- 1Faculty of Fisheries and Protection of Waters, South Bohemian Research Center of Aquaculture and Biodiversity of Hydrocenoses, Research Institute of Fish Culture and Hydrobiology, University of South Bohemia in České Budějovice, České Budějovice, Czechia
- 2Department of Cell Biology and Histology, Faculty of Biology, University of Murcia, Murcia, Spain
The present study investigated the effect of partial substitution of soybean meal (SM) for fish meal (FM) with or without addition of inulin and oligofructose in pikeperch feed. A diet containing FM was considered as the basal diet, and then three other diets were prepared by: 1) replacing 50% of FM with SM (SM50), 2) replacing 50% of FM with SM and supplementation of 2% inulin (SMI50), and 3) replacing 50% of FM with SM and supplementation of 2% oligofructose (SMO50). Each diet was fed twice daily to triplicate groups of fish (36.68 ± 0.36 g) for eight weeks. The group fed SMO50 showed the highest weight gain (WG; 85.85 ± 4.46%) among the groups fed SM, with no significant difference from the FM group (79.74 ± 2.04%; p > 0.05). Specific growth rate (SGR) showed no significant differences among fish fed SMI50 (0.81 ± 0.07%), SMO50 (1.01 ± 0.09%) and FM (1.05 ± 0.02%). However, a lower SGR (0.69 ± 0.09%) was observed in the SM50 group without the supplementation of 2% inulin or oligofructose compared to the group fed FM (p < 0.05). The groups fed SMI50 and SMO50 showed no significant difference in FCR (1.23 ± 0.10; 0.91 ± 0.05, respectively) compared to the group fed FM (0.97 ± 0.04). While, a significant difference in FCR was found between the SM50-fed group without supplementation of inulin or oligofructose (1.50 ± 0.13) and the group fed FM (0.97 ± 0.04). Survival rate and whole-body composition showed no significant difference among all groups (p > 0.05). A significant decrease in serum total cholesterol concentration was observed in the SMO50 group (2.10 ± 0.29 mmole L-1) compared to the FM-fed group (3.33 ± 0.33 mmole L-1). Serum triglyceride showed a significant decrease in the groups fed SMI50 (0.98 ± 0.17 mmole L-1) compared to the group fed SMO50 (1.93 ± 0.24 mmole L-1). The concentrations of other serum biochemical parameters (total protein, glucose, lactate dehydrogenase, alanine aminotransferase, aspartate aminotransferase, and alkaline phosphatase) were not significantly affected by the dietary treatments (p > 0.05). Among all innate immunity and antioxidant parameters, only the serum malondialdehyde (MDA) concentration of SM50-fed fish (4.25 ± 0.7 nmole mL−1) was significantly lower than in the FM-fed group (with the highest MDA concentration; 25.17 ± 3.13 nmole mL−1). Serum total antioxidant capacity (T-AOC), superoxide dismutase (SOD), and catalase (CAT) were not significantly affected by the feeding (p > 0.05). Serum D-lactate concentration was not significantly affected by the dietary treatments (p > 0.05). No significant differences were found in the relative expression of IGF-I, IGF-II, GHR genes among the studied groups (p> 0.05). The results of the present study show that 50% replacement of FM with SM supplemented with a small amount of oligofructose (2% of dry matter) does not compromise the growth performance or the immune system of pikeperch. This substitution is feasible and provides a reference for cost-optimized design of feed formulation for pikeperch.
1 Introduction
Pikeperch, Sander lucioperca (Linnaeus, 1758), is considered a species of great interest for aquaculture due to its rapid growth, high nutritional value of the meat, and high commercial value (Jankowska et al., 2003; Pěnka et al., 2021), with growing interest in intensive culture development in European countries such as Poland, Netherland and Czech Republic (Klein Breteler, 1989; Zakes, 1999; Ostaszewska et al., 2005; Schulz et al., 2006; Hamza et al., 2007; Kestemont et al., 2007; Policar et al., 2019; Imentai et al., 2022). The pikeperch is the most important predator in many aquatic ecosystems and is valued for its ability to drive unwanted fish species out of water bodies (Adámek and Opacak, 2005; Specziár, 2011). Pikeperch predation is concentrated on the species most abundant in the local community (Lappalainen et al., 2006), and cyprinids are a common stomach content (Peltonen et al., 1996).
To meet the protein requirement for adequate growth rate and feed conversion of pikeperch, at least 43% protein is required (Nyina-Wamwiza et al., 2005). This protein requirement has traditionally been met with fishmeal (FM) (Tacon and Metian, 2008) which is an excellent source of protein due to the essential amino acids, minerals, and nucleotides required for commercial production of carnivorous fish (Oliva-Teles et al., 2015). Increasing demand and competition with other users, dwindling supply, and high price have encouraged the search for more sustainable alternative ingredients to partially or fully replace FM (Lunger et al., 2007; Peng et al., 2013). In this regard, a wide range of protein sources have been evaluated to find sustainable alternative ingredients as the main protein source of plant origin (including soybeans, wheat, peas, grains or oilseeds) and animal origin (poultry meal, blood meal, feather meal) (Gatlin et al., 2007; Li et al., 2011; Badillo-Zapata et al., 2014; Pares-Sierra et al., 2014; Tangendjaja, 2015; Wang et al., 2017; Tran et al., 2019; Ye et al., 2019; Li et al., 2020).
Among all plant-based candidates, soybean meal (SM) has been suggested as one of the most promising alternatives to replace FM due to its comparatively balanced amino acid profile, high digestibility, reasonable price, sustainable supply chain, and good availability (Wang et al., 2006; Gatlin et al., 2007; Imorou Toko et al., 2008; Yue and Zhou, 2008; Lin and Luo, 2011).
Despite considerable success in partially or completely replacing fishmeal with soybean meal in fish feed (Al-Kenawy et al., 2008; Ajani et al., 2016; Rahimnejad et al., 2021; Badillo-Zapata et al., 2021), the problems with palatability, digestion, nutrient utilization, and antinutritional factors (ANFs) such as β-conglycinin, protease inhibitors, and oligosaccharides continue to limit the substitution of SM for FM in carnivorous fish diets (Mambrini et al., 1999; Papatryphon and Soares, 2001; Peres et al., 2003; Zhou et al., 2005). It is well established that high levels of SM in the diet of carnivorous fish often have negative impacts on growth rate, protein and lipid metabolism, and health status (Refstie et al., 1998; Mambrini et al., 1999; Chou et al., 2004; Tomas et al., 2005; Zhou et al., 2005; Choi et al., 2020). In addition, the inclusion of SM in fish diets can impair immune function and lead to characteristic features of intestinal inflammation and/or lesions, and abnormal vacuolization (Baeverfjord and Krogdahl, 1996; Burrells et al., 1999; Hedrera et al., 2013; Wang et al., 2016; Kokou et al., 2017).
Prebiotics are non-digestible carbohydrates that are used as dietary supplements to mitigate the effects of various anti-nutritional factors and reduce the adverse effects of replacement diets, such as diets formulated with SM (Li and Gatlin, 2004; Li and Gatlin, 2005; Burr et al., 2008). In addition, they positively affect innate immune responses via modification of the gastrointestinal microbial community (López Nadal et al., 2020), improve oxidative status by reducing oxidative damage or increasing antioxidant potential (Zhang et al., 2013; Zhang et al., 2014; Guerreiro et al., 2015), increase mineral absorption (Bongers and van den Heuvel, 2003), and improve growth rate and feed conversion (Pattipeiluhu et al., 2020).
According to previous studies, inulin and oligofructose are among the most widely used prebiotics as feed ingredients worldwide (Van Loo et al., 1999; Ringø et al., 2010). Undoubtedly, these prebiotics possess several functional and nutritional properties (Such as: fiber enrichment, reduction of serum triglycerides and blood cholesterol, bifidogenic effect, and short-chain oligomers and high solubility) that are beneficial in feed formulation (Niness, 1999) and may have a positive impact on the health status and the immune system (Watzl et al., 2005; Cerezuela et al., 2008), growth rates and/or survival (Samrongpan et al., 2008; Hoseinifar et al., 2011b), blood profiles (Hoseinifar et al., 2011a), and gastrointestinal microbiota (Ringø et al., 2006; Bakke-McKellep et al., 2007) of aquatic animals, including various fish species.
Despite the availability of some commercial aquafeeds for percids, which are mostly salmonid-targeted feeds (Stejskal et al., 2016), attention has been drawn to the inadequate formulation of specific feeds for percid fishes. In order to develop sustainable feeds for these species, their nutritional needs must be considered (Bochert, 2020). Therefore, in this study we investigated the effect of fish meal substitution by soybean meal (50%), with or without the addition of inulin and oligofructose, on growth, immune parameters, and expression of growth-related genes in pikeperch.
2Materials and methods
2.1 Experimental design and diet preparation
The formulation and composition of the diets are shown in Table 1. The prebiotics inulin (with >90% purity) and oligofructose (with >95% purity) were sourced from BENEO GmbH, Germany. A basal diet was formulated using FM (45% crude protein and 12% crude lipid). Three other diets were prepared by replacing 50% of FM with SM (SM50) and supplementation of 2% inulin or oligofructose to the SM50 diet by replacing wheat flour in the diet (SMI50 and SMO50, respectively). Fish meal and soy protein concentrate were used as the main protein sources, and a mixture of fish oil and soybean oil was used as fat sources. The ingredients were properly formulated with a well-balanced energy-to-protein ratio to keep the dry matter content of each diet at 100%. For diet preparation, the ingredients were weighed on a precision scale and then thoroughly mixed in a blender (400V HTS42 2T, RM Gastro, EU) and homogenized with the appropriate amount of water. The prepared mixture was pelletized (1.5 and 2.5 mm) using a multifunctional spiral extrusion machine (P 98 CE, BRAHER INTERNACIONAL, Spain). The pellets were dried overnight at 35°C, sealed in bags and stored at 4°C until use.
