- 1Ministry of Education (MOE) Key Laboratory of Marine Genetics and Breeding, College of Marine Life Sciences, Ocean University of China, Qingdao, China
- 2Laboratory for Marine Fisheries Science and Food Production Processes, Qingdao National Laboratory for Marine Science and Technology, Qingdao, China
- 3Laboratory of Tropical Marine Germplasm Resources and Breeding Engineering, Sanya Oceanographic Institution, Ocean University of China, Sanya, China
Dwarf surf clam, Mulinia lateralis, is widely considered as a model species for bivalves. The development of a standard culture system could greatly promote the production of high-quality individuals, yet information on the culture conditions for M. lateralis spat is still limited. This study aims to determine the suitable microalgae diet, temperature, and salinity for M. lateralis spat culture. The typically fed microalgae species, including Chlorella pyrenoidesa (Cp), Platymonas helgolandica (Ph), Dunaliella salina (Ds), Nitzschia closterium (Nc), and Chaetoceros muelleri (Cm), could be taken up by M. lateralis spat, and their filtration rates on Cp, Nc, and Cm were higher than those on Ds and Ph. For the entire spat culture, all diet trials showed similar survival percentages, while the mono-specific diet Cp exhibited the highest growth rate, suggesting that Cp was the optimal microalgae species for M. lateralis spat. Through simultaneously maximizing the growth and survival of spat, the optimal microalgae concentration and stocking density were 5 × 104 cells ml–1 and 400–600 individuals m–2 for 30–40-day-old spat as well as 1 × 105 cells ml–1 and 400–600 individuals m–2 for 40–50-day-old spat, respectively. In addition, the spat had higher growth rates and survival percentages at the temperature of 20–22°C and salinity of 22–25 ppt. The results of this study provide a basis for further culture of M. lateralis spat, and the optimized conditions will be of great significance for the construction of the standard culture system of M. lateralis.
Introduction
The dwarf surf clam, Mulinia lateralis (Say 1822), is a typical burrowing bivalve, which mainly occurs along the Atlantic coast from Canada to Mexico (Calabrese, 1970a). It usually inhabits substrate of sandy and muddy in the estuarine and intertidal zones and can be found in dense populations (Walker and Tenore, 1984). M. lateralis is a small bivalve with an adult shell length of approximately 15–20 mm and reaches sexual maturation in 2 months (Calabrese, 1969a, 1970b). In addition, this species is dioecious with an XX-female and XY-male system of sex determination and presents a continuous reproductive cycle (Calabrese, 1970b; Guo and Allen, 1994). These unique characteristics of M. lateralis have made it an attractive model species for bivalve researches (Calabrese, 1969a; Guo and Allen, 1994; Burgess and Morrison, 2010; Cripe, 2010). Some signs of progress have been made in the physiological ecology, reproduction, and artificial culture of M. lateralis, which laid a solid foundation for further research (Calabrese, 1969b; Shumway, 1983; Shumway et al., 1983; Shumway and Newell, 1984; Yang et al., 2021). While spawning induction and subsequent culture of M. lateralis can be achieved in the laboratory (Calabrese, 1969a; Rhodes et al., 1975), a major challenge impeding production of this species remains the lack of standardized culture protocols.
In order to establish a culture protocol for bivalves, many factors need to be assessed, including diet type and quantity, stocking density, and environmental parameters (such as temperature and salinity) (Cheng et al., 2020). Microalgae are the major diet source for the bivalve, which provide various nutrients to sustain normal functions including growth and survival (Avila et al., 1997; Brown et al., 1997). Nutritional quality is the primary criterion for the choice of microalgae species for bivalve culture, such as lipids, carbohydrates, and proteins (Brown et al., 1997). In addition, microalgae size, form, and mobility affect the ingestion and digestibility of bivalves and are important criteria for microalgae selection (Marshall et al., 2010). Then, according to nutrition requirements, single species or multispecies combinations of microalgae can be selected to feed bivalves. Notably, the performance of bivalve feeding on multispecies is not always better than single species, and the mixture of microalgae has positive, negative, or intermediate effects on the bivalve (Velasco, 2007; Cheng et al., 2020). For Mercenaria mercenaria, the highest levels of larval growth, development, and survival were achieved by feeding Isochrysis galbana and Chaetoceros gracilis in equal proportion for the entire larval duration (Hassan et al., 2021). For Paracentrotus lividus, the larvae fed with a combination of Rhodomonas sp. (Rho) with the selected diatom Phaeodactylum tricornutum showed identical growth performance to larvae fed with Rho monospecific diet (Gomes et al., 2021).
Food availability is an important factor affecting the feeding process and nutrition absorption, and a suitable amount of food could improve the performance of bivalves (Doroudi and Southgate, 2000; Ballesta-Artero et al., 2018; Wang et al., 2021). In Arctica islandica, the maximum growth rate (58.26 μm day–1) was observed at the microalgae concentration of 1.5 × 104 cells ml–1, and insufficient or excessive microalgae is not conducive to the growth (Ballesta-Artero et al., 2018). In addition, stocking density is also an important factor affecting the utilization of food, and density-dependent growth and survival have been reported in many bivalves (Liu et al., 2010; Nowland et al., 2019). Hence, optimizing the conditions of microalgae concentration and stocking density is necessary to ensure the food availability for the bivalve. In Clinocardium nuttallii, the stocking density of 4 larvae ml–1 combined with the microalgae concentration of 5 × 104 cells ml–1 significantly improved the larval growth and survival (Liu et al., 2010).
Numerous studies have shown that environmental factors significantly influence the culture of bivalves, especially temperature and salinity (Velez et al., 2016; Ballesta-Artero et al., 2018; Acosta et al., 2021). For example, Wang et al. (2021) reported that temperature exerted significant effects on postlarva, and temperature of 26.4–29.2°C resulted in the greatest specific growth and survival for Pinctada martensii. For Mytilus galloprovincialis, there were apparent cellular damages and a decrease in metabolic capacity when salinities were out of the optimal ranges (25–35 ppt) (Freitas et al., 2020). Therefore, the optimization of these factors is crucial for a successful and efficient culture system. In our previous study, the rearing conditions for M. lateralis have been developed by optimizing the microalgae diet and stocking density, which greatly promoted the production of high-quality larvae under laboratory conditions (Yang et al., 2021). However, very less is known about the optimal culture conditions for M. lateralis spat, which is a key stage in the transition from juvenile to adult.
In this study, the ingestion of M. lateralis spat of different microalgae diets is first assessed. Furthermore, the growth and survival of spat cultured using different microalgae diet, microalgae concentration, spat stocking density, culture temperature, and salinity are evaluated. The results will provide the optimal culture conditions for spat and underpin the establishment of the standard culture system of M. lateralis.
Materials and Methods
Experimental Animal and Culture
Mulinia lateralis used in the current experiment were obtained from the Ministry of Education (MOE) Key Laboratory of Marine Genetics and Breeding, Ocean University of China (Qingdao, China). The individuals in the spat stage were selected, which corresponds to the rapid growth period of M. lateralis life, as shown in Figure 1. They were cultured in adequately aerated seawater at a temperature of 21–22°C and salinity of 27–28 ppt. Seawater was pretreated with sand, activated carbon, and absorbent cotton as filtered seawater (FSW). The temperature and salinity of seawater were monitored using a multiparameter (YSI proplus, United States) to ensure no substantial change during the experiment. The sand (particle size: 0.25–0.5 mm) with a thickness of 3 cm was used as the habitat substrate for spat. The spat was generally fed three times a day with microalgae, and the amount of microalgae was adjusted according to the total individuals under experimental condition.
