- 1Laboratory for Ocean and Coast Geology, Third Institute of Oceanography, Ministry of Natural Resources, Xiamen, China
- 2Laboratory for Marine Geology, Qingdao National Laboratory for Marine Science and Technology, Qingdao, China
- 3College of Civil Engineering, Fuzhou University, Fuzhou, China
Owing to the development of the social economy, the geographical environment and ocean utilization patterns of urban coastal zones have changed. This change, in turn, has influenced the socio-economic development of urban coastal zones. Based on the Geographic Information System technology, the area, coastline length, and shoreland use function of reclamation areas were obtained from the geographic charts (1954–2020) and remote sensing data (1988–2017) of Quanzhou Bay. In this study, we analyzed the geomorphologic change process and the relationship between land use patterns and economic development in Quanzhou Bay from the perspectives of hydrodynamics, sediments, and human activity. Our results indicated that over the past 70 years, the bay area has reduced by 21.5%. The length of the coastline decreased from 208.36 km in 1959 to 149.11 km in 1988, whereas the shape index of the bay (SIB) decreased from 3.09 to 2.41 during the same period. Between 1988 and 2017, the coastline increased to 162.91 km, causing the SIB to increase to 2.72. The artificial index of the bay increased from 0.28 in 1959 to 0.90 in 2017. The intensity of bay the development (IBD) first increased from 0.27 in 1959 to 0.77 in 2006. During the transition to a more modern society (2006 to present), the IBD slightly decreased to 0.73 in 2017. Affected by human activity, the transformation of the reclaimed land in Quanzhou Bay can be divided into four stages that are closely linked to the economic development in the region. In the early industrialization period, reclaimed land in the region was used for agricultural production, whereas in the mid-industrialization period, it was gradually transformed into a combination of industrial (29.8%) and agricultural (56.1%) lands. In the later period of industrialization, the reclaimed land was gradually converted into urban industrial and port lands. Finally, with further refinement and upgrading of economic and industrial structures, the socio-economic and environmental benefits from coastal reclamation projects have been increasing, whereas the proportion of economic benefits (in the total benefits) has been decreasing. The results of this study can provide decision-making references for the optimization of utilization patterns and the economic development of reclamation lands in coastal areas.
Introduction
As a link between land and sea, the urban coastal zone is rich in natural resources and has a high biological productivity. It also plays an important strategic role in social and economic development as a port for international trade (Akan et al., 2020). Due to the availability of rich marine resources and rapid economic development in urban coastal zones, the population of urban coastal zones has been increasing. In 2021, the eastern seaboard of China accounted for 39.93% of the population, with an upward trend of 2.15% from 2010, whereas coastal cities in the United States of America (USA), such as New York City and Washington DC, have witnessed an increase in their populations in the last decade (data from the National Bureau of Statistics, 2021; U.S. Census Bureau, 2021). With the increasing intensity of human activity, Earth has entered the epoch of the Anthropocene era, and human activity has gradually become the dominant external force causing environmental changes on Earth (Day et al., 2021). Therefore, as a typical region evidently influenced by human activity, studying the characteristics of urban coastal zone changes can help understand the process, mechanism, and impact of anthropological activities on the environment. Notably, this is conducive to avoiding wastage of resources and can provide viable scientific references for regional sustainable development.
Changes in the geomorphology of the urban coastal zone have a certain process (Martínez et al., 2011). Due to the different degrees of economic development, cities around urban coastal zones also have different degrees of development, which results in different geomorphological changes (Aldasoro-Said and Ortiz-Lozano, 2021). In the past, the major industries in urban coastal zones were generally small-scale transportation, commerce, and agriculture, which have little impact on the evolution of urban coastal zone geomorphology (Pourkerman et al., 2020). In recent decades, most urban coastal zones in developing countries have been in the middle or late stages of industrialization. As a result of a large amount of human activity, the coastline and topography of urban coastal zones have changed significantly over a short period (Sun et al., 2020). However, in some developed countries, urban coastal zones have completed the process of industrialization, urbanization, and economic development; however, due to this development, the surrounding environmental and ecological problems have increased significantly (Naimi and Muhanna, 2021). Understanding the different stages of the impact of urban coastal zone geomorphology change factors can help us to understand human activity in the region and its effects on the environment.
During the entire development process of the urban coastal zone, the factors that affect geomorphologic evolution primarily include natural and anthropogenic factors (Feng et al., 2021). Natural factors include ocean current erosion and sediment deposition (Maren et al., 2016). With the economic development and population growth, human activity has gradually become the main factor affecting geomorphologic changes in urban coastal zones (Li and Wang, 1984). Notably, the human activities in urban coastal zones primarily include hydraulic structures and reconstruction projects, channel dredging, sand mining, artificial coast building, and aquaculture development (Giosan et al., 2013). The impact of human activities on the geomorphologic changes in urban coastal zones is primarily reflected in the changes in the hydrodynamic and sedimentary environmental conditions (Suo and Zhang, 2015). The construction of reservoirs and sluices in the upper part of the watershed can lead to a reduction in the runoff and sediment transport, causing topographic changes, such as erosion of the submerged delta or displacement of the coastline (Darwish et al., 2017; Maloney et al., 2018). Reclamation projects and artificial structures directly change the coastline shape of urban coastal zones and impede the circulation of sediment material and energy fluxes (Loh et al., 2018), resulting in changes in the velocity and amplitude of tidal currents (Yoon et al., 2020). At the same time, channel dredging and sand mining directly change the underwater topography and then, change the hydrodynamic environment of the corresponding region (Mossa et al., 2017). In addition, industrial production and aquaculture in the reclaimed area increase the influx of artificial compounds and industrial heavy metals into the bay, altering the material composition of the sediments (Fan et al., 2022). These pollutants can attach to the sediments and then, remain in the seabed and tidal flats, causing environmental degradation of coastal zones and threatening the sustainable development of the urban coastal zone society and environment (Deng et al., 2020).
In the past, the developers only considered the economic benefits of the land without considering social development and environmental protection according to the human and geographical environment of the region, which restricts the sustainable development of urban coastal zones and deters the full utilization of resources (Cao et al., 2020). At present, most of the research data on environmental evolution have poor spatial and temporal continuity, and the process of geomorphic evolution under the influences of human activity, along with the main driving factors of the process, are not fully understood (Martino et al., 2021). Future studies on the transformation process of the urban coastal zone topography and reclaimed land-use patterns are urgently needed to provide a reference for decision making to optimize the reclamation utilization pattern in coastal zones and promote sustainable development in urban coastal zone economies.
Quanzhou Bay is located on the southeast coast of China and is surrounded by well-developed cities as a typical urban coastal zone (Figure 1), with the total land area of the city being 1.10 × 104 km2 and sea area being 1.28 × 102 km2. The permanent population of the region was 8.78 × 106 in 2020 (data from Quanzhou Statistical Information Network, 2021). The sediments of Quanzhou Bay are primarily derived from the Jinjiang River, of which the annual sediment flux is 2.54 × 106 t (Lin et al., 2019). The sediment types in the bay were dominated by silt and clay, whereas the sand content was relatively low. Quanzhou Bay is a strong tidal bay, with a tidal current that has constantly alternating movement (Figures 2B,C), and its perennial wind and wave directions are primarily North-North-East (NNE) – North-East (NE) and South-South-West (SSW), respectively (Huang et al., 2018; Lin, 2020). Changes in the sea area (including coastline, shoreland, topography, reclamation projects, etc.) are closely related to local economic development and have distinct stage-specific development characteristics. Over the past 70 years, Quanzhou has undergone the entire process of transforming economic agents. Therefore, it is an ideal natural laboratory for conducting research and theory-guided practice on the topographic evolution of urban coastal zones under the impact of human activity.
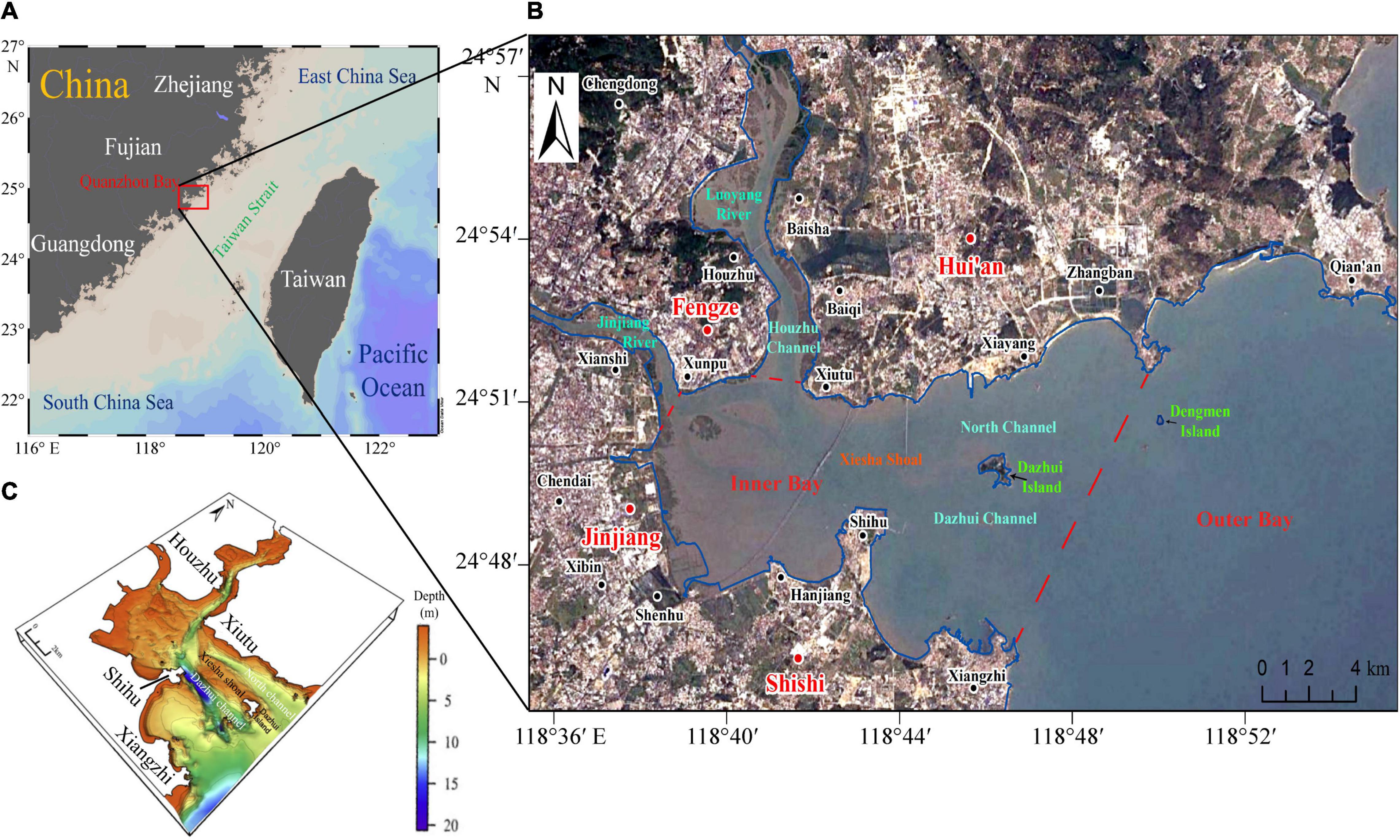
Figure 1. Overview of the study area. (A) The location of the study area (From Ocean Data View); (B) The basic information of Quanzhou Bay, including cities, islands, channels, and rivers (The background image is a satellite image of Quanzhou Bay in 2020 from Google Earth Pro); (C) Landform and topography of Quanzhou Bay (Chen et al., 2011).
