- Simon F.S. Li Marine Science Laboratory, School of Life Sciences, The Chinese University of Hong Kong, Hong Kong, Hong Kong SAR, China
Previous studies on mangrove carbon utilisation and mineralisation were focused on mangrove sesarmid crabs (Grapsoidea: Sesarmidae) because they are initial processors of mangrove organic carbon. Grapsoid crabs from other families and habitats were generally ignored although they also perform various levels of herbivory. It remains unclear how the herbivorous crabs utilise the production from mangrove or vascular plants because of the high C/N ratio and high structural carbon content of these materials. In this study, production of cellulase and expression of hepatopancreatic glycosyl hydrolase of grapsoid crabs were investigated in a more extensive way in terms of their taxa (15 species) and habitats (i.e., land margin, mangrove, mudflat, and subtidal area). Generally, low gastric endoglucanase (1.46-2.98 μmol min−1 ml−1) and β-glucosidase (0.06-0.61 μmol min−1 ml−1) activity was detected in 15 grapsoid crabs distributed along the land-sea transition. The activity of both enzymes was significantly affected by the taxonomic affiliation and habitat of the crabs: endoglucanase in sesarmids > varunids > grapsids; and land margin, mangrove and limnic habitats > mudflat and subtidal area. For β-glucosidase: sesarmids > varunids and grapsids; and land and limnic > mangrove and subtidal > mudflat. Our detection of gastric cellulases in mudflat and subtidal grapsoid crabs has rarely been reported. These results show that there is a need to re-evaluate the role of crabs from these habitats in mangrove/vascular plant carbon mineralisation. Further, low cellulase activity could be a common trait among crabs along the land-sea transition and the importance of cellulase in vascular plant carbon utilisation could have been previously over-rated. Glycosyl hydrolase family 9 (GH9) genes were identified in the hepatopancreatic transcriptome of all 15 species, indicating endogenous production of cellulase. In addition, the presence of other digestive carbohydrate-active enzymes (CAZymes), including GH5_10, GH13, GH13_24, and GH16, suggests that a capacity for the utilisation of carbon sources other than cellulose by the grapsoid crabs, which may enable sufficient assimilation of vascular plant carbon despite the low cellulase activity.
Introduction
The land-sea transition on protected shores in the tropics comprises a series of habitats, namely, the land margin, mangrove forest, mudflat and subtidal area, in which the mangrove forests, with their global NPP estimated at ∼218 ± 72 Tg C y−1 (Bouillon et al., 2008), act as one of the major producers that provide large amounts of carbon. Despite the high productivity, mangrove organic production is not highly palatable because of the high C/N ratio, high content of secondary metabolites (e.g., tannins) and high cellulose content, which make mangrove biomass unattractive to animals (Mazumder and Saintilan, 2010). However, there is robust evidence of brachyuran crabs utilising mangrove leaf litter or fresh leaves (Leh and Sasekumar, 1985; Micheli, 1993; Linton et al., 2006; Linton and Greenaway, 2007; Harada and Lee, 2016) as their major food sources. In the Indo-west-Pacific, sesarmid crabs (Grapsoidea: Sesarmidae) are key initial processors of mangrove leaf litter. By shredding or burying leaves (Lee, 1989a; Robertson and Daniel, 1989; Camilleri, 1992; Emmerson and McGwynne, 1992; Skov and Hartnoll, 2002; Mfilinge and Tsuchiya, 2008; Harada and Lee, 2016), they exert great impact on mangrove production and nutrient dynamics, with some species removing large proportions of mangrove leaf litter production (Robertson and Daniel, 1989; Emmerson and McGwynne, 1992; Harada and Lee, 2016). These crabs such as Parasesarma bidens connect mangrove and higher trophic levels by acting as prey to predators such as fish, thus linking mangrove and coastal food chains (Sheaves and Molony, 2000; Kawaida et al., 2021). Alternatively, they transform mangrove production into faecal material with improved nutritional value that may be readily assimilated by deposit-feeders such as fiddler crabs or suspension-feeders such as copepods (Lee, 1997; Werry and Lee, 2005).
Cellulose (polymer of glucose joined by β-1,4-glycosidic bonds), a structural compound in higher plants, is one of the major forms of carbon in fresh mangrove leaves and leaf litter. To cleave and breakdown cellulose into a usable energy source (glucose), endo-β-1,4-glucanase (EC 3.2.1.4), exo-1,4-β-glucanase (EC 3.2.1.91 and 3.2.1.74, exocellulase), and β-glucosidase (EC 3.2.1.21) enzymes are needed. Endo-β-1,4-glucanase hydrolyses the β-1,4-glycosidic bonds to produce oligomers with random lengths. Exo-1,4-β-glucanase cleaves cellobiose or glucose from the end of cellulose polymer. β-glucosidase cleaves glucose from the non-reducing end of cellobiose or oligomers (Watanabe and Tokuda, 2010). It was believed that herbivorous animals merely depend on microorganisms, mostly as endosymbionts, to digest cellulose before the discovery of cellulase genes in termites (Bärlocher et al., 1989; Watanabe et al., 1998). Recent works suggest that various invertebrates such as molluscs (e.g., Magallana rivularis, Pirenella cingulata, and Batillaria zonalis) and crustaceans (e.g., Parasesarma erythodactylum, Episesarma versicolor, and Gecarcoidea natalis) can produce endogenous cellulases (Adachi et al., 2012; An et al., 2014; An et al., 2015; Bui and Lee, 2015; Adachi et al., 2016).
Cellulases are carbohydrate-active enzymes (CAZymes) that belong to various glycosyl hydrolase (GH) families. There are cellulases from different GH families (Lombard et al., 2014). For example, GH7 is exclusively found in fungi while GH9, one of the most ubiquitous and well-studied cellulase GH, can be found in organisms across the entire Tree of Life. Endogenous cellulase production in grapsoid crabs was demonstrated by transcriptomic analysis (Bui and Lee, 2015; Gan et al., 2018; Gray et al., 2018). On the other hand, there are reports of terrestrial and mangrove crustaceans producing hemicellulases such as GH5_10 endo-β-1,4-mannase and xylanase, and amylase (Pavasovic et al., 2004; An et al., 2014; Linton, 2021) that break down hemicellulose and starch, in which hemicellulose can constitute up to 30% of the dicotyledonous plant cell wall (Cosgrove, 2005; Cosgrove, 2014).
Previous studies on mangrove mineralisation by macrofauna mostly focused on predominantly herbivorous mangrove sesarmids (Lee, 1989b; Robertson and Daniel, 1989; Emmerson and McGwynne, 1992; Ashton, 2002; Bui and Lee, 2015; Harada and Lee, 2016), and there is generally limited information on species that inhabit environments adjacent to mangroves such as mudflat which also receive mangroves organic matter (Fratini et al., 2000; Poon et al., 2010; Nordhaus et al., 2011; Devi et al., 2013). The presence of vascular plant and mangrove materials in the stomach of mudflat organisms shows that they are potential processors of mangrove production that facilitate mangrove carbon mineralisation (Poon et al., 2010). To re-evaluate the mangrove mineralisation capability of animals from various microhabitats of the coastline in the light of recent findings on CAZyme production, we assessed the presence of two cellulolytic enzymes, endoglucanase (EG) and β-glucosidase (BG), the optimal pH and temperature of EG, GH gene expression in the hepatopancreas, and phylogenetic analysis of the GH genes of 15 grapsoid crabs, from the superfamily of brachyurans that are widely distributed along the tropical land-sea transition.
Materials and methods
Collection and maintenance of crabs
The male individuals of 15 target species and the sites that they were collected from are shown in Table 1. Most species were collected from Ting Kok (22°28’12.5”N, 114°12’44.1”E), while individuals of Parasesarma affine were collected from Mai Po Nature Reserve (22°29’41.6”N, 114°01’54.2”E) and Episesarma versicolor from Wu Shek Kok (22°32’04.5”N, 114°12’40.6”E), Hong Kong (Figure 1). Most of the crabs distribute strictly as described in Table 1, except that mudflat species, Metaplax longipes and Metopograpsus frontalis also spend some time at lower margin of mangrove forest. All samples of these two species were collected in mudflat area. Crabs collected were maintained individually in cylindrical growth compartments (diameter 75mm x height 85 mm) containing ~30 ml of seawater, freshwater or brackish water, as appropriate, to prevent dehydration.
