- 1Ocean Networks Canada, University of Victoria, Victoria, BC, Canada
- 2Department of Biology, School of Earth and Ocean Sciences, University of Victoria, Victoria, BC, Canada
Over fifteen years ago, Ocean Networks Canada (ONC) began with the world’s first large-scale, interactive, real-time portal into the ocean, bringing continuous, real-time data to the surface for applications in scientific research, societal benefits, and supporting Canada’s ocean industry. This marked the dawn of the Internet-connected ocean, enabling a more fulsome understanding of the ocean through ocean intelligence. These open data have improved our ability to monitor and understand our changing ocean offshore all three coasts of Canada, thanks to diversity of sensor systems to monitor earthquakes and tsunamis, deep sea biodiversity, whales, hydrothermal vents, neutrinos, ocean noise, ocean acidification, forensics experiments, and the impact of climate change, including sea ice thinning in the Arctic. This pioneering approach began in the late 1990s, when scientists began developing a new way of doing ocean science that was no longer limited by weather and ship-time. They imagined a permanent presence in the ocean of sensors to allow a continuous flow of ocean data via the Internet. This big science began to take shape early this century, when a partnership between United States and Canadian institutions was established. ONC evolved out of this international collaboration with seed funding from the Canada Foundation for Innovation, while in the United States, the Ocean Observatories Initiative (OOI) was funded. ONC works closely with OOI on that span the countries’ west coast border. Recently similar observing initiatives in Europe have begun, led by EMSO, which now has a close collaboration with ONC as an Associate Member.
Introduction
Ocean Networks Canada’s infrastructure includes large telecommunication cabled networks (NEPTUNE and VENUS; Figure 1), community-based networks along all three of Canada’s ocean coasts, mobile systems on ferries, and land-based sensors that support coastal radar, weather stations, ship traffic, earthquake early warning, and tagged marine bird detection.
The cabled observatories are a node-based networks that follow the topology of the Internet protocol and supplies the power and communication capabilities required for data capture and real-time control of sensors, cameras, samplers, electro-mechanical profilers, and a seafloor crawler. Nodes connect power and Internet to sensors via junction box platforms that include step-down transformers at voltages suited to the instruments either located on the platform or connected via extension cables to sensors on the seafloor, beneath the seafloor (in boreholes and caissons), and in the water column (Figure 2). All sensors are part of a network topology that abides by the hierarchical structure of TCP/IP based communication. Communications can be any medium, from copper cable, fiber optics, point-to-point WiFi, the cellular network, micro-wave relays or satellite.
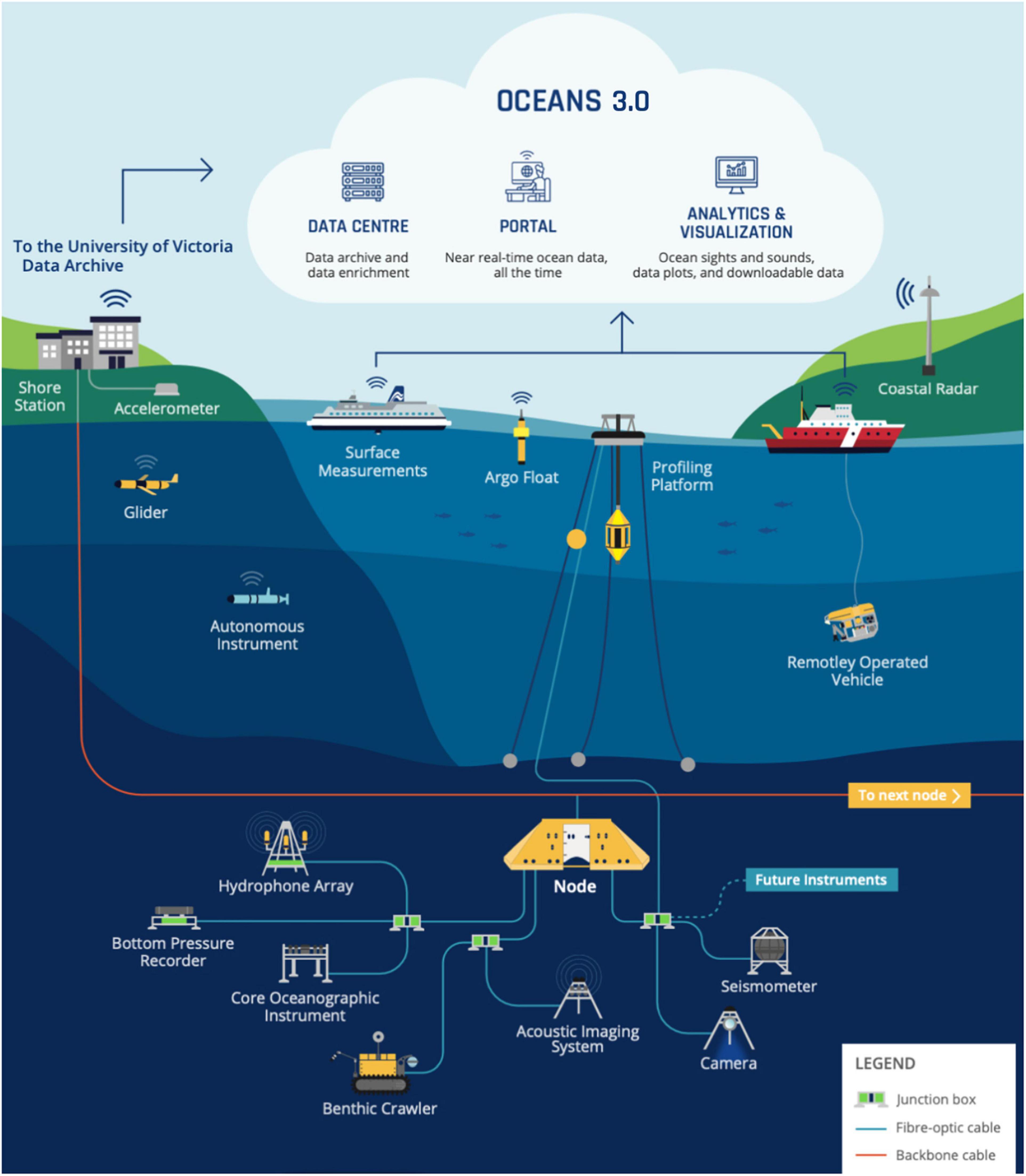
Figure 2. Schematic diagram depicting a node connected to the backbone cable and extension cables connected to platforms and sensors.
