- 1Laboratoire d’Excellence Labex-CORAIL, Institut de Recherche pour le Développement (IRD), UMR ENTROPIE (IRD-Université de La Réunion-CNRS), Nouméa, New Caledonia
- 2Department of Climate Geochemistry, Max Planck Institute for Chemistry (Otto Hahn Institute), Mainz, Germany
- 3Department of Biological Oceanography, Leibniz Institute for Baltic Sea Research Warnemünde, Rostock, Germany
- 4University of Brest, CNRS, IRD, Ifremer, LEMAR, Plouzané, France
- 5Department of Geology, Union College, Schenectady, NY, United States
- 6Division of Biological and Environmental Science and Engineering, Red Sea Research Center, King Abdullah University of Science and Technology, Thuwal, Saudi Arabia
The ability of corals to modulate their nutrition strategy in response to variable nutrient supply remains poorly understood, limiting our understanding of energy flow in coral reef ecosystems and thus our comprehension of their resilience to global changes. We used a naturally occurring nutrient gradient along the reef flat of two seabird-inhabited islets in the SW Pacific to characterize spatiotemporal fluctuations in coastal nutrient availability, and how it modulates the trophic response of the mixotrophic coral Pocillopora damicornis. The clear gradients in dissolved [NOx] and δ15N values of macroalgae and both P. damicornis tissues and symbionts observed along the reef flat during the dry and the rainy season revealed that seabird-derived-N is supplied year-round to the reef flat. Yet, nitrogen isotope values of macroalgae show that the seabirds’ effect on coral reefs varies with sites and seasons. Metrics derived from the SIBER framework revealed that coral nutrition seasonally favored autotrophy when exposed to higher seabird guano concentrations and at inshore stations, while heterotrophy dominated in corals less exposed to seabird-derived nutrient supply. P. Damicornis is therefore able to cope with large changes in nitrogen supply induced by seabird island communities by switching between autotrophy and heterotrophy. These results shed light on the flexibility of resource sharing within the coral-algae symbiosis and highlight the importance of seabird populations to the functioning of coral reef ecosystems.
Introduction
The global success of shallow-water corals relies heavily on their symbiotic association with photosynthetic intracellular dinoflagellate microalgae (Symbiodiniaceae, LaJeunesse et al., 2018). The symbiosis supplies the coral with photosynthetically fixed carbon and inorganic N (autotrophy) (Muscatine and Porter, 1977; Muscatine et al., 1981). In parallel, the coral tissues can also incorporate a wide range of food sources from dissolved organic matter to planktonic prey (heterotrophy) (Houlbrèque and Ferrier-Pagès, 2009). The dominance of one trophic strategy over the other relies on environmental factors such as depth, turbidity, or resource availability (Anthony and Fabricius, 2000; Palardy et al., 2005; Fox et al., 2019). Recent findings show for instance, that mixotrophic corals increase heterotrophy when located in shallow waters surrounding islands with elevated nearshore primary production (Fox et al., 2018). Variations in coral nutritional plasticity is considered a robust predictor of corals’ resilience to bleaching, which results from a disruption of the symbiose due to ocean warning (e.g., Grottoli et al., 2006; Conti-Jerpe et al., 2020; Thibault et al., 2021).
Elevated nutrient concentration in coastal waters surrounding remote seabird-inhabited islets was recently highlighted at several locations (Honig and Mahoney, 2016; Lorrain et al., 2017; Shatova et al., 2017; Otero et al., 2018). Reports included high NOx concentrations at coastal locations, enhanced phytoplankton productivity, and the assimilation of 15N-enriched nitrogen by coastal organisms (Wainright et al., 1998; Lorrain et al., 2017). Islands with large seabird populations have been correlated with enhanced reef productivity and functioning (Graham et al., 2018), increased plant and animal biomasses, and enhanced community response to climate change (Benkwitt et al., 2019). In particular, a previous study reported that coastal corals assimilate up to 50% of their nitrogen from guano-derived nutrients close to islets with high seabird densities (Lorrain et al., 2017). Yet, the spatiotemporal dynamics of this natural nutrient supply and its influence on coral nutrition has not been thoroughly investigated. In particular, resource sharing between the coral and the symbiont under variable nutrient supply is poorly understood.
Evaluating the trophic behavior of coral individuals is critical to evaluate their resilience to global changes; yet it is technically challenging. Conventional ex situ feeding experiments are costly, time-consuming, and are difficult to relate to in situ processes (Houlbrèque and Ferrier-Pagès, 2009). Stable isotope ratio analysis of in situ corals is therefore promising for determining the relative importance of autotrophy and heterotrophy in corals (Muscatine et al., 1989; Ferrier-Pagès et al., 2011; Conti-Jerpe et al., 2020). An analytical framework based on statistical inferences of isotope niches in biplots (Jackson et al., 2011), was recently adapted to symbiotic organisms including corals (Conti-Jerpe et al., 2020; Thibault et al., 2021). This method presents the advantage of being based on bulk CN isotope analyses, thereby providing a less expensive, faster, yet robust estimate of coral trophic strategy.
The coral genus Pocillopora includes fast-growing and competitive species that are found throughout the Pacific and the Indian Oceans. Some species of Pocillopora have shown an exceptional trophic flexibility, being able to acquire nutrients through various degrees of autotrophy and heterotrophy (e.g., P. meandrina, Fox et al., 2019) which suggests that this genus is well suited to cope with variable N-supply. Paradoxically, this genus is not capable of regulating the nutrient uptake of its symbionts (Pocillopora sp., Fox et al., 2021). This lack of internal regulation makes this genus more prone to a nutritional imbalance, leading to a shift of the symbiosis toward parasitism, exacerbating bleaching susceptibility (Baker et al., 2018; Burkepile et al., 2020). As such, Pocillopora is an interesting model organism to investigate the flexibility of coral nutrition to variable nutrient supply. This species is abundant on the D’ Entrecasteaux’s reefs, which host some of the world’s richest coral reefs in terms of endemism (Roberts, 2002), and are classified as Important Bird Area (Lorrain et al., 2017; Donald et al., 2019) and. Such remote seabird islands thus represent an ideal in situ coastal enrichment experiment to study the response of coral nutrition to naturally occurring spatiotemporal variations of the seabirds’ effect on coastal ecosystems.
Our study aimed at answering two questions. First, what is the spatial extent of seabird-derived supply of nutrients along an inshore-offshore transect and how does it vary seasonally and between two islets of various characteristics and seabird communities? Second, how does the trophic behavior of a mixotrophic coral, Pocillopora damicornis, respond to those variations in time and space. Hereafter, we present a combination of in situ data on seabird communities, coastal nutrient concentrations and isotope values that portray spatiotemporal fluctuations in the seabird-derived supply of nutrient at two different sites and seasons. We then discuss a potential effect on coral nutrition suggested by four complementary metrics applied to coral tissues and symbionts polygons in a bidimensional isotopic space.
Materials and Methods
Study Sites
Study sites are located on two coral atolls of the Coral Sea in the D’Entrecasteaux reefs located 223 km north of New Caledonia main island. The study focuses on islets located on the rim of the largest atolls of the area, Surprise and Huon (Figure 1). Both islets are made of coral debris deposits, and border 65 and 75 km reef atolls, respectively. Surprise Islet is a 24 ha round-shaped islet with a maximum elevation of 9 m. It is covered with various herbaceous plants, patches of woody vegetation and has a bare ground central plain (Caut et al., 2008). The 27 ha Huon Islet is an elongated and low islet covered with an herbaceous plant layer only. The two islets host several thousands of resident (year-long) seabird breeding pairs dominated by four genera, namely noddies (Anous sp.), bobbies (Sula sp.), frigatebirds (Fregata sp.), and the wedge-tailed shearwater Ardenna pacifica (Spaggiari et al., 2007). Reefs surrounding the two islets host benthic coral assemblages dominated by scleractinian species (Wantiez et al., 2013). As all islets in the area host seabird colonies, two reference sites were selected approximately 10 km away from each islet, away from any seabird derived nutrient supply (Figures 1B,D). Reference sites were selected on inner reef sections of similar benthic assemblages as compared to islet’s inner reefs: a substrate dominated by coral slabs, rocks and debris, that hosts massive, digitate and tabular corals as well as macroalgae (Wantiez et al., 2013). Reference samples were collected 50 m off the crest on the inner part of those reefs as in Lorrain et al. (2017).
