- 1Marine Biology Lab, Ghent University, Ghent, Belgium
- 2Institute for Agricultural and Fisheries Research, Aquatic Environment and Quality, Oostende, Belgium
Based on the principle of competitive exclusion, species occupying the same ecological niche cannot stably coexist due to strong interspecific competition for resources. Niche diversification, for instance through resource partitioning, may alleviate competition. Here, we investigate the effects of resource diversity on foraging behavior, fitness and interspecific interactions of four cryptic bacterivorous nematode species (Pm I–IV) of the Litoditis marina species complex with sympatric field distributions. Three resource (bacteria) diversity levels (low, medium, high) were used as food treatments and compared to a treatment with only Escherichia coli as food. Differences in taxis to food existed between the cryptic species and between bacterial mixtures of different diversity: all the cryptic species except Pm I showed higher attraction toward medium-diversity food. Furthermore, the cryptic species of L. marina generally exhibited higher fitness on a more diverse food resource. Resource diversity also impacted the interspecific interactions between the cryptic species. Our results show that resource diversity can alter the interspecific interactions among the cryptic species of L. marina, indicating that competitive equilibria between species are very context-dependent. Although a considerable body of evidence supports the hypotheses (e.g., “variance-in-edibility” hypothesis and the “dilution hypothesis” or “resource concentration hypothesis”) which predict a negative impact on consumers when resource diversity is increased, the benefits of a diverse resource can outweigh these disadvantages by offering a more complete and/or complementary range of nutritional resources as suggested by the “balanced diet” hypothesis.
Introduction
Resource partitioning is central to our understanding of the dynamics of species composition and coexistence in biological communities (Fridley, 2001, 2003; Shea and Chesson, 2002; Griffin et al., 2008; Northfield et al., 2010). It can promote the coexistence of species that compete for the same limiting resources (Schoener, 1974; Walter, 1991) through spatial, temporal, or qualitative differences in how these resources are used (Brown and Wilson, 1956; Pfennig et al., 2006). MacArthur and Levins (1964) pointed out that in order to coexist, each species must utilize a subset of the basic limiting resource which is qualitatively different from the subsets used by the other species. In view of this resource partitioning hypothesis, one might expect that a higher resource diversity may mitigate competition through enhanced resource partitioning. Apart from a few studies on sympatrically distributed vertebrate species (Zapata et al., 2005; Jiang et al., 2007; Barili et al., 2011; Martin and Garnett, 2013), this aspect has hitherto received surprisingly limited attention (Duffy et al., 2007; Sánchez-Hernández et al., 2017).
Resource diversity may also have an influence on the foraging behavior of organisms. Foraging behavior refers to all the methods that an organism uses to detect, acquire and utilize resources, and consists of three basic phases described as “search,” “assessment,” and “exploitation” (Kramer, 2001). Animals can perceive attractive and repellent cues in the environment, and make an assessment to either exploit the encountered resource or to keep searching (Kramer, 2001; Zhang et al., 2005). The differences in the ability to search successfully for food (Kotler and Brown, 1988; Brown et al., 1994; Krasnov et al., 2000) and/or the differences in the attraction toward various types of food (Moens et al., 1999; Newsham et al., 2004; Zhang et al., 2005) are possible mechanisms for coexistence. While food resource diversity may thus enhance species coexistence, it may have an opposite effect on the population abundances of individual consumer species. Theory tends to predict that a more diverse resource is less advantageous for a population of consumers because it is more likely to contain resources that are resistant to consumption; hence, only a part of the resource becomes truly useful (Leibold, 1989; Duffy, 2002; Hillebrand and Shurin, 2005). In addition, resource diversity may reduce both the relative and absolute abundances of preferred food available to consumers, thus forcing consumers to spend more time and energy on finding and selecting the most appropriate food within a mix of resources (Andow, 1991; Joshi et al., 2004; Keesing et al., 2006). These effects are called the “variance-in-edibility” hypothesis (Leibold, 1989; Duffy, 2002) and the “dilution or resource concentration” hypothesis (Andow, 1991; Joshi et al., 2004), respectively. By contrast, different resources may be complementary in their nutritional value (DeMott, 1998), in which case the benefits of a mixed resource can outweigh the disadvantages.
In addition, resource diversity may enable the coexistence of species that differ in their fitness or growth strategies. It is well known that even closely related species can have distinct feeding preferences and different growth rates according to the quality (Venette and Ferris, 1998; Blanc et al., 2006) and/or quantity (Michiels and Traunspurger, 2005; dos Santos et al., 2008) of available food. Furthermore, their fitness responses can vary depending on species interactions (Gansfort et al., 2018). In a scenario where two species compete for the same limiting resource, the competitively dominant species may expand its niche which reduces the available space for the inferior competitor. This may force the weaker species to utilize whatever resources are left available (Levins, 1968; Jasmin and Kassen, 2007; Pekkonen et al., 2013). When resource diversity is high, the competing species may have the possibility to alleviate the negative effects of competition by segregating resource utilization (Sánchez-Hernández et al., 2017). Although the significance of resource partitioning for species coexistence has been a long-standing topic of interest in ecology, empirical studies enhancing our understanding of this issue remain scant. As we proceed to predict responses to global change and biodiversity-ecosystem functioning relationships, adequate understanding of the ecological roles of, and interactions among the component species in a community is pivotal (Johnson et al., 1996; Donoghue, 2009). Thus, a multi-faceted framework which involves behavioral, fitness, and interaction assays is needed to provide mechanistic insights into the effects of resource diversity on coexistence. Here, we study the effects of resource (bacteria) diversity on the coexistence of four cryptic species (Pm I, Pm II, Pm III, and Pm IV) of the nematode Litoditis marina (Sudhaus, 2011). Litoditis marina is a bacterivore marine nematode species complex associated with living and decomposing macroalgae in intertidal zones (Derycke et al., 2006, 2008). This species complex is composed of cryptic species that often co-occur in the field (Derycke et al., 2006, 2008). Despite the traditional assumptions that cryptic species are ecologically equivalent due to their high phenotypic similarity, niche partitioning between these cryptic nematode species has been demonstrated with respect to microhabitat preferences (Guden et al., 2018), abiotic preferences and tolerances (De Meester et al., 2011, 2015b), competitive abilities (De Meester et al., 2011, 2015a), and dispersal strategies (De Meester et al., 2012, 2014, 2018). Furthermore, the existence of species-specific microbiomes has been illustrated in L. marina, which–among other things–may indicate that these very closely related species feed on different bacterial strains (Derycke et al., 2016). In addition to their ecological significance, the ease of collecting, handling and manipulating L. marina in the lab for experimental set-ups renders this cryptic nematode species complex an excellent research model to investigate the influence of resource diversity on the coexistence of closely related species.
To this end, we used a multi-faceted approach. First, we investigated the taxis of the cryptic species of L. marina when offered food with different levels of bacterial diversity. Taxis-to-food assays in the presence of a single bacterial population have shown that closely related nematode species can distinguish between different bacterial strains and even between different abundances of the same bacterial strain (e.g., Moens et al., 1999; Newsham et al., 2004; Zhang et al., 2005). We hypothesized that taxis to food may differ between the cryptic species of L. marina depending on bacterial diversity. Second, we tested the effect of bacterial diversity on the population and individual fitness of L. marina. Previous studies have shown that the type (i.e., strain) of bacterial food affects the fitness of bacterivorous nematodes (Grewal, 1991; Venette and Ferris, 1998; Blanc et al., 2006). Furthermore, there can be clear discrepancies between the food they preferentially move to or ingest and the food that yields the best population growth (Grewal, 1991; Carta, 2000; Blanc et al., 2006). Here, we hypothesized that the cryptic species may have different fitness responses which may also be influenced by the diversity of bacterial food offered to them. Third, we tested the effect of resource diversity on interspecific interactions between the cryptic species of L. marina. We hypothesized that a more diverse food source may help to alleviate interspecific competition by allowing more resource partitioning.
Materials and Methods
Nematode Cultures
Nematodes used in the experiments were harvested from monospecific cultures in exponential growth phase, with unidentified bacteria from the habitat as food. Monospecific cultures of four cryptic species of L. marina (Pm I–Pm IV) were raised from a single gravid female per species obtained from the field and maintained on sloppy (1%) nutrient: bacto agar (ratio of 1:4) media (Moens and Vincx, 1998). The nematode stock cultures were incubated in the dark under standardized conditions (temperature: 20°C; salinity: 25) for many generations prior to the start of our experiments. The culture inocula of Pm I, Pm II, and Pm III originate from Paulina salt marsh, Westerschelde Estuary, The Netherlands (51° 21′ N, 3° 49′ E; salinity: 24), whereas Pm IV was obtained from Lake Grevelingen, The Netherlands (51° 44′ N, 3° 57′ E; salinity: 32).
Food Resources for Nematodes
Twenty-five marine bacterial strains (Supplementary Table 1) for the present experiments were obtained from the BCCM/LMG bacterial culture collection at the Department of Biochemistry and Microbiology of Ghent University. All species were selected because they were among the most abundant bacterial taxa found in the microbiome sensu lato of specimens of at least one cryptic species of L. marina in the field (Derycke et al., 2016). Liquid cultures were prepared by isolating single colonies from the stock plates, which were then inoculated in sterile marine broth (Difco) in a 15-ml test tube. The cultures were shake-incubated for 96 h at 180 rpm and 37°C. Prior to this, several test runs and measurements were conducted using a Bio UV visible spectrophotometer at OD600 (optical density at 600 nm) to assess the bacterial growth timing. By monitoring the rate of increase in OD600, we were able to identify the exponential growth phase of the bacterial cultures. Bacterial cell densities were adjusted to 1,000 FAU (Formazin Absorption Units; ISO 7027, 1999), corresponding to an approximate cell density of 109–1010 cells ml–1 for each bacterial strain. Considering that OD 600 values are dependent on the size and shape of bacterial cells, and since the bacterial strains used in our experiments differed in cell dimensions, the reported bacterial cell densities represent estimates rather than precise measurements. Bacteria were harvested from the liquid cultures by centrifugation (2,000 rpm; 20-min); the growth medium (supernatant) was removed, and the bacteria (pellet) were washed twice in Artificial Sea Water (ASW; Dietrich and Kalle, 1957) with a salinity of 25 to remove remnants of the growth media and bacterial metabolites, and finally resuspended in ASW. The resulting bacterial suspensions of each species were then diluted in ASW to a density of ∼ 3 × 109 cells ml–1. This bacterial density supports active population growth and individual fitness of L. marina (dos Santos et al., 2008; De Meester et al., 2011).
Three food diversity treatments (henceforth referred to as “low,” “medium,” “high” diversity) were used in our experiments. Low, medium, and high-diversity mixtures consisted of 5, 10, and 15 bacterial strains, respectively. Replicates of a particular diversity treatment were assigned bacteria by a separate random draw of the appropriate number of species from our pool of 25 bacterial strains. Because we were interested in the effect of diversity, we focused on the number of bacterial strains regardless of their identity. For each treatment, culture aliquots of each component bacteria were mixed in such a way that approximately equal cell numbers of all species were present, and added up to a final density of 3 × 109 cells ml–1. A suspension of frozen-and-thawed Escherichia coli (strain K12), a known suitable food source for L. marina (Moens and Vincx, 1998; dos Santos et al., 2008; De Meester et al., 2011), was used as a positive control.
Experimental Design
A summary of the experimental design for our study is presented in Figure 1.
