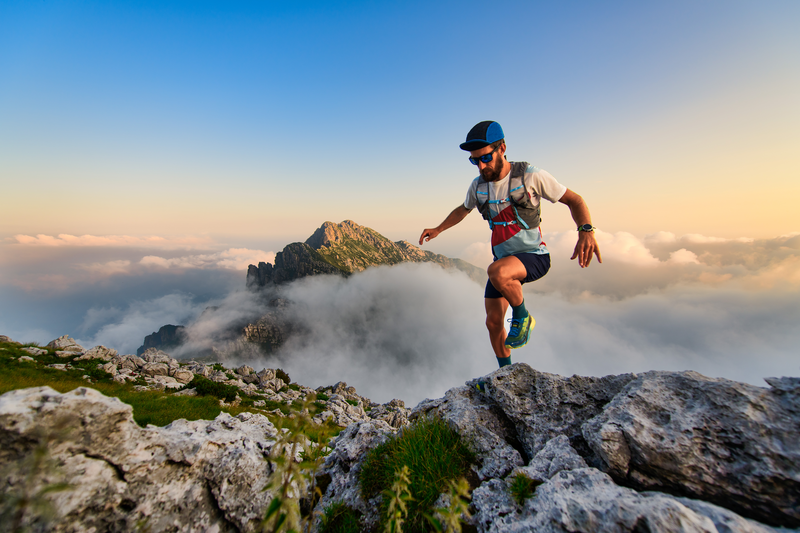
95% of researchers rate our articles as excellent or good
Learn more about the work of our research integrity team to safeguard the quality of each article we publish.
Find out more
ORIGINAL RESEARCH article
Front. Mar. Sci. , 21 January 2022
Sec. Marine Fisheries, Aquaculture and Living Resources
Volume 8 - 2021 | https://doi.org/10.3389/fmars.2021.800627
This article is part of the Research Topic Mollusk Breeding and Genetic Improvement View all 25 articles
Industrial farming is an alternative mode for Pinctada maxima juvenile cultivation to avoid mass mortality caused by natural disasters. Suitable and enough food is crucial for successful industrial bivalve farming. To investigate the feasibility of live microalga instead of spray-dried microalgal powder in P. maxima juvenile industrial farming, this study replaces a positive control live microalgal diet [Isochrysis zhanjiangensis (L-iso) and Platymonas subcordiformis (L-pla)] with spray-dried I. zhanjiangensis powder (P-iso) and P. subcordiformis powder (P-pla). Continuous feeding trials (30 days) were conducted on the P. maxima juvenile (1.2008 ± 0.0009 g initial weight and 30.12 ± 0.05 mm initial shell length), under laboratory conditions. Survival, growth performance, and intestinal microbial community were studied and compared across the groups. Results showed that survival rate (SR) did not differ significantly across the groups (ranged from 84 to 86%, P > 0.05). The growth performance in spray-dried microalgal groups, including total weight (TW), shell height (SH), absolute growth rate (AGR), and relative growth rate (RGR) for SH and TW, was slightly lower than that in live microalgal groups, while the activities of pepsin (PES), amylase (AMS), and lipase (LPS) were significantly higher (P < 0.05). The best growth performance was observed in the L-iso group, followed by the L-pla group. A 16S rRNA-based sequencing revealed that Proteobacteria was the dominant phylum in P. maxima juvenile intestinal bacterial community under controlled conditions, which accounted for 62–82% across groups. The intestinal bacteria at the genus level were more sensitive to diets, whereas Burkholderia was the dominant genus in both L-iso (66.52 ± 6.43%) and L-pla groups (54.00 ± 5.66%), while Mycoplasma, Alphaproteobacteria, and Oxyphotobacteria were in both P-iso and P-pla groups. The P-pla group got higher ACE, Chao1, and Simpson and Shannon indices (P < 0.05). The above results suggested that the spray-dried P-iso and P-pla can serve as substitutes for live microalga in P. maxima juvenile industrial farming under controlled conditions. The finding in this study provides basic data to optimize industrial farming technology and healthy management for P. maxima juvenile.
The mollusks are the second-largest animal phylum with almost 100,000 species, of which 15,000 are bivalves (Barnes et al., 1993), and bivalves culturing is considered a sustainable maricultural practice as most bivalves obtain nutrition from natural seawater without artificial food input (Zhou et al., 2006; Zheng et al., 2020). Pearl oyster Pinctada maxima is known for producing large-sized nucleated pearls, which are among the most expensive pearls worldwide (Zhao et al., 2003). The species is naturally distributed in the central Indo-Pacific region from Myanmar to the Solomon Islands, including the Philippines, China, Australia, Papua New Guinea, Indonesia, Polynesia, Micronesia, and Southern Japan (Liang et al., 2016; He et al., 2021). In China, P. maxima exists naturally along with the coastal areas of southern provinces, such as Hainan, Guangxi, and Guangdong provinces. In addition, researchers have studied the protocols for seed production and pearl culturing of P. maxima since the early 1970s, while pearl production in this species was experimentally successful in the 1980s. However, the P. maxima pearl production industry has developed at a slow pace over the past decades, for overfished wild populations and mass mortality at juvenile stages. Aiming to solve this problem, researchers improved the survival rates (SRs) by developing rearing protocols for larvae, spat, and adults, as well as introducing wild populations (Liang et al., 2016).
Raft and pile farming offshore are the dominant farming modes of bivalves that are susceptible to natural disasters and pollution of the sea area. In contrast, the industrial farming mode can effectively reduce or avoid the above disadvantages, involving a combination of culture engineering and water treatment protocols (Wang et al., 2015). Under the industrial farming mode, the animals can be fed on high-quality diets to improve survival and growth rates. Pearl oyster P. maxima cultivation in China generally occurs in inshore or nearshore estuarine areas, where salinity fluctuates due to heavy rains and runoff in the summer months. The changes in environmental factors are bad for P. maxima juvenile survival and growth, even causing mass mortality (Deng et al., 2013). Thus, the industrial farming mode is an alternative and appropriate mode for P. maxima farming. In general, the industrial farming mode involves high stocking and requires a large demand for food diets. Phytoplankton, bacteria, organic debris, and microzooplankton from the sea are the food sources for bivalves, and phytoplankton is the dominant (Wang et al., 2010). However, diets that solely comprise microalgae can hardly meet the demand in bivalve industrial farming, and large-scale microalgal culture is often susceptible to changes in water temperature and salinity. Therefore, choosing suitable food instead of live microalga, without affecting growth, physiology, and meeting nutritional requirements from bivalves, is crucial for the industrial bivalve farming mode development.
