149556e.pdf223.
Tilot V. (2019). “Assessment of deep-sea faunal communities-indicators of environmental impact,” in Environmental issues of deep-sea mining (Cham: Springer), 147–189.
Tilot V., Ormond R., Moreno-Navas J., Catala T. (2018). The benthic megafaunal assemblages of the CCZ (Eastern pacific) and an approach to their management in the face of threatened anthropogenic impacts. Front. Mar. Sci. 5. doi: 10.3389/fmars.2018.00007
Tilot V., Willaert K., Guilloux B., Chen W., Mulalap C. Y., Gaulme F., et al. (2021). Traditional dimensions of seabed resource management in the context of deep Sea mining in the pacific: Learning from the socio-ecological interconnectivity between island communities and the ocean realm. Front. Mar. Sci 8. doi: 10.3389/fmars.2021.637938
Titus G. J., Liberatore M. J. (1991). Auditing R&D planning. IEEE Trans. Eng. Manage. 38 (2), 171–177. doi: 10.1109/17.78414
Thrush S. F., Dayton P. K. (2002). Disturbance to marine benthic habitats by trawling and dredging: implications for marine biodiversity. Annu. Rev. Ecol. Syst., 449–473. doi: 10.1146/annurev.ecolsys.33.010802.150515
UKessays (2018) What factors of production can affect a construction project? retrieved on Oct 1st. Available at: https://www.ukessays.com/essays/economics/what-factors-of-production-can-affect-a-construction-project-economics-essay.php.
Vaghela B. N., Parmar M. G., Solanki H. A., Kansara B. B., Prajapati S. K., Kalubarme M. H. (2018). Multi criteria decision making (MCDM) approach for mangrove health assessment using geo-informatics technology. Int. J. Environ. Geoinformatics 5 (2), pp.114–pp.131. doi: 10.30897/ijegeo.412511
Vafaei N., Ribeiro R. A., Camarinha-Matos L. M. (2016). “Normalization techniques for multi-criteria decision making: Analytical hierarchy process case study.” in Doctoral conference on computing, electrical and industrial systems. (Cham: Springer), 261–269.
Van Dover C. L. (2011). Tighten regulations on deep-sea mining. Nature 470 (7332), 31. doi: 10.1038/470031a
Wakefield J. R., Myers K. (2018). Social cost benefit analysis for deep sea minerals mining. Mar. Policy 95, 346–355. doi: 10.1016/j.marpol.2016.06.018
Xu L., Yang J. B. (2001). Introduction to multi-criteria decision making and the evidential reasoning approach (Manchester: Manchester School of Management), 1–21.
Yedla S., Shrestha R. M. (2003). Multi-criteria approach for the selection of alternative options for environmentally sustainable transport system in Delhi. Transportation Res. Part A: Policy Pract. 37 (8), 717–729. doi: 10.1016/S0965-8564(03)00027-2
Yoshinaga T., Sato Y. (1996). Performance of an air-lift pump for conveying coarse particles. Int. J. multiphase Flow 22 (2), 223–238. doi: 10.1016/0301-9322(95)00067-4
Yüksel İ., Dagdeviren M. (2007). Using the analytic network process (ANP) in a SWOT analysis–a case study for a textile firm. Inf. Sci. 177 (16), 3364–3382. doi: 10.1016/j.ins.2007.01.001
Zhang K., Nieto A., Kleit A. N. (2015). The real option value of mining operations using mean-reverting commodity prices. Mineral Economics 28 (1-2), 11–22. doi: 10.1007/s13563-014-0048-6
Zhou X. (2012). “Fuzzy analytical network process implementation with matlab,” in MATLAB-a fundamental tool for scientific computing and engineering applications, vol. 3. (Rijeka, Croatia: InTech).
Zionts S. (1979). MCDM–if not a roman numeral, then what? Interfaces 9 (4), 94–101. doi: 10.1287/inte.9.4.94
Keywords: decision support, deep-sea mining, sustainable, fuzzy analytic network process method, multi-criteria decision making
Citation: Ma W, Du Y, Zhang K and Shen Y (2022) Application of multi-criteria decision making to sustainable deep-sea mining vertical transport plans. Front. Mar. Sci. 9:1009834. doi: 10.3389/fmars.2022.1009834
Received: 02 August 2022; Accepted: 20 October 2022;
Published: 14 November 2022.
Edited by:
Kum Fai Yuen, Nanyang Technological University, SingaporeReviewed by:
Virginie C. Tilot, Muséum National d’Histoire Naturelle, FranceCopyright © 2022 Ma, Du, Zhang and Shen. This is an open-access article distributed under the terms of the Creative Commons Attribution License (CC BY). The use, distribution or reproduction in other forums is permitted, provided the original author(s) and the copyright owner(s) are credited and that the original publication in this journal is cited, in accordance with accepted academic practice. No use, distribution or reproduction is permitted which does not comply with these terms.
*Correspondence: Yijun Shen, OTk1MzkwQGhhaW5hbnUuZWR1LmNu
Disclaimer: All claims expressed in this article are solely those of the authors and do not necessarily represent those of their affiliated organizations, or those of the publisher, the editors and the reviewers. Any product that may be evaluated in this article or claim that may be made by its manufacturer is not guaranteed or endorsed by the publisher.
Research integrity at Frontiers
Learn more about the work of our research integrity team to safeguard the quality of each article we publish.