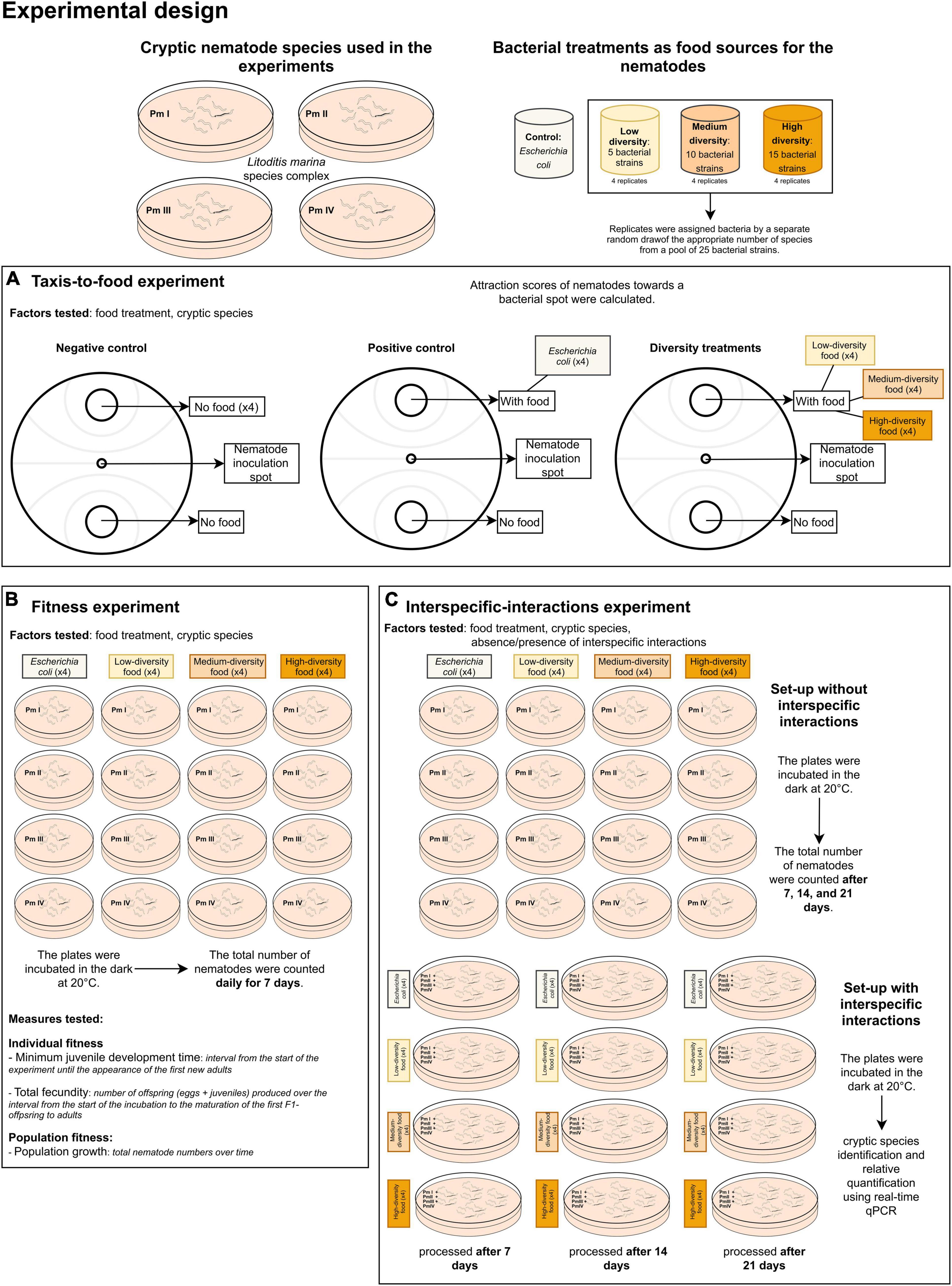
Figure 1. Summary of the experimental design showing the (A) cryptic species of L. marina and the food (bacteria) treatments used in the experiments including the set-ups for the (B) taxis-to-food, (C) fitness, and (D) interspecific interactions experiments.
Effect of Resource Diversity on Taxis to Food
To study the effect of resource diversity on taxis to food of the cryptic species of L. marina, Petri dishes (9 cm i.d.) filled with 18 ml of a 1% bacto agar were used as microcosms. Two excavations (1.5 cm diameter) were made on opposite sides of the plates at equal distance from the center; these were bottom-covered with 250 μl of agar to avoid the dispersion of test suspension underneath the surrounding agar medium (Monteiro et al., 2018). One excavation was then further filled with 150 μl of a bacterial suspension (in Artificial Sea Water) of a particular diversity level (low, medium, high) or with frozen-and-thawed Escherichia coli (strain K12) as a positive control, while the opposite spot did not have any food and was only inoculated with Artificial Sea Water (ASW). An additional treatment without any bacterial spot but with two spots of ASW was used as a negative control.
50 J4/adult nematodes were manually picked and transferred to a 20-μl drop of sterile ASW in the center of the plate. Prior to this transfer, the nematodes were bathed in ASW for 2 h to remove most adhering bacteria. The ASW drop in which nematodes had been inoculated was allowed to evaporate for 15 min under a laminar flow because the nematodes were otherwise often unable to escape its surface tension. The plates were then incubated in the dark at room temperature.
The scoring scheme illustrated in Figure 2 (Monteiro et al., 2018) was utilized to calculate an attraction score of the nematodes toward a bacterial spot. This scheme was modified from Grewal and Wright (1992) and Rodger et al. (2004) to consider the bipolar design and round shape of plates. After 5 h, the total numbers of nematodes per section on the plate were recorded and multiplied by the corresponding section score. These values were summed and divided by the total number of nematodes to obtain one score per plate. This gave an attraction score ranging from –5.0 (complete repulsion: all individuals are in the control (= ASW) spot) to 5.0 (complete attraction: all individuals are in the bacterial spot). The higher the score, the more the nematodes were attracted to the food spot. Four replicates were used for each food diversity treatment and for the controls.
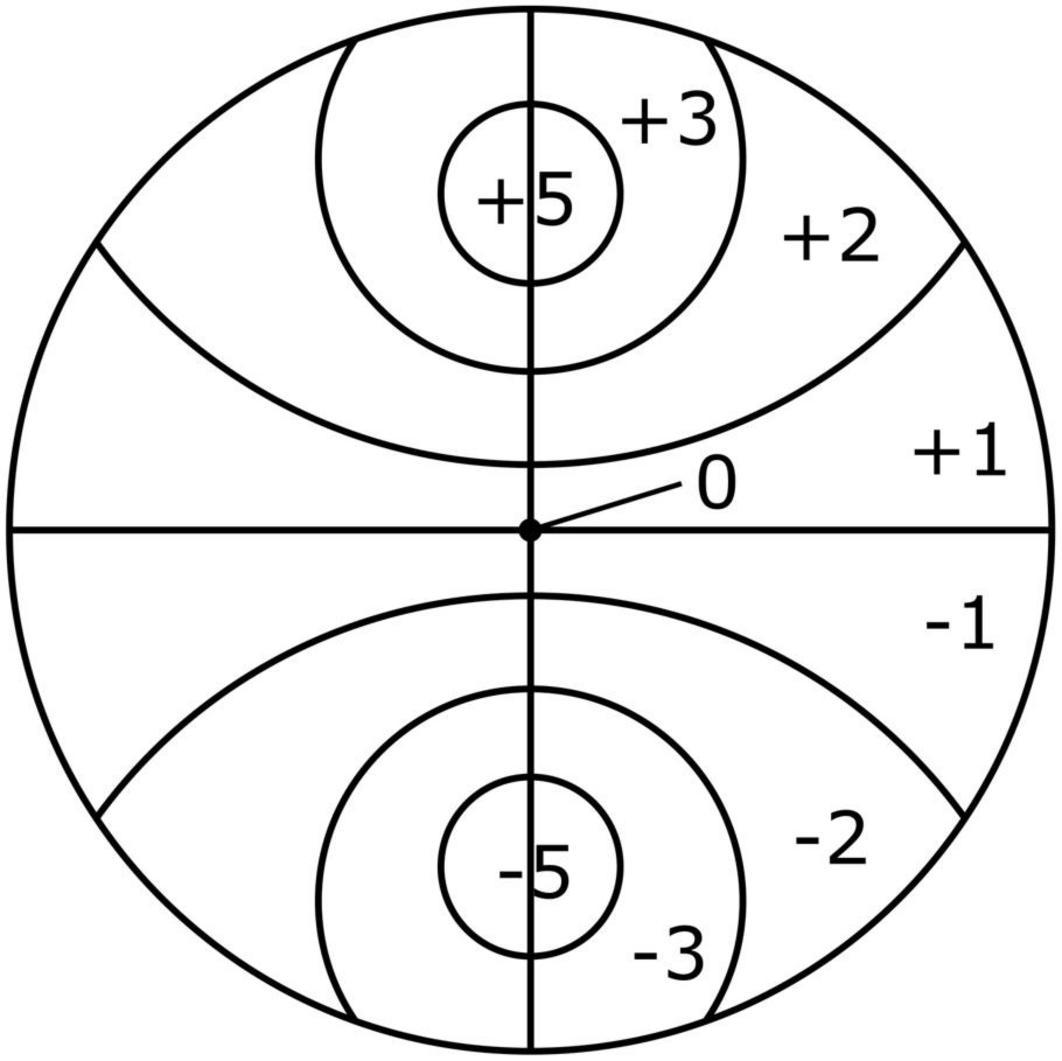
Figure 2. Scoring plate used to investigate the effects of resource diversity on taxis-to-food of the four cryptic species of L. marina (Monteiro et al., 2018).
Effect of Resource Diversity on Individual and Population Fitness
Minimum juvenile development time and total fecundity were used as measures of individual fitness. Minimum juvenile development time was taken as the interval from the occurrence of the first juveniles until the appearance of the first F1-adult (De Meester et al., 2012). Minimum development time, the interval from the start of the incubation until the appearance of the first new adults, is often used as a measure for individual fitness (Moens and Vincx, 2000). But since the reproductive strategy of L. marina varies from oviparous to viviparous depending on cryptic species and environmental conditions, we used minimum juvenile development time here instead of minimum development time (De Meester et al., 2015a). Total fecundity, which refers to the number of offspring (eggs + juveniles) produced over the interval from the start of the incubation to the maturation of the first F1-offspring to adults, was also used as a measure of individual fitness (Moens and Vincx, 2000). This is a conservative estimate of total fecundity, given that a) females might have already deposited some eggs/juveniles prior to their incubation into our experiment, and b) a (very) limited egg production may still occur beyond the day of the maturation of the first F1-offspring to adults. Total nematode numbers over time were used to analyze population growth, which was used as a measure of population fitness (De Meester et al., 2015a).
To test the effect of resource diversity on individual and population fitness of the cryptic species of L. marina, monospecific cultures were reared in Petri dishes (5 cm i.d.) with 4 ml of 1% bacto agar medium prepared with ASW with a salinity of 25 (pH buffered at 7.5–8 with TRIS–HCl in a final concentration of 5 mM). The monospecific cultures were inoculated with five adult males and five young reproductive females of a single cryptic species. Nematodes were manually picked from the stock cultures, washed in ASW (salinity of 25), and placed randomly on the Petri plates. 50-μl aliquots of bacterial suspension of the different food-diversity treatments were spread over the agar surface. Each food treatment (E. coli, low diversity, medium diversity, and high diversity) was replicated four times for each cryptic species, with replicates of a diversity level again being composed of randomly drawn bacterial strains. Total numbers of adults and juveniles were counted daily for a period of 7 days, which corresponds to at least one and at most two generations in all the treatments and species (De Meester et al., 2015a).
Effect of Resource Diversity on Interspecific Interactions
Monospecific and multispecies experiments were conducted to test whether resource diversity had an effect on interspecific interactions. The monospecific set-up was prepared as in the fitness experiment with five adult males and females of a single cryptic species in each Petri dish (5 cm i.d.). The multispecies experiments were performed by simultaneously inoculating all four cryptic species of L. marina in an additive design, resulting in a total number of 40 inoculated nematodes per microcosm. The multispecies experiments were prepared as the monospecific cultures, except for the use of Petri dishes with an inner diameter of 9 cm, filled with 15 ml of agar medium, and provision of 200 μl of bacterial food suspension, thus providing a similar amount of space and food per inoculated individual as in the monospecific experiments. Each food-diversity treatment and control (E. coli) was replicated four times for each cryptic species. All plates were incubated in the dark at a constant temperature of 20°C. The total numbers of nematodes in monospecific cultures were counted after 7, 14, and 21 days of incubation. In multispecies experiments, three independent sets of microcosms were made to analyze the cryptic species composition at the same three moments in time, because microcosms had to be sacrificed for identification of the cryptic nematode species. After counting the total numbers of adults and juveniles at each time point, cryptic species identification and relative quantification were performed using real-time qPCR of the ribosomal internal transcribed spacer (ITS) region which showed the best level of variation to develop species-specific primers (Derycke et al., 2012). For the qPCR analysis, all nematodes were extracted from the agar medium by melting it in hot distilled water (70°C) and sieving over sieves with mesh sizes of 125 μm and 32 μm to separate adults from juveniles (De Meester et al., 2014; Guden et al., 2018). Genomic DNA (gDNA) was prepared separately for each size fraction using hexadecyltrimethylammonium-bromide (CTAB) extraction (protocol based on Derycke et al., 2012). The amount of gDNA was measured using a NanoDrop 2000, and concentrations above 10 ng μl–1 were diluted to avoid interference of high concentrations of gDNA with the quality of the qPCR. Quantification of the relative abundances of each cryptic species was performed using the adjusted ΔΔCT method (Derycke et al., 2012).