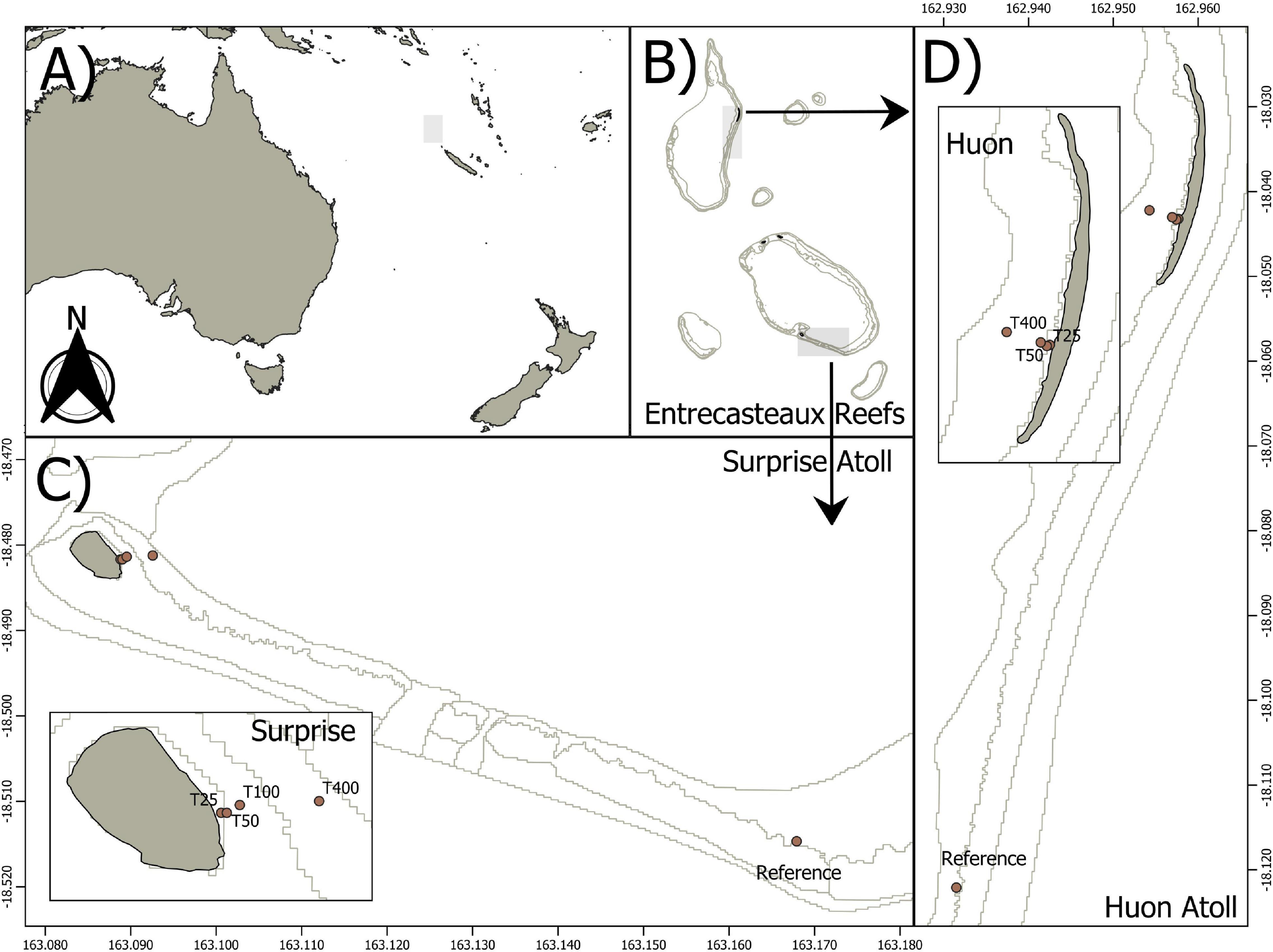
Figure 1. Study sites location. (A) Location of New Caledonia and the D’Entrecasteaux reefs in the South Pacific. (B) The two study atolls, Surprise and Huon, within the d’Entrecasteaux reefs. (C) Location of our transect and reference site within Surprise atoll. (D) Location of our transect and reference site within Huon atoll.
Sampling
Samples were collected in 2017 during the Austral rainy (March) and dry seasons (September) to account for a within-year seasonality in the coastal supply of seabird-derived nutrients. At each site and season, a transect was led perpendicular to the shore, across the reef flat. Sampling stations were defined at 25, 50, 100, and 400 m from the shore at the two sites. Samples were also collected at two reference sites, approx. 50 m away from the reef crest (Figure 1). Sampling was conducted once in each season, and along the same transect.
We measured NOx (nitrate + nitrite) concentrations in seawater to evaluate the spatial extent of the coastal enrichment in seabird-derived nutrients at the time of our sampling. Seawater was collected at the two seasons close to the coral sampled, along the two islets and at each of the four transect stations plus the reference site (N = 20). Concentration measures were duplicated or triplicated when possible.
We collected suspended particulate organic matter (POM), macroalgae fronds (Halimeda spp.), and coral samples at approximately 1.5–2 meters depth depending on the sites. POM includes photosynthetic and zooplanktonic organisms of very fast turnover rates that count in days (Torréton et al., 2002). It is therefore a good proxy of the daily availability in inorganic nitrogen. Nitrogen isotopic values of POM were analyzed from seawater samples collected in March, at the two sites and at all four transect stations plus the reference site (N = 10). Analyses were duplicated or triplicated when possible. Macroalgae samples were collected to provide an isotopic baseline of benthic primary producers. Macroalgae has fast (weekly) tissue turnover rates (Vroom et al., 2003), thus reflecting the weekly availability in inorganic nitrogen. Apical segments of Halimeda spp. fronds were collected with cutting pliers from 5 replicates per station in March, at the two sites, and at all four transect stations plus the reference site (N = 50). The September cruise only allowed for the collection of 1 macroalgae sample per site and transect station (N = 10).
We sampled the mixotrophic coral Pocillopora damicornis to get a longer time-integration of the seabird-derived nutrient supply and as a proxy of the availability in both organic and inorganic nitrogen forms. Estimated tissue turnover time in Porites lutea were 87 days for the coral tissue and 111 days for the algal symbiont (Rangel et al., 2019), and we hypothesized that these times are almost similar in P. damicornis. Apexes of five parental strains of the branching coral P. damicornis were removed with cutting pliers. On Huon’s reef, corals were found and collected beyond 25 m off the islet coast only (50, 100, and 400 m transect stations) as well as at the reference site, in both seasons (N = 41). Along Surprise, corals were only found and collected within 100 m off the islet (25, 50, and 100 m transect stations) as well as at the reference site, in both seasons (N = 40).
We estimated seabird abundances with binoculars and manual counters via exhaustive counts. Three passes were conducted on each islet by two observers that targeted two species simultaneously. For each species, the total count of individuals included all breeding pairs, chicks, as well as resting adults. Just-dried guano samples were collected on Surprise (N = 27) and Huon (N = 14) in September, under nests of the dominant breeding seabirds to provide a baseline value of the expected major source of terrestrial organic matter, and to evaluate whether guano bears a species-specific isotopic fingerprint. Samples were collected from six breeding species (five on Surprise, three on Huon), namely the red-footed booby Sula sula, the brown booby Sula leucogaster, the masked booby Sula dactylatra, the brown noddy Anous stolidus, the black noddy Anous minutus and frigate birds Fregata sp.
Nutrient Concentrations and Isotopic Analysis
Unfiltered surface seawater samples were fixed with HgCl2. NOx (NO2– + NO3–) concentrations were determined on a Bran-Luebbe III continuous flow autoanalyzer according to Raimbault et al. (1990). Coral tissues were extracted with an air-pick and homogenized with a Potter tissue grinder (Rodolfo-Metalpa et al., 2009). Zooxanthellae and coral tissue were separated by centrifugation for 10 min at 6,000 g and 4°C (Houlbrèque et al., 2003). Tissue fractions were concentrated by filtration over pre-combusted 25 mm GF/F filters (Whatman, nominal porosity = 0.7 μm), and seawater samples for POM were filtered similarly. All samples were frozen and dried at 60°C before encapsulation in tin cups. Macroalgae and guano samples were freeze-dried for 48 h. All isotopic analyses were conducted at Union College (Schenectady, NY, United States) on a Thermo Delta Advantage mass spectrometer in continuous flow connected to a Costech Elemental Analyzer via ConFlo IV. Reference standards [IAEA-N-2 ammonium sulfate (δ15N = 20.3 ± 0.2), IAEA-600 caffeine (δ13C = −27.771 ± 0.043, δ15N = 1.0 ± 0.2), IAEA-CH-6 sucrose (δ13C = −10.449‰), and an in-house acetanilide standard (δ13C = −34.07, δ15N = −0.96)] were used for isotopic corrections, and to assign the data to the appropriate isotopic scale with analytical precision better than 0.1‰.
Statistical Analyses
We explored spatial and temporal variations in δ15N values of coral tissues and symbionts, macroalgae, and suspended POM through multi-factor variance analyses using R version 3.6.1 (R Core Team, 2019). For each sample type, the δ15N value was considered the quantitative response variable. The position along the transect (including the reference site), the site and the season were considered categorical explanatory factors. Coral and macroalgae data were not normally distributed and were thus log transformed to avoid residual normality issues (Boldina and Beninger, 2016). Models were selected from full triple interaction models by step AIC procedures in the “MASS” R package v 7.3-51.5 (Venables and Ripley, 2002). Results of model selections as well as the influence of each factor combinations were compiled in an analysis of variance table. Residuals independence, normality, and variance homogeneity of all models were checked both graphically and with DurbinWatson, Shapiro-Wilk, and Bartlett tests, respectively, under “stats” v3.6.1 and “car” v3.0-3 packages (Fox and Weisberg, 2018; R Core Team, 2019). Lack of macroalgae replicates in September prevented us from using the Bartlett test homogeneity of variance, so we used a Levene test from the “car” package instead. Graphics were created with “ggplot2” v3.2.1 and “ggsignif” v0.6.0 packages (Wickham, 2016; Ahlmann-Eltze, 2019). Models’ post hoc pairwise comparisons between groups were made with Tukey tests and significant differences of interest are provided in the result section. The same procedure was used to explore temporal and spatial variations in NOx concentration, except that the model used was a Generalized Linear Model with Quasi Poisson error distribution and logit link function to meet residuals normality condition. Comparisons between δ15N values of guano from different species and sites were conducted through two-way Anovas coupled with TukeyHSD post hoc tests.