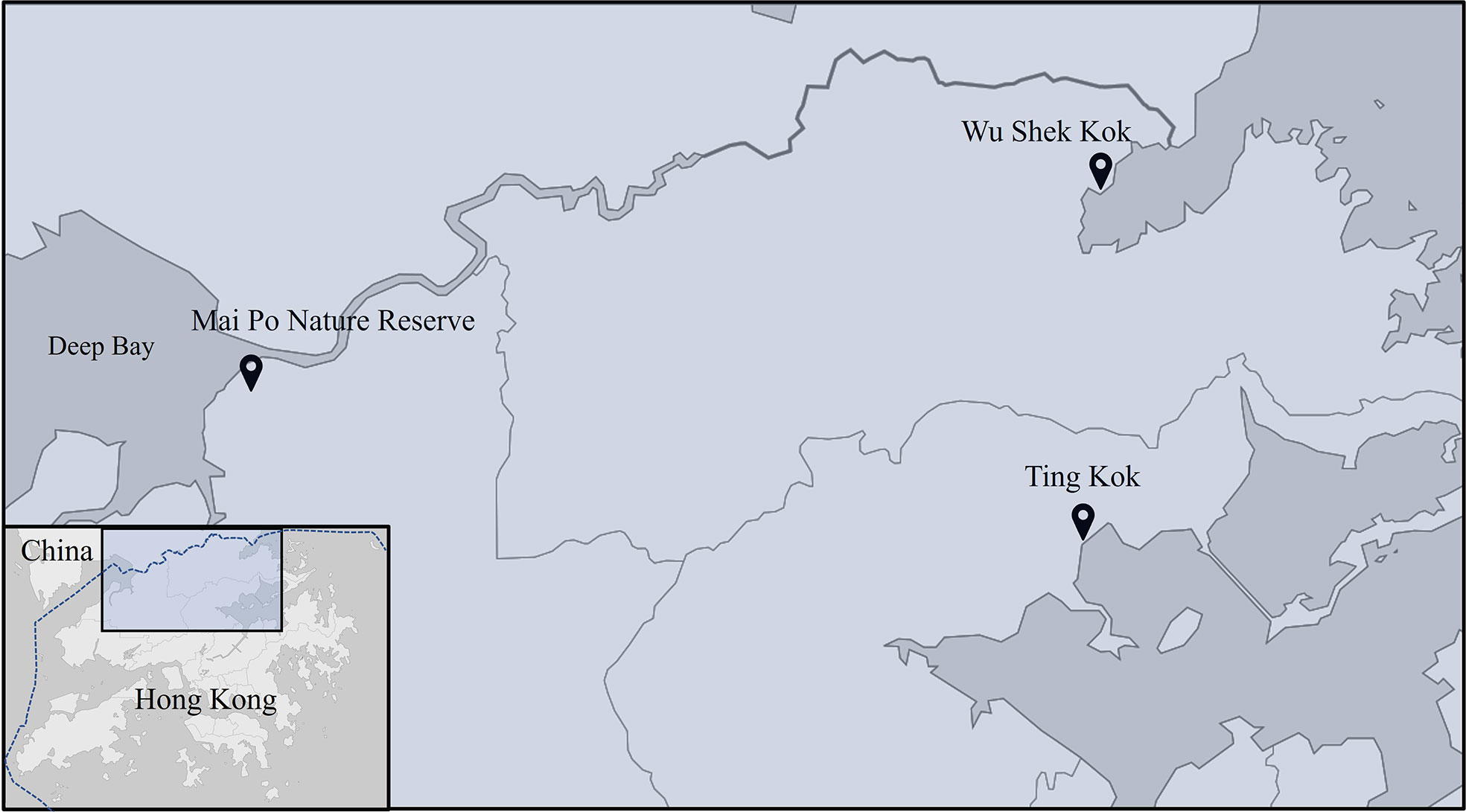
Figure 1 Map of field sites. Most crab species were collected in Ting Kok (Table 1), while Parasesarma affine and Episesarma versicolor were collected from Mai Po Nature Reserve and Wu Shek Kok, respectively, because of their high abundance at corresponding sites.
Preparation of crude enzymes
To measure the “field” stomach juice cellulase activities, gastric juice was sampled from the crabs immediately upon arrival to laboratory for the preparation of crude enzyme following the methods of Bui and Lee (2015) with some modifications. Crabs were rinsed with MilliQ water and euthanized in ice for one to 10 minutes depending on their body sizes. Gastric juice was extracted from the freshly euthanized crabs by using a syringe connected to a blunt-ended 16 to 21-gauge needle. The juice was centrifuged (13,000 g, 4°C, 10 mins). The supernatant was used as crude enzyme and stored at -20°C until use. About 70 μl was needed for biochemical characterization of cellulases.
Biochemical characterization of cellulases in the gastric juice
The activities of endo-β-1,4-glucanase and β-glucosidase of the gastric juice were determined by biochemical assay following methods of Tokuda et al. (1997) and Bui and Lee (2015) with some modifications.
Activity of endo-β-1,4-glucanase and β-glucosidase
Endo-β-1,4-glucanase (EG) activity was determined as the rate of production of reducing sugars from carboxymethyl cellulose (CMC). For each individual, five μl of gastric juice was added to 45 μl 2% CMC (Sigma) and incubated for 10 min at 37°C with 300-rpm agitation. The mixture was then transferred to ice slurry to terminate the reaction before the measurement of reducing sugars produced via the tetrazolium blue method (Jue and Lipke, 1985). β-glucosidase (BG) activity was determined as the rate of production of glucose form cellobiose (Sigma). Five μl of gastric juice was added to 45 μl of 2% cellobiose and incubated for 20 min at 37°C with 300-rpm agitation. The mixture was then transferred to ice slurry to terminate the reaction before the measurement of glucose produced using a Megazyme D-Glucose HK Assay Kit following manufacturer’s instructions.
Ten male individuals were sampled for each target species and all assays were conducted in triplicates. The amount of reducing sugar and glucose produced was corrected with the amounts of sugars in the digestive juices. One unit of EG/BG activity is defined as the amount of enzyme producing 1 μmol of reducing sugars/glucose per minute per ml of digestive juice under given assay condition. Activity was not expressed in terms of per mg of protein since the EG/GB comprises only a fraction of a mixture of various digestive enzymes in the gastric juice (Linton and Greenaway, 2004).
pH and temperature optima of endoglucanase activity
The activity of endo-β-1,4-glucanase was determined over a temperature range of 20 to 60°C and pH range of 4 to 8 based on previous reports about the optimal temperature and pH lying between the two ranges respectively. For temperature profiling, the activity was measured as mentioned above by incubating at different temperatures (20, 30, 37, 50, 60°C) using heat block. For pH profiling, 2% CMC was prepared by dissolving CMC in pH 4, 5.0, 5.5 and 6.0 0.1M citrate buffer and pH 7.0 and 8.0 Tris buffer, and the reactions were incubated at 37°C using heat block. The temperature and pH at which EG showed the highest activity among the tested temperatures and pH is determined as the optima. Three male individuals of each target species were tested for this experiment.
Data analysis
The endoglucanase activity of different species at different temperatures and pH values was compared by one-way analysis of variance (ANOVA). Levene’s test and Shapiro-Wilk’s test were used to test homogeneity of variance and normality of data set, respectively, before ANOVA. Kruskal-Wallis H test was used if the assumptions of ANOVA were not met. Significant results (P < 0.05) of ANOVA and Kruskal-Wallis H tests indicating statistical difference between treatments (pH and temperature) were further compared by applying Tukey’s Honestly Significant Difference (HSD) post-hoc-tests. Two-way ANOVA was used to analyse the effect of habitats and taxa on enzymatic activity. HSD post-hoc-test was used to determine statistical differences among species when a significant ANOVA result occurred.
