- 1School of Zoology, George S. Wise Faculty of Life Sciences, Tel Aviv University, Tel Aviv, Israel
- 2The Interuniversity Institute for Marine Sciences in Eilat, Eilat, Israel
- 3Steinhardt Museum of Natural History, Tel Aviv University, Tel Aviv, Israel
The common lionfish, Pterois miles, a notoriously invasive species known for its harmful effect on native fish communities in the Atlantic Ocean, has recently begun spreading across the Mediterranean Sea. The wide niche breadth of the lionfish has been hypothesized to facilitate its invasion success. However, it is unclear to what extent this wide niche-breadth is associated with individual-level variation and repeatable behavior over time. Large individual-level behavioral variations may allow individuals to adapt quickly to local conditions, increasing the species’ chance of invasion success and complicating mitigation efforts. In this study, we used an acoustic telemetry system in P. miles’ native Red Sea environment to explore individual-level variation in depth preference and diel activity. A wide depth range may indicate an ability to tolerate a variety of biotic and abiotic conditions, and variability in diel activity may indicate an ability to exploit multiple diet sources. We found large individual-level variability in P. miles’ activity hours; although all tracked fish were active during both sunrise and sunset, certain individuals had prolonged activity hours to variable extents. Moreover, individuals often change their patterns over time, showing low repeatability. We also found that individuals had different depth preferences and commuted between shallow and deep waters over short periods of time. This study is one of the first to explore diel activity as an individual-level trait in wild fish. The variability found in depth and diel activity is likely one of the reasons P. miles has been so successful in invading the Mediterranean Sea. In addition, this variability may impact mitigation efforts within the Mediterranean Sea as nocturnal individuals from deeper waters might replenish diurnally culled shallow-water populations.
Introduction
The common lionfish, Pterois miles, is one of the most harmful marine invasive species (Morris et al., 2009; Albins and Hixon, 2013). In the western Atlantic, invasions by P. miles and the similar red lionfish Pterois volitans, have caused a severe decline in native species diversity, density, and recruitment rate (Albins and Hixon, 2008; Green et al., 2012; Albins, 2013; Tuttle, 2017). P. miles has also invaded the Mediterranean Sea from its native Indo-Pacific origin through the Suez Canal, and has been spreading rapidly, recently reaching the Italian coast (Golani and Sonin, 1992; Azzurro et al., 2017; Stern and Rothman, 2018; Agostino et al., 2020). This species’ impact in the Mediterranean Sea has not yet been fully established (but see Agostino et al., 2020). It has been hypothesized that wide niche-breadth is one of the traits that enable the lionfish to invade so successfully (Côté et al., 2013a; Hackerott et al., 2013). However, the contribution of individual behavior to the observed population wide niche-breadth is still unclear.
Plasticity in individual behavior may be key for understanding invasiveness. For example, behavior plasticity may enable efficient tracking of local conditions and thus increase invasiveness (Nussey et al., 2007; Sagata and Lester, 2009; Gross et al., 2010; Chapple et al., 2012; Nakayama et al., 2016). In support, invasive amphipods showed higher individual-level variation in swimming activity than native populations (Bierbach et al., 2016). In addition, distinct individual behaviors can affect invasion dynamics as, for example, aggressive and better-dispersing individuals can lead the invasion front to facilitate rapid establishment (Araújo and Gonzaga, 2007; Duckworth and Badyaev, 2007; Duckworth, 2008; Araújo et al., 2011; Bolnick et al., 2011; Fogarty et al., 2011). For lionfish, there is ample evidence of population wide niche-breadth regarding diet, movement, habitat use, depth preference, and activity time (Harmelin-Vivien and Bouchon, 1976; Jud et al., 2011; Cure et al., 2012; Lee et al., 2012; Côté et al., 2013b; Andradi-Brown et al., 2017a; Gavriel and Belmaker, 2020). However, it remains unclear to what extent (1) individuals display similar behaviors and (2) individuals show repeatable behavior over time. Documenting the extent of such behavioral plasticity is critical for understanding the role of individual-level variation in invasion success.
This study explored two traits, activity time (diel activity) and depth use. Temporal activity patterns remain relatively unexplored in marine fish (Albrecht and Gotelli, 2001; Stuber et al., 2015; Alós et al., 2017). In the context of invasion, it is assumed that variable activity hours may increase the availability of dietary sources (Kocárek, 2001; Kronfeld-Schor and Dayan, 2003; Holt et al., 2004; Valeix et al., 2007). In marine fishes, temporal niches are usually conserved as most species are exclusively diurnal, nocturnal, or crepuscular (Helfman, 1978, 1986; Reebs, 2002). However, Pearly razorfish (Xyrithchys novacula) showed variations in individuals’ awakening and rest periods (Alós et al., 2017). A striking shift in diel activity was documented between Goldline rabbitfish (Siganus lineatus) populations at the Great Barrier Reef, shifting their activity from diurnal to nocturnal (Fox and Bellwood, 2011). Lionfish are traditionally described as crepuscular at a population level, but evidence of nocturnal and diurnal activity is accumulating (Harmelin-Vivien and Bouchon, 1976; Morris and Akins, 2009; Cure et al., 2012; Mctee and Grubich, 2014; McCallister et al., 2018; Gavriel and Belmaker, 2020). Individual-level variation in activity times may enable P. miles to exploit a broader range of prey sources and respond more quickly to a different distribution of prey throughout the day, thereby increasing their potential invasion success.
Depth represents a vital habitat axis dictating biotic (Larson, 1980; Golani, 1994; Dunn et al., 2010) and abiotic conditions (Longhurst, 1985; Harvey and Stewart, 1991; Malcolm et al., 2011). Thus, the range of depths inhabited can represent a behavioral axis (Van Snik Gray and Stauffer, 1999). Depth use can vary considerably between individuals. For instance, in Trumpet emperor (Lethrinus miniatus), some individuals use only shallow habitats while others exploit the entire water column (Currey et al., 2014). Lionfish show strikingly broad depth ranges from surface level to depths of 200 m (Nuttall et al., 2014; Andradi-Brown et al., 2017b; Gress et al., 2017). However, it is still not clear to what extent individuals can vary their depth and over what time scale.
Culling, i.e., targeted removal of individuals, is commonly used in the Atlantic Ocean to control lionfish populations (Morris and Creswell, 2013) and is currently being considered in multiple countries around the Mediterranean Sea (Kleitou et al., 2021; Ulman et al., 2021). Although complete eradication of lionfish is impossible, an efficient culling program can mitigate harmful effects (Côté et al., 2014a). Most culling efforts are concentrated diurnally at depths of 0–30 m (Côté et al., 2014b; Usseglio et al., 2017). However, past studies have indicated that deeper mesophotic reefs might serve as lionfish sanctuaries, replenishing shallow-water populations (Andradi-Brown et al., 2017a,b). Understanding the time scale of fish movement between shallow and deep reefs, and their nocturnal and diurnal activity, is critical for designing effective culling programs.
This study uses acoustic telemetry to explore P. mile’s individual variability in its native environment, the Red Sea. To determine diel activity and depth profiles, we tracked free-ranging lionfish, and their acceleration and depth were recorded at minute-long resolution over a period of months. We use this data to explore the variability between P. miles individuals and to assess their consistency over time. Examining the invading lionfish source population in its native habitat can help us understand whether P. miles’ behavioral variation is an inherent trait or one that only appears after invading (e.g., when the lionfish are released from their former biotic limitations (Van Valen, 1965; Bolnick et al., 2010; Tuttle et al., 2017). Moreover, this information can help scientists construct efficient management programs for invasive populations in the Mediterranean Sea.
Materials and Methods
Study Site
The study was conducted in a sub-tropical fringing reef located along the coast-line of Eilat, within the Gulf of Aqaba (Figure 1). This region, located along the northern tip of the Red Sea (29.50″N, 34.92″E), is inhabited by ichthyofauna of Indo-Pacific origin (Lieske et al., 2004; Kiflawi et al., 2006). In the Gulf of Aqaba, the common members of the Pteroinae sub-family are P. miles and the Clearfin lionfish, Pterois radiata (Golani and Darom, 1997; Brokovich, 2001; Gavriel and Belmaker, 2020). Lionfish surveys conducted within the study site found that P. miles’ mean density was 2.1 ± 1.8 (SD) individuals per 500 m2 (Gavriel and Belmaker, 2020). The Gulf of Aqaba in general, and the study site in particular, are characterized by a narrow shelf with fringing reefs, followed by a steep slope that descends to depths of 60 m within 200 m from shore (Sade et al., 2008; Tibor et al., 2010). The selected study site was spread over 2.5 km and encompassed multiple sub-habitats such as hard-bottom substrates, soft-bottom substrates, and continuous and fragmented fringing reefs.
