- 1College of Life Sciences, Sun Yat-sen University, Guangzhou, China
- 2Institute of Marine Research, Bergen, Norway
- 3Guangzhou Leader Bio-Technology Co., Ltd., Guangzhou, China
An 8-week feeding experiment was conducted to investigate whether diet supplementation of lutein could result in similar growth performance, antioxidant capacity, and immunity of Litopenaeus vannamei when compared to dietary astaxanthin. Juvenile L. vannamei (initial body weight: 0.64 ± 0.04 g) were fed with one of five isonitrogen and isolipids diets with/without lutein or astaxanthin [control group (C); the lutein (L) groups contained 0, 62.5, 75, 87.5 ppm lutein, respectively, the astaxanthin (A) group contained 50 ppm astaxanthin]. Results showed that dietary supplementation of lutein ranging from 62.5 to 75 ppm resulted in similar growth performance (WGR, SGR, FCR, and SR) of L. vannamei compared with the A group (P > 0.05). Apart from that, no statistical difference was observed in antioxidant parameters (hemolymph T-AOC, hemolymph MDA, and RNA expression level of GSH-PX, CAT), anti-inflammatory ability (Relish, Rho, and HSP70) and apoptosis-related gene expression (Caspase3) among lutein treatments ranging from 62.5 to 87.5 ppm and the A group (P > 0.05). These results indicate that a dose of 62.5–75 ppm of lutein was suitable in the diet of L. vannamei for substituting dietary astaxanthin.
Introduction
In recent years, there have been two significant factors existing in the shrimp farming industry that may affect the profit of shrimp. Firstly, water environments were deteriorated by plenty of pollutants (such as heavy metals, pesticides, bacteria, and viruses) due to industrialization’s development and human activity’s involvement, resulting in seriously high stress-induced diseases and low survival rate in the shrimp culture industry (Zhang et al., 2013). In addition, maintaining bright and appropriate body pigmentation is also a significant factor closely related to customers’ preference and shrimp’s price because it means freshness and high quality of the merchandise (Shahidi and Brown, 1998; Seidgar, 2015). In fact, shrimp and other crustaceans are incapable of bio-synthesize carotenoids de novo, which makes it essential for them to obtain carotenoids from the daily diet for body pigmentation (Boonyaratpalin et al., 2001). Previous studies paid more attention to diet supplementation with astaxanthin (3,3′-dihydroxy-β,β-carotene-4,4′-dione) on crustaceans as it helps to enhance the stress resistance of crustaceans by acting as an effective antioxidant against ROS as well as being a green additive for improving growth performance and skin pigmentation of aquatic animals (Latscha, 1989; Petit et al., 1997; Chien et al., 2003; Seabra and Pedrosa, 2010). However, diet supplementation with astaxanthin seems to considerably increase the shrimp feed cost because of the expensive synthetic process of this carotenoid pigment (Boonyaratpalin et al., 2001). Therefore, other cost-effective additives need to be identified for substituting the astaxanthin in the shrimp diets.
Crustaceans could convert many carotenoids like β-carotene, which are easy to be acquired from vegetable ingredients of the feed, into astaxanthin (Giuliano et al., 2000; Niu et al., 2014). Therefore, these green additives are of high interest in the aquaculture industry (da Costa and Miranda-Filho, 2020). For example, lutein, one of the significantly cheaper natural carotenoid pigments compared to astaxanthin, can be extracted from some vegetables like marigolds (da Costa and Miranda-Filho, 2020). Ettefaghdoost and Haghighi (2021) reported that dietary supplementation with 200 mg/kg lutein could enhance the growth performance, immune capacity and total carotenoid pigment content in different tissues of Macrobrachium nipponense.
Litopenaeus vannamei, one of the most profitable shrimp species in south China, is very popular with customers because of its delicious flesh and high nutritional value (Sirirustananun et al., 2011). Previous studies demonstrated that both lutein and astaxanthin play essential roles in growth performance, anti-resistance as well as pigmentation of aquatic animals (Kalinowski et al., 2005; Meilisza et al., 2017). However, knowledge about whether lutein can become a substitution for astaxanthin remained little understood. In our present study, diet supplementation with/without lutein or astaxanthin was compared regarding the effects of the two carotenoids on growth performance, anti-resistance and metabolism of L. vannamei. These results may provide a reference for the efficient diet formulation of L. vannamei.
Materials and Methods
Experiment Diet
In the current study, the commercial lutein (5% lutein) and astaxanthin (10% astaxanthin) were kindly provided by Guangzhou Leader Bio-Technology Co., Ltd., China. Other ingredients like fish meal were all purchased from Guangzhou Chengyi Co., Ltd., China. Five isonitrogen and isolipids experiment diets were formulated with or without lutein or astaxanthin [control group (C); the lutein (L) groups, L1-L3, contained 0, 62.5 ppm, 75 ppm, 87.5 ppm lutein, respectively, the astaxanthin (A) group contained 50 ppm astaxanthin] (Table 1). All feeds contained 405 g kg–1 crude protein and 71 g kg–1 crude lipid approximately. The diets were made according to the methods reported by Niu et al. (2012). Briefly, all ingredients presented in the Table 1 and deionized water (250 ml/kg dry ingredients mixture) were thoroughly mixed in the Hobart-type mixer, and then the diets (1.2 mm diameter) were extruded using the pelletizer (South China University of Technology, Guangdong, China). Then, diets were heated for 60 min in a ventilated oven (70°C). Afterward, the diets were stored at –20°C and were kept in dark prior to the feeding trail.
Shrimp and Experimental Conditions
Juvenile L. vannamei were obtained and cultured at the Chinese Academy of Fishery Sciences (Lingshui, China). Before the feeding experiment, shrimps were fed with the C group diet for 2 weeks to acclimate to the experiment conditions. At the beginning of the feeding trial, 800 lively shrimps (initial body weight: 0.64 ± 0.04 g) with similar size were distributed randomly into recirculating water systems with 20 cylindrical fiber tanks (300 L). Each diet was randomly assigned to quadruplicate tanks. Shrimps were fed to apparent satiation three times (at 6:00, 12:00, and 18:00; with 5–8% body weight) daily for 8 weeks.
During the feeding trial, the water temperature was maintained 26.9–28.2°C, pH 7.6–7.8, salinity 30–32‰, dissolved oxygen > 7 mg/L, total ammonia nitrogen < 0.1 mg/L, and sulfide < 0.05 mg/L.
Sample Collection
At the end of the feeding trial, shrimps were starved for 24 h and then weighed. The total number of shrimps in each tank was counted at the same time. Afterward, eight shrimps from each tank were randomly collected and anesthetized (MS-222, Sigma, St. Louis, MO, United States) to collect the hemolymph samples. Subsequently, shrimps were dissected and hepatopancreas samples were removed for enzyme and RNA expression analysis. The hemolymph sample was centrifuged (7,100 g, 10 min, 4°C) and the hemolymph was separated for antioxidant parameter analysis. All samples were collected rapidly and frozen in liquid nitrogen until analysis.
Proximate Composition Analysis
Proximate compositions of diets were assayed using the standard methods of AOAC (Horwitz et al., 2010). Briefly, diets crude protein content (N × 6.25) was detected using the Kjeldahl method (1030- Autoanalyzer; Tecator, Höganäs, Sweden). Diet crude lipid was detected by the Soxhlet extractor method (Soxtec System HT6, Tecator, Sweden). Diets moisture was analyzed by drying the diets in oven at 105°C to constant weight.
Detection of Hepatopancreas and Hemolymph Antioxidant Parameters
Hepatopancreas samples were homogenized and centrifuged according to the methods of Fang et al., 2021b). Briefly, hepatopancreas samples and phosphate buffer (1:10) were mixed and homogenized, and then centrifuged for 10 min (4°C, 1,200 g), and the supernatants were collected. Supernatants, as well as hemolymph samples, were determined for antioxidant parameters, including superoxide dismutase (SOD) (A001–1), total antioxidant capacity (T-AOC) (A015–2-1) and malondialdehyde (MDA) (A003–1). The analysis of antioxidant parameters was carried out following the kits’ instructions (Nanjing Jiancheng Bioengineering Institute, Nanjing, China).