Total RNA extraction and transcriptome sequencing
One male individual each of 14 grapsoid species were collected from Ting Kok and Mai Po, except Parasesarma pictum, as data on this species is available on the NCBI database (Accession: PRJNA524231). Crabs were dissected immediately after arriving at the laboratory. Total RNA was isolated from ~30 mg of hepatopancreas tissue using TRIzol reagent (Invitrogen), following the manufacturer’s instructions. The quality of the RNA was determined through the Nanodrop spectrophotometer and gel electrophoresis before sequencing. Library construction and sequencing (Illumina PE150, Q30 ≥ 80%) of qualifying samples were done at Novogene. The transcriptome raw data generated in this study is available on NCBI database (Accession: PRJNA866679).
The raw reads were cleaned and trimmed using Trimmomatic (Bolger et al., 2014). De novo assembly of the reads was carried out using Trinity software version 2.8.4 (Grabherr et al., 2011) with default parameters. Bacterial and viral sequences were removed using Kraken under default parameters (Wood and Salzberg, 2014). CD-HIT program was used to further remove redundancy in the final assembly (Li and Godzik, 2006; Fu et al., 2012). BUSCO was used to check the quality of the transcripts (Simão et al., 2015).
CAZyme gene identification and phylogenetic analysis
To identify GH genes, transcripts were searched against CAZy (CAZyDB.07312019 obtained from http://bcb.unl.edu/dbCAN2/download/Databases/) and NCBI nr databases (Blastx cut-off E-value < 1e-6, protein coverage ≥ 80%, bit-score ≥ 50). Sequences identified as CAZyme genes were then additionally search against the Pfam database using HMMscan (cut-off E-value < 1e-5). Transcripts with agreement of the three search results were used in further investigation.
Phylogenetic analysis
Phylogenetic analysis was used to reveal evolutionary relationships of the GH genes of the grapsoids with other taxa. Translated sequences were aligned with sequences of corresponding genes retrieved from the NCBI database using MUSCLE. The gene names and accession IDs of the sequences from NCBI were documented in the phylogenetic tree labels together with species names. Substitution model and phylogenetic analysis was performed using Randomized Axelerated Maximum Likelihood (RAxML) (Stamatakis, 2014).
Predictions on protein structure
The signal peptide and molecular weight of GHs were predicted using SignalIP (Emanuelsson et al., 2007) (http://www.cbs.dtu.dk/services/SignalP/) and Expasy (Gasteiger et al., 2005) (https://web.expasy.org/compute_pi/), respectively. Protein structures and catalytic sites of GHs were predicted using Phyre2 (Protein Homology/analogY Recognition Engine V 2.0) (Kelley et al., 2015).
Results
Biochemical properties of cellulases in the gastric juice
Endoglucanase (EG) and β-glucosidase (BG) activity were detected in the gastric juice of the 15 grapsoid species (Figure 2), demonstrating their ability to break down cellulose into assimilable glucose. The average activity of EG and BG detected ranged 1.46-2.98 μmol min−1 ml−1 and 0.06-0.61 μmol min−1 ml−1, respectively. The crabs have different temperature and pH optima for EG activity (Table 2). In addition, some species showed no significant activity difference and only little variation in activity at different temperatures (Figures 3, 4). EG of all species functioned well at pH 5-7.
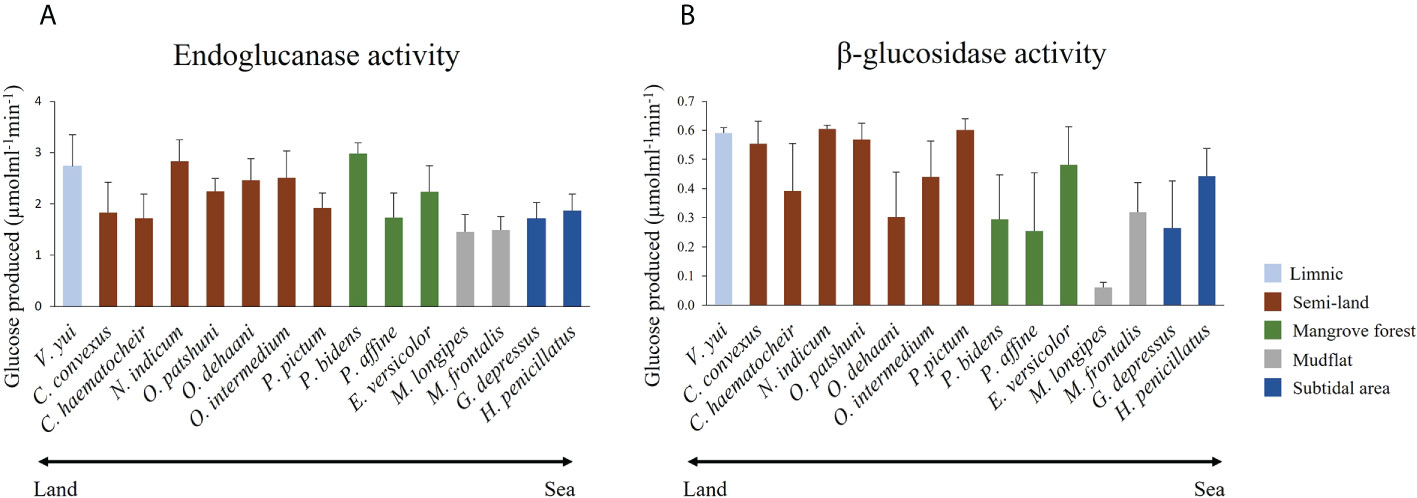
Figure 2 Mean (+SD) (A) endoglucanase and (B) b-glucosidase activities of the 15 grapsoid crabs from different habitats along the land-sea transition.
Endoglucanase activity in relation to species and habitats
The average EG activity of the sesarmids, varunids and grapsids investigated were 2.30 ± 0.06, 1.95 ± 0.08 and 1.49 ± 0.17 μmol min−1ml−1, respectively. The two-way ANOVA showed that both taxa and habitat significantly influenced EG activity, but there was no interaction between the two factors. Post-hoc comparisons suggested that EG activity followed the trend of Sesarmidae > Varunidae > Grapsidae (P < 0.05) (Table 3).
The EG activity of Parasesarma bidens was 2.98 ± 0.20 μmol min−1 ml−1, the highest among the 15 species and 30 to 100% higher than ten other species (Supplementary Table S1), including species inhabiting the land margin, mangrove forest, mudflat, and subtidal area. Previous study also showed that P. bidens exhibited higher cellulase activity when compared with mudflat and sandflat species (Kawaida et al., 2019). All the species with significantly higher activity were from land, limnic and mangrove areas, which explains the significantly higher EG activity of crabs from the land margin, mangrove and limnic habitats than those from the mudflat and subtidal area (Table 3). For the genus level 2-way ANOVA test, only the genera Parasesarma and Orisarma comprised more than one species in our study. Although Parasesarma contained members (i.e., P. bidens) with relatively higher EG activity, the overall activity became less prominent when congeners were included. Neosarmatium then became the genus with the highest activity.
β-glucosidase activity in relation to species and habitats
The average BG activity of sesarmids, varunids and grapsids were 0.41 ± 0.02, 0.39 ± 0.02 and 0.32 ± 0.05 μmol min−1 ml−1, respectively. Again, both taxa and habitat significantly influenced BG activity, but there was no interaction between them. The BG activity of sesarmids was significantly higher than those of the varunids and grapsids (Table 4). Neosarmatium indicum had the highest BG activity while M. longipes had the lowest and significantly lower activity than the other 14 species (Supplementary Table S2). The BG activity of species followed the order semi-land and limnic > mangrove and subtidal > mudflat (P < 0.05, Table 4).
GH families identified
The results on BUSCO are described in Figure 5. A total of 23 GH families were identified (Table 5). Five subfamilies of GH13 and a subfamily 10 of GH5 were identified. GH 5_10, 9, 13, 13_17, 13_24 and 16 are the GH families that include digestive enzymes, and they were analysed using Phyre2.
