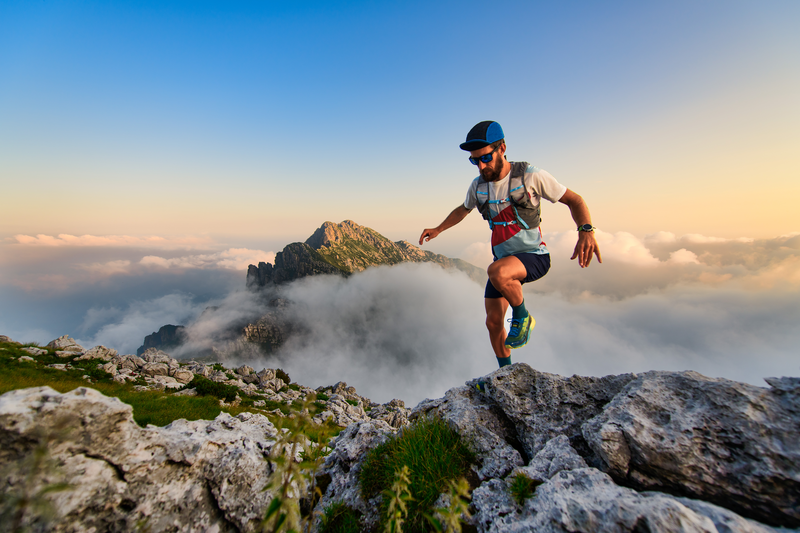
94% of researchers rate our articles as excellent or good
Learn more about the work of our research integrity team to safeguard the quality of each article we publish.
Find out more
ORIGINAL RESEARCH article
Front. Mar. Sci. , 07 October 2022
Sec. Marine Pollution
Volume 9 - 2022 | https://doi.org/10.3389/fmars.2022.1008912
This article is part of the Research Topic Coastal and Marine Environmental Quality Assessments View all 28 articles
The Mahanadi Estuarine System (MES), with a complex network of freshwater channels, rivers, and mangroves, is a leading seaport in State Odisha on the east coast of India, but subjected to intense human activity in recent years. Such anthropic impingements are known to impact sediment-dwelling biota adversely. However, information on the macrobenthic community of the MES is not well documented yet. Therefore, the primary objectives of this study (February 2013-March 2017) were to address knowledge gaps on the macrobenthic community structure vis-à-vis local environmental conditions and to evaluate the extent of anthropogenic disturbances on macrobenthos. The results from 264 benthic grab samples (van Veen, 0.04 m2; 2 replicates × 12 GPS fixed locations × 3 seasons) revealed 73 taxa representing 64 genera and 48 families of macrobenthic fauna. The polychaetes (81.41%) and crustaceans (15.42%) were significant faunal groups that contributed mainly to the benthic population and diversity. Multivariate approaches using benthic community attributes and biotic indices (AMBI and M-AMBI) as proxy measures of environmental disturbances proved effective for appraisal. The correlations between the environmental parameters (temperature, pH, salinity) and community estimates were statistically significant. Hierarchical clustering analysis disclosed three major groups (Global R 0.70; p < 0.002) influenced by tolerant/opportunist species. The lower abundance, richness, diversity, and dominance of opportunistic species mark the signs of environmental stress. The community health status remained unbalanced, as indicated by AMBI scoring. M-AMBI analysis contributed best in differentiating areas exposed to diverse impacts and indicated polluted community health status with moderate ecological quality. Our results reiterate the effective use of macrobenthos as bioindicators for ecological status and monitoring. The findings could be utilized for future monitoring assessments, translated into valuable information, and designed into well-defined sustainable management strategies for the MES.
● Comprehensive assessment of the macrobenthic fauna from a tropical mangrove-associated estuary in India.
● Differential benthic responses to anthropogenic interference examined through a suite of univariate and multivariate analyses.
● Less diverse benthic communities and more opportunistic species marked signs of local environmental stress.
● AMBI and M-AMBI indices differentiated benthic community health/ecological quality in relation to various anthropogenic impacts.
● Macrobenthos effective as bioindicators for habitat monitoring and assessment of the estuary.
● Recommendations for conservation and management of the Mahanadi estuary outlined.
Estuaries are naturally stressed bionetworks that exhibit a high degree of variability in their environmental conditions (Elliott and Quintino, 2007). These highly productive ecosystems have been the focal points for various human activities, of which rapid industrialization and indiscriminate urbanization are accountable for divergent pressures and ecosystem degradation (Lotze et al., 2006; John et al., 2017; Dash et al., 2021). Together with extreme climatic events, unprecedented demands of a rapidly increasing population for space, development, and resources have resulted in changes across global estuaries and started questioning the future of the estuaries (Kennish, 2002; Wetz and Yoskowitz, 2013; Elliott et al., 2019). Given the limitations of physicochemical approaches for determining the effects of such disturbances, the importance of macrobenthic investigations are frequently emphasized (Dauvin et al., 2016; Belal, 2019).
With extended life spans, sedentary lifestyles, and varying thresholds of sensitivity to ambient water/sediment conditions, benthic organisms are excellent bioindicators of prolonged environmental variations, mirrored through fluctuations in species composition and abundance (Veiga et al., 2016; Sany et al., 2018a). Furthermore, the distribution patterns of macrobenthos are susceptible to a wide range of anthropogenic interferences and show spatial and temporal shifts accordingly (Dash et al., 2021). Therefore, studies on the macrobenthic communities are increasingly adopted for assessing the health of the aquatic ecosystems (Mulik et al., 2017; Borja et al., 2019; Mulik et al., 2020a; Mulik et al., 2020b; Pandey et al., 2021; Dauvin et al., 2021; Subramanian et al., 2021).
Many tropical estuaries, particularly in South and Southeast Asia, are polluted and over-exploited (Bae et al., 2017; Sarathy et al., 2022). In India, the estuaries have been reported with altered or degraded environmental quality owing to the inadvertent growth of industries and metropolises on the banks of the major rivers (Sigamani et al., 2015; Feebarani et al., 2016; Mulik et al., 2017; Mitra et al., 2018; Mulik et al., 2020a; Mulik et al., 2020b). The increased loads of municipal and industrial effluents, in amounts higher than the assimilatory capacity of the system, are accumulating pollutants and causing hypoxia-like conditions (Mitra et al., 2018; Mulik et al., 2020a; Kumar et al., 2021). Despite the large deltas with some luxuriant estuarine mangrove cover along the east coast of India (in contrast to the west coast), studies that have looked at the effects of changed environmental conditions on macrobenthic fauna are largely limited (Raut et al., 2005; Ansari et al., 2017; Bhowmik and Mandal, 2021; Dash et al., 2021; Pandey et al., 2021).
The Mahanadi estuary at Paradip in the State of Odisha (Figure 1), a leading maritime gateway on the east coast of India, is not exempt from the severe anthropogenic disturbances in recent times (Nayak, 2020). Port/harbor expansion, dredging, increased marine traffic, loss of mangroves, and aquaculture development, amongst others, are some regularly seen activities in the vicinity. Such events, not to mention the additional burdens of pollution and changes in physicochemical conditions of the estuary, show adverse impacts on the sediment-dwelling benthic communities (Nayak et al., 2018), making this study highly relevant.
Figure 1 Mahanadi estuary in State Odisha on the east coast of India. The sampling stations (1-12) cover the ecotone influenced by the tides, i.e., from the river mouth to 13 km upstream. Examples of each habitat and sources of disturbances are denoted. (A) Denuded zone; (B, C) Mud flat and bird foraging zone, (D) IFFCO factory; (E) Fishing harbour, and (F) Mangrove associated zone.
Identifying the differential response of macrobenthos to environmental changes is crucial for their habitat protection. In this perspective, a suite of univariate and multivariate data analyses remains invaluable (e.g., Clarke et al., 2014; Sany et al., 2018b; Mulik et al., 2020a). Among others, the biotic indices such as AMBI (AZTI’s Marine Biotic Index) and M-AMBI (Multivariate AMBI) are robust and used widely for coastal environmental monitoring and benthic quality assessment (Borja et al., 2000; Bald et al., 2005; Muxika et al., 2007; Borja et al., 2014). In addition, these indices glean complex ecological information into easily communicable and understandable scores for possible conservation/management insights (Pinto et al., 2009).
The present study was primarily aimed to provide a state-of-the-art appraisal of the macrobenthos at Mahanadi estuary. The objectives were - (i) to identify macrobenthic communities and their composition in relation to local environmental conditions as an approach for ecological assessment and (ii) to evaluate the extent of anthropogenic disturbances on macrobenthos through the application of marine biotic indices as proxy measures of environmental degradation. This is the first long-term investigation of the hitherto poorly explored Mahanadi Estuarine System (MES). Therefore, it can form the basis for future environmental monitoring, assessment, and management of the region.
River Mahanadi, together with its three tributaries - Daya, Nuna, and Bhargavi, discharges into the Bay of Bengal in the State of Odisha on the east coast of India (20°17’08’’ N; 86°42’24’’ E) (Figure 1). The mixed semi-diurnal tides with a range of 1.45 to 2.20 m (mean, 1.29 m) reach 13 km upstream (depth 5.19 ± 1.11m) and support a rich diversity of the estuarine (mangrove) flora and fauna (Dey et al., 2013; Palei et al., 2014). According to the Indian Water Resources Information System (I-WRIS, 2021), Mahanadi is one of India’s largest and longest (494 km) river systems, with a catchment area of over 65,628 km2. The leading seaport, ‘Paradip’ - located 8 km south of the river mouth- is a hub for regional sea-borne trade. With a steadily rising population, the port township is fast developing as a core investment region for petroleum and chemicals (Hazra et al., 2020). Several industries like Indian Farmers and Fertilizers Cooperative Ltd., Paradip Phosphates Limited, Pellet Plant, Indian Oil Corporation Limited, Essar Steel, Ice manufacturing plants, Sea Food Processing units, besides many others, have sprung up in the immediate vicinity, with near denudation of once dense mangrove vegetation fringing the mudflats. Although fishing and cultivation are the traditional livelihood choices of the coastal residents, lucrative shrimp farming has gained precedence in recent years. As a result, the chemicals used for enhancing aquaculture production have been rampant. With the leaching of such substances into the waters, impacts on the estuary are imminent. Besides the untreated sewage from the township, effluents containing oil sludge, sulfur, ash, and gypsum released from industries are proven deterrent to the estuarine ecosystem (Hazra et al., opp. cit. and references therein; SPBO, 2020; Acharyya et al., 2021a; Acharyya et al., 2021b). Overall, the MES has become vulnerable to increased anthropogenic pressures and necessitates further assessment studies.