2.2 Fish and feeding trial
The feeding experiment was conducted in an experimental recirculating aquaculture system (RAS) under controlled conditions at the Faculty of Fisheries and Protection of Waters, University of South Bohemia (FFWP, USB) (Vodnany, Czech Republic). The experimental facility was equipped with twelve round tanks (150 l) with a BaseDrum 15 mechanical dram filter (Ratz Ltd, Remscheid, Germany) and a 2000 l mobile biological filter (Ekory Ltd, Jihlava, Czech Republic) and a C-0 oxygen cone (Aquacultur Fischtechnik Ltd, Nienburg, Germany). Water in the tanks was supplied at a constant flow rate of 2.5 L min-1 to change tank volume every 30 min. Seventy randomly intensive cultured pikeperch (36.68 ± 0.36 g) according to Policar et al. (2016) were distributed to each tank and fed with a commercial feed (Inicio Plus, BioMar, 3 mm) for two weeks to acclimate them to the experimental conditions. At the end of the acclimation period, four prepared experimental diets were fed to triplicate four groups twice daily (07:00 and 15:00) for 8 weeks until satiation. Feeding was stopped 24 h before weighing or blood sampling to minimize handling stress. Rearing water temperature, pH, and dissolved oxygen (DO), nitrite (NO2-), and ammonium nitrogen (NH4+-N) concentrations were maintained at 21.1 ± 0.3°C, 7.19 ± 0.06, 98.08 ± 0.64%, 0.41 ± 0.08 mg L-1, and 0.78 ± 0.29 mg L-1, respectively. The photoperiod was maintained with a 12:12 light/dark cycle.
2.3 Sample collection
At the end of 8 weeks feeding experiment, all fish were weighed individually for calculation of growth parameters, feed utilization and survival rate. Five intact fish from each tank were randomly sampled and stored at -20°C for analysis of proximate composition. Ten fish per tank were randomly captured, anesthetized (clove oil, 0.3 ml 10 l-1 water), and blood samples were collected from the caudal vein using non-heparinized syringes and allowed to clot at 4°C for 24 h. The collected blood serum was separated by centrifugation at 5,000 × g for 10 minutes and stored at -80°C for subsequent analysis of blood biochemical values, innate immunity, antioxidant parameters, and D-lactate concentration.
2.4 Whole-body composition
Whole-body composition analysis was conducted according to the standard procedures (Association of Official Analytical Chemists (AOAC), 2005). Moisture was measured by drying in a NÜVE type FN 400P oven (NÜVE, Ankara, Turkey) at 105°C to constant weight (Adineh et al., 2020). Crude protein was analyzed using the Kjeldahl method (BUCHI Labortechnik AG, type K-360, Königswinter, Germany) (Zaefarian et al., 2017). Crude lipid was determined using the extraction method of Hara and Radin (1987) with slight modifications (Zajic et al., 2013). Ash content was analyzed in a muffle furnace L 40/11 BO (Nabertherm GmbH, Lilienthal, Germany) at 550°C for 4 h (Zaefarian et al., 2017).
2.5 Blood biochemistry, immunity, and antioxidant activity
Kits using a VET-TEST 8008 analyzer (IDEXX Laboratories Inc, Maine, United States) were used to measure serum biochemical indices, including total protein (TP), triglycerides (TG), total cholesterol (CHO), glucose (GLU), alanine aminotransferase (ALT), aspartate aminotransferase (AST), alkaline phosphatase (ALP), and lactate dehydrogenase (LDH). Catalase (CAT) and superoxide dismutase (SOD) activities were determined spectrophotometrically using commercially available assay kits (ThermoFisher Scientific, CA, USA, and Sigma, 19160, respectively). Malondialdehyde (MDA) concentration was measured spectrophotometrically using commercial assay kits (Sigma-Aldrich, Missouri, USA). Lysozyme activity of the samples was measured by a turbidimetric method (Parry, 1965). Briefly, 10 µL of serum diluted 1:2 with 10 mM PBS, pH 6.2, was placed in a 96-well flat-bottomed plate. To each well, 200 µL of freeze-dried Micrococcus lysodeikticus in the above buffer (0.3 mg mL-1, Sigma) was added as a lysozyme substrate. The decrease in absorbance at 450 nm was measured over 15 min at 3 min intervals at room temperature in a plate reader. One unit of lysozyme activity was defined as an absorbance reduction of 0.001 min-1. The units of lysozyme present in serum were determined using a standard curve constructed with hen egg white lysozyme (HEWL, Sigma), and the results were expressed as units mL -1. Peroxidase activity was determined according to Quade and Roth (1997). Five µL of serum was diluted with Hanks’s buffer (HBSS) without Ca+2 or Mg+2 to a final volume of 50 µL in a flat-bottomed 96-well plate. As substrate, 100 µL of 10 mM TMB containing 0.025% of 30% H2O2 was added, and the color change reaction was stopped by adding 50 µL 2 M H2SO4. Optical density (OD) was read at 450 nm in a plate reader. Sample without serum were used as blank, and its OD value was subtracted for each sample value. One unit was defined as the amount that produced an absorbance change of 1, and the activity was expressed as units mL -1 in each sample. Total immunoglobulins (Igs) content was determined according to the method described by Siwicki and Anderson (1993). Briefly, an aliquot (0.1 mL) of each serum sample was mixed with an equal volume of a 12.0% PEG solution (polyethylene glycol, Sigma) and incubated at room temperature for 120 min and centrifuged at 5000 × g for 5 min at 4°C to precipitate immunoglobulin. The supernatant was diluted 30 times with 0.85% NaCl, and the protein content was determined by the Bradford method. The difference between the protein values of the untreated and PEG treated samples was determined as the total Igs content and expressed as mg mL -1.
2.6 Serum D-lactate concentration
Serum D-lactate concentration was determined using a commercially available kit (D-lactate colorimetric assay; Sigma-Aldrich). Absorbance at 450 nm was measured with a Wallac Victor 3 Multi-well Plate Reader (Perkin Elmer).
2.7 Gene expression
2.7.1 RNA extraction and reverse transcription
To extract total RNA, liver samples were collected from RNAlater and then the RNeasy Mini-Kit (QIAGEN, Germany) was used according to the manufacturer’s protocol. The extraction protocol included on-column treatment with DNase I (Qiagen). RNA concentration was measured spectrophotometrically using NanoDrop 2000 (Thermo Fisher Scientific, Germany) and RNA purity was assessed by 260/280 and 230/280 ratios. Reverse transcription was performed with total RNA as follows: 1 μg of total RNA was first incubated with an oligo (dT) primer (sequence: 5′−CCTGAATTCTAG AGCTCA(T)17-3′) in a 20 μL reaction at 70°C for 5 min and then cooled on ice. After addition of dNTPs (New England BioLabs Inc.), 200 U RevertAid Reverse Transcriptase (ThermoFisher Scientific, Germany), and the reaction buffer supplied with the enzyme, the resulting 30-μL reaction was heated at 42°C for 60 min and at 70°C for 15 min. A RT control, in which RevertAid Reverse Transcriptase was replaced with pure water, was added to exclude possible contamination of samples by genomic DNA. cDNA samples were subsequently stored at -20°C.
2.7.2 Relative real-time PCR
Relative real-time PCR assays were performed with gene-specific primers for growth-related genes of pikeperch, including growth hormone receptor (GHR), insulin-like growth factor-I (IGF-I), and insulin-like growth factor-I (IGF-II). Primer sequences are listed in Table 2. Assay reaction volume: 2 μL of 10x diluted cDNA, 7.5 pmol of each primer, and 1× FastStart Universal SYBRGreen Master (Rox) (Roche)] were run in a Mx3005p RT-PCR cycler (Agilent Technologies) as follows: initial denaturation at 95°C for 10 min, followed by 40 cycles of denaturation at 95°C for 17 s, primer annealing at 58, 60, 62, or 65°C for 35 s, and extension at 72°C for 25 s. PCR was performed in duplicate for all samples. The relative values of the target transcript abundance in individual samples were determined by the comparative CT method (ΔΔCT) according to Pfaffl (2001). The mRNA expression of the reference gene (GAPDH; Table 1) was used for normalization. Normalized expression of target genes is expressed as mean ± SEM relative to control.
2.8 Statistical analysis
Before the analysis, the characteristics of the data distribution and the homogeneity of the dispersion were evaluated using the Shapiro-Wilk test and Levene’s test, respectively. Then, data with normal distribution were analyzed using one-way analysis of variance (ANOVA) followed by Tukey’s honest significant difference (HSD) test. Non-homogeneous data were analyzed using a non-parametric Kruskal-Wallis test followed by multiple comparison of the mean ranks of all groups. Statistical significance was assumed at p < 0.05. Data are presented as mean ± SEM. All analyzes were performed with SPSS version 17.0 (SPSS Inc., Chicago, IL, USA).
3 Results
The group fed SMO50 showed the highest weight gain (WG; 85.85 ± 4.46%) among the groups fed SM, with no significant difference compared to the FM group; 79.74 ± 2.04% (p > 0.05; Table 3). Specific growth rate (SGR) showed no significant differences among fish fed SMI50 (0.81 ± 0.07%), SMO50 (1.01 ± 0.09%) and FM (1.05 ± 0.02%; p > 0.05). However, lower SGR (0.69 ± 0.09%) was observed in the SM50 group without supplementation of 2% inulin or oligofructose compared to the group fed FM (p < 0.05; Table 3). Similarly, a significant difference in FCR was observed between the SM50-fed group without supplementation of inulin or oligofructose (1.50 ± 0.13) and the group fed FM (0.97 ± 0.04; Table 3). The groups fed SMI50 (1.23 ± 0.10) and SMO50 (0.91 ± 0.05) showed no significant difference in FCR compared to the group fed FM (0.97 ± 0.04; Table 3). The FCR was significantly lower in the SMO50-fed group (0.91 ± 0.05) compared to the group fed SM50 (1.50 ± 0.13). The survival rate in the four groups ranged from 95.13 to 97.77%, with no significant difference observed among all groups (p > 0.05; Table 3). The proximate composition of whole-body is shown in Table 4 and no significant difference was found among all groups (p > 0.05).
