- 1College of Animal Sciences, Zhejiang University, Hangzhou, China
- 2Ocean Academy, Zhejiang University, Zhoushan, China
- 3Ocean College, Zhejiang University, Zhoushan, China
- 4School of Veterinary Science, The University of Queensland, Gatton, QLD, Australia
Single-cell proteins are attracting growing attention as viable alternatives for fishmeal (FM) in aquatic feed. Methanotroph (Methylococcus capsulatus, Bath) bacteria meal FeedKind® (FK) is a type of single cell protein with high protein content (75.14%) and desirable amino acids profile, produced by Methylococcus capsulatus (Bath) living on methane consumption. The present study evaluated the potential of replacing FM with FK in the diet of black sea bream (Acanthopagrus schlegelii). Five iso-energetic and iso-nitrogenous diets were designed with FK replacing 0, 4.13, 8.27, 16.53, and 24.80% FM protein in the basal diet (40% FM content), respectively. All the diets were fed to three replicates of fish (initial weight 6.56 ± 0.02 g) for 70 days. After the feeding trial, replacing dietary 8.27% FM protein with FK significantly improved the weight gain and specific growth rate of fish (P < 0.05), while other groups showed no significant difference in the growth performance (P > 0.05). The fish fed diets with 8.27 and 16.53% replacement levels exhibited significantly increased feeding rates. The 8.27% FK diet significantly increased the whole-body and muscle crude protein contents, apparent digestibility of crude lipid, foregut, and midgut amylase activities. The microvillus density in the midgut of fish fed the 24.80% FK diet significantly increased. The diet with 8.27% FK increased the serum triglyceride content of the fish, while the 24.80% FK diet reduced the serum triglyceride, total cholesterol, and low-density lipoprotein cholesterol contents of the fish. In conclusion, the results indicated that replacing dietary FM protein with up to 24.80% FK had no adverse effects on the growth of black sea bream, whilst replacing 8.27% FM protein with FK enhanced its growth performance and feed utilization.
Introduction
Meals obtained from animal products such as fish, cattle, and poultry are readily available and provide a variety of nutritional profiles for farmed aquatic animals. Marine proteins, such as those derived from fish, shrimp, and squid, have superior nutritional values, but their production has raised ecological and economic concerns, especially fish meal (FM) (Olsen and Hasan, 2012; Gamboa-Delgado and Márquez-Reyes, 2018; Kim et al., 2019). Plant meals are the most commonly used protein sources to replace animal proteins in feed (Kaushik et al., 2004; De Francesco et al., 2007; Cruz-Suárez et al., 2009). However, inherent characteristics of plant meals, such as the presence of anti-nutritional factors and lack of some essential amino acids (e.g., methionine, lysine), have either limited their use or required extra processing and costs (Francis et al., 2001; Gatlin et al., 2007; Miao et al., 2018). Among the unconventional sources of nutrients that have been intensely studied, single-cell protein (SCP), a bulk of dried cells that can also be termed as bioprotein, microbial protein or biomass, including microalgae, yeast, fungal, and bacterial proteins (BP), are attracting growing attention as sustainable protein sources for substituting animal- and plant-derived ingredients in aquafeeds (Anupama and Ravindra, 2000; Sánchez-Muros et al., 2014; Henry et al., 2015; Adeoye et al., 2021; Alloul et al., 2021; Jannathulla et al., 2021; Maulu et al., 2021).
In addition to high protein content (60–82%, dry matter), SCPs contain amino acid profiles similar to FM and provide fatty acids, nucleic acids, vitamins, and minerals that can support the growth and normal physiological functions of aquatic animals (Matassa et al., 2016; Gamboa-Delgado and Márquez-Reyes, 2018; Wang et al., 2020a). BPs generally contains higher methionine content (up to 3%) than algal or fungal SCPs (Erdman et al., 1977; Anupama and Ravindra, 2000). Many studies with BPs have been conducted on white leg shrimp (Penaeus vannamei) (Tlusty et al., 2017; Hamidoghli et al., 2019; Alloul et al., 2021), Florida pompano (Trachionotus carolinus) (Rhodes et al., 2015), tilapia (Oreochromis niloticus) (Maulu et al., 2021), Atlantic salmon (Salmo salar) (Storebakken et al., 2004; Berge et al., 2005; Aas et al., 2006a; Romarheim et al., 2011), rainbow trout (Oncorhynchus mykiss) (Perera et al., 1995b; Aas et al., 2006b; Øverland et al., 2006; Hardy et al., 2018), Atlantic halibut (Hippoglossus hippoglossus) (Aas et al., 2007), Japanese yellowtail (Seriola quinqueradiata) (Biswas et al., 2020), and African catfish (Clarias gariepinus) (Adeoye et al., 2021). Although based on different feed formulas, they have demonstrated that various BPs could partially or even wholly replace FM or soybean meal (SBM) in the diet without adverse effects on the growth performance or health status of various aquatic species.
FeedKind® (FK) (Calysta, Inc., Menlo Park, CA, United States) is a BP product derived from Bath. It is produced by continuous aerobic fermentation of the bacteria with methane as the sole carbon and energy source in a proprietary fermenter. The harvested biomass is subsequently centrifuged, heat inactivated, and spray dried (Biswas et al., 2020). FeedKind® has high contents of crude protein and lipid, with a well-balanced amino acids profile comparable to the FM (Table 1). Biswas et al. (2020) found that FK could replace 30% of the dietary FM protein without impacting the growth performance or feed efficiency of Japanese yellowtail.
As a popular aquaculture species in Southeast Asia, black sea bream (Acanthopagrus schlegelii) is adaptive to the intensive aquaculture on account of its characteristics of fast growth rate, high disease resistance, and tolerance to a wide range of environment (Hong and Zhang, 2003; Wang et al., 2020b). Previous study on the diet of black sea bream showed that Clostridium autoethanogenum protein (CAP) could replace FM up to 58.20% without adverse effects on the growth performance, antioxidative status, and digestive enzymes activities (Chen et al., 2020). It showed a higher possible replacement level than that in largemouth bass (Micropterus salmoides) (150 g/kg) (Yang et al., 2021). Meanwhile, there is still limited knowledge on how dietary BPs may affect black sea bream. The purpose of this study was to evaluate the potential of using FK as an alternative for FM in the diet of black sea bream based on the growth performance, feed utilization, digestive enzymes activities, intestinal and hepatic histology, and serum biochemical and antioxidative/oxidative parameters.
Materials and Methods
Experimental Diets
The methanotroph bacteria meal (FeedKind®, FK) was provided by Calysta, Inc., Menlo Park, CA, United States. Five isonitrogenous (44.8% crude protein) and isoenergetic (21 kJ/g gross energy) diets were formulated with FM protein substituted by graded levels of FK protein at 0 (FK0), 4.13 (FK4.13), 8.27 (FK8.27), 16.53 (FK16.53), and 24.80% (FK24.80) (Table 2). Crystalline DL-methionine, L-lysine, and L-arginine were added to maintain sufficient and balanced levels of these essential amino acids, according to the recommended levels from previous studies on black sea bream (Zhou et al., 2010a,b, 2011a,2011b). Taurine was added according to the recommended level by Tong et al. (2020). Non-essential amino acids were added to balance the protein level. Ca(H2PO4)2 was added to meet the available phosphorus contents required in black sea bream (Shao et al., 2008). The yttrium oxide (Y2O3) was supplemented at 0.1% for determining apparent digestibility.
After pulverizing and sifting through a 178-μm sieve, all the solid ingredients were weighed before mixing thoroughly with the lipid ingredients. The mixture was pelletized into 2.5-mm-diameter pellets using a pelletizer (Modle HKJ-218; Huarui, Wuxi, China). The pellets were then steamed for 10 min, dried for 72 h at 24°C before being stored at –20°C for subsequent feeding.
Experimental Fish and Feeding Trial
Black sea bream was provided by a fish farm (Zhoushan, China). The feeding trial was conducted in the Xixuan Fishery Science and Technology Island (Zhoushan, China). Fish were acclimated to the experimental conditions for 2 weeks in a plastic pond (5 m × 3 m × 1 m) before the feeding trial. After acclimation, 450 fish (6.56 ± 0.02 g) were randomly selected and divided into 15 fiberglass tanks. Each treatment included three replicates with 30 fish in each tank (filled with 420 L of water). Sand filtrated seawater was supplied to all the tanks at 2 L/min. The tanks were kept under natural photoperiod and continuously aerated with air stones. Water temperature was maintained at 26 ± 2°C; salinity, 27 ± 1 g/L; pH, 7.7 ± 0.1; and dissolved oxygen ≥ 5 mg/L. The fish were fed to apparent satiation two times daily at 08:00 and 16:00 for 70 days. Feces were removed 2 h after each feeding session.
Sampling
Before the feeding experiment, 30 fish were randomly collected and stored at −20°C for determining the initial whole-body crude protein content. From the 8th week, the fish feces were collected before 07:00 every day following the method of Wang et al. (2020c). Briefly, after siphoning, the feces were precipitated, filtrated, and finally collected in sealed bags and stored at −20°C for determining apparent digestibility coefficient. After the last feeding, all the fish were fasted for 24 h, anaesthetized with MS-222 (60 mg/L), and individually measured for final body weight and length. Five fish from each tank were randomly selected and preserved at −20°C for whole-body proximate composition analysis. Pooled blood was drawn from the caudal vein of the rest of the fish with 1 ml syringes. The blood samples were settled at 4°C for 2 h before being centrifuged at 10,000 g for 15 min to get the serum for biochemical analyses. Subsequently, ten fishes were dissected on ice to orderly separate the viscera, liver, and intraperitoneal fat, and then, weighed for calculating the somatic indexes. The dorsal muscle was removed for proximate composition analysis. The gastrointestinal tract was divided into stomach, foregut, midgut, and hindgut for determining the respective digestive enzyme activities. All the samples were stored at −20°C until analyses. Histological samples from the liver and midgut were collected from three fishes per tank, separated into two small parts, then respectively, fixed in 10% formalin and 2.5% glutaraldehyde solution (4°C).