The climate of Mahanadi Delta is influenced by the premonsoon (March-May), postmonsoon (September-November), and winter (December-February) seasons every year. The southwest monsoon is the major monsoon season during which southwest directional winds blow from June to September. The northwest directional winds influence the winter months (December to February). The weather is typically marked by hot and humid conditions (31.46°C) in April-June and cool and dry (28.66°C) in December-January (https://www.worldweatheronline.com/). The southwest or summer monsoon (mid-June to September) brings heavy precipitation (average, 417.75 mm). The Mahanadi in spate discharges about 45,000 m3 s-1 into the Bay of Bengal (Mohanti and Swain, 2005), reducing salinity and increasing fluvial loads in the nearshore waters. Furthermore, the southwest monsoon winds generate waves up to ~3 m high or more (Dash et al., 2020). As a result, the coast remains wave-dominated throughout the southwest monsoon and mixed wave and tide-dominated in the non-monsoon periods (Mohanti and Swain, opp. cit.).
Both biotic (macrobenthos) and abiotic (water and sediment) samples were collected trimonthly for nearly five years, covering the premonsoon, postmonsoon, and winter seasons (February 2013– March 2017). However, the pre-and postmonsoon sampling in 2016-2017 could not be completed due to logistic constraints.
A total of 12 sampling stations - from the river mouth to 13 km upstream (Figure 1), were fixed and reached with the help of a handheld GPS (Garmin Etrex 72H, Taiwan). The geographic location of the stations also represents various land-use land-cover types and habitat functioning in the vicinity. For instance, St. 1 (river mouth), St. 2 (adjacent to fishing harbor), and St. 11 (upstream area) act as migratory bird’s foraging zone (BFZ). Similarly, Sts. 3-8 (adjoining mangroves) are the mangrove-associated zone (MAZ). Intensive shrimp farming (at Sts. 3, 6, and 7) and a factory named Indian Farmers Fertiliser Cooperative Limited (IFFCO) (at St. 8) are also present in the MAZ. The remaining Sts. 9, 10, and 12 adjacent to the country boat berthing facility and Essar Steel India Limited, a leading manufacturer and supplier of steel products and iron ore pellets, stand for the mangrove denuded zone (DZ). In addition, the two bifurcates of River Mahanadi at St. 12 bring considerable freshwater influx at the confluence (Figure 1).
Altogether, 264 sediment samples (in replicates) were collected with the help of a van Veen grab (0.04 m2). After separating a small fraction of the sediment (~25g) for textural and organic matter analyses, each grab sample was transferred to a 500 μm sieve and gently washed with the seawater for macrobenthos. All specimens retained on the sieve were fixed in 5% buffered formaldehyde with 1% Rose Bengal for further processing and identification in the laboratory. Species-level identification of the macrobenthos (where possible) was carried out under a stereomicroscope (Leica, E24W, Germany) by following the standard literature (Fauvel, 1953; Fauchald, 1977; Abbott and Dance, 1982; Subba Rao et al., 1991; Blake et al., 2009; Yokoyama and Sukumaran, 2012; Muir and Hossain, 2014; Hutchings and Kupriyanova, 2018). The World Register of Marine Species (http://www.marinespecies.org/index.php) was followed to validate the scientific names. The faunal density at each station was expressed as ind. m−2. Further, to estimate the wet biomass (gm-2), preserved specimens were separated into different groups, kept on a mesh, and then moisture blotted out carefully with absorbent paper and weighed (OHAUS PAJ603 electronic balance).
Sand, silt, and clay (%) compositions in the sediment were determined by the pipette method (Krumbein and Pettijohn, 1938) and assigned the textural classes (Shepard, 1954). The organic matter (OM) (%) was estimated by the modified Walkey-Black wet-oxidation method (Gaudette et al., 1974). In the case of hydrographical parameters, salinity (psu), dissolved oxygen (DO) (mg l-1), nitrite-nitrogen () (µmol l-1), and orthophosphate () (µmol l-1) were estimated by following the standard protocols (APHA, 1989; Grasshoff et al., 1999). Water temperature (using a mercury-filled thermometer of 0.5°C sensitivity), pH (using a Hanna HI 98107 with ±0.1 accuracy), depth (m) (using echo sounder), and transparency (m) (using a Secchi disc) were measured in situ. The instruments were calibrated before recording in situ variables in the field.
The faunal diversity indices such as mean abundance, species richness (S), and Shannon-Wiener index (H’) were computed using the PRIMER v.7 software (Plymouth Routines in Multivariate Ecological Research) (Clarke and Gorley, 2015). The (square root-transformed) data were used for Bray-Curtis similarity (clustering through hierarchical group-average linking) and non-metric multidimensional scaling (nMDS) ordinations. The significance of sample groupings was tested through the Analysis of Similarities (ANOSIM), whereas confirmatory evidence of the faunal assemblages was provided through the Similarity Profile Analysis (SIMPROF) (Clarke and Ainsworth, 1993). The species abundance matrix was represented by a shade plot where the gradation of shade from grey to black is linearly proportional to the increase in species abundance (Clarke and Gorley, 2015). The unconstrained binary divisive clustering (UNCTREE) analysis further examined both sample and species associations. The environmental data were normalized and subjected to Principal Component Analysis (PCA) to distinguish the sampling sites in relation to their (local) environmental conditions. The analysis of variance (ANOVA) tests was used to probe the spatial and temporal differences. The PCA was carried out using PRIMER v.7.
AMBI and M-AMBI indices were used to assess the gradient of anthropogenic stress across the MES. The index scores were derived from the AMBI v.6 software (http://www.ambi.azti.es). AMBI index is a univariate measure that uses a ‘differential weighting’ algorithm based on the classification of benthic species into five Ecological Groups (EGs) (i.e., EGI - species very sensitive; EGII - indifferent to enrichment; EGIII - tolerant to excess OME (organic matter enrichment); EGIV - second-order opportunistic species and, EGV -first-order opportunistic species) (Grall and Glémarec, 1997). The macrobenthos of Mahanadi estuary were assigned to different EGs by following the AMBI v.6 December 2020 taxa list. In addition, a few taxa not identified up to species level or not found in the AMBI database were also assigned for their respective EGs availing the WORMS database. However, 18.7% of total taxa were either not assigned to any EG or ignored due to irrelevant species (cf. Borja and Muxika, 2005).
The scores of the AMBI index were used to categorize the ecological quality of the estuary into five classes based on a scale from 0 to 7 (0-1.2: high, 1.2-3.3: good, 3.3-4.3: moderate, 4.3-5.5: poor and, >5.5: bad). On the other hand, M-AMBI is a multimetric index that derives scores from multiple factors (species richness, Shannon-Weiner diversity index, and AMBI scores). Further, it requires setting a reference condition (Muxika et al., 2007) of a high ecological quality ratio (EQR) of environmental and biological parameters specific to the habitat (Borja et al., 2012). Reference conditions are characterized by high biological and environmental quality elements giving the site a high ecological quality ratio (EQR) compared to the impacted site (Bigot et al., 2008). The Water Framework Directive (WFD) offers four criteria to select reference conditions: (1) pristine or minor disturbance, (2) historical data, (3) predictive modelling, and (4) expert judgment (Basset et al., 2013). Since finding the less disturbed or pristine environment is as difficult as getting the historical data in the era of ‘Anthropocene,’ it is always prudent to perform predictive modelling for setting the reference condition. Therefore, an internal control with high diversity, richness, and low AMBI from the dataset was used to set the reference target, and the M-AMBI scores were calculated as suggested by Muxika et al. (2007). The scores of M-AMBI were used to qualify the samples into five grades based on a scale from 0 to 1 (>0.77: high, 0.77–0.53: good, 0.53–0.38: moderate, 0.38–0.20: poor, and <0.20: bad). Procedures adopted to estimate indices scores, algorithm, boundary limits, and community health/ecological quality classifications were based on the WFD scale of indices detailed by Equbal et al. (2017).
The pairwise Mann–Whitney U test was applied to assess differences on a seasonal scale within the faunal groups. Differences were considered significant at p<0.05 for all power test analyses. Finally, Pearsons’ correlation coefficient test was carried out to find the possible influence of environmental parameters on biotic indices (Graph Pad Software, USA).
The water temperature ranged from 21.5 to 36.0°C (mean, 28.34 ± 1.08°C), with the lowest measurements during winter and the highest during premonsoon (Table 1). The upper reaches were relatively warmer than the lower reaches of the estuary (Figure 2). The water pH was slightly alkaline - especially downstream (>7.5), and varied significantly between the seasons (two-way ANOVA F = 19.89, p < 0.01). With increasing salinity from upstream to downstream, brackish water conditions (9.26 ± 1.87 psu) prevailed along the estuary’s entire (13 km long) stretch. Seasonal salinity changed in the order of winter > premonsoon > postmonsoon. The sampling stations in the proximity of industries (e.g., Sts. 8, 9, 10) showed less DO than those adjoining mangroves (Sts. 5, 6, 7). There was a significant difference in DO between pre-and postmonsoon periods (one-way ANOVA F =4.61, p< 0.05).
Table 1 Hydrographical and sediment characteristics of Mahanadi estuary during February 2013-March 2017.
Figure 2 Spatial distribution patterns of hydrographical (water temperature, salinity, dissolved oxygen, nitrite, and orthophosphate) and sediment (sand, silt, and organic matter) variables in Mahanadi estuary.
The distribution of dissolved nutrients revealed higher concentrations of (3.17 ± 0.75 μmol l-1) and (4.22 ± 1.06 μmol l-1) at the stations close to shrimp farms and industries (e.g., Sts. 7, 8, 11). Nutrient enrichment was high during the premonsoon, followed by postmonsoon and winter seasons (Table 1). The sampling stations subjected to higher anthropogenic intervention and upstream fluvial loads (Sts. 7-12) also had a lower water column transparency. The waters are more turbid for postmonsoon (0.35 ± 0.03) than premonsoon (0.55 ± 0.05) or winter (0.95 ± 0.09). Station-wise environmental parameters for each year and season are provided as Supplementary Data (STable 1).