Hepatopancreas RNA Extraction and Expression Analysis
RNA extraction and Real-time PCR were following the methods reported in our previous articles (Fang et al., 2019). Briefly, total RNA was extracted from the hepatopancreas using Trizol ® reagent (Invitrogen, United States) following the manufacturer’s instructions. 1% agarose gel electrophoresis and spectrophotometer (NanoDrop 2,000, Thermo Fisher, United States) were used to determine RNA quality and concentration, respectively. Afterward, cDNA was synthesized using the PrimeScript™ RT reagent kit (Takara, Japan), following the manufacturer’s instructions. Real-time PCR for the target genes was performed using an SYBR ® Premix Ex Taq™ II (Takara, Japan) and quantified on the LightCycler 480 (Roche Applied Science, Basel, Switzerland).
The primers used in the current study are presented in Table 2.
Statistical Analysis
All data in the current study are presented as means ± standard error (SE). Data were analyzed by SPSS 22.0 (SPSS, Chicago, IL, United States) and followed by one-way analysis of variance (ANOVA) and Duncan’s test. P < 0.05 was regarded as significant difference among groups.
Results
Growth Performance
As shown in Table 3, diet supplementation with a suitable level of lutein or astaxanthin significantly altered the growth performance of L. vannamei. Significantly higher weight gain rate (WGR) and specific growth rate (SGR) of L. vannamei were obtained in the L1, L2, and A groups compared to the C group (P < 0.05), while no statistical difference was found between the C and L3 groups (P > 0.05). At the same time, data in the A group showed no significant difference compared with the L1 and L2 groups (P > 0.05). Feed conversion ratio (FCR) of L. vannamei in the A group was significantly lower than that in the C group (P < 0.05) and no statistical difference was observed compared with the other three lutein-supplemented groups (P > 0.05). The survival rate (SR) of L. vannamei ranged from 94.38 to 98.13% after 8 weeks of feeding treatment (P > 0.05).
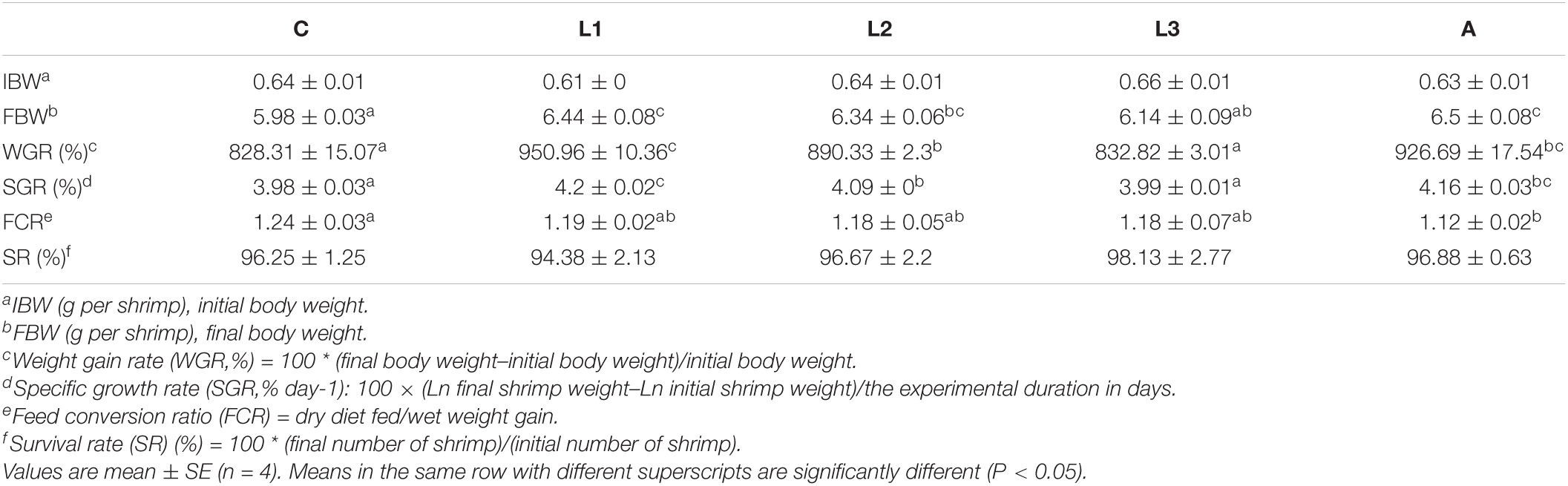
Table 3. Growth performance of L. vannamei fed diet supplementation with/without lutein or astaxanthin for 56 days.
Antioxidant Capacity
The hepatopancreas and hemolymph antioxidant parameters of L. vannamei fed with/without dietary lutein or astaxanthin are shown in Table 4. Four carotenoid pigments-supplemented groups (L1-L3, A) all had significantly lower enzyme activity of hepatopancreas T-SOD, lower level of hemolymph T-AOC and lower concentration of hemolymph MDA than the C group (P < 0.05). Meanwhile, no significant difference in hemolymph T-AOC and MDA of L. vannamei was found among the L1-3 and A groups (P > 0.05). Although there was no statistical difference in hepatopancreas MDA among five experiment groups (P > 0.05), the MDA value in four carotenoid pigment-supplied groups was still relatively lower than the control group.
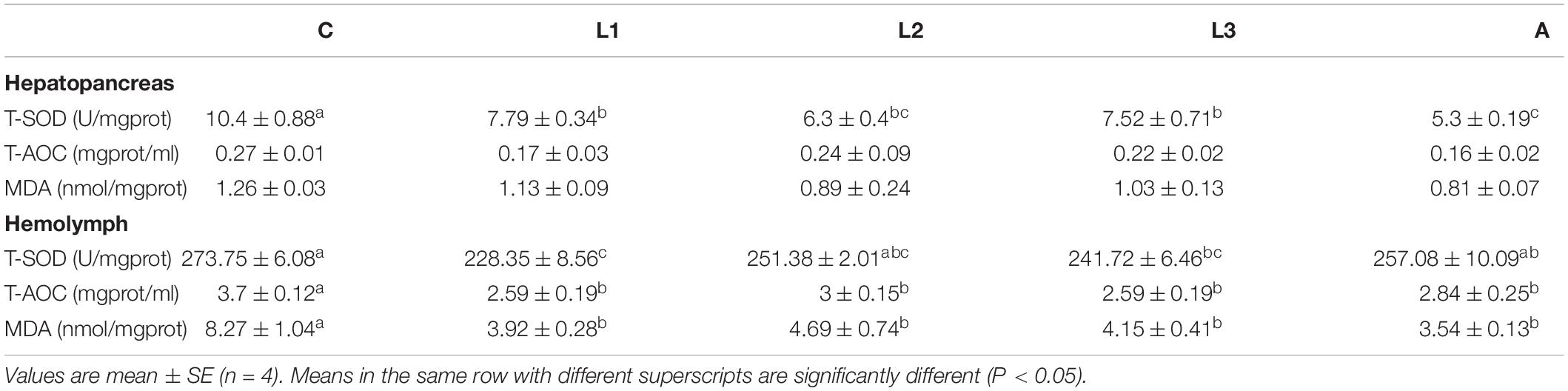
Table 4. Hepatopancreas and hemolymph antioxidant parameter of L. vannamei fed diet supplementation with/without lutein or astaxanthin for 56 days.
RNA Expression of Genes Related to Immunity
RNA expression levels of antioxidative genes (SOD, GSH-PX, and CAT) in the hepatopancreas of L. vannamei are presented in Figure 1. The RNA expression levels of hepatopancreas GSH-PX and CAT in four carotenoid pigments-supplemented groups showed significantly lower values compared to the C group (P < 0.05), and data were not statistically different among these four groups (P > 0.05). Compared to the C group, the RNA expression level of SOD was no statistically different in L. vannamei fed with/without dietary lutein or astaxanthin (P > 0.05).