We quantified the trophic strategy of corals and symbionts by plotting δ13C and δ15N on isotope biplots and analyzing isotopic niches with the SIBER (Stable Isotope Bayesian Ellipses) framework in R (Jackson et al., 2011). The number of replicates at each transect position did not allow for the computation of Standard Ellipses at this resolution (Thibault et al., 2021). Thereby, we included all transect stations and the reference site to calculate ellipses for sites and seasons only. Yet, to account for potential transect-scale variations in the signature of the lower trophic levels, our ellipses included 95% of data variations rather than 40% recommended of standard ellipses (Hoeinghaus and Zeug, 2008). However, differences between the isotope niches of both corals and symbionts were explored by season, site, and transect stations through the calculation of the distances and angles between dataset centroids, and niche segregation was statistically evaluated by Hotelling T2 tests (Turner et al., 2010). A significant niche overlap is proposed to reflect the use of similar resources by the coral and the symbiont (Turner et al., 2010). Conversely, low niche overlap indicates a decoupling of resources. The former case is associated with autotroph-dominated behavior and the latter with dominant heterotrophy. It has recently been proposed that Standard Ellipse Areas (SEAs) of coral tissues and symbionts overlapping in less than 10% could reflect heterotrophic feeding strategies (Conti-Jerpe et al., 2020). Conversely, overlaps higher than 70% may reflect autotrophic corals. The percentage of non-overlapping area between the fitted ellipses was calculated as a proxy of isotopic niche overlap, independent from the size of both coral and symbiont niches (Jackson et al., 2011). Unfortunately, samples collected 25 m off Surprise were removed from the SIBER analyses because of insufficient δ13C values obtained from the analysis.
Results
Seabird Population and Guano Nitrogen Isotope Characterization
On the two islets, seabird’s species richness and abundance were higher in September compared to March (Supplementary Table 1). On Huon, we counted 3,608 individuals from three species in March vs. 4,582 individuals from eight species in September. On Surprise, six species were observed in March for a total of 5,782 individuals while 28,630 individuals from 12 species were counted in September. Measured δ15N values varied from 6.8 ± 1.3‰ (n = 6) for the brown noddy on Surprise to 9.2 ± 1.3‰ (n = 4) for the same species on Huon (Supplementary Table 2). Neither interspecies nor between sites differences in δ15N values were significant, so that an average baseline value around 7.9 ± 1.3‰ was used.
Persistent Seabird-Derived Nutrient Gradients
Through both NOx concentrations and δ15N values of POM, macroalgae and the two coral fractions, our results demonstrate that (1) the coastal gradient in seabird-derived nutrients exists all year round at our study sites and that (2) fluctuations in the intensity of the coastal enrichment matched with spatiotemporal changes in seabird communities.
To estimate the coastal release of guano-derived nutrients, we measured the NOx concentration in surface seawater. Nutrient concentrations are reported in Supplementary Table 3 and the model describing variations in NOx concentration relative to different transect locations, sites and seasons is presented in Supplementary Table 4. The model indicates that NOx concentrations varied significantly with (i) the transect position, (ii) the site, and (iii) the season. NOx concentrations were consistently (1) higher at the 25 m sites compared to the reference sites (p < 0.001), (2) higher at Huon than at Surprise (p < 0.001) and (3) higher in September than in March (p < 0.001) (Figure 2). At the two sites and seasons, NOx concentrations were maximal at the most coastal stations and gradually decreased when distance to the coast increased.
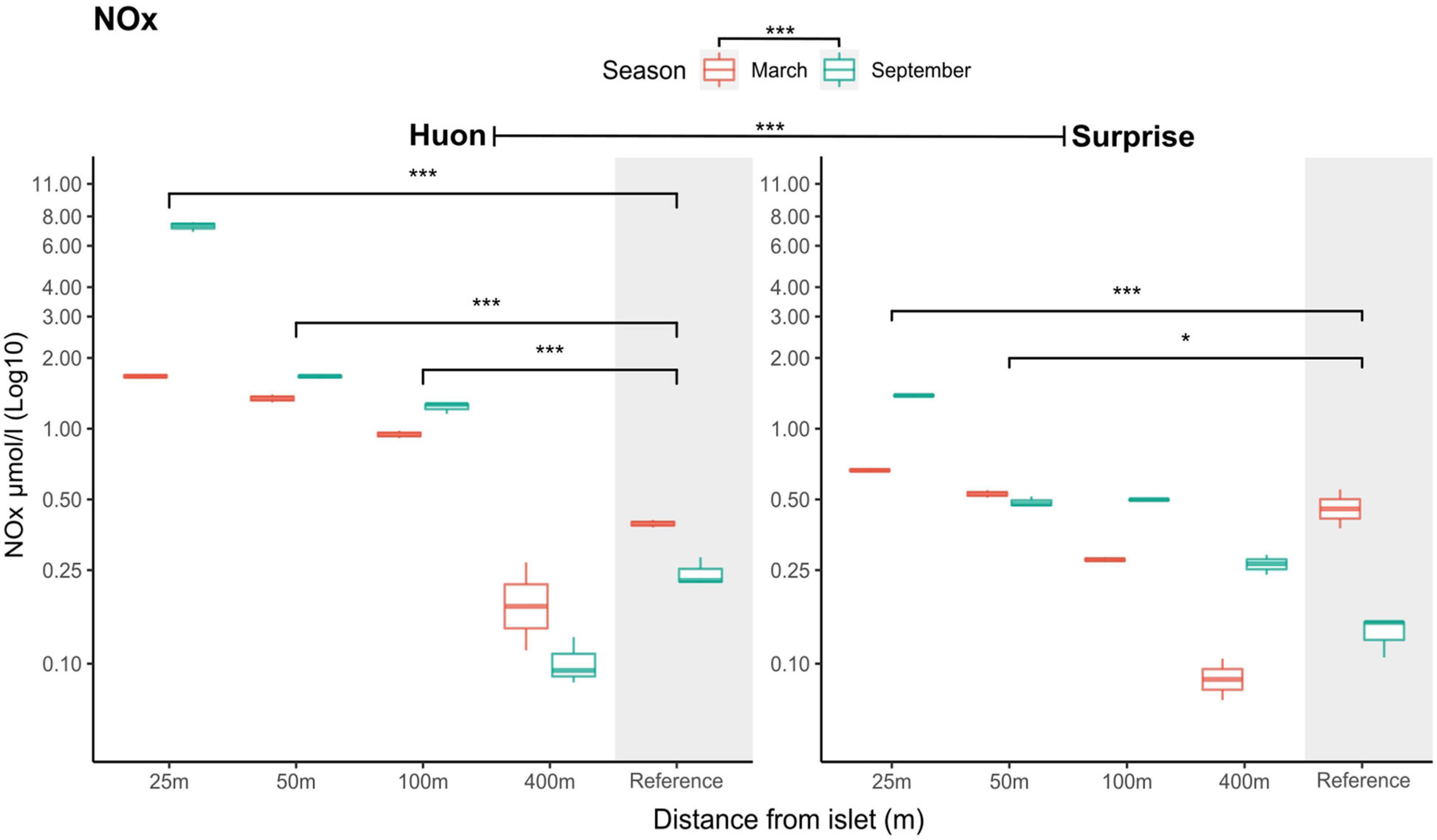
Figure 2. Spatial and temporal variations in the concentration of NOx in seawater. Asterisks show: (i) On top of the figure: a significant positive fixed effect of one season compared to the other (higher in Sept.), (ii) between site names: a significant fixed effect compared to the other site (higher at Huon), and (iii) between the reference site and the transect stations: significant differences in the transect per site effect (higher closer to shore). * Stands for p < 0.05, ** for p < 0.01, and *** for p < 0.001.
To understand the availability of seabird-derived nutrients for coastal organisms, we measured the δ15N values of POM, macroalgae, coral tissues and its symbionts (Figure 3 and Supplementary Table 5). Model testing for the influence of transect location, sites, and seasons as predictors of δ15N values provided an overview of this availability in space and time (Supplementary Table 4). Transect position, seasons and sites contributed significantly to variations in the δ15N values of all samples. The coastal gradient appeared the best predictor in all samples, as the δ15N values of POM, macroalgae, corals and symbionts decreased from inshore to offshore sites. The magnitude of the decrease then varied by site and season (Figure 3). On Surprise Islet, δ15N values were higher at 25 and 50 m compared to the reference sites in macroalgae (p < 0.001), coral tissues (p < 0.001), and corals symbionts (p < 0.001). In the same way, at Huon, stations at 50 and 100 m also showed significantly higher δ15N values in macroalgae (p < 0.001), coral tissues (p < 0.001) and coral symbionts (p < 0.001) compared to the reference site. δ15N values were significantly higher at Surprise compared to Huon in coral symbionts (p < 0.001) and tissues (p = 0.02), POM (p = 0.04), and in samples collected at 25 and 50 m in macroalgae (p < 0.001). Finally, δ15N values were higher in September in coral tissues (p < 0.001), coral symbionts (p < 0.001) and macroalgae (p < 0.001) compared to March at both sites. The only exception was the seasonality in the δ15N values of coral tissues at Huon, which was not significant (p = 0.4).