The partial or total replacement of microalgal foods by a cheap, easily handled substitute with the same nutritive qualities has been attempted by hatcheries, while studies on the nutritional requirements and artificial feed of bivalve are relatively more laggard than those on other aquatic animals. A few studies of artificial diets in bivalve species have been reported, such as bacterial proteins (Philippe and Christopher, 1994), yeasts (Nell et al., 1996), microalgal concentrates (Brown and Robert, 2002), spray-dried microalgae (Arney et al., 2015), and so on. Substituting 50% of the traditional microalgae with single-cell detritus produced from seaweed (Porphyra haitanensis) can be used as a partial microalgal substitute for the nursery culture of the tropical oyster (Crassostrea belcheri) juvenile (Tanyaros and Chuseingjaw, 2016). Palatable artificial diets have good potential for replacement of costly natural diets of the green-lipped mussel Perna canaliculus post-settlement juveniles (Gui et al., 2016a). Chlorella sp. powder, Spirulina platensis powder, yeast powder, and soybean meal are suitable protein sources in diets for pearl oyster Pinctada fucata martensii (Yang et al., 2017a). Microalgae are still the main nutrient sources for pipi clam, Paphies australis, particularly for spat and juveniles, while corn flour should be considered in the formulation of diets for laboratory grown (Mamat and Alfaro, 2014).
However, information regarding artificial feed, reliable to substitute for live microalgal feed for pearl oyster P. maxima, is rare. In this study, we have attempted to explore the feasibility of using spray-dried microalgae as daily food in P. maxima juvenile industrial farming and developed industrial farming methods and technology for P. maxima juvenile. The growth, digestive enzyme activity, and intestinal microbial community were characterized under laboratory-controlled conditions in this study. To our knowledge, this study provides the first insights into the impacts of diets on intestinal bacteria of P. maxima, where the findings can provide some information on rearing protocols and management of industrial farming mode.
The P. maxima juveniles were obtained from Sanya Maifeng Industrial Co., Ltd. (Hainan, China). Before the experiment, the juveniles were kept in an aerated cement tank (28 ± 2°C, 30.0 ± 2 psu, pH 8.0 ± 0.2) for 7 days, fed daily with a mixture of Isochrysis zhanjiangensis and Platymonas subcordiformis. A total of 30% water exchange were taken every day to promise that the water quality is good enough for P. maxima juveniles, where total ammonia-nitrogen <0.05 mg/L and DO (dissolved oxygen) ≥7 mg/L. Then, P. maxima juveniles were divided into four rearing groups in triplicates for experiments, according to different feeding strategies. Triplicate tanks (500 L) containing 300 juveniles were used for each rearing group. A total of 3,600 P. maxima juveniles were used in this study, with 1.2008 ± 0.0009 g initial weight and 30.12 ± 0.05 mm initial shell length.
The trials of 30 days were carried out. Two species of live microalgae (Isochrysis zhanjiangensis and Platymonas subcordiformis) were used in the experimental groups, respectively, the live I. zhanjiangensis group (L-iso group) and P. subcordiformis group (L-pla group). However, spray-dried microalgal powders (I. zhanjiangensis powder and P. subcordiformis powder) were used for the other two groups, namely, powder I. zhanjiangensis group (P-iso group) and powder P. subcordiformis group (P-pla group).
Microalgal stock cultures were obtained from the Microalgae Laboratories of Ocean College in Hainan University. These microalgae were cultured in 5 and 45 L glass buckets and grown at 25.0–27.0°C in Ningbo 3# nutrient medium. The photoperiod was 24:0 h (light/dark), and illumination was provided by 2-day light (40-W) fluorescent tubes. Filtered (0.45 mm) and UV-treated seawater (salinity, 30.2 psu) was used. Continuous aeration was provided to enhance growth and prevent the algae from settling. The microalgae were harvested during an exponential phase for feeding. The spray-dried microalgal powder was provided by SDIC Biotech Investment Co., Ltd. (Beijing, China).
To control the final concentration of microalgae in the experimental tanks, the feeding quantity was determined by measuring the original concentration of the different microalgal diets precisely. Prior to daily feeding, 2 g of each spray-dried diet was suspended in 1,000 ml of filtered seawater and pulsed in a commercial blender (JS39D-250, Supor, China). The liquefied mixture was filtered through a 50-μm mesh to eliminate foam and larger particles and then diluted to 15 L with filtered seawater. The diluted suspension was used in feeding. All diets were fed in culture media at a concentration of 150,000 cells/ml once every 12 h. The cell counts were made using a hemocytometer.
After the end of the experiment, the surviving pearl oyster, P. maxima, in each group was counted to calculate the SR. SR was calculated using the following equation: Survival rate = (the number of surviving individuals at the end of the experiment/the number of individuals at the beginning of the experiment) × 100%.
The shell height (SH) was measured using a Vernier caliper (an accuracy of 0.01 mm). In addition, the total weight (TW) was weighed using an electronic balance (an accuracy of 0.0001 g). The absolute growth rate (AGR) and relative growth rate (RGR) were calculated for SH and TW using the following formulas: AGR = (X2 − X1)/t, RGR = 100% × (X2 − X1)/(t × X1), where “X1” and “X2” are the average of SH (or TW) at the beginning and the end of the experiment, respectively, and “t” is the experimental days.
All experiments were complied with the standards of the Guidance of the Care and Use of Laboratory Animals in China. After the end of the culture, the intestinal tissue of each pearl oyster was dissected in a sterile environment and stored in liquid nitrogen for further bacterial community analysis, while soft tissues except the intestinal tissue and gill were used for digestive enzyme activity assay.
The frozen soft tissues were homogenized on ice in 0.2 M (w/v) of ice-cold physiological saline (Gawlicka et al., 2000), and homogenates were centrifuged at 13,000 g for 10 min at 2°C. The supernatant was separated for enzyme analysis in triplicate. The activities of pepsin (PES, E.C.3.4.23.1), amylase (AMS, E.C.3.2.1.1), and lipase (LPS, E.C.3.1.1.3) were used as biochemical indicators and assayed by the commercial kits from Nanjing Jiancheng Bioengineering Institute (Nanjing, China).
Microbial DNA was extracted from intestinal contents using the EZNA Stool DNA Kit (Omega Bio-tek, Norcross GA, United States). The final DNA concentration was quantified by using Qubit 3.0 Fluorometer (Invitrogen, United States) and agarose gel electrophoresis (Zheng et al., 2019).