Ocean Networks Canada now operates over 9,000 deep sea, coastal and land-based sensors on all three of Canada’s coasts, 24/7/365. Every day, 280 gigabytes of data are added to a rich and diverse petabyte-scale archive, most openly available on ONC’s Oceans 3.0 data portal. These FAIR (findable, accessible, interoperable, reusable) open data enable over 20,000 users around the world to build and make use of ocean intelligence. “Findable” means that data are available through the data portal, searchable by instrument type, location or device ID. “Accessible” is implemented through the data portal user interfaces and APIs, ONC’s user engagement group assists users with accessing data. “Interoperable” means that data are available using multiple format types, including open source format types. To ensure “Reusable” ONC assigns a DOI to evolving data sets for reuse and citations.
ONC conducts regular maintenance at all sites for its high service level. Data from instruments are monitored daily. When issues with data delivery or quality arise, remote troubleshooting starts. If remote troubleshooting is unsuccessful, a data gap is flagged and the instrument is scheduled for maintenance. In critical locations, to minimize data gaps, autonomous instruments are deployed to supplement the compromised cabled instruments.
Ocean Networks Canada’s data are now a decade or more in length and represent a globally unique resource. These high-resolution time series permit researchers to investigate the dynamics of ocean processes across time-scales from hours, days, and seasons, to inter-annual and decadal scales. Researchers are able to undertake critical process studies that require well-characterized ocean environments. The research highlights described here would not have been possible without the cabled technology because it provides enough power for the use of camera lights and robots, temporal resolutions that resolve high frequency processes, long and continuous time series for discovery of trends, and high resolution monitoring over a wide area range to capture processes, e.g., from the shelf to the deep sea.
Research Highlights
Ocean Networks Canada’s strategic plan (2017–2021) identified four major science themes: understanding ocean life; human and climate change impacts; seafloor/ocean/atmosphere links, and seafloor in motion. Research was enabled that advanced each of these themes and increased with the longevity of the time series. Here, some of the highlights that delivered on these themes are described.
Understanding Ocean Life
Barkley Canyon
The role of oxygen minimum zones in submarine canyon ecosystems was revealed for the first time by Domke et al. (2017), while Doya et al. (2017) showcased seasonal monitoring of deep-sea benthic life in the Barkley submarine canyon using data from a seafloor crawler controlled over the Internet using ONC’s data system, Oceans 3.0.
Campanyà-Llovet et al. (2017) investigate a range of sedimentary and near bottom water biogeochemical proxies in a depth gradient along Barkley Canyon, and discussed the potential sources (i.e., macroalgae, phytoplankton, zooplankton; resuspended sediments) and degradation states of organic matter reaching the seafloor, and their role in structuring the macrobenthic infaunal communities.