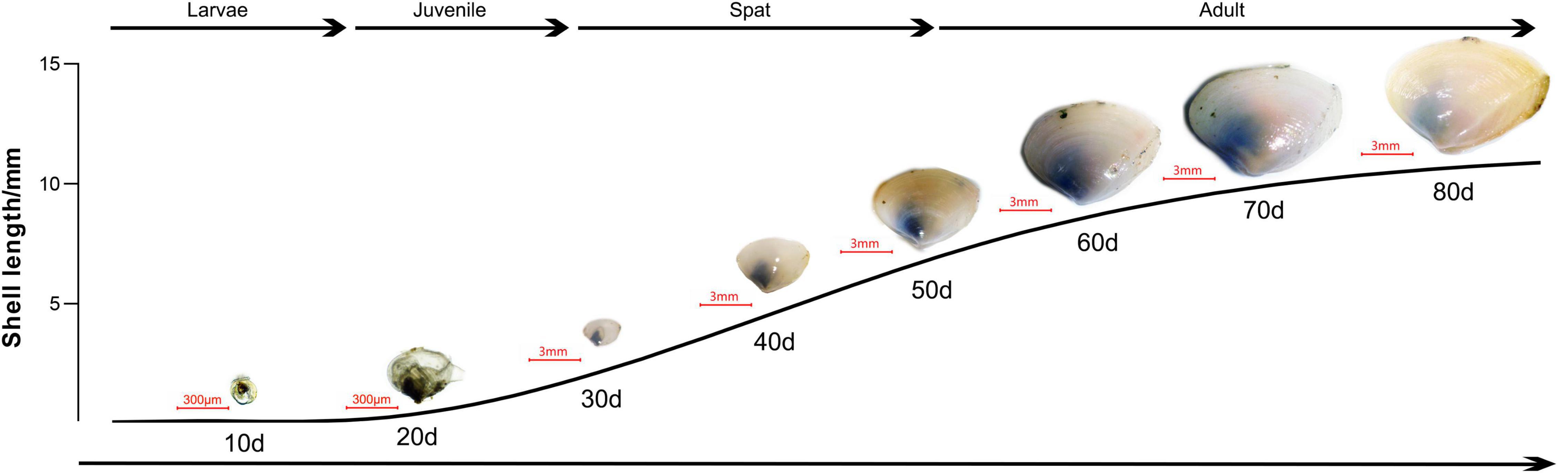
Figure 1. Main developmental stages of Mulinia lateralis, including larvae, juvenile, spat, and adult. Scale bar of 300 μm is used for the larvae and juvenile, while scale bar of 3 mm is used for spat and adult.
Microalgae Preparation
Five different microalgae species were selected in this study, including three species of Chlorophyta, namely, C. pyrenoidesa (Cp), Platymonas helgolandica (Ph), and Dunaliella salina (Ds), and two species of Bacillariophyta, namely, Nitzschia closterium (Nc) and Chaetoceros muelleri (Cm), which were commonly used for bivalve culture (Yu and Li, 2016). The characteristics of microalgae species are described in Table 1. Microalgae concentrate was purchased from Jianyang Biological Technology Ltd. (Dalian, China), which was stored at 4°C. The concentrate was diluted with FSW, and then the suspension was filtered through a 40 μm mesh screen to remove any clumps. Cell concentration of microalgae was then calculated using a cell counter (Countstar IC1000, China), and the feeding concentration was adjusted according to the experimental design.
Experimental Design
Four experiments were conducted to study the effects of microalgae diet, temperature, and salinity on the growth and survival of M. lateralis spat. The first experiment examined the filtration of different microalgae species by M. lateralis spat. The second experiment evaluated the effects of different microalgae species on the growth and survival of spat. Third, an interactive experiment was designed to explore the effects of microalgae concentration and stocking density. Finally, the effects of temperature and salinity on the growth and survival of spat were assessed, respectively. Details of each experiment were described in the following sections.
Experiment 1: Filtration of Five Microalgae Species by Mulinia Lateralis Spat
The experiment was conducted in a 1-L aquarium (10 cm × 10 cm × 10 cm) to determine the filtration rate (FR) of each microalgae species by M. lateralis spat (Supplementary Figure 1). The 30-day-old (shell length: 2.89 ± 0.09 mm) and 40-day-old (shell length: 5.84 ± 0.09) spat were collected, respectively, and washed gently with FSW. The spat were starved for 12 h before the experiment and then were randomly assigned to five aquaria filled with FSW at the temperature of 21–22°C and salinity of 27–28 ppt. There were 10 individuals in each aquarium, which were fed with one microalgae species with a concentration of 5 × 105 cells ml–1. During the experiment, adequate aeration of the seawater provided a saturated oxygen concentration and a continuous mixture of microalgae. In addition, an aquarium added with microalgae species but without spat was set as the control group to eliminate the influence of microalgae sedimentation. At 0 and 6 h after feeding, the 3 ml water samples were taken from each aquarium using the three-point sampling method, and microalgae were counted using a cell counter. Each trial was carried out in triplicates (3 aquaria). FR was calculated using the following formula (Sprung, 1984):
Where v is the volume of seawater (ml), n is the number of spat (individual), t is the duration of the experiment (h), C0 is the initial microalgae concentration (cells ml–1), C6 is the final initial microalgae concentration (cells ml–1), S is the correction factor using the control, Cc0 is the initial microalgae concentration in the control (cells ml–1), and Cc6 is the final microalgae concentration in the control (cells ml–1).
Experiment 2: Effects of Microalgae Diet on the Growth and Survival of Spat
The 30-day-old spat (shell length: 1.89 ± 0.30 mm) was collected for the diet experiment. According to microalgae species, seven trials were set for feeding experiment, including four Chlorophyta trials (Cp, Ph, Ds, and Cp + Ph + Ds) and three Bacillariophyta (Nc, Cm, and Nc + Cm). Different microalgae species were mixed at a 1:1 or 1:1:1 ratio based on the cell density. Each trial consisted of three replicates and was carried out in a 15-L aquarium (30 cm × 25 cm × 20 cm) filled with FSW, adequate aeration, 3 cm thickness substrate, a temperature of 21–22°C, and salinity of 27–28 ppt. Each aquarium contained 35 individuals. Spat was cultured in adequately aerated seawater, and seawater was renewed every 2 days. Aquaria were replaced every 10 days, which were sterilized with 0.2% potassium permanganate solution before use. All trials were fed three times a day with microalgae at a concentration of 5 × 104 cells ml–1. The number of 50-day-old spat was counted, and survival was assessed by survival percentage (%) = (the number of 50-day-old spat/35) × 100%. The spat was photographed under a stereoscope (Cnoptec SZ680, China), and 30 individuals in each microalgae trial were randomly selected to measure the shell length using ImageView software (Motic, China). Growth was calculated as the average growth rate (mm day–1) = (shell length at 50-day-old spat − shell length at 30-day-old spat)/20 day.
According to the abovementioned results, the microalgae species with the best feeding performance were selected from the Chlorophyta and Bacillariophyta, respectively. The 30-day-old spat (shell length: 1.96 ± 0.38 mm) was collected to explore the different mixing ratios of microalgae. The trials included Cm, Cm3 + Cp1, Cm1 + Cp1, Cm1 + Cp3, and Cp, and different microalgae species were mixed based on cell density. Other culture conditions and methodology for measuring the growth and survival of spat were identical to those described in the above experiment.
Experiment 3: Effects of Microalgae Concentration and Stocking Density on the Growth and Survival of Spat
The 30-day-old spat (shell length: 2.18 ± 0.29 mm) and 40-day-old spat (shell length: 4.32 ± 0.27 mm) were separately collected. The experiment for 30–40-day-old spat contained 12 trials: all combinations of three diet concentrations (1 × 104, 5 × 104, and 1 × 105 cells ml–1) and four different stocking densities (400, 600, 800, and 1,000 individuals m–2), respectively. In the experiment of 40–50-day-old spat, 12 trials were conducted: all combinations of three diet concentration levels (5 × 104, 1 × 105, and 20 × 104 cells ml–1) and four different stocking densities (400, 600, 800, and 1,000 individuals m–2). There were three replicates per trial (3 aquaria). During the whole experiment, Cp was selected as the only microalgae diet for spat. In the experiment of 30- and 40-day-old spat, survival percentage (%) = (the final number of spat/the initial number of spat in each aquarium) × 100%, and the average growth rate (mm day–1) = (the final shell length − the initial shell length)/10 days. Other experimental conditions and methodology were similar to Experiment 2.
Experiment 4: Effects of Temperature and Salinity on the Growth and Survival of Spat
Depending on the temperature of the natural distribution area,1 five experimental gradients of 18, 20, 22, 24, and 26°C were set up. The 30-day-old spat (shell length: 2.62 ± 0.31 mm) was collected for the experiment. Each trial consisted of three replicates, each make up of a 15-L aquarium filled with FSW, the salinity of 27–28 ppt, and each aquarium contained 35 individuals. For the temperature trial, a thermostatic electronic heater (BOYU HT2200, China) was equipped to maintain a steady temperature, respectively, while the 18°C trial aquarium was placed directly at room temperature controlled by an air conditioner (Green KFR-72LW, China). The seawater was preheated to the corresponding experimental temperature before use. Cp was selected as a microalgae diet for spat, and the supply of diet varied according to experiment 3. Other experimental conditions and methodology for measuring the survival and growth of spat were the same as in experiment 2.