A total of 50 ng DNA were used to generate amplicons. The V3–V4 region of the bacteria 16S ribosomal RNA gene was amplified by PCR, using the forward primers containing the sequence 341F (5′-CCTACGGGNGGCWGCAG-3′) and reverse primers containing the sequence 805R (5′-GACTACHVGGGTATCTAATCC-3′). The marker region of bacteria was amplified by PCR (thermal cycling program: 98°C for 30 s, 98°C for 10 s, followed by 35 cycles at 54°C for 30 s, 72°C for 45 s, and a final extension at 72°C for 10 min). The PCR reactions were performed in triplicate in a 12.5 μl mixture, containing 2.5 μl forward primer, 2.5 μl reverse primer, 25 μl dd H2O, and 50 ng template DNA. Amplicons were extracted from 2% agarose gel and purified using the QIAquick Gel Extraction Kit (Qiagen, Hilden, Germany) according to the instructions of the manufacturer. Purified products were subjected to Illumina MiSeq Sequencing platform (Hangzhou, Lianchuan Gene Technology Company., Ltd., China), generating paired-end reads.
Raw fasta files were demultiplexed, quality filtered, and analyzed using QIIME 2.0 (Bolyen et al., 2019). The 250 bp reads were truncated at any site of more than three sequential bases receiving a Phred quality score of <Q20. Any reads containing ambiguous base calls or barcode/primer errors were discarded. Operational taxonomic units (OTUs) were clustered with 97% similarity cutoff using UPARSE (version 7.1) (Edgar, 2013)1. Chimeric sequences were identified and removed using UCHIME. The phylogenetic affiliation of each 16S rRNA gene sequence was analyzed by SILVA Classifier (release 132) (Quast et al., 2013) against Silva 16S rRNA database using the confidence threshold of 70%. According to the SILVA (release 132) classifier, the characteristic abundance was performed with normalization by the relative abundance of each sample, and then, five indexes (ACE, Chao1, Shannon, Simpson, and Goods coverage) were calculated using QIIME2 for alpha diversity analysis. Rarefaction analysis was also performed using the Mothur program. The structures of the microbial community in different samples were compared through column diagrams and principal component analysis (PCA).
The data in this study were expressed as mean ± SE. For all statistical tests, P-values <0.05 were considered to be significant. Significant differences of each variable were first detected using the t-test in enzyme activities among groups. The least significant difference multiple-range test was used to determine the significance of differences in growth, alpha diversity metrics, and the relative abundance of phylum or genus in the intestinal microbial community. Prior to analysis, data were tested for normality using Kolmogorov-Smirnov’s test and for homogeneity of variance using Cochran’s C test. Data that did not meet the ANOVA assumptions were log-transformed before analysis, and percentage data were arcsine square-root-transformed. All statistical analyses were performed using DPS14.5 software (Hangzhou Rui Feng Information Technology Co., Ltd., Hangzhou, China).
The SR was not affected by different diets used in this study (P > 0.05, Table 1). After 30-day industrial farming, the SR in all groups ranged from 84 to 86%, without significant difference. The growth performances in L-iso, P-iso, L-pla, and P-pla were different (P > 0.05, Table 1). The best growth performance was observed in the L-iso group, followed by the L-pla group. The TW in the L-iso group was 2.6503 ± 0.1702 g, significantly higher than P-iso and P-pla groups (P < 0.05), and TM for the L-pla group (2.5002 ± 0.0201 g) significantly higher than the P-pla group (2.2601 ± 0.0301, P < 0.05). The characteristics of the difference for AGR and RGR of TW in groups were found the same as TW. The largest SH was gained in L-iso and L-pla groups (32.3747 ± 0.7783 and 30.5350 ± 2.2149 mm, respectively), significantly higher than P-iso and P-pla groups (25.8183 ± 0.9737 and 25.8000 ± 0.3885 mm, respectively). The best AGR and RGR of SH was observed in the L-iso group (0.2525 ± 0.026 mm/day and 1.0345 ± 0.1063%/day respectively), followed by the L-pla group (0.1912 ± 0.0738 mm/day, 0.7832 ± 0.3025%/day, respectively). The significant difference for AGR and RGR of SH was not existed between P-iso and P-pla groups (P < 0.05).
Table 1. The survival and growth performance of Pinctada maxima juvenile after 30-day industrial farming.
Oyster filtered out all the diets fed in the experimental groups, without exception. The microalgal species, used as a daily food in this study, did not cause a significant difference in digestive enzyme activity of P. maxima juvenile after 30-day industrial farming (P > 0.05), while the microalgal types (live or spray-dried powder) did (P < 0.05, Figure 1). The PES and LPS activities in the P-iso group were observed highest (1.81 ± 0.20 and 24.22 ± 0.57 U/mg prot, respectively), followed by the P-pla group (1.74 ± 0.17 and 22.95 ± 1.95 U/mg prot, respectively), significantly higher than L-iso and L-pla groups (P < 0.05). In addition, the highest AMS activity (1.61 ± 0.29 U/mg prot) was found in the P-pla group, followed by the P-iso group (1.51 ± 0.20 U/mg prot), significantly higher than L-iso and L-pla groups (0.57 ± 0.05 and 0.72 ± 0.07 U/mg prot, respectively, P < 0.05).
Figure 1. The digestive enzyme activity of Pinctada maxima juvenile after 30-day industrial farming. The significance level of P < 0.05 was used for all statistical tests. Different lower-case letters indicate significant differences. (A) AMS activity, AMS = amylase; (B) LPS activity, LPS = lipase; (C) PES activity, PES = pepsin.
Through the detection of intestinal microbiota, a total of 1,134,712 high-quality sequences from nine samples were obtained, with an average of 75,647 sequences per sample-based. A total of 4,872 OTUs at 97% sequence similarity were obtained, with an average of 353 OTUs in each sample. In addition, rarefaction curves indicated that the obtained sequence could reflect the majority of bacteria diversity in each sample (Figure 2).
Figure 2. Rarefaction analyses of all samples. Rarefaction curves represented the number of OUT detected in the L-iso group (L-iso 1, L-iso 2, and L-iso 3), P-iso group (P-iso 1, P-iso 2, and P-iso 3), L-pla group (L-pla 1, L-pla 2, and L-pla 3), and P-pla group (P-pla 1, P-pla 2, and P-pla 3). Sequences were clustered at 97% sequence similarity. OTU, operational taxonomic unit.
In this study, results from the analysis of alpha diversity metrics showed that the diversity and richness of the intestinal microbial community had a significant difference in different diet groups (P < 0.05, Table 2). The ACE and Chao 1 were used as estimators of community richness, and Shannon and Simpson were used as estimators of community diversity. The ACE and Chao1 ranged from 331.92 ± 20.25 to 386.40 ± 20.42 and 331.37 ± 12.48 to 382.16 ± 19.19, respectively, where Chao1 in the P-iso group was significantly lower than other groups (P < 0.05). The Simpson in P-iso and P-pla groups was 0.73 ± 0.05, 0.77 ± 0.06, respectively, significantly higher than L-iso and L-iso groups (0.56 ± 0.03 and 0.77 ± 0.06, respectively, P < 0.05).