The estuarine sediments were comprised mostly of sand (48.03 ± 6.52) and silt (51.81 ± 6.51) (Table 1), with three distinct textural classes (i.e., silty sand, sandy silt, and silt). The OM ranged from 0.07 to 6.63% and was rather high at the creek/canal confluence locations (Figure 2). The correlation between textural grades and OM was clear. For instance, sediments from the river mouth (St. 1) and upstream (St. 12) with a high sand composition contained less OM in contrast to the mangrove sediments (Sts. 2-9) with a high silt composition. Seasonally, silt was distinctive of the postmonsoon. The sediment textural classes also indicated significant spatial and temporal variations (two-way ANOVA, sand F=3.23, p < 0.05; silt F=3.18, p < 0.05; clay F=10.89, p < 0.01).
The PCA revealed significant eigenvalues (>1), and the percentage of variance was 31.9% for PC1, followed by 26.9% for PC2. While most stations in the MAZ with silt and OM were on the positive side of the two axes, the sites of the DZ with a sand abundance were on the negative side (Figure 3). Overall, the first axis had segregation of stations based on their sediment nature, and the second axis was based on their water characteristics. The stations belonging to BFZ were closely associated with the water quality (salinity, DO, pH, ) (Figure 3).
Figure 3 Principal Component Analysis (PCA) depicting the influence of environmental variables on the sampling (1-12) sites of the Mahanadi estuary during 2013-2017.The circle represents the correlation circle, and the orientation of the environmental parameters (lines) approximate their correlation to each other and to the ordination axes (DO = dissolved oxygen, Sal = salinity, OM = total organic matter, = nitrite-nitrogen, PO4- = orthophosphate, WT = water temperature). The MAZ (n = 66) – Mangrove associated zone includes St. 3, 4, 5, 6, 7, and 8, The BFZ (n = 33) – Birds foraging zone includes St. 1, 2 and 11, and the DZ (n = 33) – Denuded zone is represented by St. 9, 10 and 12.
The present study recorded 100,500 individuals from 73 taxa, 64 genera, and 48 families represented by major faunal groups such as Polychaeta, Brachiopoda, Sipuncula, Crustacea, Mollusca, Echinodermata, and Pisces. However, polychaetes (81.41%), and crustaceans (15.42%) were found to be important in terms of their abundance (Table 2). Within Polychaeta, families such as Spionidae (9 species), Capitellidae (4 species), and Nereididae (4 species) were the most diverse and dominant groups, contributing 48% to the total population. The notable species of polychaetes were nereid Perinereis cavifrons, nephtyid Micronephthys oligobranchia, spionids Dipolydora coeca, Malacoceros indicus, and Polydora cornuta. The important species of crustacean and molluscs included Victoriopisa chilkensis, Psammacoma gubernaculum, and Nassarius stolatus, with their juvenile populations (Table 2).
Table 2 Numerical abundance of macrobenthic fauna and their assignment to ecological groups (EGs-Ecological groups, NA-Not assigned, IG-Ignored, SD-standard deviation).
The macrobenthic faunal abundance ranged between 50 and 3,213 ind. m-2 (760 ± 727) (Table 2) was high at the BFZ in winter (mean, 2,288 ind. m-2) and postmonsoon (3,213 ind. m-2). In particular, the prevalence of P. cavifrons for these two seasons (773-970 ind. m-2) is noteworthy. The faunal abundance of the BFZ varied significantly between premonsoon and postmonsoon (Mann–Whitney test, p = 0.00), whereas it differed between premonsoon and winter for the MAZ (Mann–Whitney, p = 0.03). The wet weight biomass was low in upstream (e.g., 0.03 gm-2 at St. 9) than in the downstream areas (25 gm-2 at St. 2). The macrobenthic faunal abundance data (Polychaeta, Mollusca, and Crustacea) tested for seasonal, species and station wise changes together with their interaction effects revealed significant differences, particularly for the molluscs (Table 3). Station-wise data on macrobenthic faunal abundance for each season were provided in the supplementary information (STable 2).
Table 3 Result of three-way ANOVA of macrobenthic faunal abundance data (Polychaeta, Mollusca, and Crustacea): Comparing the significance of seasonal, species, station differences, and their interaction effects (*p< 0.05, **p< 0.01, ***p< 0.001).
The abundance and distribution of 49 macrobenthic species at one or more sampling stations in the estuary accounted for ≥1% of the total population. Hierarchical clustering and nMDS plots have shown a clear-cut separation of the sampling stations into three major faunal groups (Global R 0.70; p < 0.002) (Figure 4). Group I, representing the BFZ (Sts. 1, 2, and 11), was dominated by Perinereis cavifrons, Cossura coasta, and Dendronereis aestuariana species (π = 1.07, p < 0.07), whereas Group II, for the MAZ (Sts. 3-8), is characterized by Micronephtys-Victoriopisa-Heteromastus assemblage. The sampling sites of DZ (Sts. 9, 10, and 12) as Group III contained Cossura-Dipolydora-Malacoceros assemblage. The UNCTREE analysis further confirmed the presence of these three faunal clusters (BFZ - Global R: 1, B%: 31.1, π: 2.59; MAZ- Global R: 0.61, B%: 48, π:1.06; DZ - Global R: 1, B%: 64.2, π: 1.07 (Figure 5).
Figure 4 (A) Bray-Curtis similarity and (B) n-MDS ordination showing sampling (1-12) site groupings based on macrobenthic abundance data in the Mahanadi estuary (similarity: 48.7%).
Figure 5 Macrobenthic communities of Mahanadi estuary in 2013-2017: Shade plot, a visual representation of abundances (averaged over seasons) of 49 macrobenthic species accounting for ≥ 1% or ˜1% of the total abundance at one or more sampling sites. Groups are named Bird foraging zone, Mangrove associated zone, and Denuded zone with linear greyscale intensity proportional to square root transformation abundance (ind.m-2). The three significantly different sub-clusters marked by SIMPROF-powered UNCTREE analysis (X-axis) are evident.
Altogether, 61 macrobenthic species (out of 73) were categorized into their corresponding EGs (Table 2). Except for EGV (one species), the other groups were diverse and represented by 12-17 taxa. The highest faunal (mean) density was contributed by EGIV (36.4%), followed by EGII (20.8%), EGIII (20.5%), EGI (19.5%), and EGV (2.7%). The species such as Heteromastus filiformis, C. coasta, D. coeca (of EGIV), P. cavifrons (EGIII), M. oligobranchia (EGII), and V. chilkensis (EGI) were abundant, and collectively formed 60% of the total population. The faunal composition of MAZ (41.5%) and DZ (38.3%) was dominated by EGIV organisms, while BFZ (36.6%) by the EGIII (Figure 6). Seasonally, both premonsoon (41.7%) and winter (35.3.%) periods were characterized by EGIV and the postmonsoon (35.3.%) by EGIII species.
Figure 6 (A) Ecological groups associated with stations/zones of Mahanadi estuary; (B) AMBI and (C) M-AMBI seasonal variations across stations/zones in Mahanadi estuary.
According to the AMBI scores, the estuary’s ecological quality was good throughout except for St. 8, which had moderate conditions (Table 4). The AMBI scores improved from the premonsoon to the winter period. In this context, the samples with poor to moderate status from MAZ and BFZ (Sts. 2 and 7-9) in the premonsoon were represented by moderate to good status during the postmonsoon and winter (Figure 6B). The differences between premonsoon and winter were significant (Mann-Whitney, p = 0.01). Also, the sampling stations in the DZ were depicted with good ecological quality. However, in terms of community health, the estuary was unbalanced and polluted (Table 4).
Table 4 Results of AMBI, M-AMBI and biodiversity of macrobenthos from Mahanadi estuary, during February 2013-March 2017.
In contrast to AMBI, the M-AMBI scores classified around 60% of the samples as moderate and 33.3% as good quality. M-AMBI followed a trend similar to that of AMBI, improving sample grades from the premonsoon to the winter period (Figure 6C). Except for St. 12 and St. 9 (DZ), where samples were downgraded from good (premonsoon) to moderate (postmonsoon and winter) and moderate (premonsoon and postmonsoon) to poor condition (winter). A unidirectional shift in quality grades was observed at St. 2 (BFZ), where samples upgraded from poor (premonsoon) to moderate (postmonsoon) and good condition (winter). In contrast, the samples from Sts. 6, 7, and 8 (MAZ) and St. 10 (DZ) showed quality improvement only during the winter period (Figure 6C). Both spatial and temporal differences in the M-AMBI scores of the BFZ (ANOVA F=9.2, P <0.001) and the MAZ were significant (F=.3, P=0.04).
The Pearson’s correlation coefficients indicate a significant relationship between water quality parameters and benthic (AMBI and M-AMBI) indices, whereas sedimentary attributes did not show any marked association (Table 5). Among the water quality parameters, temperature, pH, and salinity were influential in assessing community health. The distribution of macrobenthos in EGI, EGIII, and EGIV seems to have been governed by temperature, pH, salinity, and DO conditions (Table 5).
As physically controlled ecosystems, estuaries are characterized by wide variabilities in their local environmental conditions. The environment, however, becomes imbalanced and stressed when exposed to increased anthropogenic stressors (Kennish, 2005; Brown et al., 2022; Suzzi et al., 2022).
Among the environmental variables observed for the MES, the water temperature was strongly marked by seasonality. In this case, other atmospheric influences, such as precipitation, nearshore sea temperatures, and river run-offs, are likely to influence its variability (Leal Filho et al., 2022). High water temperature in the premonsoon was evident in the present study. The other traceable stressors like municipal wastes, sewage, and litter from Paradip port city, fisher hamlets, and fishing harbour that enter the estuary through Atharbanki, a mangrove channel, are also found to affect the water quality to a greater extent. Nutrient enrichment at the stations close to BFZ, shrimp farms, and the fertilizer industry (Sts. 1-3 and 6-8) can be explained by avifaunal excrements and untreated effluents. The low pH in the proximity of industries (Sts. 8-9) is attributable to the influx of acidic wastes (Sundaray et al., 2009; Acharyya et al., 2021a; Acharyya et al., 2021b). On the other hand, (acidic) humic substances carried by freshwater in colloidal suspension get coagulated upon meeting the seawater and can shift the pH to an alkaline condition (Beer, 1996). The latter was apparent from the downstream measurements at the Mahanadi estuary and was similar to findings from other estuaries elsewhere (Mohanty, 2018; Habib et al., 2021; Jabir et al., 2021).