Proximate Compositions
The proximate compositions of experimental feed, fish whole-body, muscle, and feces were determined following the standard protocols of the Association of Official Analytical Chemists (AOAC, 1995). Moisture was determined by drying the sample to constant weight at 105°C in an oven. Crude protein content was determined by Kjeldahl method (N × 6.25), and crude lipid content was determined by Soxhlet extraction method with diethyl ether. Ash content was determined by combusting the sample at 550°C for 8 h in a muffle furnace. The amino acid compositions of FM, FK, and fish feces were assayed by an automatic amino acid analyzer (Hitachi L-8900, Tokyo, Japan) after acid hydrolysis. The feed and fecal samples were dried, ground, and digested with acid. After filtration and dilution, the yttrium (Y) content was determined by an inductively coupled plasma mass spectrometer (PerkinElmer ELAN DRC-e, Waltham, MA, United States).
Biochemical Assays
The supernatants of the stomach and intestine samples were obtained following the procedure of Zhou et al. (2020). Briefly, the tissues were homogenized in 9 vol (v/w) of 0.86% physiological saline. The homogenate was then centrifuged at 2,500 g for 10 min at 4°C before collecting the supernatants, which were used to determine the digestive enzymes activities. The serum was used to assay the contents of glucose (GLU), triglyceride (TG), total cholesterol (T-CHO), high density lipoprotein cholesterol (HDL-C), low density lipoprotein cholesterol (LDL-C), and malondialdehyde (MDA), as well as the activities of glutamic pyruvic transaminase (GPT), glutamic oxalacetic transaminase (GOT), superoxide dismutase (SOD), and glutathione peroxidase (GSH-Px). The biochemical assays of serum and tissues were determined using assay kits purchased from Nanjing Jiancheng Bioengineering Institute (Nanjing, China) according to the instructions of the manufacturer.
Histological Analysis of Midgut and Liver
After serial dehydration in graded alcohol, the midgut and liver tissues fixed in 10% formalin were embedded in paraffin and sectioned to 5-μm thickness. The slices were then subjected to hematoxylin and eosin (H&E) staining, and observed under a light microscope (Olympus BX61, Tokyo, Japan). After fixing with 2.5% glutaraldehyde for more than 4 h, the midgut samples were postfixed with 1% OsO4 for 1.5 h. After double fixation, the samples were first dehydrated by graded ethanol, and then, dried in Hitachi Model HCP-2 critical point dryer (Tokyo, Japan). The dehydrated samples were coated with gold-palladium (Hitachi Model E-1010 ion sputter, Tokyo, Japan), and observed in scanning electron microscope (Hitachi Model SU-8010 SEM, Tokyo, Japan). The villus height and microvillus density were measured using Image-Pro Plus 6.0 software. The number of microvilli per unit area was counted in five randomly selected non-overlapping fields of view (Wang Y. et al., 2019).
Statistical Analysis
All the data were processed by IBM SPSS Statistics 24.0 and presented as mean ± standard error (SEM). Levene’s test was used to determine the normality and homogeneity of variances. Independent-sample Kruskal-Wallis test followed by Bonferroni adjust was performed when data were not homogeneous. A one-way analysis of variance (ANOVA) followed by Duncan multiple-range test was performed to determine the statistically significant differences among different groups. The significance level was set as P < 0.05.
Results
Growth Performance and Feed Utilization
As shown in Table 3, no significant differences were found in survival rate (SR), condition factor (CF) (ANOVA, F4,10 = 1.561, P = 0.258), viscerosomatic index (VSI) (ANOVA, F4,10 = 0.650, P = 0.640), hepatosomatic index (HSI) (ANOVA, F4,10 = 0.456, P = 0.766), and intraperitoneal fat ratio (IFR) (ANOVA, F4,10 = 1.072, P = 0.420) of black sea bream among all the treatments. With the replacement level of FM by dietary FK increasing, the weight gain (WG) and specific growth rate (SGR) of fish initially increased, and then decreased after 8.27% replacement level. The WG (ANOVA, F4,10 = 103.298, P = 0.000) and SGR (ANOVA, F4,10 = 101.470, P = 0.000) of fish in the FK8.27 group were significantly higher than the FK0 group, but no significant differences were obtained among the other FK inclusion groups and the FK0 group. Compared with the FK0 group, the fish in the FK8.27 and FK16.53 groups showed a significantly increased feeding rate (FR) (ANOVA, F4,10 = 2.481, P = 0.088). The feed conversion rate (FCR) (Kruskal-Wallis, F4,10 = 5.284, P = 0.259), protein efficacy ratio (PER) (ANOVA, F4,10 = 1.141, P = 0.384), and protein productive value (PPV) (ANOVA, F4,10 = 0.185, P = 0.942) among all the groups showed no significant differences.
Whole-Body and Muscle Proximate Composition
As shown in Table 4, the whole-body crude protein content of fish in the FK8.27 group was significantly higher than other groups (ANOVA, F4,10 = 6.764, P = 0.005). Compared with the FK0 group, the whole-body ash content was significantly lower in the FK inclusion groups (ANOVA, F4,10 = 7.650, P = 0.004). Moisture (ANOVA, F4,10 = 1.615, P = 0.234) and crude lipid contents (Kruskal-Wallis, F4,10 = 5.681, P = 0.224) in the whole body of the fish were not significantly affected by dietary FK inclusion. Moisture in the muscle of the fish in the FK24.80 group was significantly higher than other groups (ANOVA, F4,10 = 7.262, P = 0.005). A significant difference was observed in the muscle crude protein content of fish between the FK8.27 and FK0 groups (Kruskal-Wallis, F4,10 = 11.732, P = 0.019). Replacing 24.80% FM protein with FK in the diet significantly decreased muscle crude lipid content than in other treatments (ANOVA, F4,10 = 11.267, P = 0.000).
Apparent Digestibility and Gastrointestinal Digestive Enzyme Activities
As listed in Table 5, the ADC of dry matter and crude lipid increased with increasing dietary FK protein replacement level to 8.27% then, decreased. The ADC of dry matter (ANOVA, F4,10 = 1.805, P = 0.198), and crude lipid (ANOVA, F4,10 = 2.955, P = 0.075) in the FK8.27 group were significantly higher than in the FK24.80 and FK0 groups, respectively. There was no significant difference in the ADC of crude protein among all the groups (Kruskal-Wallis, F4,10 = 3.523, P = 0.474).
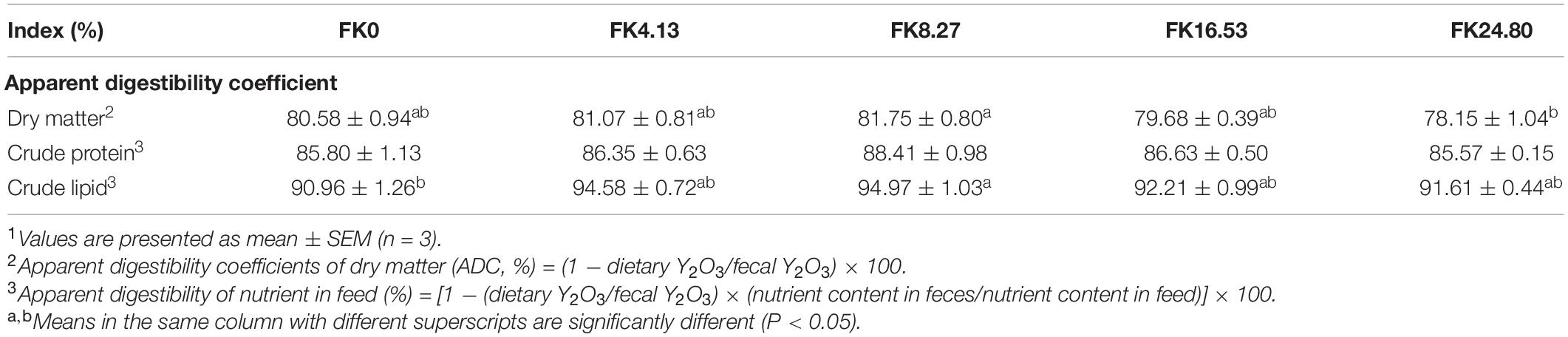
Table 5. Apparent digestibility coefficients of dry matter, protein, and lipid of black sea bream fed with different diets1.
The digestive enzyme activities in the gastrointestinal tract of all the treatments are presented in Table 6. Compared with the FK24.80 group, the FK8.27, and FK16.53 groups exhibited enhanced trypsin (ANOVA, F4,10 = 3.292, P = 0.030) and lipase (ANOVA, F4,10 = 2.970, P = 0.042) activities in the foregut. The foregut amylase activity in the FK8.27 and FK16.53 groups was also significantly higher than the other groups (ANOVA, F4,10 = 6.995, P = 0.001). The midgut amylase activity in the FK8.27 group was significantly higher than in the FK0 group (ANOVA, F4,10 = 1.557, P = 0.217), but no significant differences were found in the midgut trypsin (ANOVA, F4,10 = 0.359, P = 0.835) and lipase activities (ANOVA, F4,10 = 0.458, P = 0.765) among the treatments. The digestive enzyme activities in the stomach and hindgut were not significantly affected by dietary FK inclusion.