Table 2. OTUs, ACE, Chao 1, Simpson, Shannon, and Good’s coverage for 16s rRNA libraries of all samples.
Different diets could significantly affect the relative abundance of phylum or genus in the intestinal microbial community of P. maxima juvenile. A total of 31 phyla and 413 genera were detected in all groups (Figure 3, P < 0.05).
Figure 3. Composition of intestinal microflora of P. maxima fed with different diets. (A) Microbiota composition at the phylum level with relative abundance. (B) Microbiota composition at the genus level with relative abundance. “Others” meant the means of the sum of phylum or genus with relative abundance < 2% in L-iso, P-iso, L-pla, P-pla groups, respectively. (C) PCA analisys of bacteria community in different diet groups. The colored plot represents the bacteria from L-iso, P-iso, L-pla, and P-pla groups.
Proteobacteria was the most abundant phylum in all groups, relative abundance ranged from 62.31 ± 6.17% to 82.37 ± 9.33% (Figure 3A). In addition, Tenericutes was the core phylum both in L-pla and P-pla groups (10.19 ± 1.1% and 20.50 ± 2.05%, respectively). Furthermore, Chlamydiae (7.79 ± 0.67%) and Firmicutes (5.43 ± 0.66%) were the abundant phyla in the L-iso group, while Actinobacteria (3.28 ± 0.13%), Planctomycetes (6.40 ± 0.58%), and Chloroflexi (1.79 ± 0.11%) in the P-iso group and Cyanobacteria (10.52 ± 1.10%) in the P-pla group.
Burkholderia was the dominant genus in both L-iso (66.52 ± 6.43%) and L-pla groups (54.00 ± 5.66%), while Mycoplasma, Alphaproteobacteria, and Oxyphotobacteria were in both P-iso and P-pla groups. Furthermore, Simkaniaceae and Variovorax were the dominant genera in the L-iso group, and hence relative abundance was significantly higher than the other groups (P < 0.05). Amphritea, Cohaesibacter, and Kiloniella were the dominant genera in both P-iso and L-pla groups (Figure 3B).
In addition, the principal component analysis (PCA) indicated that the bacteria community in L-iso and L-pla groups clustered together, while P-iso and P-pla groups clustered together. The bacteria community in L-iso and L-pla groups widely differs from that in P-iso and P-pla groups (Figure 3C).
Survival and growth performances are the most important factors in the pearl oyster industry (Liao et al., 2020). In this study, we initially attempted to investigate the feasibility of spray-dried microalgae instead of live microalgae in P. maxima juvenile industrial farming. After an interview with the technician on the farm, we confirmed that the growth performance of P. maxima juveniles from industrial farming in this study was not good as that cultured in sea area, maybe due to the limited food availability, and is considered as the bottleneck for developing the culture mode for bivalves (Yang et al., 2017a,b). Similar results have been reported previously in species of some bivalves. For example, the absolute and RGRs of shell length and TW of the pearl oyster Pinctada martensii spat were lower than those cultured in the sea (Yang et al., 2015). Even so, the results in this study showed that P. maxima juvenile cultured indoor can promise the SR (ranged from 84 to 86%, no significant difference was observed) and significantly higher than those cultured in the natural sea (10%, unpublished data form farm). This is because culturing pearl oyster indoor can avoid the influence of extreme weather factors effectively, such as typhoons (usually happen in Hainan from August to October), temperature and salinity change caused by rain, and so on.
Filter-feeding bivalves usually take phytoplankton as a part of the natural diet. Isochrysis zhanjiangensis and Platymonas subcordiformis are the main microalgal species widely used in the fodder industry as good bait for aquaculture activity (Zheng et al., 2011; An et al., 2015; Cheng et al., 2020). But an operation to culture live microalgae requires dedicated staff and facilities that represent 30–50% of the costs (Gui et al., 2016b), and live microalgal growth is easily affected by environmental factors. Therefore, replacing live microalgae with a substitute and the same nutritive quality food is important and necessary in filter-feeding bivalve industrial farming. In this study, we used the spray-dried microalgal powder instead of live microalga. The results indicated that spray-dried microalgal powder can promise the P. maxima juvenile SR as live microalgae in industrial farming, improving pearl oyster production. However, the growth performance was slower than that in the live microalgal group. The difference may be caused by the spray-dried microalgal powder that was insufficient in other essential nutrients for the growth of pearl oyster juveniles, such as carbohydrate and protein, or not easy to digest as live microalga (Numaguchi, 2002; Cheng et al., 2020).
Digestive enzyme activity is often used as an indicator of digestive processes and nutritional condition of fish, including AMS, PES, and LPS, and reflects growth performance (Cahu et al., 1998). Various digestive enzymes are involved in digestive and absorptive processes and are important indicators of the growth performance of aquatic animals (Abolfathi et al., 2012). In this study, P. maxima juveniles fed with spray-dried microalgal powder displayed higher PES, AMS, and LPS activities than those with live microalgae. The phenomenon due to spray-dried microalgal powder needs more energy to digest and absorb nutrients. Thus, the growth performance is slightly lower, compared with the live microalgal group.
Intestinal microbiota, composed of a diverse and vast population of microorganisms, interacting with each other to form a complex ecological network through various types of interactions, plays key functions in host growth, physiology, and development (Nayak, 2010; Deng et al., 2012; Tremaroli and Bäckhed, 2012). In addition, intestinal microbiota activity is reported to be related to host longevity (Nayak, 2010). A disturbance in the intestinal microbial community can lead to changes in the microbial diversity and abundance of certain bacteria, resulting in beneficial or harmful effects in the aquatic animal (Gómez and Balcázar, 2008). An altered microbiota in the intestine can lead to altered immune functions of hosts and increase the risk of disease (Morgan et al., 2012). Thus, maintaining a functional and steady intestinal microbiota is important to the host. The composition of the intestinal bacterial community varies with a unique core microbiome in each specific host species. In comparison to mammals, the intestinal microbial composition in aquatic animals is susceptible to diet (De Filippo et al., 2010; Ringø et al., 2016), drugs (Zwolinska-Wcislo et al., 2011), stresses (Galley et al., 2014), and so on. The results in this study indicated that the Proteobacteria was the dominant bacteria in pearl oysters at the phylum level, consistent with the findings on oysters (Fernandez-Piquer et al., 2012; Trabal Fernández et al., 2014). Proteobacteria is the largest, most phenotypically diverse, and abundant phylum throughout all the life stages of animals (Qin et al., 2010; Sevellec et al., 2014; Zhou et al., 2018; Liao et al., 2020). Proteobacteria could catabolize feedstuff components (Jumpertz et al., 2011) and may play an important role in degrading cellulose and agar, the major components of food consumed by bivalve mollusks, as well as fixing nitrogen in the gastrointestinal tract of bivalves (Prieur et al., 1990; Harris, 1993; Newell, 2004; Liao et al., 2020). The relative abundance of Proteobacteria in spray-dried microalgal powder groups was significantly lower than that in live microalgal groups in this study, and this phenomenon may consequently result in slower growth performance of P. maxima juveniles in spray-dried microalgal powder groups.