Discussion
General activity of cellulases
The ability of the 15 grapsoid crabs to produce cellulase is congruent with the reported ubiquity of cellulases in crustaceans (Benson et al., 1999; Adachi et al., 2012; Bui and Lee, 2015; Liu et al., 2015; Adachi et al., 2016). The average activity of both endoglucanase (EG) and β-glucosidase (BG) was lower than that reported in the mangrove sesarmid Parasesarma erythodactylum (EG: 4.44 ± 2.01 μmol min−1 ml−1; BG: 0.93 ± 0.71 μmol min−1 ml−1); the higher activity in P. erythodactylum was probably caused by leaf-feeding before gastric juice extraction (Bui and Lee, 2015). Most species in this study have pH optimum of 5-6 range for EG functioning, with exception of N. indicum and species from subtidal region (G. depressus and H. penicillatus) which have pH optimum of 7. These readings were within pH 4-7 range i.e., at slightly acidic condition at which crustaceans cellulases are active (Xue et al., 1999; Linton and Greenaway, 2004; Bui and Lee, 2015). Although the pH optimum for subtidal species was 7 which could be due to the less acidic subtidal environment, the EG activity differ only slightly at different pH values.
GHs identified
The physiological function of the identified GHs is diverse such as facilitating digestion (e.g., GH5_10 and GH9) (Cragg et al., 2015; Linton, 2021), immune function (e.g., GH16) (Viborg et al., 2019), and development (e.g., GH18) (Chen et al., 2020). The crabs investigated generally possessed more than one transcript for most GH families. This could be a result of gene duplication, which is common in crustaceans. For instance, three GH9 isozymes were identified in Gecarcoidea natalis (Gan et al., 2018). The presence of transcriptomes of these GHs indicated the endogenous source of these enzymes including GH9 cellulase.
GH9 endoglucanase
The GH9 of all the species do not contain a carbohydrate-binding module, which is common in prokaryotic endoglucanases. GH9 EG of the crabs are non-processive enzymes that release the substrate before the next reaction (Watanabe and Tokuda, 2010). The crabs have 2 to 10 transcripts, just like some other crustaceans (Dammannagoda et al., 2015; Gan et al., 2018). The predicted molecular weight of EG ranged between 49.3 to 63.6 kDa, which aligns with the 44.3 to 84.8 kDa range reported by Adachi et al. (2012). The catalytic acids and bases were detected in the GH9 sequences (Supplementary Figure S1). The absence of signal peptides in some transcripts could be due to their incompleteness (Supplementary Figure S1). Confident signal peptides were not detected in some insect GH9, which were used in the phylogenetic analysis. The lack of signal peptides in protein sequences suggests that the protein might not be exported. Further investigation is needed to confirm the destination of these GH9 proteins.
Phylogenetic analysis of GH9
A monophyletic tree of GH9s (Figure 6) is evident and aligns with the previously suggested monophyletic group of animal GH9 (Davison and Blaxter, 2005). Three clades (I, II and III) (Bootstrap values: 86, 99 and 76) of homologous GH9s can be observed among the grapsoids. Gene duplication can be observed in each clade. GH9 clade III further split into two clades with confident bootstrap values (97 and 97). In each group, GH9 of crabs from the same family are usually confidently clustered together except varunids in clade I and sesarmids in clade II. Previously described GH9 of P. erythodactylum and G. natalis grouped with clade I, that of crayfish Cherax quadricarinatus seems to be closer to clades II and III, while that of the land hermit crab Coenobita rugosus is closer to clade III. Crabs possess different GH9 combinations of the three clades (Table 6). All species studied had clade II GH9, O. intermedium was the only one lacking clade I GH9 while E. versicolor and M. frontalis lacked clade III GH9. The sesarmids in clades I and II further split and clustered according to their habitats (land or mangrove).
Phylogenetic analysis of other digestive GHs
GH5, GH13, GH13_17, GH13_24 and GH16 are CAZyme families of endo-β-1,4-mannase, amylase, pancreatic amylase, and endo-β-1,3-glucanase, respectively. These enzymes break down carbohydrates such as starch and hemicellulose. The catalytic residues of these GHs except GH5_10 and GH13_17 were identified by Phyre2, but catalytic residues of GH5_10 and GH13_17 can be recognized when aligning with homologues of other organisms (Supplementary Figures S2-6). Gene duplication of these GHs is common. GH5, GH13, GH13_17, GH13_24 and GH16 of sesarmids were usually clustered together (Supplementary Figures S7-11). C. convexus has an especially high number (12) of GH5_10 transcripts while other species only have between one to four. The predicted molecular mass of GH5_10 ranged 39.9 to 44.2 kDa, which is smaller than that of the terrestrial grapsoid G. natalis (66.3 ± 0.7 kDa) (Linton, 2021). Again, phylogenetic trees show clustering according to crab families. In addition, sesarmids split according to habitats in trees of GH5_10, GH13, GH13_17 and GH13_24. Neosarmatium indicum, a borrowing crab inhabiting the landward margin of mangroves, clustered mostly with species living in the mangrove. The presence of catalytic residues in the GH16 sequenced (Supplementary Figure S6) indicates their identity as GH16_3, which could be endo-β(1,3)-glucanase (EC 3.2.1.39) and/or endo-β(1,3)/β(1,4)-glucanase activity (EC 3.2.1.6), but not GH16_4 (Viborg et al., 2019). The GH16 of grapsoids identified form confident clades with other crustaceans (Bootstrap value: 100) (Supplementary Figure S11).
GH9, cellulase activity, diet, habitat, and taxonomic affiliation
EG activity decreased along the land-sea transition, i.e., semi-land, mangrove and limnic > mudflat and subtidal area, and shows taxonomic pattern of Sesarmidae > Varunidae > Grapsidae. BG shows pattern of semi-land and limnic > mangrove and subtidal > mudflat, and Sesarmidae and Varunidae > Grapsidae. The higher EG and BG activities of land, mangroves and limnic species could be due to their herbivorous diet, feeding on the abundant litters from mangroves or other vascular plants (see Lee, 1989b; Poon et al., 2010; Devi et al., 2013; Miyake et al., 2019). Sesarmidae and varunidae contain land and/or mangrove species (Table 1), this habitat concentration may explain the significantly higher cellulase activity in the group. The availability of vascular plant or mangrove material and the dietary preferences of crabs seem to have a strong effect on the cellulase production profiles of grapsoid crabs. The effect of habitat can also be seen in the phylogenetic analysis, especially among the sesarmid crabs that land and mangrove species are separated in GH9 clades I and III. This result suggests that environment, with the corresponding implications for organic matter quality and quantity, can be a significant driving force in the evolution of GH9, which could tackle one of the biggest challenges (adaptation to vascular plant-based diet) during the evolution of terrestrialization. The same influence can be seen in GH13_17 and GH13_24 of sesarmid crabs (Supplementary Figures S9, S10). However, there must be additional factors involved as the land varunid C. convexus never clustered with the sesarmids sharing the same habitat region along the land-sea transition.
Mangrove mineralisation by grapsoid crabs
Previous studies on mangrove mineralisation focused on the leaf-eating sesarmid crabs (Lee, 1989a; Robertson and Daniel, 1989; Adachi et al., 2012; Bui and Lee, 2015). Our results showed that species from other families and habitats also possess cellulases, reflecting an under-estimation in contribution of grapsoid crabs to mangrove carbon mineralisation. Cellulase production seems to be a universal trait among brachyuran crabs, just like other crustaceans (McClintock et al., 1991; Benson et al., 1999; Tanimura et al., 2013). This result suggests that the carbon mineralising capability of crabs is more widespread than previously thought and is worth re-evaluation.