De Leo et al. (2018) published a 20-month long time-series of seafloor video imagery, acoustic Doppler backscatter, and current profiling, witnessing for the first time the exact onset of the seasonal and deep overwintering migration of Neocalanus spp. copepods. Their work used ground-truth zooplankton net cast data in the Barkley Canyon area, and proposed a conceptual model of vertical current profile structure in the canyon axis acting to focus the deep zooplankton biomass near the core of its overwintering depth (∼1,000 m).
Chauvet et al. (2019) utilized ∼2.5 years of Barkley Canyon Axis seafloor video and environmental data to describe the seasonal and inter-annual variability of tanner crab abundances. The research confirmed a hypothesis that juvenile tanner crabs perform ontogenetic migration from deep to shallow depths and offers insights about the timing of these events immediately following sea surface phytoplankton blooms occurring in spring and summer.
Seabrook et al. (2019), for the first time, described the biogeochemical links between bacterial methanotrophic production and fishery biomass. Triggered by intriguing video observations of tanner crabs “farming” on methane bubbles at Clayoquot methane seep, adult tanner crabs were collected using ROV-deployed baited traps. Combined sulfur, carbon and nitrogen stable-isotope and RNA-based molecular analyses revealed that tanner crabs partially derive their nutrition from methanotrophic bacteria—an unprecedented link between a geochemical energy and a nutrition source for human consumption.
Coastal Sites
Gasbarro et al. (2019) discovered an anomalously severe hypoxic event in Saanich inlet, after a decade of oxygen decline at a rate of 0.07 mL L–1 y–1. They used >10 years of Saanich time-series of dissolved oxygen data coupled with ROV video surveys from 2013 and 2016. Benthic megafauna was reported to follow a 56% overall decline in abundance during the extreme hypoxia events. The results also forecasted potential future scenarios under further and continuous oxygen loss in the Saanich fjor.
Leys et al. (2019) analyzed video imagery from Folger Pinnacle and showed a range of in-situ behaviors of a demosponge sponge Suberites concinnus, such as “twitches,” “ripples,” and “cringes.” This last behavior is clearly associated with sudden changes in pressure caused by severe storms. A massive storm-driven pressure anomaly in 2013 preceded several contractions of the sponge. These behavior changes due to environmental triggers set the stage for further research.
Human and Climate Change Impacts
Thomsen et al. (2017) demonstrated that carbon transfers to the deep ocean are surprisingly significant in winter months. Fjord research by Pawlowicz (2017) revealed the seasonally cyclic nature of low oxygen waters. Soontiens and Allen (2017) used ONC data to model constraints on ocean water mixing in fjords. Physical oceanographers determined that coastal currents can block the release of low-oxygen waters from fjords (Thomson et al., 2017). Ritts (2017) reported on the relationship between human-induced ocean noise and politics.
There is broad consensus that one of the oceanic consequences of climate change is an expansion and shallowing of low-oxygen zones and critical marine habitats (Breitburg et al., 2018). The work of Krogh et al. (2018) utilized time series to quantify the impacts of local sewage discharge on oxygen levels in the Salish Sea. Chu et al. (2018) used 14 months of video and oceanographic data from Saanich Inlet to describe the variability and environmental controls of benthic beta diversity. The data were used to demonstrate how sessile and mobile species respond and recover differently from seasonal hypoxia. Observations and time series from ONC’s Cambridge Bay observatory are contributing (Hu et al., 2018) to assessments of Arctic amplification and changing sea-ice thermodynamics.
A study of CO2 sequestration in the oceanic crust in the Cascadia Basin (Goldberg et al., 2018) was conducted. This included laboratory and modeling studies, potential source/transport scenarios including a carbon-negative scheme, and also economic, regulatory and project management risks analyses.
MacGillivray et al. (2019) reported on wide-scale co-operation among commercial vessel operators, Port Metro Vancouver, JASCO Applied Sciences, and ONC showed that slowing down deep sea vessels transiting through the Salish Sea reduces their underwater radiated noise. The results from this research are informing government decisions aimed at improving the habitat quality of the endangered southern resident killer whale population.
Seafloor, Ocean, and Atmospheric Links
In an astonishing study, Lelièvre et al. (2017) showed that tides and storms impacted deep-sea hydrothermal vent animals. In these same settings, Coogan et al. (2017) revealed insights about near-vent chemical processes in plume while Jackson et al. (2017) described diffuse hydrothermal flow using sound. Love et al. (2017) showed that there were variations in fluid chemistry that constrained hydrothermal vent phase separation. In 2017, Xu published three studies with co-authors that show the impact of tides on hydrothermal vents, further advancing the quantification of hydrothermal venting, and advanced the techniques in using acoustic imaging to study venting (Xu et al., 2017a,b,c). For cold methane seeps, Seabrook et al. (2018) reported on the large variation in the communities of flora and fauna they host.