Microbial identification is meaningful only when microbiota can be classified at the level of genus or species in relation to animal husbandry (Petrosino et al., 2009). Burkholderia was found as the main genus of P. maxima juvenile intestinal microbial community that from live microalgal groups, while Mycoplasma, Alphaproteobacteria, and Oxyphotobacteria in spray-dried microalgal powder groups. This phenomenon indicated the intestinal bacteria of P. maxima juvenile at genus were more sensitive to diets. Additionally, the top 10 representative genera mainly comprised of facultative anaerobes and anaerobes. Both Gram-positive and negative bacteria can produce phosphatases, helping in nutrient absorption (Ramirez and Dixon, 2003; Rasheeda et al., 2017). Although the genus observed in this study is reported to contain opportunistic pathogens, such as Burkholderia and Vibrio, all the P. maxima juvenile examined were healthy. The presence of pathogenic microbes in the intestinal of aquatic animals can be seen as a common phenomenon, playing a vital role in the internal metabolism and immune, rather than playing the role of a pathogen (Grimes et al., 1985; Pujalte et al., 2003; Valdenegro-Vega et al., 2013; Wu et al., 2013; Rasheeda et al., 2017). Alphaproteobacteria was the dominant Proteobacteria, widely distributed in marine environments (Wagner-Döbler and Biebl, 2006). Mycoplasma is the abundant member of gut microbiota in numerous vertebrate hosts and has been found to dominate in the gut of Atlantic salmon (Holben et al., 2002), farmed rainbow trout Oncorhynchus mykiss (Lyons et al., 2017), Trinidadian guppies (Sullam et al., 2015), and the long-jawed mudsucker Gillichtythys mirabilis (Bano et al., 2007).
The intestinal microbiome revealed rich biodiversity that predictably reacts to changing gut conditions, and diet components played a key role in regulating the intestinal bacterial community (Hennersdorf et al., 2016; Huyben et al., 2017). The overall intestinal bacterial diversity increased in pearl oysters P. maxima juvenile fed with spray-dried microalgal powder in this study. Therefore, the spray-dried microalgal powder leads to a high biodiversity level, benefit to maintain the intestinal micro ecological balance. The antioxidant capacity and immunity may be related to the biodiversity level of intestinal bacteria (Nie et al., 2017; Wang et al., 2017). Thus, the change of antioxidant capacity and immunity in P. maxima juveniles that feed with spray-dried microalgal powder need to be established and investigated in the future, as well as the underlying mechanisms.
In summary, this study is the first to analyze the feasibility of spray-dried microalgal powder instead of live microalga in P. maxima juvenile industrial farming. The survival, growth, and intestinal microbial community were used as an indicator for comparative profiling. The results indicated that the spray-dried microalgal powder used in this study (Isochrysis zhanjiangensis and Platymonas subcordiformis powder) can promise the SR as live microalgae, even though the growth performance is slightly lower. The characterization of the intestinal bacterial community of P. maxima juveniles under controlled conditions was first presented in this study, with Proteobacteria as the dominant phylum, and intestinal bacteria at the genus level were more sensitive to diets. However, the relationship between intestinal bacteria of P. maxima juveniles and diet needs further research. The results in this study suggested that the spray-dried I. zhanjiangensis powder and P. subcordiformis powder can serve as substitutes for live microalgae in P. maxima juvenile industrial farming under controlled conditions. The findings in this study will be helpful to develop the industrial farming mode for P. maxima juvenile, to avoid mass mortality caused by increasing pollution offshore or unexpected natural disasters. Furthermore, this study provides basic data to optimize industrial farming technology and healthy management for P. maxima juvenile.
Raw sequence data of samples in this study are available in the Sequence Read Archive database of NCBI under the SRA Accession: PRJNA790867.
The animal study was reviewed and approved by the Animal Care and Use Committee of Hainan University. Written informed consent was obtained from the owners for the participation of their animals in this study.
XZ and ZG conceptualized the study. SY and XL were responsible for the experimental operation, and prepared and wrote the original draft. HV and XZZ conducted the sample determination. XZ, ZG, AW, JL, and YS reviewed, edited, and wrote the manuscript. All authors read and approved the final manuscript.
This project was supported by the National Key Research and Development Program of China (Grant No. 2018YFD0900704) and the National Science Foundation of China (Grant No. 31772847).
The authors declare that the research was conducted in the absence of any commercial or financial relationships that could be construed as a potential conflict of interest.
All claims expressed in this article are solely those of the authors and do not necessarily represent those of their affiliated organizations, or those of the publisher, the editors and the reviewers. Any product that may be evaluated in this article, or claim that may be made by its manufacturer, is not guaranteed or endorsed by the publisher.
Abolfathi, M., Hajimoradloo, A., Ghorbani, R., and Zamani, A. (2012). Effect of starvation and refeeding on digestive enzyme activities in juvenile roach, Rutilus rutilus caspicus. Comp. Biochem. Physiol. A 161, 166–173. doi: 10.1016/j.cbpa.2011.10.020
An, X., Li, X., Li, Z., and Zhang, Y. (2015). Growth characteristics of platymonas subcordiformis and oxyrrhis marina in their co-culture systems. Nat. Environ. Pollut. Technol. 14:967.
Arney, B., Liu, W., Forster, I. P., Mckinley, R. S., and Pearce, C. M. (2015). Feasibility of dietary substitution of live microalgae with spray-dried Schizochytrium, sp. or spirulina, in the hatchery culture of juveniles of the pacific geoduck clam (Panopea generosa). Aquaculture 444, 117–133. doi: 10.1016/j.aquaculture.2015.02.014
Bano, N., Smith, A. D., Bennett, W., Vasquez, L., and Hollibaugh, J. T. (2007). Dominance of mycoplasma in the guts of the long-jawed mudsucker, Gillichthys mirabilis, from five California salt marshes. Environ. Microbiol. 9, 2636–2641. doi: 10.1111/j.1462-2920.2007.01381.x
Barnes, R., Calow, P., Olive, P., and Golding, D. (1993). The Invertebrates: A Synthesis. Cambridge: Blackwell Science Lyd.