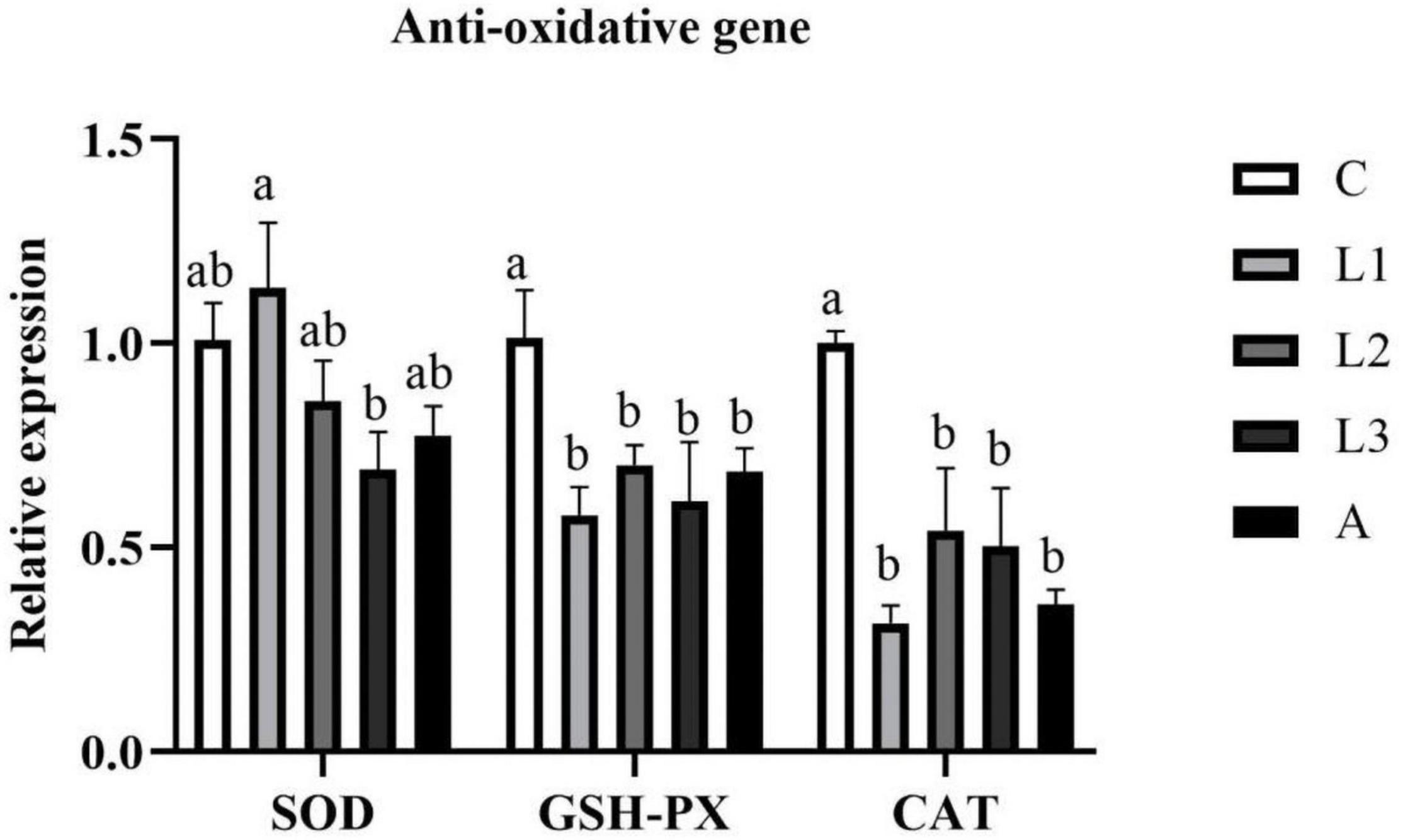
Figure 1. Hepatopancreas RNA expression level of antioxidative genes of L. vannamei fed diet supplementation with/without lutein or astaxanthin for 56 days.
Hepatopancreas RNA expression of anti-inflammatory genes (Relish, Rho, HSP70) of L. vannamei exposed to different diet treatments was shown in Figure 2. The RNA expression level of HSP70 in the A group was significantly lower than that in the C group (P < 0.05), and no statistical difference was observed compared with the other three lutein groups (P > 0.05). Significantly lower data of Relish has been observed in four carotenoid pigments-supplemented groups compared to the C group (P < 0.05). However, regarding the RNA expression level of Rho, no statistical difference was found among all the groups (P > 0.05).
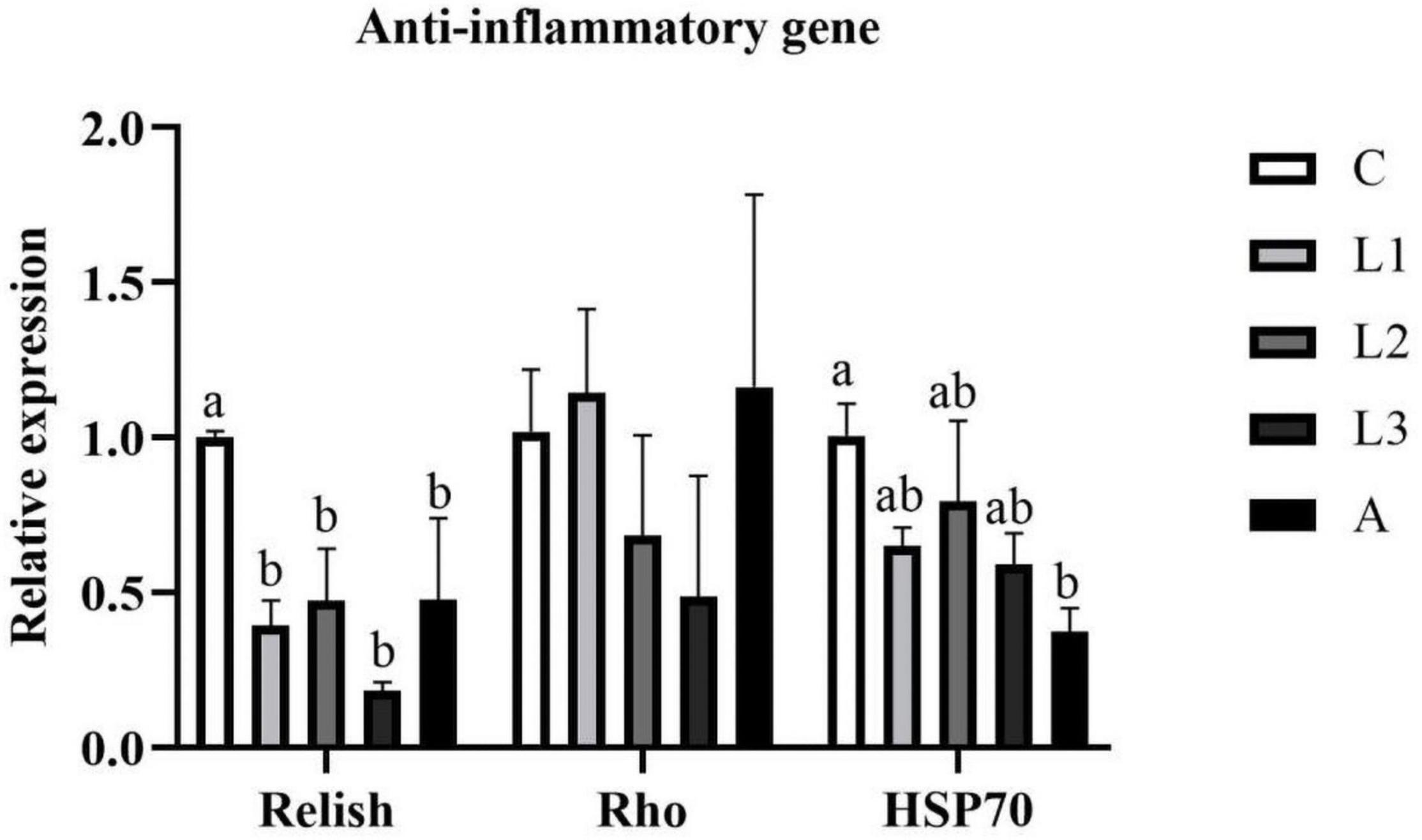
Figure 2. Hepatopancreas RNA expression level of anti-inflammatory genes of L. vannamei fed diet supplementation with/without lutein or astaxanthin for 56 days.