Data Analyses
Parametric tests (ANOVA) were used where possible and non-parametric tests (Kruskal-Wallis for univariate analyses/PERMANOVA for multivariate analyses) were conducted when the assumptions for their parametric alternatives were not met even after transformation. PERMANOVA (Permutation based Multivariate Analysis of Variance; Anderson, 2001) was performed using the Adonis function (Vegan package, Oksanen et al., 2020) based on Euclidean distances with 999 permutations. PERMDISP (Permutational Analysis of Multivariate Dispersions) was always used in conjunction with PERMANOVA to test the homogeneity of multivariate dispersions, thus checking if the observed effect was not the result of variation among the replicates (Anderson, 2004). A post hoc Tukey’s honest significant differences (HSD) test was used for the parametric ANOVA, while pairwise Wilcoxon rank-sum test was conducted for the non-parametric Kruskal-Wallis. Significant factors and interactions for PERMANOVA tests were investigated using posterior pairwise comparisons and p-values were corrected for multiple testing with the Bonferroni method. All statistical analyses were conducted using R (R Core Team, 2021).
Effect of Resource Diversity on Taxis to Food
A two-way PERMANOVA test was performed on the attraction scores of the nematodes as the dependent variable and with two fixed factors: species (4 levels: Pm I, Pm II, Pm III, and Pm IV) and treatment (5 levels: no-food control, E. coli, low diversity, medium diversity and high diversity). To investigate the effect of resource diversity in more detail, one-way ANOVA (for Pm I and Pm IV) and the non-parametric alternative (Kruskal-Wallis test; for PM II and Pm III) were conducted for each species separately.
Effect of Resource Diversity on Individual Fitness
Minimum Juvenile Development Time
A two-way ANOVA test was conducted on the minimum juvenile development time with factors: species (4 levels: Pm I, Pm II, Pm III, and Pm IV) and food treatment (4 levels: E. coli, low diversity, medium diversity and high diversity) to determine the effects of each of these factors and their interaction on the minimum juvenile development time of the four cryptic species of L. marina. Within-species analyses were performed using one-way ANOVA tests per species.
Total Fecundity
A two-way ANOVA test was performed on the square-root transformed data of total fecundity with the same two factors as under a). Within-species analyses were conducted using one-way ANOVA to test the effect of food treatment on the total fecundity within a single species of L. marina.
Effect of Resource Diversity on Population Fitness
The effect of resource diversity on the abundances of nematodes for 7 days was analyzed separately for adults and juveniles using Generalized Estimated Equation models in R (GEEGLM) (Zuur et al., 2009; De Meester et al., 2015a) because of the dependency of counts from different time moments. The most appropriate model to describe the population growth curves of both adults and juveniles was selected using Quasilikelihood under the Independence model Criterion (QIC) (Pan, 2001). In this model, the number of nematodes was the dependent variable, treatment and species were fixed factors, and time was entered as a covariable. A log-transformation of the numbers of adults and juveniles was used to account for the non-linearity of the data. Information about the dependence of the data was added to the model in the “id” argument to incorporate time-repeated measurements. Non-significant variables were eliminated from the model until a good model was attained. The model with the smallest QIC was chosen as the preferred model. The residuals were plotted against the fitted values, checking that there were no non-random patterns to validate the model. Pairwise comparisons were conducted by dummy coding in the model.
Effect of Resource Diversity on Interspecific Interactions
To test whether resource diversity had an effect on interspecific interactions, a fictitious assemblage was first “constructed” using the data of the monospecific set-up for the interspecific interaction experiment after 7, 14, and 21 days. Here, the total number of nematodes was summed to create four “fictitious” assemblages for every treatment, which reflect nematode composition without interspecific interactions. These were compared to the “real” assemblages based on the data from the multi-species cultures where interspecific interactions were present (De Meester et al., 2011, 2016). For this purpose, a three-way PERMANOVA test was conducted on the relative abundances of the cryptic species with factors: time (3 levels: 7, 14, and 21 days), food treatment (4 levels: E. coli, low-diversity, medium-diversity and high-diversity food), and absence/presence of interspecific interactions (2 levels: fictitious and real). A similar analysis was also performed using a three-way ANOVA test on the log-transformed data of the absolute abundances of nematodes. Separate analyses were conducted for adult and juvenile nematodes both for relative and absolute abundances. Posterior pairwise comparisons within PERMANOVA were performed for significant factors which influenced the relative abundances of L. marina. A Tukey Honest Significant Differences test was performed to assess the pairwise significant differences within a significant factor for the ANOVA test on the absolute abundances of nematodes.
Results
Effect of Resource Diversity on Taxis to Food
Treatment, species, and the interaction between these factors showed significant effects on taxis to food of the four cryptic species of L. marina (all p = 0.001; Figure 3). No significant pairwise differences for the interaction factor remained after Bonferroni correction (all p > 0.05). Higher attraction of nematodes was observed toward all bacterial spots (E. coli, low, medium, high diversity) compared to the negative control (no food) (all p < 0.05). In addition, the nematodes showed higher taxis to medium food diversity compared to E. coli (p = 0.0007), low (p = 0.0007) and high-diversity food treatments (p = 0.01). We also observed significantly higher taxis to food of Pm IV compared to Pm I (p = 0.043) and Pm II (p = 0.001). Within-species analyses further revealed significantly higher attraction of each cryptic species except Pm I toward a medium-diversity bacterial mix compared to the other food treatments (all p < 0.05; Pm I: p = 0.447). In Pm III, high-diversity food also yielded significantly higher attraction than E. coli and low-diversity food (both p < 0.05), and a higher taxis was observed to E. coli as a food source compared to the low-diversity food treatment (p = 0.029).
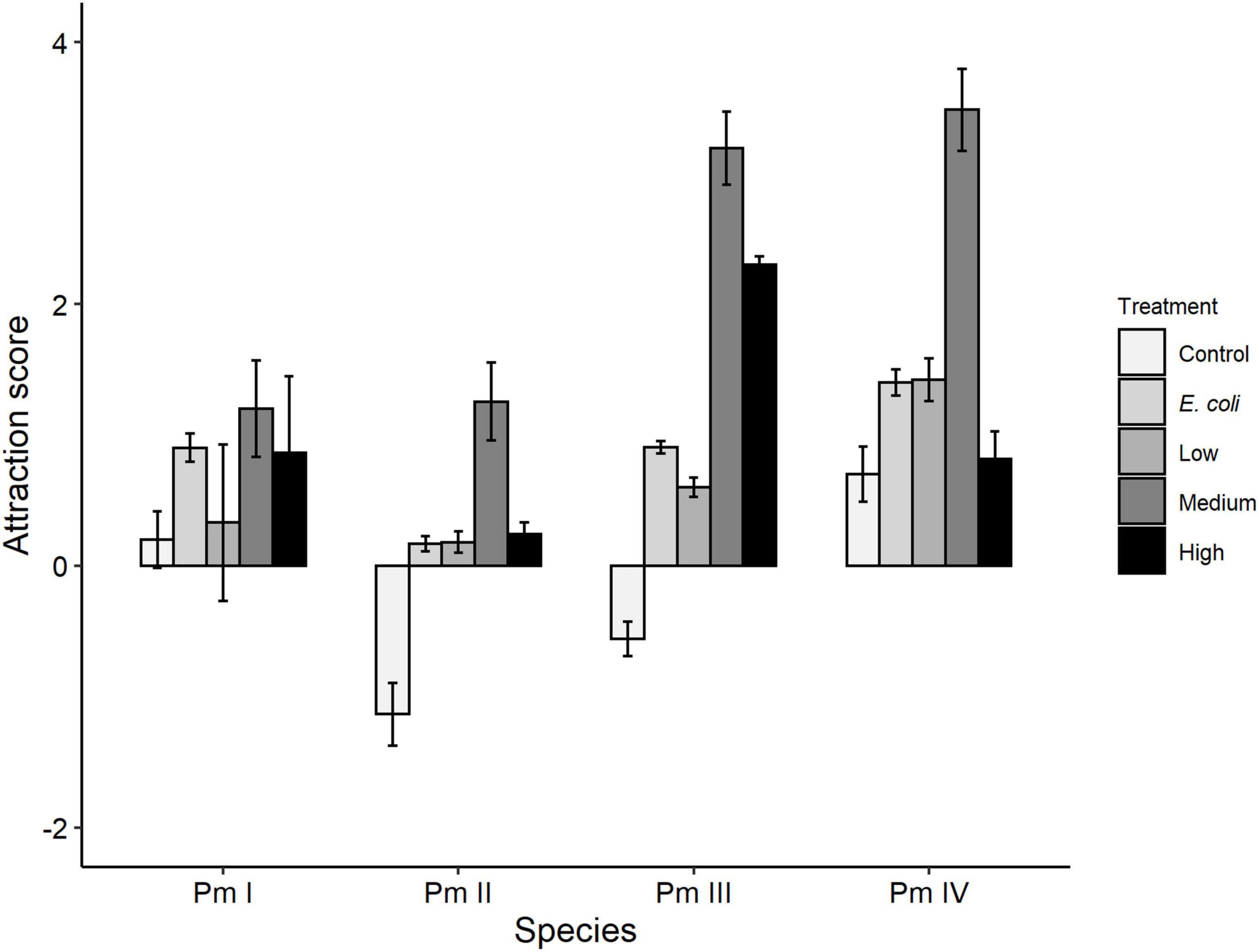
Figure 3. Taxis-to-food scores (mean ± SE) for the four cryptic species of L. marina (Pm I, Pm II, Pm III, and Pm IV) in the control (no food) and different food treatments (E. coli, low diversity, medium diversity, high diversity) (n = 4).
Effect of Resource Diversity on Individual and Population Fitness
Minimum Juvenile Development Time
There was no significant interaction effect of food treatment x species (p > 0.05) on minimum juvenile development time. By contrast, minimum juvenile development time differed significantly among food treatments (p = 0.0008) but not among nematode species (p > 0.05; Figure 4). All cryptic species except Pm II showed a similar trend of lower minimum juvenile development time in medium and high-diversity food treatments compared to the E. coli treatment (all p < 0.05). However, within-species analyses showed that such differences were only significant for Pm IV, which exhibited significantly lower minimum juvenile development time in low (p = 0.05), medium (p = 0.002) and high-diversity treatments (p = 0.002) compared to E. coli.
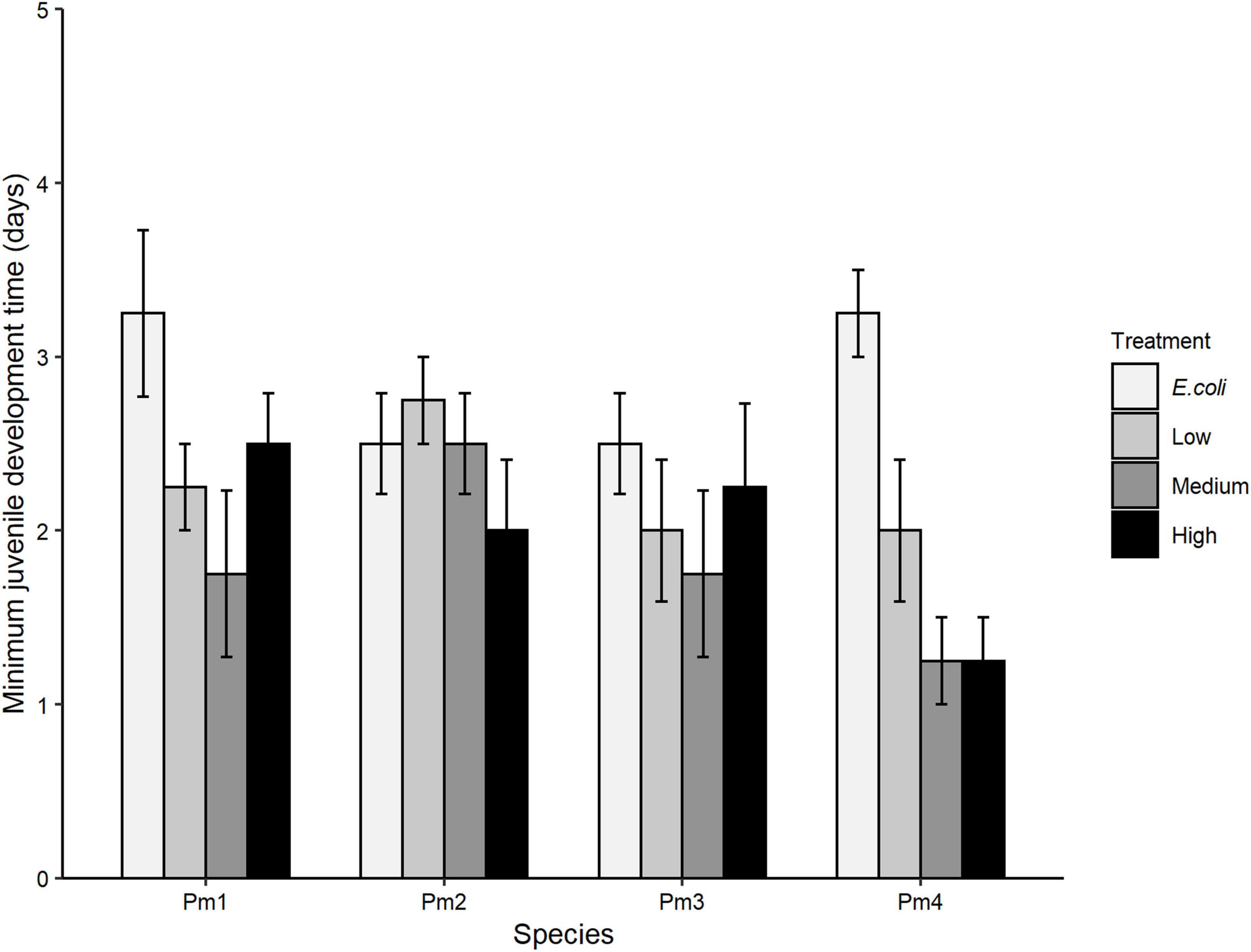
Figure 4. Minimum juvenile development time (mean ± SE) for the four cryptic species of L. marina (Pm I, Pm II, Pm III, and Pm IV) in different food treatments (E. coli, low diversity, medium diversity, and high diversity) (n = 4).