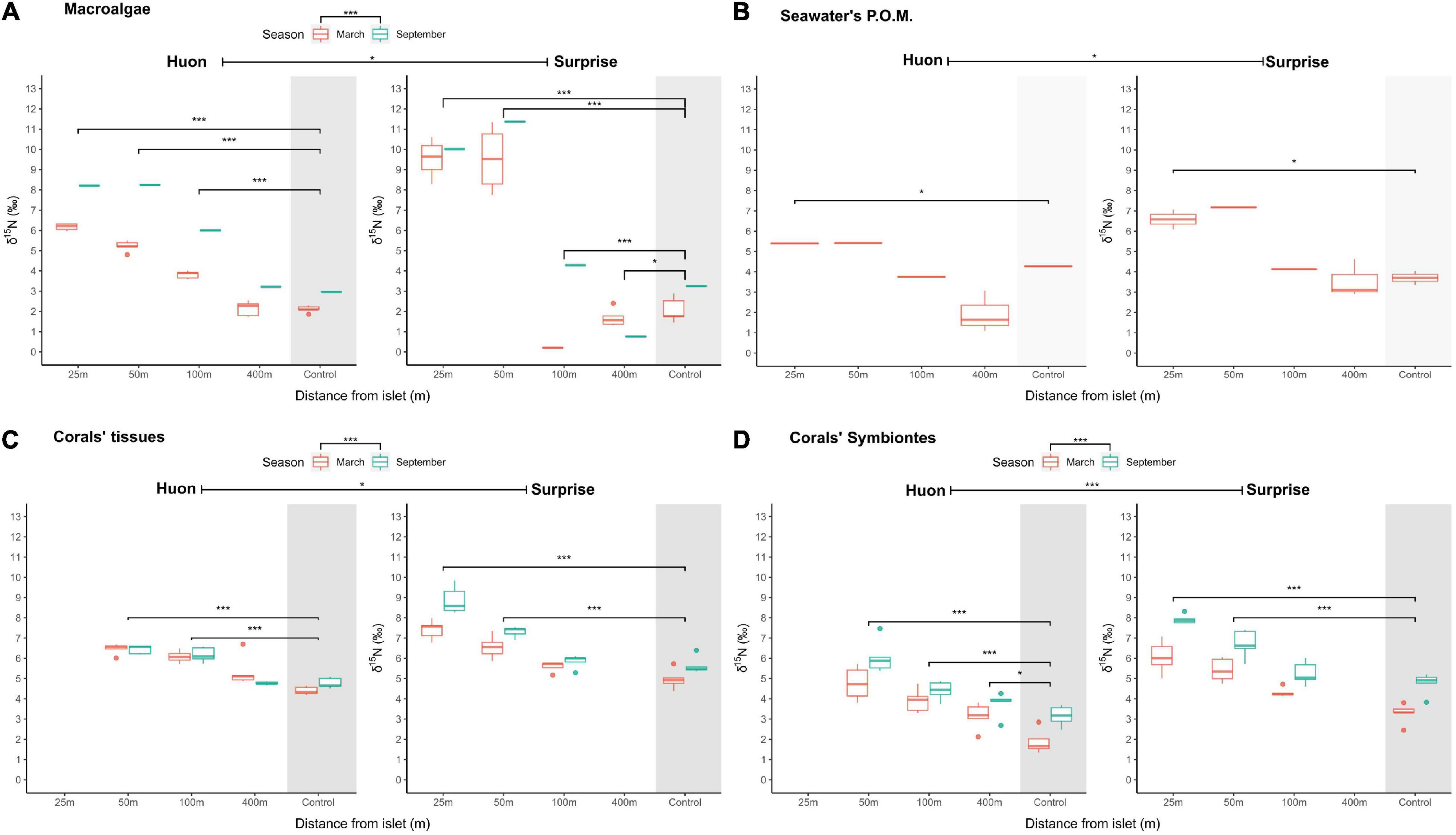
Figure 3. Spatial and temporal variations in δ15N values measured in (A) macroalgae, (B) suspended POM, (C) coral tissues and (D) coral symbionts. Asterisks are included: (i) in the legends to mark a significant positive fixed effect of one season compared to the other (higher in Sept.), (ii) next to the site names to mark a positive fixed effect compared to the other site (higher in Surprise), and (iii) between the reference site and the transect positions to mark significant differences in the transect:site effect (higher closer to shore). * Stands for p < 0.05 and *** for p < 0.001.
Nutritional Response of Pocillopora damicornis
We explored the trophic response of Pocillopora damicornis colonies to the above mentioned spatial and seasonal variations by adapting the SIBER framework to our data and hypotheses (Figure 4). This framework relies on the analysis of the isotopic niche segregation between the coral tissue and symbiont fractions. Niche overlap is considered a marker of high resource sharing between the fractions (autotrophy), suggests whereas niche segregation marks a decoupling of nutrition by the two fractions (heterotrophy). At first glance, isotopic niche segregation showed many intricate variations at all scales of our sampling design (Figure 4). Therefore, only the use of four complementary metrics allowed to get the best out of the SIBER framework in this context. To precisely describe this niche segregation, these metrics are all based on the distance between the centroids of the coral tissues and symbiont fractions in the isotopic space.
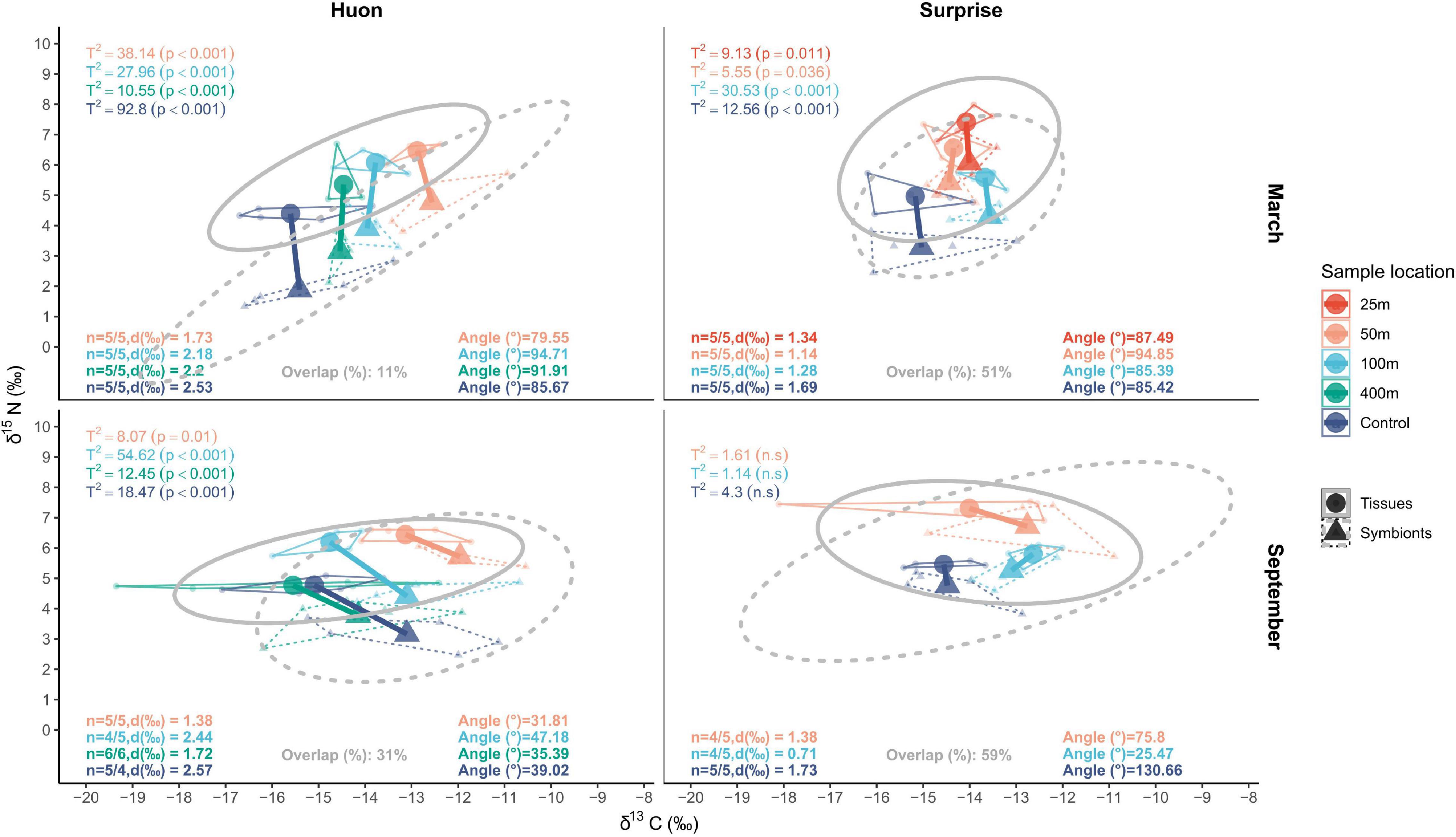
Figure 4. δ15N and δ13C biplots of corals host and symbiont at each site and season. Colors represent the different transect locations. Dotted and plain lines represent the values of the tissue and symbiont fractions, respectively. The 95% SIBER ellipses are represented as well as the corresponding overlap. The colored segments represent the distance between the centroid of the two fractions, calculated at transect location resolution. Provided distances, angles, and Hotelling test values correspond to these segments.
Results suggest an overall mixotrophy in all conditions, but with increased contribution of autotrophy: (1) at Surprise compared to Huon, (2) in September compared to March, and (3) closer to shore. First, ellipses overlap and distances between niche centroids highlighted the contribution of sites as a predictor of the coral nutritional state. At Huon, both lower ellipses overlap (Overlap: 11–31%) and higher distances between centroids (dist.: 1.3–2.57‰) revealed a higher contribution of heterotrophy compared to Surprise (Overlap: 51–61%; dist.: 0.6–1.69‰).
Second, Hotelling tests and angles of mean distance vectors revealed seasonal variations at the two sites. At Surprise, Hotelling tests were not significant in September which is a mark of autotrophy dominated behavior as opposed to March when significant tests argued for a nutritional balance dominated by heterotrophy. Angles around 45° [31.8–47.2°] suggested a higher contribution of δ13C values to the estimated heterotrophy along Huon in September, compared to angles of 90 ± 15° in March that reflected a unique contribution of δ15N values to niche segregations. However, comparing the two seasons at Huon along the δ15N axis also revealed lower distances between niche centroids, and thereby increased autotrophy, in September (Y-axis: 0.4–1.8‰) compared to March (Y-axis: 1.7–2.5‰).