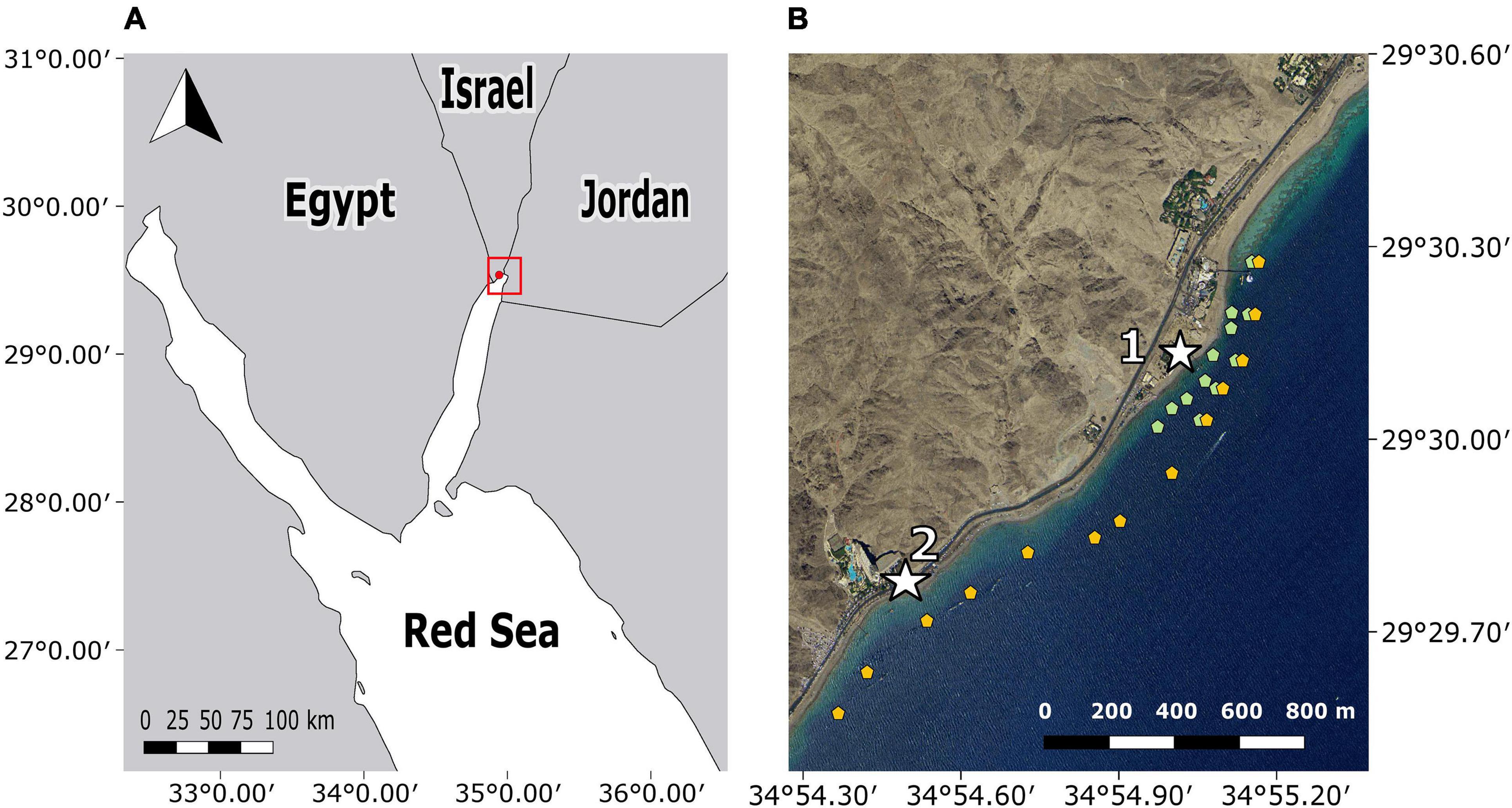
Figure 1. Map of the study area located in the northern tip of the Gulf of Aqaba. (A) Regional map of the Red Sea with the study site marked in red. (B) Receiver locations, Vemco receivers marked in orange, and Thelma Biotel receivers marked in green. The two capture sites are indicated as white stars. Site 1 is The Interuniversity Institute for Marine Sciences (IUI), site 2 is the southern beach (S.B).
Acoustic Receiver Arrays
Two acoustic telemetry systems were used to track wild lionfish activity and depth patterns. The first receiver array was composed of 13 VR2W Vemco© receivers. To avoid tag collision with other studies using the same Vemco array at the same time, we constructed another array composed of 12 TBR 700 Thelma Biotel© receivers that operated on different frequencies. Together, the arrays were spread over 2.5 km, from the Eilat Coral Nature Reserve in the north down to the Taba Border Cross in the south (Figure 1).
The area covered included the shallow reef and extended continuously toward the deeper reef with a bottom depth of about 60 m. All receivers were oriented with the hydrophone facing down. The 13 Vemco receivers were deployed from May 2017 to February 2019, in a linear form and were attached to buoys located over the reef slope with a bottom depth of 13–40 m and affixed at 6–12 m. Receivers were tuned to a frequency of 69 kHz. In addition, 12 Thelma Biotel receivers were deployed from November 2017 until February 2019 and tuned to 71 kHz. This array was composed of two parallel lines of receivers: a seaward line with five receivers and a shoreline with seven receivers. The seaward line receivers were positioned next to the Vemco receivers, and the shoreline receivers were attached to buoys at depths of 3–10 m over bottom depth of 5–20 m. The array’s configurations differed due to the lower detection range of the Thelma Biotel receivers (see section “Data Validation”), which required a denser configuration. We located the Thelma Biotel array next to the Inter-University Institution (IUI, Site 1; Figure 1) as this site has a high density of lionfish and could be easily accessed. Neither array provided pinpoint localizations as this kind of data necessitates overlapping reception of at least three receivers. Receivers were retrieved bi-monthly for short periods (up to 36 h) when detection data was downloaded, batteries replaced, and hydrophones cleaned.
Tag Specifications
We used two types of internal implantation transmitters from two manufacturers. Transmissions could only be recorded on the corresponding company’s equipment. Thelma Biotel© AD-LP9L transmitters (9 mm diameter, 28.5 mm long, 2.5 g, operates on a frequency of 71 kHz, power output of 142 dB) were equipped with tri-axial acceleration and depth sensors. The tags were programmed to transmit every minute for the first 5 months and every 5 min for an additional 12 months. The transmission ratio was 2:1 acceleration to depth, respectively. The acceleration sensor had a range of 0–3.465 m/s2. The depth sensor has a resolution of 0.34 cm and a range of 0–81.6 m. The second type of transmitters used were Vemco© V9AP-2x (9 mm diameter, 48 mm long, 3.6 g, operates on a frequency of 69 kHz, power output of 151 dB) equipped with tri-axial acceleration and depth sensors. The acceleration sensor values ranged between 0 and 4.9 m/s2, and the depth sensor had a resolution of 15 cm and a range of 0–34 m. The tags were programmed to transmit every minute for the first 70 days and every 5 min for an additional 287 days. Again, the transmission ratio was 2:1 for acceleration to depth. In the “sensitivity analyses” below, we examine how the difference in maximal depth between tags may impact the result.
Fish Collecting and Tagging
During this study, 11 P. miles were tagged (dates and locations specified in Table 1). Fish were captured at night by trained SCUBA divers using soft monophyletic hand nets. The fish were then transported to a lab located within 1 km from all capturing sites and kept in a 700 L water tank with fresh seawater circulation until being tagged the following morning (up to 10 h from capture). For the tag implantations, the fish were anesthetized with clove oil, and the tags were surgically implanted in the peritoneal cavity of the fish (protocol by Ghanawi et al., 2013). The tag weight to body weight ratio (mean = 1.3%, range 0.8–1.9%) was kept low to assure that tags did not alter fish activity and behavior and were consistent with ratios from previous telemetry research on this species (Bacheler et al., 2015). To facilitate recovery from the surgical procedure, fish were returned to the tank for at least 36 h after the tag implantation. After the recovery period, the fish were released to the sea at their original capture point (±50 m). Fish inserted with Vemco transmitters (five fish) were tagged during May 2017, and those with Thelma Biotel transmitters (six fish) were tagged between December 2017 and June 2018. All surgical procedures and fish handling procedures were reviewed and approved by the BGU Experimentation Ethics Review Committee, authorization number IL-18-04-2018c.
Data Validation
Fish detection was screened manually, and detections from dead fish (i.e., showing no change in location, depth, or acceleration values) were removed. Data collected in the 48 h following the fish release back to nature was removed from the analyses to avoid periods of acclimation, as were false detection attributed to tags with unknown ID numbers (ghost tags). Finally, weeks with fewer than 10 detections were omitted. Additional filtering was applied separately for each analysis (see below). We estimated the array reception areas and found that the conservative detections range was 120 m for each Vemco receiver and 50 m for each Thelma Biotel receiver (see Supplementary Material 1).