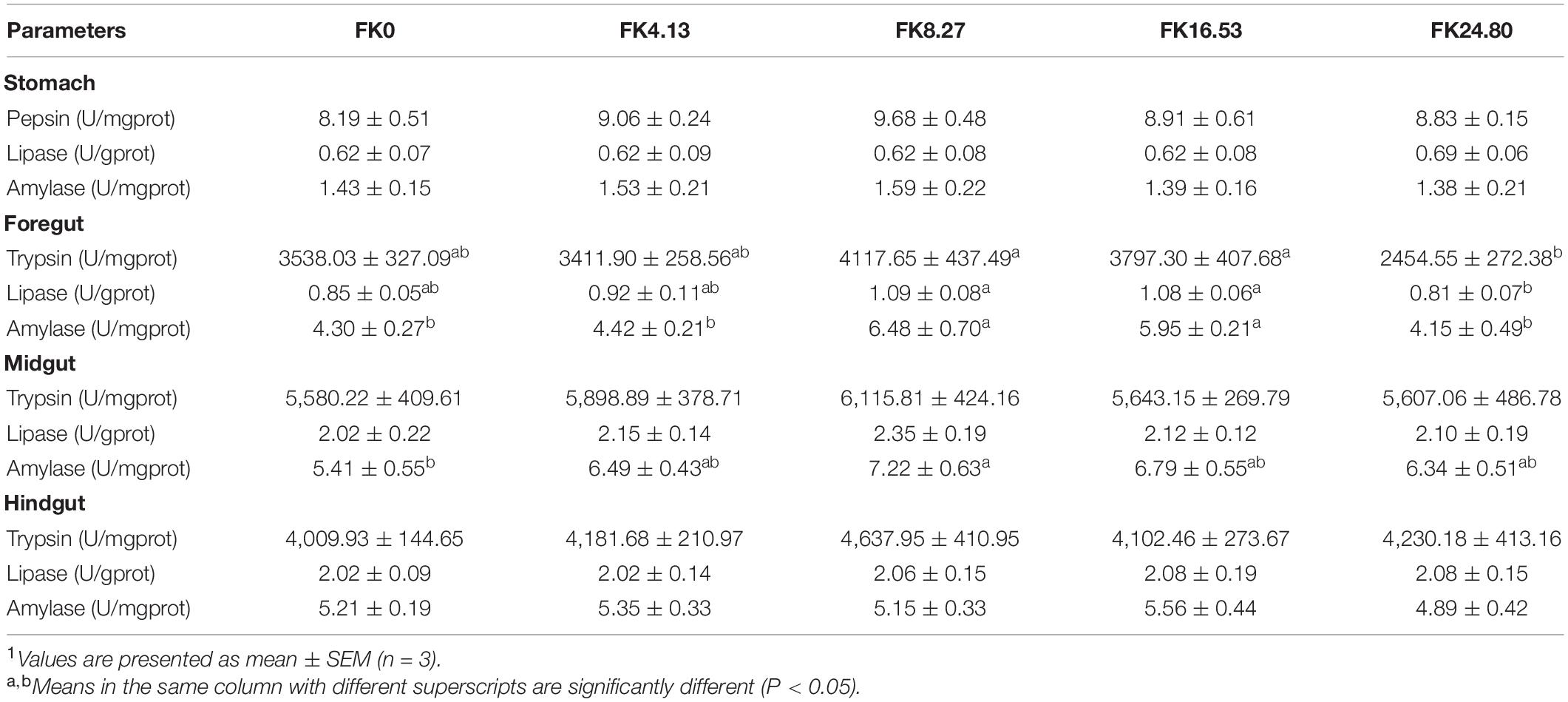
Table 6. Digestive enzyme activities in gastrointestinal tract of black sea bream fed with different diets1.
Serum Biochemical and Antioxidative/Oxidative Parameters
The effects of dietary FK on serum biochemical and antioxidative/oxidative parameters of black sea bream are listed in Table 7. The serum TG concentration increased with increasing dietary FK protein replacement level to 8.27%, then decreased (ANOVA, F4,10 = 10.405, P = 0.000). The serum T-CHO content of fish in the FK24.80 group was lower than in the FK0, FK4.13, and FK8.27 groups (ANOVA, F4,10 = 2.819, P = 0.048). Replacing 24.80% FM protein with FK in the diet significantly decreased the serum LDL-C level (Kruskal-Wallis, F4,10 = 12.323, P = 0.015). The serum GPT activity of fish in the FK8.27 group was significantly lower than in the FK24.80 group (ANOVA, F4,10 = 2.752, P = 0.055). No significant differences in glucose (ANOVA, F4,10 = 0.927, P = 0.472), HDL-C (Kruskal-Wallis, F4,10 = 3.504, P = 0.477), and MDA contents (ANOVA, F4,10 = 0.280, P = 0.888), as well as GOT (ANOVA, F4,10 = 0.435, P = 0.781), SOD (ANOVA, F4,10 = 0.275, P = 0.891), and GSH-Px (ANOVA, F4,10 = 1.384, P = 0.272) activities were found in the fish serum among all the groups.
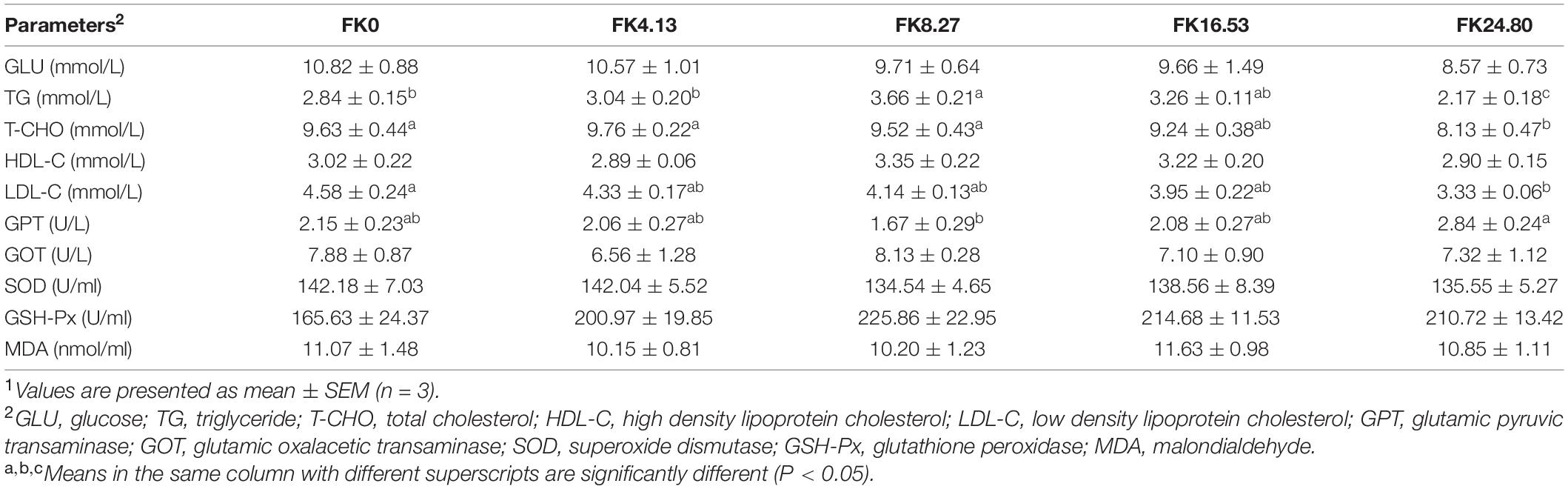
Table 7. Serum biochemical and antioxidative/oxidative parameters of black sea bream fed with different diets1.
Intestinal and Hepatic Histological Observation
The midgut histological structures of fish in the FK0, FK8.27, and FK24.80 groups are presented in Figure 1. The experimental diets did not affect the integrity of midgut intestinal mucosa morphology, with no visible damage. Each mucosal fold was composed of a simple lamina propria with abundant goblet cells and intraepithelial leucocytes (IELs). Different from the other two groups, the FK24.80 group showed expansion of central lacteal in the lamina propria of some villus. The villus height of fish fed with the FK8.27 diet was significantly higher than the FK24.80 diet, whereas both groups showed similar villus height to the control group (ANOVA, F4,10 = 4.047, P = 0.039). The intestinal microvilli in all the groups were arranged neatly and tightly. When the FK protein replacement level increased to 24.8%, the midgut microvillus presented a higher density (ANOVA, F4,10 = 7.258, P = 0.009). For all the fish sampled, the nuclei of hepatocytes had normal and spherical shapes, with clear hepatocyte boundaries (Figure 2).