Third, combination of the metrics also suggested that isotope niche segregations presented transect-scale variations. In the most heterotrophic setting, i.e., at Huon in March, a gradual shift toward more autotrophy appeared with proximity to the islet. This is what the gradually lowering distance between coral host and symbiont niche centroids (a proxy for increasing autotrophy) suggests at this site and season. Conversely, in the most autotrophic setting at Surprise in September, no clear pattern was observed as coral host and symbiont niches overlapped. At Huon in September, the distance between niche centroids gradually decreased with distance to the shore suggesting an increased autotrophy toward the islet coast. Only samples collected at 100 m, which are missing one host sample replicate, added noise to this nutritional shift pattern. The same results were found at Surprise in March, where this distance also increases gradually from 50 m to the reference site, but 25 m samples had a distance value closer to the reference. Yet, in both situations, Hotelling tests statistics (T2 < 10) and p- values (> 0.01) suggested a lower niche segregation, or a higher autotrophy, at inshore stations.
Discussion
The ability of shallow water corals to adjust their nutrition between autotrophy and heterotrophy plays a crucial role in their ability to cope with ongoing global warming (e.g., Grottoli et al., 2006; Conti-Jerpe et al., 2020; Thibault et al., 2021). Here, we used a naturally occurring nutrient gradient along two seabird islets of the SW Pacific to study the spatiotemporal variability of seabird derived-N supply and its influence on the nutritional response of the mixotrophic coral Pocillopora damicornis using stable nitrogen (and carbon) isotope ratios.
Seabirds Are an Extrinsic Source of Inorganic Nitrogen for Oligotrophic Reefs
The two reef flats display a similar nutrient concentration gradient along an inshore offshore transect. The section of the transect closest to the shore shows NOx concentration above 1 μM, an elevated value for a coral reef lying in oligotrophic water (Bell, 1992; Kleypas et al., 1999), whereas the oceanic section of the transects and the reference sites had concentrations within the range typically reported on tropical oligotrophic coral reefs (i.e., <1 μM; Bell, 1992; Kleypas et al., 1999). The transect stations closer to the shore (25, 50, and 100 m) present higher NOx concentrations than offshore and reference stations and are associated with high δ15N values of macroalgae and coral (from 6 to 11‰, Figures 2, 3). This result suggests that a concentrated source of 15N-enriched-N is present in the ecosystem. This trend has been previously described by Lorrain et al. (2017) and attributed to the assimilation of seabird-derived N by marine organisms in line with the high density of seabird nesting on these islets. Indeed, seabird guano is enriched in 15N because of the high seabird trophic position and preferential volatilization of 14N, so that seabird droppings have elevated δ15N values (Wainright et al., 1998). The δ15N of just-dried bird droppings measured in this study was relatively uniform across the six species of seabird sampled (Supplementary Table 2). The high ammonia content in seabird droppings makes guano prone to isotopic fractionation caused by the propensity of 14N ammonia to volatilize quickly, leaving behind 15N enriched ammonia (Mizutani et al., 1985; Walters et al., 2019). For comparison, older dried guano collected in 2015 at the same site had δ15N values of 11.5 ± 1.2‰ for the same seabird species (Lorrain et al., 2017), which confirms a 15N enrichment during the drying process (i.e., volatilization). Macroalgae are autotrophic organisms that assimilate the dissolved inorganic nitrogen available in their environment to perform photosynthesis. Therefore, the stable nitrogen isotope value of their tissues is used as a proxy to map nitrogen enrichments in coastal ecosystems (e.g., Costanzo et al., 2005; Geeraert et al., 2020). Here, δ15N values recorded in macroalgae along our inshore-offshore transects demonstrate that a local enrichment in seabird-derived inorganic nitrogen happens within 50 m off Surprise and 100 m off Huon. The high macroalgae δ15N values close to the shore (up to 11‰) also suggest that guano (7.9 ± 1.3‰) must have undergone fractionation before being supplied to the reef and that the source of NOx present on the transect unlikely derived directly from unaltered bird droppings.
On the oceanic section of the transects and at the reference sites, macroalgae recorded lower δ15N values (approx. 3‰, Figure 3) than those typically reported for subthermocline nitrate, which range from 5.5 to 7.0‰ in the western Pacific Ocean (Casciotti et al., 2008; Sigman et al., 2008; Rafter et al., 2012; Ren et al., 2015). This suggests that a 15N-depleted source is present at the oceanic ends of our study sites, which was expected as the SW Pacific is described as a hotspot for N2-fixation (Bonnet et al., 2018). There, surface cyanobacteria assimilate atmospheric N2 in their biomass and tend to lower the δ15N value (fixed N is typically around -2 to 0‰) of the nitrate pool through ammonification and nitrification (Wada and Hattori, 1976; Minagawa and Wada, 1986; Carpenter et al., 1997). Our data thus suggests that N2-fixation is a significant source of N in the surface waters of the Coral Sea.
At both sites, the gradient observed in NOx concentrations and in macroalgae and coral δ15N values is present during both Austral dry (September) and rainy (March) seasons. Previously in October 2015, a strong NOx concentration gradient was also reported at Surprise and Reynard Islets, two islets hosting important seabird colonies (Lorrain et al., 2017). This strongly suggests that seabirds supply inorganic nitrogen to reef flats all year-round along densely populated islands.
The Seabird-Derived Nutrient Supply Varies With Sites and Seasons
Macroalgae and both coral tissue and symbiont fractions recorded higher δ15N values at Surprise compared to Huon. POM δ15N values presented the same patterns as macroalgae along the transect and among sites, thus reflecting variations in inorganic δ15N sources. Higher NOx concentrations were found at Huon, but NOx can be highly variable in seawater (e.g., Torréton et al., 2010) and can even reach up to 20 μM at Surprise (Lorrain et al., 2017). Conversely, δ15N values of POM, macroalgae, and corals that reflect changes in the N-sources at the scale of the week (or several weeks) suggest that those higher values at Surprise are real and driven by local processes. As no significant differences were found in the δ15N values of guano samples by species or sites, it is unlikely that site-specific seabird communities drive this difference. Thus, the site effect on δ15N values in reef organisms is certainly the result of site-specific alterations of seabird-derived nutrients along the land-sea continuum as suggested by the 15N enrichment between seabird guano and macroalgae tissues samples. While the biochemical transformations undergone by nitrogen along the various land-sea pathways were beyond the scope of this study, two differences between our sites were striking and deserved mention. The first one was the much larger number of seabirds inhabiting Surprise compared to Huon (Supplementary Table 1). The second was the vegetation cover that is limited to a few herbaceous species on Huon while Surprise is covered with dense shrubs and trees. The combination of increased guano deposition and fractionation of nitrogen by plant roots (Schmidt et al., 2005; Hobbie and Ouimette, 2009) may lead to the release of 15N enriched nutrient along Surprise compared to Huon.
At our two sites, seasonal fluctuations were also observed in both the NOx and the isotopic gradients. Both gradients become steeper during the dry season (September), when sea surface temperature (SST) and rainfall are low (Supplementary Figure 1). On the contrary, during the Austral wet season (March), these gradients appear smoother when conditions are wetter and warmer. Further, seasonality in this 15N enrichment is mostly observable in macroalgae and coral symbiont samples (and not in coral tissues), suggesting that this 15N enrichment occurred in the inorganic forms of nitrogen. Once again, while we lack macroalgae replicates in September, the similarity between seasonal variations in macroalgae and coral symbionts δ15N values gives weight to this statement. Seasonal variations in NOX samples were also recorded at the reference sites. As these fluctuations were equivalent to the standard deviation of replicate measurements (s.d. up to 0.4 μM), we believe that they could be the result of sampling stochasticity only. However, variations in the isotope values recorded at the two reference sites (up to 1.5‰) argue for a seasonal change in the dominant N source given the typical analytical precision of 0.1 ‰. An increase in primary production during the dry season was recorded at our study sites in 2017 (Supplementary Figure 2) which might have allowed for increased N assimilation and fractionation by both coral symbionts and phytoplankton. Still, the persistent coastal 15N enrichment at both seasons argues for a simultaneous increase in the supply of terrigenous nitrogen along the transects. Increased precipitation and runoff leading to rapid export of N to the reef (Tait et al., 2014; Xu et al., 2019) cannot explain such seasonality as the highest δ15N increase in coastal organisms has been measured during the austral dry season (September), when rainfall and sea surface temperatures were the lowest (Supplementary Figure 1). A reasonable hypothesis for the seasonality in the seabird-derived N could thus involve the brackish groundwater lens found on most coral islets (Comte et al., 2010, 2014). Brackish groundwater lenses are typically located a few meters under the surface of the islet and develop when rainwater infiltrates the porous calcium carbonate framework of the islet and remains above the saltwater intrusion due to its lower density. It is probable that the seabird-derived N transits through this water mass before reaching the reef (Santos et al., 2012). If this holds true, then rainfall and seabird-N supply fluctuation may become decoupled, reducing any direct connection between rainfall and N-supply (Michael et al., 2005; Taniguchi et al., 2019).
Seabird-Derived Nutrient Supplies Modulate Pocillopora damicornis Nutrition
Seasonal and site-specific dynamics as well as the steep NOx gradients found adjacent to the seabird nesting islets all modulate the nutrient supply available to the corals located on the surrounding reef flats. We used the analytic framework on Stable Isotope Bayesian Ellipses in R (SIBER, Jackson et al., 2011) to determine the trophic response of P. damicornis to this fluctuating nutrient supply at various scales (Conti-Jerpe et al., 2020). The analysis of the 95% ellipses (Thibault et al., 2021), at the two locations and during the two seasons, revealed a general mixotrophic nutrition strategy with a relatively high overlap between the coral and the symbiont ellipses: 11–61% (Figure 4). This finding is in agreement with previous works based on bulk and essential amino-acid (AAESS) δ13C values of the species P. meandrina (Fox et al., 2018, 2019) in the central Pacific, indicating that mixotrophy could be a shared feature across the species of the genus Pocillopora (Lyndby et al., 2020). We also looked at three other metrics associated with the mean distance vectors between the centroids of the coral and symbiont components. Combination of these metrics suggests that coral nutritional state varies together with fluctuations in the availability of seabird-derived nutrients on coastal waters. Indeed, while POM, macroalgae and coral tissues recorded a higher 15N enrichment at Surprise compared to Huon, P. damicornis appeared consistently more autotroph at Surprise. While NOx concentrations and macroalgae δ15N value were higher in Austral dry season compared to the wet season, corals were more autotrophic during the Austral dry season. Finally, inshore samples were more autotroph than those collected offshore. This fine scale modulation was intricate with the seasonal and site effects, but a gradual shift toward more autotrophy at coastal -“N-enriched”- stations was visible in the most heterotrophic settings of our study design.