Based on the salinity of the natural distribution area (see text footnote 1), seven experimental gradients of 16, 19, 22, 25, 28, 31, and 34 ppt were designed to evaluate the growth and survival of spat under laboratory conditions. The 30-day-old spat (shell length: 2.85 ± 0.44 mm) was collected for the experiment, each aquarium was filled with FSW, and the temperature was regulated at 21–22°C. The experimental seawater of different salinities was prepared by mixing the freshwater, FSW, and sea crystal (HAIBAWANG, China). Other experimental conditions and measurements were identical to those of the temperature experiment.
Data Analysis
The ingestion rate, growth rate, and survival percentage of spat were presented as the mean ± standard deviation (SD). All data were expressed in a chart format by the GraphPad8.0 software. Data analysis and curve fitting were conducted using the SPSS23 software. The analysis of variance (ANOVA) followed by post hoc comparison of means based on Tukey’s test was conducted to determine significant differences (p < 0.05). The normality of data was confirmed by Kolmogorov-Smirnov’s test and homogeneity of variances by Levene’s test. Non-linear regression analysis was carried out to evaluate the effects of temperature and salinity in our experiment.
Results
Ingestion of Microalgae by Mulinia lateralis Spat
All microalgae used in this study could be ingested by M. lateralis spat, as shown in Figure 2. For 30-day-old spat, the FRs of Cp [5.66 ± 0.89 × 105 cells (ind × h)–1], Nc [5.65 ± 1.23 × 105 cells (ind × h)–1], and Cm [4.30 ± 1.82 × 105 cells (ind × h)–1] were higher than Ds [1.47 ± 0.31 × 105 cells (ind × h)–1] and Ph [0.85 ± 0.23 × 105 cells (ind × h)–1], respectively. The ANOVA test showed that there was a significant difference among different trials (F = 9.123, p < 0.01, Supplementary Table 1). For 40-day-old spat, the FRs of different microalgae ranging from high to low were Cp [19.56 ± 4.11 × 105 cells (ind × h)–1], Nc [9.32 ± 4.30 × 105 cells (ind × h)–1], Cm [8.67 ± 2.1 × 105 cells (ind × h)–1], Ds [6.94 ± 1.04 × 105 cells (ind × h)–1], and Ph [2.25 ± 0.27 × 105 cells (ind × h)–1]. The ANOVA test was also highly significant (F = 9.809, p < 0.01, Supplementary Table 1). In summary, the spat had high FR of Cp, Nc, and Cm, and the FR of microalgae increased as M. lateralis spat grew.
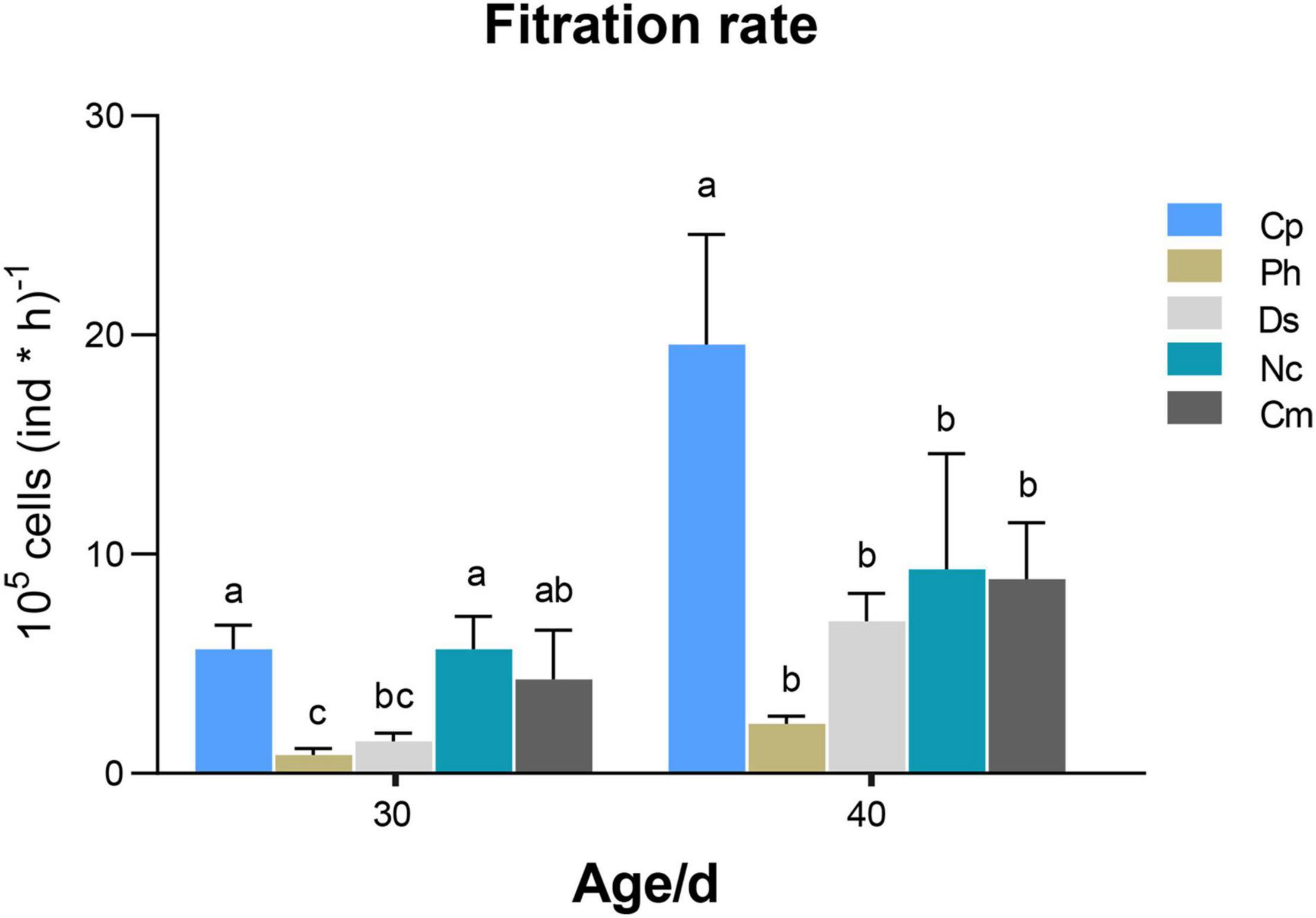
Figure 2. Filtration rate (FR) of M. lateralis spat fed by different microalgae diets at different ages, different letters represent significant differences between different trials (p < 0.05). Cp, Chlorella pyrenoidesa; Ph, Platymonas helgolandica; Ds, Dunaliella salina; Nc, Nitzschia closterium; Cm, Chaetoceros muelleri.
Effects of Microalgae Species on the Growth and Survival of Mulinia lateralis Spat
Both single species and mixed microalgae diets were used to feed M. lateralis spat aiming to observe their effects on survival and growth (Figure 3A). Among the diet trials, the survival percentage of spat fed with different diets ranged from 94.29 ± 2.33% to 99.05 ± 1.35%, and there was no significant difference (F = 1.769, p > 0.05, Supplementary Table 2). Meanwhile, spat fed with Cp showed the highest average growth rate among these trials (Figure 4), and the ANOVA test showed a significant difference (F = 46.574, p < 0.01, Supplementary Table 2). In the single-species diet experiment, the growth rates of spat-fed different species of Chlorophyta ranging from high to low were 0.266 ± 0.038 mm day–1 (Cp), 0.179 ± 0.034 mm day–1 (Ph), and 0.159 ± 0.029 mm day–1 (Ds), while the growth rates of spat-fed Bacillariophyta were 0.166 ± 0.035 mm day–1 (Cm) and 0.152 ± 0.023 mm day–1 (Nc), respectively. In the mixed diet experiment, spat fed with a mixture of three Chlorophyta (Cp + Ph + Ds) had a growth rate of 0.232 ± 0.030 mm day–1, while spat fed with a mixture of two Bacillariophyta (Cm + Nc) had a growth rate of 0.155 ± 0.029 mm day–1. In addition, the growth rate of spat increased significantly with the increase in the proportion of Cp (F = 25.538, p < 0.01, Supplementary Table 3), while the survival percentage was almost not affected (Figure 3B).