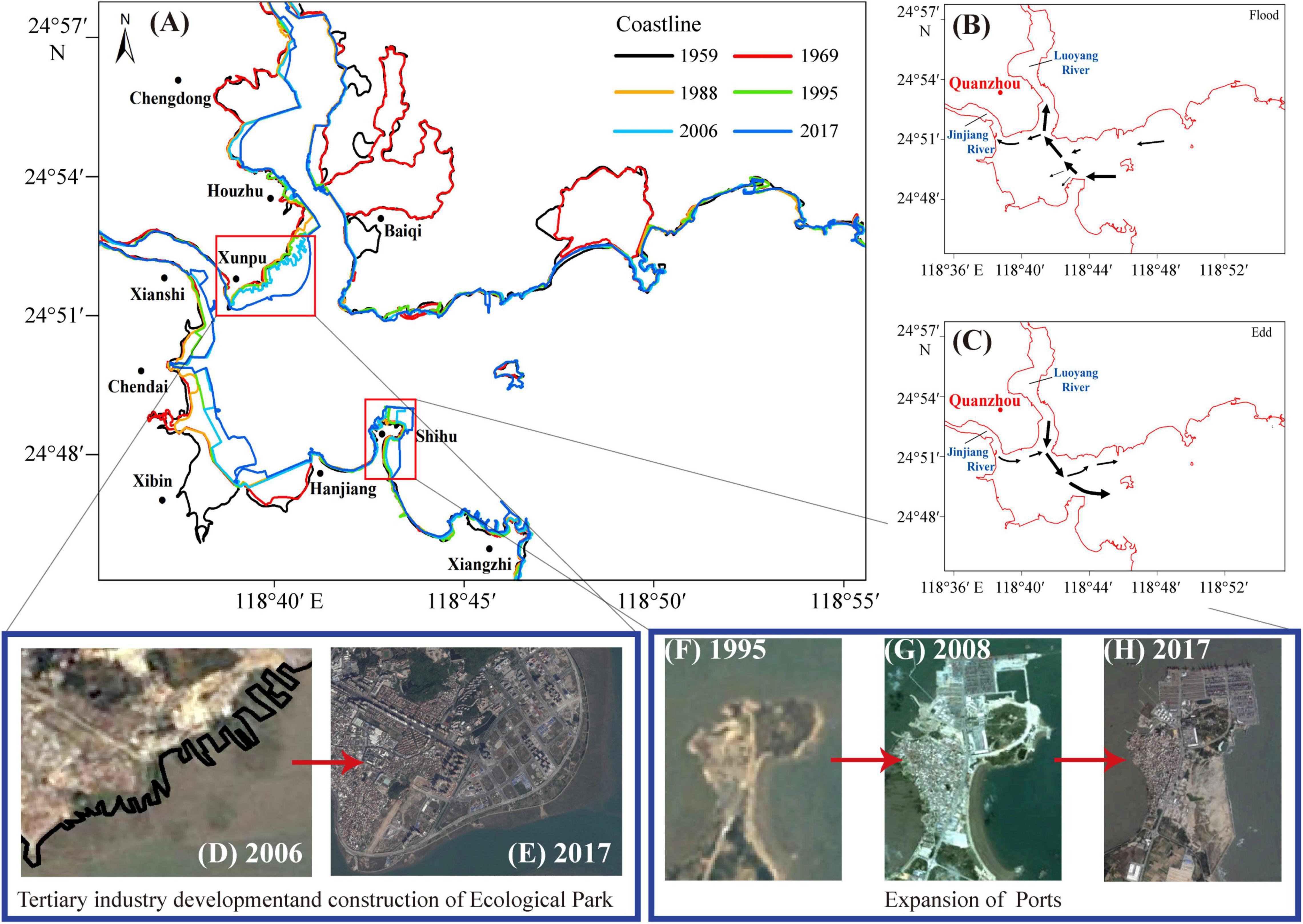
Figure 2. Tidal current direction, coastline and reclamation changes in Quanzhou Bay. (A) Coastline change; (B) Flood current; (C) Ebb current; (D,E) Urban expansion in Fengze; (F–H) Shihu Port expansion [(D–H) images are from Google Earth Pro].
Based on the historical geographic charts, satellite images, and economic data of Quanzhou Bay, in this study, we discussed the topographic change processes and main influencing factors of urban coastal zones under the influence of human activity over the past 70 years. The research results can provide a strategic reference for estimating future economic development in coastal cities and promoting sustainable development in urban coastal zones.
Materials and Methods
Marine Map and Satellite Image Data
In this study, six large-scale geographic charts (for the period 1954–2017) of Quanzhou Bay were selected as the source of bathymetric and coastline data (Table 1), and the lowest theoretical datum was used for bathymetric data (Liu et al., 2020). The remote sensing data were satellite images from 1988, 1995, 2006, and 2017, respectively (from Google Earth) (Table 2).
All charts were converted to the WGS84 coordinate system and Mercator projection using ArcGis (Environmental Systems Research Institute, United States), to eliminate discrepancies caused by the differences in geographic coordinate systems and projection methods. The digital elevation model and raster data files were constructed using digital bathymetry data, according to Chen et al. (2020). The remote sensing information was referenced to a 1:35,000 chart and covered the entire research area. Each image had more than eight control points to carry on the geometric correction; then, the information for the coastline, including the reclamation method used in the region, was extracted. By comparing the charts of different years and the data extracted from remote sensing (Li et al., 2018b), changes in the isobath and coastline were analyzed, and the rates of erosion and deposition were obtained to determine the evolutionary processes in the regional topography.
Parameters
Shape Index of the Bay
By calculating the ratio of the circumferences of the bay and the circle of the equal area and comparing the similarity between the shape of the bay and the circle, the complexity of the shape of the bay was determined (Chen et al., 2020). The SIB was calculated as follows:
where P is the perimeter of the bay (m) and A is the area of the bay (m2).
Artificial Index of the Bay
The artificial coastline index indicates the degree of transformation from the natural coastline to the artificial coastline. Notably, it reflects the degree of interference of human activity with the natural coastline (Li et al., 2018b). The artificial index of the bay AIB was calculated as follows:
where T is the artificial coastline length and L is the total coastline length of the study area.
Intensity of Bay Development
The IBD represents the intensity of human activity in the coastal zone (Song et al., 2019), which can be calculated as follows:
where i is the type of coastline, li is the length of the type i coastline, n is the sum of the types of coastlines, L is the length of the coastline in the study area, and pi is the environmental impact factor of the type i coastline (Table 3). Notably, the higher the pi-value, the greater the negative impact of human activity on the bay.
Real Gross Domestic Product per Capita
Real GDP per capita is intrinsically linked to the process of urban industrialization and is related to the structure of the economy. It can objectively reflect the level of social development and the degree of development over a certain period (Wu, 2020). The structural changes and degree of industrialization of urban coastal zones can be determined by calculating the gross national product in different periods. The real GDP per capita can be expressed by the following equation:
Results
Based on the data analysis and calculation, the geomorphological changes in Quanzhou Bay primarily include the sea area, length and types of coastlines, and shoreland reclamation land use patterns. These changes can be divided into different stages based on their different characteristics.
Changes in Coastline and Sea Area
Coastline and Sea Area Changes From 1954 to 1989
The period from 1954 to 1989 was the main period of reclamation in the bay. According to the results of coastline extraction and classification (Figure 2A), during this period, the coastline length decreased by 7.28 km from 1959 to 1969 and 51.97 km from 1969 to 1988, and the sea area decreased by 13.34 and 45.62 km2, respectively.
This period can be divided into two sub-stages based on coastline length change. The first sub-stage was from 1954 to 1969. In this period, the main reclamation projects were the Xibin Farm Reclamation (along the north bank of the Luoyang River) and the urban expansion of the Baiqi village, with reclaimed areas of 2.83, 0.78, and 1.54 km2, respectively. The length of the urban coastline increased by 11.01 km, whereas that of the aquaculture and reclamation coastline increased by 4.63 and 1.34 km, respectively (Table 4). The second sub-stage was from 1969 to 1988. The reclamation projects primarily included the Wuyi, Qiyi, and Chengdong reclamations, along with the extension of the Xibin Farms reclamation on the west side of Hanjiang, covering areas of 17.49, 13.03, 7.54, and 3.02 km2, respectively. The natural coastline was reduced by 46.7% owing to the transformation to the artificial concrete coastline. After the reclamation project along the Luoyang River was completed, the berm coastline was reduced to 14.19 km. With the reconstruction of Houzhu Port and the development of coastal aquaculture, the port coastline and aquaculture coastline increased by 5.23 and 8.29 km, respectively (Table 4).
Coastline and Sea Area Changes From 1988 to 2017
A decrease in reclamation activity and a slight increase in coastline length occurred from 1988 to 2017 (Table 5). In 1988–1995, 1995–2006, and 2006–2017, the coastline length increased by 2.66, 7.55, and 3.59 km, respectively, whereas the sea area decreased by 3.3, 5.26, and 10.32 km2, respectively.
The major reclamation works between 1988 and 2006 were carried out at the coasts of the Jinjiang River, Houzhu, and the northern part of Shihu, with a total reclaimed area of 2.77 km2. During this period, the natural coastline decreased by 41.11 km, whereas the urban coastline increased by 42.82 km (Table 4).
The area of reclamation projects in Quanzhou Bay increased from 2006 to 2017. The reclamation projects were primarily located at the north bank of Luoyang River and the coast from Chendai to Hanjiang, covering an area of 4.09 and 3.84 km2, respectively (Table 4). At the same time, the construction of the flood control seawall along the south bank of the Luoyang River was lengthened. The Shihu Port was further expanded, and the coastline was thus, straightened, turning the eastern coastline into an entirely artificial coastline. The natural coastline declined to 15.94 km, and part of the urban coastline was converted into port and berm coastlines. The expansion of Shihu Port led to a significant increase in the port coastline (from 13.59 to 23.20 km) and an increase in the aquaculture coastline (from 15.68 to 16.39 km) (Table 4).
Change of Topography in Different Periods
Changes in Isobath in Different Periods
Variations in isobaths in Quanzhou Bay primarily occurred in the Houzhu, Dazhui, and northern channels, among which the variations in isobaths at 0, 5, 10, and 20 m were more significant. The changes in the isobath can be divided into two periods (Figure 3).
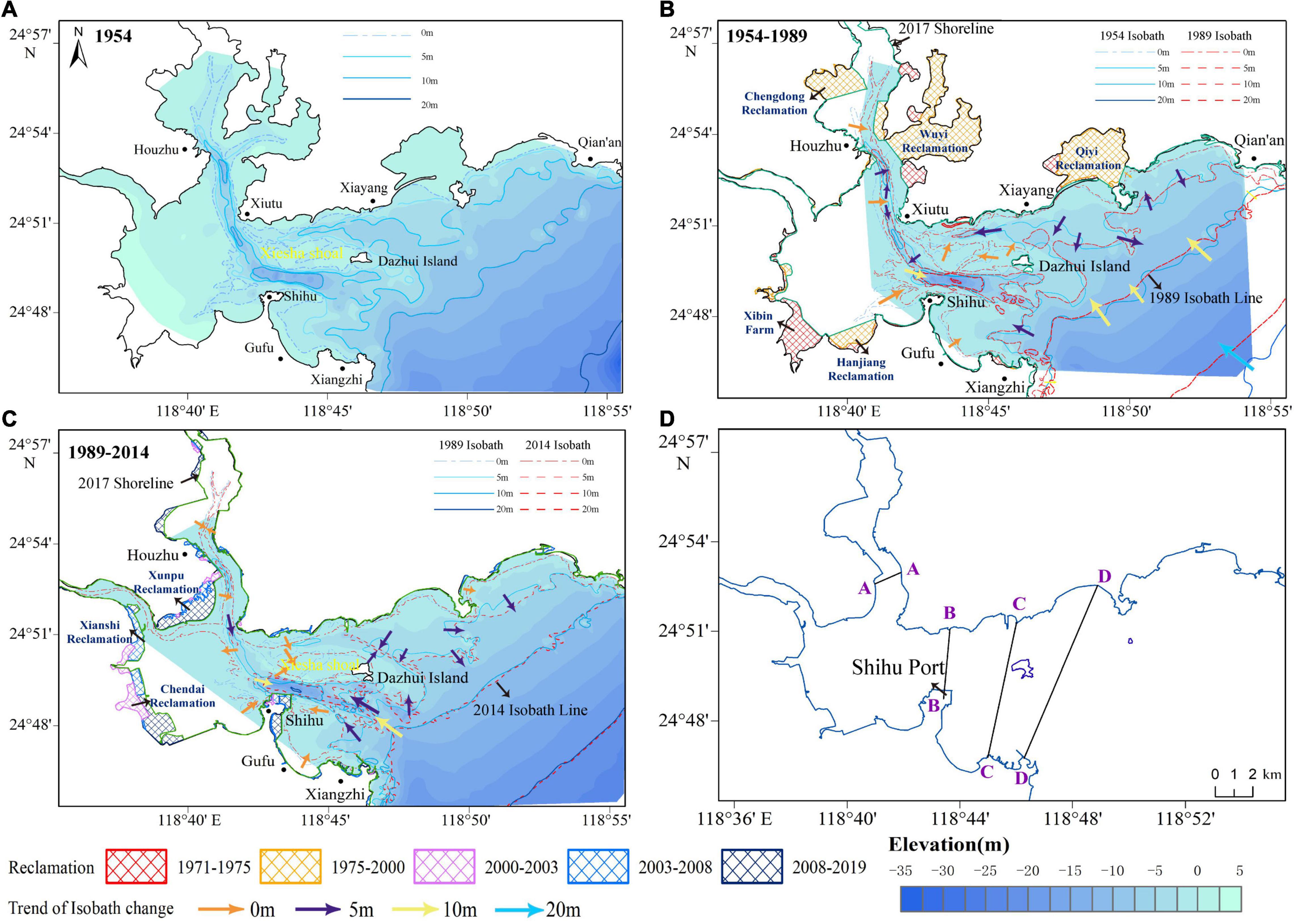
Figure 3. Variation of isobath in Quanzhou Bay from 1954 to 2017. (A) 1954; (B) 1954–1988; (C) 1988–2017; (D) position of characteristic section. The arrows indicate changes in the isobath.