Bolyen, E., Rideout, J. R., Dillon, M. R., Bokulich, N. A., Abnet, C. C., Al-Ghalith, G. A., et al. (2019). Reproducible, interactive, scalable and extensible microbiome data science using QIIME 2. Nat. Biotechnol. 37, 852–857. doi: 10.1038/s41587-019-0209-9
Brown, M., and Robert, R. (2002). Preparation and assessment of microalgal concentrates as feeds for larval and juvenile pacific oyster (Crassostrea gigas). Aquaculture 207, 289–309. doi: 10.1016/s0044-8486(01)00742-6
Cahu, C., Zambonino Infante, J., Peres, A., Quazuguel, P., and Gall, M. (1998). Algal addition in sea bass (Dicentrarchus labrax) larvae rearing: effect on digestive enzymes. Aquaculture 161, 479–489. doi: 10.1016/s0044-8486(97)00295-0
Cheng, P., Zhou, C., Chu, R., Chang, T., Xu, J., Ruan, R., et al. (2020). Effect of microalgae diet and culture system on the rearing of bivalve mollusks: nutritional properties and potential cost improvements. Algal Res. 51:102076. doi: 10.1016/j.algal.2020.102076
De Filippo, C., Cavalieri, D., Di Paola, M., Ramazzotti, M., Poullet, J. B., Massart, S., et al. (2010). Impact of diet in shaping gut microbiota revealed by a comparative study in children from Europe and rural Africa. Proc. Natl. Acad. Sci. U.S.A. 107, 14691–14696. doi: 10.1073/pnas.1005963107
Deng, Y., Fu, S., Liang, F., Du, X., and Xie, S. (2013). Growth and survival of pearl oyster pinctada maxima spat reared under different environmental conditions. J. Shellfish Res. 32, 675–679.
Deng, Y., Jiang, Y. H., Yang, Y., He, Z., Luo, F., and Zhou, J. (2012). Molecular ecological network analyses. BMC Bioinformatics 13:113. doi: 10.1186/1471-2105-13-113
Edgar, R. C. (2013). UPARSE: Highly accurate OTU sequences from microbial amplicon reads. Nat. Methods 10, 996–998. doi: 10.1038/nmeth.2604
Fernandez-Piquer, J., Bowman, J. P., Ross, T., and Tamplin, M. L. (2012). Molecular analysis of the bacterial communities in the live Pacific oyster (Crassostrea gigas) and the influence of postharvest temperature on its structure. J. Appl. Microbiol. 112, 1134–1143. doi: 10.1111/j.1365-2672.2012.05287.x
Galley, J. D., Nelson, M. C., Yu, Z., Dowd, S. E., and Bailey, M. T. (2014). Exposure to a social stressor disrupts the community structure of the colonic mucosa-associated microbiota. BMC Microbiol. 14:189. doi: 10.1186/1471-2180-14-189
Gawlicka, A., Parent, B., Horn, M. H., Ross, N., Opstad, I., and Torrissen, O. J. (2000). Activity of digestive enzymes in yolk-sac larvae of Atlantichalibut (Hippoglossus hippoglossus): indication of readiness for first feeding. Aquaculture 184, 303–314. doi: 10.1016/s0044-8486(99)00322-1
Gómez, G. D., and Balcázar, J. L. (2008). A review on the interactions between gutmicrobiota and innate immunity of fish. Pathog. Dis. 52, 145–154. doi: 10.1111/j.1574-695x.2007.00343.x
Grimes, D. J., Brayton, P., Colwell, R. R., and Gruber, S. H. (1985). Vibrios as autochthonous flora of neritic sharks. Syst. Appl. Microbiol. 6, 221–226.
Gui, Y., Kaspar, H. F., Zamora, L. N., Dunphy, B. J., and Jeffs, A. G. (2016a). Capture efficiency of artificial food particles of post-settlement juveniles of the greenshellTM mussel, Perna canaliculus. Aquaculture 464, 1–7. doi: 10.1016/j.aquaculture.2016.06.011
Gui, Y., Zamora, L., Dunphy, B. J., and Jeffs, A. G. (2016b). Evaluation of the formulated diet MySpat for feeding hatchery-reared spat of the green-lipped mussel, Perna canaliculus (Gmelin, 1791). Aquac. Res. 42, 3907–3912. doi: 10.1111/are.12841
Harris, J. (1993). The presence, nature, and role of gut microflora in aquatic invertebrates: a synthesis. Microb. Ecol. 25, 195–231. doi: 10.1007/BF00171889
He, G., Liu, X., Xu, Y., Liang, J., Deng, Y., Zhang, Y., et al. (2021). Repeated exposure to simulated marine heatwaves enhances the thermal tolerance in pearl oysters. Aquat. Toxicol. 239:105959. doi: 10.1016/j.aquatox.2021.105959
Hennersdorf, P., Kleinertz, S., Theisen, S., Abdul-Aziz, M. A., Mrotzek, G., Palm, H. W., et al. (2016). Microbial diversity and parasitic load in tropical fish of different environmental conditions. PLoS One 11:e151594. doi: 10.1371/journal.pone.0151594
Holben, W. E., Williams, P., Saarinen, M., Sarkilahti, L. K., and Apajalahti, J. H. A. (2002). Phylogenetic analysis of intestinal microflora indicates a novel mycoplasma phylotype in farmed and wild salmon. Microb. Ecol. 44, 175–185. doi: 10.1007/s00248-002-1011-6
Huyben, D., Nyman, A., Vidakoviæ, A., Passoth, V., Moccia, R., Kiessling, A., et al. (2017). Effects of dietary inclusion of the yeasts Saccharomyces cerevisiae and Wickerhamomyces anomalus on gut microbiota of rainbow trout. Aquaculture 473, 528–537.