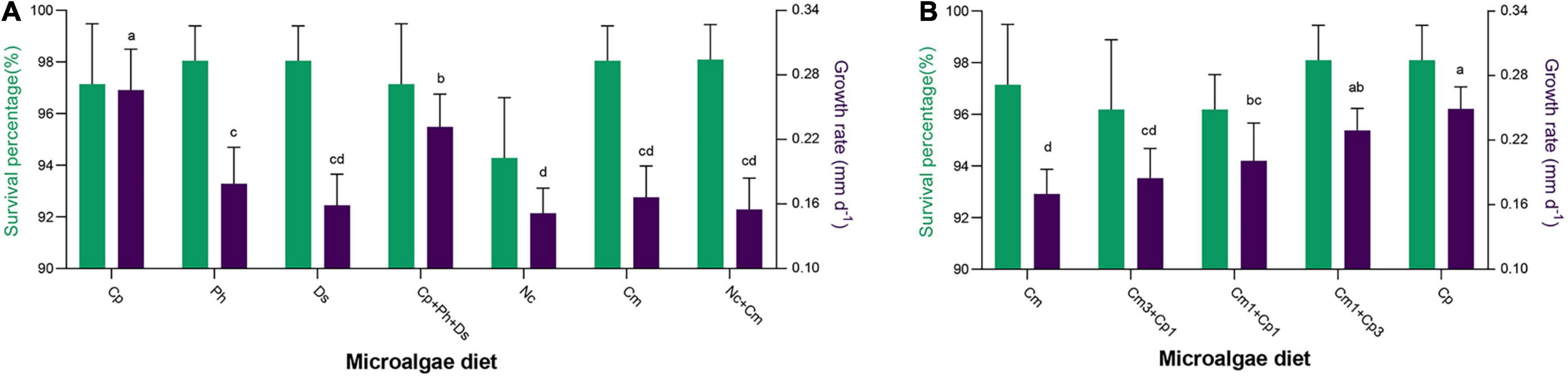
Figure 3. Effects of different microalgae species (A) and ratios (B) on the survival and growth of M. lateralis spat, different letters represent significant differences between different trials (p < 0.05). The green part indicates the survival percentage; the purple part indicates the growth rate.
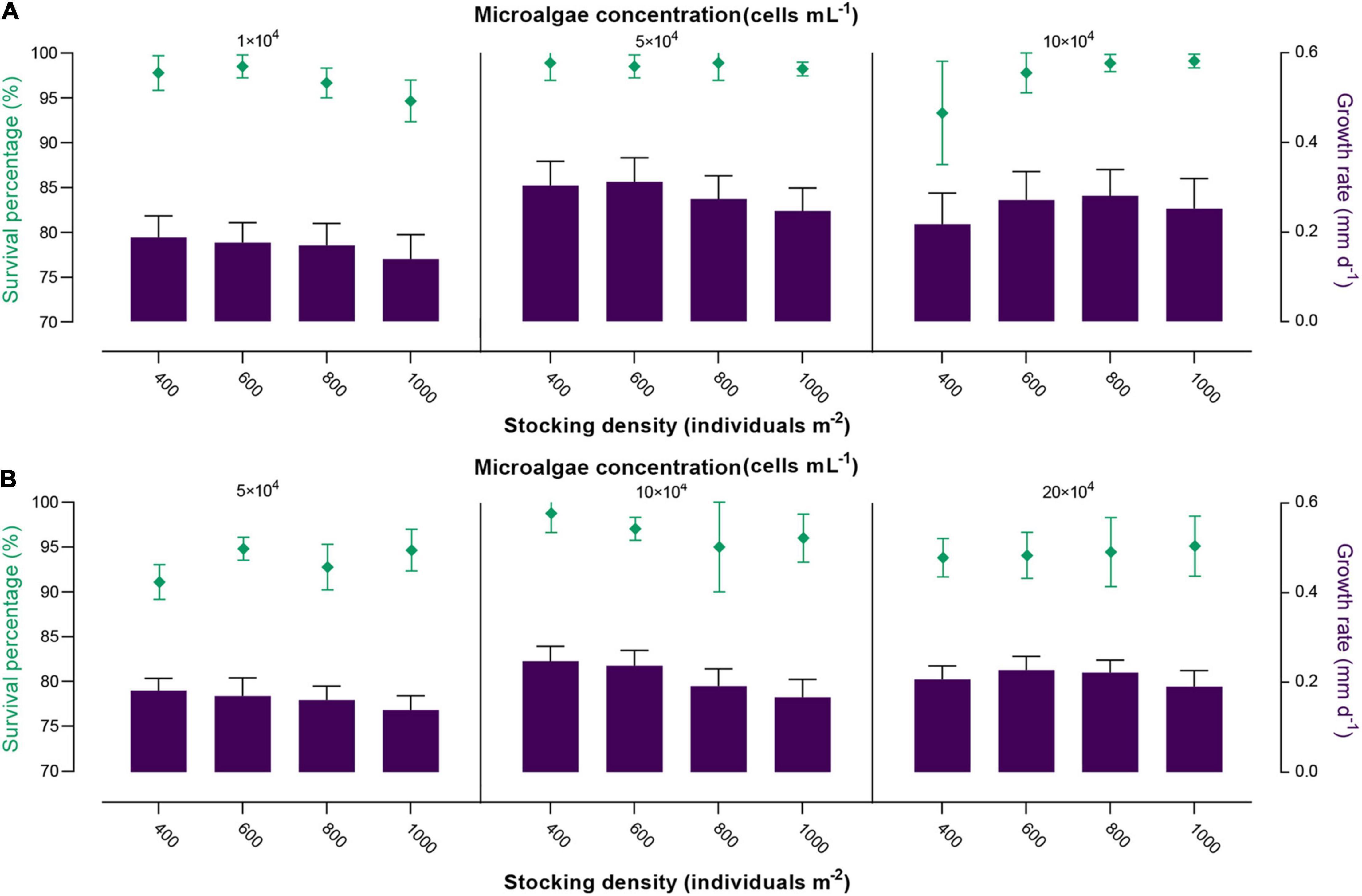
Figure 4. Effects of different microalgae concentration and stocking density on the survival and growth of 30–40-day-old spat (A) and 40–50-day-old spat (B) of M. lateralis. The green part indicates the survival percentage; the purple part indicates the growth rate.
Effects of Microalgae Concentration and Stocking Density on the Growth and Survival of Mulinia lateralis Spat
In 30–40-day-old experiment (Figure 4A), the survival percentage of spat was ≥ 93.33 ± 4.71%, and the growth rate varied between 0.140 ± 0.054 mm day–1 and 0.312 ± 0.052 mm day–1, among these trials. Two-way ANOVA showed that there was no significant effect of microalgae concentration and stocking density on the survival of spat, and there was no interaction between them (p > 0.05, Table 2). In contrast, these two factors significantly affected the growth rate of spat throughout the experiment, and interaction was detected between them (p < 0.01, Table 2). The maximum growth rate was 0.312 ± 0.052 mm day–1 at microalgae concentration of 5 × 104 cells ml–1 and a stocking density of 600 individuals m–2, followed by 5 × 104 cells ml–1 × 400 individuals m–2 (0.304 ± 0.053 mm d–1). In 40–50-day-old experiment (Figure 4B), the survival percentage of spat varied between 91.11 ± 1.57% and 98.77 ± 1.75%. Meanwhile, the effect of microalgae concentration on survival was significant (p < 0.05, Table 2), and higher survival percentages were observed at a microalgae concentration of 1 × 105 cells ml–1. The spat growth in this period varied significantly with microalgae concentration, stocking density, and the interaction between them (p < 0.01, Table 2). High growth rates were found in two trials: 1 × 105 cells ml–1 × 400 individuals m–2 (0.247 ± 0.033 mm day–1) and 1 × 105 cells ml–1 × 600 individuals m–2 (0.237 ± 0.033 mm day–1). According to the survival and growth, the optimal culture condition for 30–40-day-old spat was 5 × 104 cells ml–1 × 400–600 individuals m–2, and for 40–50-day-old spat 1 × 105 cells ml–1 × 400–600 individuals m–2.