From 1954 to 1989 (Figures 3A,B), after the large reclamation projects and the construction of artificial water control projects in the upper reaches of the river, the 0-m isobath from the Luoyang River estuary to Xiutu moved to the central axis of the channel. At the position of Gufu to Xiangzhi, the 0-m isobath moved seaward, with an average of 0.12 km. The 0-m isobath in the Xiesha Shoal extended outward, leading to a 0.15-km wide valley. The balance between erosion and deposition was maintained along the coast from Xianyang to Qian’an. In the Houzhu Channel, the isobath of 5 m was interrupted and contracted. The isobath of 10 m disappeared in the Houzhu Channel and moved landward on the direct line from Xiangzhi to Qian’an at the same time. The 20-m isobath at the southeast of the bay moved 2 km on average.
From 1989 to 2014 (Figures 3B,C), in the Houzhu Channel, the 0-m isobath continued to move toward the central axis of the channel. In the east of Shihu, the 0-m isobath retracted westward. The 0-m isobath of nearby the Gufu to Xiangzhi path moved seaward 0.2 km on an average. The area of the 5-m deep area of the Houzhu Channel was reduced. A channel appeared in the south of Xunpu, with an average width of 0.3 km. Meanwhile, two artificial channels with depths of 5 and 10 m appeared on the north and south of Dazhui Island, respectively. The position near the 20 m isobath maintained a balance between erosion and deposition.
Changes of Erosion and Deposition in Different Periods
The erosion and deposition changes of the seabed were obtained according to the information of the planar distribution of water depth and the longitudinal profiles of four characteristic locations (Figures 3D, 4A), which indicated different characteristics at different periods.
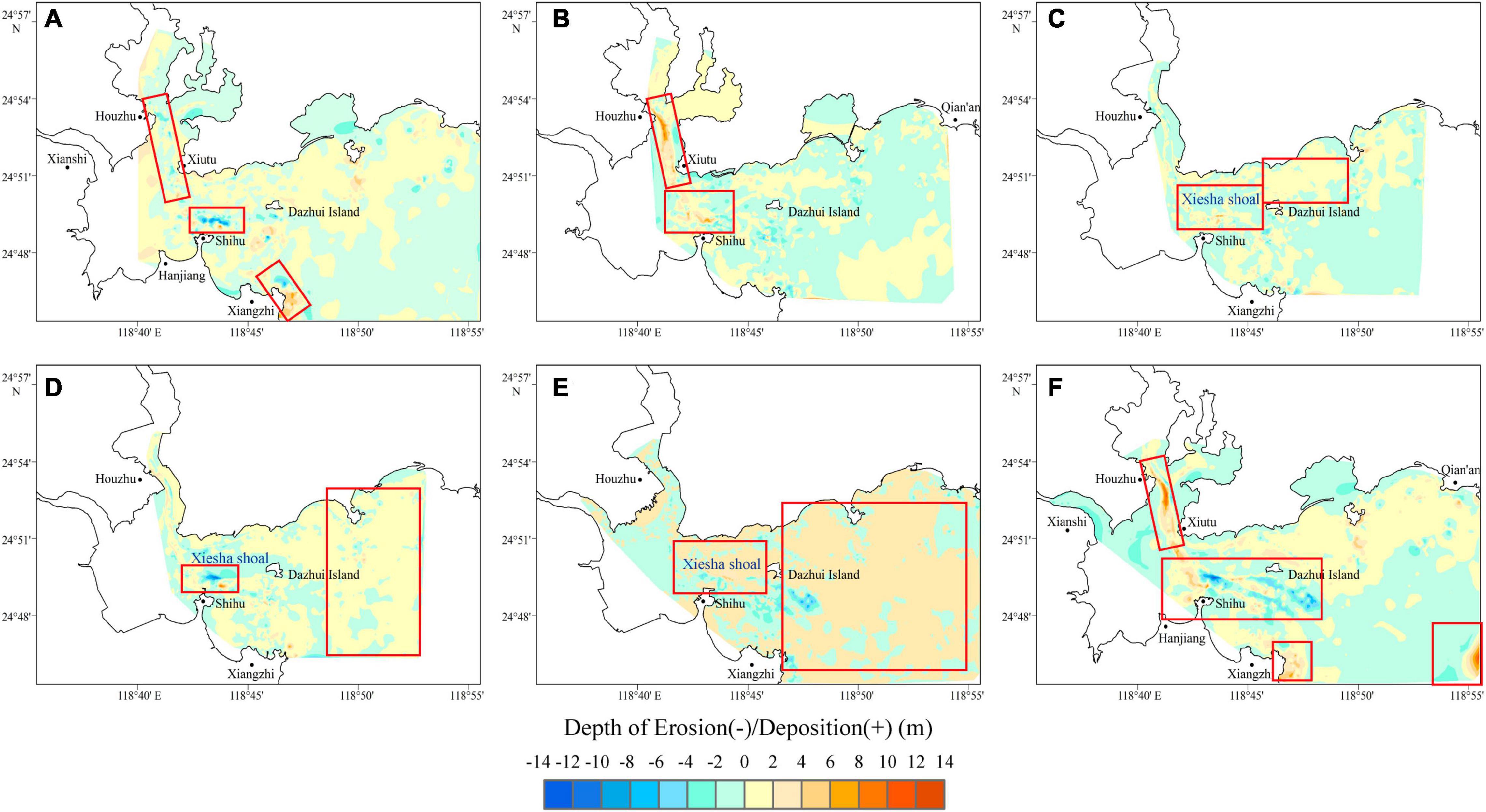
Figure 4. Variation of water depth in Quanzhou Bay from 1954 to 2017. (A) 1954–1968; (B)1968–1989; (C) 1989–1998; (D) 1998–2007; (E) 2007–2014; (F) 1954–2014. Most of the blank areas in the bay were mudflats over 0 meters, and the blank area at the entrance of the bay was the repeated bathymetric information.
Erosion during the period 1954–1968 ranged from –12.33 to 0 m (a negative value indicates the depth of erosion), whereas deposition during this period ranged from 0 to 9.47 m (Figure 4A). The west side of Xiutu and the northern part of Dazhui Island were raised by 1 m due to deposition. The maximum deposition area was located to the east of Xiangzhi, with an average annual deposition rate of 0.68 m/yr. Notably, the main eroding areas were located in the east of Houzhu and north of Shihu and Xiangzhi. The largest eroding sites were located to the north of Shihu (as section B-B) (Figure 5B), which indicated an eroding depth of approximately 2 m, with an average annual erosion rate of 0.88 m/yr.
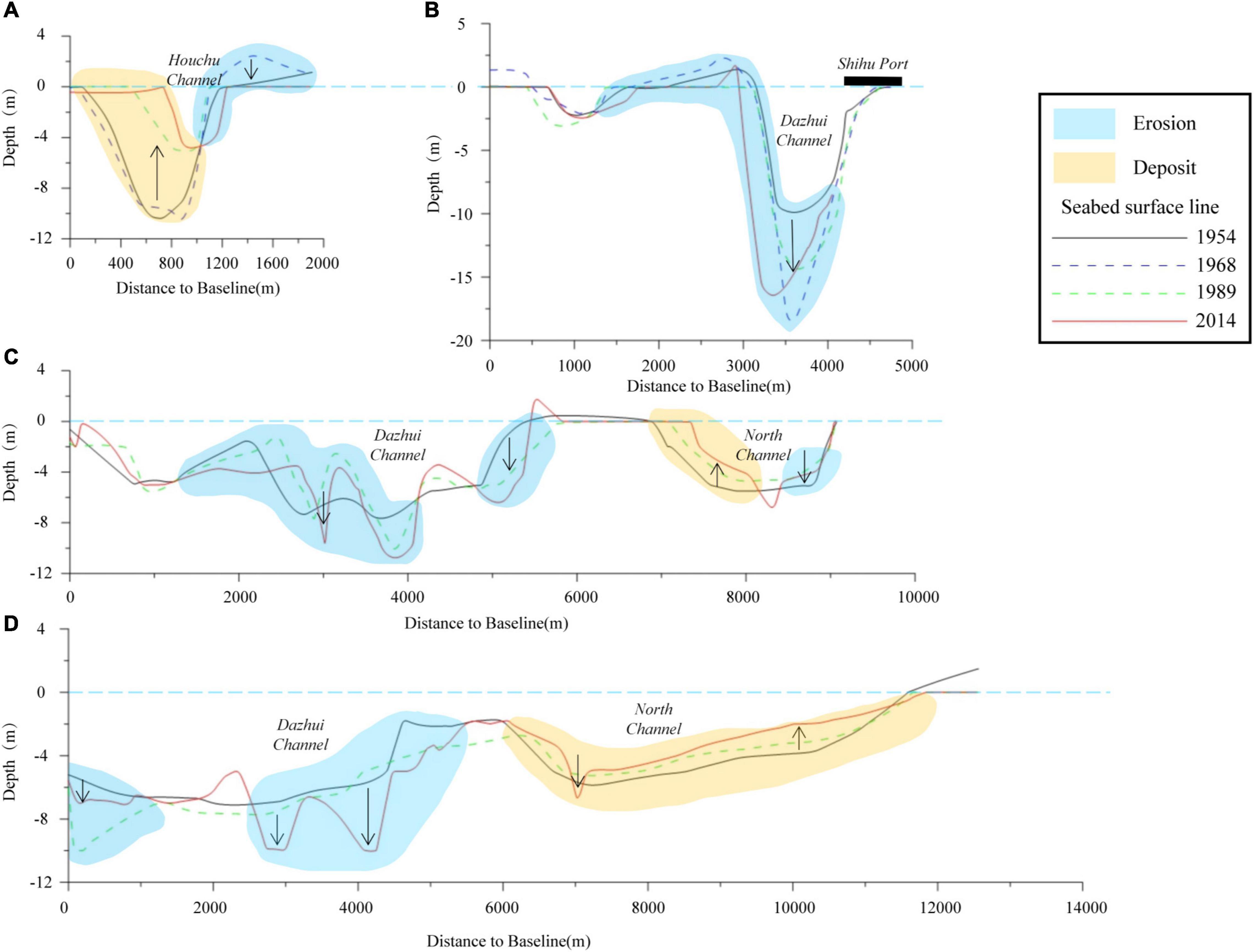
Figure 5. Cross-shore topography variations along four profiles during the four different stages of industrialization from 1954 to 2017. The upward arrow indicates deposition, and the downward arrow indicates erosion. The elements in the section (A–D) are explained in part labels, where the blue areas represent erosional areas, the orange areas represent deposital areas, and different types of lines represent the seabed surfaces in the section for different years.