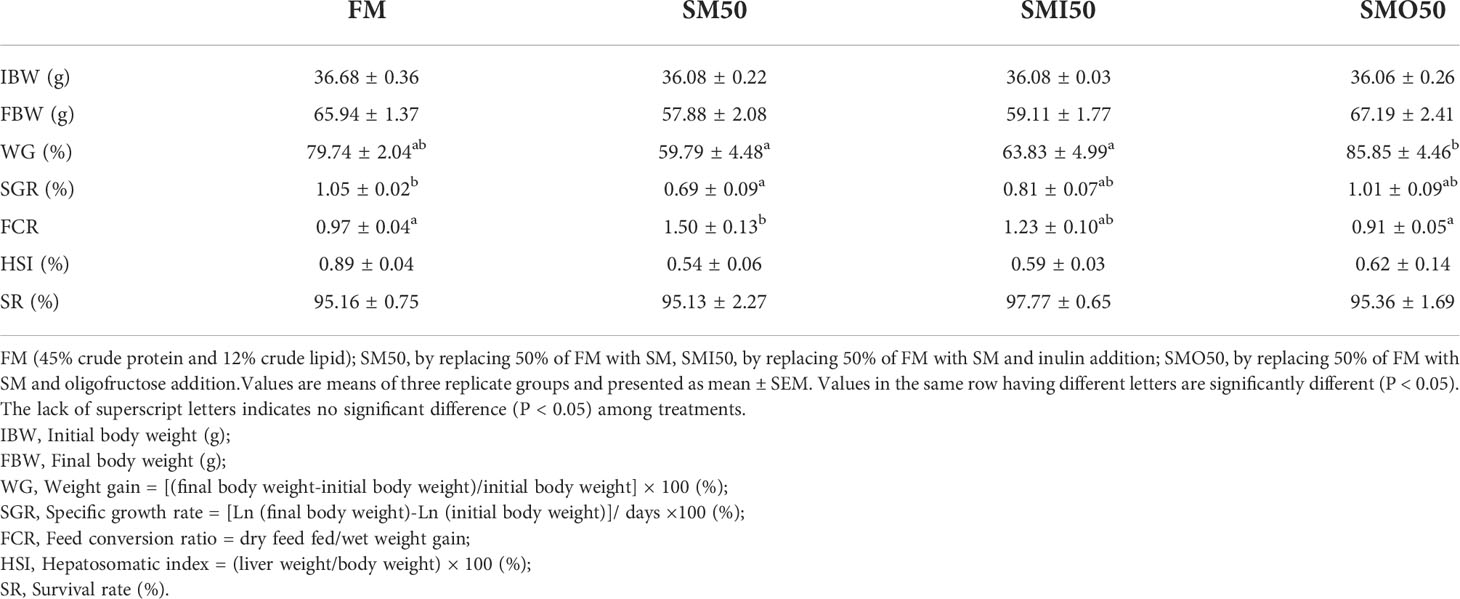
Table 3 Growth performance, feed utilization and survival of pikeperch fed the experimental diets for 8 weeks.
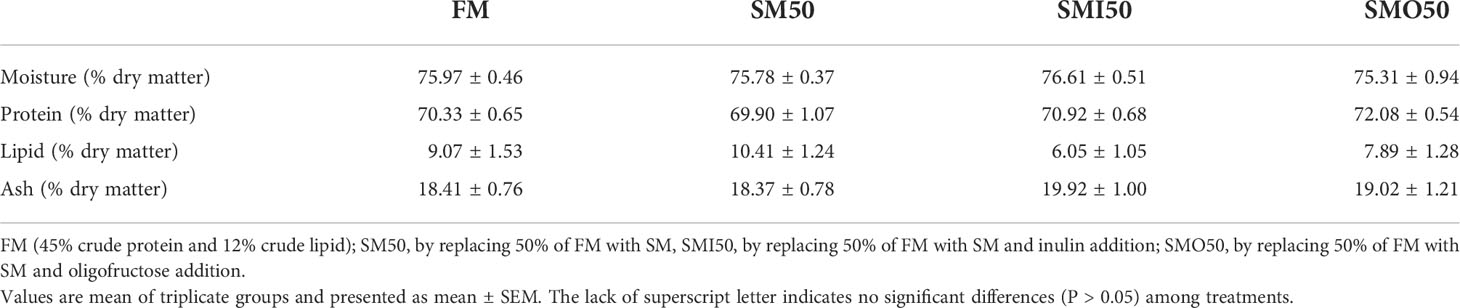
Table 4 Whole-body composition of pikeperch fed the experimental diets for 8 weeks (% dry matter basis).
Replacement of FM with SM resulted in a significant decrease in serum total cholesterol concentration (T- CHO) in the SMO50 group (2.10 ± 0.29 mmole L-1) compared to the FM-fed group (3.33 ± 0.33 mmole L-1; Table 5). There were no significant differences between the other SM-fed groups and the FM-fed group (p > 0.05). Serum triglyceride showed a significant reduction in the groups fed SMI50 (0.98 ± 0.17 mmole L-1) compared to the group fed SMO50 (1.93 ± 0.24 mmole L-1; Table 5). No significant difference was observed in triglycerides between the other groups (p > 0.05; Table 5). The concentrations of other serum biochemical parameters (triglyceride: TG, total protein: TP, glucose: GLU, lactate dehydrogenase: LDH, alanine aminotransferase: ALT, aspartate aminotransferase: AST, and alkaline phosphatase: ALKP) remained unchanged (p > 0.05; Table 5).
Among the parameters of innate immunity and antioxidants, lysozyme activity (LYZ), peroxidase, and total immunoglobulin (T-Ig) showed no significant differences among the studied groups (p > 0.05; Table 6). In contrast, a significant difference was observed in the serum malondialdehyde (MDA) concentration of the group fed FM (25.17 ± 3.13 nmole mL−1; Table 6) compared to the groups fed SM50, SMI50, and SMO50 (ranging from 4.25 to 14.77 nmole mL−1; Table 6). Total antioxidant capacity (T-AOC), superoxide dismutase (SOD) and catalase (CAT) in serum were not significantly affected by the feeding (p > 0.05; Table 6). Serum D-lactate concentration was not significantly affected by the dietary treatments (p > 0.05; Figure 1). The results of gene expression analysis of growth-related genes, IGF-I, IGF-II, and GHR showed no significant difference among the studied groups (P > 0.05; Figure 2).
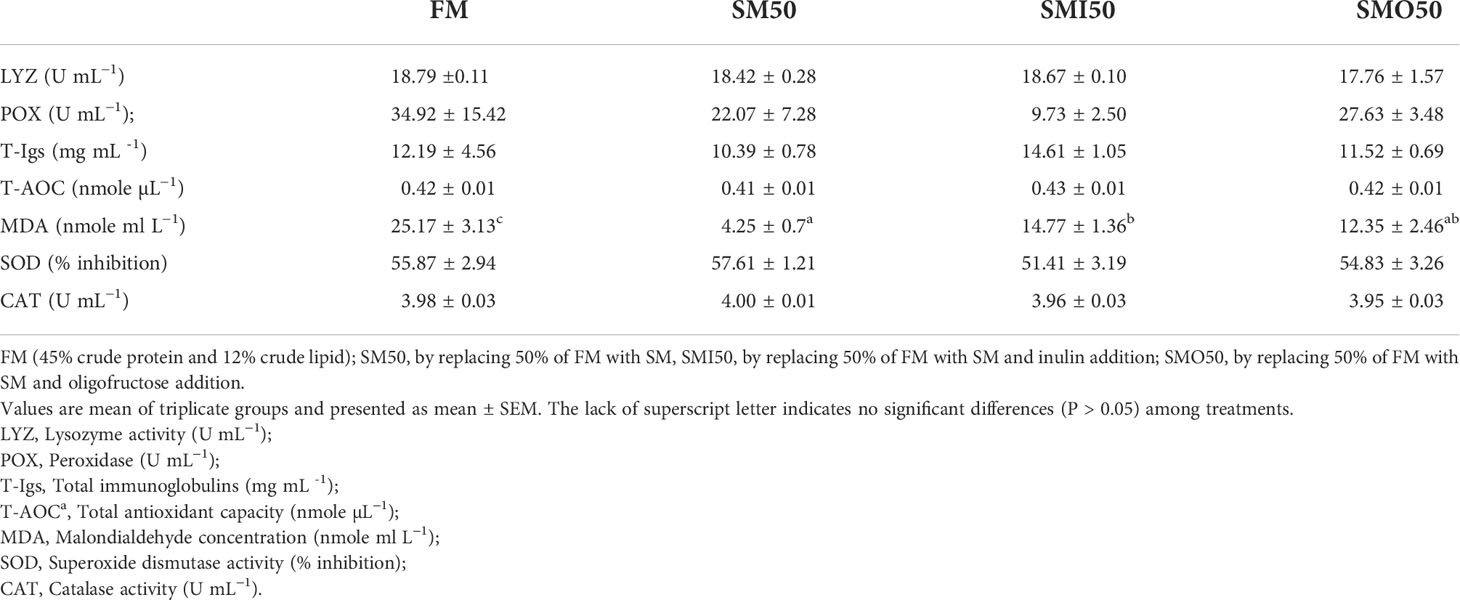
Table 6 Serum innate immunity and antioxidant parameters of pikeperch fed the experimental diets for 8 weeks.