Research spanning the entire water column, from beneath the sediment interface to the atmosphere, are contributing to a wide range of interdisciplinary results. Li et al. (2018) extensively used the coastal observatory data (ADCP, Echo-sounder, and CODAR) to assess the role of nonlinear internal waves in the Strait of Georgia. Wang et al. (2019) took advantage of in situ measurements of dissolved oxygen concentration from ONC sensors mounted aboard a ferry transiting the Strait of Georgia to estimate primary production and respiration rates. These are high resolution rate estimates which are otherwise difficult to resolve at scales necessary to understand biophysical regulation of atmosphere-ocean interactions and productivity at the base of the marine food web.
One of the most comprehensive studies to date of seafloor gas venting was carried out by Riedel et al. (2018) who estimated that about 1 gram of methane per square meter of seafloor—which totals about 100,000 tons of methane—is released along the Cascadia Margin off BC, Washington, and Oregon each year. They used all of ONC’s water column sonar data from both single- and multi-beam systems and integrated the seafloor multi-beam sonar at Clayoquot Slope that continuously scans for bubbles escaping the seafloor. Furthermore, they used all of the publicly available ship-based data from the NOAA and OOI archives as well as a large non-published data set from Natural Resources Canada. This resulted in the first margin wide quantification of natural gas escaping the seafloor.
Zhang et al. (2019) used video footage of fluids escaping the Grotto vent at Endeavor ridge to study short-period variations in vertical fluxes of hydrothermal plumes. Quantitative analysis of digital video images of plumes using the particle image velocimetry method allowed the comparison of vents from mid-ocean ridges around the globe. Davis and Villinger (2019) used high resolution temperature data measured 1 m below the seafloor to study thermal properties of the sediments. They found that temperature variations are not only due to changes in bottom water temperature, but also observed adiabatic temperature changes due to tidal loading. The unique data set was acquired using a new instrument that was mainly developed to monitor acceleration, tilt, and pressure at the ocean floor.
Wang et al. (2019) analyzed ONC British Columbia Ferries time series of surface ocean measurements during transits across the Salish Sea. The analyses combines dissolved oxygen measurements with physical parameters to determine the relative role of physical transport, air-sea diffusion, and biological productivity for interpreting observed changes in the dissolved oxygen concentrations in near surface waters. The results of this work demonstrate the usefulness of the dataset for quantifying biological productivity at a daily timescale and the importance of measuring diurnal changes in dissolved oxygen for assessing the Net Ecosystem Productivity of the ecosystem—a key indicator for ecological assessment and environmental change.
Duke (2019) presented a study of the marine carbon cycle that utilizes the high resolution time series of biogeochemical properties to better understand the factors that affect inorganic carbon species distribution, seasonal dynamics in biological productivity, and ocean acidification in the Arctic. Both pH and pCO2 were measured by in situ sensors on the regional community observatory operated by ONC. These results significantly contribute to the assessment of these sensors for operating in Arctic cold waters and for addressing seasonal changes in under-ice plankton productivity.
Seafloor in Motion
Gao et al. (2017) researched the thermal conditions on a segment of the Juan de Fuca Ridge that has implications for seismic and tsunami hazards. Rathnayaka and Gao (2017) presented the broad scale seismic structure of the Cascadia subduction zone. Davis et al. (2017) made a major discovery, using seismometer data—seafloor tilt is induced by ocean tides. A new real-time tsunami detection algorithm was developed and reported on by Chierici et al. (2017), while Grilli et al. (2017) used a tsunami algorithm for validation purposes. Tolkova et al. (2017) presented a new approach for rapid forecasting of tsunamis.
McGuire et al. (2018) studied the effect of dynamic stress transients on borehole seismic-geodetic observations at Clayoquot Slope. They found that the Alaska Earthquake did not trigger slow slip and tremor on the updip portion of the Cascadia megathrust. This is a significant since other subduction zones such as Hikurangi and Nankai are known to produce slow-slip events under similar circumstances. Lay et al. (2018) studied the complex rupture of the 2018 Mw 7.9 Gulf of Alaska Earthquake. Gao et al. (2018) investigated rupture scenarios of the Cascadia megathrust and their effect on tsunami generation. Gao (2018) examined the effect of along-strike and downdip variations in physical properties of the oceanic plate on seismicity of the Cascadia subduction zone. Rohr et al. (2018) used the Nootka fault zone as a case study to explore mechanisms for strike-slip fault initiation. Williamson and Newman (2018) assessed the suitability for a near-field tsunami early warning using open-ocean instrumentation.