After the construction of extensive reclamation projects from 1968 to 1989 (Figure 4B), erosion and deposition in Quanzhou Bay were significantly different. Erosion ranged from –8.05 to 0 m, whereas deposition was observed to be ∼12.32 m. During this period, the main sedimentation area was located in Houzhu Channel. As shown in section A-A (Figure 5A), the maximum deposition depth was 6 m, with an average annual deposition rate of 0.59 m/year. Massive deposition occurred from the northern part of Shihu to Xiutu, with a deposition range of 1–2 m in section C-C (Figure 5C). The depth of water along the coastline from Xiutu to Qian’an generally increased, and the largest eroded area appeared to the south of Dazhui Island, with an average annual erosion rate of 0.38 m/yr.
From 1989 to 1999, the erosion ranged from –6.92 to 0 m, whereas the deposition ranged from 0 to 9.30 m (Figure 4C). Notably, the main deposition areas were primarily concentrated in the inner bay. The Houzhu Channel subsidence was located on the south side of the channel, and the depositions in the north of Xiangzang and northeast of Dazhui Island were in the range of 1–2 m. The deposition in the northern part of Shihu was the largest, with an annual average sedimentation rate of 0.93 m/yr. The main eroding areas were in Houzhu Port, the eastern part of Shihu, and the Xiesha Shoal. The largest erosion occurred in the southern part of Dazhui Island, with an average annual erosion rate of 0.69 m/yr.
From 1999 to 2007 (Figure 4D), erosion ranged from –11.66 to 0 m, whereas deposition ranged from 0 to 9.29 m. During this period, the entire Houzhu Channel area was still deposited. There were small deposits in the northern part of Shihu and the eastern part of Xiangzhi. The largest deposition occurred in the northern part of Shihu, with an average annual deposition rate of 1.16 m/yr. The main eroding area was located in the Xiesha Shoal, with an average annual erosion rate of 1.46 m/yr.
From 2007 to 2017 (Figure 4E), erosion ranged from –10.72 to 0 m, whereas deposition ranged from 0 to 6.64 m. During this time, the Xiesha Shoal area was the main deposition area. There was a large area of deposition at the entrance of the bay, and the amount of deposition in this area increased compared with the previous period. The maximum deposition location was from the Xiesha Shoal to the Dazhui Island Channel, with an average annual deposition rate of 0.66 m/yr. The total water level in the Houzhu Channel decreased, and the maximum eroding area was in the southwest of Dazhui Island, with an average annual erosion rate of 1.07 m/yr. There were two artificial channels in the bay (Figure 5D), the changes in the estuary and the bay coast were small, and the balance of erosion and deposition was maintained.
There were two artificial channels in the bay (Figure 5D), the changes in the estuary and the bay coast were small, and the balance of erosion and deposition was maintained. During the period 1954–2014, it can be seen that the seabed changes in the inner bay of Quanzhou Bay were mainly concentrated in the area around Houzhu and Shihu Port (Figure 4F). The main features of the two areas are different degrees of deposition and obvious traces of artificial dredging.
Changes of Land Use Pattern in the Reclaimed Area Around Quanzhou Bay
According to Chanali’s theory of industrialization stage (Jin et al., 2018), the industrialization development in Quanzhou Bay over the past 70 years has occurred in multiple stages; the land use functions of reclaimed areas under different stages also differs (Figure 6A).
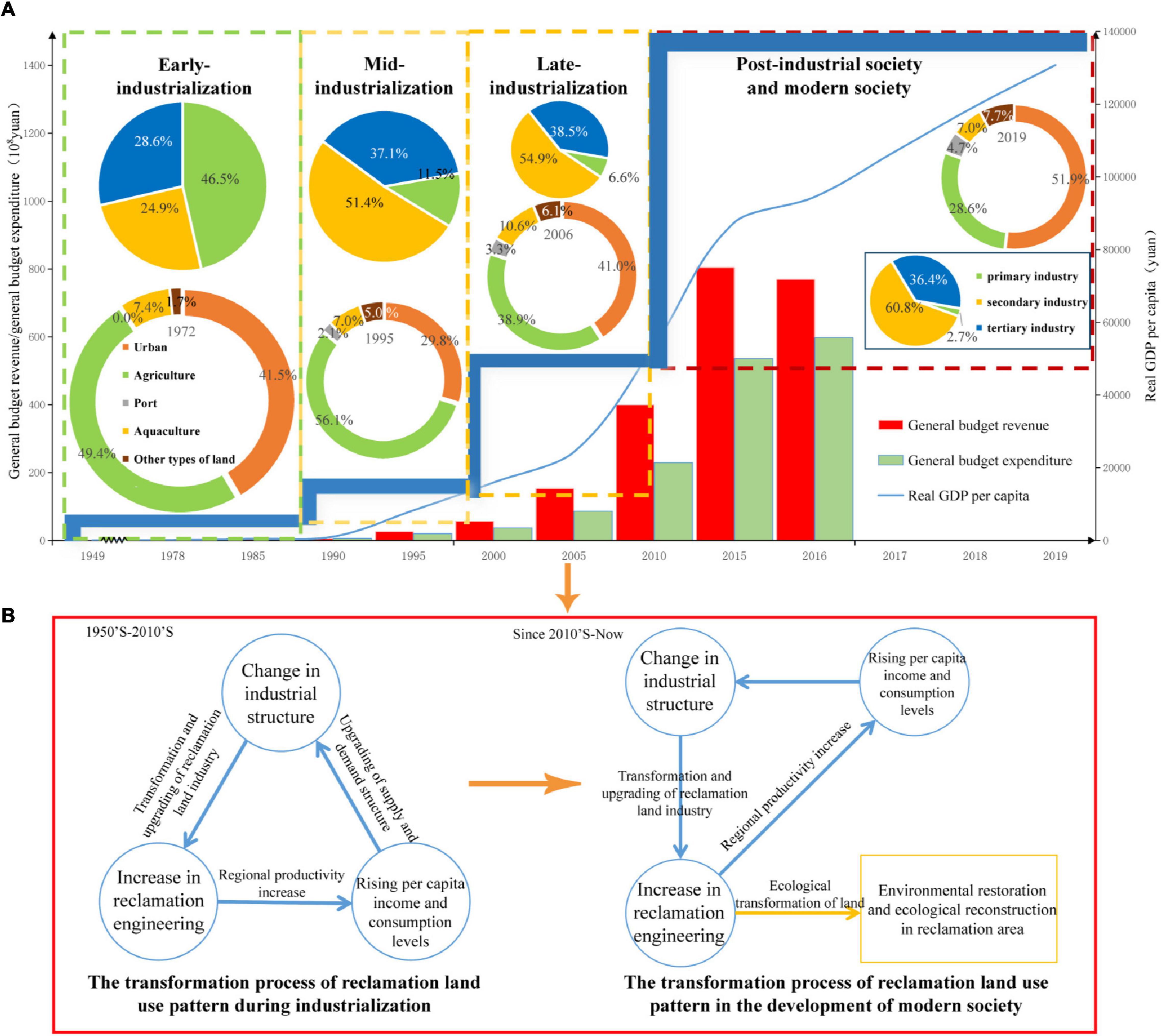
Figure 6. (A) The stages division of land reclamation with economic structure in Quanzhou Bay. The pie chart shows the proportion of different industries; the circular chart shows the proportion of different land uses; GDP, population, General budget revenue, and General budget expenditure data from http://tjj.quanzhou.gov.cn/tjzl/tjsj/. (B) Conversion model of reclaimed land in Quanzhou Bay.
From the 1950s to the mid-1980s, Quanzhou was in the early stage of industrialization, with the per capita GDP increasing from 60 Chinese yuan to 6,000 Chinese yuan. During this period, the economic structure of Quanzhou was primarily composed of the primary industry. Notably, Quanzhou was in the early stage of economic development between the 1950s and 1960s. Reclamation projects were all carried out for agricultural production. The area of agricultural land accounted for 49.4% of the total reclaimed area, indicating that the reclaimed land was primarily used for agricultural production, and the total arable land area increased (Figure 7C and Table 6). However, the output values of the secondary and tertiary industries were relatively low, owing to the shortage of raw materials, low production levels, and low product values. Therefore, the primary industry grew faster than the other two industries (Figure 7A). Until the 1970s, large-scale reclamation projects were conducted. Part of the reclaimed land was used for agriculture and animal husbandry, and part of it was used for industrial development. During this time, the factories of saltworks, small-scale food, and handicrafts were established and began production, resulting in a rapid increase in the secondary industry output value. Even then, the primary industry remained as a pillar industry during this time (Figure 7A).
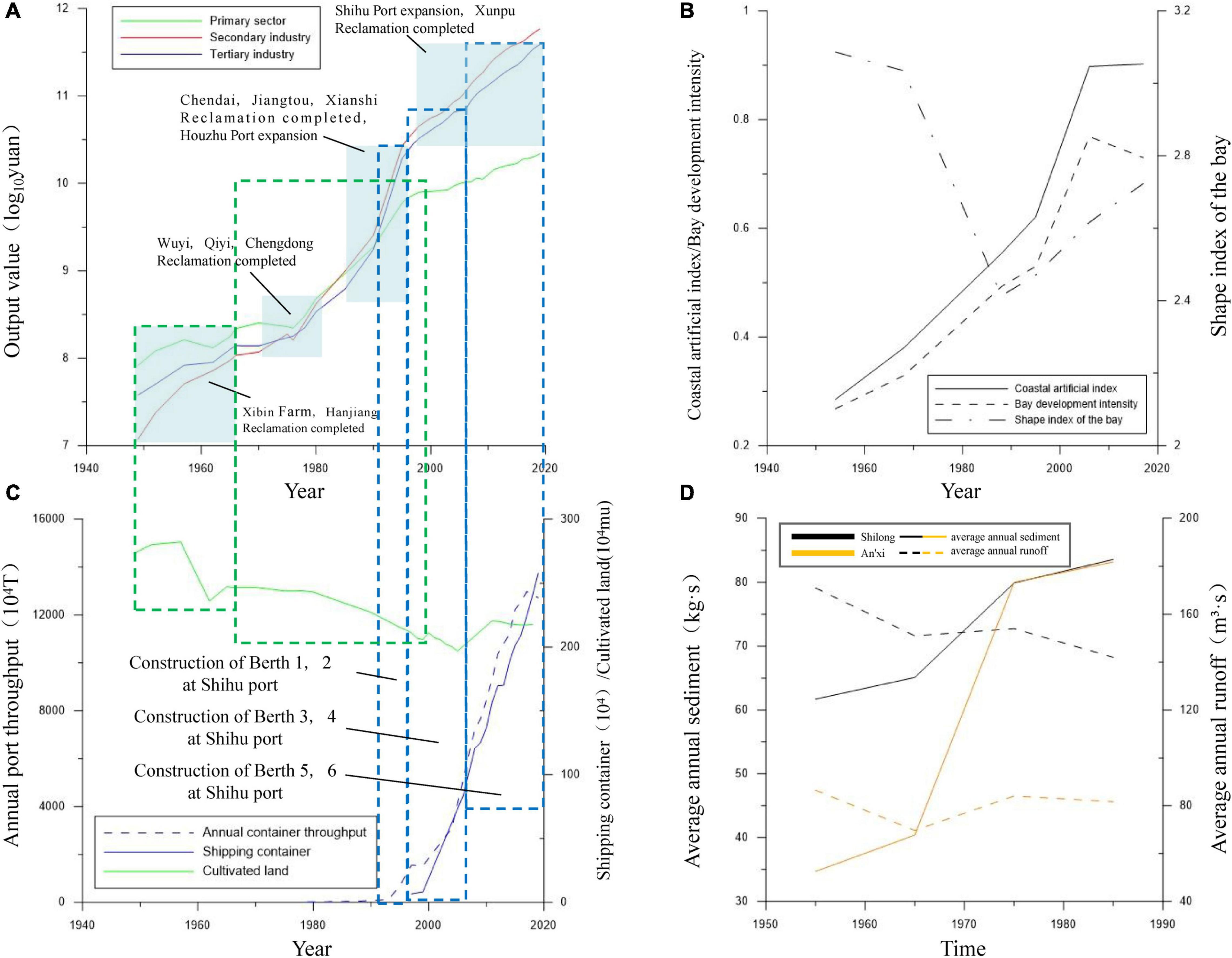
Figure 7. Changes of industrial output value and correlation coefficient. (A) Annual changes in the output value of the three major industries (economy data from http://tjj.quanzhou.gov.cn/tjzl/tjsj/). (B) CHANGES of coastline parameters. (C) Area of cultivated land, shipping throughput and the number of containers, (D) Sediment transport and annual runoff variation.