Jumpertz, R., Le, D. S., Turnbaugh, P. J., Trinidad, C., Bogardus, C., Gordon, J. I., et al. (2011). Energy-balance studies reveal associations between gut microbes, caloric load, and nutrient absorption in humans. Am. J. Clin. Nutr. 94, 58–65. doi: 10.3945/ajcn.110.010132
Liang, F., Xie, S., Fu, S., Li, J., and Deng, Y. (2016). Growth pattern and biometric relationship of pearl oyster Pinctada maxima cultured in Beibu Bay, China. J. Appl. Aquac. 28, 110–118. doi: 10.1080/10454438.2016.1172535
Liao, Y., Cai, C., Yang, C., Zheng, Z., Wang, Q., Du, X., et al. (2020). Effect of protein sources in formulated diets on the growth, immune response, and intestinal microflora of pearl oyster Pinctada fucata martensii. Aquac. Rep. 16:100253. doi: 10.1016/j.aqrep.2019.100253
Lyons, P. P., Turnbull, J. F., Dawson, K. A., and Crumlish, M. (2017). Effects of low-level dietary microalgae supplementation on the distal intestinal microbiome of farmed rainbow trout Oncorhynchus mykiss (Walbaum). Aquac. Res. 48, 2438–2452. doi: 10.1111/are.13080
Mamat, N. Z., and Alfaro, A. C. (2014). Evaluation of microalgal and formulated diets for the culture of the New Zealand pipi clam Paphies australis. Int. Aquat. Res. 6, 1–13.
Morgan, X., Tickle, T., Sokol, H., Gevers, D., Devaney, K., Ward, D., et al. (2012). Dysfunction of the intestinal microbiome in inflammatory bowel disease and treatment. Genome Biol. 13:R79. doi: 10.1186/gb-2012-13-9-r79
Nayak, S. K. (2010). Role of gastrointestinal microbiota in fish. Aquac. Res. 41, 1553–1573. doi: 10.1111/j.1365-2109.2010.02546.x
Nell, J. A., Diemar, J. A., and Heasman, M. P. (1996). Food value of live yeasts and dry yeast-based diets fed to Sydney rock oyster Saccostrea commercialis, spat. Aquaculture 145, 235–243. doi: 10.1016/s0044-8486(96)01337-3
Newell, R. I. R. (2004). Ecosystem influences of natural and cultivated populations of suspension-feeding bivalve molluscs: a review. J. Shellfish Res. 23, 51–61.
Nie, L., Zhou, Q. J., Qiao, Y., and Chen, J. (2017). Interplay between the gut microbiota and immune responses of ayu (Plecoglossus altivelis) during Vibrio anguillarum infection. Fish Shellfish Immunol. 68, 479–487. doi: 10.1016/j.fsi.2017.07.054
Numaguchi, K. (2002). Effect of an artificial diet on early spat growth of the Japanese pearl oyster Pinctada fucata martensii. Fisheries 68, 694–696. doi: 10.1046/j.1444-2906.2002.00446.x
Petrosino, J., Highlander, S., Luna, R., Gibbs, R., and Versalovic, J. (2009). Metagenomic pyrosequencing and microbial identification. Clin. Chem. 55, 856–866. doi: 10.1373/clinchem.2008.107565
Philippe, A. D., and Christopher, J. L. (1994). Use of a probiotic for the culture of larvae of the pacific oyster (Crassostrea gigas, thunberg). Aquaculture 119, 25–40. doi: 10.1016/0044-8486(94)90441-3
Prieur, D., Mevel, G., Nicolas, J. L., Plusquellec, A., and Vigneulle, M. (1990). Interactions between bivalve molluscs and bacteria in the marine environment. Oceanogr. Mar. Biol. Annu. Rev. 28, 277–352.
Pujalte, M. J., Sitjã-Bobadilla, A., Ã-Lvarez-Pellitero, P., and Garay, E. (2003). Carriage of potentially fish-pathogenic bacteria in Sparus aurata cultured in Mediterranean fish farms. Dis. Aquat. Organ. 54, 119–126. doi: 10.3354/dao054119
Qin, J., Li, R., Raes, J., Arumugam, M., Burgdorf, K. S., Manichanh, C., et al. (2010). A human gut microbial gene catalogue established by metagenomic sequencing. Nature 464, 59–65. doi: 10.1038/nature08821
Quast, C., Pruesse, E., Yilmaz, P., Gerken, J., Schweer, T., Yarza, P., et al. (2013). The SILVA ribosomal RNA gene database project: improved data processing and web-based tools. Nucl. Acids Res. 41, D590–D596. doi: 10.1093/nar/gks1219
Ramirez, R. F., and Dixon, B. A. (2003). Enzyme production by obligate intestinal anaerobic bacteria isolated from oscars (Astronotus ocellatus), angelfish (Pterophyllum scalare) and southern flounder (Paralichthys lethostigma). Aquaculture 227, 417–426. doi: 10.1016/s0044-8486(03)00520-9
Rasheeda, M. K., Rangamaran, V. R., Srinivasan, S., Ramaiah, S. K., Gunasekaran, R., Jaypal, S., et al. (2017). Comparative profiling of microbial community of three economically important fishes reared in sea cages under tropical offshore environment. Mar. Genom. 34, 57–65. doi: 10.1016/j.margen.2017.04.003
Ringø, E. Z. Z. V., Dimitroglou, A., Foey, A., Davies, S., Owen, M., Lauzon, H. L., et al. (2016). Effect of dietary components on the gut microbiota of aquatic animals. A never-ending story? Aquac. Nutr. 22, 219–282.
Sevellec, M., Pavey, S. A., Boutin, S., Filteau, M., Derome, N., and Bernatchez, L. (2014). Microbiome investigation in the ecological speciation context of lake whitefish (Coregonus clupeaformis) using next-generation sequencing. J. Evol. Biol. 27, 1029–1046. doi: 10.1111/jeb.12374
Sullam, K. E., Rubin, B. E. R., Dalton, C. M., Kilham, S. S., Flecker, A. S., and Russell, J. A. (2015). Divergence across diet, time and populations rules out parallel evolution in the gut microbiomes of Trinidadian guppies. ISME J. 9, 1508–1522. doi: 10.1038/ismej.2014.231
Tanyaros, S., and Chuseingjaw, S. (2016). A partial substitution of microalgae with single cell detritus produced from seaweed (Porphyra haitanensis) for the nursery culture of tropical oyster (Crassostrea belcheri). Aquac. Res. 47, 2080–2088.
Trabal Fernández, N., Mazón-Suástegui, J. M., Vázquez-Juárez, R., Ascencio-Valle, F., and Romero, J. (2014). Changes in the composition and diversity of the bacterial microbiota associated with oysters (Crassostrea corteziensis, Crassostrea gigas and Crassostrea sikamea) during commercial production. FEMS Microbiol. Ecol. 88, 69–83. doi: 10.1111/1574-6941.12270
Tremaroli, V., and Bäckhed, F. (2012). Functional interactions between the gut microbiota and host metabolism. Nature 489, 242–249. doi: 10.1038/nature11552
Valdenegro-Vega, V., Naeem, S., Carson, J., Bowman, J., Tejedor Del Real, J., and Nowak, B. (2013). Culturable microbiota of ranched southern bluefin tuna (Thunnus maccoyii Castelnau). J. Appl. Microbiol. 115, 923–932. doi: 10.1111/jam.12286
Wagner-Döbler, I., and Biebl, H. (2006). Environmental biology of the marine Roseobacter lineage. Annnu. Rev. Microbiol. 60, 255–280. doi: 10.1146/annurev.micro.60.080805.142115
Wang, A., Shi, Y., Wang, Y., and Gu, Z. (2010). The Biology and Breeding of New Technologies of Pinctada Martensii. Beijing: Chinese Agricultural Science and Technology Press.