Analyses
Activity
To assess if individuals possess similar activity patterns, we used Generalized Additive Models (GAMs, Hastie, 2017) and tested if including individual identity increased model performance. Activity hours were estimated using the acceleration data collected from tagged P. miles. The underlying assumption was that a concentration of high acceleration values could accurately represent lionfish activity and compensate for the low acceleration values expected for an ambush predator (Fishelson, 1997; Albins and Lyons, 2012). We transformed the acceleration values to a binomial variable (zero indicating a non-active state and one indicating an active state) in the following way: median acceleration values (m/s2) were calculated for bins of 30 min periods, bins with less than three detections were removed from the analysis. Then we defined the 70th percentile of the bins with higher acceleration values as active half hours and the rest as non-active half hours for each fish. This method enabled us to standardize the activity definitions and account for variation in acceleration measurements across the different manufacturers (see section “Tag Specifications”).
As the response was the presence or absence of activity (1/0) in each 30-min bin, we used binomial family GAM. The primary predictor was “time from sunrise,” modeled via cubic spline smoother, meaning that time was treated as cyclic [knot number (k) was defined to be 24, i.e., the day was split into 24 sections]. Other predictors used to explain some of the variations between individuals were “depth (m),” calculated as the median depth (m) for each 30-min bin, and applied in the model using an adaptive smoother (Wood, 2006), and “season” modeled as a fixed effect (December–February, March–May, June–August, and September–November). “Fish length” (TL in cm), and “capture site” (categorical variable, n = 2) were tested as well but excluded from the analysis in a preliminary model selection process. The variable “time from sunset” was also tested and produced similar results to “time from sunrise” and was thus omitted. We tested this model once with “fish ID” added as a random effect to the intercept only (similar activity pattern to all fish) and once as an interaction with the “time from sunrise” (creating separate activity curves for each “fish ID”). To identify the models best supported by the data, we used the corrected Akaike Information Criteria (Sugiura, 1978; Akaike, 1987). This criterion prioritizes models based on the maximum variation explained by the minimum variables and thus balances predictive power and overfitting (Akaike, 1987).
To assess consistency in activity hours for the same individual between days, we used repeatability scores. To compare activity across days and individuals, we calculated an index of daily activity peaks, defined as the hour of the highest density of active detections, as estimated by a kernel density smoother. Since lionfish show a general pattern of two activity peaks around sunrise and sunset, we performed the analyses separately for each period to obtain one index for the time of the highest morning activity and one index for the highest evening activity. To ensure the quality of the analysis, we only included days that had a full activity profile with at least 20 h between the first and last detections of the day and with at least one detection every 4 h.
The repeatability score was quantified using a mixed-effect model approach (Dingemanse and Dochtermann, 2013; Alós et al., 2017) as:
The between individual component (BIC) represents the average variance found between individuals and was calculated in the model as the variance of “fish ID” random intercepts. The within individual component (WIC) is the average variance found within an individual and represents the variance associated with measurement error in the model (Roughgarden, 1972; Bolnick et al., 2002; Nakagawa and Schielzeth, 2010; Dingemanse and Dochtermann, 2013; Alós et al., 2017). The repeatability score ranges from 0 (low) to 1 (high) repeatability.
The models’ response variables were “morning activity peak” or “evening activity peak.” Predictors used to explain some of the WIC were “season” and “capture site,” treated as fixed effects. We calculated adjusted repeatability scores, meaning that the variance explained by the fixed effects was not included in the denominator of the repeatability calculation (Dingemanse and Dochtermann, 2013). The confidence intervals for the adjusted repeatability score were constructed using parametric bootstraps, and likelihood ratio tests were used to calculate the p-value.
Depth
To estimate if individuals vary in their depth use, we tested whether including individual identity increased GAM performance. We used the median depth (m) of each 30-min bin as the response variable. The predictor variables included “time from sunrise” and “season” modeled as described above. “Activity” (0/1 for each 30 min bin) was used as fix effect. “Fish length” and “capture site” were also tested but were excluded in a preliminary model selection process. We tested this model compared to a similar model with “fish ID” added as a random effect (using AICc scores; Akaike, 1987; Hastie, 2017).
To assess individual consistency in depth preferences, we examined each individual’s maximum daily depth and daily depth range and calculated the repeatability score. These indices were calculated based only on days with 18 h or more between the first and last detection and at least one detection every 6 h. These criteria are slightly less strict than those used for the daily activity indices as it is easier to characterize the daily depth profile than it is to characterize activity levels that usually have at least two peaks. Adjusted repeatability scores were quantified using mixed-effect models as described above for activity (Dingemanse and Dochtermann, 2013; Alós et al., 2017). The models’ response variables were “maximum depth” or “depth range.” Predictors used to explain some of the WIC were “season” and “capture site,” treated as fixed effects.
Sensitivity Analyses
We performed several sensitivity analyses to assure the robustness of our results. (1) To ensure that active periods defined at a scale of 30-min bins (see section “Activity”) do not represent only the periods in which fish were detected (e.g., due to low reception while hiding), we compared the density plot of the periods defined as active to the density plot of all the detections. (2) The number of detections and the tracking period were considerably higher for fish number eight (71% of all detections). Thus, activity patterns and repeatability scores might be affected by this single individual. We performed two additional analyses and compared the results to those obtained when using all the data. In the first, we excluded fish number eight entirely. In the second, we used only the first 2 months of data for this individual. This way, we retained a balanced portion of this individual’s detections compared to other individuals tagged. (3) Transmitters from both manufacturers lower their transmit frequencies after a certain period (see section “Tag Specifications”). We repeated all analyses with data collected only from high transmit frequency periods to ensure this did not affect the results. (4) Three of the fish in this study were tagged with Vemco tags with a limited depth range of 34 m. As a result, the depth ranges for these fish are truncated, which can influence the perceived values of BIC and WIC (see section “Depth”). This “pseudo-repeatability” is expected to decrease the WIC, and, as a result, the repeatability score estimated might be inflated (Dingemanse and Dochtermann, 2013). To control for the possibility of biased repeatability scores, we also constructed depth models only for the four fish with the full depth range. The results of all sensitivity analyses are presented in Supplementary Material 2.
Results
Fish Tagged
During this study, 11 P. miles were tagged, 5 were tagged with Vemco transmitters, and 6 with Thelma Biotel (Table 1). Out of those, eight fish survived, and their detections were analyzed. The number of detections for each fish ranged from 3,861 to 2,300,697 and the tracking period ranged between 20 and 272 days.
Activity Patterns
The activity of all P. miles tracked, as emerged from the telemetry data, generally peaked around dusk and dawn, with most activities ±2 h from sunrise/sunset. However, when examining individual activity plots, it became clear that there was some P. miles activity level almost throughout the entire day (Figures 2A,B), as some individuals tended to nocturnal activity (e.g., fish #5), some toward diurnal activity (e.g., fish #1), and others stayed mainly crepuscular (Figures 2A,B). In addition, the model fitting separate activity curves for each individual was better supported by the data (Table 2, ΔAICc = 438), indicating individual variation in diel activity.
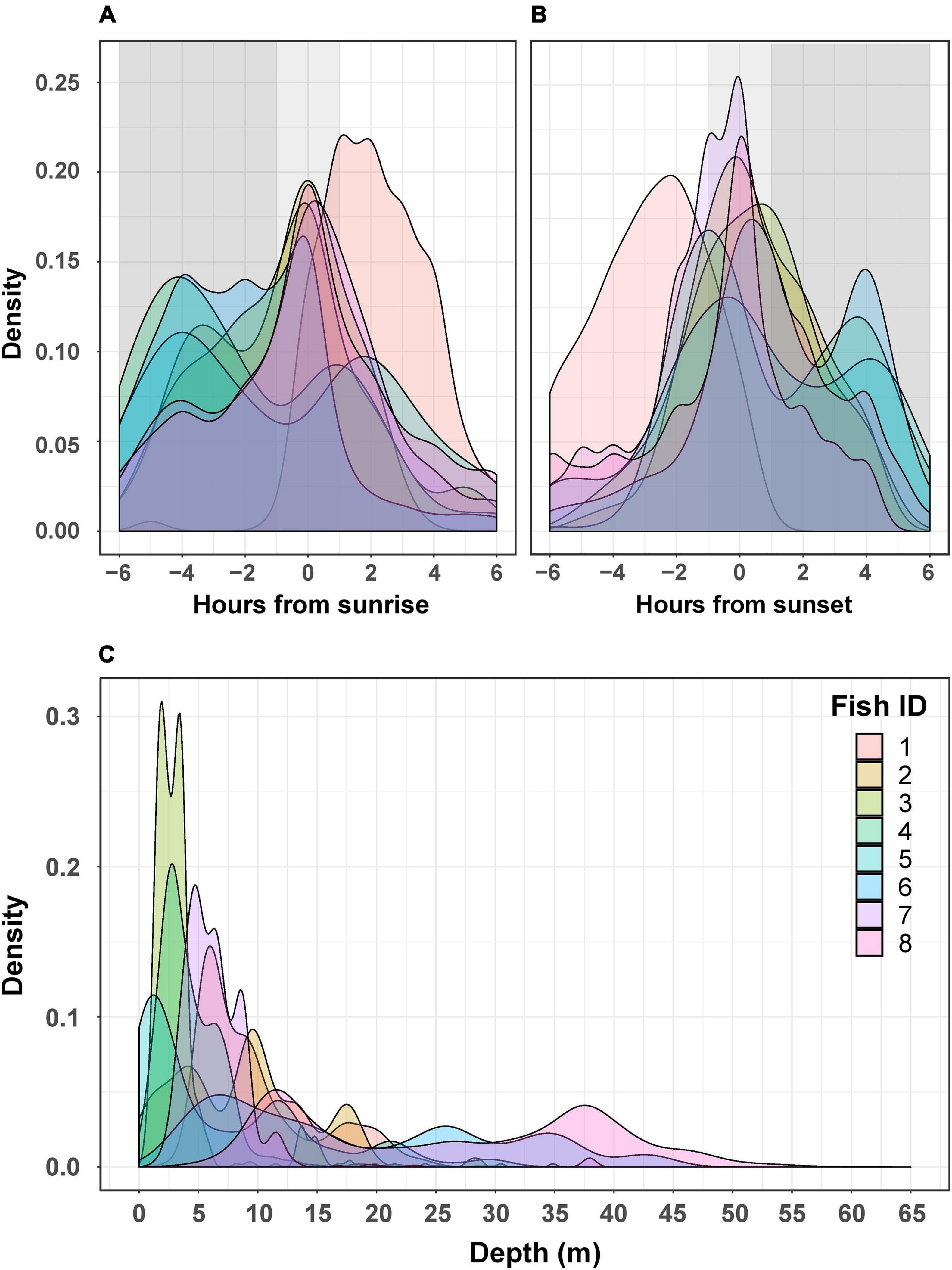
Figure 2. Activity (A,B) and depth (C) for each tagged P. miles. The top two graphs depict the density of active detection relative to time from sunrise (A) and time from sunset (B). Active detections were defined as the 30% most active half hours for each fish. The shaded dark gray areas represent night, and the light gray represents crepuscular hours (±1 h from sunset/sunrise). The bottom graph (C) shows the densities of detected depths for each individual.