From the mid-1980s to the late-1990s, Quanzhou entered the middle stage of industrialization, and the per capita GDP increased to 18,000 Chinese yuan. With the Reform and Opening-up Policies, urban industrial land accounted for half of the reclaimed land, whereas small-scale processing industries began to construct factories and began operation. Thus, the output value of the secondary industry gradually approached that of the primary industry, leading to the beginning of changes in the industrial structure of this region (Figure 7A). Furthermore, the pace of transformation and upgrading of traditional industries accelerated with increasing foreign investment. During this period, the area of agricultural land gradually decreased, whereas that of urban land continued to increase (Table 6). The rapid development of labor-intensive industries, such as regional textile shoes, clothing, and craft products, led to the rapid expansion of the secondary industry. Furthermore, the reconstruction of Houzhu Port and the growth of the shipping-based transportation industry led to a rapid increase in the tertiary industry. By the 1990s, there was an evident change that took place in the regional demand, production, and foreign trade structures, and the demand for reclaimed land had also changed from agriculture and aquaculture to cities, ports, and industrial parks. Notably, the reclaimed land in the Jinjiang River estuary has been primarily used for aquaculture and industrial production. The urban industrial land in the reclaimed area was 17.95 km2, and the aquaculture land increased by two times (Table 6). Furthermore, the annual port capacity and the number of container freight transport increased slightly, due to the expansion of berths at Houzhu Port, and the output value of all three industries increased during this time, with the secondary industry overtaking the primary industry as the main industry (Figures 7A,C).
In the 2000s–2010s, Quanzhou entered the stage of late industrialization. The reclaimed land was primarily around the expansion of Shihu Port and the expansion of the regional town of Hanjiang (Figures 2F,G), where ports and service industries became major industries in the reclaimed areas. The proportion of agricultural land in the reclaimed area decreased from 56.1 to 38.9%. Moreover, the traditional manufacturing industries around the urban coastal zone gradually changed to technology-intensive industries, such as the petrochemical, ship-building, and electronic information industries, leading to rapid growth in the output value of the secondary industries. Commercial and ecological construction began along the Xunpu coast, whereas new 100,000-ton berths were added to Houzhu Port and 1–4# berths were built at the Shihu Port (Figures 2D,E). The port handling capacity and the number of container transports increased rapidly (Figure 7C), and the output values of the second and tertiary industries were higher than that of the primary industry.
In the past 10 years, Quanzhou has entered the transition period between the post-industrial and modern society. The newly reclaimed land was primarily used for expanding cities and building ports (Figures 2F,H), whereas the old reclaimed land was gradually transformed into the residential, financial business, and foreign-funded industrial areas, with an urban industrial land share of 51.9% (Table 6). With the expansion of the port and further refinement of the tertiary industry, the number of transport containers increased, whereas the growth rates of the output values of the second and tertiary industries increased again. The oil, handicraft-related industries, and ports around the reclamation project are currently integrated, resulting in increased industrial relevance, transportation industry operating rate, and degree of industrial convergence (Figure 7C).
Discussion
Evolution Process and Influence Mechanism of Urban Coastal Zone
In this study, the geomorphologic evolution of Quanzhou Bay presented different characteristics at different industrialization stages. At different stages, the factors affecting the geomorphologic evolution were different.
Evolution of Urban Coastal Zone Environment Before Industrialization
From pre-industrialization to the early stage of industrialization, the reclamation projects were concentrated only in a few locations, which had little influence on the shape and topography of the urban coastal zone (Zhu et al., 2017). Thus, we could deduce that the evolution of the urban coastal zone environment is primarily controlled by natural factors, which include hydrodynamics (e.g., river discharge, waves, and tides) and sediment characteristics (e.g., supply, composition, and distribution) (Cao et al., 2021). Except for extreme weather conditions, the natural evolution of urban coastal zones tends to remain stable (Lin, 2020; Sun and Niu, 2021). Under the influence of extreme weather, e.g., typhoons and floods, the sediment particle input increases greatly in a short time, whereas the change in hydrodynamics during typhoons causes sediment resuspension and redistribution that affects the evolution of underwater topography (Lin et al., 2019).
Under natural conditions, hydrodynamics is the main factor that controls the changes in the coastline and topography of urban coastal zones (Li et al., 2020; Zarzuelo et al., 2021). The influence of hydrodynamics on topography results in the erosion of the seabed and coastline by natural ocean currents (Oiwane et al., 2011). As a typical strong tidal bay, the top of Quanzhou Bay is dominated by the river hydrodynamics of the Jinjiang and Luoyang rivers, whereas the middle and river-mouth areas are dominated by ocean dynamics (Wang, 2011). During the flood tide, the flood tidal current and river flow directions are opposite, and the two actions cancel each other, which lowers the flow velocity, reduces the erosion ability, and intensifies sediment accumulation (Feng et al., 2015; Du et al., 2016). During the ebb period, the ebb-tidal current and river flow are in the same direction, and the velocity of the total flow increases, which leads to the enhancement of the scouring capacity of the estuary (Styles et al., 2016). Notably, the bottom seabed on the north side of the Shihu Port was eroded by strong tidal currents caused by a sudden change in the topography of the cape (Lubke, 1985). The same process occurred in the area east of Xiangzhi, where the coastline was eroded and moved landward (Figure 2).
Sediment supply is also an important factor that affects the evolution of erosion and deposition in the bay. Generally, under natural conditions, the distribution of sediments in urban coastal zones remains stable (Martino et al., 2021). In our study area, the sediment particles flow down the Jinjiang River and converge with the Luoyang River into the bay area, where the traction load deposited in the estuary of Jinjiang has formed a large area of tidal flats, and the suspended load has been deposited in the weak hydrodynamic environment (Wang et al., 2015). At the same time, in the bay, the grain size distribution of sediments is primarily controlled by the hydrodynamic force (the grain size distribution was deduced to be inversely proportional to the force); thus, the changes in hydrodynamic conditions will affect the distribution of sediments, resulting in erosion or deposition in the coastline and changes in the topography.
At the beginning of industrialization (1960s) in the Quanzhou Bay, Liaodong Bay, Hangzhou Bay, and other urban coastal zones, topography changes were primarily controlled by natural factors (Zhang and Zhang, 2004; Yan et al., 2017). Notably, natural factors are still an important factor affecting the topography of urban coastal zones. Excessive erosion of the coastline will lead to the failure of flood protection facilities in coastal cities and will thus, increase the risk of flood disasters in the city (Mohamed Rashidi et al., 2021).
Period From Early to Middle Stages of Industrialization
From early industrialization to mid-industrialization (1950s–1990s), human activity has become the main controlling factor of environmental evolution in the urban coastal zone. Notably, one of the reasons for the changes in topography has been the change in hydrodynamics in the region (Liu et al., 2014). The development of industrialization in urban coastal zones changes the shape of the coastline and the area of the sea, which leads to hydrodynamic changes (Yang and Chui, 2020). Specifically, coastal construction in urban coastal zones has transformed much of the natural coastline into an artificial coastline and changed the original hydrodynamic strength and direction (Chen et al., 2011; Ondoa et al., 2018), thereby affecting the development of the urban coastal zone topography. In the meantime, extensive reclamation projects have reduced the area of the urban coastal zone, caused a decrease in tidal influx, and weakened the tidal intensity in the area. By the 2000s, the tidal energy at the Luoyang River and south of Xiutu decreased by 64.1 and 16%, respectively (Wang, 2007). Notably, the construction of reservoirs and sluice gates in the upper reaches of the Jinjiang and Luoyang rivers reduced the runoff volume and weakened the influence of runoff on tidal movements (Ying et al., 2018). Moreover, the reclamation embankment constructed during the Wuyi Reclamation Project reduced the tidal current velocity and increased the sediment flux (Tan et al., 2021), resulting in deposits that are 6 m in thickness in the Houzhu Channel. Due to the weakening of the hydrodynamics in the inner bay, the bay mouth was primarily eroded due to ocean hydrodynamics, which caused the 20 m isobath to move landward.
Another factor that influenced topographic changes was the increased amount of sediments, which were primarily derived from river discharge and erosion along the urban coastal zone. In the early and middle stages of industrialization, massive deforestation and excavation in the mountains in the upstream areas have caused serious soil erosion (Wang et al., 2011). Based on hydrological data from the Anxi and Shilong stations located along the Jinjiang River basin, a significant increase in sediment concentration was found in the Jinjiang River between the 1950s and 1980s (Figure 7D). The sediments discharged by the Jinjiang River, combined with those discharged by the Luoyang River, were deposited in the estuaries and formed a widely tidal flat, whereas the suspended load of sediments deposited in the weak hydrodynamic environment of the Houzhu Channel resulted in the continuous deposition of tidal flats in the channel (to the west) (Liu et al., 2014). Meanwhile, the Dazhui channel was gradually silted up (Figure 5B), owing to weakening hydrodynamics and increasing sediment flux. In addition, during the construction of the reclamation project, some of the filling structures were rushed into the bay by the water flow, resulting in an increase in the sediment concentration in the coastal water. However, human activities also affect the distribution of sediments (Radhouan et al., 2021). Notably, the hydrodynamic force in the bay has decreased, resulting in the tidal hydrodynamics not being sufficient enough to transport the fine-grained sediments out of the Houzhu Channel; thus, the particles carried by currents were accumulated on the banks of both the channels (Figure 5A). The shoal in the channel expanded rapidly outward, resulting in the disappearance of a 10-m deep groove in the Houzhu Channel and a significant extension of the 0 m isobath toward the center of the channel, which destroyed the original balance of erosion and deposition in Quanzhou Bay.
During this period, there was a great demand for land in urban coastal zones (Yu et al., 2020). During this period, the reclamation area in Quanzhou Bay was the largest during the entire process of industrialization. A typical example of this is Osaka Bay, which has an area of approximately 160 km2 built over the centuries (Martín-Antón et al., 2020). During the period of industrialization, extensive reclamation activities in these urban coastal zones destroyed the original wetlands and freshwater resources. Additionally, sea reclamation caused the coastline length and sea area of the urban coastal zone to decrease, which in turn, affected the economic activity in the region (Guo et al., 2019). Most of the reclamation projects shorten the length and curvature of the coastline, resulting in a lower SIB (Figure 7B). Notably, with increased human activity, the natural coastline decreased, whereas the urban, port, and aquaculture coastlines increased. The AIB increased from 0.28 to 0.55, and the IBD increased from 0.27 to 0.49 (Table 5). An artificial coastline was also common in other industrial urban coastal zones in the nineteenth century, such as Maine Bay and New York Harbor (Köster et al., 2007; Guo et al., 2019). This indicates that, as the economy grows and the total reclaimed area increases, the levels of artificiality and development of coastlines gradually increase.
Period From Late Industrialization to Modern Society Era
From the later period of industrialization to the age of modern society (1990s–till date), the area of reclamation decreased. The topography changed primarily in the Houzhu Channel and northern Shihu. With the decrease in tidal hydrodynamic force and water exchange capacity, the venturi effect of the tidal channel between the inner and outer urban coastal zones weakened (Wang, 2011), which in turn, weakened the marine erosion in the Shoal and increased the deposition area. During this period, industrial and economic development along the coast altered the material composition of the sediments. Owing to the establishment of printing, dyeing, textile, and electroplating industries along the coast, the wastewater from the related industries and domestic sewage were discharged into the bay through sewage pipes or open channels, resulting in continuous increases in organic matter and heavy metal elements (e.g., Zn and Pb); this changes the physical composition of sediments in the Quanzhou Bay (Homira, 2021). Some ocean construction projects, such as sand mining and dredging, have also directly changed the seabed (Rahmawan et al., 2017). Since 2010, to maintain the use of the channel, dredging operations have been carried out in areas of the channel where deposition is severe and the water depth does not reach the required standard, resulting in artificially excavated channels in the Dazhui and North channels that are 10 and 5 m deep, respectively (Figures 5C,D). Simultaneously, the depositions in the channels facilitated the suspension and redistribution of surrounding sediments, which resulted in the deposition of the coastal sediments and periodic fluctuation in the terrain in the channel (Liu et al., 2014).