Cellulases are the major members of GH9. The presence of GH9 transcriptomes in the hepatopancreas of the crabs indicated endogenous contribution to cellulase production. Further investigation is needed to reveal the dependence of crabs on symbiotic cellulases. The total cellulase activity of P. erythodactylum (EG: 4.44 ± 2.01 μmol min−1 ml−1; BG: 0.93 ± 0.71 μmol min−1 ml−1) was considered to be low while our results are even lower when compared to organisms such as sea hare and krill (Table 7) although the methods of activity determination were not exactly the same. At the same time, most of the EG and BG activity of land and mangrove species in this study is comparable to or higher than that of predominantly herbivorous land crabs, e.g., G. natalis (EG: 2.304 ± 0.116 μmol min−1 ml−1, pH 7; BG: 0.521 ± 0.253 μmol min−1 ml−1, pH 5.5) and Cardisoma hirtipes (EG: 1.534 ± 0.206 μmol min−1 ml−1, pH 5.5; BG: 1.961 ± 0.074 μmol min−1 ml−1, pH 7) (Linton and Greenaway, 2004), except that the BG activity of C. hirtipes is several fold those of the BG activities of the crabs we studied. Therefore, low cellulase activity seems to be common among crabs, even for those predominantly herbivorous species. Firstly, the low activity of cellulases especially BG implies the possibility that carbon in cellulose may not be the main target of the crabs’ physiological machinery. Linton (2020) suggested that the function of EG could be for breaking down cell walls to obtain the more digestible cellular contents. Secondly, the low cellulase activity may be already sufficient if carbon is the main target. Mangrove and vascular plants are rich carbon sources, indicated by their high carbon content (Jones et al., 2020). With the continuous provision of such a carbon source from land and mangrove plants along the land-sea transition, it is possible that even low cellulase production is enough for the crabs to obtain sufficient energy if they ingest significant amounts of these vascular plant sources.
The presence of both EG and BG in the gastric juice ensures the digestion of cellulose to glucose and the assimilation of refractory carbon like cellulose when available. Irrespective of the extent, mangrove carbon mineralisation and assimilation would occur once the crabs ingest mangrove materials. There is solid evidence of grapsoid crabs consuming mangrove material, including gut content analysis of P. bidens and M. frontalis (Poon et al., 2010), and laboratory observation of P. affine (Lee, 1989b), Episesarma versicolor (Thongtham et al., 2008), and Chiromantes haematocheir and Orisarma dehaani (Miyake et al., 2019). While some species prefer aged mangrove leaf litter, some readily consume freshly available material, e.g., Neosarmatium trispinosum (Harada and Lee, 2016). Therefore, land, mangrove, and mudflat grapsoid species could also be heavily involved in mangrove carbon mineralisation. N. indicum, P. pictum and M. longipes are also likely to do so because they may also consume mangrove like some of their congeners (Lee, 1989b; Nordhaus et al., 2011; Harada and Lee, 2016). Even M. frontalis inhabiting the lower mangrove margin and mudflat has 10-40% vascular plant material in their gut contents. With results indicating most of these species producing EG and BG, we confirm that there could be significant utilisation and assimilation of mangrove carbon through species that are not mangrove sesarmids, a process that is rarely reported. As mentioned, V. yui probably has vascular plants as a major component of its gut contents like Varuna litterata (Devi et al., 2013), implying that V. yui could also mineralise and assimilate mangrove carbon if mangrove leaf litter is available. Other land species are considered as predominantly herbivorous. The high leaf litter standing crop of the mangrove Kandelia obovata observed where V. yui and semi-land species were collected suggests this scenario is highly possible. Stable isotope analysis could be used to indicate the mangrove assimilation and utilisation by these crabs.
There is limited data on the diet of the subtidal species (Depledge, 1989), but they are believed to be suspension-feeding, mainly utilising phytoplankton or fine particulate matter. Subtidal species might use cellulase to digest the POM derived from mangrove leaf litter already processed by sesarmid crabs (Werry and Lee, 2005). It is possible for subtidal species to mineralise and utilise mangrove carbon in the form of POM, or leaf litter if available, albeit only occasionally.
Other GHs compensating for the low cellulase activity
The presence of other carbohydrate-digestive enzymes allows the crabs to assimilate carbon not just from cellulose but also other carbohydrates such as hemicellulose and starch. This may explain the low cellulase activity of some of the crabs. GH5_10 endo-β-1,4-mannase is the only hemicellulase identified in this study. It targets mannans such as galactomannan and glucomannan, which can contribute up to 5% of the plant primary cell wall (Scheller and Ulvskov, 2010). GH5_10 was also reported from bivalves (Xu et al., 2002; Larsson et al., 2006), gastropods (Ootsuka et al., 2006; Zahura et al., 2011), and crustacean such as Gecarcoidea natalis (Gan et al., 2018; Linton, 2021). Linton (2021) suggested that mannan might be an important carbon source to terrestrial crustaceans. GH13 amylase, GH13_24 amylase and GH13_17 α-glucosidase release α-glucose from different substrates such as starch and sucrose, which are the carbohydrates abundant in plants. GH16_3 β-1,3-glucanase cleave β-1,3-glucans if secreted to the stomach. GH5_10 endo-β-1,4-mannase, GH13_24 amylase and GH16 β-1,3-glucanase have already been described in three terrestrial crustaceans, including G. natalis (Linton, 2021). The expression of various GHs in the hepatopancreas of grapsoid crabs suggests the availability of various forms of carbon sources to the crabs, and cellulose may not be the only carbon source utilised. It is possible that symbiotic gut cellulolytic bacteria may play a role in cellulose digestion. For instance, previous studies have already indicated the presence of cellulolytic gut bacteria in various organisms such as bivalve Barnea birmanica (Balasubramanian et al., 1979), shipworm species from the genera Bankia, Lyrodus and Teredo (Betcher et al., 2012), and terrestrial snail Helix pomatia (Stradine and Whitaker, 1963).
Low BG activity in Metaplax longipes
For Metaplax longipes, its very low β-glucosidase activity (0.06 ± 0.02 μmol min−1 ml−1) makes its capacity for mangrove carbon mineralisation doubtful. The β-glucosidase activity of M. longipes is significantly lower than the other 14 species in this study. Compound-specific stable isotope analysis indicated that diet of M. longipes is mainly composed of deposited phytoplankton and microphytobenthos (S.Y. Lee et al., unpublished data). The carbon stable isotope value of M. longipes muscle from Ting Kok (-16.6 ± 3.3‰, C.Y. Lee and S.Y. Lee, unpublished data) also reflected the low dependence on vascular plant or mangrove materials, where predominantly herbivorous crabs have higher carbon stable isotopic value (e.g., δ13C of Parasesarma bidens muscle: −25.7 ± 0.8‰ (Kristensen et al., 2017)). On the other hand, other GHs and cellulolytic bacteria may compensate the low activity of cellulase as the tool to assimilate carbon from mangrove or vascular plant as mentioned above.
Other important GHs
Other GHs such as the various subfamilies of GH18 identified in this study may also be important for the crabs in food utilisation. Digestive GH18 chitinases could help the crabs obtain carbon from the chitinous cell wall of fungi or crustacean shells. The GH38 lysosomal α-mannosidase of Tenebrio molitor (Accession: KP892646.1) is secreted into the midgut and acts as digestive enzyme (Moreira et al., 2015). It is possible that GH38 works in the same manner in the crabs, but further confirmation is needed. There is still a lot to be explored for the GHs (e.g., their role in the immune system). However, the lacking data of crabs on databases such as CAZy database could be an obstacle, i.e., the data on arthropods is strongly dominated by insects. It is crucial to expand the crustacean database for future investigations on non-insect taxa.
Conclusion
This comprehensive study indicates that endogenous cellulase production of grapsoid crabs is common along the land-sea transition, giving these species the capacity for mineralising and utilising mangrove carbon, more so for land, mangrove and mudflat species due to their relatively higher EG activity. We also provide evidence of the potential of subtidal grapsoids for utilising mangrove production. Although the cellulase activity of the land and mangrove crabs are comparable to those of other land crabs, the level of activity is generally low, which seems to be a common phenomenon among crabs. Our results on the expression of various GH families in the hepatopancreas of grapsoids suggest that cellulose is not the only carbon source available to the crabs. They may also depend on other carbohydrates such as starch and mannan, which may explain the low cellulase activity among these crustaceans. Future studies on mangrove carbon assimilation by these crabs could further assess their role in mangrove carbon utilisation in connected habitats along the land-sea transition, which has significant implications for the study of the global mangrove carbon budget. Other than endogenous production, symbiotic cellulases might also contribute to the apparent cellulase production of the crabs. Further clarification of the role of gut cellulolytic bacteria of the crabs could also be another fruitful area of future investigation.