When examining morning and evening activity peaks across days, we found that most activity peaks indeed occurred at sunrise or sunset (Figures 3A,B). However, in some cases, the maximum activity peak was as far as 6 h from sunrise or sunset. Some fish had distributions of daily activity peaks that resembled the distribution of all fish combined (Figures 3A,B), indicating low temporal repeatability. Accordingly, the adjusted repeatability scores for the morning and evening activity peaks were 0.21 (0.00–0.46, 95% CI) and 0.27 (0.003–0.53, 95% CI), respectively (Table 3). When examining the BIC and WIC, we found that the low repeatability scores were due to relatively high WIC (4.33 and 4.81 for the morning and evening activity peaks, respectively; Table 3) almost three times larger than the BIC.
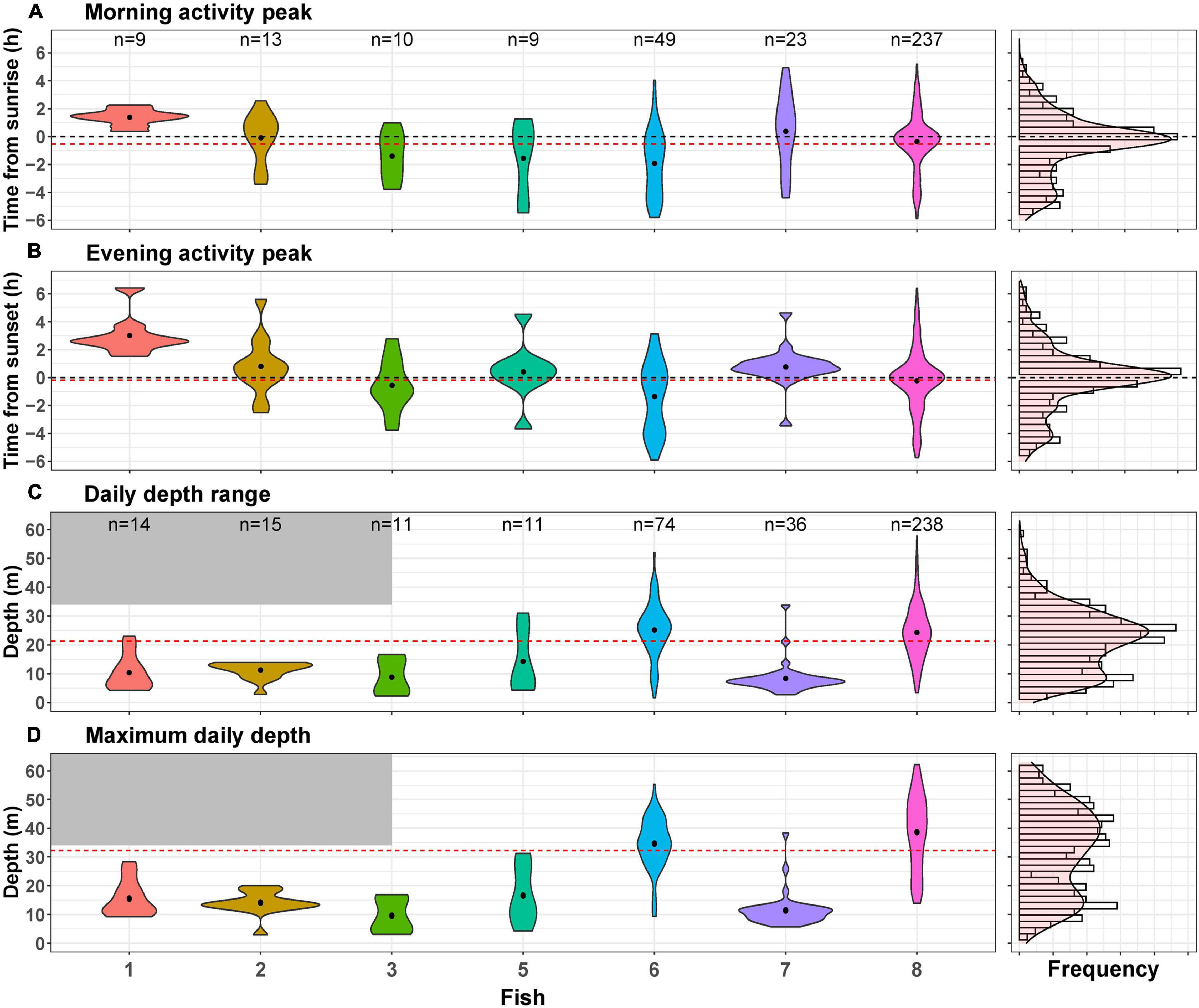
Figure 3. Distribution of the four daily indices across all individuals (right) and separately for each tagged individual (left). Dots in the violin plots represent the mean value for each fish, and the red dashed lines indicate the mean value for all fish. “n” represents the number of days used for each violin plot. (A) Morning activity peak, sunrise is denoted by a dashed black line. (B) Evening activity peak, sunset denoted by a dashed black line. (C) Daily depth range and (D) maximum daily depth. The gray shaded areas denote the Vemco transmitter depth limitation.
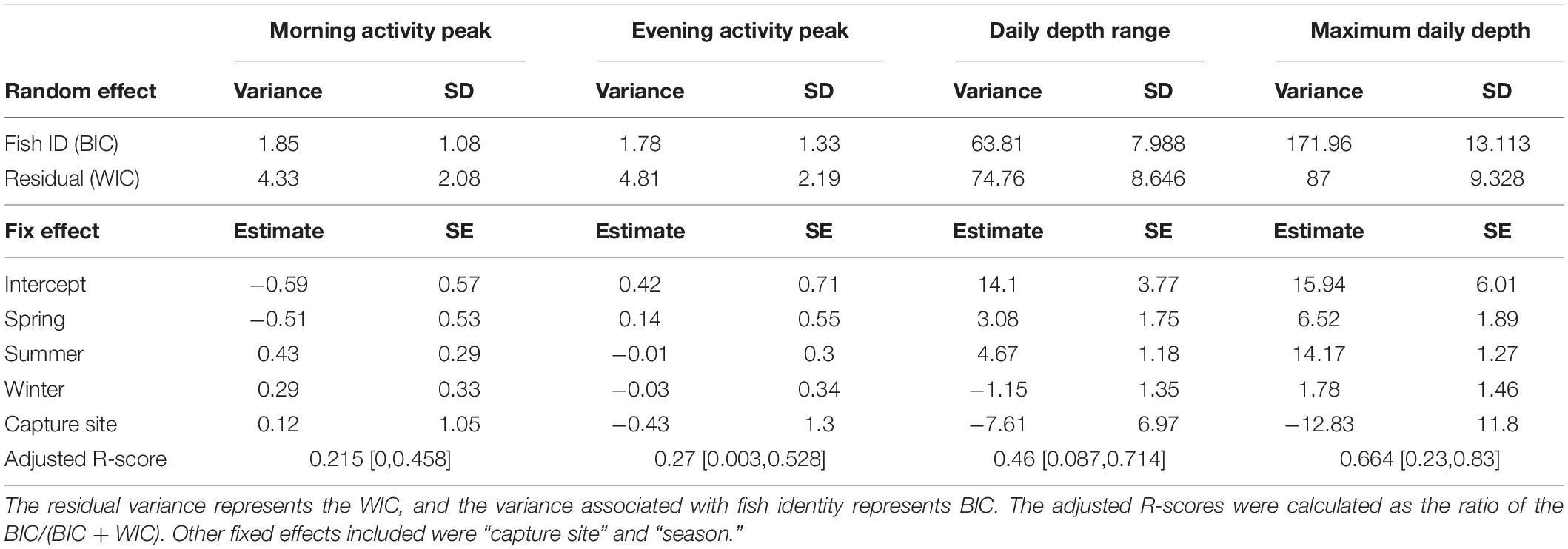
Table 3. Mixed effect model results including adjusted R-score, between-individual (BIC), and within-individual variation (WIC) for the daily indices (morning activity peak, evening activity peak, daily depth range, and maximum daily depth).