Lintern D.G. et al. (2019) described ONC infrastructure from the Fraser Delta and ONC hydrophone records from turbidity currents, and emphasized the need for in-situ real-time observations for these types of hazards. Lintern G. et al. (2019) described ONC’s Community Observatory in Kitimat Arm for its potential for live hazard warning system for underwater landslides and induced tsunamis.
The effectiveness of ocean observatories for gas hydrate research was published by Scherwath et al. (2019), with documentation of various studies that were realized with ONC data, and included spectacular three-dimensional images from the structural light imaging system, a laser camera, on the seafloor crawler Wally.
Forward to 2030
Ocean Networks Canada recently completed its Strategic Plan 2030, titled “Advancing our Knowledge of the Ocean at a Critical Time.” This ambitious plan sets out three major goals as well as strategies for delivering ocean intelligence for science, society, and industry.
Advance ocean observing. Ocean observation is a critical foundation for understanding our changing ocean and climate and the ocean’s key role in buffering and moderating the Earth’s climate. ONC’s deep-sea, coastal, and land-based infrastructure provides real-time, long-duration, high-quality data of high spatial and time resolution. ONC plans to advance ocean observing by continuing and expanding the community-based ocean observing and international partnerships; equitably increasing workforce capacity; filling gaps in Canada’s ocean observing networks to address high-priority national, provincial, and regional needs; and evolve the observing networks and data access. At the same time, ONC will build on existing time-series data; and enhance ocean knowledge sharing through learning, education, media communications, storytelling, and the arts.
Ocean Networks Canada’s approach to ocean observing is a commitment to ensure that no data are stranded, measuring at the highest temporal resolution possible, and deliver data in real-time or near real-time. Unlike autonomous systems that mainly rely on preserving battery power, which limits temporal resolution, the ONC approach provides data at time resolutions from daily to seasonal to decadal. The advantage provided by ONC’s NEPTUNE observatory is, for example, that dynamic changes associated with intermittent climate change impact events (e.g., heat extremes) can be studied in the detail needed to discern natural vs. climate-related variability. The real-time data can also be re-purposed to be used not only for science, but also for real-time valued added products needed for marine safety.
Develop and deliver ocean intelligence. Unlocking the immense potential of ocean intelligence requires increasingly innovative data interpretation tools to help us understand complex systems. ONC uses the International Council for Science World Data System as a world-leading standard. The global blue economy depends upon sustainable use of the ocean. Advanced data products are required to address and monitor the health of the ocean, inform safer shipping and ocean transportation, and provide vital ocean insights for communities. ONC plans to continue collaborations with local and Indigenous communities, public safety organizations, and governments to make ocean intelligence accessible to all stakeholders, from researchers, students, Indigenous partners, coastal communities and industry players, to policy-makers.
Ocean-based solutions for climate change mitigation and coastal resilience. As we face the growing impacts of climate change, coastal communities are disproportionately threatened by sea-level rise, storm surge, ocean warming and acidification, and declining seafood stocks. ONC will work with partners to enable solutions for coastal resilience and make major contributions to advance a sustainable ocean economy through the development of climate change mitigation and adaptation technologies that include carbon dioxide removal, forecasting coastal sea level rise and storm surges, monitoring ocean acidification in the vicinity of vulnerable ocean industries like shellfish farms, and transferring observing technologies to Indigenous coastal communities so that they expand upon their knowledge systems to grow their stewardship efforts.
Author Contributions
All authors listed have made a substantial, direct, and intellectual contribution to the work, and approved it for publication.
Funding
Ocean Networks Canada is supported by the Canada Foundation for Innovation, the Governments of Canada and British Columbia, and the University of Victoria.
Conflict of Interest
The authors declare that the research was conducted in the absence of any commercial or financial relationships that could be construed as a potential conflict of interest.
Publisher’s Note
All claims expressed in this article are solely those of the authors and do not necessarily represent those of their affiliated organizations, or those of the publisher, the editors and the reviewers. Any product that may be evaluated in this article, or claim that may be made by its manufacturer, is not guaranteed or endorsed by the publisher.