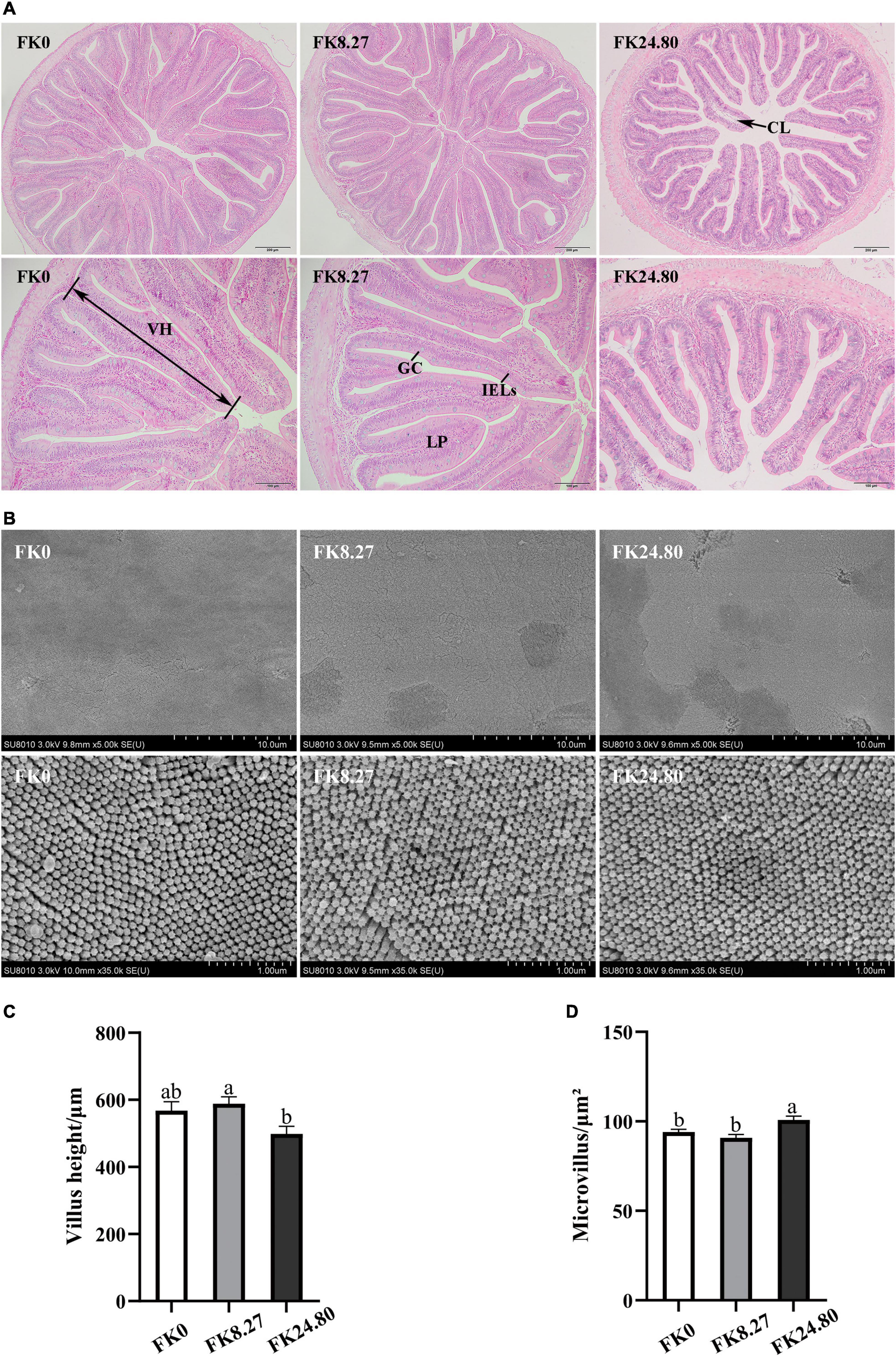
Figure 1. Intestinal histomorphological observation and statistics of black sea bream fed FK0, FK8.27, and FK24.80 diets for 10 weeks. (A) Representative histomorphological images from hematoxylin and eosin-stained midgut transverse section (100×/200×), single arrows indicate vacuoles in the lamina propria; (B) representative scanning electron microscope images of ultrastructure of the midgut epithelium surface (5.00 k×/35.0 k×); (C) mean (±SEM) height of villus in midgut (n = 3); (D) mean (±SEM) number of microvillus per μm2 in midgut (n = 3). a,bMeans with different superscripts are significantly different (P < 0.05). VH, villus height; LP, lamina propria; GC, goblet cell; IELs, intraepithelial leucocytes; CL, central lacteal.
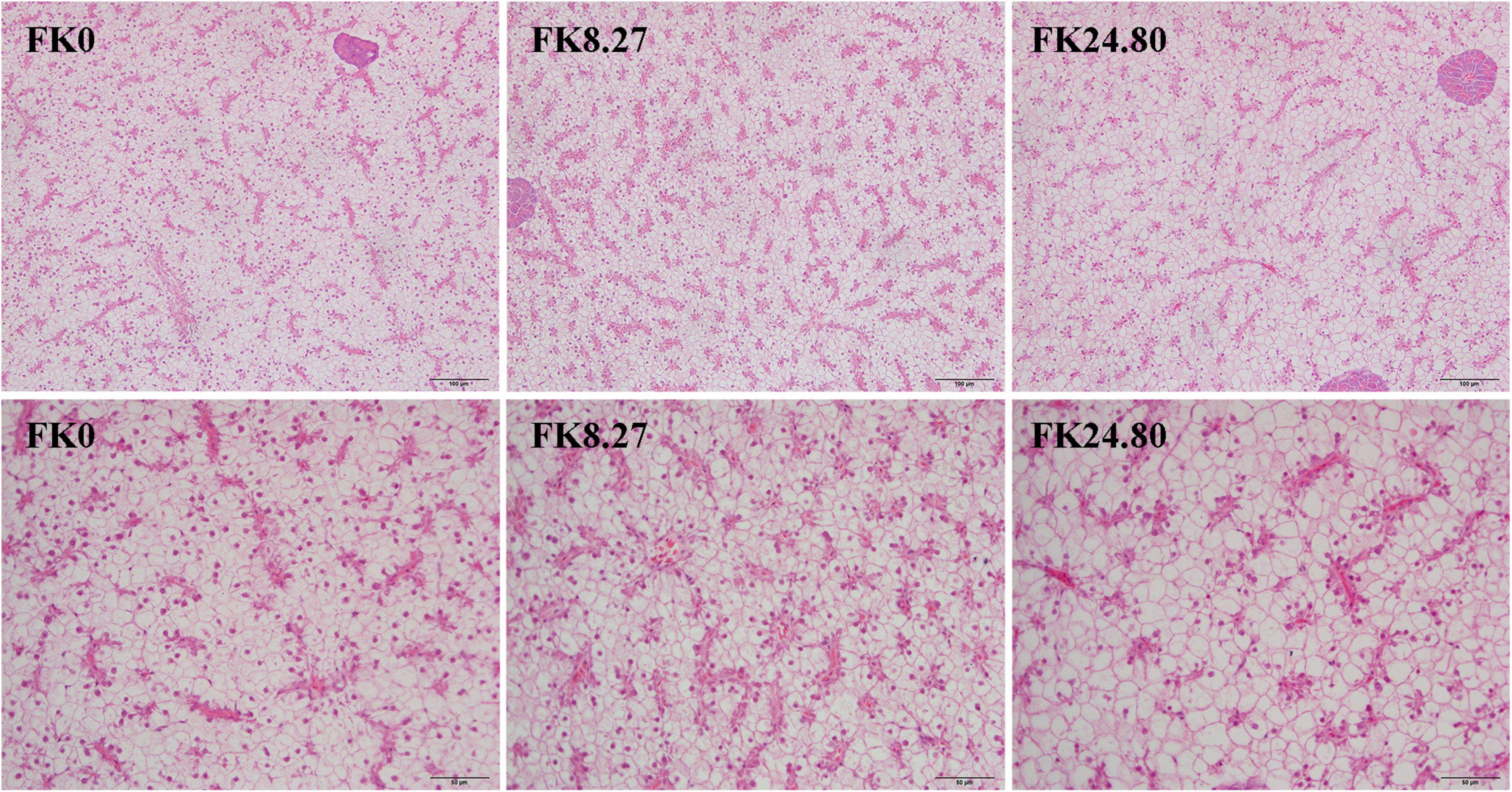
Figure 2. Representative histomorphological images from hematoxylin and eosin-stained liver of black sea bream fed FK0, FK8.27, and FK24.80 diets for 10 weeks (200×/400×).
Discussion
The present study assessed the viability of using methanotroph bacteria meal as an FM alternative in the formulated diet of black sea bream. Among the experimental diets, the levels of taurine and essential amino acids were balanced and sufficient for the growth of black sea bream. The improved WG and SGR of fish in the FK8.27 group may be ascribed to the synergistic effects of combining appropriate levels of two ingredients, i.e., FM and FK, with high nutritional values and complementary amino acids in the diet (Adeoye et al., 2021). Similar results were also obtained in African catfish fed diet with 30% FM protein replaced by a BP product (Yenprotide manufactured by Yenher Agro-Products Sdn. Bhd., Malaysia) (Adeoye et al., 2021), Atlantic salmon fed diets with 18 and 36% bacterial protein meal containing a mix of Bath, Alcaligenes acidovorans, Bacillus brevis, and Bacillus firmus (BPM) (Aas et al., 2006a), as well as Nile tilapia (Oreochromis nilotica) and Malaysian Mahseer (Tor tambroides) fed with diets incorporating 1:2 (w/w) of phototrophic purple bacteria (Rhodovulum sulfidophilum and Marichromatium sp., respectively) (Banerjee et al., 2000; Chowdhury et al., 2016). Chowdhury et al. (2016) reported that the extracellular enzymes of phototrophic purple bacteria could benefit the early digestion and metabolism of fish. These enzymes may be responsible for the improved fish growth. Further studies on the functions of extracellular enzymes in FK are needed to verify this speculation. Furthermore, the improved growth of fish fed the FK8.27 diet in this study could be explained by the higher FR with unchanged levels of feed utilization (FCR, PER, and PPV) of the fish. Good attractability and palatability of diet can promote the onset and continuation of feed ingestion in most aquatic animals, and thus increase feed intake (Grasso and Basil, 2002; Rønnestad et al., 2013). Low molecular weight (<1,000 Da) substances such as amino acids and nucleotides can stimulate the olfactory and gustatory sensory cells of fish (Gamboa-Delgado and Márquez-Reyes, 2018). Previous studies reported that dietary supplementation of nucleotides improved the feed intake, growth, immune response, and stress tolerance in fish (Rumsey et al., 1992; Li and Gatlin, 2006) and shrimp (Li et al., 2007; Biswas et al., 2012). Microorganisms have high nucleotide content, making them efficient palatability agents (Gamboa-Delgado and Márquez-Reyes, 2018). The increased FR of black sea bream fed the FK diet can be attributed to improved palatability caused by a high nucleotide level.