Depth Pattern
Fish were detected at depths of 0–64 m (Figure 2C); however, this is likely an underestimation of the fish’s full depth range as the tags’ transmits were depth-limited (see section “Materials and Methods”). We found that different fish had depths they tended to inhabit. Thus, the model that included “fish ID” as a random effect was better supported by the data than the model assuming all individuals had similar depth preferences (ΔAICc = 9234, Table 4). Nevertheless, individuals were not restricted to a specific depth, and individuals displayed varying depth patterns over time (Figures 2C, 4). The greatest daily depth range for any individual fish was 60 m (fish #8).
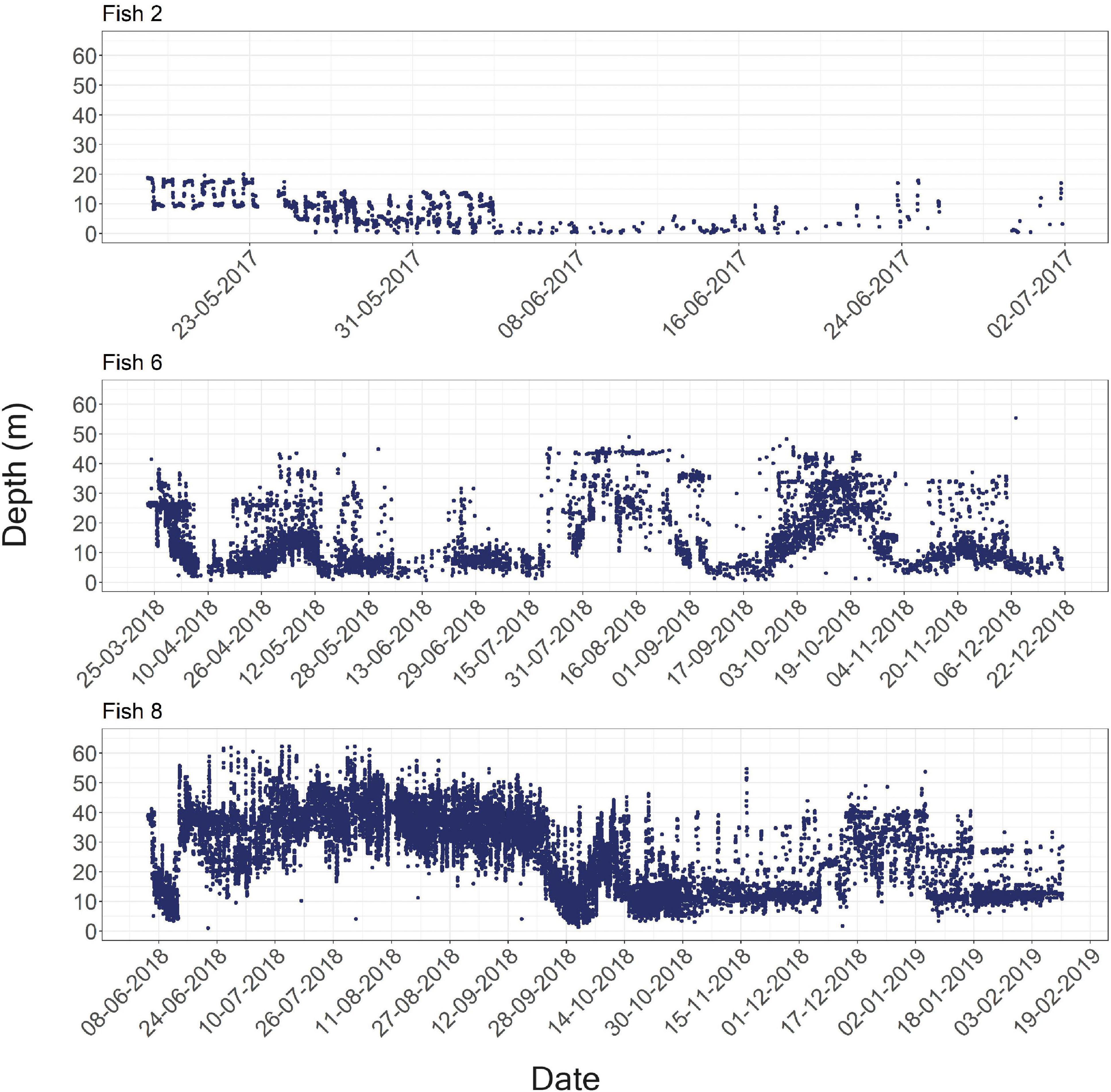
Figure 4. Examples of the raw depth recorded for the entire period each fish was detected. Fish numbers two, six, and eight are shown above, and data for all fish in the study appear in Supplementary Material 4.
We also examined depth pattern consistency and found that the daily depth range and the maximum daily depth varied considerably between individuals and within each specific fish over time (Figures 3C,D). Adjusted repeatability scores were 0.46 (0.08–0.71, 95% CI) for daily depth range and 0.64 (0.23–0.83, 95% CI) for maximum depth (Table 3). These scores represent medium to high repeatability. However, when examining the BIC and WIC, we found that the daily depth range index had similar BIC and WIC (63 and 75, respectively) and that for maximal depth, the BIC (172) was almost double the WIC (87, Table 3). These results imply that the relatively high repeatability scores for depth are attributed to the large differences between individuals (represented by BIC) that mask the changes in individual depth-use over time (represented by WIC).
Discussion
We found that within their native range, P. miles individuals show high variability in both depth use and activity time. All P. miles tracked were active at both sunrise and sunset, but some individuals tended to also be active during the day or night (Figures 2A,B), and individuals also utilized variable depths (Figures 2C, 4). Moreover, despite the small sample size (n = 8), we observed considerable within-individual variation between days along the day/night and depth axes. Activity peaks varied substantially across days for single individuals (Figure 3 and Table 3), and individuals varied their depth unpredictably over time. This behavioral flexibility demonstrated within the species’ native range may indicate a pre-adaptation allowing this species to become a successful invader in both the Atlantic and the Mediterranean.
Although lionfish as a species have been extensively studied (Morris et al., 2009; Cure et al., 2012; McCallister et al., 2018), only a few studies have specifically assessed variation between individuals. Evidence for individual variation comes from acoustic telemetry home-range studies. In two different studies, lionfish’s home-range in invaded seas ranged from 360–12,812 m2 (McCallister et al., 2018) to 158–4051 m2 (Dahl and Patterson, 2020), suggesting high variation among individuals. In addition, a study in North Carolina found large variability in the number of receivers that detected each lionfish, indirectly implying variable home-ranges (Bacheler et al., 2015). Here, we extend the findings of the large variability in home-range between individuals to both depth use and diel activity.
Lionfish’s ability to be active at different hours throughout the day and night may increase the number of potential prey species, especially in an invaded range where the native fish are naïve (Agostino et al., 2020). Usually, marine species have highly conserved diel activity patterns (Helfman, 1978, 1986; Reebs, 2002; Pickholtz et al., 2018; but see Fox and Bellwood, 2011). Even among crepuscular species that need to adjust to dark and light conditions, individual variation is unusual (Helfman, 1986; Løkkeborg et al., 2000). For example, Ling fish (Molva molva) show a consistent crepuscular activity pattern with little variation among individuals (Løkkeborg et al., 2000). In this context, the diel flexibility of P. miles is impressive as it occurs both between individuals and within individuals over time.
Lionfish depth profiles show that some individuals occupy different depths across days (fish #6 and fish #8), while others consistently inhabit shallow areas (fish #5 and fish #7). The reason for the large variation in depth-use patterns between individuals can either be distinct personalities and depth preferences, or the depth distribution of habitats at different locations. For example, over the southern sites, where fish preferred mostly shallow depths, there is no deep reef but rather a soft bottom substrate that might be less appealing for lionfish. The depth-related repeatability scores suggest conserved individual depth use (Table 3). Nevertheless, these high repeatability scores originated from the high variation between individuals (BIC) that obscured the variation within individuals over time (WIC). Thus, the results also demonstrate that depth shifts within individuals are common and can occur over short time scales. For example, fish #8 crossed more than 60 m in a single day (Figure 3C).