References
Breitburg, D., Levin, L. A., Oschlies, A., Grégoire, M., Chavez, F. P., Conley, D. J., et al. (2018). Declining oxygen in the global ocean and coastal waters. Science 359:eaam7240. doi: 10.1126/science.aam7240
Campanyà-Llovet, N., Snelgrove, P. V. R., and Parrish, C. C. (2017). Rethinking the importance of food quality in marine benthic food webs. Prog.Oceanogr. 156, 240–251. doi: 10.1016/j.pocean.2017.07.006
Chauvet, P., Metaxas, A., and Matabos, M. (2019). Interannual variation in the population dynamics of juveniles of the deep-sea crab Chionoecetes tanneri. Front. Mar. Sci. 6:50. doi: 10.3389/fmars.2019.00050
Chierici, F., Embriaco, D., and Pignagnoli, L. (2017). A new real-time tsunami detection algorithm. J. Geophys. Res. 122, 636–652. doi: 10.1002/2016JC012170
Chu, J. W. F., Curkan, C., and Tunnicliffe, V. (2018). Drivers of temporal beta diversity of a benthic community in a seasonally hypoxic fjord. R. Soc. Open Sci. 5:172284. doi: 10.1098/rsos.172284
Coogan, L. A., Attar, A., Mihaly, S. F., Jeffries, M., and Pope, M. (2017). Near-vent chemical processes in a hydrothermal plume: insights from an integrated study of the Endeavour segment. Geochem. Geophys. Geosyst. 18, 1641–1660. doi: 10.1002/2016GC006747
Davis, E. E., and Villinger, H. (2019). Subseafloor temperature variations influenced by variations in bottom water temperature and pressure: new high resolution observations and implications. J. Geophys. Res. 124, 76–87. doi: 10.1029/2018JB016151
Davis, E. E., Heesemann, M., Lambert, A., and He, J. (2017). Seafloor tilt induced by ocean tidal loading inferred from broadband seismometer data from the Cascadia subduction zone and Juan de Fuca Ridge. Earth Planet. Sci. Lett. 463, 243–252. doi: 10.1016/j.epsl.2017.01.042
De Leo, F. C., Ogata, B., Sastri, A. R., Heesemann, M., Mihály, S., Galbraith, M., et al. (2018). High-frequency observations from a deep-sea cabled observatory reveal seasonal overwintering of Neocalanus spp. in Barkley Canyon, NE Pacific: insights into particulate organic carbon flux. Prog. Oceanogr. 169, 120–137. doi: 10.1016/j.pocean.2018.06.001
Domke, L., Lacharité, M., Metaxas, A., and Matabos, M. (2017). Influence of an oxygen minimum zone and macroalgal enrichment on benthic megafaunal community composition in a NE Pacific submarine canyon. Mar. Ecol. 38, e12481. doi: 10.1111/maec.12481
Doya, C., Chatzievangelou, D., Bahamon, N., Purser, A., De Leo, F. C., Juniper, S. K., et al. (2017). Seasonal monitoring of deep-sea megabenthos in Barkley Canyon cold seep by internet operated vehicle (IOV). PLoS One 12:e0176917. doi: 10.1371/journal.pone.0176917
Duke, P. J. (2019). Describing Seasonal Marine Carbon System Processes in Cambridge Bay Nunavut using an Innovative Sensor Platform. Calgary, AB: University of Calgary.
Gao, D., Wang, K., Davis, E. E., Jiang, Y., Insua, T. L., and He, J. (2017). Thermal state of the Explorer segment of the Cascadia subduction zone: implications for seismic and tsunami hazards. Geochem. Geophys. Geosyst. 18, 1569–1579. doi: 10.1002/2017GC006838
Gao, D., Wang, K., Insua, T. L., Sypus, M., Riedel, M., and Sun, T. (2018). Defining megathrust tsunami source scenarios for northernmost Cascadia. Nat. Hazards 94, 445–469. doi: 10.1007/s11069-018-3397-6
Gao, H. (2018). Three-dimensional variations of the slab geometry correlate with earthquake distributions at the Cascadia subduction system. Nat. Commun. 9:1204. doi: 10.1038/s41467-018-03655-5
Gasbarro, R., Chu, J. W. F., and Tunnicliffe, V. (2019). Disassembly of an epibenthic assemblage in a sustained severely hypoxic event in a northeast Pacific basin. J. Mar. Syst. 198:103184. doi: 10.1016/j.jmarsys.2019.103184
Goldberg, D., Aston, L., Bonneville, A., Demirkanli, I., Evans, C., Fisher, A., et al. (2018). Geological storage of CO2 in sub-seafloor basalt: the CarbonSAFE pre-feasibility study offshore Washington State and British Columbia. Energy Proc. 146, 158–165. doi: 10.1016/j.egypro.2018.07.020