As shown in Figure 3, the hepatopancreas RNA expression level of Caspase 3 in four carotenoid pigments groups were significantly lower compare to the C group (P < 0.05), and the data were not statistically different among these four groups (P > 0.05). Apart from that, significantly lower RNA expression level of Caspase 2 was found in the L3 and A groups than in C, L1 and L2 groups (P < 0.05).
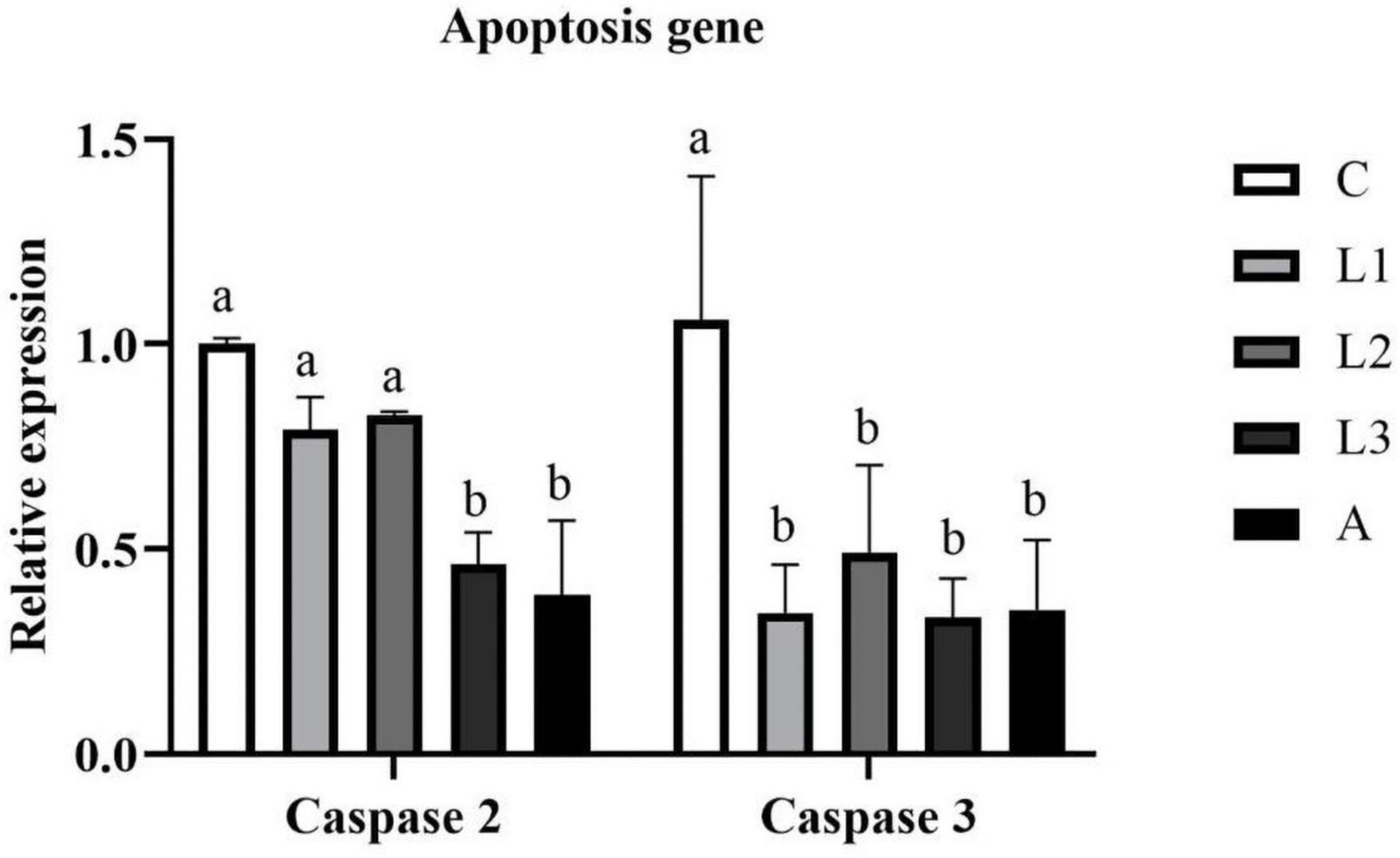
Figure 3. Hepatopancreas RNA expression level of apoptosis genes of L. vannamei fed diet supplementation with/without lutein or astaxanthin for 56 days.
RNA Expression of Genes Related to Digestive and Metabolic Enzymes
As shown in Figure 4, diet supplementation with lutein or astaxanthin was unable to alter the hepatopancreas RNA expression level of digestive enzyme genes (chymotrypsin and trypsin) of L. vannamei (P > 0.05).
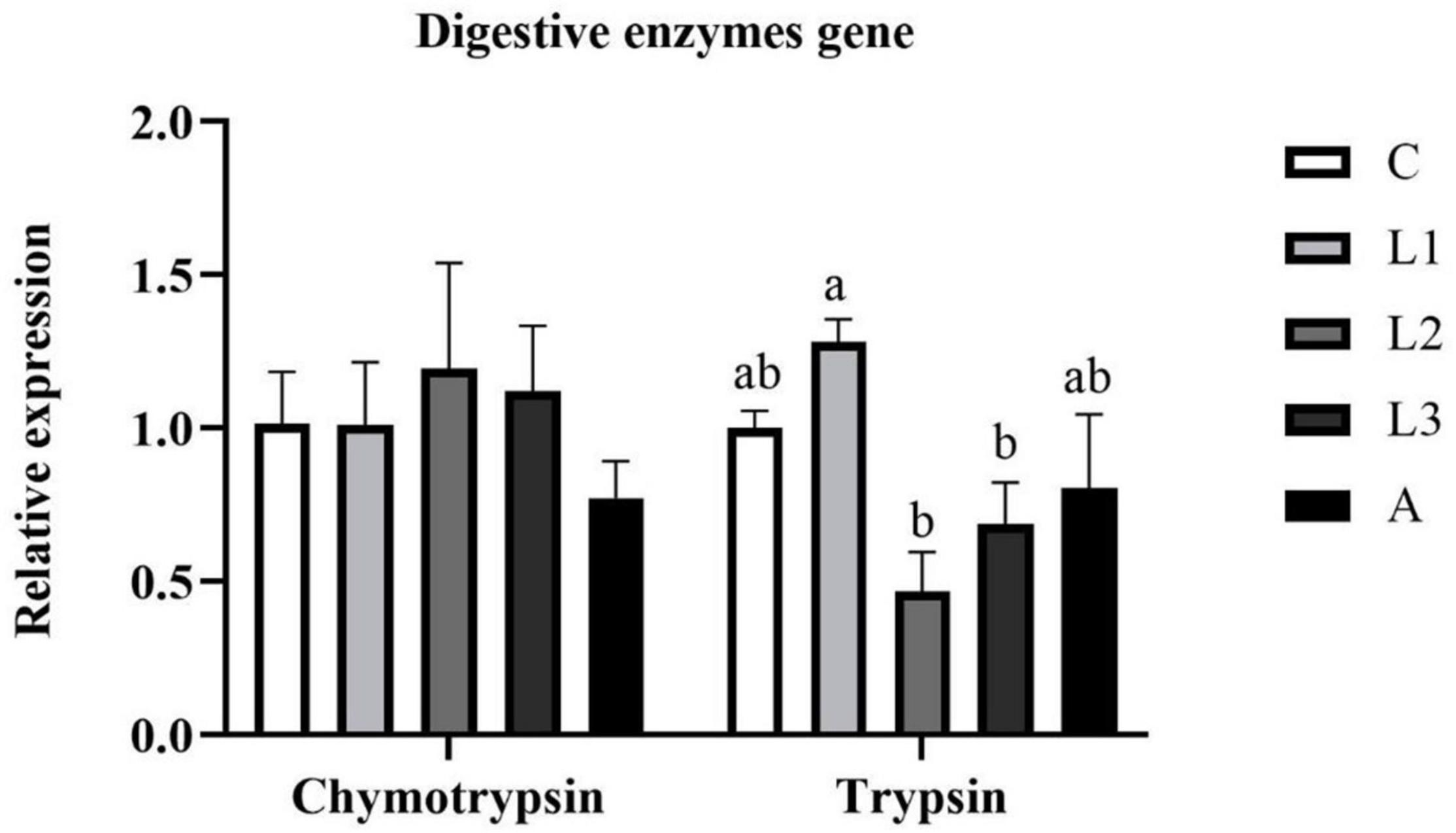
Figure 4. Hepatopancreas RNA expression level of digestive enzyme genes of L. vannamei fed diet supplementation with/without lutein or astaxanthin for 56 days.