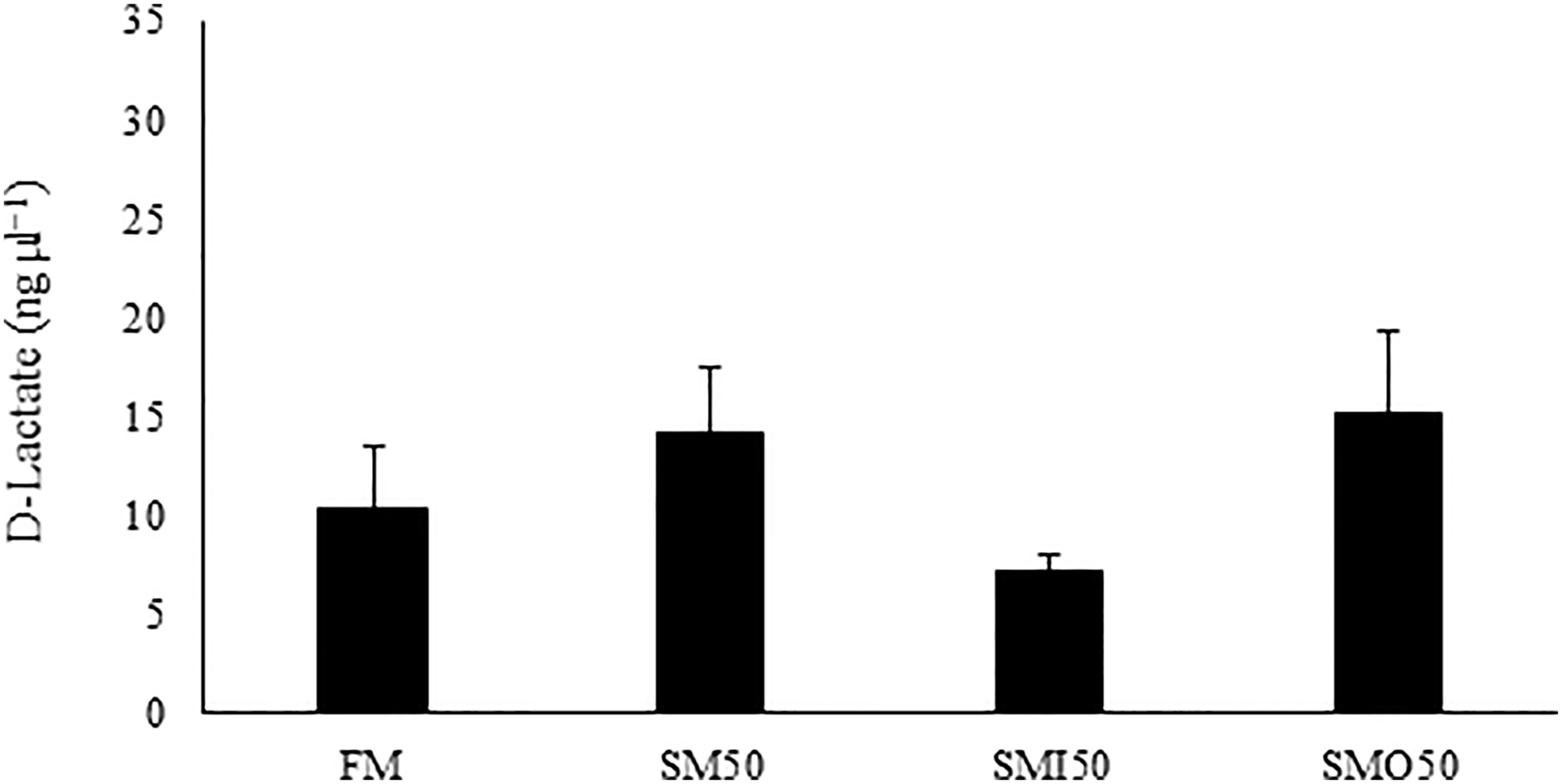
Figure 1 Serum D-lactate concentration (ng µl−1) in pikeperch fed the experimental diets for 8 weeks. FM (45% crude protein and 12% crude lipid); SM50, by replacing 50% of FM with SM, SMI50, by replacing 50% of FM with SM and inulin addition; SMO50, by replacing 50% of FM with SM and oligofructose addition. The lack of superscript letter indicates no significant differences (P > 0.05) among treatments.
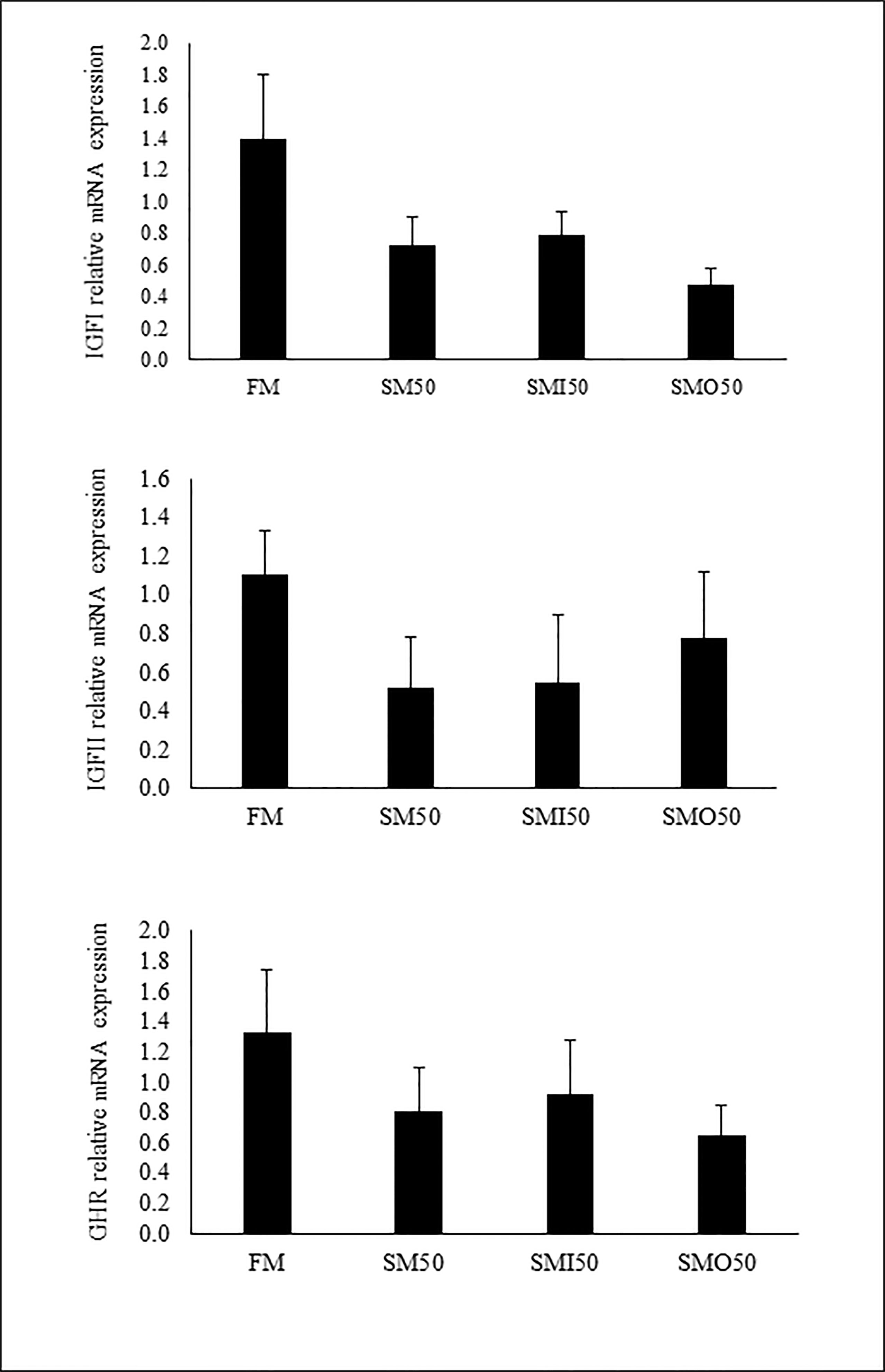
Figure 2 Relative mRNA expression in liver of pikeperch fed the experimental diets for 8 weeks of A) IGF-I, B) IGF-II, C) GHR gene. FM (45% crude protein and 12% crude lipid); SM50, by replacing 50% of FM with SM, SMI50, by replacing 50% of FM with SM and inulin addition; SMO50, by replacing 50% of FM with SM and oligofructose addition. The lack of superscript letter indicates no significant differences (P > 0.05) among treatments.
4 Discussion
Different effects have been reported in various omnivorous and carnivorous fish species due to dietary soybean supplementation (Viola et al., 1982; Shiau et al., 1989; Shiau et al., 1990; Webster et al., 1992; Shimeno et al., 1993; Refstie et al., 2000). Previous studies on carnivorous fish have shown conflicting results on the replacement of FM with SM, including the possibility of replacing FM with only a small amount of SM (Krogdahl et al., 2010; Merrifield et al., 2011) and a large tolerance to the replacement of FM (Webster et al., 1992; Zhang et al., 2018).
In the present study, replacing 50% of FM with SM did not impair the growth performance of pikeperch. This result is consistent with a study in which FM was replaced with up to 100% SM in the diet of an omnivorous fish, Pacific fat sleeper (Dormitator latifrons), and showed adequate growth without effects on body composition and survival (Badillo-Zapata et al., 2021). Replacement of FM up to 70% with crude protein from toasted soybean meal also did not compromise growth of carnivorous blue catfish, Ictalurus furcatus (Webster et al., 1992). Similar to our results, Zhang et al. (2018) reported no significant changes in growth performance of Japanese sea bass, Lateolabrax japonicus, when 50% of FM was replaced with SM. In contrast to these reports, an impaired growth performance was revealed in some carnivorous fish species such as Atlantic salmon, Salmo salar (> 20%; Krogdahl et al., 2003) and cobia Rachycentron canadum (> 40%; Chou et al., 2004) when FM was partially replaced with SM. Among the groups fed SM50, SMI50, and SMO50, the highest weight gain was observed in the group fed SMO50, highlighting the beneficial effect of oligofructose on the growth rate of carnivorous fish (Mahious et al., 2006).
According to the present results, the specific growth rate significantly decreased in the SM50-fed group, and supplementation of the prebiotics (inulin and oligofructose) significantly improved the specific growth rate. In the current study, there was a significant increase in FCR in the SM50-fed group compared to the FM-fed group. While, oligofructose supplementation resulted in significantly lower FCR in the SMO50-fed group (0.91 ± 0.05; close to the FM-fed group) compared to the SM50-fed group (1.50 ± 0.13). A study on Atlantic salmon showed a reduction in feed conversion when 40% of FM was replaced with SM (Refstie et al., 1998). In contrast, no negative effects on growth and FCR were observed in rainbow trout, Oncorhynchus mykiss when 60% of FM was replaced with SM (Barnes et al., 2012). Similarly, an inclusion level of SM up to 40% does not seem to adversely affect growth, feed efficiency, and protein utilization in cobia (Chou et al., 2004). In Carassius auratus gibelio ♀ × Cyprinus carpio ♂, the best growth responses and feed efficiency were obtained with a limited substitution to < 38.52-41.81% and 40.75%, respectively (Liu et al., 2020).