The east-flowing rivers in India, the Brahmaputra, Ganges, Irrawaddy, Godavari, Mahanadi, Krishna, and Cauvery, contribute significantly to the total freshwater discharge into the Bay of Bengal (BoB) (from 1.5 × 1012 m3 to 1.83 × 1013 m3 per year) (Varkey et al., 1996; Thadathil et al., 2002). These rivers have a maximum climatological discharge between July and September when the southwest monsoon rainfall is at its peak and decreases gradually to a minimum during the winter and premonsoon (Sandeep and Pant, 2019). Hence, salinity with an increasing gradient towards the mouth of the estuary was low for the wet (monsoon) season and high for the dry (winter) season. Higher DO at Sts. 5-7 of the MAZ could be due to sustained autotrophic production in the waters. This process originates through nutrient-rich outwelling from the adjacent mangroves during inundation regimes (Cohen et al., 2004; Prasad and Ramanathan, 2008). However, land-based sulphate leaching from the gypsum dumps (heaped close to the fertilizer industry), and industrial effluents, besides others, are the drivers of near hypoxia conditions at Sts. 8-10 (cf. Nayak et al., 2018; Taillardat et al., 2020; Sharma et al., 2022). Depletion in DO is a strong indicator of poor water quality (Costa et al., 2018). Such low DO can prolong hypoxia and affect major estuarine ecosystem-level processes, including fluxes and rates linked to carbon and nitrogen biogeochemical cycling. Therefore, maintaining the best possible conditions across the river basins, including coastal and offshore waters, is essential (Karydis and Kitsiou, 2013; Barletta et al., 2019) and crucial for ecosystem functioning and maintaining estuarine biodiversity (Ghosh et al., 2022).
Fine sediment deposition is difficult in the regions where strong water currents persist (Mitchell, 2020). Low fine particle accretion, sandy texture, and lesser OM at seaward (St. 1) and near confluence sites (Sts. 10, 12) support this phenomenon in the study area. Similarly, mud (silt and clay) accruals occurred mainly in sheltered seascapes (mangrove-fringed sites, Sts. 3-8) due to weak tidal currents and land-based fine particle sources. Muddy sediments retained more OM (>2%) than sandy sediments because of the better adsorption capacity of the fine-grained particles (Gaonkar et al., 2021; Haddout et al., 2022). Furthermore, litter from mangrove vegetation (leaves, propagules, and twigs) and subsurface root growth significantly deliver organic carbon to mangrove sediments (Alongi, 1998). Therefore, sites adjoining mangroves revealed higher OM arising from autogenic sources and outwelling (Hossain et al., 2014; Mohanty et al., 2019).
Reliable information on aquatic resources is key to improving their management (Karydis and Kitsiou, 2013). Furthermore, the competence in accommodating changes (episodic or permanent) to managerial plans requires an in-depth understanding of the drivers of water quality deviations and the availability of natural resources at different timescales (Costa et al., 2018). Anthropogenic activities have significantly threatened the Mahanadi estuary’s water quality and biota. As a result, continuous monitoring and potential corrective measures are required to mitigate such effects.
Studies on the macrobenthos of the MES and adjacent mangrove waterways/intertidal mudflats are confined mainly to taxonomic accounts, without much inference to the local environmental conditions (Deb, 1998; Pattanayak and Haldar, 1998; Rao, 1998; Surya Rao and Maitra, 1998; Nayak et al., 2018; Tudu et al., 2018; Mohanty et al., 2022). In recent years, industrial expansion has become disproportionately rapid along major waterways due to well-connected transport hubs and habitation sites, as is the case for the MES. The complex network of creeks and canals acting as vectors and sink for the effluents released from the port town, fishing harbour/landing centers, industrial units, etc. (Rodgers et al., 2020) shows significant consequences on the benthic communities.
Macrobenthic diversity and dominance patterns in the Mahanadi estuary reflected varying degrees of natural and man-induced stressors. Spatial and temporal benthic faunal distribution appeared to be primarily structured by sediment texture, OM, salinity, and pH. For instance, the abundance of polychaetes in the estuary could represent their tolerance to a wide array of (shifting/changing) environmental conditions (Sanchis et al., 2021). The deposit-feeding P. cavifrons is an important link between detritus accumulation and higher trophic levels. As an opportunist, P. cavifrons can repopulate in defaunated areas where the perturbations like dredging (St. 2, opposite to fishing harbour) and mangrove denudation (sites in the proximity of industries) occur. Furthermore, the capitellids, including Heteromastus, spionids, and Magelona spp., exhibit a natural proliferation in fine-muddy sediments (Afli et al., 2008; Sivadas and Ingole, 2016), while Paraprionospio and Magelona spp. survive in hypoxic and lesser competitive environments inhospitable to their predators and competitors (Sivadas et al., 2021). The impoverished faunal trends noticed towards the estuary mouth (St. 1) and upstream (St.12) also confirm the observations of Shirodkar and Nayak (2010). Macrobenthos in the proximity of shrimp farms and industries (IFFCO, Essar Steel) could represent the impacted environmental conditions as pollution indicators (Borja et al., 2000; Dauvin et al., 2012; Albano et al., 2013; Shivarudrappa et al., 2019). Seasonally varied diversity indices are perceptible, with maximum abundance in the winter (Kundu et al., 2010). The findings of this study were consistent with other regional (Mulik et al., 2017; Dias et al., 2018; Rehitha et al., 2019; Sivadas et al., 2021; Subramanian et al., 2021; Kumar et al., 2022; Rehitha et al., 2022) and global (Abrogueña et al., 2021; Bravo et al., 2021; Dauvin et al., 2021; Salimi et al., 2021; Sánchez-Ovando et al., 2021; Kanhai, 2022) benthic evaluations.
An estuary is a dynamic environment where the macrobenthos are adapted to live in widely shifting environmental conditions over relatively short distances (Ortega et al., 2018). The benthic assemblages of Mahanadi estuary are distinct in relation to the local environmental settings. For example, the heterogeneity in Micronephtys-Victoriopisa-Heteromastus assemblage of the MAZ could represent anthropic interventions (through industries, ferry traffic, mangrove destruction, and shrimp farms) modifying the habitat and its preference for the sediment composed of silty-sand and high OM. Also, Cossura-Dipolydora-Malacoceros assemblage of the DZ, under similar man-made disturbances (boat berthing, Essar steel), occupied the sediment with silt and high OM. Therefore, each zone showed the macrofaunal responses to diverse stressors in the vicinity. The effect could be revealed through a low diversity, which testifies to the relationship between biodiversity loss and human-induced disturbances (D’Alessandro, 2020).
Apart from the anthropogenic interventions, monsoon-mediated perturbations also lead to a high degree of substrate heterogeneity and changes in the structure and function of biological communities in the estuary (Sivadas et al., 2011). The benthic community attributes such as abundance, richness, and diversity fluctuate mostly through defaunation, migration, and spawning of species with recovery followed by stable conditions, recruitment, and resettlement (Gaonkar et al., 2013; Sivadas et al., 2021). As a result, tolerant or opportunist species often dominate these communities, exhibiting a highly dynamic population under specific situations (Sivadas et al., 2016). The health assessment of the MES based on the biotic indices demonstrated a high agreement with inferences extracted from benthic community structures against natural or anthropogenic disturbances.
The dominance of P. cavifrons, Heteromastus filiformis, and Dipolydora coeca in organically rich and contaminated sediments consequently changed the ecological quality status of MAZ and DZ from poor to moderate. In contrast, good to moderate conditions at sandy sediments (BFZ) suggest that low organic content is also favourable to EGI and EGIII forms (Sigamani et al., 2015). Overall, the present study affirms both MAZ and DZ as polluted, with benthic communities of tolerant (EGIII) and second-order opportunistic (EGIV) species. The Odisha Pollution Control Board (OSPCB) estimates untreated domestic wastewater discharge from urban settlements in the Mahanadi basin at 3,45,000 m3 (m3 = 1000 liters) per day, producing biochemical oxygen demand (BOD) load of about 68.8 tonnes daily. The water quality index (WQI) of the Paradip coastal waters (riverine and estuarine stretch) also indicated a deteriorating trend from “grade C” in 2013-15 to “grade D” in 2017 (SPCB report card, 2020).
The benthic indices obtained through quality-based evaluation in the present study reflect different stages of the estuarine environmental conditions. Large-scale variability in the environmental parameters and ecological quality status of the benthic habitat at each station (or zone) could be attributable to the heterogeneous MES (Elliott and Quintino, 2007). Temporal variation of macrobenthic communities was evident through either increased or decreased benthic indices depending upon life strategy (r-selected and k-selected) and niche performance (sensitive, indifferent and tolerant) of the individual species (Equbal et al., 2017). The improved sample quality between premonsoon and winter is suggestive of satisfactory performance by the benthic indices during stable, less turbulent, and low seasonal impact periods (Kröncke and Reiss, 2010; Karakassis et al., 2013; Sigamani et al., 2015; Bae et al., 2016; Chan et al., 2016; Feebarani et al., 2016; Sivadas et al., 2016; Mulik et al., 2017). In fact, for many estuaries on the east coast of India, premonsoon exhibit the most stressed conditions with excess and continuous accumulation of contaminants (Mitra et al., 2018). However, a good flush-out of pollutants is only possible during the monsoon season (Mulik et al., 2020a), aided further by a breakdown of contaminants owing to changing redox chemistry and consequences on benthic-pelagic coupling (Ghosh et al., 2022).
The applied biotic indices evaluated the benthic quality status by discriminating the highly disturbed from the less disturbed areas of the estuary. While the robustness of the M-AMBI (compared to AMBI) in sample quality assessment was explained by several researchers (Khan et al., 2014; Equbal et al., 2017; Pandey et al., 2021), a few others have observed its reverse performance due to lack of reference conditions and spatial benthic variability, heterogeneity within the estuarine complexes (Sigamani et al., 2015). According to Borja and Tunberg (2011), both AMBI and M-AMBI are sensitive to detecting human-mediated disturbances modified by natural disturbances. However, for regions strongly influenced by season, coastal dynamics, and anthropogenic disturbances, the univariate AMBI could show a high degree of variability than the multimetric M-AMBI (Kröncke and Reiss, 2010). The temporal variability of AMBI was indeed found to be less than M-AMBI (Equbal et al., 2018). Nevertheless, the marine biotic index, especially the M-AMBI, appeared to be more robust and realistic in grading the habitat quality into different classes. Therefore M-AMBI index can be used as far as the assessment of benthic habitat quality of the MES is concerned.