In the present study, replacing FM protein up to 24.80% with FK protein in the diet did not significantly affect the growth performance and feed utilization of black sea bream, which may be because the substitution level of FK was not too high. In our previous study of black sea bream, no significant effects were found on the growth performance of fish fed diets with up to 58.20% FM replaced by CAP (Chen et al., 2020). Similar results were also obtained in Pacific white shrimp (Litopenaeus vannamei, 50% FM replacement) and smallmouth grunt (Haemulon chrysargyreum, 30% FM replacement) fed with Methylobacterium extorquens BP diets (Tlusty et al., 2017), African catfish fed with 60 or 100% BP diets (Adeoye et al., 2021), and rainbow trout fed with 27% BPM diet (Aas et al., 2006b). Nevertheless, in the study of Storebakken et al. (2004), dietary 50% FM amino acids replaced by BPM exhibited negative effects on the growth of Atlantic salmon. Aas et al. (2007) found that Atlantic halibut fed 9% BPM diet performed similarly as the control group, whereas the growth performance and feed utilization were reduced in the fish fed 18% BPM diet. Reduced growth performance was also found in Japanese yellowtail when more than 50% dietary FM was replaced by FK (Biswas et al., 2020). Apart from the differences of fish species and FM content in the basal diets of different experiments, compared to the present study, the adverse results may be because high levels of dietary BPs could reduce the digestibility and absorption of nitrogen, total amino acids, as well as several essential and non-essential amino acids (Perera et al., 1995a,b; Storebakken et al., 2004; Øverland et al., 2006). Hence, overuse of BPs in diets may limit the amino acids supply for fish growth. In addition, Sharif et al. (2021) proposed that the adverse effects on the fish growth may be due to the high concentration of nucleic acids in the BPs. Although dietary nucleic acids may improve the growth and immunity of fish, a high dietary level (10%) can affect the palatability of diets, increase the uric acid level in the serum and adversely affect feed intake, growth and feed utilization of fish (Tacon and Cooke, 1980; Li and Gatlin, 2006). FK contains 9% nucleic acids (Calysta data), and thus FK24.80 contributed less than 1% nucleic acids to the feed. Based on the unchanged FR, there was no apparent palatability-mediated aversion for the FK24.80 diet in the present study. Therefore, it can be inferred that the nucleic acids in the experimental diets had no negative effects on the feed intake and growth of black sea bream. The effects of FK replacement levels higher than 24.80% on black sea bream could be further studied to determine the maximum dietary FK concentration that can maintain the normal growth of fish. On the other hand, low digestibility ascribed to microbial cell walls and membranes impeding enzymatic digestion could also account for the poor performance at relatively high BP inclusion levels (Kiessling and Askbrandt, 1993). Previous studies found that the ADCs of dry matter and energy significantly decreased as the dietary SCP level increased in sea bass (Dicentrarchus labrax) (Oliva-Teles and Gonçalves, 2001), and Atlantic salmon (Berge et al., 2005). Rumsey et al. (1991) demonstrated that the energy and nitrogen digestibility of rainbow trout increased after removing all the yeast cell wall components and separating nitrogen into amino acids and nucleic acids fractions. In this study, the FK24.80 group also presented the lowest ADC of dry matter, though no significant differences in the ADCs of dry matter and crude protein were found between FK inclusion groups and the control. Further study on the FK and its autolysate as dietary protein sources is needed to explain the factors affecting its digestion, especially at a high inclusion level.
In the present study, dietary 8.27% FM protein replaced by FK significantly increased the crude protein content in the whole-body and muscle of black sea bream. This suggests that the combination of FM and appropriate level of FK in the diet could contribute to superior growth performance and nutrient absorption. Adeoye et al. (2021) also found that catfish fed with 30% BP included diet had higher body protein content than the control. In addition, the foregut and midgut amylase activities of fish in the FK8.27 group were higher than those in the control group, indicating that the capacity of the fish to digest carbohydrates was enhanced and more protein was saved for growth. With the increase of FK replacement level, the whole-body and muscle crude lipid contents of fish first increased and then decreased, with the fish in the FK8.27 group showing the highest crude lipid content. This result could be related to the higher lipase activity in the foregut and the ADC of crude lipid of fish fed the FK8.27 diet, which suggests enhanced lipid digestion of fish. Many studies have also demonstrated the protein-sparing effect of lipid (Yigit et al., 2002; Ai et al., 2004; Aliyu-Paiko et al., 2010; Li et al., 2017; Wang L. et al., 2019). Furthermore, the lipid composition of FK is different from that of the FM, being rich in phospholipids, consisting mainly of 16:0 and 16:1 fatty acids (Calysta data). Tocher et al. (2008) proposed that dietary phospholipids could improve the digestion and absorption of lipids and other nutrients, and facilitate the transport efficiency of fatty acids and lipids from the intestine to the rest of the body by promoting lipoprotein synthesis. The higher serum TG content in the FK8.27 group also indicates a more active lipid metabolism of the fish. It could be speculated that the combination of FM and suitable level of FK in the diet could benefit the digestion and retention of lipids in the body of the fish. Similar results were found in rainbow trout (Hauptman et al., 2014), red drum (Sciaenops ocellatus) (Rosales et al., 2017), and sunshine bass (female white bass Morone chrysops × male striped bass M. saxatilis) (Gause and Trushenski, 2011). In addition, Adeoye et al. (2021) and Hamidoghli et al. (2019) hypothesized thathigher lipid contents could be ascribed to an attempt to compensate for the imbalance of BP amino acids by promoting protein deamination, resulting in the non-nitrogenous or carbonaceous components of the diet being deposited as lipids. However, the muscle crude lipid content of fish in the FK24.80 group was instead lower than the other groups in this study. This may be due to BPs non-starch polysaccharides (NSP) content, which is assumed to interfere with nutrients digestion and absorption by increasing digesta viscosity (Storebakken et al., 1998; Leenhouwers et al., 2006; Duan et al., 2017). The specific mechanisms need further exploration.
In the present study, black sea bream fed with representative levels of dietary FK were taken for histological observation, including FK0, FK8.27, and FK24.80. The midgut was chosen because of its highest digestive enzyme activities among the three parts of the intestine. The results showed that dietary FK did not negatively affect the integrity of fish midgut intestinal mucosa and hepatic morphology. No significant differences in midgut villus height were observed between the FK inclusion groups and the control group, while the microvillus in the FK24.80 group presented a higher density than the FK0 and FK8.27 groups. Similarly, previous studies reported that dietary BPs did not damage the intestinal morphology of Atlantic salmon (Storebakken et al., 2004; Berge et al., 2005) and African catfish (Adeoye et al., 2021). The increased microvillus density provided a larger nutrient absorptive surface in the fish fed the FK24.80 diet, which may compensate for the relatively lower villus height. Dietary nucleotides, which increased with the increasing FK levels, were demonstrated to influence gut motility, thus increasing the transit time of digesta and may stimulate the increase of microvilli density (Kim et al., 1968). Furthermore, dietary nucleotides and phospholipids could improve intestinal health and ameliorate intestinal injury (Sturm and Dignass, 2002; Li and Gatlin, 2006), which may benefit the growth of microvilli in the FK24.80 group.
Serum biochemical parameters are widely used as indicators of the general nutritional condition and physiological status of fish (Congleton and Wagner, 2006). In this study, the measured parameters, such as GLU, HDL-C, and MDA contents, GPT, GOT, SOD, and GSH-Px activities, did not show any obvious changes in fish fed the FK included diets. Similar results were found in African catfish fed dietary BP obtained from fermentation of agricultural wastes (Adeoye et al., 2021), Japanese yellowtail fed dietary FK (Biswas et al., 2020), and black sea bream fed dietary CAP (Chen et al., 2020). Serum TG, T-CHO, and LDL-C are indicators relating to lipid metabolism of fish. In the present study, the serum TG concentration increased with increasing dietary FK protein replacement level to 8.27% then decreased. Replacing 24.80% FM protein with FK in the diet significantly decreased the serum TG, T-CHO, and LDL-C levels of fish. This result was consistent with the gut lipase activity, suggesting that the lipid hydrolysis level increased first and then decreased with the increasing dietary FK levels. It may also be related to the dietary lipid composition, as FK had a different fatty acid profile from FM. Studies found that dietary phospholipids could lower plasma lipoprotein levels, and bioactive components in bacterial meal lipids could lower blood cholesterol (Øverland et al., 2010). Nevertheless, referring to other studies on black sea bream, the values of these parameters were all within the normal ranges (Jin et al., 2017, 2019; Wang et al., 2020b). These results manifested that feeding FK to black sea bream did not elicit apparent adverse effects on the fish physiological health and antioxidative/oxidative status.