Replacing 50% of FM with SM, either with or without probiotics, did not result in significant changes in whole-body composition in the present study. Consistent with the present results, whole body proximate compositions were not affected when 0, 70, 80, 90, and 100% of fish meal protein was replaced with dehulled soybean meal in red sea bream (Pagrus major) diets (Kader et al., 2012). A previous study on sea bass is consistent with these results (Tibaldi et al., 2006) when fish were fed either a FM-based diet (control) or an isoprotein, isolipid and isocaloric soy replacement diet. The diets consisted of replacement of FM protein with: 25% toasted, dehulled, and solvent-extracted soybean meal; 50% dehulled and toasted soy seeds subjected to dry extrusion and mechanical oil extraction; 50% enzyme-treated soybean meal; and an inclusion of 60% composed of 30% toasted, dehulled, and solvent-extracted soybean meal and 30% enzyme-treated soybean meal.
Hematological indices are commonly used to evaluate the physiological and health status of fish in studies focused on replacing fishmeal with soybean in the diet (Ajani et al., 2016; Rahimnejad et al., 2021; Xu et al., 2022). Despite lowering blood cholesterol levels by replacing animal proteins with soy protein in various animal models (Cho et al., 2007), it has been claimed that a formulated diet containing 45% SM with or without Heat-Killed Lactobacillus plantarum has no effect on total cholesterol level in amberjack, Seriola dumerili (Dawood et al., 2015). Similarly, our results showed no significant change in serum total cholesterol levels when FM was replaced with SM. However, a significant decrease was observed in the oligofructose supplemented group fed SM50 (2.10 ± 0.29), which underlines the probable effect of oligofructose supplementation on cholesterol levels in fish (Hoseinifar et al., 2011a). In agreement with the present study, serum total cholesterol was not significantly decreased when 40% FM was replaced by SM in the diet for Japanese sea bass, Lateolabrax japonicas (Rahimnejad et al., 2019). However, increasing the substitution of SM for FM from 40% to 80% resulted in a decrease in serum total cholesterol in this species (Rahimnejad et al., 2019). Similarly, replacing FM with SM (47.6%) in the diet of rainbow trout lowered plasma cholesterol levels (Yamamoto et al., 2010). The reported reductions could be due to either interruption of intestinal uptake of dietary bile acids and cholesterol (Makino et al., 1988) or enhancement of LDL-R transcription in hepatocytes (Cho et al., 2007). The capacity of the antioxidant system is a key indicator reflecting health status and oxidative stress in fish (Deng et al., 2015). Therefore, the effects of soybean meal on the innate immunity and antioxidant parameters of pikeperch were also investigated. In the present study, there were no statistically significant differences in LYZ, POX, T-Ig and T-AOC. In addition, two representatives of the first line defense antioxidants, SOD and CAT, did not change significantly in the studied groups. However, MDA as one of the most important indicators of lipid peroxidation with a high level of biotoxicity to cells (Parvez and Raisuddin, 2005) significantly increased in the FM-fed group with the highest MDA value (25.17 ± 3.13 nmole ml L−1). The lower MDA value in the groups fed SM50, SMI50, and SMO50 could be attributed to the activity of other first line defense antioxidants such as glutathione peroxidase (GPx) or second line defense antioxidants such as ascorbic acid, uric acid, and glutathione (Ighodaro and Akinloye, 2018). On the other hand, soybeans contain phenolic compounds and flavonoids, of which isoflavones are the most abundant (Liu et al., 2005). The isoflavones have been shown to act as potent antioxidants and may be responsible for preventing lipid peroxidation (Jha et al., 1985).
Serum D-lactate is a well-established marker that indirectly assesses intestinal mucosal barrier function (Zhang et al., 2018). In the present study, no significant differences in serum D-lactate concentration were observed among all groups, indicating that the barrier function of the intestinal mucosa was not disturbed after replacing FM with SM.
Biotechnology elements (growth-related genes) such as GHR, IGF-I, and IGF-II are also used as biomolecular tools to evaluate growth response in fish studies (Picha et al., 2008) and to evaluate the efficacy of commercial feeds and plant proteins (Mzengereza et al., 2021). The present data indicate that the relative expression of the growth-related genes GHR, IGF-I, and IGF-II was not affected by the replacement of FM. In agreement with the present results, no significant changes in the relative expression of IGF-I and IGF- II were reported in red sea bream liver upon replacement of 24% of fish meal with soybean meal (Mzengereza et al., 2021).
5 Conclusion
The results of the present study show that the substitution of 50% of FM by SM with a small amount of oligofructose does not compromise the growth performance or the immune system of pikeperch. This substitution is feasible and provides a reference for cost-optimised design of feed formulations for pikeperch.
Data availability statement
The original contributions presented in the study are included in the article/supplementary material. Further inquiries can be directed to the corresponding author.
Ethics statement
This study was performed according to the following national and international guidelines for animal welfare protection (EU-harmonized Animal Welfare Act of the Czech Republic; law No. 166/1996 and no. 246/1992). This study was performed under RTD capacity permits issued to No. 58672/2020-MZE-18134 and No. 33446/2020-MZE-18134. All samples were done with the appropriate permission of the Departmental Expert Committee for the Authorization of Experimental Projects of the Ministry of Education, Youth and Sports of the Czech Republic, permit no. MSMT-8155/2022-4 for project NAZV QK22020144.
Author contributions
HD, conceptualization, investigation, visualization, methodology, data curation, writing, review, and editing. HD, LC, AI, and ME, formal analysis. OM, TPe, and JK, samples collection and interpretation of data. SR, conceptualization, and design of the study and review. TPo, funding acquisition, project administration, and resources. All authors contributed to the article and approved the submitted version.
Funding
This study was financially supported by the Ministry of Agriculture of the Czech Republic, project NAZV QK22020144 and by the “Comunidad Autónoma Región de Murcia-Fundación Séneca (ThinkInAzul). España. Unión europea”.
Conflict of interest
The authors declare that the research was conducted in the absence of any commercial or financial relationships that could be construed as a potential conflict of interest.
Publisher’s note
All claims expressed in this article are solely those of the authors and do not necessarily represent those of their affiliated organizations, or those of the publisher, the editors and the reviewers. Any product that may be evaluated in this article, or claim that may be made by its manufacturer, is not guaranteed or endorsed by the publisher.
References
Adámek Z., Opacak A. (2005). Prey selectivity in pike (Esox lucius), zander (Sander lucioperca) and perch (Perca fluviatilis) under experimental conditions. Biologia 60 (5), 567–570.
Adineh H., Harsij M., Jafaryan H., Asadi M. (2020). The effects of microencapsulated garlic (Allium sativum) extract on growth performance, body composition, immune response and antioxidant status of rainbow trout (Oncorhynchus mykiss) juveniles. J. Appl. Anim. Res. 48, 372–378. doi: 10.1080/09712119.2020.1808473
Ajani E. K., Orisasona O., Omitoyin B. O., Osho E. F. (2016). Total replacement of fishmeal by soybean meal with or without methionine fortification in the diets of Nile tilapia. Oreochrom. niloticus. J. Fish. Aquat. Sci. 11, 238–243. doi: 10.3923/jfas.2016.238.243
Al-Kenawy D., El-Nagar G., Abou Zead M. Y. (2008). “Total replacement of fishmeal with soybean meal in diets for Nile tilapia in pre-fertilized ponds,” in Proceedings of the 8th International Symposium on Tilapia in Aquaculture, Egypt. 773–785 (The World Fish Center). Available at: https://digitalarchive.worldfishcenter.org/bitstream/handle/20.500.12348/1546/6%20Diaa%20Kenaway.pdf?sequence1.
Association of Official Analytical Chemists (AOAC) (2005). Official methods of analysis. 16th ed (Washington, DC, USA).