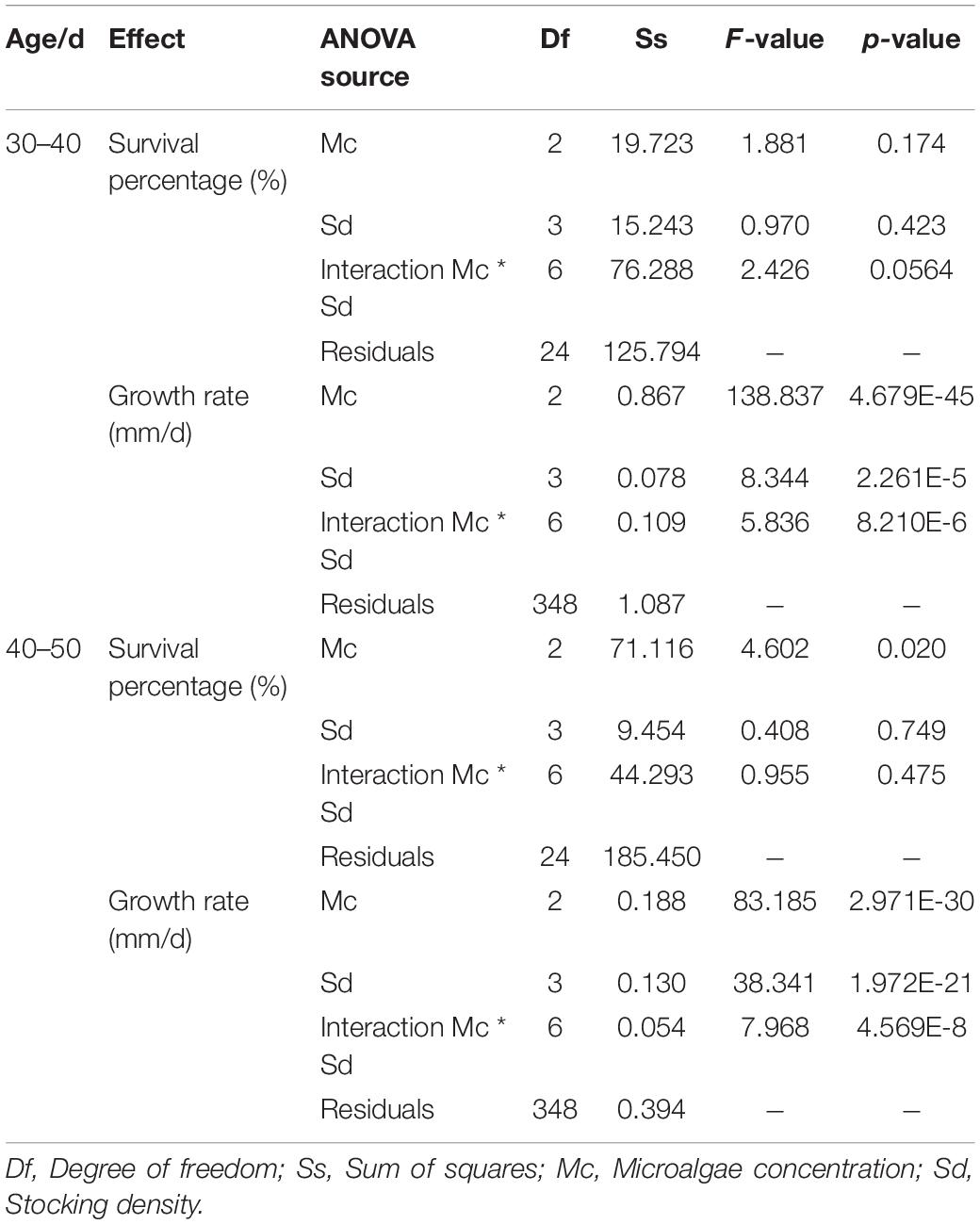
Table 2. Two-way ANOVA table testing the effects of microalgae concentration and stocking density on the survival and growth of Mulinia lateralis spat.
Effects of Temperature and Salinity on the Growth and Survival of Mulinia lateralis Spat
During the spat culture, the relationship between spat survival and temperature was cubic (R2 = 0.993, p = 0.007), with survival percentage varying from 61.90 ± 4.86% to 99.05 ± 1.35% (Figure 5A). Spat survival gradually decreased with increasing temperature, especially at a temperature over 22°C. The ANOVA test showed that there was a significant difference among different trials (p < 0.01, Supplementary Table 4). With the increase in temperature, the growth of spat increased at first and then decreased, and spat at 20–24°C showed high average growth rates (0.230 ± 0.041–0.253 ± 0.046 mm day–1). As shown in Figure 5B, a quadratic relationship was found between the survival percentage and salinity (R2 = 0.863, p = 0.019), and the spat had high survival percentages (93.33 ± 3.56–97.14 ± 2.33%), especially at a salinity of 22–25 ppt. At the same time, the spat had high growth rates (0.211 ± 0.045–0.232 ± 0.048 mm day–1) at a salinity of 19–28 ppt. The ANOVA test indicated that the salinity significantly affected the survival and growth of spat (p < 0.05, Supplementary Table 5). As a result, the better conditions for spat culture were at the temperature of 20–22°C and the salinity of 22–25 ppt.
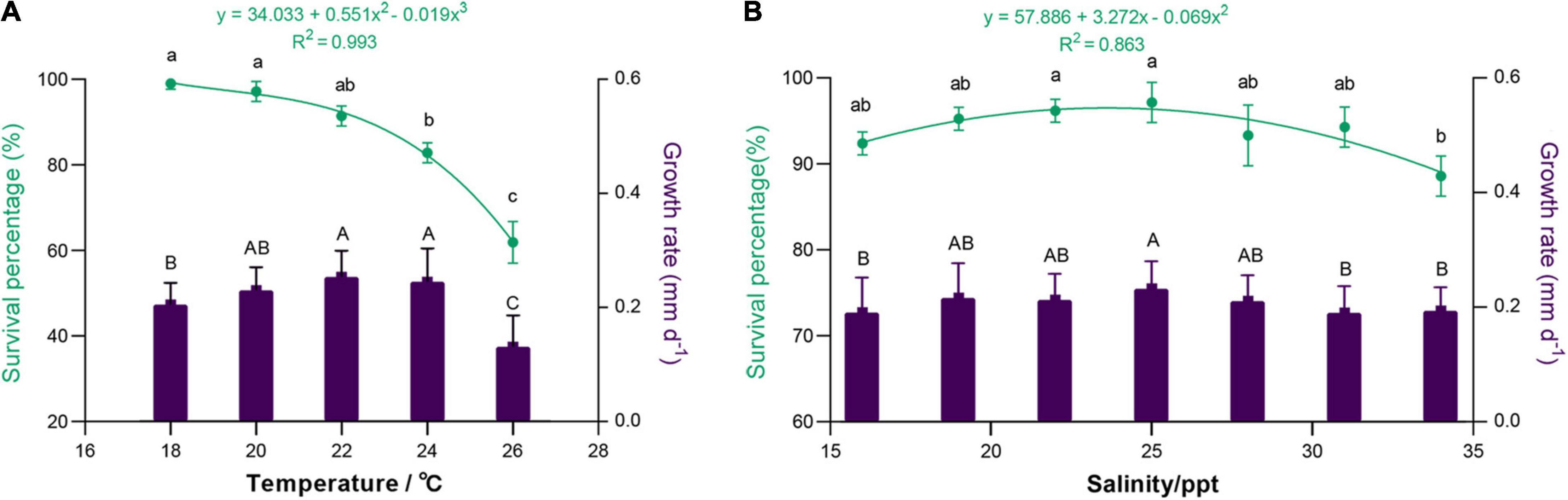
Figure 5. Survival and growth of M. lateralis spat cultured at different temperatures (A) and salinities (B). The green part indicates the survival percentage; the purple part indicates the growth rate. Different letters represent significant differences between different trials (p < 0.05).
Discussion
In M. lateralis, all five microalgae species could be ingested by spat, but the FRs on different microalgae showed significant differences. For filter-feeding bivalves, FR is an important indicator to evaluate their feeding ability (Maquirang et al., 2020). The cell size and form of microalgae influence the filtration of microalgae in the bivalve (Cheng et al., 2020). For example, Wang et al. (2015) found that Platymonas subcordiformis was easier to sink due to its large cell size, which affects the feeding of Lutraria sieboldii juvenile. The feeding of Crassostrea hongkongensis on three microalgae showed that the long flagella of C. muelleri could damage the gill of C. hongkongensis during feeding, leading to a reduction in the palatability of microalgae (Fu et al., 2017). In this study, the cells of Cp, Nc, and Cm are relatively small, while Ds and Ph are larger. Moreover, except Ds, other microalgae have fibrous or siliceous cell walls, for example, Nc has a crescent form while Cm has four longer setae. This may explain the high FRs of M. lateralis spat on Cp, Nc, and Cm and the relatively low FRs on Ds and Ph.