In addition to the changes in topography, the environment of Quanzhou Bay changed significantly during this period, because unplanned human activity (e.g., large-scale exploitation of sea sand, excessive discharge of industrial sewage, and excessive aquaculture) led to the gradual deterioration of the ecological environment in the urban coastal zones in the bay. Furthermore, with the increasing population in the reclaimed area and the rapid development of secondary and tertiary industries along the coast, the discharge of pollutants and organic matter into the bay has increased (Yan et al., 2020). Notably, the weakened hydrodynamics has limited the exchange of a large number of pollutants with seawater, affecting the dilution and diffusion of these pollutants, which has resulted in a significant increase in heavy metals and organic carbon in the bottom sediment (Yan et al., 2020). As a result, the water quality of the bay has deteriorated, and correspondingly, the environmental quality of the surrounding wetlands has declined significantly; this may have an impact on the cultural environment of the relevant marine organisms. Moreover, the large amount of coastal aquaculture has changed the pattern of water, sediment, and nutrient transport, which has, in turn, changed the natural environment where marine organisms live, resulting in the uneven distribution of hydrobionts and a decrease in the number of benthic organisms annually (Ge et al., 2021). Moreover, excessive aquaculture destroys the surrounding tidal flats and wetlands. Since the middle stage of industrialization, the aquaculture coastline in the coastal reclaimed areas has increased (Table 4), resulting in a large number of artificially excavated trench-ridge marks in the intertidal zone of the reclaimed area. This has completely destroyed the waterways of flood and ebb tides and sediment transport paths in the bay.
During this period, the reclamation works were large in number but small in area. Notably, during this period, the straight coastline area became complex and twisted again, resulting in an increase in the SIB (Figure 2 and Table 5). The change in coastline type was primarily due to the change in the land function of the reclamation area. Over the course of this duration, natural coastlines have been transformed into artificial coastlines, with the percentage of natural coastlines decreasing from 44 to 10% (Table 4). There are two main reasons for this change. First, with the increased demand for the shipping industry, the Shihu Port has been expanding toward the sea, and thus, the coastline of ports has increased. However, in the later stage of industrialization, aquaculture increased due to economic growth, and part of the city coastline was transformed into aquaculture areas. In the process of the transformation of the bay area into a modern society, formerly large-scale aquaculture areas increased, whereas small-scale aquaculture production ceased. Notably, the IBD increased at first and then, decreased because of the difference in pi of different coastlines. This change in coastline and shoreland land-use patterns indicates that human activity in urban coastal zones has changed with economic development. In the later stage of industrialization, we observed that the land use in the urban coastal zone was primarily cities and industrial land (Table 6). Compared with post-industrial Japan, the purpose of reclamation projects has changed from farmland to airports and residential buildings (Kitazume, 2012). In the modern society, 95% of the coastline in Tokyo Bay has been transformed from natural to artificial (Atsushi and Jota, 2020). In some developed countries, the transformation of old reclamation areas is now a focus of urban coastal zone development; for example, Spain has also included parts of the reclamation areas in old industrial areas in reconstruction planning (Nogués and Arroyo, 2015). Thus, through the comparison of different urban coastal zones, we were able to deduce that shoreland land-use changes with economic changes.
Stage-Specific Development Mechanism of Coupling Relationship Between Human Activity and Geographical Environment in Urban Coastal Zones
As observed through the changes of the urban coastal zone geomorphology, it was evident that there was a stage change in the geomorphology features, such as coastline, isobath, and shoreland land use. This phase of change in the urban coastal zone during the stage of industrialization has a certain relevance (Kakisina et al., 2015).
Before industrialization, the cities around the urban coastal zone were small and sparsely populated, mostly dominated by agriculture and small shipping businesses. With the construction of reclamation projects, the productivity of urban coastal zones increased, and the per capita income and consumption level also increased, which led to the upgrading of the demand structure (Wu, 2020). At the same time, the change in the demand structure led to the transformation of resources of the industrial sectors, with an increased consumer demand. Thus, the economic structure was transformed, and the land use pattern of the reclamation area changed (Figure 6A).
Economic development has led to the functional transformation of shoreland reclamation land, further causing changes in the industrial structure of the entire region (Li et al., 2018a; Qiu et al., 2021). During the whole process of the industrialization of the urban coastal zone in the bay, we observed two different patterns of reclamation (Figure 6B). From the initial stage of industrialization to the later stage of industrialization, the reclamation project increased the economic benefits of and development in the area, leading to the transformation of the regional economic industry. Furthermore, the transformation of the economic industry increased the demand for land and promoted new reclamation activities (Figure 6B). In modern society, the awareness of people regarding environmental protection has increased, and locals no longer undertake reclamation projects without definite plans. At the same time, the per capita GDP and economic output of the reclamation area indicated an increase year by year, but the percentage of economic efficiency in the area indicated a decrease (Figure 6A), which may be due to the increasing cost of resources for ecological restoration and environmental improvement.
Future Development and Planning of Urbanization in the Urban Coastal Zone
In the past few decades, many reclamation projects have been carried out around the urban coastal zones of China, owing to the increase in population and the demand for land (Li et al., 2018a). Due to large-scale reclamation projects, the shape and economic model of urban coastal zones have significantly changed and are extensively developed (Qiu et al., 2021). From the example of Quanzhou Bay, since the 1970s, the morphology of the urban coastal zones of China has changed rapidly; the proportion of natural coastline and the area of urban coastal zones have been decreasing, and regional differences have become more pronounced (Suo and Zhang, 2015). With the continuous intervention of human activity, most urban coastal zones have experienced different degrees of channel deposition and environmental pollution (Feng et al., 2015). The number of channels in urban coastal zones that have not reached the design baseline has also been increasing (data from the China Dredging Association, 2021). Without manual intervention, these channels would not fit the transport demand, which may affect the economic development of cities around the urban coastal zone. However, the quality of water in the urban coastal zone may continue to decline the increasing deposition in the bay, which is caused by the weakened hydrodynamics in the bay and decreased water exchange rate between the inner and outer seas (Deng et al., 2020; Li et al., 2020). This may affect the development of aquaculture and production of agriculture around urban coastal zones, hindering the development of coastal economy and industrial upgrading and transformation in the region. In the future, if unreasonable planning surrounds urban development policies, the urban coastal zone environment will deteriorate further.
At present, the exhaustion of resources in urban coastal zones is a widespread problem, which is a concern not only in China but also in other parts of the world, e.g., the Tokyo and New York bays (Atsushi and Jota, 2020; O’Neil et al., 2020). The environmental degradation of these urban coastal zones after industrialization has urged the local governments to invest considerable resources in environmental management (Maiolo et al., 2020). The ecological problems of urban coastal zones are gradually becoming important issues for countries globally, and thus, the focus in urban coastal zones has gradually changed from economic development to environmental restoration (Oyetibo et al., 2019).
The development of urban coastal zones is unstoppable. To avoid the negative effects from the resource development of urban coastal zones and the aggravation of the surrounding environmental problems, it is necessary to coordinate with the surrounding ecological environment during development. Therefore, development in such areas should focus on marine spatial planning for future development. In terms of pollutants in the sediments, the input of heavy metals and man-made organic matter into the bay should be reduced. For economically developed regions, large heavy industry enterprises should be upgraded to eliminate the industries that use old technologies to reduce the emissions of harmful pollutants (Choi et al., 2021). Therefore, it is necessary to use advanced clean production technologies and strong environmental protection measures in heavy industry production to minimize environmental pollution. For regions that are still in the middle of the industrialization stage, industrial production areas should be planned in advance while considering the carrying capacity of the urban coastal zone environment and increasing production (Shen et al., 2020). In terms of ecological restoration, an economically developed urban coastal zone should be able to repair the damaged ecology over time. For example, the “Regeneration Action Plan” that was first implemented in Tokyo Bay was executed in Japan and included in the United States Wetland Protection Law (Steiner et al., 1994; Tokunaga et al., 2020). During the development of urban coastal zones, we should consider adopting a development plan that has a balance of ecology and development (Chen et al., 2011). It is more important to formulate a regional development strategic plan and carry out the spatial layout in the future development of urban coastal zones, which can coordinate with the regional ecological environment. Notably, in accordance with the principle of ecological priority, it is necessary to protect the original ecological resources, such as wetlands and forests, as much as possible. When formulating an effective bay development policy, the Integrated Coastal Zone Management (ICZM) system should be established along with ecological restoration (Caviedes et al., 2020). Simultaneous with the development of urban coastal zones, parameters, such as environmental impact assessment and ecological index of urban coastal zones, must be introduced, to comprehensively evaluate the construction of urban coastal zones. At present, this indicator is also widely used in urban coastal zone assessments worldwide, which has improved the environment of urban coastal zones and reduced the risks posed by human activities to the ecology of these zones (Reija et al., 2021). However, in the future, we should also consider the different socio-economic development levels and geographical locations of each region and select appropriate indicators, to evaluate the development of urban coastal zones in light of the specific situations in the urban coastal zones. Furthermore, to promote harmonious development between human beings and the environment of urban coastal zones, we should restore the vegetation in the coastal area, construct the bay nature reserve, and strengthen the protection and utilization of coastal wetland resources and biological resources.
Notably, it is important to pre-plan the use and activities that affect the marine environment while paying attention to long-term policies oriented toward the conservation of ecosystem services provided by urban coastal zone ecosystems. Since 2020, a project called Blue Bay has been carried out in Quanzhou Bay to improve the ecology of its urban coastal zones, with a total investment of 351 million Chinese yuan (data from The Jinjiang Government, 2021). This project aims to protect the marine ecological environment through different measures, including the control of Spartina alterniflora (Loisel), restoration of the mangrove ecological zones, creation of bird habitat, and ecological transformation of the coast; thus, the wetland ecosystem in the protected area was gradually restored, the self-sustainability of urban coastal zone ecosystems was improved, biodiversity and ecological balance were maintained, and regional economic development was promoted. Thus, only timely measures can guarantee the future sustainable development of urban coastal zones.
Conclusion
In this study, we analyzed the topographic changes and conversion process of reclaimed land function in Quanzhou Bay, based on the coastline, water depth, and economic data. The results indicate that there are distinct spatial and temporal differences in coastline length and function in the urban coastal zones of the bay. Before industrialization, the coastline changes were dominated by natural evolution and remained stable overall. During the industrialization period, human activity was the main factor that influenced coastline changes in the bay, with a significant increase in development and artificiality. At the same time, the negative impact of human activity on the development of urban coastal zones also increased. In summary, the topographic changes observed in the urban coastal zones in the bay are the result of both natural and human activity, and the main factors include hydrodynamics, sediments, and human activity, among which the weakening of hydrodynamic force caused by the reclamation project and the increasing sediment flux are the main reasons for the changes in the seabed observed in the urban coastal zones. Moreover, the land use pattern of the reclaimed area was closely related to the development process of industrialization, and the entire process was divided into four stages. With the development of industrialization, land use patterns of the reclaimed areas gradually transformed from agriculture into industry, such as transportation and services of tertiary industries, finally transforming into areas of ecological and environmental construction and restoration. The economic benefits of reclaimed land gradually decreased, whereas the social benefits gradually increased. With the development of society, the development and utilization of coastal areas increased; this must be applied globally. Thus, the natural environment and economic structure around urban coastal zones will further change globally. Based on the factors affecting the evolution of urban coastal zones, the development of these zones in the future should be reasonably planned while controlling the number of reclamation projects, and the resources of the zones must be developed using effective ecological concepts. The findings of this study can provide decisions for the rational planning and economical use of resources to reduce the negative impact of human activities on the environmental development of urban coastal zones.