Badillo-Zapata D., Lazo J. P., Herzka S. Z., Viana M. T. (2014). The effect of substituting fishmeal with poultry by-product meal in diets for Totoaba macdonaldi juveniles. Aquac. Res. 47, 1778–1789. doi: 10.1111/are.12636
Badillo-Zapata D., Musin G., Palma-Cancino D., Guerrero-Galván S., Chong-arrillo O., Vega-Villasante F. (2021). Total or partial replacement of fishmeal with soybean meal in the diet of the pacific fat sleeper Dormitator latifrons juveniles. Lat. Am. J. Aquat. Res. 49 (1), 40–47. doi: 10.3856/vol49-issue1-fulltext-2564
Baeverfjord G., Krogdahl Å. (1996). Development and regres- sion of soybean meal induced enteritis in Atlantic salmon, Salmo salar l., distal intestine: A comparison with the intestines of fasted fish. J. Fish. Dis. 19, 375–387. doi: 10.1111/j.1365-2761.1996.tb00376.x
Bakke-McKellep A. M., Penn M. H., Salas P. M., Refstie S., Sperstad S., Landsverk T., et al. (2007). Effects of dietary soybean meal, inulin and oxytetracycline on gastrointestinal histological characteristics, distal intestine cell proliferation and intestinal microbiota in Atlantic salmon (Salmo salar l.). Br. J. Nutr. 97, 699–713. doi: 10.1017/S0007114507381397
Barnes M. E., Brown M. L., Rosentrater K. A., Sewell J. R. (2012). An initial investigation replacing fish meal with a commercial fermented soybean meal product in the diets of juvenile rainbow trout. Open J. Anim. Sci. 2, 234–243. doi: 10.4236/ojas.2012.24033
Bochert R. (2020). Comparative performance, biochemical composition, and fatty acid analysis of Eurasian perch (Perca fluviatilis) during grow-out in RAS fed different commercial diets. J. Appl. Aquacult. 34 (1), 1–15. doi: 10.1080/10454438.2020.1828217
Bongers A., van den Heuvel E. G. H. M. (2003). Prebiotics and the bioavailability of minerals and trace elements. Food Rev. Int. 19, 397–422. doi: 10.1081/FRI-120025482
Burrells C., Williams P. D., Southgate P. J., Crampton V. O. (1999). Immunological, physiological and pathological responses of rainbow trout (Oncorhynchus mykiss) to increasing dietary concentrations of soybean proteins. Vet. Immunol. Immunopathol. 72, 277–288. doi: 10.1016/S0165-2427(99)00143-9
Burr G., Hume M., Neill W. H., Gatlin D. M. (2008). Effects of prebiotics on nutrient digestibility of a soybean-meal-based diet by red drum Sciaenops ocellatus (Linnaeus). Aquac. Res. 39, 1680–1686. doi: 10.1111/j.1365-2109.2008.02044.x
Cerezuela R., Cuesta A., Meseguer J., Esteban M. A. (2008). Effects of inulin on gilthead seabream (Sparus aurata l.) innate immune parameters. Fish. Shellfish Immunol. 24, 663–668. doi: 10.1016/j.fsi.2007.10.002
Choi D. G., He M., Fang H., Wang X. L., Li X. Q., Leng X. J. (2020). Replacement of fish meal with two fermented soybean meals in diets for rainbow trout (Oncorhynchus mykiss). Aquacult. Nutr. 2637–, 46. doi: 10.1111/anu.12965
Cho S. J., Juillerat M. A., Lee C. H. (2007). Cholesterol lowering mechanism of soybean protein hydrolysate. J. Agric. Food Chem. 55, 10599–10604. doi: 10.1021/jf071903f
Chou R. L., Her B. Y., Su M. S., Hwang G., Wu Y. H., Chen H. Y. (2004). Substituting fish meal with soybean meal in diets of juvenile cobia Rachycentron canadum. Aquaculture 229, 325–333. doi: 10.1016/S0044-8486(03)00395-8
Dawood M. A., Koshio S., Ishikawa M., Yokoyama S. (2015). Effects of partial substitution of fish meal by soybean meal with or without heat-killed Lactobacillus plantarum (LP20) on growth performance, digestibility, and immune response of amberjack, Seriola dumerili juveniles. BioMed. Res. Int. 2015, 514196. doi: 10.1155/2015/514196
Deng J. M., Mai K. S., Chen L. Q., Mi H. F., Zhang L. (2015). Effects of replacing soybean meal with rubber seed meal on growth, antioxidant capacity, non-specific immune response, and resistance to Aeromonas hydrophila in tilapia (Oreochromis niloticus o. aureus). Fish. Shellfish Immunol. 44, 436–444. doi: 10.1016/j.fsi.2015.03.018
Gatlin D. M., Barrows I. I. I., Brown P., Dabrowski K., Gaylord T. G., Hardy R. W., et al. (2007). Expanding the utilization of sustainable plant products in aquafeeds: a review. Aquacult. Res. 38, 551–579. doi: 10.1111/j.1365-2109.2007.01704.x
Guerreiro I., Couto A., Perez-Jimenez A., Oliva-Teles A., Enes P. (2015). Gut morphology and hepatic oxidative status of European sea bass (Dicentrarchus labrax) juveniles fed plant feedstuffs or fishmeal-based diets supplemented with shortchain fructo-oligosaccharides and xylo-oligosaccharides. Br. J. Nutr. 114, 1975–1984. doi: 10.1017/S0007114515003773
Hamza N., Mhetli M., Kestemont P. (2007). Effects of weaning age and diets on ontogeny of digestive activities and structures of pikeperch (Sander lucioperca) larvae. Fish. Physiol. Biochem. 33, 121–133. doi: 10.1007/s10695-006-9123-4
Hara A., Radin N. S. (1987). Lipid extraction of tissues with a low toxicity solvent. Anal. Biochem. 90, 420–426. doi: 10.1016/0003-2697(78)90046-5
Hedrera M. I., Galdames J. A., Jimenez-Reyes M. F., Reyes A. E., Avendaño-Herrera R., Romero J., et al. (2013). Soybean meal induces intestinal inflammation in zebrafish larvae. PloS One 8 (7), e69983. doi: 10.1371/journal.pone.0069983
Hoseinifar S. H., Mirvaghefi A., Merrifield D. L., Amiri B. M., Yelghi S., Bastami K. D. (2011a). The study of some haematological and serum biochemical parameters of juvenile beluga (Huso huso) fed oligofructose. Fish. Physiol. Biochem. 37 (1), 91–96. doi: 10.1007/s10695-010-9420-9
Hoseinifar S., Mirvaghefi A., Mojazi amiri B., Rostami H., Merrifield D. (2011b). The effects of oligofructose on growth performance, survival and autochthonous intestinal microbiota of beluga (Huso huso) juveniles. Aquac. Nutr. 17, 498–504. doi: 10.1111/j.1365-2095.2010.00828.x
Ighodaro O. M., Akinloye O. A. (2018). First line defence antioxidants-superoxide dismutase (SOD), catalase (CAT) and glutathione peroxidase (GPX): Their fundamental role in the entire antioxidant defence grid. Alexandria J. Med. 54 (4), 287–293. doi: 10.1016/j.ajme.2017.09.001
Imentai A., Gilannejad N., Martınez-Rodrıguez G., Lopez F. J. M., Martınez F. P., Penka T., et al. (2022). Effects of first feeding regime on gene expression and enzyme activity in pikeperch (Sander lucioperca) larvae. Front. Mar. Sci. 9. doi: 10.3389/fmars.2022.864536
Imorou Toko I., Fiogbe D. E., Kestemont P. (2008). Mineral status of African catfish (Clarias gariepinus) fed diets containing graded levels of soybean or cottonseed meals. Aquaculture 275, 298–305. doi: 10.1016/j.aquaculture.2007.11.038
Jankowska B., Zakes Z., Zmijewski T., Szczepkowski M. (2003). Fatty acid profile and meat utility of wild and cultured zander, Sander lucioperca (L.). Electron. J. Pol. Agric. Univ. 6, 1–7.
Jha H. C., von Recklinghausen G., Zilliken F. (1985). Inhibition of in vitro microsomal lipid peroxidation by isoflavonoids. Biochem. Pharmacol. 34 (9), 1367–1369. doi: 10.1016/0006-2952(85)90672-0
Kader M. A., Bulbul M., Koshio S., Ishikawa M., Yokoyama S. (2012). Effect of complete replacement of fishmeal by dehulled soybean meal with crude attractants supplementation in diets for red sea bream, Pagrus major. Aquaculture 350–353, 109–116. doi: 10.1016/j.aquaculture.2012.04.009
Kestemont P., Xueliang X., Hamza N., Maboudou J., Imorou Toko I. (2007). Effect of weaning age and diet on pikeperch larviculture. Aquaculture 264, 194–204. doi: 10.1016/j.aquaculture.2006.12.034
Klein Breteler J. G. P. (1989). “Intensive culture of pike-perch fry with live food,” in Aquaculture- a biotechnology in progress, vol. 203-207 . Eds. De Pauw N., Jaspers E., Ackefors H., Wilkins N. (Bredene Belgium: E.A.S.).
Kokou F., Sarropoulou E., Cotou E., Kentouri M., Alexis M., Rigos G. (2017). Effects of graded dietary levels of soy protein concentrate supplemented with methionine and phosphate on the immune and antioxidant responses of gilthead sea bream (Sparus aurata l.). Fish. Shellfish Immunol. 64, 111–121. doi: 10.1016/j.fsi.2017.03.017
Krogdahl A., Bakke-McKellep A. M., Baeverfjord G. (2003). Effects of graded levels of standard soybean meal on intestinal structure, mucosal enzyme activities, and pancreatic response in Atlantic salmon (Salmo salar l.). Aquac. Nutr. 9, 361–371. doi: 10.1046/j.1365-2095.2003.00264.x
Krogdahl A., Penn M., Thorsen J., Refstie S., Bakke A. M. (2010). Important antinutrients in plant feedstuffs for aquaculture: an update on recent findings regarding responses in salmonids. Aquacult. Res. 41, 333–344. doi: 10.1111/j.1365-2109.2009.02426.x
Lappalainen J., Olin M., Vinni M. (2006). Pikeperch cannibalism: Effects of abundance, size and condition. Ann. Zool. Fenn. 43 (1), 35–44.