Microalgae are the main food source for bivalves, as they could provide abundant nutrition for the whole life cycle (Brown et al., 1997). In this study, there are significant differences in the growth of spat fed by different microalgae, and the spat fed by Cp had the highest growth rate (0.266 ± 0.038 mm day–1). These results may be due to differences in the nutrient content of microalgae diets. The microalgae Cp used in the study is a species of Chlorella, which has the highest protein content (>50%) among the microalgae species used in this study, and is also rich in essential amino acids, pigments, and other biomolecules, especially the Chlorella growth factor and chlorellin (Wang and Zhang, 2012; Ahmad et al., 2020). Many studies have shown that the presence of features, such as GCF, chlorellin, and strong antioxidant activity, produces improvements in growth performance, feed utilization, survival, disease resistance, inhibition of bacterial quorum sensing, immunity, stress tolerance, gut microbiota, and aquatic bioremediation (An et al., 2016; Ahmad et al., 2020). For example in Pinctada fucata martensii, Yang et al. (2017) reported that Chlorella sp. improved the growth performance, immunity and antioxidant, and biomineralization capacity of the juvenile by measuring the growth rate, digestive enzyme activities, antioxidant capacities, and expression levels of immune- and antioxidant-related genes of juvenile fed with different diets. A similar situation also occurred in Hyriopsis cumingii, i.e., the growth of mussel fed with C. pyrenoidosa was higher than that of those fed with Monoraphidium contortum (Chen et al., 2021). However, some studies also reported vibrant nutritional effects of Chlorella at varying supplementation levels in different species, including favorable and unfavorable effects of Chlorella diet (Ahmad et al., 2020). Therefore, for the culture of each bivalve species, it is vital to determine the suitable microalgae diet.
Previous studies have suggested that, in most cases, it is better to feed mixed microalgae rather than a single species, as they may provide more balanced nutrition (Cheng et al., 2020). At the larvae stage of M. lateralis, the mixed diet of Isochrysis galbana and Platymonas helgolandica as diet significantly improved the larval survival and growth (Yang et al., 2021). Similar studies have been reported in many species, such as Pinna nobilis (Prado et al., 2020) and M. mercenaria (Hassan et al., 2021). However, M. lateralis spat fed a mixed diet grew poorly or had no obvious advantages compared with the single microalgae Cp. Some researchers reported that a mixture of microalgae has positive, negative, or intermediate effects, depending on the type of microalgae selected (Velasco, 2007; Cheng et al., 2020). In other words, the addition of other microalgae species reduces the proportion of preferred species in the culture water, resulting in less absorption of species that meet the nutrition requirement for bivalves. In this study, the performance of spat feeding on mixed microalgae was not as good as that of feeding on the single microalgae Cp. If there are some other suitable microalgae, the effects of mixed diet are worthy of further research to support the culture of M. lateralis.
Food availability is an important factor affecting the bivalve, especially the concentration of microalgae is directly related to the feeding process (Ballesta-Artero et al., 2018; Militz et al., 2021). Numerous studies have proven that the growth and survival of bivalves will be reduced if they take too much or too little microalgae, such as researches in P. margaritifera (Linard et al., 2011), M. edulis (Thomsen et al., 2013), and M. galloprovincialis (Weston et al., 2021). Stocking density is another important factor affecting food availability, and density-dependent growth and survival have been reported for many bivalves under culture conditions (Liu et al., 2010; Nowland et al., 2019). In P. martensii, the interaction between microalgae concentration and stocking density was evaluated, and the optimal culture conditions of 50.85 cells μl–1 and 1.6 ind ml–1 were obtained to maximize the growth and survival (Wang et al., 2021). Our study indicated that these two factors significantly affected the growth and survival of spat, and their interaction constituted significant portions in the variability of growth for spat. The combined analysis of two factors showed that the optimal culture condition for 30–40-day-old spat was 5 × 104 cells ml–1 × 400–600 individuals m–2, and for 40–50-day-old spat 1 × 105 cells ml–1 × 400–600 individuals m–2. To rule out the possibility of food availability as a limited factor, bivalves reared at higher densities necessarily require higher food input to maintain optimal growth. However, high density will increase the competition of bivalve for food and space and generate more metabolic wastes to pollute the water quality, especially the static rearing system, which ultimately affect the bivalve performance (Raghavan and Gopinathan, 2008; Velasco and Barros, 2008). Therefore, balancing the relationship between microalgae concentration and stocking density is vital to sustainable culture of bivalve. These results will provide the basis for optimizing the artificial culture of M. lateralis.
As bivalves are ectotherms, the temperature is a key factor affecting their growth, development, and survival, which was proved in Callista chione (Purroy et al., 2018), Arctica islandica (Ballesta-Artero et al., 2018), and Crassostrea gigas (Trigg et al., 2020). For bivalves, the increase in temperature has a significantly positive effect within a certain range, which is mainly related to the metabolic activities of bivalves (Matoo et al., 2013; Freitas et al., 2019; Prado et al., 2020). On the contrary, when the temperature exceeds this range, the activity of enzymes required for various physiological and biochemical processes will be reduced, thus inhibiting the normal growth of bivalves. Therefore, it is essential to determine the appropriate temperature range of the bivalve culture. Our results are consistent with these researches, with the increase in temperature, the growth and survival of spat increased first and then decreased, and spat had the higher performance at the temperature of 20–22°C. In a previous study, Calabrese (1970b) reported that the optimal temperature was 25 ± 1°C for embryo and 27.5°C for D-shaped larva, which was somewhat different from our study. The reason may be that M. lateralis used in Calabrese (1970b) was from the wild populations, which had better adaptability to the environment than the long-term cultured populations in our experiment. Another reason is the differences in subjects and duration. The study of Calabrese focused on the larval development lasting for 48 h, while our experiment continued to track the culture of spat for 30 days.
Salinity is another important environmental factor in the artificial culture of bivalves (Gosling, 2015). In this study, salinity produced apparent effects on the growth and survival of M. lateralis spat. This is probably because the osmolarity changes caused by salinity exceed the ability of spat to regulate itself (Berger and Kharazova, 1997). In the bivalve, intracellular osmolarity is maintained by regulating ion and amino acid concentrations, and this process incurs considerable energy costs (Hawkins and Hilbish, 1992). In M. galloprovincialis (Freitas et al., 2019) and Ruditapes philippinarum (Velez et al., 2016), excessive energy consumption during osmotic pressure regulation produced negative effects on multiple processes, such as metabolism, redox, and immunity, which is a cause of mortality. In general, M. lateralis spat possesses a wide salinity range of 19–28 ppt, which is similar to other burrowing bivalves, such as Tegillarca granosa (16.5–30 ppt), Meretrix meretrix (9.2–29.3 ppt), and R. philippinarum (23.0–35.5 ppt) (Bao and You, 2004). This may be related to the living environment of burrowing bivalves, which mainly inhabit near estuarine benthic and intertidal areas with large salinity fluctuation. Furthermore, burrowing bivalves have evolved various behaviors in response to the changes in salinity, such as retracting deeper into sediments or quickly closing their shells (Sokolova et al., 2000; Haider et al., 2017).
The spat is a key stage in the transition from the juvenile to the adult stage. Providing the most suitable culture conditions can promote the successful cultivation of high-quality spat to a greater extent and lay a solid foundation for further maturation and reproduction of M. lateralis. The results of this study provide valuable new information on the choice of microalgae diet, temperature, and salinity. For applicability, the optimal conditions determined in this study were validated in our laboratory and became an important part of the standard culture protocol of M. lateralis. Of course, other aspects need to be further optimized in future research to develop a culture system for M. lateralis.
Conclusion
This study highlights the complex influences of three factors, namely, microalgae diet, temperature, and salinity on the ingestion, growth, and survival of the dwarf surf clam M. lateralis spat. Through simultaneously maximizing the growth and survival, the optimal culture conditions for M. lateralis spat, including microalgae species, microalgae concentration, stocking density, temperature, and salinity, were attained. The results from this study have accomplished a necessary foundation for M. lateralis spat culture, which would greatly promote the establishment of a standard culture system of M. lateralis.
Data Availability Statement
The original contributions presented in the study are included in the article/Supplementary Material, further inquiries can be directed to the corresponding author/s.
Author Contributions
ZY, XH, and ZB conceived and designed the experiments. ZY, HW, ML, MT, XW, and AZ performed the experiments. ZY and XH analyzed the data and drafted the manuscript. ZY, XH, JH, and ZB contributed to writing, review, and editing. All authors contributed to the article, revised the final manuscript and approved the submitted version.