Hepatopancreas RNA expression levels of metabolic enzyme genes [Hexokinase (HK) and Fatty acid synthase (FAS)] of L. vannamei fed diet supplemented with/without lutein or astaxanthin were shown in Figure 5. Regarding the RNA expression level of HK, the data in the L1 and L2 groups were significantly higher than that of the C and A groups (P < 0.05). In addition, the RNA expression level of FAS in the L2 and A groups was higher than that in the C group (P < 0.05), and statistical difference was not found between the L2 and A group (P > 0.05).
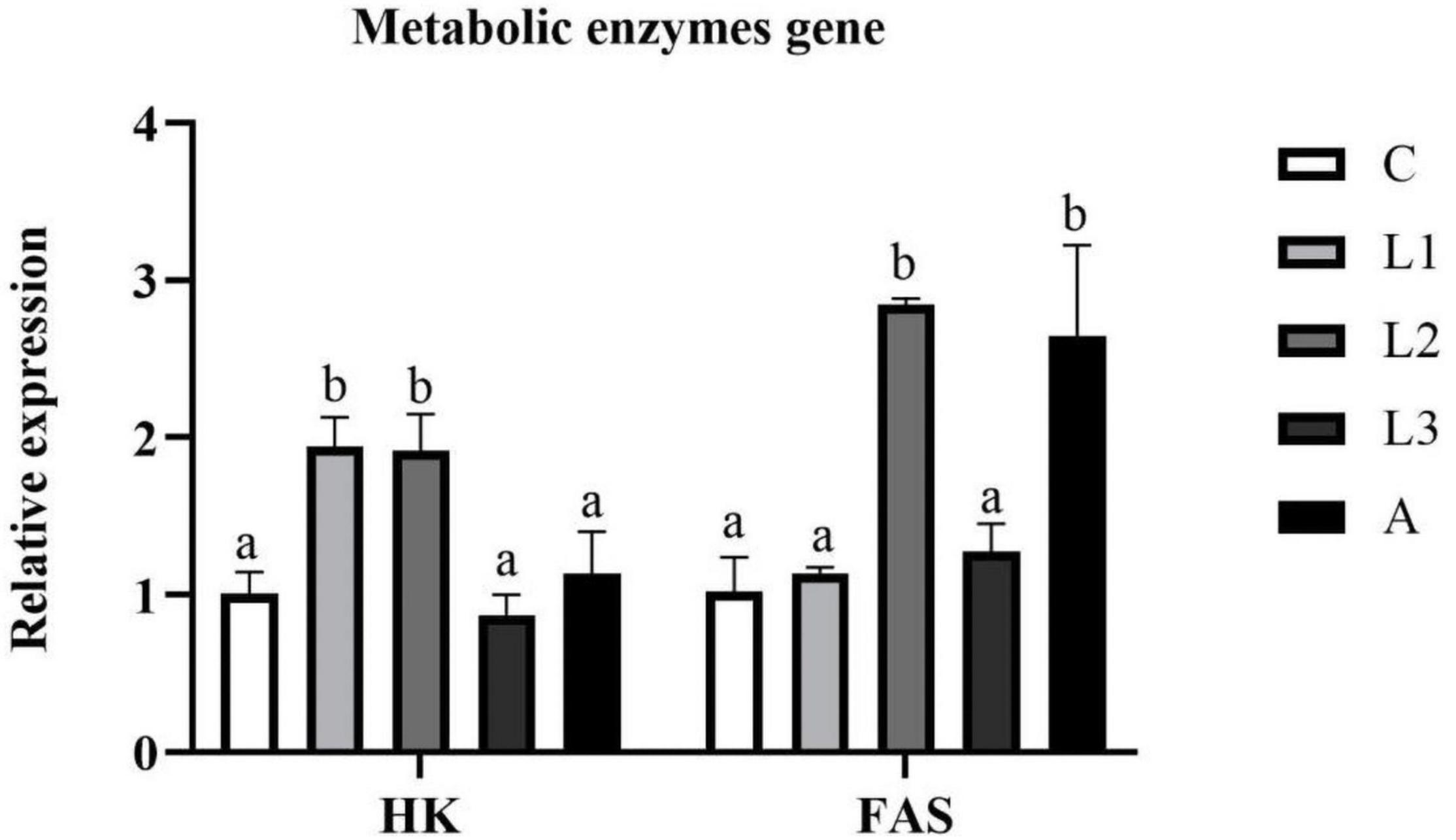
Figure 5. Hepatopancreas RNA expression level of metabolic enzymes genes of L. vannamei fed diet supplementation with/without lutein or astaxanthin for 56 days.
Discussion
Diet supplementation with carotenoid pigment could improve the growth performance of the aquatic animals. For example, dietary astaxanthin supplementation could optimize the growth performance of Marsupenaeus japonicus (Wang et al., 2018), Paralithodes camtschaticus (Daly et al., 2013), Trachinotus ovatus (Fang et al., 2021a) and Micropterus salmoides (Xie S. et al., 2020). In addition, diet supplementation with β-carotene also enhanced the growth performance of Penaeus monodon (Niu et al., 2014), Piaractus mesopotamicus (Bacchetta et al., 2019) and Oreochromis niloticus × O. aureus (Hu et al., 2006). Similar results were shown in the present study. Carotenoid pigment-supplemented groups except for the L3 group all showed significantly higher growth performance (WGR and SGR) of L. vannamei than the C group. There were two main reasons for why carotenoid pigments could improve the growth performance of crustaceans. Firstly, the carotenoid pigment could regulate the metabolism of aquatic animals and thus promote the digestion of nutrients (Baron et al., 2008; Zhang et al., 2013). In addition, the carotenoid pigment could shorten the molt cycle interval of crustaceans and regulate the NADPH metabolism for reducing energy consumption, resulting in enhanced growth performance (Hertrampf and Piedad-Pascual, 2012; Mao et al., 2017). Meanwhile, in the current study, similar growth performances (WGR and SGR), FCR, and SR of L. vannamei were found in the L1-2 groups and A group, indicating that diet supplementation with lutein ranging from 62.5 to 75 ppm could substitute astaxanthin in the diets of L. vannamei. However, previous studies also showed that carotenoid pigment was unable to alter the growth performance of aquatic animals. For example, dietary astaxanthin could not improve the growth performance of Portunus trituberculatus (Han et al., 2018), Eriocheir sinensis (Jiang et al., 2020), Symphysodon spp. (Song et al., 2017), and Pagrus pagrus (Nogueira et al., 2021). These results might be attributed to the culture environment, aquatic animal species and carotenoid pigment doses.