Li P., Gatlin D. M. (2004). Dietary brewers yeast and the prebiotic GrobioticTM AE influence growth performance, immune responses and resistance of hybrid striped bass (Morone chrysops x m. saxatilis) to streptococcus iniae infection. Aquaculture 231, 445–456. doi: 10.1016/j.aquaculture.2003.08.021
Li P., Gatlin D. M. (2005). Evaluation of the prebiotic GroBiotic®-a and brewers yeast as dietary supplements for sub-adult hybrid striped bass (Morone chrysops x m. saxatilis) challenged in situ with mycobacterium marinum. Aquaculture (Amsterdam), 197–205. doi: 10.1016/j.aquaculture.2005.03.005
Li Y., Kortner T. M., Chikwati E. M., Belghit I., Lock E. Y., Krogdahl A. (2020). Total replacement of fish meal with black soldier fly (Hermetia illucens) larvae meal does not compromise the gut health of Atlantic salmon (Salmo salar). Aquaculture 520, 734957. doi: 10.1016/j.aquaculture.2020.734967
Lin S., Luo L. (2011). Effects of different levels of soybean meal inclusion in replacement for fish meal on growth, digestive enzymes and transaminase activities in practical diets for juvenile tilapia, Oreochromis niloticus × O. aureus. Anim. Feed Sci. Technol. 168, 80–87. doi: 10.1016/j.anifeedsci.2011.03.012
Li M. H., Robinson E. H., Bosworth B. G., Oberle D. F., Lucas P. M. (2011). Use of corn gluten feed and cottonseed meal to replace soybean meal and corn in diets for pond-raised channel catfish. N. Am. J. Aquac. 73, 153–158. doi: 10.1080/15222055.2011.568857
Liu J., Chang S. K., Wiesenborn D. (2005). Antioxidant properties of soybean isoflavone extract and tofu in vitro and in vivo. J. Agric. Food Chem. 23(6), 2333–2340. doi: 10.1021/jf048552e
Liu X., Han B., Xu J., Zhu J., Hu J., Wan W., et al. (2020). Replacement of fishmeal with soybean meal affects the growth performance, digestive enzymes, intestinal microbiota and immunity of Carassius auratus gibelio♀ × Cyprinus carpio♂. Aquac. Rep. 18, 100472. doi: 10.1016/j.aqrep.2020.100472
López Nadal A., Ikeda-Ohtsubo W., Sipkema D., Peggs D., McGurk C., Forlenza M., et al. (2020). Feed, microbiota, and gut immunity: Using the zebrafish model to understand fish health. Front. Immunol. 5. doi: 10.3389/fimmu.2020.00114
Lunger A. N., McLean E., Craig S. R. (2007). The effects of organic protein supplementation upon growth, feed conversion and texture quality parameters of juvenile cobia (Rachycentron canadum). Aquaculture 264, 342–352. doi: 10.1016/j.aquaculture.2006.12.012
Mahious A. S., Gatesoupe F. J., Hervi M., Metaille R., Ollevier F. (2006). Effect of dietary inulin and oligosaccharides as prebiotics for weaning turbot, Psetta maxima (Linnaeus, c. 1758). Aquacult. Int. 14, 219–229. doi: 10.1007/s10499-005-9003-4
Makino S., Nakashima H., Minami K., Moriyama R., Takao S. (1988). Bile acid-binding protein from soybean seed: isolation, partial characterization and insulin-stimulating activity. Agric. Biol. Chem. 52 (3), 803–809. doi: 10.1080/00021369.1988.10868729
Mambrini M., Roem A. J., Cravedi J. P., Lalles J. P., Kaushik S. J. (1999). Effects of replacing fish meal with soy protein concentrate and of DLmethionine supplementation in high-energy, extruded diets on the growth and nutrient utilization of rainbow trout, Oncorhynchus mykiss. J. Anim. Sci. 77, 2990–2999. doi: 10.2527/1999.77112990x
Merrifield D. L., Olsen R. E., Myklebust R., Ringø E. (2011). “Dietary effect of soybean (Glycine max) products on gut histology and microbiota of fish,” in Soybean and nutrition. Ed. El-Shemy H. (InTech), London. doi: 10.5772/20101
Mzengereza K., Ishikawa M., Koshio S., Yokoyama S., Yukun Z., Shadrack R. S., et al. (2021). Growth performance, growth- related genes, digestibility, digestive enzyme activity, immune and stress responses of de novo camelina meal in diets of red seabream (Pagrus major). Animals 11, 3118. doi: 10.3390/ani11113118
Niness K. R. (1999). Inulin and oligofructose: what are they? J. Nutr. 129, 1402S–1406S. doi: 10.1093/jn/129.7.1402S
Nyina-Wamwiza L., Xu X. L., Blanchard G., Kestemont P. (2005). Effect of dietary protein, lipid and carbohydrate ratio on growth, feed efficiency and body composition of pikeperch Sander lucioperca fingerlings. Aquacult. Res. 36, 486–492. doi: 10.1111/j.1365-2109.2005.01233.x
Oliva-Teles A., Enes P., Peres H. (2015). “Replacing fishmeal and fish oil in industrial aquafeeds for carnivorous fish,” in Feed and feeding practices in aquaculture (Cambridge, UK: Woodhead Publishing), 203–233. doi: 10.1016/B978-0-08-100506-4.00008-8
Ostaszewska T., Dabrowski K., Czuminska K., Olech W., Olejniczak M. (2005). Rearing of pike-perch larvae using formulated diets-first success with starter feeds. Aquac. Res. 36, 1167–1176. doi: 10.1111/j.1365-2109.2005.01332.x
Papatryphon E., Soares J. H. (2001). Optimizing the levels of feeding stimulants for use in high-fish meal and plant feedstuff-based diets for striped bass, Morone saxatilis. Aquaculture 202, 279–288. doi: 10.1016/S0044-8486(01)00778-5
Pares-Sierra G., Durazo E., Ponce M. A., Badillo-Zapata D., Correa-Reyes G., Viana M. T. (2014). Partial to total replacement of fishmeal by poultry byproduct meal in diets for juvenile rainbow trout (Oncorhynchus mykiss) and their effect on fatty acids from muscle tissue and the time required to retrieve the effect. Aquac. Res. 45, 1459–1469. doi: 10.1111/are.12092
Parry R. (1965). A rapid and sensitive assay of muramidase. Proc. Soc Exp. Biol. Med. 119, 384–386. doi: 10.3181/00379727-119-30188
Parvez S., Raisuddin S. (2005). Protein carbonyls: novel biomarkers of exposure to oxidative stress-inducing pesticides in freshwater fish Channa punctata (Bloch). Environ. Toxicol. Phar. 20, 112–117. doi: 10.1016/j.etap.2004.11.002
Pattipeiluhu S. M., Loupatty J. W., Pattipeilohy C., Sambonu R. W. (2020). Feed efficiency in the growth of humpback grouper cromileptes altivelis with prebiotics dietary modulation. IOP Conf. Ser. Earth Environ. Sci. 584, 12066. doi: 10.1088/1755-1315/584/1/012066
Peltonen H., Rita H., Ruuhijarvi J. (1996). Diet and prey selection of pikeperch (Stizostedion lucioperca (L.)) in lake vesijarvi analysed with a logit model. Ann. Zool. Fenn. 33, 481–487.
Peng M., Xu W., Ai Q., Mai K., Liufu Z., Zhang K. (2013). Effects of nucleotide supplementation on growth, immune responses and intestinal morphology in juvenile turbot fed diets with graded levels of soybean meal (Scophthalmus maximus l.). Aquaculture 392–395, 51–58. doi: 10.1016/j.aquaculture.2013.02.002
Pěnka T., Malinovskyi O., Křišťan J., Imentai A., Policar T. (2021). Effect of density and mixed culture of largemouth bass (Micropterus salmoides) and pikeperch (Sander lucioperca) on growth, survival and feed conversion rate in intensive culture. Czech J. Anim. Sci. 66 (10), 428–440. doi: 10.17221/59/2021-CJAS
Peres H., Lim C., Klesius P. H. (2003). Nutritional value of heat-treated soybean meal for channel catfish (Ictalurus punctatus). Aquaculture 225, 67–82. doi: 10.1016/S0044-8486(03)00289-8
Pfaffl M. W. (2001). A new mathematical model for relative quantification in real-time RTPCR. Nucleic Acids Res. 29 (9), e45. doi: 10.1093/nar/29.9.e45
Picha M. E., Turano M. J., Beckman B. R., Borski R. J. (2008). Endocrine biomarkers of growth and applications to aquaculture: A minireview of growth hormone, insulin-like growth factor (IGF)-i, and IGF-binding proteins as potential growth indicators in fish. N. Am. J. Aquac. 70, 196–211. doi: 10.1577/A07-038.1
Policar T., Blecha M., Křišťan J., Mráz J., Velíšek J., Stará A., et al. (2016). Comparison of production efficiency and quality of differently cultured pikeperch (Sander lucioperca l.) juveniles as a valuable product for ongrowing culture. Aquacult. Int. 241607 –, 1626. doi: 10.1007/s10499-016-0050-9
Policar T., Schaefer F. J., Panana E., Meyer S., Teerlinck S., Toner D., et al. (2019). Recent progress in European percid fish culture production technology–tackling bottlenecks. Aquacult. Int. 27, 1151–1174. doi: 10.1007/s10499-019-00433-y
Quade M. J., Roth J. A. (1997). A rapid, direct assay to measure degranulation of bovine neutrophil primary granules. Vet. Immunol. Immunopathol. 58, 239–248. doi: 10.1016/S0165-2427(97)00048-2
Rahimnejad S., Lu K., Wang L., Song K., Mai K., Davis D. A., et al. (2019). Replacement of fish meal with Bacillus pumillus SE5 and Pseudozyma aphidis ZR1 fermented soybean meal in diets for Japanese seabass (Lateolabrax japonicus). Fish. Shellfish Immunol. 84, 987–997. doi: 10.1016/j.fsi.2018.11.009
Rahimnejad S., Zhang J. J., Wang L., Sun Y., Zhang Ch. (2021). Evaluation of Bacillus pumillus SE5 fermented soybean meal as a fish meal replacer in spotted seabass (Lateolabrax maculatus) feed. Aquaculture 531, 735975. doi: 10.1016/j.aquaculture.2020.735975
Refstie S., Korsøen Ø.J., Storebakken T., Baeverfjord G., Lein I., Roem A. J. (2000). Differing nutritional responses to dietary soybean meal in rainbow trout Oncorhynchus mykiss and Atlantic salmon Salmo salar. Aquaculture 190, 49–63. doi: 10.1016/S0044-8486(00)00382-3
Refstie S., Storebakken T., Roem A. J. (1998). Feed consumption and conversion in Atlantic salmon (Salmo salar) fed diets with fish meal, extracted soybean meal or soybean meal with reduced content of oligosaccharides, trypsin inhibitors, lectins and soya antigens. Aquaculture 162, 301–312. doi: 10.1016/S0044-8486(98)00222-1
Ringo E., Olsen R. E., Gifstad T.Ø., Dalmo R. A., Amlund H., Hemre G. I., et al. (2010). Prebiotics in aquaculture: a review. Aquac. Nutr. 16, 117–136. doi: 10.1111/j.1365-2095.2009.00731.x
Ringo E., Sperstad S., Myklebust R., Mayhew T. M., Olsen R. E. (2006). The effect of dietary inulin on bacteria associated with hindgut of Arctic charr (Salvelinus alpinus l.). Aquacult. Res. 37, 891–897. doi: 10.1111/j.1365-2109.2006.01509.x
Samrongpan C., Areechon N., Yoonpundh R., Srisapoome P. (2008). “Effects of mannan-oligosaccharide on growth, survival and disease resistance of Nile tilapia (Oreochromis niloticus) fry,” in Proceeding of 8th International Symposium on Tilapia in Aquaculture. Dept of Aquaculture, Faculty of Fisheries, Kasetsart University, Bangkok 10900, Thailand . 345–354 (Cairo, Egypt). Available at: https://ag.arizona.edu/azaqua/ista/ISTA8/FinalPapers/PDF/27%20Chinnapat%20Samrongpan.pdf.