Considering the Mahanadi estuary’s polluted health status, some holistic management initiatives are required immediately. The government can enforce authority and share responsibilities with local stakeholders, academic institutions, and non-governmental organizations as partners (Romañach et al., 2018). Regular monitoring of the environmental conditions and mangrove cover through advanced research (by observing heavy metal concentrations, application of Remote Sensing and GIS, and others) and citizen science approaches are essential (Zauki et al., 2019; Wolswijk et al., 2020; Durango-Cordero et al., 2021; Gopalakrishnan et al., 2021; Wolswijk et al., 2022). Afforestation of degraded mangrove zones, embankment of tidal rivers - without affecting zonation/succession of the wetland species, and biodiversity conservation (as mandated by the Department of Forest and Environment, Government of Odisha) are some crucial endeavours in the right direction. Moreover, the impact of local pollution on human health should be apprised through appropriate awareness campaigns. The approaches of this kind would be able to lessen the present-day waste discharge, habitat fragmentation, and ecological functioning damages.
The present study unveiled the macrobenthic community of the mangrove-associated MES and demonstrated the impacts of anthropogenic intervention through an ecological approach. Significant sources of anthropic stressors (municipal wastes, sewage, litter, acidic discharges from industries, nutrients, and shrimp farm run-offs) were influential in structuring the macrobenthic assemblages of the MES on spatial and temporal scales. The dominance of opportunistic (second-order opportunists, EGIV) and tolerant (EGIII) species marked the signs of environmental stress, regardless of the sources of disturbance, i.e., natural or anthropogenic. The higher (mean) abundance of opportunists and tolerant species in the MAZ and DZ was mainly caused by OM enrichment that was further modified by the industrial and shrimp farming effluents. Marine biotic indices, especially the M-AMBI, informed the MES’s current state of ecological quality. The improved benthic community health from moderate to good in the premonsoon and winter was largely due to stable environmental conditions, allowing multiple species to co-exist in the light of reduced tolerant/opportunist species. The findings of this study also identified a set of environmental factors (water temperature, nutrient enrichment, salinity, DO, pH) influencing the macrobenthic assemblages of the MES. Evaluating the structure of macrobenthos in relation to anthropogenic/natural pressures and impacts provided the much-needed breadth of knowledge to help the management authorities formulate appropriate conservation strategies/policies. Given the importance of aquatic ecosystems for livelihood and climate change mitigation, the coastal and estuarine ecosystems must be protected so that their goods and services are sustainably utilized in the present and also availed by future generations.
The original contributions presented in the study are included in the article/Supplementary Material. Further inquiries can be directed to the corresponding authors.
AN: Methodology, Field Investigation, Laboratory analysis, Data curation. JE: Software, Data curation, writing. SS: Field Investigation, Laboratory analysis, Figures. BD: Field Investigation, Laboratory analysis, Figures. GT: Software validation, manuscript preparation. DR: Conceptualization, Original draft preparation, and writing. BS: review and editing. PB: review and editing. All authors contributed to the article and approved the submitted version.
The University Grants Commission, New Delhi, India (File No. 41-64/2012 (SR) dated 11-JUL-2012) funded the research. The Open Access funding was provided by the Mangrove Research Unit (MARU), Institute of Oceanography and Environment, Universiti Malaysia Terengganu, Malaysia.
The University Grants Commission, New Delhi, India (File No. 41-64/2012 (SR) dated 11-JUL-2012) funded the research. The authors are grateful to the Principal Chief Conservator of Forests (Wildlife) and Chief Wildlife Warden, Government of Odisha, for their kind permission to conduct this research work at Mahanadi estuary. Laboratory facilities at the Department of Zoology, Ravenshaw University, are appreciated. Thanks to the reviewers for the suggestions and comments that immensely helped to improve the manuscript.
The authors declare that the research was conducted in the absence of any commercial or financial relationships that could be construed as a potential conflict of interest.
All claims expressed in this article are solely those of the authors and do not necessarily represent those of their affiliated organizations, or those of the publisher, the editors and the reviewers. Any product that may be evaluated in this article, or claim that may be made by its manufacturer, is not guaranteed or endorsed by the publisher.
The Supplementary Material for this article can be found online at: https://www.frontiersin.org/articles/10.3389/fmars.2022.1008912/full#supplementary-material
Abrogueña J. B. R., Joydas T. V., Pappathy M., Cali N. A., Alcaria J., Shoeb M. (2021). Structure and composition of the macrobenthic community associated to shallow mangrove-seagrass habitat along the Southern Red Sea coast, Saudi Arabia. Egypt. J. Aquat. Res. 47, 61–66. doi: 10.1016/J.EJAR.2020.10.001
Acharyya T., Sudatta B. P., Raulo S., Singh S., Srichandan S., Baliarsingh S. K., et al. (2021a). “A systematic review of biogeochemistry of Mahanadi river estuary: Insights and future research direction,” in Estuarine biogeochemical dynamics of the East coast of India (Switzerland: Springer, Cham), 57–80.
Acharyya T., Sudatta B. P., Srichandan S., Baliarsingh S. K., Lotliker A. A., Raulo S., et al. (2021b). Deciphering long-term seasonal and tidal water quality trends in the Mahanadi estuary. J. Coast. Conserv. 25, 1–16. doi: 10.1007/s11852-021-00843-2
Afli A., Ayari R., Zaabi S. (2008). Ecological quality of some Tunisian coast and lagoon locations, by using benthic community parameters and biotic indices. Estuar. Coast. Shelf Sci. 80, 269–280. doi: 10.1016/J.ECSS.2008.08.010
Albano M. J., da Cunha Lana P., Bremec C., Elías R., Martins C. C., Venturini N., et al. (2013). Macrobenthos and multi-molecular markers as indicators of environmental contamination in a south American port (Mar del Plata, southwest Atlantic). Mar. Pollut. Bull. 73, 102–114. doi: 10.1016/J.MARPOLBUL.2013.05.032
Ansari K. G. M. T., Pattnaik A. K., Rastogi G., Bhadury P. (2017). Multiple spatial scale analysis provide an understanding of benthic macro-invertebrate community structure across a lagoonal ecosystem. Wetlands 37, 277–287. doi: 10.1007/s13157-016-0866-0
APHA (1989). Standard methods for the examination of water and wastewater. 17th Ed (Washington DC: American Public Health Association), 1550.
Bae M. J., Chun J. H., Chon T. S., Park Y. S. (2016). Spatio-temporal variability in benthic macroinvertebrate communities in headwater streams in south Korea. Water 8 (3), 99. doi: 10.3390/w8030099
Bae H., Lee J. H., Song S. J., Park J., Kwon B. O., Hong S., et al. (2017). Impacts of environmental and anthropogenic stresses on macrozoobenthic communities in Jinhae Bay, Korea. Chemosphere 171, 681–691. doi: 10.1016/J.CHEMOSPHERE.2016.12.112
Bald J., Borja A., Muxika I., Franco J., Valencia V. (2005). Assessing reference conditions and physico-chemical status according to the European water framework directive: A case-study from the Basque country (Northern Spain). Mar. Pollut. Bull. 50, 1508–1522. doi: 10.1016/J.MARPOLBUL.2005.06.019
Barletta M., Lima A. R. A., Costa M. F. (2019). Distribution, sources and consequences of nutrients, persistent organic pollutants, metals and microplastics in south American estuaries. Sci. Total Environ. 651, 1199–1218. doi: 10.1016/J.SCITOTENV.2018.09.276
Basset A., Barbone E., Borja A., Elliott M., Jona-Lasinio G., Marques J. C., et al. (2013). Natural variability and reference conditions: Setting type-specific classification boundaries for lagoon macroinvertebrates in the Mediterranean and Black Seas. Hydrobiologia 704 (1), 325–345. doi: 10.1007/s10750-012-1273-z
Beer T. (1996). Environmental Oceanography. 2nd ed (Boca Raton: CRC Press). doi: 10.1201/9780203756980
Belal A. A. M. (2019). Macro-benthic invertebrates as a bio-indicator for water and sediment quality in Suez Bay, Red Sea. Egypt. J. Aquat. Res. 45, 123–130. doi: 10.1016/j.ejar.2019.03.003
Bhowmik M., Mandal S. (2021). Do seasonal dynamics influence traits and composition of macrobenthic assemblages of Sundarbans estuarine system, India? Oceanologia 63, 80–98. doi: 10.1016/j.oceano.2020.10.002
Bigot L., Grémare A., Amouroux J. M., Frouin P., Maire O., Gaertner J. C. (2008). Assessment of the ecological quality status of soft-bottoms in Reunion Island (tropical southwest Indian Ocean) using AZTI marine biotic indices. Mar. Pollut. Bull. 56, 704–722. doi: 10.1016/J.MARPOLBUL.2007.12.020
Blake J. A., Maciolek N. J., Ota A. Y., Williams I. P. (2009). Long-term benthic infaunal monitoring at a deep-ocean dredged material disposal site off northern California. Deep Sea Res. Part II Top. Stud. Oceanogr. 56, 1775–1803. doi: 10.1016/J.DSR2.2009.05.021
Borja A., Chust G., Muxika I. (2019). Forever young: The successful story of a marine biotic index. 1st ed (United Kingdom: Elsevier Ltd). doi: 10.1016/bs.amb.2019.05.001