Therefore, our results portray three contrasted scenarios experienced by seabird island corals. First, at Huon and during the Austral wet season, the release of seabird-derived nutrients onto the reef is “minimum.” Coral’s nutrition is dominated by heterotrophy as oceanic oligotrophic water is dominant on the reef. This situation brings to light a fine scale effect of seabird-derived nutrient supply allowing for increased autotrophic nutrition in corals lying close to the seabird-derived nutrient supply (50–100 m). Second, in the Austral dry season at Surprise, the coastal supply of seabird-derived nutrients is “maximal.” Seabird-derived inorganic nitrogen becomes dominant on the reef, so that coral’s nutrition is balanced toward dominant autotrophy. This marks a site-dependence in the nutritional state of P. damicornis which was recently shown in another Pocillopora species (P. acuta, Price et al., 2021). Third, corals collected at Surprise in the wet season and Huon in the dry season present mixotroph nutritional states that vary with the proximity to the nutrient supply. Vertical mean distance vectors in Austral wet season on Surprise’s reef showed that the shift toward more heterotrophy was driven by the seasonal shift in the nitrogen source.
However, isotopic niches of corals fractions collected at Huon during the dry season showed increased symbiont δ13C values (Supplementary Table 6), which is another marker of increased photosynthetic activity (Muscatine et al., 1989; Fox et al., 2018). Experimental studies reported that elevated temperature (>30°C) may slow nutrient uptake in Pocilloporid corals (Godinot et al., 2011; Ezzat et al., 2016; Burkepile et al., 2020; Foo and Asner, 2020), which could have happened at our sites during the austral hot and wet season. However, mean sea surface temperature reached a maximum of 29°C during the wet season of 2017 in our study area according to satellite sensing. It is thus very likely that the greater supply of seabird-derived NOx to the coastal reef during the Austral dry season (September) dominantly drives the seasonality in coral nutrition at our two sites.
The modulation of the coral trophic strategy as a response to seabird-derived nutrient supply is best explained by the increased supply of seabird-derived nutrients. It suggests that P. damicornis increases its reliance on dissolved inorganic N-sources (mostly NOx) when this resource dominates in the ecosystem (Grover et al., 2003). In Fox et al. (2021) P. acuta colonies were cultivated under increasing nitrate concentration and symbionts continuously assimilated nitrate independently of the coral, increasing their density. If nitrate supplementation alone has been shown to be mostly detrimental to corals, Ezzat et al. (2015) demonstrated that a combined enrichment of phosphate and ammonium at an appropriate stoichiometry (i.e., close to that of the coral tissues) was beneficial to the symbiosis. Although we did not measure phosphate or ammonium concentrations in this study, seabird guano is known to be rich in those two elements that are commonly found elevated along densely populated seabird islands (Lindeboom, 1984; Honig and Mahoney, 2016; Otero et al., 2018; Savage, 2019). We thus suggest that the coastal seabird-derived nutrient supply favors an increase in P. damicornis symbiont density and chlorophyll synthesis, enhancing the photosynthesis (Ezzat et al., 2016) and thereby their autotrophic nutrition.
Trophic plasticity is considered a major predictor of resilience to climate change in mixotrophic corals (Grottoli et al., 2006). Indeed, some species can cope with increased turbidity and reduced light availability by supplementing their nutrient assimilation with heterotrophic feeding (Anthony and Fabricius, 2000). In the face of increased nearshore/oceanic primary production, this plasticity increases the resistance potential of corals (Fox et al., 2018). Does this plasticity reflect an acclimation potential or an adaptation to environmental fluctuations? The question still holds, but our results suggest that the natural supply of seabird-derived inorganic nutrients along the land-sea continuum is both persistent and variable. We show that the nutritional regime of the species is modulated by these natural fluctuations. Historical fluctuations in island seabird colonies may have participated in providing a fluctuating environment to coastal corals in terms of nutrient availability. We believe that the relative plasticity of P. damicornis with respect to ongoing environmental changes (Hoadley et al., 2015) could partly originate from fluctuations along the land-sea continuum. Our results highlight two research fronts that would improve our understanding of coral island ecosystems and their sensitivity to ongoing changes. The first concerns the origin of the nutrient supply and the intricate mechanisms shaping the transport and alteration of seabird-derived nutrients along the land-sea continuum. The second relates to its ultimate effects, and the benefit to corals’ physiology from such fluctuating drivers of nutrition on their resistance/resilience to climate change.
Our study provides a comprehensive characterization of a natural supply of coastal nutrients along the shores of remote islets hosting massive seabird colonies. The in situ sampling design, including two seasons, shows that this seabird nutrient supply is persistent yet variable over time. Samples collected along coast-ocean transects demonstrate its major contribution to the local nutrient pool by providing limiting nutrients in an oligotrophic context. Replicated measurements of NOx concentrations map the small spatial extent of this nutrient supply, while nitrogen stable isotopes mark its assimilation by reef organisms of variable nutritional requirements. Finally, the trophic niches occupied by corals and symbionts in stable isotope biplots shows that this supply modulates the nutritional regime of a mixotrophic coral at a fine scale.
Data Availability Statement
All data associated with this manuscript can be found deposited in DataVerse Repository 10.23708/INWSD6.
Author Contributions
MT, FH, ND, NC, and AL conceived the ideas and designed methodology. FH, VM, FB, and AR collected the data. DG led the laboratory analyses. MT analyzed the data and led the writing of the manuscript. All authors contributed critically to the drafts and gave final approval for publication.
Funding
Financial support was provided by the LabEx CORAIL/MER and INSU LEFE/EC2CO, through the IGUANE and GUANACO projects, respectively. NN Duprey benefitted from the Paul Crutzen Post-doctoral fellowship and M THIBAULT from the IRD Post-doctoral fellowship. The U.S. National Science Foundation funded Union College’s isotope ratio mass spectrometer and peripherals (NSF-MRI #1229258).
Conflict of Interest
The authors declare that the research was conducted in the absence of any commercial or financial relationships that could be construed as a potential conflict of interest.
Publisher’s Note
All claims expressed in this article are solely those of the authors and do not necessarily represent those of their affiliated organizations, or those of the publisher, the editors and the reviewers. Any product that may be evaluated in this article, or claim that may be made by its manufacturer, is not guaranteed or endorsed by the publisher.
Acknowledgments
Samples were collected during the cruises POSTBLANCO (sampling authorization N 2017-409 and 2017-575/GNC from the Government of New Caledonia) and TARA ENTRECASTEAUX (sampling authorization N 2017-2067/GNC from the Government of New Caledonia and the Parc Naturel de la Mer de Corail) on board of the RV Alis (IRD) and TARA. We thank the crews of the N/O Alis and Tara, the Government of New Caledonia and the Parc Naturel de la Mer de Corail for permission to access to the reef. We thank the LAMA laboratory, Philippe Gérard and the US IMAGO for nutrient analyses, and Anouk Verheyden and Madelyn Miller from the Union College Stable Isotope Laboratory for preparing and analyzing the stable isotope samples.