Schulz C., Günther S., Wirth M., Rennert B. (2006). Growth performance and body composition of pike perch (Sander lucioperca) fed varying formulated and natural diets. Aquacult. Int. 14, 577–586. doi: 10.1007/s10499-006-9056-z
Shiau S. Y., Kwok C. C., Kwang J. Y., Chen C. M., Lee S. L. (1989). Replacement of fishmeal with soybean meal in male tilapia (Oreochromis niloticus × o. aureus) fingerling diets at a suboptimal protein level. J. World Aquacult. Soc 20, 230–235. doi: 10.1111/j.1749-7345.1989.tb01007.x
Shiau S. Y., Lin S. F., Yu S. L., Lin A. L., Kwok C. C. (1990). Defatted and full-fat soybean meal as partial replacement for fishmeal in tilapia (Oreochromis niloticus × o. aureus) diets at low protein level. Aquaculture 86401–407. doi: 10.1016/0044-8486(90)90328-K
Shimeno S., Kumon M., Ando H., Ukawa M. (1993). The growth performance and body composition of young yellowtail fed with diets containing defatted soybean meals for a long period. Nippon Suisan Gakkai shi. 59, 821–825. doi: 10.2331/suisan.59.821
Siwicki A. K., Anderson D. P. (1993). “Nonspecific defense mechanisms assay in fish. II. potential killing activity of neutrophils and macrophages, lysozyme activity in serum and organs and total immunoglobulin (T- ig) levels in serum,” in Fish diseases diagnosis and prevention’s methods (Olsztyn, Poland: FAO-Project GCP/INT/526/JPN; IFI), 105–112.
Specziár A. (2011). Size-dependent prey selection in piscivorous pikeperch Sander lucioperca and Volga pikeperch Sander volgensis haped by bimodal prey size distribution. Fish. Biol. 79 (7), 1895–1917. doi: 10.1111/j.1095-8649
Stejskal V., Kouril J., Policar T., Svobodová Z. (2016). Splenic lipidosis in intensively cultured perch, Perca fluviatilis l. J. Fish. Dis. 39, 87–93. doi: 10.1111/jfd.12327
Tacon A. G. J., Metian M. (2008). Global overview on the use of fish meal and fish oil in industrially compounded aquafeeds: Trends and future prospects. Aquaculture 285, 146–158. doi: 10.1016/j.aquaculture.2008.08.015
Tangendjaja B. (2015). Quality control of feed ingredients for aquaculture. Feed feeding practices aquacult. (Amsterdam), 141–169. doi: 10.1016/b978-0-08-100506-4.00006-4
Teng T., Zhao X., Li C., Guo J., Wang Y., Pan C., et al. (2020). Cloning and expression of IGF-I, IGF-II, and GHR genes and the role of their single-nucleotide polymorphisms in the growth of pikeperch (Sander lucioperca). Aquacult. Int. 28, 1547–1561. doi: 10.1007/s10499-020-00542-z
Tibaldi E., Uni Z., Francesco M., Luzzana U., Harpaz S., Tulli F., et al. (2006). Effects of the partial substitution of dietary fish meal by differently processed soybean meals on growth performance, nutrient digestibility and activity of intestinal brush border enzymes in the European seabass (Dicentrarchus labrax). Aquaculture 261, 182–193. doi: 10.1016/j.aquaculture.2006.06.026
Tomas A., de la Gandara F., Garcıa-Gomez A., Perez L., Jover M. (2005). Utilization of soybean meal as an alternative protein source in the Mediterranean yellowtail, Seriola dumerili. Aquac. Nutr. 11, (333–340). doi: 10.1111/j.1365-2095.2005.00365.x
Tran N., Chu L., Chan C. Y., Genschick S., Phillips M. J., Shula-Kefi A. (2019). Fish supply and demand for food security in Sub-Saharan Africa: an analysis of the Zambian fish sector. Mar. Policy 99, 343–350. doi: 10.1016/j.marpol.2018.11.009
Van Loo J., Cummings J., Delzenne N., Franck A., Hopkins M., MacFarlane G., et al. (1999). Functional food properties of non-digestible oligosaccharide: a consensus report from the ENDO project (DGXII AIRII-CT94–1095). Br. J. Nutr. 81121–132. doi: 10.1017/s0007114599000252
Viola S., Mokady U., Rappapor U., Arieli Y. (1982). Partial and complete replacement of fishmeal by soybean meal in feeds for intensive culture of carp. Aquaculture 26, 223–236. doi: 10.1016/0044-8486(82)90158-2
Wang Y., Kong L. J., Li C., Bureau D. P. (2006). Effect of replacing fish meal with soybean meal on growth, feed utilization and carcass composition of cuneate drum (Nibea miichthioides). Aquaculture 261, 1307–1313. doi: 10.1016/j.aquaculture.2006.08.045
Wang Y., Wang L., Zhang C. X., Song K. (2017). Effects of substituting fishmeal with soybean meal on growth performance and intestinal morphology in orange-spotted grouper (Epinephelus coioides). Aquac. Rep. 5, 52–57. doi: 10.1016/j.aqrep.2016.12.005
Wang L., Zhou H., He R., Xu W., Mai K., He G. (2016). Effects of soybean meal fermentation by Lactobacillus plantarum P8 on growth, immune responses, and intestinal morphology in juvenile turbot (Scophthalmus maximus l.). Aquaculture 464, 87–94. doi: 10.1016/j.aquaculture.2016.06.026
Watzl B., Girrbach S., Roller M. (2005). Inulin, oligofructose and immunomodulation. Br. J. Nutr. 93 (S1), S49–S55. doi: 10.1079/BJN20041357
Webster C. D., Yancey D. H., Tidewell J. H. (1992). Effect of partially or totally replacing fish meal with soybean meal on growth of blue catfish (Ictalurus furcatus). Aquaculture 103, 141–152. doi: 10.1016/0044-8486(92)90408-D
Xu Q., Yang Z., Chen S., Zhu W., Xiao S., Liu J., et al. (2022). Effects of replacing dietary fish meal by soybean meal Co-fermented using bacillus subtilis and enterococcus faecium on serum antioxidant indices and gut microbiota of crucian carp Carassius auratus. Fishes 7, 54. doi: 10.3390/fishes7020054
Yamamoto T., Iwashita Y., Matsunari H., Sugita T., Furuita H., Akimoto A., et al. (2010). Influence of fermentation conditions for soybean meal in a non-fish meal diet on the growth performance and physiological condition of rainbow trout Oncorhynchus mykiss. Aquaculture 309, 173–180. doi: 10.1016/j.aquaculture.2010.09.021
Ye H., Xu M., Liu Q., Sun Z., Zou C., Chen L., et al. (2019). Effects of replacing fish meal with soybean meal on growth performance, feed utilization and physiological status of juvenile obscure puffer. Takifugu obscurus Comp. Biochem. Physiol. Part - C: Toxicol. Pharmacol. 216, 75–81. doi: 10.1016/j.cbpc.2018.11.006
Yue Y. R., Zhou Q. C. (2008). Effect of replacing soybean meal with cottonseed meal on growth, feed utilization, and hematological indexes for juvenile hybrid tilapia, Oreochromis niloticus × o. aureus. Aquaculture 284, 185–189. doi: 10.1016/j.aquaculture.2008.07.030
Zaefarian A., Yeganeh S., Adhami B. (2017). Dietary effects of garlic powder (Allium sativum) on growth, blood indices, carcass composition, and lysozyme activity in brown trout (Salmo caspius) and resistance against Yersinia ruckeri infection. Aquac. Int. 25, 1987–1996. doi: 10.1007/s10499-017-0169-3
Zajic T., Mraz J., Samples S., Pickova J. (2013). Fillet quality changes as a result of purging of common carp (Cyprinus carpio l.) with special regard to weight loss and lipid profile. Aquaculture 400, 111–119. doi: 10.1016/j.aquaculture.2013.03.004
Zakes Z. (1999). The effect of body size and water temperature on the results of intensive rearing of pike-perch Stizostedion lucioperca (L.) fry under controlled conditions. Arch. Pol. Fish. 7, 187–199. doi: 10.2478/v10086-012-0020-4
Zhang C. N., Li X. F., Xu W. N., Jiang G. Z., Lu K. L., Wang L. N., et al. (2013). Combined effects of dietary fructo-oligosaccharide and Bacillus licheniformis on innate immunity, antioxidant capability and disease resistance of triangular bream (Megalobrama terminalis). Fish. Shellfish Immunol. 35, 1380–1386. doi: 10.1016/j.fsi.2013.07.047
Zhang C., Rahimnejad S., Wang Y., Lu K., Song K., Wang L., et al. (2018). Substituting fish meal with soybean meal in diets for Japanese seabass (Lateolabrax japonicus): effects on growth, digestive enzymes activity, gut histology, and expression of gut inflammatory and transporter genes. Aquaculture 483, 173–182. doi: 10.1016/j.aquaculture.2017.10.029
Zhang C. N., Tian H. Y., Li X. F., Zhu J., Cai D. S., Xu C., et al. (2014). The effects of fructo-oligosaccharide on the immune response, antioxidant capability and HSP70 and HSP90 expressions in blunt snout bream (Megalobrama amblycephala yih) under high heat stress. Aquaculture 433, 458–466. doi: 10.1016/j.aquaculture.2014.07.007
Keywords: antioxidant capacity, fish meal replacement, gene, innate immunity, pikeperch, prebiotic
Citation: Dadras H, Chupani L, Imentai A, Malinovskyi O, Esteban MA, Penka T, Kolářová J, Rahimnejad S and Policar T (2022) Partial replacement of fish meal by soybean meal supplemented with inulin and oligofructose in the diet of pikeperch (Sander lucioperca): Effect on growth and health status. Front. Mar. Sci. 9:1009357. doi: 10.3389/fmars.2022.1009357
Received: 05 August 2022; Accepted: 29 September 2022;
Published: 14 October 2022.
Edited by:
Amalia Pérez-Jiménez, University of Granada, SpainReviewed by:
H-Michael Habte-Tsion, University of Maine, United StatesSM Sharifuzzaman, University of Chittagong, Bangladesh
Copyright © 2022 Dadras, Chupani, Imentai, Malinovskyi, Esteban, Penka, Kolářová, Rahimnejad and Policar. This is an open-access article distributed under the terms of the Creative Commons Attribution License (CC BY). The use, distribution or reproduction in other forums is permitted, provided the original author(s) and the copyright owner(s) are credited and that the original publication in this journal is cited, in accordance with accepted academic practice. No use, distribution or reproduction is permitted which does not comply with these terms.
*Correspondence: Hadiseh Dadras, ZGFkcmFzQGZyb3YuamN1LmN6; ZmguZGFkcmFzQGdtYWlsLmNvbQ==