Conclusion
In conclusion, this study found that dietary FM protein can be partly (24.80%) replaced with FK without adverse impacts on growth performance, feed utilization, intestinal and hepatic histology, serum biochemical and antioxidative/oxidative parameters in black sea bream. The combination of dietary FM and appropriate FK level (e.g., FK8.27) can contribute to superior growth performance and nutrient absorption of black sea bream. Further research on the higher dietary replacement level in various aquatic species and the bioactive components (e.g., nucleic acids, phospholipids, NSPs) is essential for evaluating the nutritional value and exploring the functional mechanism of SCP products.
Data Availability Statement
The raw data supporting the conclusions of this article will be made available by the authors, without undue reservation.
Ethics Statement
The animal study was reviewed and approved by the Institutional Animal Care and Use Committee at Zhejiang University.
Author Contributions
QS designed the study and provided the fund support. BX conducted the experiment, analyzed the data, and wrote the manuscript. YL and KC participated in the feeding trial and subsequent analysis. LW, GS, AT, and YS helped to take samples and revised the manuscript. YY, LZ, and SU helped to analyze the samples and results. All the authors contributed to the article and approved the submitted version.
Funding
This work was supported by China National Ministry of Science and Technology (Funding No. 2020YFD0900801).
Conflict of Interest
The authors declare that the research was conducted in the absence of any commercial or financial relationships that could be construed as a potential conflict of interest.
Publisher’s Note
All claims expressed in this article are solely those of the authors and do not necessarily represent those of their affiliated organizations, or those of the publisher, the editors and the reviewers. Any product that may be evaluated in this article, or claim that may be made by its manufacturer, is not guaranteed or endorsed by the publisher.
Acknowledgments
We are grateful to the Key Lab of Mari-culture and Breeding of Zhejiang Province and China-Norwegian Joint Laboratory of Nutrition and Feed for Marine Aquaculture for providing the rearing system and logistical support during the feeding trial. Thanks for the technical support for light microscopy by the Experimental Teaching Center of Zhejiang University College of Animal Sciences and the Core Facility Platform of Zhejiang University School of Medicine. Thanks for the technical support for electron microscopy by Bio-Ultrastructure Analysis Lab of Analysis Center of Agrobiology and Environmental Sciences, Zhejiang University.
References
Aas, T. S., Grisdale-Helland, B., Terjesen, B. F., and Helland, S. J. (2006a). Improved growth and nutrient utilisation in Atlantic salmon (Salmo salar) fed diets containing a bacterial protein meal. Aquaculture 259, 365–376. doi: 10.1016/j.aquaculture.2006.05.032
Aas, T. S., Hatlen, B., Grisdale-Helland, B., Terjesen, B. F., Bakke-McKellep, A. M., and Helland, S. J. (2006b). Effects of diets containing a bacterial protein meal on growth and feed utilisation in rainbow trout (Oncorhynchus mykiss). Aquaculture 261, 357–368. doi: 10.1016/j.aquaculture.2006.07.033
Aas, T. S., Hatlen, B., Grisdale-Helland, B., Terjesen, B. F., Penn, M., Bakke-McKellep, A. M., et al. (2007). Feed intake, growth and nutrient utilization in Atlantic halibut (Hippoglossus hippoglossus) fed diets containing a bacterial protein meal. Aquac. Res. 38, 351–360. doi: 10.1111/j.1365-2109.2007.01672.x
Adeoye, A. A., Akegbejo-samsons, Y., Fawole, F. J., Olatunji, P. O., Muller, N., Wan, A. H. L., et al. (2021). From waste to feed: dietary utilisation of bacterial protein from fermentation of agricultural wastes in African catfish (Clarias gariepinus) production and health. Aquaculture 531:735850. doi: 10.1016/j.aquaculture.2020.735850
Ai, Q., Mai, K., Li, H., Zhang, C., Zhang, L., Duan, Q., et al. (2004). Effects of dietary protein to energy ratios on growth and body composition of juvenile Japanese seabass, Lateolabrax japonicus. Aquaculture 230, 507–516. doi: 10.1016/j.aquaculture.2003.09.040
Aliyu-Paiko, M., Hashim, R., and Shu-Chien, A. C. (2010). Influence of dietary lipid/protein ratio on survival, growth, body indices and digestive lipase activity in Snakehead (Channa striatus, Bloch 1793) fry reared in re-circulating water system. Aquac. Nutr. 16, 466–474. doi: 10.1111/j.1365-2095.2009.00683.x
Alloul, A., Wille, M., Lucenti, P., Bossier, P., Van Stappen, G., Vlaeminck, S. E., et al. (2021). Purple bacteria as added-value protein ingredient in shrimp feed: Penaeus vannamei growth performance, and tolerance against Vibrio and ammonia stress. Aquaculture 530:735788. doi: 10.1016/j.aquaculture.2020.735788
Anupama, and Ravindra, P. (2000). Value-added food: single cell protein. Biotechnol. Adv. 18, 459–479. doi: 10.1016/S0734-9750(00)00045-48
AOAC (1995). Official Methods of Analysis of the Association of Official Analytical Chemists. Washington, DC: Association of Official Analytical Chemists.
Banerjee, S., Azad, S. A., Vikineswary, S., Selvaraj, O. S., and Mukherjee, T. K. (2000). Phototrophic bacteria as fish feed supplement. Asian-Australasian J. Anim. Sci. 13, 991–994. doi: 10.5713/ajas.2000.991
Berge, G. M., Baeverfjord, G., Skrede, A., and Storebakken, T. (2005). Bacterial protein grown on natural gas as protein source in diets for Atlantic salmon. Salmo salar, saltwater. Aquaculture 244, 233–240. doi: 10.1016/j.aquaculture.2004.11.017
Biswas, A., Takakuwa, F., Yamada, S., Matsuda, A., Saville, R. M., LeBlanc, A., et al. (2020). Methanotroph (Methylococcus capsulatus, Bath) bacteria meal as an alternative protein source for Japanese yellowtail, Seriola quinqueradiata. Aquaculture 529:735700. doi: 10.1016/j.aquaculture.2020.735700
Biswas, G., Korenaga, H., Nagamine, R., Kono, T., Shimokawa, H., Itami, T., et al. (2012). Immune stimulant effects of a nucleotide-rich baker’s yeast extract in the kuruma shrimp, Marsupenaeus japonicus. Aquaculture 366-367, 40–45. doi: 10.1016/j.aquaculture.2012.09.001
Chen, Y., Sagada, G., Xu, B., Chao, W., Zou, F., Ng, W. K., et al. (2020). Partial replacement of fishmeal with Clostridium autoethanogenum single-cell protein in the diet for juvenile black sea bream (Acanthopagrus schlegelii). Aquac. Res. 51, 1000–1011. doi: 10.1111/are.14446
Chowdhury, A. J. K., Zakaria, N. H., Abidin, Z. A. Z., and Rahman, M. M. (2016). Phototrophic purple bacteria as feed supplement on the growth, feed utilization and body compositions of Malaysian Mahseer, Tor tambroides juveniles. Sains Malaysiana 45, 135–140.
Congleton, J. L., and Wagner, T. (2006). Blood-chemistry indicators of nutritional status in juvenile salmonids. J. Fish Biol. 69, 473–490. doi: 10.1111/j.1095-8649.2006.01114.x
Cruz-Suárez, L. E., Tapia-Salazar, M., Villarreal-Cavazos, D., Beltran-Rocha, J., Nieto-López, M. G., Lemme, A., et al. (2009). Apparent dry matter, energy, protein and amino acid digestibility of four soybean ingredients in white shrimp Litopenaeus vannamei juveniles. Aquaculture 292, 87–94. doi: 10.1016/j.aquaculture.2009.03.026
De Francesco, M., Parisi, G., Pérez-Sánchez, J., Gómez-Réqueni, P., Médale, F., Kaushik, S. J., et al. (2007). Effect of high-level fish meal replacement by plant proteins in gilthead sea bream (Sparus aurata) on growth and body/fillet quality traits. Aquac. Nutr. 13, 361–372. doi: 10.1111/j.1365-2095.2007.00485.x
Duan, Y., Zhang, Y., Dong, H., Wang, Y., Zheng, X., and Zhang, J. (2017). Effect of dietary Clostridium butyricum on growth, intestine health status and resistance to ammonia stress in Pacific white shrimp Litopenaeus vannamei. Fish Shellfish Immunol. 65, 25–33. doi: 10.1016/j.fsi.2017.03.048
Erdman, M. D., Bergen, W. G., and Adinarayana Reddy, C. (1977). Amino acid profiles and presumptive nutritional assessment of single cell protein from certain lactobacilli. Appl. Environ. Microbiol. 33, 901–905. doi: 10.1128/aem.33.4.901-905.1977
Francis, G., Makkar, H. P. S., and Becker, K. (2001). Antinutritional factors present in plant-derived alternate fish feed ingredients and their effects in fish. Aquaculture 199, 197–227.