Funding
This study was supported by the grants of the Chinese Ministry of Science and Technology through the National Key Research and Development Program of China (2018YFD0901402), the Major Basic Research Projects of Shandong Natural Science Foundation (ZR2018ZA0748), the China Agriculture Research System of MOF and MARA, and Earmarked Fund for Agriculture Seed Improvement Project of Shandong Province (2020LZGC016).
Conflict of Interest
The authors declare that the research was conducted in the absence of any commercial or financial relationships that could be construed as a potential conflict of interest.
Publisher’s Note
All claims expressed in this article are solely those of the authors and do not necessarily represent those of their affiliated organizations, or those of the publisher, the editors and the reviewers. Any product that may be evaluated in this article, or claim that may be made by its manufacturer, is not guaranteed or endorsed by the publisher.
Supplementary Material
The Supplementary Material for this article can be found online at: https://www.frontiersin.org/articles/10.3389/fmars.2021.823112/full#supplementary-material
Supplementary Figure 1 | Experimental design used to test the effects of different microalgae diet on the ingestion rates of M. lateralis spat at different ages.
Supplementary Figure 2 | Morphological observation of microalgae concentrates under microscope.
Footnotes
References
Acosta, E. J., Rodríguez-Forero, A., Werding, B., and Kunzmann, A. (2021). Effect of density, temperature and diet on the growth, survival and development of larvae and juveniles of Isostichopus sp. Aquac. Res. 52, 611–624. doi: 10.1111/are.14918
Ahmad, M. T., Shariff, M., Yusoff, F. M. D., Goh, Y. M., and Banerjee, S. (2020). Applications of microalga Chlorella vulgaris in aquaculture. Rev. Aquacult. 12, 328–346. doi: 10.1111/raq.12320
An, B. K., Kim, K. E., Jeon, J. Y., and Lee, K. W. (2016). Effect of dried Chlorella vulgaris and Chlorella growth factor on growth performance, meat qualities and humoral immune responses in broiler chickens. Springerplus 5:718. doi: 10.1186/s40064-016-2373-4
Avila, C., Grenier, S., Tamse, C., and Kuzirian, A. (1997). Biological factors affecting larval growth in the nudibranch Mollusc Hermissenda crassicornis (Eschscholtz, 1831). J. Exp. Mar. Biol. Ecol. 218, 243–262. doi: 10.1016/S0022-0981(97)00077-4
Ballesta-Artero, I., Janssen, R., van der Meer, J., and Witbaard, R. (2018). Interactive effects of temperature and food availability on the growth of Arctica islandica (Bivalvia) juveniles. Mar. Environ. Res. 133, 67–77. doi: 10.1016/j.marenvres.2017.12.004
Bao, Y., and You, Z. (2004). Influences of several environmental factors on growth in marine shellfish larvae. Fish. Sci. 23, 39–41.
Berger, V. J., and Kharazova, A. D. (1997). Mechanisms of salinity adaptations in marine molluscs. Hydrobiologia 355, 115–126. doi: 10.1007/978-94-017-1907-0_12
Brown, M., Jeffrey, S., Volkman, J., and Dunstan, G. (1997). Nutritional properties of microalgae for mariculture. Aquaculture 151, 315–331. doi: 10.1016/S0044-8486(96)01501-3
Burgess, R. M., and Morrison, G. E. (2010). A short-exposure, sublethal, sediment toxicity test using the marine bivalve Mulinia lateralis: statistical design and comparative sensitivity. Environ. Toxicol. Chem. 13, 571–580. doi: 10.1002/etc.5620130405
Calabrese, A. (1969a). “Mulinia lateralis: Molluscan fruit fly?,” in Proceedings of the National Shellfisheries Association, (Waldoboro: National shellfisheries association), 65–66.
Calabrese, A. (1969b). Individual and combined effects of salinity and temperature on embryos and larvae of the coot clam, Mulinia lateralis (Say). Biol. Bull. US. 137, 417–428. doi: 10.2307/1540164
Calabrese, A. (1970a). The Early Life History and Larval Ecology of the Coot Clam, Mulinia lateralis (Say) (Mactridae:Pelecypoda). Ph.D thesis, Connecticut: The university of Connecticut.
Calabrese, A. (1970b). Reproductive cycle of the coot clam, Mulinia lateralis (Say), in Long Island Sound. Veliger 12, 265–269.
Chen, Q., Jiang, X., Han, Q., Sheng, P., Chai, Y., Peng, R., et al. (2021). Growth, calcium content, proximate composition, and fatty acid composition of triangle sail mussel (Hyriopsis cumingii) fed five different microalgal diets. Aquaculture 530:735719. doi: 10.1016/j.aquaculture.2020.735719
Cheng, P., Zhou, C., Chu, R., Chang, T., Xu, J., Ruan, R., et al. (2020). Effect of microalgae diet and culture system on the rearing of bivalve mollusks: nutritional properties and potential cost improvements. Algal. Res. 51:102076. doi: 10.1016/j.algal.2020.102076
Cripe, G. M. (2010). Contaminated sediment testing with the bivalve Mulinia lateralis: culture refinement for organism availability. Environ. Toxicol. Chem. 25:1332–1336. doi: 10.1897/05-271R.1
Doroudi, M., and Southgate, P. (2000). The influence of algal ration and larval density on growth and survival of blacklip pearl oyster Pinctada margaritifera (L.) larvae. Aquac. Res. 31, 621–626. doi: 10.1046/j.1365-2109.2000.318483.x
Freitas, R., Leite, C., Pinto, J., Costa, M., Monteiro, R., Henriques, B., et al. (2019). The influence of temperature and salinity on the impacts of lead in Mytilus galloprovincialis. Chemosphere 235, 403–412. doi: 10.1016/j.chemosphere.2019.05.221
Freitas, R., Silvestro, S., Coppola, F., Meucci, V., Battaglia, F., Intorre, L., et al. (2020). Combined effects of salinity changes and salicylic acid exposure in Mytilus galloprovincialis. Sci. Total Environ. 715:136804. doi: 10.1016/j.scitotenv.2020.136804
Fu, J., Lan, W., Li, T., Li, Q., Fan, H., and Huang, L. (2017). Research of clearance rate and filtration rate of Crassostrea hongkongensis feeding on three different phytoplankton. Haiyang Xuebao 39, 62–69. doi: 10.3969/j.issn.0253-4193.2018.08.008
Gomes, A., Lourenco, S., Santos, P. M., Raposo, A., Mendes, S., Goncalves, S. C., et al. (2021). Effects of single and mixed-diatom diets on growth, condition, and survival of larvae of the sea urchin Paracentrotus lividus (Lamarck, 1816). Aquacult. Int. 29, 1069–1090. doi: 10.1007/s10499-021-00676-8
Guo, X., and Allen, S. K. (1994). Sex determination and polyploid gigantism in the dwarf surfclam (Mulinia lateralis Say). Genetics 138, 1199–1206. doi: 10.1093/genetics/138.4.1199
Haider, F., Sokolov, E. P., and Sokolova, I. M. (2017). Effects of mechanical disturbance and salinity stress on bioenergetics and burrowing behavior of the soft shell clam Mya arenaria. J. Exp. Biol. 221:jeb172643. doi: 10.1242/jeb.172643
Hassan, M. M., Parks, V., and Laramore, S. (2021). Optimizing microalgae diets for hard clam, Mercenaria mercenaria, larvae culture. Aquacult. Rep. 20:100716. doi: 10.1016/j.aqrep.2021.100716
Hawkins, A., and Hilbish, T. (1992). The costs of cell volume regulation: protein metabolism during hyperosmotic adjustment. J. Mar. Biol. Assoc. U.K 72, 569–578. doi: 10.1017/S002531540005935X
Linard, C., Gueguen, Y., Moriceau, J., Soyez, C., Hui, B., Raoux, A., et al. (2011). Calcein staining of calcified structures in pearl oyster Pinctada margaritifera and the effect of food resource level on shell growth. Aquaculture 313, 149–155. doi: 10.1016/j.aquaculture.2011.01.008
Liu, W., Gurney-Smith, H., Beerens, A., and Pearce, C. (2010). Effects of stocking density, algal density, and temperature on growth and survival of larvae of the basket cockle, Clinocardium nuttallii. Aquaculture 299, 99–105. doi: 10.1016/j.aquaculture.2009.11.023