Nutrient digestion and metabolism capacity in aquatic animals are also crucial parameters for evaluating a diet additive. In the present study, no significant differences in RNA expression level of Chymotrypsin and Trypsin were found between the four carotenoid pigment treatment groups and the C group, indicating that dietary carotenoid pigment was unable to alter the protein digestive capacity of L. vannamei. In terms of metabolic enzyme genes, HK is a critical rate-limiting enzyme in regulating the glycolysis cycle; FAS plays a significant role in catalyzing the synthesis of the fatty acids (Chirala and Wakil, 2004; Guo et al., 2008). In the present study, RNA expression level of HK in the L1 and L2 groups was higher than in other groups, indicating that diet supplementation of lutein ranging from 62.5 to 75 ppm could improve the capacity of carbohydrate metabolism. In addition, RNA expression level of FAS in the L2 and A groups was significantly higher than in other groups, meaning that lutein and astaxanthin could improve the fat synthesis ability of L. vannamei.
During the feeding trial, the shrimp might be affected by several uncontrollable external factors, such as water temperature change, bacterial contaminations in aquatic water and feed, as well as stress caused by the feeding process. In this situation, oxidative burst would occur in cells to produce numerous reactive oxygen species (ROS) to protect the organism from oxidative stress (Wilhelm Filho, 2007; Banh et al., 2016). However, ROS overproduction may cause DNA damage and other severe detriments in cells (Piconi et al., 2006; de Souza Grinevicius et al., 2016). If cells were exposed to excess ROS, they would activate the antioxidant system and upregulate the expression of antioxidant genes and enzymes, like SOD, GSH-PX, and CAT (Ighodaro and Akinloye, 2018). In addition, T-AOC was also a significant parameter to reflect the antioxidant capacity of crustaceans (Dai et al., 2018). Since carotenoid pigment contains ionone rings with hydroxyl and keto, they could remove ROS and protect cells from various environmental stress (Chien et al., 2003). In the current study, compared to the control group, significantly lower levels of hepatopancreas T-SOD activity, hemolymph T-AOC and RNA expression of GSH-Px and CAT were observed in four carotenoids pigments-supplemented groups, indicating lutein and astaxanthin could act as an antioxidant for scavenging ROS and thus protecting cells from oxidative stress. As a result, the cells unnecessarily stimulate the antioxidant system and produce more antioxidant enzymes. These results were similar to the previous results reproted by Zhang et al. (2013), Xie J. et al. (2020), and Ettefaghdoost and Haghighi (2021). MDA is a product of lipid peroxidation, which can be used to evaluate the damage degree of cell structure and function (Çilingir Yeltekin and Oğuz, 2018). The present study demonstrated that significantly lower hemolymph MDA was obtained in four carotenoid pigment treatment groups than the C group, indicating lutein and astaxanthin could improve the antioxidant capacity of L. vannamei. No significant statistical difference in hemolymph T-AOC, MDA, and hepatopancreas RNA expression level of GSH-PX, CAT between dietary lutein treatment groups (L1-3) and the astaxanthin group was found in the present study, indicating that dietary supplementation with lutein ranging from 62.5 to 87.5 ppm could obtain similar antioxidant effects in L. vannamei compared to astaxanthin.
The antioxidant property of lutein and astaxanthin had been widely reported (Zhang et al., 2013; Rahman et al., 2016; Song et al., 2017). However, few studies focused on anti-inflammation and other immune functions of these carotenoid pigments. The NF-κB pathway is an essential moderator related to inflammatory responses, and Relish as well as Rho are two essential NF-κB family proteins (Ko et al., 2017; Xie et al., 2018). In addition, HSP70 could also exert the anti-inflammatory property by stimulating its IL-10 producing T cells (Wendling et al., 2000; Tanaka et al., 2007). Lower expression of inflammatory cytokines or higher expression of anti-inflammatory cytokines can suppress the inflammatory response (Zhao et al., 2020). In the present study, significantly lower RNA expression level of Relish was found in four carotenoid pigment treatment groups than in the C group, indicating lutein and astaxanthin could improve the anti-inflammation capacity of L. vannamei by regulating the NF-κB pathway. Caspases, which belong to the cysteine proteases family, are essential regulators of programmed cell death (apoptosis) (Taylor et al., 2008). In general, Caspase 2 was a factor which mainly responded to stress-induced apoptosis, while Caspase 3 frequently acted as a proenzyme and was activated to destroy various specific cellular structures and proteins (Dorstyn et al., 2012; Gao et al., 2013). The present study demonstrated significantly lower RNA expression level of Caspase 3 in four carotenoid pigment treatment groups than in the control group, indicating diet supplementation of lutein or astaxanthin could inhibit the apoptosis of L. vannamei. In addition, no statistical differences in Relish, HSP70 and Caspase 3 were observed among L1-L3 and A groups, meaning that diet supplementation of lutein ranging from 62.5 to 87.5 ppm could stimulate similar immune response in L. vannamei compared to diet supplemented with astaxanthin.
Conclusion
Overall, diet supplementation of lutein ranging from 62.5 to 75 ppm could result in similar growth performance, antioxidant capacity and immune response of L. vannamei compared with diet supplemented with 50 ppm astaxanthin. Therefore, dietary lutein was suggested to be suitable in the diet of L. vannamei for substituting astaxanthin.
Data Availability Statement
The original contributions presented in the study are included in the article/supplementary material, further inquiries can be directed to the corresponding author/s.
Ethics Statement
The animal study was reviewed and approved by Experimental Animal Ethics Committee of Sun Yat-sen University.
Author Contributions
HF, YL, and LT designed the study. HZ provided relative experiment material. HF, XH, and JN analyzed data. HF carried out the experiment and wrote this manuscript. All authors contributed to the article and approved the submitted version.
Funding
This work was supported by the Fund of China Agriculture Research System of MOF and MARA 48 (CARS 48), Project of Science and Technology of Guangdong Province (2019B110209005), Project of Science and Technology of Guangdong Province (2021B0202050002), and Youth Science and Technology Innovation Talent of Guangdong TeZhi Plan Talent (2019TQ05N129).
Conflict of Interest
HZ was employed by company Guangzhou Leader Bio-Technology Co., Ltd.
The remaining authors declare that the research was conducted in the absence of any commercial or financial relationships that could be construed as a potential conflict of interest.
Publisher’s Note
All claims expressed in this article are solely those of the authors and do not necessarily represent those of their affiliated organizations, or those of the publisher, the editors and the reviewers. Any product that may be evaluated in this article, or claim that may be made by its manufacturer, is not guaranteed or endorsed by the publisher.
References
Bacchetta, C., Rossi, A. S., Cian, R. E., Drago, S. R., and Cazenave, J. (2019). Dietary β-carotene improves growth performance and antioxidant status of juvenile Piaractus mesopotamicus. Aquac. Nutr. 25, 761–769. doi: 10.1111/anu.12893
Banh, S., Wiens, L., Sotiri, E., and Treberg, J. R. (2016). Mitochondrial reactive oxygen species production by fish muscle mitochondria: potential role in acute heat-induced oxidative stress. Comp. Biochem. Physiol. B Biochem. Mol. Biol. 191, 99–107. doi: 10.1016/j.cbpb.2015.10.001
Baron, M., Davies, S., Alexander, L., Snellgrove, D., and Sloman, K. A. (2008). The effect of dietary pigments on the coloration and behaviour of flame-red dwarf gourami, Colisa lalia. Anim. Behav. 75, 1041–1051.
Boonyaratpalin, M., Thongrod, S., Supamattaya, K., Britton, G., and Schlipalius, L. E. (2001). Effects of β-carotene source, Dunaliella salina, and astaxanthin on pigmentation, growth, survival and health of Penaeus monodon. Aquac. Res. 32, 182–190.
Chien, Y. H., Pan, C. H., and Hunter, B. (2003). The resistance to physical stresses by Penaeus monodon juveniles fed diets supplemented with astaxanthin. Aquaculture 216, 177–191.