Gamboa-Delgado, J., and Márquez-Reyes, J. M. (2018). Potential of microbial-derived nutrients for aquaculture development. Rev. Aquac. 10, 224–246. doi: 10.1111/raq.12157
Gatlin, D. M., Barrows, F. T., Brown, P., Dabrowski, K., Gaylord, T. G., Hardy, R. W., et al. (2007). Expanding the utilization of sustainable plant products in aquafeeds: a review. Aquac. Res. 38, 551–579. doi: 10.1111/j.1365-2109.2007.01704.x
Gause, B., and Trushenski, J. (2011). Replacement of fish meal with ethanol yeast in the diets of sunshine bass. N. Am. J. Aquac. 73, 97–103. doi: 10.1080/15222055.2011.544939
Grasso, F. W., and Basil, J. A. (2002). How lobsters, crayfishes, and crabs locate sources of odor: current perspectives and future directions. Curr. Opin. Neurobiol. 12, 721–727. doi: 10.1016/S0959-4388(02)00388-384
Hamidoghli, A., Yun, H., Won, S., Kim, S. K., Farris, N. W., and Bai, S. C. (2019). Evaluation of a single-cell protein as a dietary fish meal substitute for whiteleg shrimp Litopenaeus vannamei. Fish. Sci. 85, 147–155. doi: 10.1007/s12562-018-1275-1275
Hardy, R. W., Patro, B., Pujol-Baxley, C., Marx, C. J., and Feinberg, L. (2018). Partial replacement of soybean meal with Methylobacterium extorquens single-cell protein in feeds for rainbow trout (Oncorhynchus mykiss Walbaum). Aquac. Res. 49, 2218–2224. doi: 10.1111/are.13678
Hauptman, B. S., Barrows, F. T., Block, S. S., Gibson Gaylord, T., Paterson, J. A., Rawles, S. D., et al. (2014). Evaluation of grain distillers dried yeast as a fish meal substitute in practical-type diets of juvenile rainbow trout. Oncorhynchus mykiss. Aquaculture 432, 7–14. doi: 10.1016/j.aquaculture.2014.03.026
Henry, M., Gasco, L., Piccolo, G., and Fountoulaki, E. (2015). Review on the use of insects in the diet of farmed fish: past and future. Anim. Feed Sci. Technol. 203, 1–22. doi: 10.1016/j.anifeedsci.2015.03.001
Hong, W., and Zhang, Q. (2003). Review of captive bred species and fry production of marine fish in China. Aquaculture 227, 305–318. doi: 10.1016/S0044-8486(03)00511-518
Jannathulla, R., Sravanthi, O., Moomeen, S., Gopikrishna, G., and Dayal, J. S. (2021). Microbial products in terms of isolates, whole-cell biomass, and live organisms as aquafeed ingredients: production, nutritional values, and market potential—a review. Aquac. Int. 29, 623–650. doi: 10.1007/s10499-021-00644-642
Jin, M., Pan, T., Cheng, X., Zhu, T. T., Sun, P., Zhou, F., et al. (2019). Effects of supplemental dietary L-carnitine and bile acids on growth performance, antioxidant and immune ability, histopathological changes and inflammatory response in juvenile black seabream (Acanthopagrus schlegelii) fed high-fat diet. Aquaculture 504, 199–209. doi: 10.1016/j.aquaculture.2019.01.063
Jin, M., Yuan, Y., Lu, Y., Ma, H., Sun, P., Li, Y., et al. (2017). Regulation of growth, tissue fatty acid composition, biochemical parameters and lipid related genes expression by different dietary lipid sources in juvenile black seabream, Acanthopagrus schlegelii. Aquaculture 479, 25–37. doi: 10.1016/j.aquaculture.2017.05.017
Kaushik, S. J., Covès, D., Dutto, G., and Blanc, D. (2004). Almost total replacement of fish meal by plant protein sources in the diet of a marine teleost, the European seabass, Dicentrarchus labrax. Aquaculture 230, 391–404. doi: 10.1016/S0044-8486(03)00422-428
Kiessling, A., and Askbrandt, S. (1993). Nutritive value of two bacterial strains of single-cell protein for rainbow trout (Oncorhynchus mykiss). Aquaculture 109, 119–130. doi: 10.1016/0044-8486(93)90209-H
Kim, S. W., Less, J. F., Wang, L., Yan, T., Kiron, V., Kaushik, S. J., et al. (2019). Meeting global feed protein demand: challenge, opportunity, and strategy. Annu. Rev. Anim. Biosci. 7, 221–243. doi: 10.1146/annurev-animal-030117-014838
Kim, T. S., Shulman, J., and Levine, R. A. (1968). Relaxant effect of cyclic adenosine 3′, 5′-monophosphate on the isolated rabbit ileum. J. Pharmacol. Exp. Ther. 163, 36–42.
Leenhouwers, J. I., Adjei-Boateng, D., Verreth, J. A. J., and Schrama, J. W. (2006). Digesta viscosity, nutrient digestibility and organ weights in African catfish (Clarias gariepinus) fed diets supplemented with different levels of a soluble non-starch polysaccharide. Aquac. Nutr. 12, 111–116. doi: 10.1111/j.1365-2095.2006.00389.x
Li, P., and Gatlin, D. M. (2006). Nucleotide nutrition in fish: current knowledge and future applications. Aquaculture 251, 141–152. doi: 10.1016/j.aquaculture.2005.01.009
Li, P., Lawrence, A. L., Castille, F. L., and Gatlin, D. M. (2007). Preliminary evaluation of a purified nucleotide mixture as a dietary supplement for Pacific white shrimp Litopenaeus vannamei (Boone). Aquac. Res. 38, 887–890. doi: 10.1111/j.1365-2109.2007.01761.x
Li, W., Wen, X., Huang, Y., Zhao, J., Li, S., and Zhu, D. (2017). Effects of varying protein and lipid levels and protein-to-energy ratios on growth, feed utilization and body composition in juvenile Nibea diacanthus. Aquac. Nutr. 23, 1035–1047. doi: 10.1111/anu.12471
Matassa, S., Boon, N., Pikaar, I., and Verstraete, W. (2016). Microbial protein: future sustainable food supply route with low environmental footprint. Microb. Biotechnol. 9, 568–575. doi: 10.1111/1751-7915.12369
Maulu, S., Liang, H., Ge, X., Yu, H., Huang, D., and Ke, J. (2021). Effect of dietary Clostridium autoethanogenum protein on growth, body composition, plasma parameters and hepatic genes expression related to growth and AMPK / TOR / PI3K signaling pathway of the genetically improved farmed tilapia (GIFT: Oreochromis niloticus) juveniles. Animal Feed Sci. Technol. 276:114914. doi: 10.1016/j.anifeedsci.2021.114914
Miao, S., Zhao, C., Zhu, J., Hu, J., Dong, X., and Sun, L. (2018). Dietary soybean meal affects intestinal homoeostasis by altering the microbiota, morphology and inflammatory cytokine gene expression in northern snakehead. Sci. Rep. 8:113. doi: 10.1038/s41598-017-18430-18437
Oliva-Teles, A., and Gonçalves, P. (2001). Partial replacement of fishmeal by brewers yeast (Saccaromyces cerevisae) in diets for sea bass (Dicentrarchus labrax) juveniles. Aquaculture 202, 269–278. doi: 10.1016/S0044-8486(01)00777-773
Olsen, R. L., and Hasan, M. R. (2012). A limited supply of fishmeal: impact on future increases in global aquaculture production. Trends Food Sci. Technol. 27, 120–128. doi: 10.1016/j.tifs.2012.06.003
Øverland, M., Romarheim, O. H., Hovin, M., Storebakken, T., and Skrede, A. (2006). Apparent total tract digestibility of unprocessed and extruded diets containing basic and autolyzed bacterial protein meal grown on natural gas in mink and rainbow trout. Anim. Feed Sci. Technol. 129, 237–251. doi: 10.1016/j.anifeedsci.2005.12.017
Øverland, M., Tauson, A. H., Shearer, K., and Skrede, A. (2010). Evaluation of methane-utilising bacteria products as feed ingredients for monogastric animals. Arch. Anim. Nutr. 64, 171–189. doi: 10.1080/17450391003691534
Perera, W. M. K., Carter, C. G., and Houlihan, D. F. (1995a). Apparent absorption efficiencies of amino acids in rainbow trout, Oncorhynchus mykiss (Walbaum), fed diets containing bacterial single-cell protein. Aquac. Nutr. 1, 95–103. doi: 10.1111/j.1365-2095.1995.tb00024.x
Perera, W. M. K., Carter, C. G., and Houlihan, D. F. (1995b). Feed consumption, growth and growth efficiency of rainbow trout (Oncorhynchus mykiss (Walbaum) fed on diets containing a bacterial single-cell protein. Br. J. Nutr. 73, 591–603. doi: 10.1079/bjn19950061
Rhodes, M. A., Zhou, Y., and Davis, D. A. (2015). Use of dried fermented biomass as a fish meal replacement in practical diets of Florida pompano, Trachinotus carolinus. J. Appl. Aquac. 27, 29–39. doi: 10.1080/10454438.2014.959834
Romarheim, O. H., Øverland, M., Mydland, L. T., Skrede, A., and Landsverk, T. (2011). Bacteria grown on natural gas prevent soybean meal-induced enteritis in atlantic salmon. J. Nutr. 141, 124–130. doi: 10.3945/jn.110.128900
Rønnestad, I., Yúfera, M., Ueberschär, B., Ribeiro, L., Sæle, Ø, and Boglione, C. (2013). Feeding behaviour and digestive physiology in larval fish: current knowledge, and gaps and bottlenecks in research. Rev. Aquac. 5, S59–S98. doi: 10.1111/raq.12010