Maquirang, J. R. H., Pedroso, F. L., Apines-Amar, M. J., Piñosa, L. A. G., Rendaje, D. C., Cadangin, J. F., et al. (2020). Ingestion, digestion, growth and survival of green mussel Perna viridis pediveliger larvae fed different microalgae. Fisheries Sci. 86, 97–105. doi: 10.1007/s12562-019-01367-3
Marshall, R., McKinley, S., and Pearce, C. M. (2010). Effects of nutrition on larval growth and survival in bivalves. Rev. Aquacult. 2, 33–55. doi: 10.1111/j.1753-5131.2010.01022.x
Matoo, O. B., Ivanina, A. V., Ullstad, C., Beniash, E., and Sokolova, I. M. (2013). Interactive effects of elevated temperature and CO2 levels on metabolism and oxidative stress in two common marine bivalves (Crassostrea virginica and Mercenaria mercenaria). Comp. Biochem. Phys. A 164, 545–553. doi: 10.1016/j.cbpa.2012.12.025
Militz, T. A., Braley, R. D., and Southgate, P. C. (2021). Factors influencing the capacity for pediveliger larvae of the giant clam, Tridacna noae, to ingest and digest cells of microalgae concentrates. Aquaculture 533:736121. doi: 10.1016/j.aquaculture.2020.736121
Nowland, S. J., O’Connor, W. A., and Southgate, P. C. (2019). Optimizing stocking density and microalgae ration improves the growth potential of tropical black-lip oyster, Saccostrea echinata, larvae. J. World Aquacult. Soc. 50, 728–737. doi: 10.1111/jwas.12581
Prado, P., Cabanes, P., Catanese, G., Carella, F., Carrasco, N., Grau, A., et al. (2020). Growth of juvenile Pinna nobilis in captivity conditions: dietary and pathological constraints. Aquaculture 522:735167. doi: 10.1016/j.aquaculture.2020.735167
Purroy, A., Milano, S., Schöne, B. R., Thébault, J., and Peharda, M. (2018). Drivers of shell growth of the bivalve, Callista chione (L. 1758)-Combined environmental and biological factors. Mar. Environ. Res. 134, 138–149. doi: 10.1016/j.marenvres.2018.01.011
Raghavan, G., and Gopinathan, C. P. (2008). Effects of diet, stocking density and environmental factors on growth, survival and metamorphosis of clam, Paphia malabarica (Chemnitz) larvae. Aquac. Res. 39, 928–933. doi: 10.1111/j.1365-2109.2008.01951.x
Rhodes, E. W., Calabrese, A., Cable, W. D., and Landers, W. S. (1975). The development of methods for rearing the coot clam, Mulinia lateralis, and three species of coastal bivalves in the laboratory in Culture of Marine Invertebrate Animals. (Eds) L. S, Walter, and H. C, Matoira. (Berlin: Springer US), 273–282. doi: 10.1007/978-1-4615-8714-9_18
Shumway, S. E. (1983). Factors affecting oxygen consumption in the coot clam Mulinia lateralis (Say). Ophelia 22, 143–171. doi: 10.1080/00785326.1983.10426592
Shumway, S. E., and Newell, R. C. (1984). Energy resource allocation in Mulinia lateralis (Say), an opportunistic bivalve from shallow water sediments. Ophelia 23, 101–118. doi: 10.1080/00785326.1984.10426607
Shumway, S. E., Scott, T. M., and Shick, M. J. (1983). The effects of anoxia and hydrogen sulphide on survival, activity and metabolic rate in the coot clam, Mulinia lateralis (Say). J. Exp. Mar. Biol. Ecol. 71, 135–146. doi: 10.1016/0022-0981(93)90069-Z
Sokolova, I. M., Bock, C., and Pörtner, H. O. (2000). Resistance to freshwater exposure in White Sea Littorina spp. I: anaerobic metabolism and energetics. J. Comp. Physiol. B. 170, 91–103. doi: 10.1007/s003600050264
Sprung, M. (1984). Physiological energetics of mussel larvae (Mytilus edulis). I. Shell growth and biomass. Marine ecology progress series. Oldendorf 17, 283–293.
Thomsen, J., Casties, I., Pansch, C., Körtzinger, A., and Melzner, F. (2013). Food availability outweighs ocean acidification effects in juvenile Mytilus edulis: laboratory and field experiments. Global Change Biol. 19, 1017–1027. doi: 10.1111/gcb.12109
Trigg, S. A., Mitchell, K. R., Thompson, R. E., Eudeline, B., Vadopalas, B., Timmins-Schiffman, E. B., et al. (2020). Temporal proteomic profiling reveals insight into critical developmental processes and temperature-influenced physiological response differences in a bivalve mollusc. BMC Genomics 21:723. doi: 10.1186/s12864-020-07127-3
Velasco, L. (2007). Energetic physiology of the Caribbean scallops Argopecten nucleus and Nodipecten nodosus fed with different microalgal diets. Aquaculture 270, 299–311. doi: 10.1016/j.aquaculture.2007.04.005
Velasco, L. A., and Barros, J. (2008). Experimental larval culture of the Caribbean scallops Argopecten nucleus and Nodipecten nodosus. Aquac. Res. 39, 603–618. doi: 10.1111/j.1365-2109.2008.01917.x
Velez, C., Figueira, E., Soares, A. M., and Freitas, R. (2016). Combined effects of seawater acidification and salinity changes in Ruditapes philippinarum. Aquat. Toxicol. 176, 141–150. doi: 10.1016/j.aquatox.2016.04.016
Walker, R. L., and Tenore, K. R. (1984). Growth and production of the dwarf surf clam Mulinia lateralis (Say 1822) in a Georgia estuary. Gulf Res. Rep. 7, 357–363. doi: 10.18785/grr.0704.07
Wang, B., Liu, Z., Liu, Z., Liu, S., and Feng, X. (2015). Studies on large scale artificial breeding of Lutraria sieboldii. J. Guangdong Ocean Univ. 1, 35–42.
Wang, H., Zhang, Y., Wang, L., Zhu, C., Zhang, J., and Wu, N. (2021). Growth and survival of Pinctada martensii (Dunker) postlarvae under concurrent variation in temperature, algal ration and stocking density. Aquacult. Rep. 20:100668. doi: 10.1016/j.aqrep.2021.100668
Wang, X., and Zhang, X. (2012). Optimal extraction and hydrolysis of Chlorella pyrenoidosa proteins. Bioresour. Technol. 126, 307–313. doi: 10.1016/j.biortech.2012.09.059
Weston, K., Jahangard, S., Ingram, B. A., Miller, A. D., Jennings, G., and Sherman, C. D. (2021). Factors affecting settlement, growth and metamorphosis of hatchery-produced Australian blue mussel larvae. Aquacult. Int. 29, 1963–1977. doi: 10.1007/s10499-021-00728-z
Yang, C., Hao, R., Deng, Y., Liao, Y., Wang, Q., Sun, R., et al. (2017). Effects of protein sources on growth, immunity and antioxidant capacity of juvenile pearl oyster Pinctada fucata martensii. Fish Shellfish Immun. 67, 411–418. doi: 10.1016/j.fsi.2017.06.037
Yang, Z., Huang, X., Wang, H., Pan, H., Wang, X., Teng, M., et al. (2021). Effects of microalgae diets and stocking density on larval growth, survival and metamorphosis of dwarf surfclam, Mulinia lateralis. Aquaculture 536:736440. doi: 10.1016/j.aquaculture.2021.736440
Keywords: Mulinia lateralis, spat culture, microalgae diet, temperature, salinity
Citation: Yang Z, Wang H, Li M, Teng M, Wang X, Zhao A, Huang X, Hu J and Bao Z (2022) Optimizing Microalgae Diet, Temperature, and Salinity for Dwarf Surf Clam, Mulinia lateralis, Spat Culture. Front. Mar. Sci. 8:823112. doi: 10.3389/fmars.2021.823112
Received: 03 December 2021; Accepted: 28 December 2021;
Published: 25 January 2022.
Edited by:
Benjamin Costas, University of Porto, PortugalReviewed by:
Jilin Xu, Ningbo University, ChinaTeresa Maria Coelho Baptista, Polytechnic Institute of Leiria, Portugal
Copyright © 2022 Yang, Wang, Li, Teng, Wang, Zhao, Huang, Hu and Bao. This is an open-access article distributed under the terms of the Creative Commons Attribution License (CC BY). The use, distribution or reproduction in other forums is permitted, provided the original author(s) and the copyright owner(s) are credited and that the original publication in this journal is cited, in accordance with accepted academic practice. No use, distribution or reproduction is permitted which does not comply with these terms.
*Correspondence: Xiaoting Huang, eHRodWFuZ0BvdWMuZWR1LmNu