Data availability statement
The raw data supporting the conclusions of this article will be made available by the authors, without undue reservation. The hepatopancreatic transcriptome data presented in the study are deposited in the NCBI database, accession number: PRJNA866679. https://www.ncbi.nlm.nih.gov/bioproject/PRJNA866679.
Author contributions
Both authors conceived the ideas, designed the experiments, wrote the manuscript and gave final approval for publication.
Funding
CYL is supported by a PhD scholarship from The Chinese University of Hong Kong.
Acknowledgments
We would like to thank Dr. Kayan Ma for helping with transcriptomic analysis.
Conflict of interest
The authors declare that the research was conducted in the absence of any commercial or financial relationships that could be construed as a potential conflict of interest.
Publisher’s note
All claims expressed in this article are solely those of the authors and do not necessarily represent those of their affiliated organizations, or those of the publisher, the editors and the reviewers. Any product that may be evaluated in this article, or claim that may be made by its manufacturer, is not guaranteed or endorsed by the publisher.
Supplementary material
The Supplementary Material for this article can be found online at: https://www.frontiersin.org/articles/10.3389/fmars.2022.1002502/full#supplementary-material
References
Adachi K., Tanimura K., Mitsui T., Morita T., Yosho I., Ikejima K., et al. (2016). Cellulolytic activity in the hepatopancreas of Chionoecetes opilio and Chionoecetes japonicus: Enzymatic adaptations to deep sea environment. Fish. Sci. 82, 835–841. doi: 10.1007/s12562-016-1014-8
Adachi K., Toriyama K., Azekura T., Morioka K., Tongnunui P., Ikejima K. (2012). Potent cellulase activity in the hepatopancreas of mangrove crabs. Fish. Sci. 78, 1309–1314. doi: 10.1007/s12562-012-0547-8
An T., Dong Z., Lv J., Liu Y., Wang M., Wei S., et al. (2015). Purification and characterization of a salt-tolerant cellulase from the mangrove oyster, Crassostrea rivularis. ABBS 47, 299–230. doi: 10.1093/abbs/gmv015
An T., Lyu J., Jia W., Wang M., Wei S., Zhang Y. (2014). Role of macrobenthic fauna in mangrove carbon fluxes indicated by their cellulase and hemicellulase activities. Mar. Biol. Res. 10, 934–940. doi: 10.1080/17451000.2013.863355
Ashton E. C. (2002). Mangrove sesarmid crab feeding experiments in peninsular Malaysia. J. Exp. Mar. Biol. Ecol. 273, 97–119. doi: 10.1016/S0022-0981(02)00140-5
Balasubramanian T., Lakshmanaperumalsamy P., Chandramohan D., Natarajan R. (1979). Cellulolytic activity of streptomycetes isolated from the digestive tract of a marine borer. IJMS 8, 111–114.
Bärlocher F., Arsuffi T. L., Newell S. Y. (1989). Digestive enzymes of the saltmarsh periwinkle Littorina irrorata (Mollusca: Gastropoda). Oecologia 80, 39–43. doi: 10.1007/BF00789929
Benson L. K., Rice S. A., Johnson B. R. (1999). Evidence of cellulose digestion in the wood boring isopod Sphaeroma terebrans. Fla. Sci. 62, 128–144. doi: 10.1017/S095283690200050X
Betcher M. A., Fung J. M., Han A. W., O'Connor R., Seronay R., Concepcion G. P., et al. (2012). Microbial distribution and abundance in the digestive system of five shipworm species (Bivalvia: Teredinidae). PloS One 7, e45309. doi: 10.1371/journal.pone.0045309
Bolger A. M., Lohse M., Usadel B. (2014). Trimmomatic: A flexible trimmer for illumina sequence data. Bioinformatics 30, 2114–2120. doi: 10.1093/bioinformatics/btu170
Bouillon S., Borges A. V., Castañeda-Moya E., Diele K., Dittmar T., Duke N. C., et al. (2008). Mangrove production and carbon sinks: A revision of global budget estimates. Global Biogeochem. Cycles. 22. doi: 10.1029/2007GB003052
Bui T. H. H., Lee S. Y. (2015). Endogenous cellulase production in the leaf litter foraging mangrove crab Parasesarma erythodactyla. Comp. Biochem. Physiol. 179, 27–36. doi: 10.1016/j.cbpb.2014.09.004
Camilleri J. (1992). Leaf-litter processing by invertebrates in a mangrove forest in Queensland. Mar. Biol. 114, 139–145. doi: 10.1007/BF00350863
Chen W., Jiang X., Yang Q. (2020). Glycoside hydrolase family 18 chitinases: The known and the unknown. Biotechnol. Adv. 43, 107553. doi: 10.1016/j.biotechadv.2020.107553
Cosgrove D. J. (2005). Growth of the plant cell wall. Nat. Rev. Mol. Cell. Biol. 6, 850–861. doi: 10.1038/nrm1746
Cosgrove D. J. (2014). Re-constructing our models of cellulose and primary cell wall assembly. Curr. Opin. Plant Biol. 22, 122–131. doi: 10.1016/j.pbi.2014.11.001
Cragg S. M., Beckham G. T., Bruce N. C., Bugg T. D. H., Distel D. L., Dupree P., et al. (2015). Lignocellulose degradation mechanisms across the tree of life. Curr. Opin. Chem. Biol. 29, 108–119. doi: 10.1016/j.cbpa.2015.10.018
Dammannagoda L. K., Pavasovic A., Prentis P. J., Hurwood D. A., Mather P. B. (2015). Expression and characterization of digestive enzyme genes from hepatopancreatic transcripts from redclaw crayfish (Cherax quadricarinatus). Aquac. Nutr. 21, 868–880. doi: 10.1111/anu.12211
Davison A., Blaxter M. (2005). Ancient origin of glycosyl hydrolase family 9 cellulase genes. Mol. Biol. Evol. 22, 1273–1284. doi: 10.1093/molbev/msi107
Depledge M. H. (1989). Observations on the feeding behaviour of Gaetice depressus (Grapsidae: Varuninae) with special reference to suspension feeding. Mar. Biol. 100, 253–260. doi: 10.1007/BF00391966
Devi P. L., Gopi Nair D., Joseph A., Nair D. G., Joseph A. (2013). Habitat ecology and food and feeding of the herring bow crab Varuna litterata (Fabricius 1798) of Cochin backwaters, kerala, India. Arthopods 2, 172–188.