The ability of individuals to move between shallow and mesophotic reefs has substantial implications for the effectiveness of shallow reef culling (Andradi-Brown et al., 2017a,b). Lionfish removal is typically restricted to shallow depths (Côté et al., 2014b; Usseglio et al., 2017), including in the Mediterranean Sea (Kleitou et al., 2021). Our findings demonstrate frequent movements of lionfish between shallow and mesophotic habitats over short time intervals. These findings raise the possibility that culled shallow-water populations can be replenished, even daily, with individuals from deeper waters. In support, a study integrating data from numerous western Atlantic locations found that shallow water culling programs did not always alter the depth abundance distribution of lionfish (Andradi-Brown et al., 2017b). At the same time, large individual-level mobility may also imply that shallow water culling can impact the lionfish population at deeper depths (similar to an edge effect in marine protected areas; Ohayon et al., 2021).
Past studies have demonstrated lionfish’s ability to react to culling efforts. For example, lionfish in culled areas in the Bahamas tended to be less active during daytime compared to lionfish inhabiting non-culled reefs (Côté et al., 2014b). In the Atlantic Ocean, non-culled mesophotic lionfish had a similarly high alert rate to divers as lionfish in culled shallow areas (Andradi-Brown et al., 2017a). Understanding both the location-specific level of connectivity and the ability of individuals to change behaviors in response to fishing is critical to designing and implementing optimal culling programs.
This study has several caveats; the most prominent one is the small sample size. While larger sample sizes would have provided a more detailed understanding of the observed patterns and may lead to increased depth range and activity hour estimates, even from the current sample size, the variation in activity and depth of P. miles between and within individuals is clear and striking. Another caveat is that some of the study fish were tagged with transmitters with limited maximal depth (34 m). Although excluding those fish did not change the results (see Supplementary Material 2), the variability and range of depth estimates presented here are likely underestimated. In addition, the use of two acoustic telemetry systems possessing different detection ranges and array configuration limited our ability to conduct movement and home range assessments, preventing direct comparison to former studies exploring those traits in the invaded range. Finally, we only examined two important behavioral axes. Estimating additional traits, such as diet or space-use patterns, could provide complementary views of this species’ behavior.
This study was conducted within the native environment of P. miles in the Red Sea. The “ecological release” theory posits that invasive species may be freed from the biotic limitations in their native range and thus appear as generalist species (Van Valen, 1965; Bolnick et al., 2002, 2010, 2011; Pickholtz et al., 2018). The observation of flexible behaviors and wide niche-breadth for P. miles in the native range suggests that the plasticity of lionfish in the invaded ranges is not solely the result of “ecological release.” Instead, the behavioral flexibility appears to be a pre-adaptation that may have facilitated its invasion success.
Data Availability Statement
The raw data supporting the conclusions of this article will be made available by the authors, without undue reservation.
Ethics Statement
The animal study was reviewed and approved by the Institutional Animal Care and Use Committee, Ben Gurion University (authorization number IL-18-04-2018c).
Author Contributions
TG, RP, and JB conceived of the presented idea. TG performed the fieldwork, analyses, and wrote the manuscript. RP contributed to the design and implementation of the research and the analysis of the results. JB supervised the findings of this work. All authors discussed the results and contributed to the final manuscript.
Conflict of Interest
The authors declare that the research was conducted in the absence of any commercial or financial relationships that could be construed as a potential conflict of interest.
Publisher’s Note
All claims expressed in this article are solely those of the authors and do not necessarily represent those of their affiliated organizations, or those of the publisher, the editors and the reviewers. Any product that may be evaluated in this article, or claim that may be made by its manufacturer, is not guaranteed or endorsed by the publisher.
Acknowledgments
This study has been made possible thanks to the facilities and the professional team of The Interuniversity Institute for Marine Sciences (IUI) in Eilat. We wish to thank Moshe Kiflawi for his support and all those who helped with fieldwork, especially Noam Sommerfeld, Ori Frid, Hezi Buba, Mai Lazrus, and Shahar Malamud. We would also like to thank Daphna Shapiro Goldberg for her important insights.
Supplementary Material
The Supplementary Material for this article can be found online at: https://www.frontiersin.org/articles/10.3389/fmars.2021.790930/full#supplementary-material
References
Agostino, D. D., Jimenez, C., Reader, T., Hadjioannou, L., Heyworth, S., Aplikioti, M., et al. (2020). Behavioural traits and feeding ecology of Mediterranean lionfish and naiveté of native species to lionfish predation. Mar. Ecol. Prog. Ser. 638, 123–135. doi: 10.3354/meps13256
Akaike, H. (1987). “Factor analysis and AIC,” in Selected Papers of Hirotugu Akaike, eds E. Parzen, K. Tanabe, and G. Kitagawa (New York, NY: Springer), 371–386.
Albins, M. A. (2013). Effects of invasive Pacific red lionfish Pterois volitans versus a native predator on Bahamian coral-reef fish communities. Biol. Invasions 15, 29–43.
Albins, M. A., and Hixon, M. A. (2008). Invasive Indo-Pacific lionfish Pterois volitans reduce recruitment of Atlantic coral-reef fishes. Mar. Ecol. Prog. Ser. 367, 233–238.
Albins, M. A., and Hixon, M. A. (2013). Worst case scenario: potential long-term effects of invasive predatory lionfish (Pterois volitans) on Atlantic and Caribbean coral-reef communities. Environ. Biol. Fishes 96, 1151–1157. doi: 10.1007/s10641-011-9795-1
Albins, M. A., and Lyons, P. J. (2012). Invasive red lionfish Pterois volitans blow directed jets of water at prey fish. Mar. Ecol. Prog. Ser. 448, 1–5.
Albrecht, M., and Gotelli, N. (2001). Spatial and temporal niche partitioning in grassland ants. Oecologia 126, 134–141. doi: 10.1007/s004420000494
Alós, J., Martorell-Barceló, M., and Campos-Candela, A. (2017). Repeatability of circadian behavioural variation revealed in free-ranging marine fish. R. Soc. Open Sci. 4:160791. doi: 10.1098/rsos.160791
Andradi-Brown, D. A., Grey, R., Hendrix, A., Hitchner, D., Hunt, C. L., Gress, E., et al. (2017a). Depth-dependent effects of culling—do mesophotic lionfish populations undermine current management? R. Soc. Open Sci. 4:170027. doi: 10.1098/rsos.170027
Andradi-Brown, D. A., Vermeij, M. J., Slattery, M., Lesser, M., Bejarano, I., Appeldoorn, R., et al. (2017b). Large-scale invasion of western Atlantic mesophotic reefs by lionfish potentially undermines culling-based management. Biol. Invasions 19, 939–954.
Araújo, M. S., Bolnick, D. I., and Layman, C. A. (2011). The ecological causes of individual specialisation. Ecol. Lett. 14, 948–958.
Araújo, M. S., and Gonzaga, M. O. (2007). Individual specialization in the hunting wasp Trypoxylon (Trypargilum) albonigrum (Hymenoptera, Crabronidae). Behav. Ecol. Sociobiol. 61, 1855–1863. doi: 10.1007/s00265-007-0425-z
Azzurro, E., Stancanelli, B., Di Martino, V., and Bariche, M. (2017). Range expansion of the common lionfish Pterois miles (Bennett, 1828) in the Mediterranean Sea: an unwanted new guest for Italian waters. Bioinvasions Rec. 6, 95–98. doi: 10.3391/bir.2017.6.2.01
Bacheler, N. M., Whitfield, P. E., Muñoz, R. C., Harrison, B. B., Harms, C. A., and Buckel, C. A. (2015). Movement of invasive adult lionfish Pterois volitans using telemetry: importance of controls to estimate and explain variable detection probabilities. Mar. Ecol. Prog. Ser. 527, 205–220.
Bierbach, D., Laskowski, K. L., Brandt, A.-L., Chen, W., Jourdan, J., Streit, B., et al. (2016). Highly variable, unpredictable activity patterns in invasive, but not native amphipod species. Aquat. Ecol. 50, 261–271.
Bolnick, D. I., Amarasekare, P., Araújo, M. S., Bürger, R., Levine, J. M., Novak, M., et al. (2011). Why intraspecific trait variation matters in community ecology. Trends Ecol. Evol. 26, 183–192. doi: 10.1016/j.tree.2011.01.009
Bolnick, D. I., Ingram, T., Stutz, W. E., Snowberg, L. K., Lau, O. L., and Paull, J. S. (2010). Ecological release from interspecific competition leads to decoupled changes in population and individual niche width. Proc. R. Soc. B Biol. Sci. 277, 1789–1797. doi: 10.1098/rspb.2010.0018
Bolnick, D. I., Svanbäck, R., Fordyce, J. A., Yang, L. H., Davis, J. M., Hulsey, C. D., et al. (2002). The ecology of individuals: incidence and implications of individual specialization. Am. Nat. 161, 1–28. doi: 10.1086/343878
Brokovich, E. (2001). The Community Structure and Biodiversity of Reef Fishes at the Northern Gulf of Aqaba (Red Sea) with Relation to Their Habitat. Faculty of Life Sciences. Tel Aviv-Yafo: Tel Aviv University, 116.