Borja Á., Dauer D. M., Grémare A. (2012). The importance of setting targets and reference conditions in assessing marine ecosystem quality. Ecol. Indic. 12, 1–7. doi: 10.1016/J.ECOLIND.2011.06.018
Borja A., Franco J., Pérez V. (2000). A marine biotic index to establish the ecological quality of soft-bottom benthos within European estuarine and coastal environments. Mar. Pollut. Bull. 40, 1100–1114. doi: 10.1016/S0025-326X(00)00061-8
Borja Á., Marín S., Núñez R., Muxika I. (2014). Is there a significant relationship between the benthic status of an area, determined by two broadly-used indices, and best professional judgment? Ecol. Indic. 45, 308–312. doi: 10.1016/J.ECOLIND.2014.04.015
Borja A., Muxika I. (2005). Guidelines for the use of AMBI (AZTI’s marine biotic index) in the assessment of the benthic ecological quality. Mar. Pollut. Bull. 7, 787–789. doi: 10.1016/j.marpolbul.2005.04.040
Borja A., Tunberg B. G. (2011). Assessing benthic health in stressed subtropical estuaries, eastern Florida, USA using AMBI and m-AMBI. Ecol. Indic. 11, 295–303. doi: 10.1016/J.ECOLIND.2010.05.007
Bravo G., Livore J. P., Battini N., Gastaldi M., Lauretta D., Brogger M., et al. (2021). Rocky reef biodiversity survey: Punta Pardelas, Argentina. Biodivers. Data J. 9, e72081. doi: 10.3897/BDJ.9.e72081
Brown A. M., Bass A. M., Pickard A. (2022). Anthropogenic-estuarine interactions cause disproportionate greenhouse gas production: A review of the evidence base. Mar. Pollut. Bull. 174, 113240. doi: 10.1016/j.marpolbul.2021.113240
Chan A. K. Y., Xu W. Z., Liu X. S., Cheung S. G., Shin P. K. S. (2016). Sediment characteristics and benthic ecological status in contrasting marine environments of subtropical Hong Kong. Mar. Pollut. Bull. 103, 360–370. doi: 10.1016/J.MARPOLBUL.2015.12.032
Clarke K. R., Ainsworth M. (1993). A method of linking multivariate community structure to environmental variables. Mar. Ecol. Prog. Ser. 92, 205–205. doi: 10.3354/meps092205
Clarke K. R., Tweedley J. R., Valesini F. J. (2014). Simple shade plots aid better long-term choices of data pre-treatment in multivariate assemblage studies. J. Mar. Biolog. Assoc. U.K. 94, 1–16. doi: 10.1017/S0025315413001227
Cohen M. C. L., Lara R. J., Szlafsztein C., Dittmar T. (2004). Mangrove inundation and nutrient dynamics from a GIS perspective. Wetl. Ecol. 12, 81–86. doi: 10.1023/B:WETL.0000021668.25445.41
Costa C. R., Costa M. F., Dantas D. V., Barletta M. (2018). Interannual and seasonal variations in estuarine water quality. Front. Mar. Sci. 5. doi: 10.3389/fmars.2018.00301
D’Alessandro M., Porporato E. M. D., Esposito V., Giacobbe S., Deidun A., Nasi F., et al. (2020). Common patterns of functional and biotic indices in response to multiple stressors in marine harbours ecosystems. Environ. Pollut. 259, 113959. doi: 10.1016/J.ENVPOL.2020.113959
Dash C., Jaiswal M. K., Pati P., Patel N. K., Singh A. K., Shah R. A. (2020). Fluvial response to late quaternary sea level changes along the Mahanadi delta, east coast of India. Quat. Int. 553, 60–72. doi: 10.1016/j.quaint.2020.07.033
Dash B., Rout S. S., Lovaraju A., Charan Kumar B., Bharati A., Ganesh T., et al. (2021). Macrobenthic community of a tropical Bay system revisited: Historical changes in response to anthropogenic forcing. Mar. Pollut. Bull. 171, 112775. doi: 10.1016/j.marpolbul.2021.112775
Dauvin J. C., Alizier S., Rolet C., Bakalem A., Bellan G., Gesteira J. L. G., et al. (2012). Response of different benthic indices to diverse human pressures. Ecol. Indic. 12, 143–153. doi: 10.1016/J.ECOLIND.2011.03.019
Dauvin J. C., Andrade H., De-La-Ossa-Carretero J. A., Del-Pilar-Ruso Y., Riera R. (2016). Polychaete/amphipod ratios: An approach to validating simple benthic indicators. Ecol. Indic. 63, 89–99. doi: 10.1016/j.ecolind.2015.11.055
Dauvin J. C., Fersi A., Pezy J. P., Bakalem A., Neifar L. (2021). Macrobenthic communities in the tidal channels around the Gulf of Gabès, Tunisia. Mar. Pollut. Bull. 162, 111846. doi: 10.1016/J.MARPOLBUL.2020.111846
Dey M., Chowdhury C., Pattnaik A. A., Ganguly D., Mukhopadhyay S. K., De T. K., et al. (2013). Comparison of Monsoonal change of water quality parameters between 1983 and 2008 in a tropical estuary in Northeastern India: Role of phytoplankton and community metabolism. Mar. Ecol. 34, 14–29. doi: 10.1111/j.1439-0485.2012.00519.x
Dias H. Q., Sukumaran S., Srinivas T., Mulik J. (2018). Ecological quality status evaluation of a Monsoonal tropical estuary using benthic indices: comparison via a seasonal approach. Environ. Sci. Pollut. Res. 25, 22672–22688. doi: 10.1007/s11356-018-2344-0
Durango-Cordero J., Satyanarayana B., Chan J. C. W., Bogaert J., Dahdouh-Guebas F. (2021). Distinguishing original and non-original stands at the Zhanjiang Mangrove National Nature Reserve (P.R. China): Remote sensing and GIS for conservation and ecological research. Remote Sens. 13, 2781. doi: 10.3390/rs13142781
Elliott M., Day J. W., Ramachandran R., Wolanski E., Fang Q., Sheehan M. R., et al. (2019). A synthesis: What is the future for coasts, estuaries, deltas and other transitional habitats in 2050 and beyond? Coasts Estuaries Futur. 2019, 1–28. doi: 10.1016/B978-0-12-814003-1.00001-0
Elliott M., Quintino V. (2007). The estuarine quality paradox, environmental homeostasis and the difficulty of detecting anthropogenic stress in naturally stressed areas. Mar. Pollut. Bull. 54, 640–645. doi: 10.1016/j.marpolbul.2007.02.003
Equbal J., Lakra R. K., Savurirajan M., Satyam K., Thiruchitrambalam G. (2018). Testing performances of marine benthic biotic indices under the strong seasonality in the tropical intertidal habitats, South Andaman, India. Mar. Pollut. Bull. 135, 266–282. doi: 10.1016/J.MARPOLBUL.2018.07.034
Equbal J., Thiruchitrambalam G., Lakra R. K., Savurirajan M., Satyam K. (2017). Assessing the ecological quality of the Port Blair coast (South Andaman, India) using different suites of benthic biotic indices. Mar. Biolog. Assoc. U.K. 97 (5), 1007–1021. doi: 10.1017/S0025315417000753
Fauchald K. (1977). The polychaete worms. definitions and keys to the orders, families and genera. Natural History Museum Los Angeles County Sci. Ser. 28, 1–188. http://hdl.handle.net/10088/3435
Fauvel P. (1953). “The fauna of India including Pakistan, Ceylon, Burma and Malaya,” in Annelida: Polychaeta (Delhi: The Indian Press), 507.
Feebarani J., Joydas T. V., Damodaran R., Borja A. (2016). Benthic quality assessment in a naturally- and human-stressed tropical estuary. Ecol. Indic. 67, 380–390. doi: 10.1016/j.ecolind.2016.03.005
Gaonkar C. V., Nasnodkar M. R., Matta V. M. (2021). Assessment of metal enrichment and contamination in surface sediment of Mandovi estuary, Goa, West coast of India. Environ. Sci. Pollut. Res. 28 (41), 57872–57887. doi: 10.1007/s11356-021-14610-1
Gaonkar U. V., Sivadas S. K., Ingole B. S. (2013). Effect of tropical rainfall in structuring the macrobenthic community of Mandovi estuary, west coast of India. J. Mar. Biolog. Assoc. U.K. 93 (7), 1727–1738. doi: 10.1017/S002531541300026X
Gaudette H. E., Flight W. R., Toner L., Folger D. W. (1974). An inexpensive titration method for the determination of organic carbon in recent sediments. J. Sediment. Res. 44, 249–253. doi: 10.1306/74D729D7-2B21-11D7-8648000102C1865D
Ghosh A., Woodward E. M. S., Nelson C. E., Saha R., Bhadury P. (2022). Nitrogen driven niche differentiation in bacterioplankton communities of northeastern coastal Bay of Bengal. Environ. Res. Commun. 4, 1–15. doi: 10.1088/2515-7620/ac5a69
Gopalakrishnan L., Satyanarayana B., Chen D., Wolswijk G., Amir A. A., Vandegehuchte M. B., et al. (2021). Using historical archives and landsat imagery to explore changes in the mangrove cover of Peninsular Malaysia between 1853 and 2018. Remote Sens. 13, 3403. doi: 10.3390/rs13173403
Grall J., Glémarec M. (1997). Using biotic indices to estimate macrobenthic community perturbations in the Bay of Brest. Estuar. Coast. Shelf Sci. 44, 43–53. doi: 10.1016/S0272-7714(97)80006-6
Grasshoff K., Kremling K., Ehrardt M. (1999). Methods of seawater analysis. 3rd ed. Vol. 407 (Weinhein: VerlagChemie).
Habib S., Hossain M. B., Hossain M. S., Jolly Y. N., Sarker S. (2021). Ecological risk evaluation in bottom-surface sediments and sub-surface water in the subtropical Meghna estuarine system. Heliyon 7, e08324. doi: 10.1016/J.HELIYON.2021.E08324
Haddout S., Priya K. L., Casila J. C. C., Samad B. (2022). Assessment of heavy metal contamination in the surficial sediments from the Sebou river estuary, Morocco, environ. Forensics 23:1-2, 23–31. doi: 10.1080/15275922.2021.1887970
Hazra S., Das S., Ghosh A., Raju P. V., Patel A. (2020). The Mahanadi delta: A rapidly developing delta in India. In Deltas Anthropocene Palgrave Macmillan Cham, 53–77. doi: 10.1007/978-3-030-23517-8_3
Hossain M. S., Chowdhury S. R., Navera U. K., Hossain M. A. R., Imam B., Sharifuzzaman S. M. (2014). Opportunities and strategies for ocean and river resources management. Dhaka: Background paper for preparation of the 7th five year plan. Plann. Commission Ministry Planning Bangladesh. 1–66.
Hutchings P., Kupriyanova E. (2018). Cosmopolitan polychaetes–fact or fiction? personal and historical perspectives. Invertebr. Syst. 32, 1–9. doi: 10.1071/IS17035
I-WRIS (2021) Water resources information system of India. Available at: https://indiawris.gov.in/wris/#/Basin (Accessed 16 January2022, 021).