Data Availability Statement
The original contributions presented in the study are included in the article/supplementary material, further inquiries can be directed to the corresponding author/s.
Author Contributions
YhL conceived and designed the study, supervised the work, and co-wrote the manuscript. XX completed the extraction of bathymetric data from the chart, the calculation, and analysis of topographic change parameters and co-wrote the manuscript. FS and JH contributed to the analysis of the variation of water depth data and the interpretation of related parameter data. LW analyzed the cause of the change of sediment composition. XZ and WC collected and consolidated relevant economic data. YtL extracted the coastline information from satellite data and interpretation. BZ provided charts and explained the changes in the coastline. All authors contributed to the article and approved the submitted version.
Funding
This work was supported by the National Science Foundation of China (41976050) and the Scientific Research Foundation of the Third Institute of Oceanography, MNR (TIO2019028 and TIO2015014).
Conflict of Interest
The authors declare that the research was conducted in the absence of any commercial or financial relationships that could be construed as a potential conflict of interest.
Publisher’s Note
All claims expressed in this article are solely those of the authors and do not necessarily represent those of their affiliated organizations, or those of the publisher, the editors and the reviewers. Any product that may be evaluated in this article, or claim that may be made by its manufacturer, is not guaranteed or endorsed by the publisher.
References
Akan, Ç, McWilliams, J. C., and Uchiyama, Y. (2020). Topographic and coastline influences on surf Eddies. Ocean. Model. 147:101565. doi: 10.1016/j.ocemod.2019.101565
Aldasoro-Said, G., and Ortiz-Lozano, L. (2021). Marine resource dependence in rural coastal communities south of the Reef Corridor of the Southwest Gulf of Mexico. Ocean Coast. Manage. 211:105778. doi: 10.1016/j.ocecoaman.2021.105778
Atsushi, K., and Jota, K. (2020). Coastal urbanization alters carbon cycling in Tokyo Bay. Sci. Rep. 10:20413. doi: 10.1038/s41598-020-77385-4
Cao, C., Cai, F., Qi, H. S., Zheng, Y. L., and Lu, H. Q. (2021). Characteristics of underwater topography, geomorphology and sediment source in Qinzhou Bay. Water 13:1392. doi: 10.3390/w13101392
Cao, W. T., Zhou, Y. Y., Li, R., and Li, X. C. (2020). Mapping changes in coastlines and tidal flats in developing islands using the full time series of Landsat images. Remote Sens. Environ. 239:111665. doi: 10.1016/j.rse.2020.111665
Caviedes, V., Arenas-Granados, P., and Barragán-Muñoz, J. M. (2020). Regional public policy for integrated coastal zone management in Central America. Ocean Coast. Manage. 186:105114. doi: 10.1016/j.ocecoaman.2020.105114
Chen, B., Yu, W. W., Liu, W. H., and Liu, Z. H. (2011). An assessment on restoration of typical marine ecosystems in China-achievements and lessons. Ocean Coast. Manage. 57, 53–61. doi: 10.1016/j.ocecoaman.2011.11.007
Chen, K. L., Dong, H. Y., Jia, L. W., and He, Z. X. (2020). Depocentre transfer in the Lingdingyang estuary: interferencesInterferences from natural and anthropogenic forcings. Ocean Coast. Manage. 185:105064. doi: 10.1016/j.ocecoaman.2019.105064
China Dredging Association (2021). Available online at: http://www.chida.org/ (accessed March 23, 2021).
Choi, H. J., Cho, S. J., Hwang, T., Nam, J., and Hwang, C. S. (2021). Cumulative impact assessment for marine spatial planning: a case study of the Gyeonggi Bay in south Korea. J. Coast. Res. 114, 360–364. doi: 10.2112/jcr-si114-073.1
Darwish, K., Smith, S. E., Torab, M., Monsef, H., and Hussein, O. (2017). Geomorphological changes along the Nile Delta coastline between 1945 and 2015 detected using satellite remote sensing and GIS. J. Coast. Res. 33, 786–794. doi: 10.2112/jcoastres-d-16-00056.1
Day, J. W., Gunn, J. D., and Burger, J. R. (2021). Diminishing opportunities for sustainability of coastal cities in the Anthropocene: a review. Front. Env. Sci. 9:663275. doi: 10.3389/fenvs.2021.663275
Deng, G. F., Shen, Y. M., Li, C. P., and Tang, J. (2020). Computational investigation on hydrodynamic and sediment transport responses influenced by reclamation projects in the Meizhou Bay, China. Front. Earth. Sci. Prc. 14:493–511. doi: 10.1007/s11707-019-0758-8
Du, J. L., Yang, S. L., and Feng, H. (2016). Recent human impacts on the morphological evolution of the Yangtze River delta foreland: a review and new perspectives. Estuar. Coast. Shelf Sci. 181, 160–169. doi: 10.1016/j.ecss.2016.08.025
Fan, Y. F., Chen, X. L., Chen, Z. B., Zhou, X. X., Lu, X., and Liu, J. (2022). Pollution characteristics and source analysis of heavy metals in surface sediments of Luoyuan Bay, Fujian. Environ. Res. 203:111911. doi: 10.1016/j.envres.2021.111911
Feng, L., He, J., Ai, J. Y., Sun, X., Bian, F. Y., and Zhu, X. D. (2015). Evaluation for coastal reclamation feasibility using a comprehensive hydrodynamic framework: a case study in Haizhou Bay. Mar. Pollut. Bull. 100, 182–190. doi: 10.1016/j.marpolbul.2015.08.051
Feng, R. D., Wang, F. Y., and Wang, K. Y. (2021). Spatial-temporal patterns and influencing factors of ecological land degradation-restoration in Guangdong-Hong Kong-Macao Greater Bay Area. Sci. Total Environ. 794:148671. doi: 10.1016/j.scitotenv.2021.148671
Ge, B. M., Zhou, J., Yang, R. P., Jiang, S. H., Yang, L., and Tang, B. P. (2021). Lower land use intensity promoted soil macrofaunal biodiversity on a reclaimed coast after land use conversion. Agric. Ecosyst. Environ. 306:107208. doi: 10.1016/j.agee.2020.107208
Giosan, L., Constantinescu, S., Filip, F., and Deng, B. (2013). Maintenance of large deltas through channelization: nature vs. humans in the Danube delta. Anthropocene 1, 35–45. doi: 10.1016/j.ancene.2013.09.001
Guo, Q. D., Pu, R. L., Tapley, K., Cheng, J., Li, J. L., and Jiao, T. (2019). Impacts of coastal development strategies on long-term coastline changes: a comparison between Tampa Bay, USA and Xiangshan Harbor, China. Pap. Appl. Geogr. 5, 126–139. doi: 10.1080/23754931.2019.1654405
Homira, A. (2021). Ecological risk assessment of heavy metals in sediment, fish, and human hair from Chabahar Bay, Makoran, Iran. Mar. Pollut. Bull. 169:112345. doi: 10.1016/j.marpolbul.2021.112345
Huang, F. M., Huang, B. Q., Huang, J. L., and Li, S. H. (2018). Measuring land change in coastal zone around a rapidly urbanized bay. Int. J. Env. Res. Public Health 15:1059. doi: 10.3390/ijerph15061059
Jin, W. F., Zhou, C. S., and Luo, L. J. (2018). Impact of land input on economic growth at different stages of development in Chinese cities and regions. Sustainability 10:2847. doi: 10.3390/su10082847
Kakisina, T. J., Anggoro, S., Hartoko, A., and Suripin. (2015). Analysis of the impact of land use on the degradation of coastal areas at Ambon Bay-mollucas province Indonesia. Procedia Environ. Sci. 23, 266–273. doi: 10.1016/j.proenv.2015.01.040
Kitazume, M. (2012). Ground improvements in Haneda/Tokyo international airport expansion project. Proc. Inst. Civ. Eng.Ground. Improv. 165, 77–86. doi: 10.1680/grim.10.00031
Köster, D., Lichter, J., Lea, P. D., and Nurse, A. (2007). Historical eutrophication in a river-estuary complex in mid-coast Maine. Ecol. Appl. 17, 765–778. doi: 10.1890/06-0815
Li, G. D., Xu, K. H., Xue, Z. G., Liu, H. R., and Bentley, S. J. (2020). Hydrodynamics and sediment dynamics in Barataria Bay, Louisiana, USA. Estuar. Coast. Shelf Sci. 249:107090. doi: 10.1016/j.ecss.2020.107090
Li, J. T., Liu, Y. S., and Yang, Y. Y. (2018b). Land use change and effect analysis of tideland reclamation in Hangzhou Bay. J. Mt. Sci. 15, 394–405. doi: 10.1007/s11629-017-4542-5
Li, J. L., Pu, R. L., Yuan, Q. X., Liu, Y. C., Feng, B. X., Guo, Q. D., et al. (2018a). Spatiotemporal change patterns of coastlines in Xiangshan Harbor (Zhejiang, China) during the past 40 years. J. Coast. Res. 34, 1418–1428. doi: 10.2112/jcoastres-d-17-00150.1
Li, Z. J., and Wang, G. Y. (1984). The change of coast-line of the Jinjiang River mouth and the declination of Quanzhou Port (in Chinese with English abstract). Earth Sci. 3, 157–163.
Lin, Y. P. (2020). The Material “Source And Sink” Process Of Quanzhou Bay Under the Impact of Typhoon Matmo (2014) (in Chinese with English abstract). Academic thesis. Xiamen: Third Institute of Oceanography. doi: 10.27057/d.cnki.ggjhy.2020.000002
Lin, Y. P., Li, Y. H., Zheng, B. X., Yin, X. J., Wang, L., He, J., et al. (2019). Evolution of sedimentary organic matter in a small river estuary after the typhoon process: a case study of Quanzhou Bay. Sci. Total Environ. 686, 290–300. doi: 10.1016/j.scitotenv.2019.05.452
Liu, C. L., Chang, J., Chen, M. J., and Zhang, T. (2020). Dynamic monitoring and its influencing factors analysis of coastline in the Laizhou Bay since 1985. J. Coast. Res. 105, 18–22. doi: 10.2112/jcr-si105-004.1
Liu, H., Kang, H. X., and Yin, B. S. (2014). Sediment transport in the Quanzhou Bay. Adv. Mater. Res. 2914, 2388–2391.
Loh, P. S., Cheng, L. X., Yuan, H. W., Yang, L., Lou, Z. H., Jin, A. M., et al. (2018). Impacts of human activity and extreme weather events on sedimentary organic matter in the Andong salt marsh, Hangzhou Bay, China. Cont. Shelf Res. 154, 55–64. doi: 10.1016/j.csr.2018.01.005
Lubke, R. A. (1985). Erosion of the beach at St Francis Bay, Eastern Cape, South Africa. Biol. Conserv. 32, 99–127. doi: 10.1016/0006-3207(85)90080-1
Maloney, J. M., Bentley, S. J., Xu, K., Obelcz, J., Georgiou, I. Y., and Miner, M. D. (2018). Mississippi river subaqueous delta is entering a stage of retrogradation. Mar. Geol. 400, 12–23. doi: 10.1016/j.margeo.2018.03.001
Maren, D. S., Oost, A. P., Wang, Z. B., and Vos, P. C. (2016). The effect of land reclamations and sediment extraction on the suspended sediment concentration in the Ems Estuary. Mar. Geol. 376, 147–157. doi: 10.1016/j.margeo.2016.03.007
Martín-Antón, M. del Campo, J. M., Negro, V., and Frades, J. L. Moreno Blasco, L. J.Jiménez Verdejo, J. R. (2020). Land use and port-city integration in reclamation areas: a comparison between Spain and Japan. J. Coast. Res. 95, 278–282. doi: 10.2112/si95-054.1
Martínez, C., Quezada, M., and Rubio, P. (2011). Historical changes in the shoreline and littoral processes on a headland bay beach in central Chile. Geomorphology 135, 80–96. doi: 10.1016/j.geomorph.2011.07.027
Martino, G. D., Innangi, S., Sacchi, M., and Tonielli, R. (2021). Seafloor morphology changes in the inner-shelf area of the Pozzuoli Bay, Eastern Tyrrhenian Sea. Mar. Geophys. Res. 42, 1–15. doi: 10.1007/s11001-021-09434-0
Maiolo, M. Alvise Mel, R., and Sinopoli, S. (2020). A stepwise approach to beach restoration at Calabaia Beach. Water 12:2677. doi: 10.3390/w12102677
Mohamed Rashidi, R. A. H., Jamal, M. H., Hassan, M. Z., Mohd, S. S. S., Mohd, S. S. L., and Abd, H. M. R. (2021). Coastal structures as beach erosion control and sea level rise adaptation in Malaysia: a review. Water 13:1741. doi: 10.3390/w13131741
Mossa, J., Chen, Y. H., Walls, S. P., Kondolf, G. M., and Wu, C. Y. (2017). Anthropogenic landforms and sediments from dredging and disposing sand along the Apalachicola River and its floodplain. Geomorphology 294, 119–134. doi: 10.1016/j.geomorph.2017.03.010
Naimi, A., and Muhanna, S. (2021). Economic diversification trends in the gulf: the case of Saudi Arabia. Circ. Econ. Sustain. 28, 1–10. doi: 10.1007/s43615-021-00106-0
Nogués, S., and Arroyo, N. L. (2015). Alternative approach to prioritization of Brownfield reclamation attending to urban development potentialities: case study in a depressed industrial district in Northern Spain. J. Urban Plan. Dev. 142:05015002. doi: 10.1061/(asce)up.1943-5444.0000272
Oiwane, H., Tonai, S., Kiyokawa, S., Nakamura, Y., Suganuma, Y., and Tokuyama, H. (2011). Geomorphological development of the Goto Submarine Canyon, northeastern East China Sea. Mar. Geol. 288, 49–60.