Total Fecundity
Treatment, species, and the interaction between these factors showed significant effects on the total fecundity of the cryptic species of L. marina (all p < 0.05; Figure 5). Pm I had the lowest total number of offspring and Pm III the highest. The significant interaction between treatment and species showed that the total fecundity of Pm III was significantly higher compared to Pm I in all food treatments, but only higher compared to Pm II and Pm IV in the high-diversity treatment (all p < 0.05). Furthermore, Pm II had a significantly lower total fecundity in the E. coli treatment compared to Pm III (p = 0.002), but a higher total fecundity compared to Pm I in low-diversity (p = 0.03), medium-diversity (p = 0.009) and high-diversity food treatments (p = 0.002). Within-species analyses showed that food treatment had significant effects on the total fecundity of Pm I (p = 0.02) which exhibited significantly higher fecundity at high food diversity compared to low food diversity (p = 0.02). The total fecundity Pm III was also influenced by food treatment, which was significantly higher in high-diversity compared to E. coli (p = 0.005), low-diversity (p = 0.0006) and medium-diversity food treatments (p = 0.01). No significant effect of food treatment was found on the total fecundity of Pm II and Pm IV (both p > 0.05).
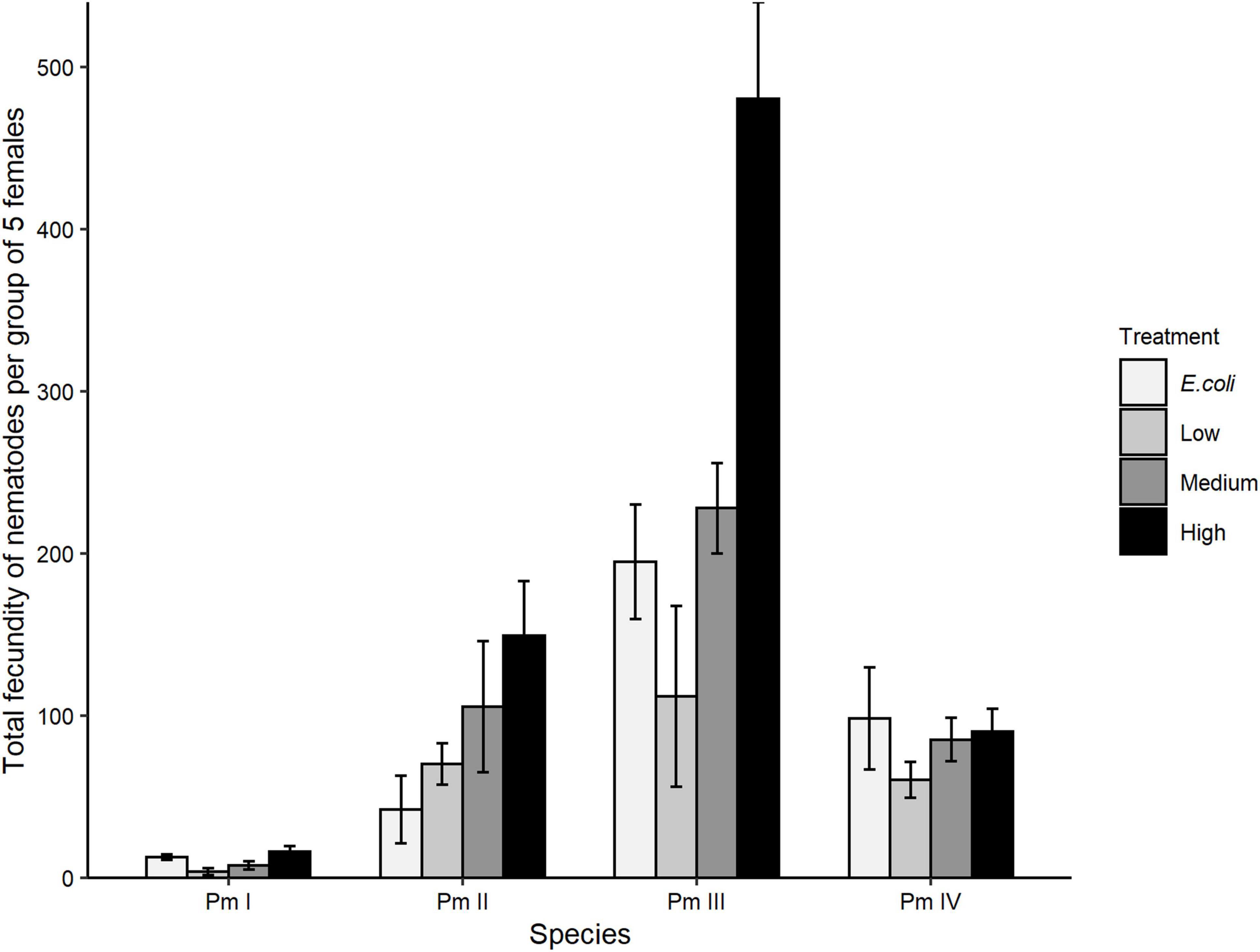
Figure 5. Total fecundity: total number of offspring (eggs + juveniles) produced over the interval from the start of the incubation to the maturation of the first F1-offspring to adults (mean ± SE) for the four cryptic species of L. marina in different food treatments (E. coli, low diversity, medium diversity, and high diversity) (n = 4).
Population Growth
The population growth of nematodes during the first 7 days of incubation was significantly influenced by the interaction of time, treatment and species, as well as by all three individual factors (Table 1). Abundances of adults and juveniles followed very similar trends which showed treatment and species-specific increases of nematodes over time; here we only present the effect of resource diversity on the population growth of adults (Figure 6). In general, all species performed better at high food diversity compared to the low-diversity and E. coli treatments. These differences were significant for Pm I (both p ≤ 0.001) and Pm IV (both p ≤ 0.05). In addition, Pm II performed better at low, medium and high-diversity food treatments compared to E. coli (all p < 0.05). Pm III exhibited significantly higher population growth at a more diverse food source compared to E. coli (all p < 0.05), while Pm IV performed best at high-diversity food compared to all the other food treatments (all p < 0.05).
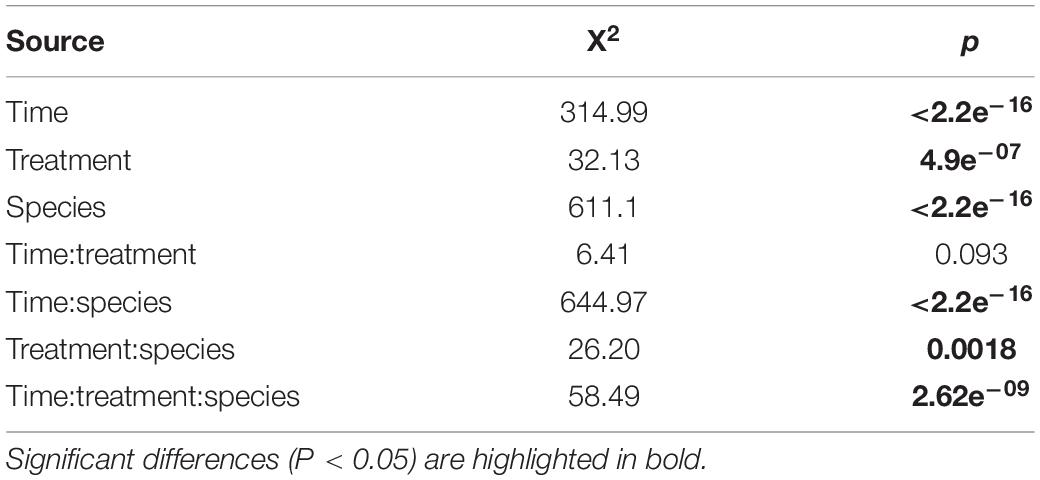
Table 1. Results of the Wald statistic of the GEEGLM: Effect of treatment (E. coli, low, medium, and high) and species (Pm I, Pm II, Pm III, and Pm IV) on the total number of adults over time.
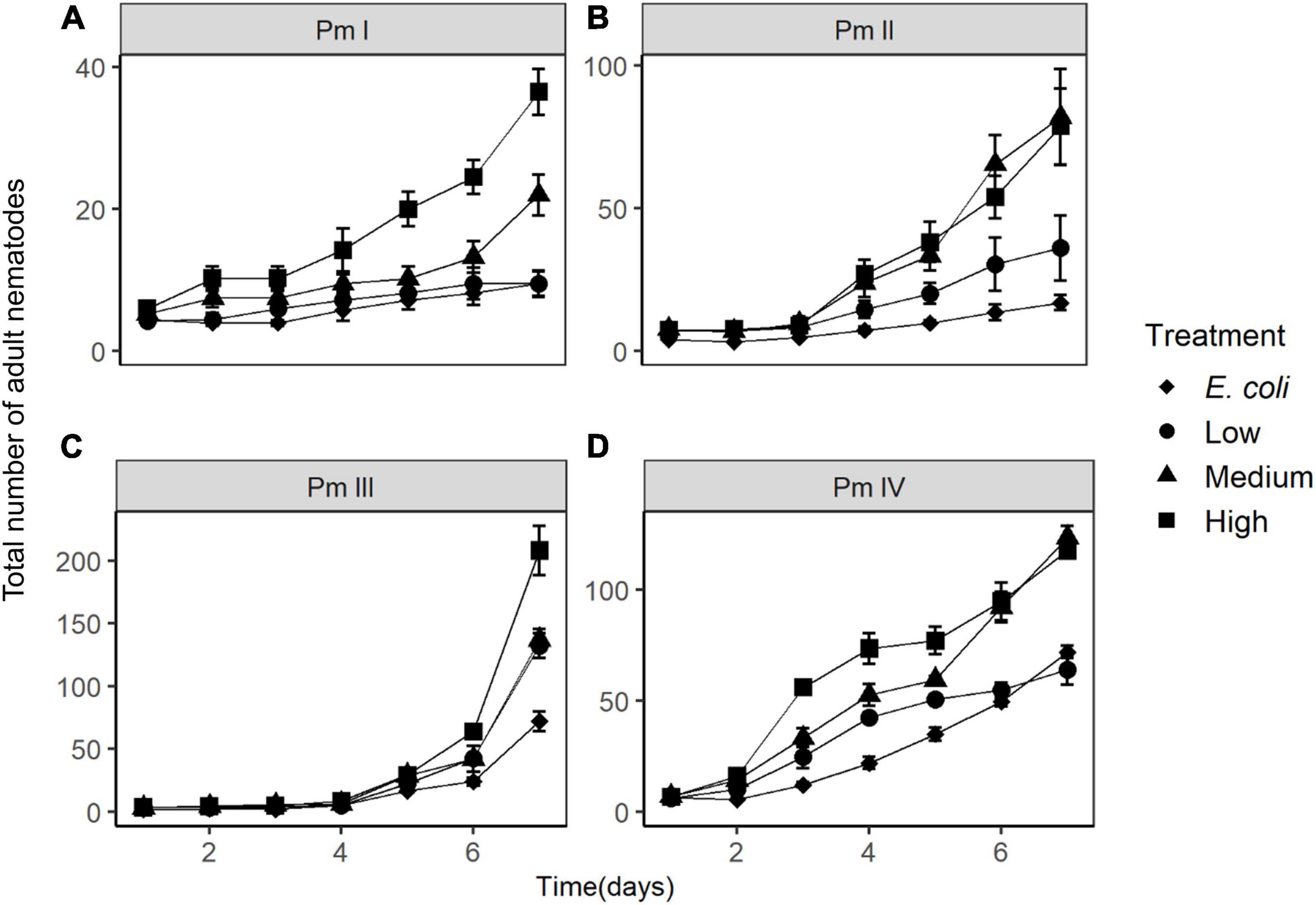
Figure 6. Total number of adult nematodes over time (mean ± SE) in different food treatments (E. coli, low diversity, medium diversity, and high diversity) for the four cryptic species of L. marina: (A) Pm I, (B) Pm II, (C) Pm III, and (D) Pm IV (n = 4). Note the different y-axis scales in the panels.