Rosales, M., Castillo, S., Pohlenz, C., and Gatlin, D. M. (2017). Evaluation of dried yeast and threonine fermentation biomass as partial fish meal replacements in the diet of red drum Sciaenops ocellatus. Anim. Feed Sci. Technol. 232, 190–197. doi: 10.1016/j.anifeedsci.2017.08.014
Rumsey, G. L., Hughes, S. G., Smith, R. R., Kinsella, J. E., and Shetty, K. J. (1991). Digestibility and energy values of intact, disrupted and extracts from brewer’s dried yeast fed to rainbow trout (Oncorhynchus mykiss). Anim. Feed Sci. Technol. 33, 185–193. doi: 10.1016/0377-8401(91)90059-90052
Rumsey, G. L., Winfree, R. A., and Hughes, S. G. (1992). Nutritional value of dietary nucleic acids and purine bases to rainbow trout (Oncorhynchus mykiss). Aquaculture 108, 97–110. doi: 10.1016/0044-8486(92)90321-B
Sánchez-Muros, M. J., Barroso, F. G., and Manzano-Agugliaro, F. (2014). Insect meal as renewable source of food for animal feeding: a review. J. Clean. Prod. 65, 16–27. doi: 10.1016/j.jclepro.2013.11.068
Shao, Q., Ma, J., Xu, Z., Hu, W., Xu, J., and Xie, S. (2008). Dietary phosphorus requirement of juvenile black seabream, Sparus macrocephalus. Aquaculture 277, 92–100. doi: 10.1016/j.aquaculture.2008.01.029
Sharif, M., Zafar, M. H., Aqib, A. I., Saeed, M., Farag, M. R., and Alagawany, M. (2021). Single cell protein: sources, mechanism of production, nutritional value and its uses in aquaculture nutrition. Aquaculture 531:735885. doi: 10.1016/j.aquaculture.2020.735885
Storebakken, T., Baeverfjord, G., Skrede, A., Olli, J. J., and Berge, G. M. (2004). Bacterial protein grown on natural gas in diets for Atlantic salmon, Salmo salar, in freshwater. Aquaculture 241, 413–425. doi: 10.1016/j.aquaculture.2004.07.024
Storebakken, T., Kvien, I. S., Shearer, K. D., Grisdale-Helland, B., Helland, S. J., and Berge, G. M. (1998). The apparent digestibility of diets containing fish meal, soybean meal or bacterial meal fed to Atlantic salmon (Salmo salar): evaluation of different faecal collection methods. Aquaculture 169, 195–210. doi: 10.1016/S0044-8486(98)00379-372
Sturm, A., and Dignass, A. U. (2002). Modulation of gastrointestinal wound repair and inflammation by phospholipids. Biochim. Biophys. Acta - Mol. Cell Biol. Lipids 1582, 282–288. doi: 10.1016/S1388-1981(02)00182-188
Tacon, A. G. J., and Cooke, D. J. (1980). Nutritional value of dietary nucleic acids to trout. Nutr. Rep. Int. 22, 631–640.
Tlusty, M., Rhyne, A., Szczebak, J. T., Bourque, B., Bowen, J. L., Burr, G., et al. (2017). A transdisciplinary approach to the initial validation of a single cell protein as an alternative protein source for use in aquafeeds. PeerJ 2017:e3170. doi: 10.7717/peerj.3170
Tocher, D. R., Bendiksen, E. Å, Campbell, P. J., and Bell, J. G. (2008). The role of phospholipids in nutrition and metabolism of teleost fish. Aquaculture 280, 21–34. doi: 10.1016/j.aquaculture.2008.04.034
Tong, S., Wang, L., Kalhoro, H., Volatiana, J. A., and Shao, Q. (2020). Effects of supplementing taurine in all-plant protein diets on growth performance, serum parameters, and cholesterol 7α-hydroxylase gene expression in black sea bream, Acanthopagrus schlegelii. J. World Aquac. Soc. 51, 990–1001. doi: 10.1111/jwas.12611
Wang, C., Chuprom, J., Wang, Y., and Fu, L. (2020a). Beneficial bacteria for aquaculture: nutrition, bacteriostasis and immunoregulation. J. Appl. Microbiol. 128, 28–40. doi: 10.1111/jam.14383
Wang, L., Xu, B., Sagada, G., Ng, W. K., Chen, K., Zhang, J., et al. (2020b). Dietary berberine regulate lipid metabolism in muscle and liver of black sea bream (Acanthopagrus schlegelii) fed normal or high-lipid diets. Br. J. Nutr. 125, 481–493. doi: 10.1017/S0007114520003025
Wang, L., Yin, N., Sagada, G., Hua, Y., Li, H., Zhang, J., et al. (2020c). Partial replacement of fishmeal with corn gluten meal, pea protein isolate and their mixture in diet of black sea bream (Acanthopagrus schlegelii) juveniles: effects on growth performance, feed utilization and haematological parameters. Aquac. Res. 51, 2071–2083. doi: 10.1111/are.14558
Wang, L., Zhang, W., Gladstone, S., Ng, W. K., Zhang, J., and Shao, Q. (2019). Effects of isoenergetic diets with varying protein and lipid levels on the growth, feed utilization, metabolic enzymes activities, antioxidative status and serum biochemical parameters of black sea bream (Acanthopagrus schlegelii). Aquaculture 513:734397. doi: 10.1016/j.aquaculture.2019.734397
Wang, Y., Li, C., Pan, C., Liu, E., Zhao, X., and Ling, Q. (2019). Alterations to transcriptomic profile, histopathology, and oxidative stress in liver of pikeperch (Sander lucioperca) under heat stress. Fish Shellfish Immunol. 95, 659–669. doi: 10.1016/j.fsi.2019.11.014
Yang, P., Li, X., Song, B., He, M., Wu, C., and Leng, X. (2021). The potential of Clostridium autoethanogenum, a new single cell protein, in substituting fish meal in the diet of largemouth bass (Micropterus salmoides): growth, feed utilization and intestinal histology. Aquac. Fish. (in press). doi: 10.1016/j.aaf.2021.03.003
Yigit, M., Yardim, Ö, and Koshio, S. (2002). The protein sparing effects of high lipid levels in diets for rainbow trout (Oncorhynchus mykiss, W. 1792) with special reference to reduction of total nitrogen excretion. Isr. J. Aquac. - Bamidgeh 54, 79–88. doi: 10.46989/001c.20314
Zhou, F., Shao, J., Xu, R., Ma, J., and Xu, Z. (2010a). Quantitative L-lysine requirement of juvenile black sea bream (Sparus macrocephalus). Aquac. Nutr. 16, 194–204. doi: 10.1111/j.1365-2095.2009.00651.x
Zhou, F., Xiong, W., Xiao, J. X., Shao, Q. J., Bergo, O. N., Hua, Y., et al. (2010b). Optimum arginine requirement of juvenile black sea bream, Sparus macrocephalus. Aquac. Res. 41, 418–430. doi: 10.1111/j.1365-2109.2009.02474.x
Zhou, F., Shao, Q. J., Xiao, J. X., Peng, X., Ngandzali, B. O., Sun, Z., et al. (2011a). Effects of dietary arginine and lysine levels on growth performance, nutrient utilization and tissue biochemical profile of black sea bream, Acanthopagrus schlegelii, fingerlings. Aquaculture 319, 72–80. doi: 10.1016/j.aquaculture.2011.06.001
Zhou, F., Xiao, J. X., Hua, Y., Ngandzali, B. O., and Shao, Q. J. (2011b). Dietary L-methionine requirement of juvenile black sea bream (Sparus macrocephalus) at a constant dietary cystine level. Aquac. Nutr. 17, 469–481. doi: 10.1111/j.1365-2095.2010.00823.x
Zhou, L., Li, H., Qin, J. G., Wang, X., Chen, L., Xu, C., et al. (2020). Dietary prebiotic inulin benefits on growth performance, antioxidant capacity, immune response and intestinal microbiota in Pacific white shrimp (Litopenaeus vannamei) at low salinity. Aquaculture 518:734847. doi: 10.1016/j.aquaculture.2019.734847
Keywords: black sea bream, FeedKind®, single-cell protein, growth, feed utilization, histology
Citation: Xu B, Liu Y, Chen K, Wang L, Sagada G, Tegomo AF, Yang Y, Sun Y, Zheng L, Ullah S and Shao Q (2021) Evaluation of Methanotroph (Methylococcus capsulatus, Bath) Bacteria Meal (FeedKind®) as an Alternative Protein Source for Juvenile Black Sea Bream, Acanthopagrus schlegelii. Front. Mar. Sci. 8:778301. doi: 10.3389/fmars.2021.778301
Received: 16 September 2021; Accepted: 26 October 2021;
Published: 29 November 2021.
Edited by:
Min Gu, Shandong University, Weihai, ChinaReviewed by:
Hualiang Liang, Freshwater Fisheries Research Center, Chinese Academy of Fishery Sciences, ChinaKhor Waiho, University of Malaysia Terengganu, Malaysia
Copyright © 2021 Xu, Liu, Chen, Wang, Sagada, Tegomo, Yang, Sun, Zheng, Ullah and Shao. This is an open-access article distributed under the terms of the Creative Commons Attribution License (CC BY). The use, distribution or reproduction in other forums is permitted, provided the original author(s) and the copyright owner(s) are credited and that the original publication in this journal is cited, in accordance with accepted academic practice. No use, distribution or reproduction is permitted which does not comply with these terms.
*Correspondence: Qingjun Shao, qjshao@zju.edu.cn