Chapple, D. G., Simmonds, S. M., and Wong, B. B. (2012). Can behavioral and personality traits influence the success of unintentional species introductions? Trends Ecol. Evol. 27, 57–64. doi: 10.1016/j.tree.2011.09.010
Côté, I. M., Akins, L., Underwood, E., Curtis-Quick, J., and Green, S. J. (2014a). Setting the record straight on invasive lionfish control: culling works. PeerJ Prepr. 2:e398v391.
Côté, I. M., Darling, E. S., Malpica-Cruz, L., Smith, N. S., Green, S. J., Curtis-Quick, J., et al. (2014b). What doesn’t kill you makes you wary? Effect of repeated culling on the behaviour of an invasive predator. PLoS One 9:e94248. doi: 10.1371/journal.pone.0094248
Côté, I. M., Green, S. J., and Hixon, M. A. (2013a). Predatory fish invaders: insights from Indo-Pacific lionfish in the western Atlantic and Caribbean. Biol. Conserv. 164, 50–61. doi: 10.1111/1365-2656.12250
Côté, I. M., Green, S. J., Morris, J. A. Jr., Akins, J. L., and Steinke, D. (2013b). Diet richness of invasive Indo-Pacific lionfish revealed by DNA barcoding. Mar. Ecol. Prog. Ser. 472, 249–256. doi: 10.3354/meps09992
Cure, K., Benkwitt, C. E., Kindinger, T. L., Pickering, E. A., Pusack, T. J., Mcilwain, J. L., et al. (2012). Comparative behavior of red lionfish Pterois volitans on native Pacific versus invaded Atlantic coral reefs. Mar. Ecol. Prog. Ser. 467, 181–192. doi: 10.3354/meps09942
Currey, L. M., Heupel, M. R., Simpfendorfer, C. A., and Williams, A. J. (2014). Sedentary or mobile? Variability in space and depth use of an exploited coral reef fish. Mar. Biol. 161, 2155–2166. doi: 10.1007/s00227-014-2497-4
Dahl, K. A., and Patterson, W. F. (2020). Movement, home range, and depredation of invasive lionfish revealed by fine-scale acoustic telemetry in the northern Gulf of Mexico. Mar. Biol. 167, 1–22.
Dingemanse, N. J., and Dochtermann, N. A. (2013). Quantifying individual variation in behaviour: mixed-effect modelling approaches. J. Anim. Ecol. 82, 39–54. doi: 10.1111/1365-2656.12013
Duckworth, R. A. (2008). Adaptive dispersal strategies and the dynamics of a range expansion. Am. Nat. 172, S4–S17.
Duckworth, R. A., and Badyaev, A. V. (2007). Coupling of dispersal and aggression facilitates the rapid range expansion of a passerine bird. Proc. Natl. Acad. Sci. U.S.A. 104, 15017–15022. doi: 10.1073/pnas.0706174104
Dunn, M. R., Griggs, L., Forman, J., and Horn, P. (2010). Feeding habits and niche separation among the deep-sea chimaeroid fishes Harriotta raleighana, Hydrolagus bemisi and Hydrolagus novaezealandiae. Mar. Ecol. Prog. Ser. 407, 209–225.
Fishelson, L. (1997). Experiments and observations on food consumption, growth and starvation in Dendrochirus brachypterus and Pterois volitans (Pteroinae, Scorpaenidae). Environ. Biol. Fishes 50, 391–403. doi: 10.1023/a:1007331304122
Fogarty, S., Cote, J., and Sih, A. (2011). Social personality polymorphism and the spread of invasive species: a model. Am. Nat. 177, 273–287. doi: 10.1086/658174
Fox, R. J., and Bellwood, D. R. (2011). Unconstrained by the clock? Plasticity of diel activity rhythm in a tropical reef fish, Siganus lineatus. Funct. Ecol. 25, 1096–1105. doi: 10.1111/j.1365-2435.2011.01874.x
Gavriel, T., and Belmaker, J. (2020). Little spatial and temporal segregation between coexisting lionfishes (Pterois miles and Pterois radiata) in the Red Sea. Isr. J. Ecol. Evol. 1, 1–9.
Ghanawi, J., Monzer, S., and Saoud, I. P. (2013). Anaesthetic efficacy of clove oil, benzocaine, 2-phenoxyethanol and tricaine methanesulfonate in juvenile marbled spinefoot (Siganus rivulatus). Aquac. Res. 44, 359–366.
Golani, D. (1994). Niche separation between colonizing and indigenous goatfish (Mullidae) along the Mediterranean coast of Israel. J. Fish Biol. 45, 503–513.
Golani, D., and Sonin, O. (1992). New records of the Red Sea fishes, Pterois miles (Scorpaenidae) and Pteragogus pelycus (Labridae) from the eastern Mediterranean Sea. Jpn. J. Ichthyol. 39, 167–169. doi: 10.1007/bf02906001
Green, S. J., Akins, J. L., Maljković, A., and Côté, I. M. (2012). Invasive lionfish drive Atlantic coral reef fish declines. PLoS One 7:e32596. doi: 10.1371/journal.pone.0032596
Gress, E., Andradi-Brown, D. A., Woodall, L., Schofield, P. J., Stanley, K., and Rogers, A. D. (2017). Lionfish (Pterois spp.) invade the upper-bathyal zone in the western Atlantic. PeerJ 5:e3683. doi: 10.7717/peerj.3683
Gross, K., Pasinelli, G., and Kunc, H. P. (2010). Behavioral plasticity allows short-term adjustment to a novel environment. Am. Nat. 176, 456–464. doi: 10.1086/655428
Hackerott, S., Valdivia, A., Green, S. J., Côté, I. M., Cox, C. E., Akins, L., et al. (2013). Native predators do not influence invasion success of Pacific lionfish on Caribbean reefs. PLoS One 8:e68259. doi: 10.1371/journal.pone.0068259
Harmelin-Vivien, M., and Bouchon, C. (1976). Feeding behavior of some carnivorous fishes (Serranidae and Scorpaenidae) from Tulear (Madagascar). Mar. Biol. 37, 329–340.
Harvey, B., and Stewart, A. (1991). Fish size and habitat depth relationships in headwater streams. Oecologia 87, 336–342. doi: 10.1007/BF00634588
Hastie, T. J. (2017). “Generalized additive models,” in Statistical Models in S, ed. S. N. Wood (Boca Raton, FL: Routledge), 249–307.
Helfman, G. S. (1978). Patterns of community structure in fishes: summary and overview. Environ. Biol. Fishes 3, 129–148.
Helfman, G. S. (1986). “Fish behaviour by day, night and twilight,” in The Behaviour of Teleost Fishes, ed. T. J. Pitcher (Boston, MA: Springer), 366–387. doi: 10.1007/s00359-007-0247-5
Holt, R. D., Barfield, M., and Gomulkiewicz, R. (2004). Temporal variation can facilitate niche evolution in harsh sink environments. Am. Nat. 164, 187–200. doi: 10.1086/422343
Jud, Z. R., Layman, C. A., Lee, J. A., and Arrington, D. A. (2011). Recent invasion of a Florida (USA) estuarine system by lionfish Pterois volitans/P. miles. Aquat. Biol. 13, 21–26.
Kiflawi, M., Belmaker, J., Brokovich, E., Einbinder, S., and Holzman, R. (2006). The determinants of species richness of a relatively young coral-reef ichthyofauna. J. biogeogr. 33, 1289–1294.
Kleitou, P., Rees, S., Cecconi, F., Kletou, D., Savva, I., Cai, L. L., et al. (2021). Regular monitoring and targeted removals can control lionfish in Mediterranean Marine Protected Areas. Aquat. Conserv. Mar. Freshw. Ecosyst. 31, 2870–2882.
Kocárek, P. (2001). Diurnal activity rhythms and niche differentiation in a carrion beetle assemblage (Coleoptera: Silphidae) in Opava, the Czech Republic. Biol. Rhythm Res. 32, 431–438. doi: 10.1076/brhm.32.4.431.1333
Kronfeld-Schor, N., and Dayan, T. (2003). Partitioning of time as an ecological resource. Annu. Rev. Ecol. Evol. Syst. 34, 153–181. doi: 10.1098/rsos.160175
Larson, R. J. (1980). Competition, habitat selection, and the bathymetric segregation of two rockfish (Sebastes) species. Ecol. Monogr. 50, 221–239.