Jabir T., Vipindas P. V., Jesmi Y., Divya P. S., Adarsh B. M., Miziriya H. N., et al. (2021). Influence of environmental factors on benthic nitrogen fixation and role of sulfur reducing diazotrophs in a eutrophic tropical estuary. Mar. Pollut. Bull. 165, 112126. doi: 10.1016/j.marpolbul.2021.112126
John S. E., Rajimol T. R., Mohan S V., Maya K., Padmalal D. (2017). Environmental degradation of a tropical estuary due to human interferences-a case study from Southern Kerala, SW India. Arab. J. Geosci. 10, 1–15. doi: 10.1007/s12517-017-3112-z
Kanhai A. (2022). Spatial and seasonal variation in polychaete communities according to their trophic categories in southwest Tobago, West Indies. Neotrop. Biodivers. 8 (1), 8–20. doi: 10.1080/23766808.2021.2021051
Karakassis I., Dimitriou P. D., Papageorgiou N., Apostolaki E. T., Lampadariou N., Black K. D. (2013). Methodological considerations on the coastal and transitional benthic indicators proposed for the water framework directive. Ecol. Indic. 29, 26–33. doi: 10.1016/J.ECOLIND.2012.12.020
Karydis M., Kitsiou D. (2013). Marine water quality monitoring: A review. Mar. Pollut. Bull. 77, 23–36. doi: 10.1016/J.MARPOLBUL.2013.09.012
Kennish M. J. (2002). Environmental threats and environmental future of estuaries. Environ. Conserve. 29, 78–107. doi: 10.1017/S0376892902000061
Kennish M. J. (2005). “Estuaries, anthropogenic impacts,” in Encyclopedia of earth sciences series (Netherlands: Springer), 434–436). doi: 10.1007/978-3-319-48657-4_140-2
Khan S. A., Manokaran S., Lyla P. S. (2014). Assessment of ecological quality of Vellar and Uppanar estuaries, southeast coast of India, using benthos. Indian J. Geo-Marine Sci. 43, 1989–1995.
Kröncke I., Reiss H. (2010). Influence of macrofauna long-term natural variability on benthic indices used in ecological quality assessment. Mar. Pollut. Bull. 60, 58–68. doi: 10.1016/J.MARPOLBUL.2009.09.001
Krumbein W. C., Pettijohn F. J. (1938). Manual of sedimentary petrography (New York: Appleton Century Crofts Inc.), 549.
Kumar M. R., Krishnan K. A., Vimexen V., Faisal A. K., Mohind M., Arun V. (2022). Heavy metal impression in surface sediments and factors governing the fate of macrobenthic communities in tropical estuarine ecosystem, India. Environ. Sci. Pollut. Res. 29, 38567–38590. doi: 10.1007/s11356-021-18394-2
Kumar C., Ghosh A., Yash Debnath M., Bhadury P. (2021). Seasonal dynamicity of environmental variables and water quality index in the lower stretch of the River Ganga. Environ. Res. Commun. doi: 10.1088/2515-7620/ac10fd
Kundu S., Mondal N., Lyla P. S., Ajmal Khan S. (2010). Biodiversity and seasonal variation of macro-benthic infaunal community in the inshore waters of Parangipettai coast. Environ. Monit. Assess. 163 (1), 67–79. doi: 10.1007/s10661-009-0817-9
Leal Filho W., Nagy G. J., Martinho F., Saroar M., Erache M. G., Primo A. L., et al. (2022). Influences of Climate Change and Variability on Estuarine Ecosystems: An Impact Study in Selected European, South American and Asian Countries. Int. J. Environ. Res. Public Health 19 (1), 585. doi: 10.3390/ijerph19010585
Lotze H. K., Lenihan H. S., Bourque B. J., Bradbury R. H., Cooke R. G., Kay M. C., et al. (2006). Depletion, degradation, and recovery potential of estuaries and coastal seas. Science 312, 1806–1809. doi: 10.1126/science.1128035
Mitchell S. (2020). Sediment transport and Marine Protected Areas. Marine Protected Areas, 587–598. doi: 10.1016/B978-0-08-102698-4.00030-7
Mitra S., Ghosh S., Satpathy K. K., Bhattacharya B. D., Sarkar S. K., Mishra P., et al. (2018). Water quality assessment of the ecologically stressed Hooghly river estuary, India: A multivariate approach. Mar. Pollut. Bull. 126, 592–599. doi: 10.1016/j.marpolbul.2017.09.053
Mohanti M., Swain M. R. (2005). “Mahanadi river delta, east coast of India: an overview on evolution and dynamic processes,” in Department of Geology (Vani Vihar: Utkal University).
Mohanty B. (2018). Taxonomy and Ecology of Brachyuran Crabs of Devi Estuary and Associated Mangrove Environment, Astarang, East Coast of India (Doctoral dissertation, Ravenshaw University).
Mohanty B., Nayak A., Dash B., Rout S. S., Charan Kumar B., Patnaik L., et al. (2019). Biodiversity and ecological considerations of brachyuran crabs (Crustacea: Decapoda) from Devi estuary–mangrove region on the east coast of India. Reg. Stud. Mar. Sci. 32, 100865. doi: 10.1016/j.rsma.2019.100865
Mohanty B., Raut D., Dev Roy M. K., Patnaik L., Rout S. S., Dash B., et al. (2022). Brachyuran Crabs of Devi estuary: Taxonomic perspective (India: Odisha Biodiversity Board), 120.
Muir A. I., Hossain M. M. M. (2014). The intertidal polychaete (Annelida) fauna of the Sitakunda coast (Chittagong, Bangladesh), with notes on the Capitellidae, Glyceridae, Lumbrineridae, Nephtyidae, Nereididae and Phyllodocidae of the “Northern Bay of Bengal ecoregion”. ZooKeys 1, 1–419. doi: 10.3897/zookeys.419.7557
Mulik J., Sukumaran S., Dias H. Q. (2020a). Can the ecological status of three differentially impacted Monsoonal tropical estuaries in NW India, be adequately assessed by a common estuarine benthic index? Ecol. Indic. 119, 106807. doi: 10.1016/J.ECOLIND.2020.106807
Mulik J., Sukumaran S., Dias H. Q. (2020b). Is the benthic index AMBI impervious to seasonality and data transformations while evaluating the ecological status of an anthropized Monsoonal estuary? Ocean Coast. Manage. 186, 105080. doi: 10.1016/J.OCECOAMAN.2019.105080
Mulik J., Sukumaran S., Srinivas T., Vijapure T. (2017). Comparative efficacy of benthic biotic indices in assessing the ecological quality status (EcoQS) of the stressed Ulhas estuary, India. Mar. Pollut. Bull. 120, 192–202. doi: 10.1016/J.MARPOLBUL.2017.05.014
Muxika I., Borja Á., Bald J. (2007). Using historical data, expert judgement and multivariate analysis in assessing reference conditions and benthic ecological status, according to the European water framework directive. Mar. Pollut. Bull. 55, 16–29. doi: 10.1016/J.MARPOLBUL.2006.05.025
Nayak A. (2020). Benthic macrofauna of Mahanadi estuary Odisha, East coast of India, doctoral thesis submitted to Ravenshaw university. 406. Available at: http://hdl.handle.net/10603/355684.
Nayak A., Charan Kumar B., Lovaraju A., Raut D., Sanghamitra Rout S., Dash B., et al. (2018). Benthic infauna from mudflats of Atharbanki mangrove waterway in Odisha, India. J. Mar. Biol. Assoc. India. 60, 33–39. doi: 10.6024/jmbai.2018.60.1.2026-05
Ortega I., Colling L. A., Dumont L. F. C. (2018). Response of soft-bottom macrobenthic assemblages to artisanal trawling fisheries in a subtropical estuary. estuar. Coast. Shelf Sci. 207, 142–153. doi: 10.1016/J.ECSS.2018.04.007
Palei N. C., Rath B. P., Pradhan S. D., Swain K. K., Pati M. (2014). The water birds of Paradeep Phosphate limited (PPL) campus of Jagatsinghpur District in Odisha, India. World J. Zool. 9 (3), 208–213. doi: 10.5829/idosi.wjz.2014.9.3.8552
Pandey V., Venkatnarayanan S., Kumar P. S., Ratnam K., Jha D. K., Rajaguru S., et al. (2021). Assessment of ecological health of Swarnamukhi river estuary, southeast coast of India, through AMBI indices and multivariate tools. Mar. Pollut. Bull. 164, 112031. doi: 10.1016/J.MARPOLBUL.2021.112031
Pattanayak J. G., Haldar B. P. (1998). Other groups. In Fauna of Mahanadi estuary, Orissa, Estuarine Ecosystem Series, Rec. Zool. Surv. India 3, 215–218.
Pinto R., Patrício J., Baeta A., Fath B. D., Neto J. M., Marques J. C. (2009). Review and evaluation of estuarine biotic indices to assess benthic condition. Ecol. Indic. 9, 1–25. doi: 10.1016/J.ECOLIND.2008.01.005
Prasad M., Ramanathan A. L. (2008). Dissolved organic nutrients in the Pichavaram mangrove waters of east coast of India. Indian J. Geo-Marine Sci. 37, 141–145.
Rao C. A. N. (1998). Polychaete, in Fauna of Mahanadi estuary, Orissa. Estuar. Ecosystem Series Rec. Zool. Surv. India. 3, 199–209.