Grilli, S. T., Guérin, C.-A., Shelby, M., Grilli, A. R., Moran, P., Grosdidier, S., et al. (2017). Tsunami detection by high frequency radar beyond the continental shelf: ii. extension of time correlation algorithm and validation on realistic case studies. Pure Appl. Geophys. 174, 3003–3028. doi: 10.1007/s00024-017-1619-6
Hu, X., Sun, J., Chan, T. O., and Myers, P. G. (2018). Thermodynamic and dynamic ice thickness contributions in the canadian arctic archipelago in NEMO-LIM2 numerical simulations. Cryosphere 12, 1233–1247. doi: 10.5194/tc-12-1233-2018
Jackson, D. R., Ivakin, A. N., Xu, G., and Bemis, K. G. (2017). Sonar observation of diffuse hydrothermal flows. Earth Space Sci. 4, 230–239. doi: 10.1002/2016EA000245
Krogh, J., Ianson, D., Hamme, R. C., and Lowe, C. J. (2018). Risks of hypoxia and acidification in the high energy coastal environment near Victoria, Canada’s untreated municipal sewage outfalls. Mar. Pollut. Bull. 133, 517–531. doi: 10.1016/j.marpolbul.2018.05.018
Lay, T., Ye, L., Bai, Y., Cheung, K. F., and Kanamori, H. (2018). The 2018 MW 7.9 gulf of alaska earthquake: multiple fault rupture in the pacific plate. Geophys. Res. Lett. 45, 9542–9551. doi: 10.1029/2018GL079813
Lelièvre, Y., Legendre, P., Matabos, M., Mihály, S., Lee, R. W., Sarradin, P.-M., et al. (2017). Astronomical and atmospheric impacts on deep-sea hydrothermal vent invertebrates. Proc. R. Soc. B 284:20162123. doi: 10.1098/rspb.2016.2123
Leys, S. P., Mah, J. L., McGill, P. R., Hamonic, L., De Leo, F. C., and Kahn, A. S. (2019). Sponge behavior and the chemical basis of responses: a post-genomic view. Integr. Comp. Biol. 59, 751–764. doi: 10.1093/icb/icz122
Li, L., Pawlowicz, R., and Wang, C. (2018). Seasonal variability and generation mechanisms of nonlinear internal waves in the strait of georgia. J. Geophys. Res. 123, 5706–5726. doi: 10.1029/2017JC013563
Lintern, D. G., Mosher, D. C., and Scherwath, M. (2019). Advancing from subaqueous mass movement case studies to providing advice and mitigation. Geol. Soc. Lond. Spec. Publ. 477, 1–14. doi: 10.1144/SP477-2018-190
Lintern, G., Blais-Stevens, A., Stacey, C., Shaw, J., Bobrowsky, P., Conway, K., et al. (2019). Providing multidisciplinary scientific advice for coastal planning in Kitimat Arm, British Columbia. Geol. Soc. Lond. Spec. Publ. 477, 567–581. doi: 10.1144/SP477.40
Love, B., Lilley, M., Butterfield, D., Olson, E., and Larson, B. (2017). Rapid variations in fluid chemistry constrain hydrothermal phase separation at the Main Endeavour Field. Geochem. Geophys. Geosyst. 18, 531–543. doi: 10.1002/2016GC006550
MacGillivray, A. O., Li, Z., Hannay, D. E., Trounce, K. B., and Robinson, O. M. (2019). Slowing deep-sea commercial vessels reduces underwater radiated noise. J. Acoust. Soc. Am. 146, 340–351. doi: 10.1121/1.5116140
McGuire, J. J., Collins, J. A., Davis, E., Becker, K., and Heesemann, M. (2018). A lack of dynamic triggering of slow slip and tremor indicates that the shallow cascadia megathrust offshore vancouver island is likely locked. Geophys. Res. Lett. 45, 11095–11103. doi: 10.1029/2018GL079519
Pawlowicz, R. (2017). Seasonal cycles, hypoxia, and renewal in a coastal fjord (Barkley Sound, British Columbia). Atmos. Ocean 55, 264–283. doi: 10.1080/07055900.2017.1374240
Rathnayaka, S., and Gao, H. (2017). Crustal-scale seismic structure from trench to forearc in the Cascadia subduction zone. J. Geophys. Res. 122, 7311–7328. doi: 10.1002/2017JB014299
Riedel, M., Scherwath, M., Römer, M., Veloso, M., Heesemann, M., and Spence, G. D. (2018). Distributed natural gas venting offshore along the Cascadia margin. Nat. Commun. 9:3264. doi: 10.1038/s41467-018-05736-x
Ritts, M. (2017). Amplifying environmental politics: ocean noise. Antipode 49, 1406–1426. doi: 10.1111/anti.12341