Effect of Resource Diversity on Interspecific Interactions
The cryptic species composition of L. marina in real and fictitious assemblages at every time moment showed very similar patterns for adults and juveniles. Here, we only present the results for adults, which demonstrated that the relative abundances of nematodes were influenced by the interplay between time and interspecific interactions (p = 0.001), and between resource diversity and interspecific interactions (p = 0.002), as well as by the separate effects of time, interspecific interactions and resource diversity (all p < 0.005) (Table 2 and Figure 7).
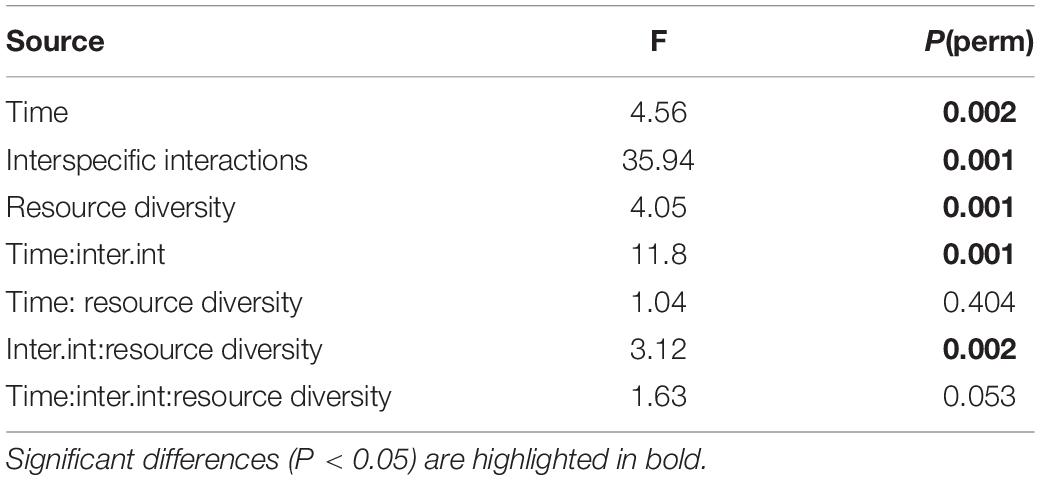
Table 2. PERMANOVA results from the analysis of relative abundances of Pm I, Pm II, Pm III, and Pm IV as a function of time, resource diversity and interspecific interactions.
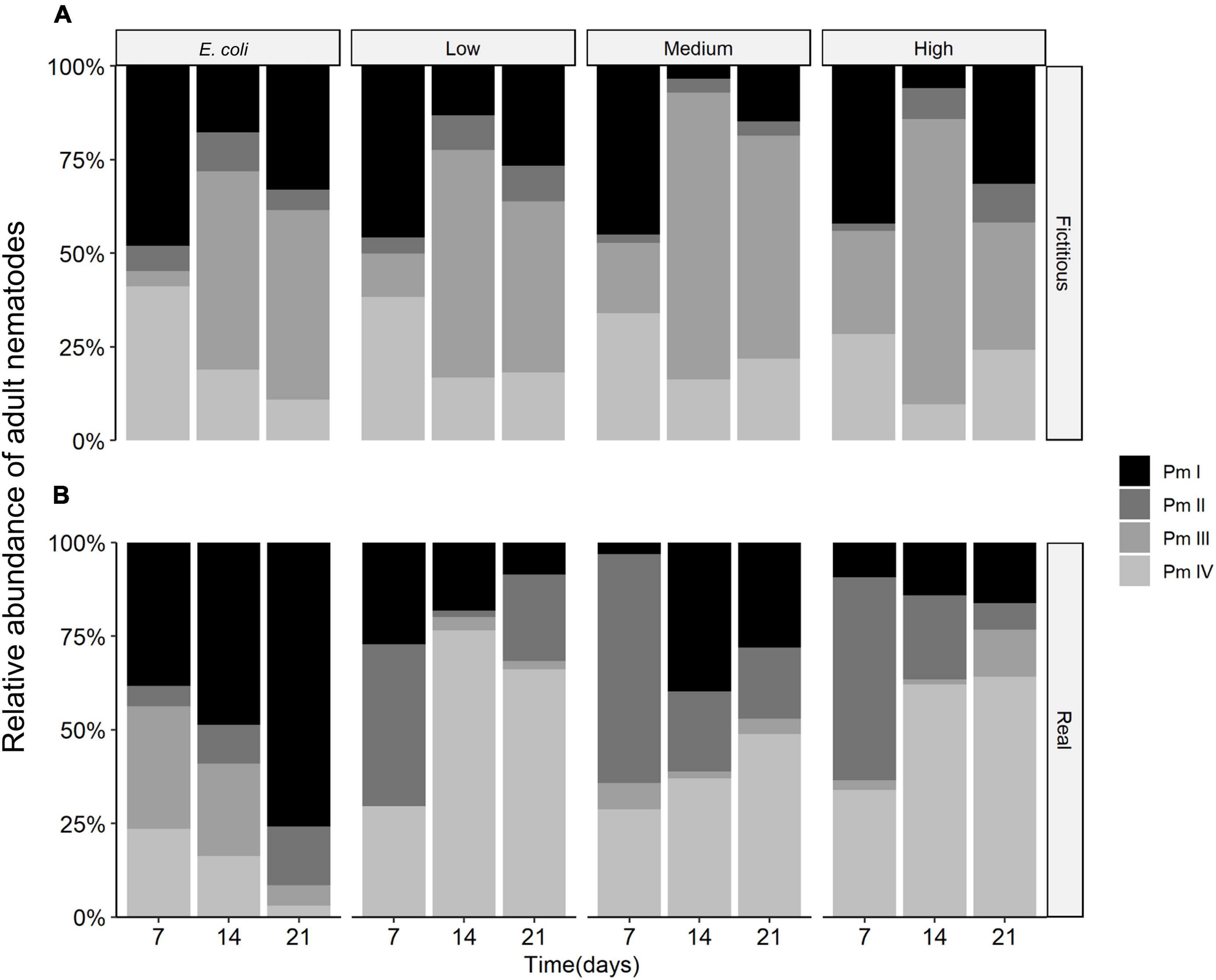
Figure 7. Average relative abundances of adult nematodes in (A) fictitious (based on the results of the monospecific cultures) and (B) real multispecies cultures at each time moment (7, 14, and 21 days) in different food treatments (E. coli, low diversity, medium diversity, and high diversity) (n = 4).
Both Pm I and Pm III showed higher relative abundances than the other two cryptic species in both fictitious and real assemblages with E. coli as a food source. Pm III also exhibited high relative abundances in fictitious assemblages in low, medium and high-diversity food treatments, yet, it showed the lowest relative abundances among all the cryptic species in real assemblages, i.e., in the presence of interspecific interactions, in all food treatments except the one with E. coli. By contrast, Pm II showed higher relative abundances in the two most diverse food treatments of the (real) multispecies experiment than what could be expected based on monospecific cultures. Pm IV also showed high relative abundances in the presence of interspecific interactions, exhibiting the highest relative abundances of all cryptic species in low and high-diversity treatments. In the medium-diversity food, all cryptic species except Pm III performed well in real multispecies cultures.
Like species composition, the absolute abundances of adults of all nematode species were significantly affected by time, interspecific interactions, resource diversity, and the two-way interactions between these factors (all p < 0.05; Table 3 and Figure 8). Higher absolute nematode abundances were found in fictitious (summed monospecific) than in real assemblages in all food-diversity treatments (all p < 0.05). The lowest abundances were observed with E. coli as the food source, whereas the highest abundances were found in the low-diversity food treatment (all p < 0.05) both in fictitious and real assemblages. The absolute abundances of nematodes were also significantly higher in the high-diversity compared to the medium-diversity food treatment (p = 0.007) in real (multispecies) but not in fictitious assemblages (both p > 0.05).
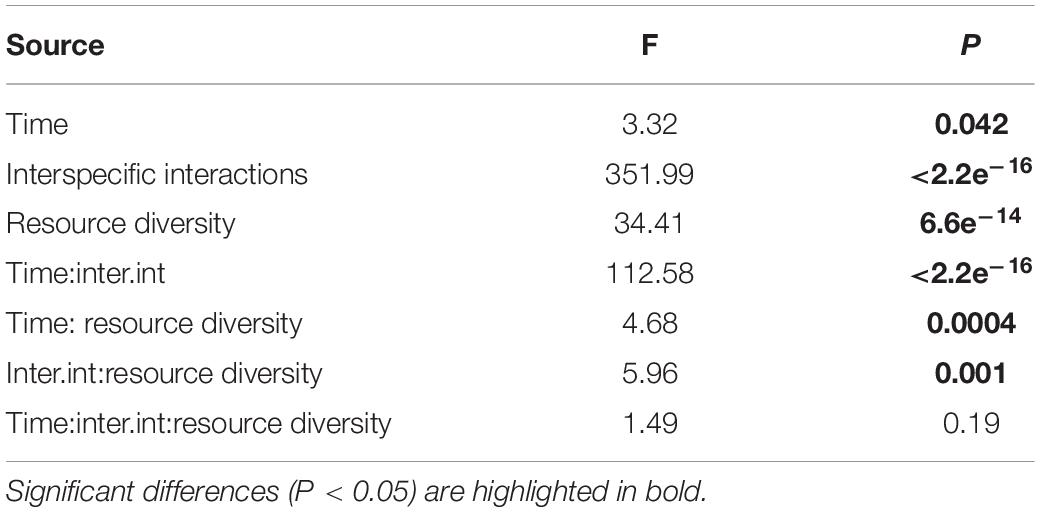
Table 3. ANOVA results from the analysis of absolute abundances of adult nematodes as a function of time, resource diversity and interspecific interactions.