Lee, S., Buddo, D. S., and Aiken, K. A. (2012). “Habitat preference in the invasive lionfish (Pterois volitans/miles) in Discovery Bay, Jamaica: use of GIS in management strategies,” in Proceedings of the 64rd Gulf and Caribbean Fisheries Institute, Puerto Morelos, 39–48.
Lieske, E., Fiedler, K. E., and Myers, R. F. (2004). Coral Reef Guide: Red Sea to Gulf of Aden, South Oman; the Definitive Guide to Over 1200 Species of Underwater Life. London: Collins.
Løkkeborg, S., Skajaa, K., and Fernö, A. (2000). Food-search strategy in ling (Molva molva L.): crepuscular activity and use of space. J. Exp. Mar. Biol. Ecol. 247, 195–208. doi: 10.1016/s0022-0981(00)00148-9
Longhurst, A. R. (1985). Relationship between diversity and the vertical structure of the upper ocean. Deep Sea Res. A 32, 1535–1570.
Malcolm, H. A., Jordan, A., and Smith, S. D. (2011). Testing a depth-based habitat classification system against reef fish assemblage patterns in a subtropical marine park. Aquat. Conserv. Mar. Freshw. Ecosyst. 21, 173–185.
McCallister, M., Renchen, J., Binder, B., and Acosta, A. (2018). Diel activity patterns and movement of invasive lionfish (Pterois volitans/P. miles) in the Florida keys identified using acoustic telemetry. Gulf Caribb. Res. 29, 27–40. doi: 10.18785/gcr.2901.13
Mctee, S. A., and Grubich, J. R. (2014). Native densities, distribution, and diurnal activity of Red Sea lionfishes (Scorpaenidae). Mar. Ecol. Prog. Ser. 508, 223–232.
Morris, J. A., and Akins, J. L. (2009). Feeding ecology of invasive lionfish (Pterois volitans) in the Bahamian archipelago. Environ. Biol. Fish. 86:389. doi: 10.1007/s10641-009-9538-8
Morris, J., and Creswell, L. (2013). Invasive Lionfish: A Guide to Control and Management. Marathon, FL: Gulf and Caribbean Fisheries Institute Special Publication.
Morris, J. A. Jr., Akins, J., Barse, A., Cerino, D., Freshwater, D., Green, S., et al. (2009). “Biology and ecology of the invasive lionfishes, Pterois miles and Pterois volitans,” in Proceedings of the 61st Gulf and Caribbean Fisheries Institute, Gosier, 409–414.
Nakagawa, S., and Schielzeth, H. (2010). Repeatability for Gaussian and non-Gaussian data: a practical guide for biologists. Biol. Rev. 85, 935–956. doi: 10.1111/j.1469-185X.2010.00141.x
Nakayama, S., Laskowski, K. L., Klefoth, T., and Arlinghaus, R. (2016). Between-and within-individual variation in activity increases with water temperature in wild perch. Behav. Ecol. 27, 1676–1683.
Nussey, D., Wilson, A., and Brommer, J. (2007). The evolutionary ecology of individual phenotypic plasticity in wild populations. J. Evol. Biol. 20, 831–844. doi: 10.1111/j.1420-9101.2007.01300.x
Nuttall, M. F., Johnston, M. A., Eckert, R. J., Embesi, J. A., Hickerson, E. L., and Schmahl, G. P. (2014). Lionfish (Pterois volitans [Linnaeus, 1758] and P. miles [Bennett, 1828]) records within mesophotic depth ranges on natural banks in the Northwestern Gulf of Mexico. Bioinvasions Rec. 3, 111–115.
Ohayon, S., Granot, I., and Belmaker, J. (2021). A meta-analysis reveals edge effects within marine protected areas. Nat. Ecol. Evol. 5, 1301–1308. doi: 10.1038/s41559-021-01502-3
Pickholtz, R. S., Kiflawi, M., Friedlander, A. M., and Belmaker, J. (2018). Habitat utilization by an invasive herbivorous fish (Siganus rivulatus) in its native and invaded range. Biol. Invasions 20, 3499–3512. doi: 10.1007/s10530-018-1790-4
Reebs, S. G. (2002). Plasticity of diel and circadian activity rhythms in fishes. Rev. Fish Biol. Fish. 12, 349–371.
Sade, A. R., Hall, J. K., Tibor, G., Niemi, T. M., Ben-Avraham, Z., Al-Zoubi, A. A., et al. (2008). The Israel national bathymetric survey: northern Gulf of ‘Aqaba/Elat Poster. Isr. J. Earth Sci. 57, 139–144. doi: 10.1560/ijes.57.2.139
Sagata, K., and Lester, P. J. (2009). Behavioural plasticity associated with propagule size, resources, and the invasion success of the Argentine ant Linepithema humile. J. Appl. Ecol. 46, 19–27. doi: 10.1111/j.1365-2664.2008.01523.x
Stern, N., and Rothman, S. B. (2018). “Iron lion zion: the successful, albeit lingered, invasion of the lionfish in the Israeli Mediterranean Sea,” in Lionfish Invasion and Its Management in the Mediterranean Sea, eds M. F. Hüseyinoğlu and B. Öztürk (Istanbul: Turkish Marine Research Foundation (TUDAV)), 121.
Stuber, E. F., Dingemanse, N. J., Kempenaers, B., and Mueller, J. C. (2015). Sources of intraspecific variation in sleep behaviour of wild great tits. Anim. Behav. 106, 201–221.
Sugiura, N. (1978). Further analysts of the data by akaike’s information criterion and the finite corrections: further analysts of the data by akaike’s. Commun. Stat. Theory Methods 7, 13–26. doi: 10.1080/03610927808827599
Tibor, G., Niemi, T. M., Ben-Avraham, Z., Al-Zoubi, A., Sade, R. A., Hall, J. K., et al. (2010). Active tectonic morphology and submarine deformation of the northern Gulf of Eilat/Aqaba from analyses of multibeam data. Geo Mar. Lett. 30, 561–573. doi: 10.1007/s00367-010-0194-y
Tuttle, L. J. (2017). Direct and indirect effects of invasive lionfish on coral-reef cleaning mutualists. Mar. Ecol. Prog. Ser. 569, 163–172. doi: 10.3354/meps12092
Tuttle, L. J., Sikkel, P. C., Cure, K., and Hixon, M. A. (2017). Parasite-mediated enemy release and low biotic resistance may facilitate invasion of Atlantic coral reefs by Pacific red lionfish (Pterois volitans). Biol. Invasions 19, 563–575. doi: 10.1007/s10530-016-1342-8
Ulman, A., Harris, H. E., Doumpas, N., Deniz Akbora, H., Mabruk, A., Azzurro, E., et al. (2021). Low pufferfish and lionfish predation in their native and invaded ranges suggests human control mechanisms may be necessary to control their Mediterranean abundances. Front. Mar. Sci. 8:670413. doi: 10.3389/fmars.2021.670413
Usseglio, P., Selwyn, J. D., Downey-Wall, A. M., and Hogan, J. D. (2017). Effectiveness of removals of the invasive lionfish: how many dives are needed to deplete a reef? PeerJ 5:e3043. doi: 10.7717/peerj.3043
Valeix, M., Chamaillé-Jammes, S., and Fritz, H. (2007). Interference competition and temporal niche shifts: elephants and herbivore communities at waterholes. Oecologia 153, 739–748. doi: 10.1007/s00442-007-0764-5
Van Snik Gray, E., and Stauffer, J. R. (1999). Comparative microhabitat use of ecologically similar benthic fishes. Environ. Biol. Fishes 56, 443–453. doi: 10.1023/a:1007536019444
Van Valen, L. (1965). Morphological variation and width of ecological niche. Am. Nat. 99, 377–390. doi: 10.1086/282379
Keywords: repeatability, invasion, acoustic telemetry, Red Sea, native range, Gulf of Aqaba
Citation: Gavriel T, Pickholtz R and Belmaker J (2021) Large Individual-Level Variability in Diel Activity and Depth Use for the Common Lionfish (Pterois miles). Front. Mar. Sci. 8:790930. doi: 10.3389/fmars.2021.790930
Received: 07 October 2021; Accepted: 24 November 2021;
Published: 23 December 2021.
Edited by:
Periklis Kleitou, Marine and Environmental Research Lab (MER), CyprusReviewed by:
José Lino Vieira De Oliveira Costa, University of Lisbon, PortugalPaul Carl Sikkel, University of Miami, United States
Copyright © 2021 Gavriel, Pickholtz and Belmaker. This is an open-access article distributed under the terms of the Creative Commons Attribution License (CC BY). The use, distribution or reproduction in other forums is permitted, provided the original author(s) and the copyright owner(s) are credited and that the original publication in this journal is cited, in accordance with accepted academic practice. No use, distribution or reproduction is permitted which does not comply with these terms.
*Correspondence: Tal Gavriel, dGFsZ2F2QGdtYWlsLmNvbQ==
†ORCID: Tal Gavriel, orcid.org/0000-0001-8657-0060; Renanel Pickholtz, orcid.org/0000-0001-7319-991X; Jonathan Belmaker, orcid.org/0000-0002-5618-7359