Chirala, S. S., and Wakil, S. J. (2004). Structure and function of animal fatty acid synthase. Lipids 39, 1045–1053. doi: 10.1007/s11745-004-1329-9
Çilingir Yeltekin, A., and Oğuz, A. R. (2018). Antioxidant responses and DNA damage in primary hepatocytes of Van fish (Alburnus tarichi, Güldenstadt 1814) exposed to nonylphenol or octylphenol. Drug Chem. Toxicol. 41, 415–423. doi: 10.1080/01480545.2018.1461899
Dai, J., Zhang, L., Du, X., Zhang, P., Li, W., Guo, X., et al. (2018). Effect of lead on antioxidant ability and immune responses of crucian carp. Biol. Trace Elem. Res. 186, 546–553. doi: 10.1007/s12011-018-1316-z
Daly, B., Swingle, J. S., and Eckert, G. L. (2013). Dietary astaxanthin supplementation for hatchery-cultured red king crab, P aralithodes camtschaticus, juveniles. Aquac. Nutr. 19, 312–320. doi: 10.1111/j.1365-2095.2012.00963.x
de Souza Grinevicius, V. M. A., Kviecinski, M. R., Santos Mota, N. S. R., Ourique, F., Porfirio Will, Castro, L. S. E., et al. (2016). Piper nigrum ethanolic extract rich in piperamides causes ROS overproduction, oxidative damage in DNA leading to cell cycle arrest and apoptosis in cancer cells. J. Ethnopharmacol. 189, 139–147. doi: 10.1016/j.jep.2016.05.020
Dorstyn, L., Puccini, J., Wilson, C. H., Shalini, S., Nicola, M., Moore, S., et al. (2012). Caspase-2 deficiency promotes aberrant DNA-damage response and genetic instability. Cell Death Differ. 19, 1288–1298. doi: 10.1038/cdd.2012.36
Ettefaghdoost, M., and Haghighi, H. (2021). Impact of different dietary lutein levels on growth performance, biochemical and immuno-physiological parameters of oriental river prawn (Macrobrachium nipponense). Fish Shellfish Immunol. 115, 86–94. doi: 10.1016/j.fsi.2021.05.024
Fang, H., Xie, J., Liao, S., Guo, T., Xie, S., Liu, Y., et al. (2019). Effects of Dietary Inclusion of Shrimp Paste on Growth Performance, Digestive Enzymes Activities, Antioxidant and Immunological Status and Intestinal Morphology of Hybrid Snakehead (Channa maculata ♀ × Channa argus ♂). Front. Physiol. 10:1027. doi: 10.3389/fphys.2019.01027
Fang, H., Zhao, W., Xie, J., Yin, P., Zhuang, Z., Liu, Y., et al. (2021b). Effects of dietary lipid levels on growth performance, hepatic health, lipid metabolism and intestinal microbiota on Trachinotus ovatus. Aquac. Nutr. 27:13296. doi: 10.1111/anu.13296
Fang, H., Xie, J., Zhao, W., Liu, Z., Liu, Y., Tian, L., et al. (2021a). Study Supplementation of Astaxanthin in High-fat Diet on Growth Performance, Antioxidant ability, Anti-inflammation, Non-specific Immunity and Intestinal Structure of Juvenile Trachinotus Ovatus. United States: Wiley. 1–12. doi: 10.1111/anu.13386
Gao, D., Zhang, X., Wang, H., Wang, Y., and Min, W. (2013). Molecular cloning, immunohistochemical localization, characterization and expression analysis of caspase-9 from the purse red common carp (Cyprinus carpio) exposed to cadmium. Aquat. Toxicol. 142, 53–62. doi: 10.1016/j.aquatox.2013.07.017
Giuliano, G., Aquilani, R., and Dharmapuri, S. (2000). Metabolic engineering of plant carotenoids. Trends Plant Sci. 5, 406–409. doi: 10.1016/s1360-1385(00)01749-0
Guo, B., Wang, F., Hou, C. Q., Dong, S., and Sun, H. (2008). Effects of acute temperature fluctuation on HK and PK activity, HSP70 relative content in Litopenaeus vannamei. J. Fish. Sci. China 15, 885–889.
Han, T., Li, X., Wang, J., Wang, C., Yang, M., and Zheng, P. (2018). Effects of dietary astaxanthin (AX) supplementation on pigmentation, antioxidant capacity and nutritional value of swimming crab, Portunus trituberculatus. Aquaculture 490, 169–177. doi: 10.1016/j.aquaculture.2018.02.030
Hertrampf, J. W., and Piedad-Pascual, F. (2012). Handbook on Ingredients for Aquaculture Feeds. Germany: Springer Science & Business Media.
Horwitz, W., Latimer, G. W., and AOAC International. (2010). Official Methods of Analysis of AOAC International. Gaithersburg: AOAC International. 1997.
Hu, C.-J., Chen, S.-M., Pan, C.-H., and Huang, C.-H. (2006). Effects of dietary vitamin A or β-carotene concentrations on growth of juvenile hybrid tilapia, Oreochromis niloticus×O. aureus. Aquaculture 253, 602–607. doi: 10.1016/j.aquaculture.2005.09.003
Ighodaro, O. M., and Akinloye, O. A. (2018). First line defence antioxidants-superoxide dismutase (SOD), catalase (CAT) and glutathione peroxidase (GPX): their fundamental role in the entire antioxidant defence grid. Alexandria J. Med. 54, 287–293. doi: 10.1016/j.ajme.2017.09.001
Jiang, X., Zu, L., Wang, Z., Cheng, Y., Yang, Y., and Wu, X. (2020). Micro-algal astaxanthin could improve the antioxidant capability, immunity and ammonia resistance of juvenile Chinese mitten crab, Eriocheir sinensis. Fish Shellfish Immunol. 102, 499–510. doi: 10.1016/j.fsi.2020.05.021
Kalinowski, C. T., Robaina, L. E., Fernandez-Palacios, H., Schuchardt, D., and Izquierdo, M. S. (2005). Effect of different carotenoid sources and their dietary levels on red porgy (Pagrus pagrus) growth and skin colour. Aquaculture 244, 223–231. doi: 10.1016/j.aquaculture.2004.11.001
Ko, E.-Y., Cho, S.-H., Kwon, S.-H., Eom, C.-Y., Jeong, M. S., Lee, W., et al. (2017). The roles of NF-κB and ROS in regulation of pro-inflammatory mediators of inflammation induction in LPS-stimulated zebrafish embryos. Fish Shellfish Immunol. 68, 525–529. doi: 10.1016/j.fsi.2017.07.041
Latscha, T. (1989). The role of astaxanthin in shrimp pigmentation. in Advances in Tropical Aquaculture, Workshop at Tahiti. French Polynesia 20:1989.
Mao, X., Guo, N., Sun, J., and Xue, C. (2017). Comprehensive utilization of shrimp waste based on biotechnological methods: a review. J. Clean. Prod. 143, 814–823.