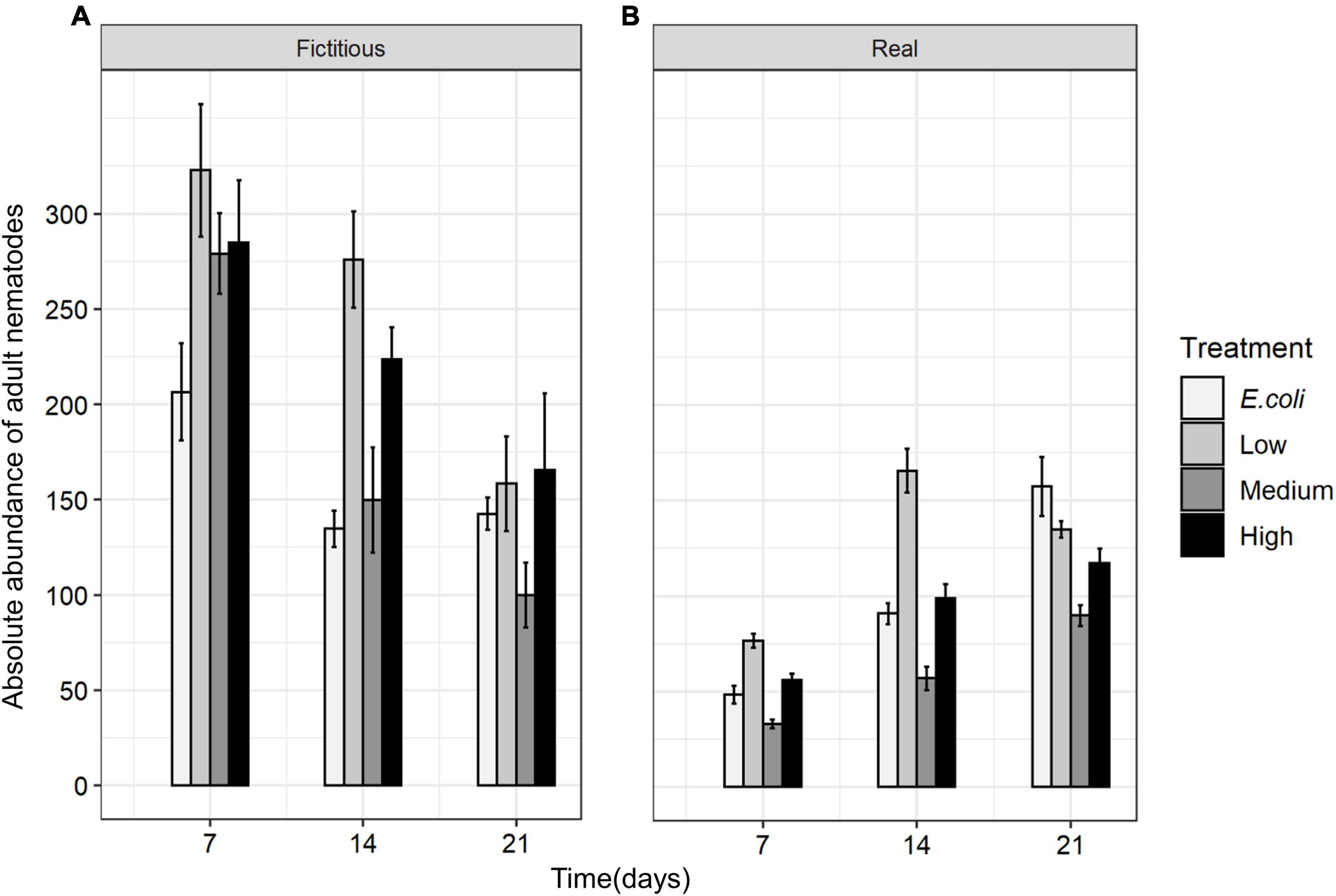
Figure 8. Total absolute abundances of adult nematodes in (A) fictitious (summed based on the results of the monospecific cultures) and (B) real multispecies cultures at each time moment (7, 14, and 21 days) in different food treatments (E. coli, low diversity, medium diversity, and high diversity) (n = 4).
Discussion
In this study, we show that food resource diversity may promote the coexistence of the cryptic species of L. marina through its differential effects on taxis to food and fitness of nematodes and its impact on interspecific interactions. A summary of the results for each cryptic species can be found in Table 4.
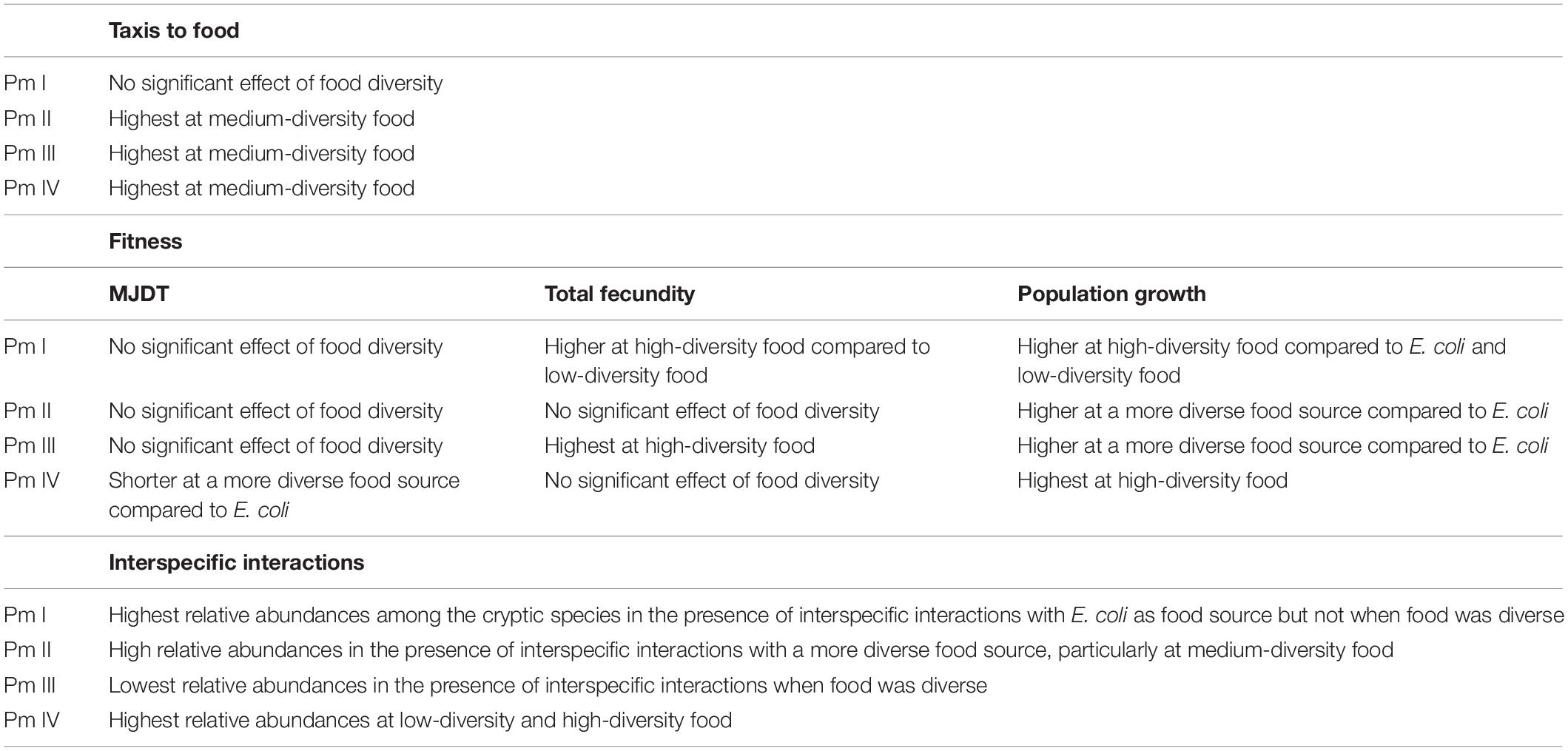
Table 4. Summary of the results for taxis-to-food, individual and population fitness, and interspecific interactions for each cryptic species of L. marina.
Differential Responses of Closely Related Nematode Species to Different Levels of Resource Diversity
To our knowledge, the present study is the first to demonstrate taxis to food of nematodes using mixtures of bacterial strains at different levels of diversity. All four cryptic species consistently showed their highest attraction toward the medium-diversity food bacterial mixture, and in three of the four (Pm II, Pm III, and Pm IV), their chemotaxis at this food resource diversity significantly exceeded that toward E. coli and both the low-diversity and high-diversity food. Pm III also exhibited preference toward high-diversity food compared to the E. coli and low-diversity food treatments (Figure 3). These results are particularly striking because different replicates of any given diversity treatments were composed of different combinations of bacterial strains, underscoring the importance of the observed diversity effect. Different bacterial mixtures may elicit distinct bacterial quorum sensing signals and/or produce different types or concentrations of attractants, which may influence the sensory ability of nematodes (Grewal and Wright, 1992; Köthe et al., 2003; Beale et al., 2006). However, in nature, nematodes are exposed to a range of microbiota growing in close proximity to each other and hence to complex mixtures of infochemicals produced by bacteria and other microbial organisms. In this respect, it is relevant to note that the freshwater nematode Bursilla monohystera was attracted to cyanobacterial biofilms and to some of the volatile organic compounds (VOC) they produce. Bursilla monohystera did not respond to any single VOC but a mixture of VOC did elicit a pronounced chemotaxis of the nematode (Höckelmann et al., 2004). Nematodes can sense various signals through several olfactory sensory neurons (Bargmann and Horvitz, 1991; Bargmann et al., 1993), which we hypothesized may function optimally under conditions where a sufficient diversity of stimuli is present, but suboptimally when too large a mixture of slightly different stimuli has to be “processed”. If so, this might explain why cryptic L. marina species were more attracted to mixtures of 10 bacterial strains than to lower and higher-diversity mixtures. Another interesting aspect which remains largely unexplored is the relationship between marine nematodes and fungi, and the potential of the latter as food sources and/or as attractants for nematodes. Although fungal-feeding nematodes are lacking in marine nematode benthic assemblages, except for some algae-associated Aphelenchoididae (Moens et al., 2013), there is evidence from barcoding studies of some association between fungi and marine nematodes (Bhadury et al., 2009, 2011; Bhadury and Austen, 2010) indicating that a fungal microbiome indeed exists in these organisms.
But did the observed taxis-to-food patterns concur with any fitness responses of these nematodes to resource diversity? We evaluated both population-level and individual nematode fitness, the latter on the basis of two traits which both contribute to the rate of population increase, i.e., minimum juvenile development time and fecundity. In general, there was a trend of shorter juvenile development time (Figure 4) and higher fecundity (Figure 5) at high and medium-diversity food; however, only some of these effects were statistically significant (Table 4). In addition, we observed a higher fecundity of Pm III compared to the other cryptic species, in accordance to De Meester et al. (2015a), yet, our study revealed that this was more pronounced in the high-diversity food treatment.
At the population level, each bacterial mixture supported population growth of the nematodes (preliminary analyses also showed that the individual bacterial strains can support nematode growth), but a more diverse food source enhanced the population growth of L. marina, especially for Pm I and Pm IV (Table 4 and Figure 6). Pm II and Pm III exhibited a similar response, but performed better in all bacterial mixtures (low, medium and high-diversity food) than on the single-strain resource E. coli. Our findings appear to corroborate the “balanced diet” hypothesis, which suggests that a more diverse resource offers a more complete and/or complementary range of nutritional resources, leading to a higher production of consumer biomass (DeMott, 1998). By contrast, both the “variance-in-edibility” hypothesis and the “dilution hypothesis” or “resource concentration hypothesis” predict a negative impact on consumers when resource diversity is increased and are supported by a considerable body of evidence (e.g., Andow, 1991; Steiner, 2001; Wilsey and Polley, 2002; Hillebrand and Cardinale, 2004; Duffy et al., 2005), whereas examples of the balanced diet effect of diversity on consumers are more scarce. Nevertheless, several studies have demonstrated enhanced herbivore growth and biomass accumulation with mixed diets of primary producers than with single-species diets (e.g., Pfisterer et al., 2003; Worm et al., 2006). However, in the rapidly changing and ephemeral habitat where L. marina lives, the coexistence of the cryptic species is likely not only determined by resource availability, but also by the responses of the cryptic species and their microbiomes to environmental changes (Derycke et al., 2006; De Meester et al., 2015a) and/or their ability to disperse to suitable patches with more favorable conditions (De Meester et al., 2012), such as a site with high resource diversity.
Differential Effects of Resource Diversity on Interspecific Interactions
Resource diversity can also affect the response of cryptic species to competition. We found that competitive interactions between the cryptic species of L. marina were substantial, as evidenced by the fact that when all four cryptic species were inoculated together, the total abundance of nematodes was lower than the sum of the abundances of the four species in the monospecific experiments (Figure 8). These competitive interaction effects corroborate the findings of previous research (De Meester et al., 2011, 2015b). In addition, the total numbers of nematodes were lower with E. coli as food compared to a more diverse food source both in real and fictitious assemblages, showing that nematode abundances of the mixed species assemblages were also clearly influenced by resource diversity. Based on the principle of competitive exclusion, species occupying the same ecological niche cannot stably coexist due to strong interspecific competition for resources (Volterra, 1926; Gause, 1936). Niche diversification, for instance through resource partitioning, may alleviate competition (Sánchez-Hernández et al., 2017).
Interspecific interactions also had an effect on the species composition of the nematode assemblages. In real assemblages (multi-species experiments), Pm I was the competitively strongest species in the E. coli food treatment in which Pm IV almost became excluded after 21 days. Pm I and Pm IV are the two most closely related cryptic species and they seldom co-occur in the field (Derycke et al., 2006, 2008), so we expected substantial competition between them. The present results also corroborate that when simultaneously inoculated in a homogeneous environment without spatial structure and with E. coli as a food source, Pm I is the strongest competitor, followed by Pm III, while Pm II and Pm IV are poor competitors (Figure 7).