Wang, Q., Yang, C., Du, X., Liu, X., Sun, R., and Deng, Y. (2015). Growth performance and biochemical composition of juvenile pearl oyster Pinctada martensii fed on artificial diets. Aquac. Int. 24, 995–1005. doi: 10.1007/s10499-015-9966-8
Wang, X., Sun, Y., Wang, L., Li, X., Qu, K., and Xu, Y. (2017). Synbiotic dietary supplement affects growth, immune responses and intestinal microbiota of Apostichopus japonicus. Fish Shellfish Immunol. 68, 232–242. doi: 10.1016/j.fsi.2017.07.027
Wu, S. G., Tian, J. Y., Gatesoupe, F. J., Li, W. X., Zou, H., Yang, B. J., et al. (2013). Intestinal microbiota of gibel carp (Carassius auratus gibelio) and its origin as revealed by 454 pyrosequencing. World J. Microbiol. Biotechnol. 29, 1585–1595. doi: 10.1007/s11274-013-1322-4
Yang, C., Hao, R., Deng, Y., Liao, Y., Wang, Q., Sun, R., et al. (2017a). Effects of protein sources on growth, immunity and antioxidant capacity of juvenile pearl oyster Pinctada fucata martensii. Fish Shellfish Immunol. 67, 411–418. doi: 10.1016/j.fsi.2017.06.037
Yang, C., Luo, S., Wang, Q., Deng, Y., and Du, X. (2015). Evaluation of the applicability of a microencapsulated diet developed for the pearl oyster, Pinctada martensii. J. Fish. Sci. China 22, 442–449.
Yang, C., Wang, Q., Hao, R., Liao, Y., Du, X., and Deng, Y. (2017b). Effects of replacing microalgae with an artificial diet on pearl production traits and mineralization-related gene expression in pearl oyster Pinctada fucata martensii. Aquac. Res. 48, 5331–5337.
Zhao, B., Zhang, S., and Qian, P. Y. (2003). Larval settlement of the silver- or goldlip pearl oyster Pinctada maxima (Jameson) in response to natural biofilms and chemicalcues. Aquaculture 220, 883–901. doi: 10.1016/s0044-8486(02)00567-7
Zheng, J., Hao, J., and Wang, B. (2011). Bioremediation of aquiculture wastewater by microalgae Isochrysis zhanjiangensis and production of the biomass material. Key Eng. Mater. 460–461, 491–495. doi: 10.4028/www.scientific.net/kem.460-461.491
Zheng, X., Fu, Z., Lin, S., Yang, R., Wang, A., Gu, Z., et al. (2020). Which is the major trigger in aquatic environment for pearl oyster Pinctada fucata martensii sperm from gonad: ammonia ion or pH ? Aquaculture 520:734673. doi: 10.1016/j.aquaculture.2019.734673
Zheng, X., Yang, R., Hu, J., Lin, S. Q., Gu, Z. F., and Ma, Z. H. (2019). The gut microbiota community and antioxidant enzymes activity of barramundi reared at seawater and freshwater. Fish Shellfish Immunol. 89, 127–131. doi: 10.1016/j.fsi.2019.03.054
Zhou, M., Liang, R., Mo, J., Yang, S., Gu, N., Wu, Z., et al. (2018). Effects of brewer’s yeast hydrolysate on the growth performance and the intestinal bacterial diversity of largemouth bass (Micropterus salmoides). Aquaculture 484, 139–144. doi: 10.1016/j.aquaculture.2017.11.006
Zhou, Y., Yang, H. S., Zhang, T., Liu, S. L., Zhang, S. M., Liu, Q., et al. (2006). Influence of filtering and biodeposition by the cultured scallop Chlamys farreri on benthic-pelagic coupling in a eutrophic bay in China. Mar. Ecol. Prog. Ser. 317, 127–141. doi: 10.3354/meps317127
Zwolinska-Wcislo, M. K. G. P., Sliwowski, Z., Urbanczyk, K., Drozdowicz, D., Konturek, S. J., Pawlik, W. W., et al. (2011). Antibiotic treatment with ampicillin accelerates the healing of colonic damage impaired by aspirin and coxib in the experimental colitis. Importance of intestinal bacteria, colonic microcirculation and proinflammatory cytokines. J. Physiol. Pharmacol. 62, 357–368.
Keywords: Pinctada maxima, juvenile, industrial farming, microalga substitution, spray-dried microalga powder
Citation: Yang S, Li X, Vasquez HE, Wang A, Shi Y, Li J, Zhang X, Zheng X and Gu Z (2022) Comparative Profiling of Survival, Growth, and Intestinal Microbial Community of Pearl Oyster Pinctada maxima Juvenile in the Industrial Farming: The Feasibility of Using Spray-Dried Microalgae Powder. Front. Mar. Sci. 8:800627. doi: 10.3389/fmars.2021.800627
Received: 23 October 2021; Accepted: 06 December 2021;
Published: 21 January 2022.
Edited by:
Liqiang Zhao, Guangdong Ocean University, ChinaReviewed by:
Liang Junping, Henan Normal University, ChinaCopyright © 2022 Yang, Li, Vasquez, Wang, Shi, Li, Zhang, Zheng and Gu. This is an open-access article distributed under the terms of the Creative Commons Attribution License (CC BY). The use, distribution or reproduction in other forums is permitted, provided the original author(s) and the copyright owner(s) are credited and that the original publication in this journal is cited, in accordance with accepted academic practice. No use, distribution or reproduction is permitted which does not comply with these terms.
*Correspondence: Xing Zheng, emhlbmd4aW5nX2VkdUAxNjMuY29t; Zhifeng Gu, aG51Z3VAMTYzLmNvbQ==
†These authors have contributed equally to this work and share first authorship
Disclaimer: All claims expressed in this article are solely those of the authors and do not necessarily represent those of their affiliated organizations, or those of the publisher, the editors and the reviewers. Any product that may be evaluated in this article or claim that may be made by its manufacturer is not guaranteed or endorsed by the publisher.
Research integrity at Frontiers
Learn more about the work of our research integrity team to safeguard the quality of each article we publish.