Meilisza, N., Jusadi, D., Zairin, M. Jr., Artika, I. M., Priyo Utomo, N. B., Kadarini, T., et al. (2017). Digestibility, growth and pigmentation of astaxanthin, canthaxanthin or lutein diets in Lake Kurumoi rainbowfish, Melanotaenia parva (Allen) cultured species. Aquac. Res. 48, 5517–5525. doi: 10.1111/are.13372
Niu, J., Li, C.-H., Liu, Y.-J., Tian, L.-X., Chen, X., Huang, Z., et al. (2012). Dietary values of astaxanthin and canthaxanthin in Penaeus monodon in the presence and absence of cholesterol supplementation: effect on growth, nutrient digestibility and tissue carotenoid composition. Br. J. Nutr. 108, 80–91. doi: 10.1017/S0007114511005423
Niu, J., Wen, H., Li, C.-H., Liu, Y.-J., Tian, L.-X., Chen, X., et al. (2014). Comparison effect of dietary astaxanthin and β-carotene in the presence and absence of cholesterol supplementation on growth performance, antioxidant capacity and gene expression of Penaeus monodon under normoxia and hypoxia condition. Aquaculture 422, 8–17. doi: 10.1016/j.aquaculture.2013.11.013
Nogueira, N., Canada, P., Caboz, J., Andrade, C., and Cordeiro, N. (2021). Effect of different levels of synthetic astaxanthin on growth, skin color and lipid metabolism of commercial sized red porgy (Pagrus pagrus). Anim. Feed Sci. Technol. 276:114916. doi: 10.1016/j.anifeedsci.2021.114916
da Costa, D. P., and Miranda-Filho, K. C. (2020). The use of carotenoid pigments as food additives for aquatic organisms and their functional roles. Rev. Aquac. 12, 1567–1578. doi: 10.1002/mnfr.201600469
Petit, H., Nègre-Sadargues, G., Castillo, R., and Trilles, J.-P. (1997). The effects of dietary astaxanthin on growth and moulting cycle of postlarval stages of the prawn, Penaeus japonicus (Crustacea, Decapoda). Comp. Biochem. Physiol. PA Physiol. 117, 539–544.
Piconi, L., Quagliaro, L., Assaloni, R., Da Ros, R., Maier, A., Zuodar, G., et al. (2006). Constant and intermittent high glucose enhances endothelial cell apoptosis through mitochondrial superoxide overproduction. Diabetes. Metab. Res. Rev. 22, 198–203. doi: 10.1002/dmrr.613
Rahman, M. M., Khosravi, S., Chang, K. H., and Lee, S.-M. (2016). Effects of dietary inclusion of astaxanthin on growth, muscle pigmentation and antioxidant capacity of juvenile rainbow trout (Oncorhynchus mykiss). Prev. Nutr. food Sci. 21:281. doi: 10.3746/pnf.2016.21.3.281
Seabra, L. M. J., and Pedrosa, L. F. C. (2010). Astaxanthin: structural and functional aspects. Rev. Nutr. 23, 1041–1050. doi: 10.1590/s1415-52732010000600010
Seidgar, M. (2015). The effects of Fairy Shrimp Phallocryptus spinosa (Branchiopoda: Anostraca) as live food on reproduction performances and color of freshwater ornamental fish prawns. Int. J. Aquat. Sci. 6, 37–44.
Shahidi, F., and Brown, J. A. (1998). Carotenoid pigments in seafoods and aquaculture. Crit. Reveiws Food Sci. 38, 1–67. doi: 10.1080/10408699891274165
Sirirustananun, N., Chen, J.-C., Lin, Y.-C., Yeh, S.-T., Liou, C.-H., Chen, L.-L., et al. (2011). Dietary administration of a Gracilaria tenuistipitata extract enhances the immune response and resistance against Vibrio alginolyticus and white spot syndrome virus in the white shrimp Litopenaeus vannamei. Fish Shellfish Immunol. 31, 848–855. doi: 10.1016/j.fsi.2011.07.025
Song, X., Wang, L., Li, X., Chen, Z., Liang, G., and Leng, X. (2017). Dietary astaxanthin improved the body pigmentation and antioxidant function, but not the growth of discus fish (Symphysodon spp.). Aquac. Res. 48, 1359–1367. doi: 10.1007/s10295-018-2104-7
Tanaka, K.-I., Namba, T., Arai, Y., Fujimoto, M., Adachi, H., Sobue, G., et al. (2007). Genetic evidence for a protective role for heat shock factor 1 and heat shock protein 70 against colitis. J. Biol. Chem. 282, 23240–23252. doi: 10.1074/jbc.M704081200
Taylor, R. C., Cullen, S. P., and Martin, S. J. (2008). Apoptosis: controlled demolition at the cellular level. Nat. Rev. Mol. cell Biol. 9, 231–241. doi: 10.1038/nrm2312
Wang, W., Ishikawa, M., Koshio, S., Yokoyama, S., Sakhawat Hossain, M., and Moss, A. S. (2018). Effects of dietary astaxanthin supplementation on juvenile kuruma shrimp, Marsupenaeus japonicus. Aquaculture 491, 197–204. doi: 10.1016/j.aquaculture.2018.03.025
Wendling, U., Paul, L., van der Zee, R., Prakken, B., Singh, M., and van Eden, W. (2000). A conserved mycobacterial heat shock protein (hsp) 70 sequence prevents adjuvant arthritis upon nasal administration and induces IL-10-producing T cells that cross-react with the mammalian self-hsp70 homologue. J. Immunol. 164, 2711–2717. doi: 10.4049/jimmunol.164.5.2711
Wilhelm Filho, D. (2007). Reactive oxygen species, antioxidants and fish mitochondria. Front Biosci 12, 1229–1237. doi: 10.2741/2141
Xie, J., Fang, H., He, X., Liao, S., Liu, Y., Tian, L., et al. (2020). Study on mechanism of synthetic astaxanthin and Haematococcus pluvialis improving the growth performance and antioxidant capacity under acute hypoxia stress of golden pompano (Trachinotus ovatus) and enhancing anti-inflammatory by activating Nrf2-ARE path. Aquaculture 518:734657. doi: 10.1016/j.aquaculture.2019.734657
Xie, S., Yin, P., Tian, L., Yu, Y., Liu, Y., and Niu, J. (2020). Dietary Supplementation of Astaxanthin Improved the Growth Performance, Antioxidant Ability and Immune Response of Juvenile Largemouth Bass (Micropterus salmoides) Fed High-Fat Diet. Mar. Drugs 18:642. doi: 10.3390/md18120642
Xie, S., Fang, W., Wei, D., Liu, Y., Yin, P., Niu, J., et al. (2018). Dietary supplementation of Haematococcus pluvialis improved the immune capacity and low salinity tolerance ability of post-larval white shrimp, Litopenaeus vannamei. Fish Shellfish Immunol. 80, 452–457. doi: 10.1016/j.fsi.2018.06.039
Zhang, J., Liu, Y., Tian, L., Yang, H., Liang, G., Yue, Y., et al. (2013). Effects of dietary astaxanthin on growth, antioxidant capacity and gene expression in P acific white shrimp L itopenaeus vannamei. Aquac. Nutr. 19, 917–927. doi: 10.1111/anu.12037
Zhao, W., Fang, H.-H., Gao, B.-Y., Dai, C.-M., Liu, Z.-Z., Zhang, C.-W., et al. (2020). Dietary Tribonema sp. supplementation increased growth performance, antioxidant capacity, immunity and improved hepatic health in golden pompano (Trachinotus ovatus). Aquaculture 529:735667. doi: 10.1016/j.aquaculture.2020.735667
Keywords: lutein, L. vannamei, astaxanthin, growth performance, antioxidant capacity, immunity
Citation: Fang H, He X, Zeng H, Liu Y, Tian L and Niu J (2021) Replacement of Astaxanthin With Lutein in Diets of Juvenile Litopenaeus vannamei: Effects on Growth Performance, Antioxidant Capacity, and Immune Response. Front. Mar. Sci. 8:803748. doi: 10.3389/fmars.2021.803748
Received: 28 October 2021; Accepted: 16 November 2021;
Published: 13 December 2021.
Edited by:
Jun Wang, Chinese Academy of Fishery Sciences (CAFS), ChinaReviewed by:
Mingchun Ren, Chinese Academy of Fishery Sciences (CAFS), ChinaChangle Qi, Huzhou University, China
Houguo Xu, Chinese Academy of Fishery Sciences (CAFS), China
Copyright © 2021 Fang, He, Zeng, Liu, Tian and Niu. This is an open-access article distributed under the terms of the Creative Commons Attribution License (CC BY). The use, distribution or reproduction in other forums is permitted, provided the original author(s) and the copyright owner(s) are credited and that the original publication in this journal is cited, in accordance with accepted academic practice. No use, distribution or reproduction is permitted which does not comply with these terms.
*Correspondence: Jin Niu, bml1ajNAbWFpbC5zeXN1LmVkdS5jbg==