However, the competitive hierarchy among these four species is not fixed. All four species can co-exist for longer time periods when dispersal opportunities are present (spatial structure) (De Meester et al., 2014). In addition, the outcome of the competition depends on the precise species combination and on environmental factors such as salinity and temperature (De Meester et al., 2011, 2015a). The present study demonstrates that resource diversity also affects the composition of assemblages of cryptic L. marina species. In strong contrast with the E. coli treatment, Pm IV exhibited the highest relative abundances of all species in low and high-diversity food treatments in multispecies experiments; Pm I and Pm II also occurred in high abundances. On the other hand, Pm III exhibited the lowest relative abundances at all resource diversity levels except the E. coli treatment in multispecies experiments (real assemblage), although it exhibited the highest relative abundances in fictitious assemblages, demonstrating that this species suffered from interspecific interactions irrespective of resource diversity (Figure 7). Derycke et al. (2016) argued that Pm I and Pm III partly feed on different bacterial strains, and that Pm III is a much less generalist feeder than Pm I. Since monospecific cultures of Pm III performed well on each of the bacterial diets offered here, suitable food for Pm III was sufficiently available in all food regimes. Given the poor performance of Pm III in the low, medium and high-diversity food treatments, other species were likely more successful at utilizing these resources than Pm III. When a competitively dominant species expands its niche, the niche space available for other species is reduced, resulting in selection pressure to the inferior competitor to use whatever resources are left available (Levins, 1968; Jasmin and Kassen, 2007; Pekkonen et al., 2013). In this case, there may be a pronounced effect of competition on selective feeders since their preferred food may become limiting in the presence of other cryptic species, whereas more generalist feeders may be able to minimize competition in a more diverse food environment through enhanced resource partitioning. When resource diversity is high, the competing species may segregate in resource utilization by specialization, which may minimize the negative effects of competition (Sánchez-Hernández et al., 2017).
In the field, the local diversity of marine nematodes is typically high (Heip et al., 1985; Moens et al., 2013). Despite their diversity, quantitative and qualitative information on the feeding ecology of nematodes at the species, genus and even family level, remain scant (Montagna, 1984; Moens et al., 2014; Wu et al., 2019). Marine nematodes have traditionally been classified by the morphology of their stoma, and, to a lesser extent, based on pharyngeal musculature (Wieser, 1953). However, our findings show that even very closely related nematodes with highly similar stoma, such as the cryptic species of L. marina, can have differential responses to resource diversity, which can impact the outcome of interspecific interactions. This indicates that the link between morphology and feeding, although informative, is neither as absolute nor as straightforward as proposed by Wieser’s classification (Moens et al., 2004), which has significant implications from a functional perspective and in understanding how resource partitioning affects the composition and diversity of nematode communities.
Conclusion
We demonstrate that resource diversity may promote the coexistence of different cryptic species of Litoditis marina through its differential effects on taxis to food and on nematode fitness, both at the individual and population level, and its impact on their interspecific interactions. Taxis to food in some species was influenced by resource diversity. In addition, the cryptic species of L. marina generally showed higher fitness on a more diverse food resource. Our results corroborate the “balanced diet” hypothesis, which suggests that a more diverse resource offers a more complete and/or complementary range of nutritional resources. Resource diversity also had an impact on the interspecific interactions of the cryptic species of L. marina, showing that the outcome of competition among these closely related species is highly context-dependent and that the coexistence of closely related species such as L. marina in the field may be mediated among other things by resource diversity.
Data Availability Statement
All data of the study are available in the Integrated Marine Information System (IMIS) database (VLIZ): https://doi.org/10.14284/486.
Author Contributions
RG was heavily involved in all aspects of the present work and performed all the lab work and data analyses. RG and TM conceived this study and its sampling design. TM and SD contributed intensively to the writing of the manuscript. All authors contributed to the article and approved the submitted version.
Funding
RG benefitted from a Ph.D. Scholarship from Ghent University (BOF) during the period of this study. The results of this study were obtained using infrastructure of EMBRC Belgium, funded by the Flemish Science Fund FWO (project G0H3817N). Specific funding for the laboratory experiments was provided by the FWO through project 3G.0192.09 and by Ghent University through GOA-project (17GOA-026).
Conflict of Interest
The authors declare that the research was conducted in the absence of any commercial or financial relationships that could be construed as a potential conflict of interest.
Publisher’s Note
All claims expressed in this article are solely those of the authors and do not necessarily represent those of their affiliated organizations, or those of the publisher, the editors and the reviewers. Any product that may be evaluated in this article, or claim that may be made by its manufacturer, is not guaranteed or endorsed by the publisher.
Acknowledgments
Nele De Meester and Luana Monteiro are gratefully acknowledged for their valuable inputs on the experimental design. Annick Van Kenhove and Annelien Rigaux helped during experimental procedures.
Supplementary Material
The Supplementary Material for this article can be found online at: https://www.frontiersin.org/articles/10.3389/fmars.2021.777425/full#supplementary-material
References
Anderson, M. (2004). PERMDISP: A FORTRAN Computer Program for Permutational Analysis of Multivariate Dispersions (for Any Two-factor ANOVA Design) Using Permutation. Auckland: Department of Statistics, University of Auckland.
Anderson, M. J. (2001). A new method for non-parametric multivariate analysis of variance. Austral Ecol. 26, 32–46. doi: 10.1046/j.1442-9993.2001.01070.x
Andow, D. A. (1991). Vegetational diversity and arthropod population response. Annu. Rev. Entomol. 36, 561–586. doi: 10.1146/annurev.en.36.010191.003021
Bargmann, C. I., and Horvitz, H. R. (1991). Chemosensory neurons with overlapping functions direct chemotaxis to multiple chemicals in C. elegans. Neuron 7, 729–742. doi: 10.1016/0896-6273(91)90276-6
Bargmann, C. I., Hartwieg, E., and Horvitz, H. R. (1993). Odorant-selective genes and neurons mediate olfaction in C. elegans. Cell 74, 515–527. doi: 10.1016/0092-8674(93)80053-H
Barili, E., Agostinho, A. A., Gomes, L. C., and Latini, J. D. (2011). The coexistence of fish species in streams: relationships between assemblage attributes and trophic and environmental variables. Environ. Biol. Fishes 92, 41–52. doi: 10.1007/s10641-011-9814-2
Beale, E., Li, G., Tan, M. W., and Rumbaugh, K. P. (2006). Caenorhabditis elegans senses bacterial autoinducers. Appl. Environ. Microbiol. 72, 5135–5137. doi: 10.1128/AEM.00611-06
Bhadury, P., and Austen, M. C. (2010). Barcoding marine nematodes: an improved set of nematode 18S rRNA primers to overcome eukaryotic co-interference. Hydrobiologia 641, 245–251. doi: 10.1007/s10750-009-0088-z
Bhadury, P., Bik, H., Lambshead, J. D., Austen, M. C., Smerdon, G. R., and Rogers, A. D. (2011). Molecular diversity of fungal phylotypes co-amplified alongside nematodes from coastal and deep-sea marine environments. PLoS One 6:e26445. doi: 10.1371/journal.pone.0026445
Bhadury, P., Bridge, P. D., Austen, M. C., Bilton, D. T., and Smerdon, G. R. (2009). Detection of fungal 18S rRNA sequences in conjunction with marine nematode 18S rRNA amplicons. Aquat. Biol. 5, 149–155. doi: 10.3354/ab00145
Blanc, C., Sy, M., Djigal, D., Brauman, A., Normand, P., and Villenave, C. (2006). Nutrition on bacteria by bacterial-feeding nematodes and consequences on the structure of soil bacterial community. Eur. J. Soil Biol. 42, 70–78. doi: 10.1016/j.ejsobi.2006.06.003
Brown, J. S., Kotler, B. P., and Mitchell, W. A. (1994). Foraging theory, patch use, and the structure of a Negev Desert granivore community. Ecology 75, 2286–2300.
Carta, L. K. (2000). Bacterial-feeding nematode growth and preference for biocontrol isolates of the bacterium Burkholderia cepacia. J. Nematol. 32, 362–369.
De Meester, N., Derycke, S., and Moens, T. (2012). Differences in time until dispersal between cryptic species of a marine nematode species complex. PLoS One 7:e42674. doi: 10.1371/journal.pone.0042674
De Meester, N., Derycke, S., Bonte, D., and Moens, T. (2011). Salinity effects on the coexistence of cryptic species: a case study on marine nematodes. Mar. Biol. 158, 2717–2726. doi: 10.1007/s00227-011-1769-5
De Meester, N., Derycke, S., Rigaux, A., and Moens, T. (2014). Active dispersal is differentially affected by inter- and intraspecific competition in closely related nematode species. Oikos 124, 561–570. doi: 10.1111/oik.01779
De Meester, N., Dos Santos, G. A. P., Rigaux, A., Valdes, Y., Derycke, S., and Moens, T. (2015b). Daily temperature fluctuations alter interactions between closely related species of marine nematodes. PLoS One 10:e0131625. doi: 10.1371/journal.pone.0131625
De Meester, N., Derycke, S., Rigaux, A., and Moens, T. (2015a). Temperature and salinity induce differential responses in life histories of cryptic nematode species. J. Exp. Mar. Bio. Ecol. 472, 54–62. doi: 10.1016/j.jembe.2015.07.002
De Meester, N., Gingold, R., Rigaux, A., Derycke, S., and Moens, T. (2016). Cryptic diversity and ecosystem functioning: a complex tale of differential effects on decomposition. Oecologia 182, 559–571. doi: 10.1007/s00442-016-3677-3
De Meester, N., Van Daele, T., Van Malderen, J., Monteiro, L., Van Colen, C., Derycke, S., et al. (2018). Active and species-specific dispersal behaviour in a marine nematode cryptic species complex. Mar. Ecol. Prog. Ser. 600, 71–83. doi: 10.3354/meps12658
DeMott, W. R. (1998). Utilization of a cyanobacterium and a phosphorus-deficient green alga as complementary resources by daphnids. Ecology 79, 2463–2481.
Derycke, S., Backeljau, T., Vlaeminck, C., Vierstraete, A., Vanfleteren, J., Vincx, M., et al. (2006). Seasonal dynamics of population genetic structure in cryptic taxa of the Pellioditis marina complex (Nematoda: Rhabditida). Genetica 128, 307–321. doi: 10.1007/s10709-006-6944-0
Derycke, S., De Meester, N., Rigaux, A., Creer, S., Bik, H., Thomas, W. K., et al. (2016). Coexisting cryptic species of the Litoditis marina complex (Nematoda) show differential resource use and have distinct microbiomes with high intraspecific variability. Mol. Ecol. 25, 2093–2110. doi: 10.1111/mec.13597
Derycke, S., Remerie, T., Backeljau, T., Vierstraete, A., Vanfleteren, J., Vincx, M., et al. (2008). Phylogeography of the Rhabditis (Pellioditis) marina species complex: evidence for long-distance dispersal, and for range expansions and restricted gene flow in the northeast Atlantic. Mol. Ecol. 17, 3306–3322. doi: 10.1111/j.1365-294X.2008.03846.x
Derycke, S., Sheibani Tezerji, R., Rigaux, A., and Moens, T. (2012). Investigating the ecology and evolution of cryptic marine nematode species through quantitative real-time PCR of the ribosomal ITS region. Mol. Ecol. Resour. 12, 607–619. doi: 10.1111/j.1755-0998.2012.03128.x
Dietrich, G., and Kalle, K. (1957). Allgemeine Meereskunde: eine Einführung in die Ozeanographie, 8th Edn. Berlin: Gebrüder Bornträger.
Donoghue, M. J. (2009). A phylogenetic perspective on the distribution of plant diversity. Light Evol. 2, 247–262. doi: 10.17226/12501
dos Santos, G. A. P., Derycke, S., Fonsêca-Genevois, V. G., Coelho, L. C. B. B., Correia, M. T. S., and Moens, T. (2008). Differential effects of food availability on population growth and fitness of three species of estuarine, bacterial-feeding nematodes. J. Exp. Mar. Bio. Ecol. 355, 27–40. doi: 10.1016/j.jembe.2007.11.015
Duffy, J. E. (2002). Biodiversity and ecosystem function: the consumer connection. Oikos 99, 201–219. doi: 10.1034/j.1600-0706.2002.990201.x
Duffy, J. E., Cardinale, B. J., France, K. E., McIntyre, P. B., Thébault, E., and Loreau, M. (2007). The functional role of biodiversity in ecosystems: incorporating trophic complexity. Ecol. Lett. 10, 522–538. doi: 10.1111/j.1461-0248.2007.01037.x
Duffy, J. E., Richardson, J. P., and France, K. E. (2005). Ecosystem consequences of diversity depend on food chain length in estuarine vegetation. Ecol. Lett. 8, 301–309. doi: 10.1111/j.1461-0248.2005.00725.x
Fridley, J. D. (2001). The influence of species diversity on ecosystem productivity: how, where, and why? Oikos 93, 514–526.