Supplementary Material
The Supplementary Material for this article can be found online at: https://www.frontiersin.org/articles/10.3389/fmars.2021.790408/full#supplementary-material
References
Anthony, K. R. N., and Fabricius, K. E. (2000). Shifting roles of heterotrophy and autotrophy in coral energetics under varying turbidity. J. Exp. Mar. Biol. Ecol. 252, 221–253. doi: 10.1016/S0022-0981(00)00237-9
Baker, D. M., Freeman, C. J., Wong, J. C. Y., Fogel, M. L., and Knowlton, N. (2018). Climate change promotes parasitism in a coral symbiosis. ISME J. 12, 921–930. doi: 10.1038/s41396-018-0046-8
Bell, P. R. F. (1992). Eutrophication and coral reefs—some examples in the Great Barrier Reef lagoon. Water Res. 26, 553–568. doi: 10.1016/0043-1354(92)90228-V
Benkwitt, C. E., Wilson, S. K., and Graham, N. A. J. (2019). Seabird nutrient subsidies alter patterns of algal abundance and fish biomass on coral reefs following a bleaching event. Glob. Change Biol. 25, 2619–2632. doi: 10.1111/gcb.14643
Boldina, I., and Beninger, P. G. (2016). Strengthening statistical usage in marine ecology: linear regression. J. Exp. Mar. Biol. Ecol. 474, 81–91. doi: 10.1016/j.jembe.2015.09.010
Bonnet, S., Caffin, M., Berthelot, H., Grosso, O., Benavides, M., Helias-Nunige, S., et al. (2018). In depth characterization of diazotroph activity across the Western Tropical South Pacific hot spot of N&It;sub>2&It;/sub> fixation. Biogeosci. Discuss. 15, 4215–4232. doi: 10.5194/bg-2017-567
Burkepile, D. E., Shantz, A. A., Adam, T. C., Munsterman, K. S., Speare, K. E., Ladd, M. C., et al. (2020). Nitrogen identity drives differential impacts of nutrients on coral bleaching and mortality. Ecosystems 23, 798–811. doi: 10.1007/s10021-019-00433-2
Carpenter, E. J., Harvey, H. R., Fry, B., and Capone, D. G. (1997). Biogeochemical tracers of the marine cyanobacterium Trichodesmium. Deep Sea Res. Part Oceanogr. Res. Pap. 44, 27–38. doi: 10.1016/S0967-0637(96)00091-X
Casciotti, K. L., Trull, T. W., Glover, D. M., and Davies, D. (2008). Constraints on nitrogen cycling at the subtropical North Pacific Station ALOHA from isotopic measurements of nitrate and particulate nitrogen. Deep Sea Res. Part II Top. Stud. Oceanogr. 55, 1661–1672. doi: 10.1016/j.dsr2.2008.04.017
Caut, S., Angulo, E., and Courchamp, F. (2008). Dietary shift of an invasive predator: rats, seabirds and sea turtles. J. Appl. Ecol. 45, 428–437. doi: 10.1111/j.1365-2664.2007.01438.x
Comte, J.-C., Banton, O., Join, J.-L., and Cabioch, G. (2010). Evaluation of effective groundwater recharge of freshwater lens in small islands by the combined modeling of geoelectrical data and water heads: recharge of freshwater lens in small islands. Water Resour. Res. 46, 1–10. doi: 10.1029/2009WR008058
Comte, J.-C., Join, J.-L., Banton, O., and Nicolini, E. (2014). Modelling the response of fresh groundwater to climate and vegetation changes in coral islands. Hydrogeol. J. 22, 1905–1920. doi: 10.1007/s10040-014-1160-y
Conti-Jerpe, I. E., Thompson, P. D., Wong, C. W. M., Oliveira, N. L., Duprey, N. N., Moynihan, M. A., et al. (2020). Trophic strategy and bleaching resistance in reef-building corals. Sci. Adv. 6:eaaz5443. doi: 10.1126/sciadv.aaz5443
Costanzo, S. D., Udy, J., Longstaff, B., and Jones, A. (2005). Using nitrogen stable isotope ratios (δ15N) of macroalgae to determine the effectiveness of sewage upgrades: changes in the extent of sewage plumes over four years in Moreton Bay, Australia. Mar. Pollut. Bull. 51, 212–217. doi: 10.1016/j.marpolbul.2004.10.018
Donald, P. F., Fishpool, L. D. C., Ajagbe, A., Bennun, L. A., Bunting, G., Burfield, I. J., et al. (2019). Important bird and biodiversity areas (IBAs): the development and characteristics of a global inventory of key sites for biodiversity. Bird Conserv. Int. 29, 177–198.
Ezzat, L., Maguer, J.-F., Grover, R., and Ferrier-Pagès, C. (2015). New insights into carbon acquisition and exchanges within the coral–dinoflagellate symbiosis under NH 4 + and NO 3 - supply. Proc. R. Soc. Lond. B Biol. Sci. 282:20150610. doi: 10.1098/rspb.2015.0610
Ezzat, L., Towle, E., Irisson, J.-O., Langdon, C., and Ferrier-Pagès, C. (2016). The relationship between heterotrophic feeding and inorganic nutrient availability in the scleractinian coral T. reniformis under a short-term temperature increase. Limnol. Oceanogr. 61, 89–102. doi: 10.1002/lno.10200
Ferrier-Pagès, C., Peirano, A., Abbate, M., Cocito, S., Negri, A., Rottier, C., et al. (2011). Summer autotrophy and winter heterotrophy in the temperate symbiotic coral Cladocora caespitosa. Limnol. Oceanogr. 56, 1429–1438. doi: 10.4319/lo.2011.56.4.1429
Foo, S. A., and Asner, G. P. (2020). Sea surface temperature in coral reef restoration outcomes. Environ. Res. Lett. 15:074045. doi: 10.1088/1748-9326/ab7dfa
Fox, J., and Weisberg, S. (2018). An R Companion to Applied Regression, 3rd Edn. San Diego, CA: SAGE Publications, Inc.
Fox, M. D., Elliott Smith, E. A., Smith, J. E., and Newsome, S. D. (2019). Trophic plasticity in a common reef-building coral: Insights from δ 13 C analysis of essential amino acids. Funct. Ecol. 33, 2203–2214. doi: 10.1111/1365-2435.13441
Fox, M. D., Nelson, C. E., Oliver, T. A., Quinlan, Z. A., Remple, K., Glanz, J., et al. (2021). Differential resistance and acclimation of two coral species to chronic nutrient enrichment reflect life-history traits. Funct. Ecol. 35, 1081–1093. doi: 10.1111/1365-2435.13780
Fox, M. D., Williams, G. J., Johnson, M. D., Radice, V. Z., Zgliczynski, B. J., Kelly, E. L. A., et al. (2018). Gradients in primary production predict trophic strategies of mixotrophic corals across spatial scales. Curr. Biol. 28, 3355–3363.e4. doi: 10.1016/j.cub.2018.08.057
Geeraert, N., Duprey, N. N., McIlroy, S. E., Thompson, P. D., Goldstein, B. R., LaRoche, C., et al. (2020). The anthropogenic nitrogen footprint of a tropical lagoon: spatial variability in Padina sp. δ15N values1. Pac. Sci. 74:19. doi: 10.2984/74.1.2
Godinot, C., Houlbrèque, F., Grover, R., and Ferrier-Pagès, C. (2011). Coral uptake of inorganic phosphorus and nitrogen negatively affected by simultaneous changes in temperature and pH. PLoS One 6:e25024. doi: 10.1371/journal.pone.0025024
Graham, N. A. J., Wilson, S. K., Carr, P., Hoey, A. S., and Jennings, S. (2018). Seabirds enhance coral reef productivity and functioning in the absence of invasive rats. Nature 559, 250–253.
Grottoli, A. G., Rodrigues, L. J., and Palardy, J. E. (2006). Heterotrophic plasticity and resilience in bleached corals. Nature 440, 1186–1189. doi: 10.1038/nature04565
Grover, R., Maguer, J.-F., Allemand, D., and Ferrier-Pagés, C. (2003). Nitrate uptake in the scleractinian coral Stylophora pistillata. Limnol. Oceanogr. 48, 2266–2274. doi: 10.4319/lo.2003.48.6.2266
Hoadley, K. D., Pettay, D. T., Grottoli, A. G., Cai, W.-J., Melman, T. F., Schoepf, V., et al. (2015). Physiological response to elevated temperature and pCO2 varies across four Pacific coral species: understanding the unique host+symbiont response. Sci. Rep. 5:18371. doi: 10.1038/srep18371
Hobbie, E. A., and Ouimette, A. P. (2009). Controls of nitrogen isotope patterns in soil profiles. Biogeochemistry 95, 355–371. doi: 10.1007/s10533-009-9328-6
Hoeinghaus, D. J., and Zeug, S. C. (2008). Can stable isotope ratios provide for community-wide measures of trophic structure? Ecology 89, 2353–2357. doi: 10.1890/07-1143.1
Honig, S. E., and Mahoney, B. (2016). Evidence of seabird guano enrichment on a coral reef in Oahu, Hawaii. Mar. Biol. 163:22. doi: 10.1007/s00227-015-2808-4
Houlbrèque, F., and Ferrier-Pagès, C. (2009). Heterotrophy in tropical Scleractinian Corals. Biol. Rev. 84, 1–17. doi: 10.1111/j.1469-185X.2008.00058.x
Houlbrèque, F., Tambutté, E., and Ferrier-Pagès, C. (2003). Effect of zooplankton availability on the rates of photosynthesis, and tissue and skeletal growth in the scleractinian coral Stylophora pistillata. J. Exp. Mar. Biol. Ecol. 296, 145–166. doi: 10.1016/S0022-0981(03)00259-4
Jackson, A. L., Inger, R., Parnell, A. C., and Bearhop, S. (2011). Comparing isotopic niche widths among and within communities: SIBER – Stable isotope bayesian ellipses in R. J. Anim. Ecol. 80, 595–602. doi: 10.1111/j.1365-2656.2011.01806.x
Kleypas, J. A., Mcmanus, J. W., and Meñez, L. A. B. (1999). Environmental Limits to coral reef development: where do we draw the line? Am. Zool. 39, 146–159. doi: 10.1093/icb/39.1.146
LaJeunesse, T. C., Parkinson, J. E., Gabrielson, P. W., Jeong, H. J., Reimer, J. D., Voolstra, C. R., et al. (2018). Systematic revision of symbiodiniaceae highlights the antiquity and diversity of coral endosymbionts. Curr. Biol. 28, 2570–2580.e6. doi: 10.1016/j.cub.2018.07.008
Lindeboom, H. J. (1984). The nitrogen pathway in a penguin rookery. Ecology 65, 269–277. doi: 10.2307/1939479
Lorrain, A., Houlbrèque, F., Benzoni, F., Barjon, L., Tremblay-Boyer, L., Menkes, C., et al. (2017). Seabirds supply nitrogen to reef-building corals on remote Pacific islets. Sci. Rep. 7:3721. doi: 10.1038/s41598-017-03781-y
Lyndby, N., Holm, J., Wangpraseurt, D., Grover, R., Rottier, C., Kühl, M., et al. (2020). Effect of temperature and feeding on carbon budgets and O2 dynamics in Pocillopora damicornis. Mar. Ecol. Prog. Ser. 652, 49–62. doi: 10.3354/meps13474
Michael, H. A., Mulligan, A. E., and Harvey, C. F. (2005). Seasonal oscillations in water exchange between aquifers and the coastal ocean. Nature 436, 1145–1148. doi: 10.1038/nature03935
Minagawa, M., and Wada, E. (1986). Nitrogen isotope ratios of red tide organisms in the East China Sea: a characterization of biological nitrogen fixation. Mar. Chem. 19, 245–259. doi: 10.1016/0304-4203(86)90026-5
Mizutani, H., Kabaya, Y., and Wada, E. (1985). Ammonia volatilization and high 15N/14N ratio in a penguin rookery in Antarctica. Geochem. J. 19, 323–327.