Raut D., Ganesh T., Murty N. V. S. S., Raman A. V. (2005). Macrobenthos of Kakinada bay in the Godavari delta, East coast of India: Comparing decadal changes. Estuar. Coast. Shelf Sci. 62, 609–620. doi: 10.1016/j.ecss.2004.09.029
Rehitha T. V., Madhu N. V., Vineetha G., Vipindas P. V., Resmi P., Revichandran C. (2019). Spatio-temporal variability in macrobenthic communities and trophic structure of a tropical estuary and its adjacent coastal waters. Environ. Monit. Assess. 191 (6), 1–18. doi: 10.1007/s10661-019-7460-x
Rehitha T. V., Vineetha G., Madhu N. V. (2022). Ecological habitat quality assessment of a tropical estuary using macrobenthic functional characteristics and biotic indices. Environ. Sci. Pollut. Res. 29, 1–18. doi: 10.1007/s11356-022-19295-8
Rodgers K., McLellan I., Peshkur T., et al. (2020). The legacy of industrial pollution in estuarine sediments: Spatial and temporal variability implications for ecosystem stress. Environ Geochem Health 42, 1057–1068. doi: 10.1007/s10653-019-00316-4
Romañach S. S., DeAngelis D. L., Koh H. L., Li Y., Teh S. Y., Raja Barizan R. S., et al. (2018). Conservation and restoration of mangroves: Global status, perspectives, and prognosis. Ocean Coast. Manage. 154, 72–82. doi: 10.1016/J.OCECOAMAN.2018.01.009
Salimi E., Sakhaei N., Nurinezhad M., Savari A., Ghaemmaghami S. S. (2021). Composition, biomass and secondary production of the macrobenthic invertebrate assemblage in a mangrove forest in Nayband Bay, Persian Gulf. Reg. Stud. Mar. Sci. 42, 101636. doi: 10.1016/J.RSMA.2021.101636
Sánchez-Ovando J. P., Benítez-Villalobos F., Bastida-Zavala J. R. (2021). Early development of two species of Spirobranchus Blainville, 1818 (Polychaeta: Serpulidae) from the Southern Mexican Pacific. Invertebr. Reprod. Dev. 65, 188–200. doi: 10.1080/07924259.2021.1932614
Sanchis C., Soto E. H., Quiroga E. (2021). The importance of a functional approach on benthic communities for aquaculture environmental assessment: Trophic groups – a polychaete view. Mar. Pollut. Bull. 167, 112309. doi: 10.1016/J.MARPOLBUL.2021.112309
Sandeep K. K., Pant V. (2019). Riverine freshwater plume variability in the Bay of Bengal using wind sensitivity experiments. Deep Sea Res. Part II Top. Stud. Oceanogr. 168, 104649. doi: 10.1016/J.DSR2.2019.104649
Sany T. S. B., Hashim R., Tajfard M., Rahman M. A. (2018a). Assessing the ecological health status using macrobenthic communities of tropical coastal water. Hum. Ecol. Risk Assess. (HERA) 24, 1761–1785. doi: 10.1080/10807039.2017.1422974
Sany T. S. B., Tajfard M., Hashim R., Rezayi M., Rahman M. A., Karlen D. J. (2018b). The response of macrobenthic communities to environmental variability in tropical coastal waters. Estuaries Coast. 41, 1178–1192. doi: 10.1007/s12237-017-0346-7
Sarathy P. P., Bharathidasan V., Murugesan P., Selvaraj P., Punniyamoorthy R. (2022). Seasonal carbonate system vis-à-vis pH and salinity in selected tropical estuaries: Implications on polychaete diversity and composition towards predicting ecological health. Oceanologia 64, 346–362. doi: 10.1016/J.OCEANO.2022.01.001
Sharma D., Rao K., Ramanathan A. L. (2022). A systematic review on the impact of urbanization and industrialization on Indian coastal mangrove ecosystem. Coast. Ecosyst. 38, 175–199. doi: 10.1007/978-3-030-84255-0_8
Shepard F. P. (1954). Nomenclature based on sand-silt-clay ratios. J. sediment. Res. 24, 151–158. doi: 10.1306/D4269774-2B26-11D7-8648000102C1865D
Shirodkar S. R., Nayak V. N. (2010). Distribution and seasonal abundance of macro benthos of Gangavali estuary, Uttar Kannada, West coast of India. J. Ecobiotechnol. 2 (5), 80–84. Available at: https://updatepublishing.com/journal/index.php/jebt/article/view/41.
Shivarudrappa S. K., Rakocinski C. F., Briggs K. B. (2019). Vertical distribution of macrobenthos in hypoxia-affected sediments of the northern Gulf of Mexico: Applying functional metrics. Estuaries Coast. 42, 250–263. doi: 10.1007/s12237-018-0446-z
Sigamani S., Perumal M., Arumugam S., Preetha Mini Jose H. M., Veeraiyan B. (2015). AMBI indices and multivariate approach to assess the ecological health of Vellar–Coleroon estuarine system undergoing various human activities. Mar. Pollut. Bull. 100, 334–343. doi: 10.1016/J.MARPOLBUL.2015.08.028
Sivadas S. K., Gupta G. V. M., Kumar S., Ingole B. S. (2021). Trait-based and taxonomic macrofauna community patterns in the upwelling ecosystem of the southeastern Arabian Sea. Mar. Environ. Res. 170, 105431. doi: 10.1016/J.MARENVRES.2021.105431
Sivadas S. K., Ingole B. S. (2016). Biodiversity and biogeography pattern of benthic communities in the coastal basins of India. Mar. Biol. Res. 12 (8), 797–816. doi: 10.1080/17451000.2016.1203949
Sivadas S., Ingole B., Nanajkar M. (2011). Temporal variability of macrofauna from a disturbed habitat in Zuari estuary, west coast of India. Environ. Monit. Assess. 173 (1), 65–78. doi: 10.1007/s10661-010-1371-1
Sivadas S. K., Nagesh R., Gupta G. V. M., Gaonkar U., Mukherjee I., Ramteke D., et al. (2016). Testing the efficiency of temperate benthic biotic indices in assessing the ecological status of a tropical ecosystem. Mar. Pollut. Bull. 106 (1-2), 62–76. doi: 10.1016/j.marpolbul.2016.03.026
State Pollution Control Board, Odisha (2020). Health card of Paradeep coastal stretch. State Pollution Control Board, Odisha, Coastal Management Cell, Integrated Coastal Zone Management Programme. Odisha, State Pollution Control Board
Subba Rao N. V., Surya Rao K. V., Maitra S. (1991). Marine molluscs. In Fauna of Orissa, esturine ecosystem series, rec. Zool. Surv. India. 1, 1–175.
Subramanian R., Retnamma J., Nagarathinam A., Loganathan J., Singaram P., Chandrababu V. (2021). Seasonality of macrobenthic assemblages and the biotic environmental quality of the largest Monsoonal estuary along the west coast of India. Environ. Sci. Pollut. Res. 28 (28), 37262–37278. doi: 10.1007/s11356-021-13144-w
Sundaray S. K., Nayak B. B., Bhatta D. (2009). Environmental studies on river water quality with reference to suitability for agricultural purposes: Mahanadi river estuarine system, India–a case study. Environ. Monit. Assess. 155 (1), 227–243. doi: 10.1007/s10661-008-0431-2
Surya Rao K. V., Maitra S. (1998). Mollusca In Fauna of Mahanadi estuary Orissa, estuarine ecosystem series, Rec. Zool. Surv. India 3, 161–197.
Suzzi A. L., Gaston T. F., McKenzie L., Mazumder D., Huggett M. J. (2022). Tracking the impacts of nutrient inputs on estuary ecosystem function. Sci. Total Environ. 811, 152405. doi: 10.1016/j.scitotenv.2021.152405
Taillardat P., Marchand C., Friess D. A., Widory D., David F., Ohte N., et al. (2020). Respective contribution of urban wastewater and mangroves on nutrient dynamics in a tropical estuary during the monsoon season. Mar. Pollut. Bull. 160, 111652. doi: 10.1016/j.marpolbul.2020.111652
Thadathil P., Gopalakrishna V. V., Muraleedharan P. M., Reddy G. V., Araligidad N., Shenoy S. (2002). Surface layer temperature inversion in the Bay of Bengal. Deep Sea Res. Part I Oceanogr. Res. 49, 1801–1818. doi: 10.1016/S0967-0637(02)00044-4
Tudu P. C., Yennawar P., Ghorai N., Tripathy B., Mohapatra A. (2018). An updated checklist of marine and estuarine mollusc of Odisha coast. Indian J. Geo-Marine Sci. 47, 1537–1560.
Varkey M. J., Murty V. S. N., Suryanarayana A. (1996). Physical oceanography of the Bay of Bengal and Andaman Sea. Oceanogr. Mar. Biol. 5, 413. Available at: https://updatepublishing.com/journal/index.php/jebt/article/view/41.
Veiga P., Torres A. C., Aneiros F., Sousa-Pinto I., Troncoso J. S., Rubal M. (2016). Consistent patterns of variation in macrobenthic assemblages and environmental variables over multiple spatial scales using taxonomic and functional approaches. Mar. Environ. Res. 120, 191–201. doi: 10.1016/j.marenvres.2016.08.011
Wetz M. S., Yoskowitz D. W. (2013). An “extreme” future for estuaries? effects of extreme climatic events on estuarine water quality and ecology. Mar. Pollut. Bull. 69, 7–18. doi: 10.1016/j.marpolbul.2013.01.020
Wolswijk G., Satyanarayana B., Siau Y. F., Ali A. N. B., Saliu I. S., Fisol M. A. B., et al. (2020). Distribution of mercury in sediments, plant and animal tissues in Matang Mangrove Forest Reserve, Malaysia. J. Hazard. Mater. 387, 121665. doi: 10.1016/j.jhazmat.2019.121665
Wolswijk G., Trullols A. B., Hugé J., Otero V., Satyanarayana B., Lucas R., et al. (2022). Can mangrove silviculture be carbon neutral? Remote Sens. 14, 2920. doi: 10.3390/rs14122920
Yokoyama H., Sukumaran S. (2012). First records of three Paraprionospio species (Polychaeta: Spionidae) from Indian waters. Cah. Biol. Mar. 53, 279–287. Available at: http://drs.nio.org/drs/handle/2264/4047.
Keywords: macrobenthos, pollution monitoring, Mahanadi estuary, Odisha, Bay of Bengal, AMBI, M-AMBI
Citation: Nayak A, Equbal J, Rout SS, Dash B, Thiruchitrambalam G, Bhadury P, Satyanarayana B and Raut D (2022) Macrobenthic community of an anthropogenically influenced mangrove associated estuary on the East coast of India: An approach for ecological assessment. Front. Mar. Sci. 9:1008912. doi: 10.3389/fmars.2022.1008912
Received: 01 August 2022; Accepted: 20 September 2022;
Published: 07 October 2022.
Edited by:
Meilin Wu, South China Sea Institute of Oceanology (CAS), ChinaReviewed by:
Thilagam Harikrishnan, Pachaiyappa’s College for Men, IndiaCopyright © 2022 Nayak, Equbal, Rout, Dash, Thiruchitrambalam, Bhadury, Satyanarayana and Raut. This is an open-access article distributed under the terms of the Creative Commons Attribution License (CC BY). The use, distribution or reproduction in other forums is permitted, provided the original author(s) and the copyright owner(s) are credited and that the original publication in this journal is cited, in accordance with accepted academic practice. No use, distribution or reproduction is permitted which does not comply with these terms.
*Correspondence: Behara Satyanarayana, c2F0eWFtMjE0OUBnbWFpbC5jb20=; Dipti Raut, cmF1dC5kaXB0aTJAZ21haWwuY29t
Disclaimer: All claims expressed in this article are solely those of the authors and do not necessarily represent those of their affiliated organizations, or those of the publisher, the editors and the reviewers. Any product that may be evaluated in this article or claim that may be made by its manufacturer is not guaranteed or endorsed by the publisher.
Research integrity at Frontiers
Learn more about the work of our research integrity team to safeguard the quality of each article we publish.