Ondoa, G. A., Onguéné, R., Eyango, M. T., Duhaut, T., Mama, C., Angnuureng, D. B., et al. (2018). Assessment of the evolution of Cameroon coastline: an overview from 1986 to 2015. J. Coast. Res. 81, 122–129. doi: 10.2112/si81-016.1
O’Neil, J. M., Newton, R. J., Bone, E. K., Birney, L. B., Green, A. E., Merrick, B., et al. (2020). Using urban harbors for experiential, environmental literacy: case studies of New York and Chesapeake Bay. Reg. Stud. Mar. Sci. 33:100886. doi: 10.3389/10.1016/j.rsma.2019.100886
Oyetibo, G. O., Miyauchi, K., Huang, Y., Ohtsubo, W. I., Chien, M. F., Ilori, M. O., et al. (2019). Comparative geochemical evaluation of toxic metals pollution and bacterial communities of industrial effluent tributary and a receiving estuary in Nigeria. Chemosphere 227, 638–646. doi: 10.1016/j.chemosphere.2019.04.048
Pourkerman, M., Marriner, N., Morhange, C., Djamali, M., Spada, G., Amjadi, S., et al. (2020). Geoarchaeology as a tool to understand ancient navigation in the northern Persian Gulf and the harbour history of Siraf. J. Archaeol. Sci. Rep. 33:102539. doi: 10.1016/j.jasrep.2020.102539
Qiu, L. F., Zhang, M., Zhou, B. B., Cui, Y. Z., Yu, Z. L., Liu, T., et al. (2021). Economic and ecological trade-offs of coastal reclamation in the Hangzhou Bay. China Ecol. Indic. 125:107477. doi: 10.1016/j.ecolind.2021.107477
Quanzhou Statistical Information Network (2021). Information Statistics. Available online at: http://tjj.quanzhou.gov.cn/tjzl/tjsj/ (accessed January 10, 2021).
Radhouan, E. Z., Lamia, Y., Takwa, W., Sylvie, C., Michel, G., Lamjed, M., et al. (2021). Surface sediment enrichment with trace metals in a heavily human-impacted lagoon (Bizerte Lagoon, Southern Mediterranean Sea): spatial distribution, ecological risk assessment, and implications for environmental protection. Mar. Pollut. Bull. 169:112512. doi: 10.1016/j.marpolbul.2021.112512
Rahmawan, G. A., Husrin, S., and Prihantono, J. (2017). Bathymetry changes analysis in serang district waters caused by seabed sand exploitation. E J. Ilmu dan Teknol. Kelautan Tropis 9, 45–55. doi: 10.29244/jitkt.v9i1.17916
Reija, H., Asko, I., Tarmo, P., Anne, K., Anu, P., Maila, K., et al. (2021). Data integration and participatory process in developing integrated coastal zone management (ICZM) in the northern Baltic Sea. J. Coast. Conserv. 25:47. doi: 10.1007/s11852-021-00833-4
Shen, H., Bi, K., Gao, Y., and Wang, M. (2020). How does the traditional heavy industry use ecotechnology to achieve the ecological innovation goal? Analysis based on expert survey in China’s shipbuilding industry. Sustainability 12:6624. doi: 10.3390/su12166624
Song, Y., Li, D., and Hou, X. Y. (2019). Characteristics of mainland coastline changes in Southeast Asia during the 21st Century. J. Coast. Res. 36, 261–275. doi: 10.2112/jcoastres-d-19-00018.1
Steiner, F., Pieart, S., Cook, E., Rich, J., and Coltman, V. (1994). State wetlands and riparian area protection programs. Environ. Manage. 18, 183–201. doi: 10.1007/bf02393761
Styles, R., Brown, M., Brutsché, K., Li, H. H., Beck, T., and Sánchez, A. (2016). Long-term morphological modeling of Barrier Island tidal inlets. J. Mar. Sci. Eng. 4:5. doi: 10.3390/jmse4040065
Sun, X., Zhang, L., Lu, S. Y., Tan, X. Y., Chen, K. L., Zhao, S. Q., et al. (2020). A new model for evaluating sustainable utilization of coastline integrating economic output and ecological impact: a case study of coastal areas in Beibu Gulf, China. J. Clean. Prod. 271:122423. doi: 10.1016/j.jclepro.2020.122423
Sun, Z., and Niu, X. (2021). Variation tendency of coastline under natural and anthropogenic disturbance around the abandoned Yellow River Delta in 1984–2019. Remote Sens. 13:3391. doi: 10.3390/rs13173391
Suo, A. N., and Zhang, M. H. (2015). Sea areas reclamation and coastline change monitoring by remote sensing in coastal zone of Liaoning in China. J. Coast. Res. 73, 725–729. doi: 10.2112/si73-124.1
Tan, Z. H., Chen, H. B., Xu, Y. N., and Guan, N. (2021). Analysis of hydrodynamics and sediment conditions around East Coast Sea Area in Bay of Bengal. IOP Conf. Ser. Earth Environ. Sci. 621:012081. doi: 10.2112/jcoastres-d-15-00194.1
The Jinjiang Government (2021). Available online at: http://www.jinjiang.gov.cn/ (accessed August 20, 2021).
Tokunaga, K., Sugino, H., Nomura, H., and Michida, Y. (2020). Norms and the willingness to pay for coastal ecosystem restoration: a case of the Tokyo Bay intertidal flats. Ecol. Econ. 169:106423. doi: 10.1016/j.ecolecon.2019.106423
U.S. Census Bureau (2021). Demographic Data. Available online at: https://www.census.gov/data.html (accessed October 20, 2021).
Wang, A. J. (2007). Impact of human activities on depositional process of tidal flat in Quanzhou Bay of China. Chin. Geogr. Sci 17, 265–269. doi: 10.1007/s11769-007-0265-9
Wang, A. J. (2011). Hydrodynamics and associated sediment transport over coastal wetlands in Quanzhou Bay, China. China Ocean Eng. 25, 59–72. doi: 10.1007/s13344-011-0005-x
Wang, C., Sun, Q., Jiang, S., and Wang, J. K. (2011). Evaluation of pollution source of the bays in Fujian Province. Procedia Environ. Sci. 10, 685–690. doi: 10.1016/j.proenv.2011.09.110
Wang, J., Cao, Y. C., Liu, H. M., and Gao, Y. J. (2015). Formation conditions and sedimentary model of over-flooding lake deltas within continental lake basins: an example from the paleogene in the Jiyang Subbasin, Bohai Bay Basin. Acta Geol. Sin. Eng. 89, 270–284. doi: 10.1111/1755-6724.12410
Wu, M. R. (2020). Measurement of regional industrial ecological efficiency in China and an analysis of its influencing factors. J. World Econ. Res. 9, 43–50. doi: 10.11648/j.jwer.20200901.16
Yan, X., Hu, Y., Chang, Y., Zhang, D., Liu, M., Guo, J., et al. (2017). Monitoring wetland changes both outside and inside reclamation areas for coastal management of the northern Liaodong Bay, China. Wetlands 37, 885–897. doi: 10.1007/s13157-017-0922-4
Yan, Y., Han, L., Yu, R. L., Hu, G. R., Zhang, W. F., Cui, J. Y., et al. (2020). Background determination, pollution assessment and source analysis of heavy metals in estuarine sediments from Quanzhou Bay, southeast China. Catena 187:104322. doi: 10.1016/j.catena.2019.104322
Yang, Y., and Chui, T. F. M. (2020). The role of the Pearl River flow in deep bay hydrodynamics and potential impacts of flow variation and land reclamation. J. Hydro Environ. Res. 34, 1–10. doi: 10.1016/j.jher.2020.11.001
Ying, C., Li, R. J., Li, X. W., and Liu, Y. (2018). Anthropogenic influences on the tidal prism and water exchange in Yueqing Bay, Zhejiang, China. J. Coast. Res. 85, 961–965. doi: 10.2112/si85-193.1
Yoon, H. H., Chun, S. S., and Hong, S. H. (2020). Rapid change in sedimentary facies from wave-to tide-dominated macrotidal flat in the Sinduri Bay, west coast of Korea. J. Coast. Res. 95, 728–732. doi: 10.2112/si95-142.1
Yu, X., Zhang, Z., Feng, A., Gu, D., Zhang, R., Xia, P., et al. (2020). Recent history of metal contamination in the Fangcheng Bay (Beibu Gulf, South China) utilizing spatially-distributed sediment cores: Responding to local urbanization and industrialization. Mar. Pollut. Bull. 158:111418. doi: 10.1016/j.marpolbul.2020.111418
Zarzuelo, C., Ruiz, A. L., and Sánchez, M. O. (2021). The role of waves and heat exchange in the hydrodynamics of multi-basin bays: the example of Cádiz Bay (Southern Spain). J. Geophys. Res. Oceans 126:e2020JC016346. doi: 10.1029/2020jc016346
Zhang, L. P., and Zhang, M. X. (2004). Spatial and temporal evolution of eroding coast in Hangzhou Bay, China. J. Coast. Res. 37, 67–74.
Keywords: human activity, geomorphology, coupling relationship, stage development, urban coastal zone, reclamation land, Quanzhou Bay
Citation: Xiao X, Li Y, Shu F, Wang L, He J, Zou X, Chi W, Lin Y and Zheng B (2022) Coupling Relationship of Human Activity and Geographical Environment in Stage-Specific Development of Urban Coastal Zone: A Case Study of Quanzhou Bay, China (1954–2020). Front. Mar. Sci. 8:781910. doi: 10.3389/fmars.2021.781910
Received: 23 September 2021; Accepted: 08 December 2021;
Published: 07 January 2022.
Edited by:
Johannes Karstensen, GEOMAR Helmholtz Center for Ocean Research Kiel, Helmholtz Association of German Research Centres (HZ), GermanyReviewed by:
Maria De Andres, University of Cádiz, SpainDaniel Rittschof, Duke University, United States
Copyright © 2022 Xiao, Li, Shu, Wang, He, Zou, Chi, Lin and Zheng. This is an open-access article distributed under the terms of the Creative Commons Attribution License (CC BY). The use, distribution or reproduction in other forums is permitted, provided the original author(s) and the copyright owner(s) are credited and that the original publication in this journal is cited, in accordance with accepted academic practice. No use, distribution or reproduction is permitted which does not comply with these terms.
*Correspondence: Binxin Zheng, emhlbmdiaW54aW5AdGlvLm9yZy5jbg==