Rohr, K. M. M., Furlong, K. P., and Riedel, M. (2018). Initiation of strike-slip faults, serpentinization, and methane: the nootka fault zone, the juan de fuca-explorer plate boundary. Geochem. Geophys. Geosyst. 19, 4290–4312. doi: 10.1029/2018GC007851
Scherwath, M., Thomsen, L., Riedel, M., Römer, M., Chatzievangelou, D., Schwendner, J., et al. (2019). Ocean observatories as a tool to advance gas hydrate research. Earth Space Sci. 6, 2644–2652. doi: 10.1029/2019EA000762
Seabrook, S., De Leo, F. C., and Thurber, A. R. (2019). Flipping for food: the use of a methane seep by tanner crabs (Chionoecetes tanneri). Front. Mar. Sci. 6:43. doi: 10.3389/fmars.2019.00043
Seabrook, S., De Leo, F. C., Baumberger, T., Raineault, N., and Thurber, A. R. (2018). Heterogeneity of methane seep biomes in the Northeast Pacific. Deep Sea Res. Part II 150, 195–209. doi: 10.1016/j.dsr2.2017.10.016
Soontiens, N., and Allen, S. E. (2017). Modelling sensitivities to mixing and advection in a sill-basin estuarine system. Ocean Modelling 112, 17–32. doi: 10.1016/j.ocemod.2017.02.008
Thomsen, L., Aguzzi, J., Costa, C., De Leo, F., Ogston, A., and Purser, A. (2017). The oceanic biological pump: rapid carbon transfer to depth at continental margins during winter. Sci. Rep. 7:10763. doi: 10.1038/s41598-017-11075-6
Thomson, R. E., Spear, D. J., Krassovski, M. V., Hourston, R. A. S., Juhász, T. A., and Mihály, S. F. (2017). Buoyancy-driven coastal current blocks ventilation of an anoxic fjord on the Pacific coast of Canada. J. Geophys. Res. 122, 2976–2998. doi: 10.1002/2016JC012512
Tolkova, E., Nicolsky, D., and Wang, D. (2017). A response function approach for rapid far-field tsunami forecasting. Pure Appl. Geophys. 174, 3249–3327. doi: 10.1007/s00024-017-1612-0
Wang, C., Pawlowicz, R., and Sastri, A. R. (2019). Diurnal and seasonal variability of near-surface oxygen in the strait of georgia. J. Geophys. Res. 124, 2418–2439. doi: 10.1029/2018JC014766
Williamson, A. L., and Newman, A. V. (2018). Suitability of open-ocean instrumentation for use in near-field tsunami early warning along seismically active subduction zones. Pure Appl. Geophys. 176, 3247–3262. doi: 10.1007/s00024-018-1898-6
Xu, G., Jackson, D. R., and Bemis, K. G. (2017a). The relative effect of particles and turbulence on acoustic scattering from deep sea hydrothermal vent plumes revisited. J. Acoust. Soc. Am. 141, 1446–1458. doi: 10.1121/1.4974828
Xu, G., Jackson, D. R., Bemis, K. G., and Ivakin, A. (2017b). Long-term, quantitative observations of seafloor hydrothermal venting using an imaging sonar. J. Acoust. Soc. Am. 142:2504. doi: 10.1121/1.5014145
Xu, G., Larson, B. I., Bemis, K. G., and Lilley, M. D. (2017c). A preliminary 1-D model investigation of tidal variations of temperature and chlorinity at the Grotto mound, Endeavour Segment, Juan de Fuca Ridge. Geochem. Geophys. Geosyst. 18, 75–92. doi: 10.1002/2016GC006537
Keywords: ocean observing, marine life, climate change, marine geoscience, marine hazards, ocean data
Citation: Moran K, Juniper SK, Bligh S, Loock D, Kulin I, Paulson M and Pirenne B (2022) Canada’s Internet-Connected Ocean. Front. Mar. Sci. 8:805134. doi: 10.3389/fmars.2021.805134
Received: 29 October 2021; Accepted: 27 December 2021;
Published: 01 February 2022.
Edited by:
Juan José Dañobeitia, ERIC Foundation, ItalyReviewed by:
Christoph Waldmann, University of Bremen, GermanyCopyright © 2022 Moran, Juniper, Bligh, Loock, Kulin, Paulson and Pirenne. This is an open-access article distributed under the terms of the Creative Commons Attribution License (CC BY). The use, distribution or reproduction in other forums is permitted, provided the original author(s) and the copyright owner(s) are credited and that the original publication in this journal is cited, in accordance with accepted academic practice. No use, distribution or reproduction is permitted which does not comply with these terms.
*Correspondence: Kate Moran, b25jc2VjQG9jZWFubmV0d29ya3MuY2E=