Emanuelsson O., Brunak S., von Heijne G., Nielsen H. (2007). Locating proteins in the cell using TargetP, SignalP and related tools. Nat. Protoc. 2, 953–971. doi: 10.1038/nprot.2007.131
Emmerson W. D., McGwynne L. E. (1992). Feeding and assimilation of mangrove leaves by the crab Sesarma meinerti de man in relation to leaf-litter production in mgazana, a warm-temperate southern African mangrove swamp. J. Exp. Mar. Biol. Ecol. 157, 41–53. doi: 10.1016/0022-0981(92)90073-J
Fratini S., Cannicci S., Abincha L. M., Vannini M. (2000). Feeding, temporal, and spatial preferences of Metopograpsus thukuhar (Decapoda; grapsidae): An opportunistic mangrove dweller. J. Crustac. Biol. 20, 326–333. doi: 10.1163/20021975-99990044
Fu L., Niu B., Zhu Z., Wu S., Li W. (2012). CD-HIT: Accelerated for clustering the next-generation sequencing data. Bioinformatics 28, 3150–3152. doi: 10.1093/bioinformatics/bts565
Gan H. M., Austin C., Linton S. (2018). Transcriptome-guided identification of carbohydrate active enzymes (CAZy) from the Christmas island red crab, Gecarcoidea natalis and a vote for the inclusion of transcriptome-derived crustacean CAZys in comparative studies. Mar. Biotechnol. 20, 654–665. doi: 10.1007/s10126-018-9836-2
Gasteiger E., Hoogland C., Gattiker A., Duvaud S., Wilkins M. R., Appel R. D., et al. (2005). "Protein identification and analysis tools on the ExPASy server," in the proteomics protocols handbook. Ed. J. M. Walker (New Jersey USA: Humana Press) pp. 571–607. Available at: https://link.springer.com/protocol/10.1385/1-59259-890-0:571#citeas
Grabherr M. G., Haas B. J., Yassour M., Levin J. Z., Thompson D. A., Amit I., et al. (2011). Full-length transcriptome assembly from RNA-seq data without a reference genome. Nat. Biotechnol. 29, 644–652. doi: 10.1038/nbt.1883
Gray M., Linton S. M., Allardyce B. J. (2018). cDNA sequences of GHF9 endo-β-1,4-glucanases in terrestrial Crustacea. Gene 642, 408–422. doi: 10.1016/j.gene.2017.11.030
Harada Y., Lee S. Y. (2016). Foraging behavior of the mangrove sesarmid crab Neosarmatium trispinosum enhances food intake and nutrient retention in a low-quality food environment. Estuar. Coast. Shelf Sci. 174, 41–48. doi: 10.1016/j.ecss.2016.03.017
Jones A. R., Raja Segaran R., Clarke K. D., Waycott M., Goh W. S. H., Gillanders B. M. (2020). Estimating mangrove tree biomass and carbon content: A comparison of forest inventory techniques and drone imagery. Front. Mar. Sci. 6. doi: 10.3389/fmars.2019.00784
Jue C. K., Lipke P. N. (1985). Determination of reducing sugars in the nanomole range with tetrazolium blue. J. Biochem. Biophys. Methods 11, 109–115. doi: 10.1016/0165-022x(85)90046-6
Kawaida S., Nanjo K., Ohtsuchi N., Kohno H., Sano M. (2019). Cellulose digestion abilities determine the food utilization of mangrove estuarine crabs. Estuar. Coast. Shelf Sci. 222, 43–52. doi: 10.1016/j.ecss.2019.04.004
Kawaida S., Nanjo K., Ohtsuchi N., Kohno H., Sano M. (2021). Crabs assimilating cellulose materials drive the detritus food chain in a mangrove estuary. Food Webs. 26, e00180. doi: 10.1016/j.fooweb.2020.e00180
Kelley L. A., Mezulis S., Yates C. M., Wass M. N., Sternberg M. J. E. (2015). The Phyre2 web portal for protein modeling, prediction and analysis. Nat. Protoc. 10, 845–858. doi: 10.1038/nprot.2015.053
Kristensen E., Lee S. Y., Mangion P., Quintana C. O., Valdemarsen T. (2017). Trophic discrimination of stable isotopes and potential food source partitioning by leaf-eating crabs in mangrove environments. Limnol. Oceanogr. 62, 2097–2112. doi: 10.1002/lno.10553
Larsson A. M., Anderson L., Xu B., Muñoz I. G., Usón I., Janson J. C., et al. (2006). Three-dimensional crystal structure and enzymic characterization of β-mannanase Man5A from blue mussel Mytilus edulis. J. Mol. Biol. 357, 1500–1510.75–87. doi: 10.1016/0272-7714(89)90074-7
Lee S. Y. (1989a). Litter production and turnover of the mangrove Kandelia candel (L.) druce in a Hong Kong tidal shrimp pond. Estuar. Coast. Shelf Sci. 29, 75–87. doi: 10.1016/0272-7714(89)90074-7
Lee S. Y. (1989b). The importance of sesarminae crabs chiromanthes spp. and inundation frequency on mangrove (Kandelia candel (L.) druce) leaf litter turnover in a Hong Kong tidal shrimp pond. J. Exp. Mar. Biol. Ecol. 131, 23–43. doi: 10.1016/0022-0981(89)90009-9
Lee S. Y. (1997). Potential trophic importance of the faecal material of the mangrove sesarmine crab. Sesarma massa. Mar. Ecol. Prog. Ser. 159, 275–284. doi: 10.1016/j.ecss.2010.02.016
Leh C. M. U., Sasekumar A. (1985). The food of sesarmid crabs in Malaysia mangrove forests. MNJ 39, 135–145. Available at: https://cir.nii.ac.jp/crid/1571698600090048000.
Li W., Godzik A. (2006). Cd-hit: A fast program for clustering and comparing large sets of protein or nucleotide sequences. Bioinformatics 22, 1658–1659. doi: 10.1093/bioinformatics/btl158
Linton S. M. (2020). Review: The structure and function of cellulase (endo-β-1,4-glucanase) and hemicellulase (β-1,3-glucanase and endo-β-1,4-mannase) enzymes in invertebrates that consume materials ranging from microbes, algae to leaf litter. Comp. Biochem. Physiol. B Biochem. Mol. Biol. 240, 110354. doi: 10.1016/j.cbpb.2019.110354
Linton S. M. (2021). Presence and activity of endo-β-1,4-mannase, an important digestive carbohydrase within the digestive fluid of terrestrial crustaceans. J. Comp. Physiol. B Biochem. Syst. Environ. Physiol. 191, 243–253. doi: 10.1007/s00360-021-01342-4
Linton S. M., Greenaway P. (2004). Presence and properties of cellulase and hemicellulase enzymes of the gecarcinid land crabs Gecarcoidea natalis and Discoplax hirtipes. J. Exp. Biol. 207, 4095–4104. doi: 10.1242/jeb.01252
Linton S. M., Greenaway P. (2007). A review of feeding and nutrition of herbivorous land crabs: adaptations to low quality plant diets. J. Comp. Physiol. B Biochem. Syst. Environ. Physiol. 177, 269–286. doi: 10.1007/s00360-006-0138-z
Linton S. M., Greenaway P., Towle D. W. (2006). Endogenous production of endo-beta-1,4-glucanase by decapod crustaceans. J. Comp. Physiol. B. 176, 339–348. doi: 10.1007/s00360-005-0056-5
Linton S. M., Shirley A. J. (2011). Isozymes from the herbivorous gecarcinid land crab, Gecarcoidea natalis that possess both lichenase and endo-β-1,4-glucanase activity. Comp. Biochem. Physiol. B Biochem. Mol. Biol. 160, 44–53. doi: 10.1016/j.cbpb.2011.05.007
Liu W., Tanimura A., Yamada K., Toyohara H., Chew L., Hanamura Y., et al. (2015). Occurrence of cellulose activities in planktonic crustaceans inhabiting mangrove areas in Malaysia. Jpn. Agric. Res. Q. 49, 293–299. doi: 10.6090/jarq.49.293
Lombard V., Golaconda Ramulu H., Drula E., Coutinho P. M., Henrissat B. (2014). The carbohydrate-active enzymes database (CAZy) in 2013. Nucleic Acids Res. 42, D490–D495. doi: 10.1093/nar/gkt1178
Mazumder D., Saintilan N. (2010). Mangrove leaves are not an important source of dietary carbon and nitrogen for crabs in temperate Australian mangroves. Wetlands 30, 375–380. doi: 10.1007/s13157-010-0021-2
McClintock J. B., Klinger T. S., Marion K., Hsueh P. (1991). Digestive carbohydrases of the blue crab Callinectes sapidus (Rathbun): Implications in utilization of plant-derived detritus as a trophic resource. J. Exp. Mar. Biol. Ecol. 148, 233–239. doi: 10.1016/0022-0981(91)90084-A