Muscatine, L., and Porter, J. W. (1977). Reef corals: mutualistic symbioses adapted to nutrient-poor environments. BioScience 27, 454–460. doi: 10.2307/1297526
Muscatine, L., McCloskey, L. R., and Marian, R. E. (1981). Estimating the daily contribution of carbon from zooxanthellae to coral animal respiration1. Limnol. Oceanogr. 26, 601–611. doi: 10.4319/lo.1981.26.4.0601
Muscatine, L., Porter, J. W., and Kaplan, I. R. (1989). Resource partitioning by reef corals as determined from stable isotope composition. Mar. Biol. 100, 185–193. doi: 10.1007/BF00391957
Otero, X. L., De La Peña-Lastra, S., Pérez-Alberti, A., Ferreira, T. O., and Huerta-Diaz, M. A. (2018). Seabird colonies as important global drivers in the nitrogen and phosphorus cycles. Nat. Commun. 9:246. doi: 10.1038/s41467-017-02446-8
Palardy, J., Grottoli, A., and Matthews, K. (2005). Effects of upwelling, depth, morphology and polyp size on feeding in three species of Panamanian corals. Mar. Ecol. Prog. Ser. 300, 79–89. doi: 10.3354/meps300079
Price, J. T., McLachlan, R. H., Jury, C. P., Toonen, R. J., and Grottoli, A. G. (2021). Isotopic approaches to estimating the contribution of heterotrophic sources to Hawaiian corals. Limnol. Oceanogr. 66, 2393–2407. doi: 10.1002/lno.11760
R Core Team (2019). R: A Language and Environment for Statistical Computing. Vienna: R Foundation for statistival computing.
Rafter, P. A., Sigman, D. M., Charles, C. D., Kaiser, J., and Haug, G. H. (2012). Subsurface tropical Pacific nitrogen isotopic composition of nitrate: Biogeochemical signals and their transport: tropical Pacific nitrate isotopes. Glob. Biogeochem. Cycles 26:GB1003. doi: 10.1029/2010GB003979
Raimbault, P., Slawyk, G., Coste, B., and Fry, J. (1990). Feasibility of using an automated colorimetric procedure for the determination of seawater nitrate in the 0 to 100 nM range: examples from field and culture. Mar. Biol. 104, 347–351. doi: 10.1007/BF01313277
Rangel, M. S., Erler, D., Tagliafico, A., Cowden, K., Scheffers, S., and Christidis, L. (2019). Quantifying the transfer of prey δ15N signatures into coral holobiont nitrogen pools. Mar. Ecol. Prog. Ser. 610, 33–49. doi: 10.3354/meps12847
Ren, H., Studer, A. S., Serno, S., Sigman, D. M., Winckler, G., Anderson, R. F., et al. (2015). Glacial-to-interglacial changes in nitrate supply and consumption in the subarctic North Pacific from microfossil-bound N isotopes at two trophic levels. Paleoceanography 30, 1217–1232. doi: 10.1002/2014PA002765
Roberts, C. M. (2002). Marine biodiversity hotspots and conservation priorities for tropical reefs. Science 295, 1280–1284. doi: 10.1126/science.1067728
Rodolfo-Metalpa, R., Martin, S., Ferrier-Pagès, C., and Gattuso, J.-P. (2009). Response of the temperate coral Cladocora caespitosa to mid-and long-term exposure to pCO 2 and temperature levels projected for the year 2100 AD. Biogeosciences 7, 289–300. doi: 10.5194/bgd-6-7103-2009
Santos, I. R., Eyre, B. D., and Glud, R. N. (2012). Influence of porewater advection on denitrification in carbonate sands: evidence from repacked sediment column experiments. Geochim. Cosmochim. Acta 96, 247–258. doi: 10.1016/j.gca.2012.08.018
Savage, C. (2019). Seabird nutrients are assimilated by corals and enhance coral growth rates. Sci. Rep. 9:4284. doi: 10.1038/s41598-019-41030-6
Schmidt, O., Quilter, J. M., Bahar, B., Moloney, A. P., Scrimgeour, C. M., Begley, I. S., et al. (2005). Inferring the origin and dietary history of beef from C, N and S stable isotope ratio analysis. Food Chem. 91, 545–549. doi: 10.1016/j.foodchem.2004.08.036
Shatova, O. A., Wing, S. R., Hoffmann, L. J., Wing, L. C., and Gault-Ringold, M. (2017). Phytoplankton community structure is influenced by seabird guano enrichment in the Southern Ocean. Estuar. Coast. Shelf Sci. 191, 125–135. doi: 10.1016/j.ecss.2017.04.021
Sigman, D. M., Karsh, K. L., and Casciotti, K. L. (2008). “Ocean process tracers: nitrogen isotopes in the ocean,” in Encyclopedia of Ocean Science, 2nd Edn, eds J. H. Steele, K. K. Turekian, and S. A. Thorpe (Amsterdam: Elsevier), 17.
Spaggiari, J., Barré, N., Baudat-Franceschi, J., and Borsa, P. (2007). “New Caledonian seabirds,” in Compendium of Marine Species from New Caledonia, 2nd Edn, eds C. Payri and B. Richer de Forges (Nouméa: IRD).
Tait, D. R., Erler, D. V., Santos, I. R., Cyronak, T. J., Morgenstern, U., and Eyre, B. D. (2014). The influence of groundwater inputs and age on nutrient dynamics in a coral reef lagoon. Mar. Chem. 166, 36–47. doi: 10.1016/j.marchem.2014.08.004
Taniguchi, M., Dulai, H., Burnett, K. M., Santos, I. R., Sugimoto, R., Stieglitz, T., et al. (2019). Submarine groundwater discharge: updates on its measurement techniques, geophysical drivers, magnitudes, and effects. Front. Environ. Sci. 7:141. doi: 10.3389/fenvs.2019.00141
Thibault, M., Lorrain, A., and Houlbrèque, F. (2021). Comment on trophic strategy and bleaching resistance in reef-building corals. Sci. Adv. 7:eabd9453. doi: 10.1126/sciadv.abd9453
Torréton, J., Pagès, J., and Talbot, V. (2002). Relationships between bacterioplankton and phytoplankton biomass, production and turnover rate in Tuamotu atoll lagoons. Aquat. Microb. Ecol. 28, 267–277. doi: 10.3354/ame028267
Torréton, J.-P., Rochelle-Newall, E., Pringault, O., Jacquet, S., Faure, V., and Briand, E. (2010). Variability of primary and bacterial production in a coral reef lagoon (New Caledonia). Mar. Pollut. Bull. 61, 335–348. doi: 10.1016/j.marpolbul.2010.06.019
Turner, T. F., Collyer, M. L., and Krabbenhoft, T. J. (2010). A general hypothesis-testing framework for stable isotope ratios in ecological studies. Ecology 91, 2227–2233. doi: 10.1890/09-1454.1
Venables, W. N., and Ripley, B. D. (2002). Modern Applied Statistics with S, 4th Edn. New York, NY: Springer.
Vroom, P. S., Smith, C. M., Coyer, J. A., Walters, L. J., Hunter, C. L., Beach, K. S., et al. (2003). Field biology of Halimeda tuna (Bryopsidales, Chlorophyta) across a depth gradient: comparative growth, survivorship, recruitment, and reproduction. Hydrobiologia 501, 149–166.
Wada, E., and Hattori, A. (1976). Natural abundance of 15N in particulate organic matter in the North Pacific Ocean. Geochim. Cosmochim. Acta 40, 249–251. doi: 10.1016/0016-7037(76)90183-6
Wainright, S. C., Haney, J. C., Kerr, C., Golovkin, A. N., and Flint, M. V. (1998). Utilization of nitrogen derived from seabird guano by terrestrial and marine plants at St. Paul, Pribilof Islands, Bering Sea, Alaska. Mar. Biol. 131, 63–71. doi: 10.1007/s002270050297
Wantiez, L., Frolla, P., Goroparawa, D., and Keller, F. (2013). Communaut s Biologiques et Habitats Coralliens des Atolls d’Entrecasteaux. Etat des lieux 2012. Maintien de l’int grit du bien. Gouvernement de la Nouvelle-Cal donie. Universit de la Nouvelle-Cal donie.
Walters, W. W., Chai, J., and Hastings, M. G. (2019). Theoretical phase resolved ammonia–ammonium nitrogen equilibrium isotope exchange fractionations: applications for tracking atmospheric ammonia gas-to-particle conversion. ACS Earth Space Chem. 3, 79–89. doi: 10.1021/acsearthspacechem.8b00140
Keywords: seabird, island, coral reef, stable isotopes, nitrogen, SIBER
Citation: Thibault M, Houlbreque F, Duprey NN, Choisnard N, Gillikin DP, Meunier V, Benzoni F, Ravache A and Lorrain A (2022) Seabird-Derived Nutrients Supply Modulates the Trophic Strategies of Mixotrophic Corals. Front. Mar. Sci. 8:790408. doi: 10.3389/fmars.2021.790408
Received: 06 October 2021; Accepted: 29 November 2021;
Published: 04 January 2022.
Edited by:
Matthew Lewis, Bangor University, United KingdomCopyright © 2022 Thibault, Houlbreque, Duprey, Choisnard, Gillikin, Meunier, Benzoni, Ravache and Lorrain. This is an open-access article distributed under the terms of the Creative Commons Attribution License (CC BY). The use, distribution or reproduction in other forums is permitted, provided the original author(s) and the copyright owner(s) are credited and that the original publication in this journal is cited, in accordance with accepted academic practice. No use, distribution or reproduction is permitted which does not comply with these terms.
*Correspondence: Martin Thibault, bWFydGluLnRoaWJhdWx0QGlyZC5mcg==