Mfilinge P. L., Tsuchiya M. (2008). Effect of temperature on leaf litter consumption by grapsid crabs in a subtropical mangrove (Okinawa, Japan). J. Sea Res. 59, 94–102. doi: 10.1016/j.seares.2007.07.004
Micheli F. (1993). Feeding ecology of mangrove crabs in north Eastern Australia: Mangrove litter consumption by Sesarma messa and Sesarma smithii. J. Exp. Mar. Biol. Ecol. 171, 165–186. doi: 10.1016/0022-0981(93)90002-6
Miyake K., Ura K., Chida S., Ueda Y., Baba Y., Kusube T., et al. (2019). Guaiacol oxidation activity of herbivorous land crabs, Chiromantes haematocheir and Chiromantes dehaani. J. Biosci. Bioeng. 128, 316–322. doi: 10.1016/j.jbiosc.2019.02.012
Moreira N. R., Cardoso C., Ribeiro A. F., Ferreira C., Terra W. R. (2015). Insect midgut α-mannosidases from family 38 and 47 with emphasis on those of Tenebrio molitor. Insect Biochem. Mol. Biol. 67, 94–104. doi: 10.1016/j.ibmb.2015.07.012
Nordhaus I., Salewski T., Jennerjahn T. C. (2011). Food preferences of mangrove crabs related to leaf nitrogen compounds in the segara anakan lagoon, Java, Indonesia. J. Sea Res. 65, 414–426. doi: 10.1016/j.seares.2011.03.006
Ootsuka S., Saga N., Suzuki K., Inoue A., Ojima T. (2006). Isolation and cloning of an endo-β-1,4-mannanase from pacific abalone Haliotis discus hannai. J. Biotechnol. 125, 269–280. doi: 10.1016/j.jbiotec.2006.03.008
Pavasovic M., Richardson N. A., Anderson A. J., Mann D., Mather P. B. (2004). Effect of pH, temperature and diet on digestive enzyme profiles in the mud crab, Scylla serrata. Aquaculture 242, 641–654. doi: 10.1016/j.aquaculture.2004.08.036
Poon D. Y. N., Chan B. K. K., Williams G. A. (2010). Spatial and temporal variation in diets of the crabs Metopograpsus frontalis (Grapsidae) and Perisesarma bidens (Sesarmidae): Implications for mangrove food webs. Hydrobiologia 638, 29–40. doi: 10.1007/s10750-009-0005-5
Robertson A. I., Daniel P. A. (1989). The influence of crabs on litter processing in high intertidal mangrove forests in tropical Australia. Oecologia 78, 191–198. doi: 10.1007/BF00377155
Scheller H. V., Ulvskov P. (2010). Hemicelluloses. Annu. Rev. Plant Biol. 61, 263–289. doi: 10.1146/annurev-arplant-042809-112315
Sheaves M., Molony B. (2000). Short-circuit in the mangrove food chain. Mar. Ecol. Prog. Ser. 199, 97–109. doi: 10.3354/meps199097
Simão F. A., Waterhouse R. M., Ioannidis P., Kriventseva E. V., Zdobnov E. M. (2015). BUSCO: Assessing genome assembly and annotation completeness with single-copy orthologs. Bioinformatics 31, 3210–3212. doi: 10.1093/bioinformatics/btv351
Skov M. W., Hartnoll R. G. (2002). Paradoxical selective feeding on a low-nutrient diet: Why do mangrove crabs eat leaves? Oecologia 131, 1–7. doi: 10.1007/s00442-001-0847-7
Stamatakis A. (2014). RAxML version 8: A tool for phylogenetic analysis and post-analysis of large phylogenies. Bioinformatics 30, 1312–1313. doi: 10.1093/bioinformatics/btu033
Stradine G. A., Whitaker D. R. (1963). On the origin of the cellulase and chitinase of Helix pomatia. Biochem. Mol. Biol. 41, 1621–1626. doi: 10.1139/o63-185
Tanimura A., Liu W., Yamada K., Kishida T., Toyohara H. (2013). Animal cellulases with a focus on aquatic invertebrates. Fish. Sci. 79, 1–13. doi: 10.1007/s12562-012-0559-4
Thongtham N., Kristensen E., Puangprasan S. Y. (2008). Leaf removal by sesarmid crabs in bangrong mangrove forest, phuket, thailand; with emphasis on the feeding ecology of Neoepisesarma versicolor. Estuar. Coast. Shelf Sci. 80, 573–580. doi: 10.1016/j.ecss.2008.09.017
Tokuda G., Watanabe H., Matsumoto T., Noda H. (1997). Cellulose digestion in the wood-eating higher termite, Nasutitermes takasagoensis (Shiraki): distribution of cellulases and properties of endo-β-1,4-gIucanase. Zool. Sci. 14, 83–93. doi: 10.2108/zsj.14.83
Tsuji A., Sato S., Kondo A., Tominaga K., Yuasa K. (2012). Purification and characterization of cellulase from north pacific krill (Euphausia pacifica). analysis of cleavage specificity of the enzyme. Comp. Biochem. Physiol. B Biochem. Mol. Biol. 163, 324–333. doi: 10.1016/j.cbpb.2012.08.005
Tsuji A., Tominaga K., Nishiyama N., Yuasa K. (2013). Comprehensive enzymatic analysis of the cellulolytic system in digestive fluid of the Sea hare Aplysia kurodai. efficient glucose release from sea lettuce by synergistic action of 45 kDa endoglucanase and 210 kDa ß-glucosidase. PloS One 8, e65418. doi: 10.1371/journal.pone.0065418
Viborg A. H., Terrapon N., Lombard V., Michel G., Czjzek M., Henrissat B., et al. (2019). A subfamily roadmap of the evolutionarily diverse glycoside hydrolase family 16 (GH16). J. Biol. Chem. 294, 15973–15986. doi: 10.1074/jbc.RA119.010619
Watanabe H., Nakamura M., Tokuda G., Yamaoka I., Scrivener A. M., Noda H. (1997). Site of secretion and properties of endogenous endo-β-1,4-glucanase components from Reticulitermes speratus (Kolbe), a Japanese subterranean termite. Insect Biochem. Mol. Biol. 27, 305–313. doi: 10.1016/S0965-1748(97)00003-9
Watanabe H., Noda H., Tokuda G., Lo N. (1998). A cellulase gene of termite origin. Nature 394, 330–331. doi: 10.1038/28527
Watanabe H., Tokuda G. (2010). Cellulolytic systems in insects. Annu. Rev. Entomol. 55, 609–632. doi: 10.1146/annurev-ento-112408-085319
Werry J., Lee S. Y. (2005). Grapsid crabs mediate link between mangrove litter production and estuarine planktonic food chains. Mar. Ecol. Prog. Ser. 293, 165–176. doi: 10.3354/meps293165
Wood D. E., Salzberg S. L. (2014). Kraken: Ultrafast metagenomic sequence classification using exact alignments. Genome Biol. 15, 1–12. doi: 10.1186/gb-2014-15-3-r46
Xue X. M., Anderson A. J., Richardson N. A., Anderson A. J., Xue G. P., Mather P. B. (1999). Characterisation of cellulase activity in the digestive system of the redclaw crayfish (Cherax quadricarinatus). Aquaculture 180, 373–386. doi: 10.1016/S0044-8486(99)00213-6
Xu B., Hägglund P., Stålbrand H., Janson J. C. (2002). Endo-β-1,4-mannanases from blue mussel, Mytilus edulis: Purification, characterization, and mode of action. J. Biotechnol. 92, 267–277. doi: 10.1016/s0168-1656(01)00367-4
Keywords: mangrove, grapsoid crabs, herbivory, cellulase, glycosyl hydrolase
Citation: Lee CY and Lee SY (2022) Widespread occurrence of endogenous cellulase production and glycosyl hydrolase in grapsoid crabs along the land-sea transition indicates high potential for mineralisation of mangrove production. Front. Mar. Sci. 9:1002502. doi: 10.3389/fmars.2022.1002502
Received: 25 July 2022; Accepted: 07 September 2022;
Published: 27 September 2022.
Edited by:
Jian-Wen Qiu, Hong Kong Baptist University, Hong Kong SAR, ChinaReviewed by:
Hong Wooi Teoh, Xiamen University, MalaysiaRohit Rai, Lovely Professional University, India
Copyright © 2022 Lee and Lee. This is an open-access article distributed under the terms of the Creative Commons Attribution License (CC BY). The use, distribution or reproduction in other forums is permitted, provided the original author(s) and the copyright owner(s) are credited and that the original publication in this journal is cited, in accordance with accepted academic practice. No use, distribution or reproduction is permitted which does not comply with these terms.
*Correspondence: Shing Yip Lee, am9lc3lsZWVAZS5jdWhrLmVkdS5oaw==