Fridley, J. D. (2003). Diversity effects on production in different light and fertility environments: an experiment with communities of annual plants. J. Ecol. 91, 396–406. doi: 10.1890/13-1666.1
Gansfort, B., Uthoff, J., and Traunspurger, W. (2018). Interactions among competing nematode species affect population growth rates. Oecologia 187, 75–84. doi: 10.1007/s00442-018-4109-3
Gause, G. F. (1936). The struggle for existence. Soil Sci. 41:159. doi: 10.1097/00010694-193602000-00018
Grewal, P. S. (1991). Influence of bacteria and temperature on the reproduction of Caenorhabditis elegans (Nematoda: Rhabditidae) infesting mushrooms (Agaricus Bisporus). Nematologica 37, 72–82.
Grewal, P., and Wright, D. (1992). Migration of Caenorhabditis elegans larvae towards bacteria and the nature of the bacterial stimulus. Fundam. Appl. Nematol. 15, 159–166.
Griffin, J., Haye, K., Hawkins, S., Thompson, R., and Jenkins, S. (2008). Predator diversity and ecosystem functioning: density modifies the effect of resource partitioning. Ecology 89, 298–305. doi: 10.1890/07-1220.1
Guden, R. M., Vafeiadou, A. M., De Meester, N., Derycke, S., and Moens, T. (2018). Living apart-together: microhabitat differentiation of cryptic nematode species in a saltmarsh habitat. PLoS One 13:e0204750. doi: 10.1371/journal.pone.0204750
Heip, C., Vincx, M., and Vranken, G. (1985). The ecology of marine nematodes. Oceanogr. Mar. Biol. Annu. Rev. 23, 399–489.
Hillebrand, H., and Cardinale, B. J. (2004). Consumer effects decline with prey diversity. Ecol. Lett. 7, 192–201. doi: 10.1111/j.1461-0248.2004.00570.x
Hillebrand, H., and Shurin, J. B. (2005). “Biodiversity and aquatic food webs,” in Aquatic Food Webs: An Ecosystem Approach, eds A. Belgrano, U. M. Scharler, J. Dunne, and R. E. Ulanowicz (Oxford: Oxford University Press), 184–198.
Höckelmann, C., Moens, T., and Jüttner, F. (2004). Odor compounds from cyanobacterial biofilms acting as attractants and repellents for free-living nematodes. Limnol. Oceanogr. 49, 1809–1819. doi: 10.4319/lo.2004.49.5.1809
Jasmin, J. N., and Kassen, R. (2007). On the experimental evolution of specialization and diversity in heterogeneous environments. Ecol. Lett. 10, 272–281. doi: 10.1111/j.1461-0248.2007.01021.x
Jiang, T., Feng, J., Sun, K., and Wang, J. (2007). Coexistence of two sympatric and morphologically similar bat species Rhinolophus affinis and Rhinolophus pearsoni. Prog. Nat. Sci. 18, 523–532. doi: 10.1016/J.PNSC.2007.12.005
Johnson, K. H., Vogt, K. A., Clark, H. J., Schmitz, O. J., and Vogt, D. J. (1996). Resilience and stability of ecosystems. Trends Ecol. Evol. 11, 372–377.
Joshi, J., Otway, S. J., Koricheva, J., Pfisterer, A. B., Alphei, J., Roy, B. A., et al. (2004). “Bottom-up effects and feedbacks in simple and diverse experimental Grassland Communities,” in Insects And Ecosystem Function, eds W. W. Weisser and E. Siemann (Berlin: Springer- Verlag), 115–134. doi: 10.1007/978-3-540-74004-9_6
Keesing, F., Holt, R. D., and Ostfeld, R. S. (2006). Effects of species diversity on disease risk. Ecol. Lett. 9, 485–498. doi: 10.1111/j.1461-0248.2006.00885.x
Köthe, M., Antl, M., Huber, B., Stoecker, K., Ebrecht, D., Steinmetz, I., et al. (2003). Killing of Caenorhabditis elegans by Burkholderia cepacia is controlled by the cep quorum-sensing system. Cell. Microbiol. 5, 343–351. doi: 10.1046/j.1462-5822.2003.00280.x
Kotler, B. P., and Brown, J. S. (1988). Environmental heterogeneity and the coexistence of desert rodents. Annu. Rev. Ecol. Syst. 19, 281–307.
Kramer, D. L. (2001). “Foraging behavior,” in Evolutionary Ecology: Concepts and Case Studies, eds C. W. Fox, D. A. Roff, and D. J. Fairbairn (New York, NY: Oxford University Press), 232–246.
Krasnov, B. R., Shenbrot, G. I., Rios, L. E., and Lizurume, M. E. (2000). Does food-searching ability determine habitat selection? Foraging in sand of three species of gerbilline rodents. Ecography (Cop.) 23, 122–129.
Leibold, M. A. (1989). Resource edibility and the effects of predators and productivity on the outcome of trophic interactions. Am. Nat. 134, 922–949.
Levins, R. (1968). Evolution In Changing Environments: Some Theoretical Explorations. Princeton, NJ: Princeton University Press.
MacArthur, R., and Levins, R. (1964). Competition, habitat selection, and character displacement in a patchy environment. Proc. Natl. Acad. Sci. U.S.A. 51, 1207–1210. doi: 10.1073/pnas.51.6.1207
Martin, R. A., and Garnett, S. C. (2013). Relatedness and resource diversity interact to influence the intensity of competition. Biol. J. Linn. Soc. 110, 689–695. doi: 10.1111/bij.12146
Michiels, I. C., and Traunspurger, W. (2005). Impact of resource availability on species composition and diversity in freshwater nematodes. Oecologia 142, 98–103. doi: 10.1007/s00442-004-1700-6
Moens, T., and Vincx, M. (1998). On the cultivation of free-living marine and estuarine nematodes. Helgoländer Meeresunters 52, 115–139.
Moens, T., and Vincx, M. (2000). Temperature, salinity and food thresholds in two brackish-water bacterivorous nematode species: assessing niches from food absorption and respiration experiments. J. Exp. Mar. Bio. Ecol. 243, 137–154. doi: 10.1016/S0022-0981(99)00114-8
Moens, T., Braeckman, U., Derycke, S., Fonseca, G., Gallucci, F., Ingels, J., et al. (2013). “Ecology of free-living marine nematodes,” in Handbook of Zoology: Gastrotricha, Cycloneuralia and Gnathifera, Vol. 2: Nematoda, ed. A. Schmidt-Rhaesa (Berlin: De Gruyter), 109–152. doi: 10.1371/journal.pone.0186140
Moens, T., Vafeiadou, A. M., De Geyter, E., Vanormelingen, P., Sabbe, K., and De Troch, M. (2014). Diatom feeding across trophic guilds in tidal flat nematodes, and the importance of diatom cell size. J. Sea Res. 92, 125–133. doi: 10.1016/j.seares.2013.08.007
Moens, T., Verbeeck, L., De Maeyer, A., Swings, J., and Vincx, M. (1999). Selective attraction of marine bacterivorous nematodes to their bacterial food. Mar. Ecol. Prog. Ser. 176, 165–178. doi: 10.3354/meps176165
Moens, T., Yeates, G. W., and De Ley, P. (2004). Use of carbon and energy sources by nematodes. Nematol. Monogr. Perspect. 2, 529–545.
Montagna, P. (1984). In situ measurement of meiobenthic grazing rates on sediment bacteria and edaphic diatoms. Mar. Ecol. Prog. Ser. 18, 119–130. doi: 10.3354/meps018119
Monteiro, L. C., Van Butsel, J., De Meester, N., Traunspurger, W., Derycke, S., and Moens, T. (2018). Differential heavy-metal sensitivity in two cryptic species of the marine nematode Litoditis marina as revealed by developmental and behavioural assays. J. Exp. Mar. Bio. Ecol. 502, 203–210. doi: 10.1016/j.jembe.2017.05.016
Newsham, K. K., Rolf, J., Pearce, D. A., and Strachan, R. J. (2004). Differing preferences of Antarctic soil nematodes for microbial prey. Eur. J. Soil Biol. 40, 1–8. doi: 10.1016/j.ejsobi.2004.01.004
Northfield, T. D., Snyder, G. B., Ives, A. R., and Snyder, W. E. (2010). Niche saturation reveals resource partitioning among consumers. Ecol. Lett. 13, 338–348. doi: 10.1111/j.1461-0248.2009.01428.x
Oksanen, J., Blanchet, F. G., Friendly, M., Kindt, R., Legendre, P., McGlinn, D., et al. (2020). vegan: Community Ecology Package. R package version 2.5-7. Available online at: https://CRAN.R-project.org/package=vegan (accessed June 1, 2021).
Pan, W. (2001). Akaike’s information criterion in generalized estimating equations. Biometrics 57, 120–125. doi: 10.1111/j.0006-341x.2001.00120.x
Pekkonen, M., Ketola, T., and Laakso, J. T. (2013). Resource availability and competition shape the evolution of survival and growth ability in a bacterial community. PLoS One 8:e76471. doi: 10.1371/journal.pone.0076471
Pfennig, D. W., Rice, A. M., and Martin, R. A. (2006). Ecological opportunity and phenotypic plasticity interact to promote character displacement and species coexistence. Ecology 87, 769–779. doi: 10.1890/05-0787
Pfisterer, A. B., Diemer, M., and Schmid, B. (2003). Dietary shift and lowered biomass gain of a generalist herbivore in species-poor experimental plant communities. Oecologia 135, 234–241. doi: 10.1007/s00442-002-1169-0
R Core Team (2021). R: A Language And Environment For Statistical Computing. Vienna: R Foundation for Statistical Computing.
Rodger, S., Griffiths, B. S., Mcnicol, J. W., Wheatley, R. W., and Young, I. M. (2004). the impact of bacterial diet on the migration and navigation of Caenorhabditis elegans. Microb. Ecol. 48, 358–365. doi: 10.1007/s00248-003-0201-1
Sánchez-Hernández, J., Gabler, H. M., and Amundsen, P. A. (2017). Prey diversity as a driver of resource partitioning between river-dwelling fish species. Ecol. Evol. 7, 2058–2068. doi: 10.1002/ece3.2793
Schoener, T. W. (1974). Resource partitioning in ecological communities. Science 185, 27–39. doi: 10.1126/science.185.4145.27
Shea, K., and Chesson, P. (2002). Community ecology theory as a framework for biological invasions. Trends Ecol. Evol. 17, 170–176.
Steiner, C. F. (2001). The effects of prey heterogeneity and consumer identity on the limitation of trophic-level biomass. Ecology 82, 2495–2506.
Sudhaus, W. (2011). Phylogenetic systematisation and catalogue of paraphyletic “Rhabditidae” (Secernentea, Nematoda). J. Nematode Morphol. Syst. 14, 113–178.
Venette, R. C., and Ferris, H. (1998). Influence of bacterial type and density on population growth of bacterial-feeding nematodes. Soil Biol. Biochem. 30, 949–960. doi: 10.1016/S0038-0717(97)00176-4
Volterra, V. (1926). Fluctuations in the abundance of a species considered mathematically. Nature 118, 558–560. doi: 10.1038/118558a0
Walter, G. H. (1991). What is resource partitioning? J. Theor. Biol. 150, 137–143. doi: 10.1016/S0022-5193(05)80327-3
Wieser, W. (1953). Die beziehung zwischen mundh ö hlengestalt, ernährungsweise und vorkommen bei freilebenden marinen nematoden. Ark. Zool. 4, 439–484.
Wilsey, B. J., and Polley, H. W. (2002). Reductions in grassland species evenness increase dicot seedling invasion and spittle bug infestation. Ecol. Lett. 5, 676–684. doi: 10.1046/j.1461-0248.2002.00372.x
Worm, B., Barbier, E. B., Beaumont, N., Duffy, J. E., Folke, C., Halpern, B. S., et al. (2006). Impacts of biodiversity loss on ocean ecosystem services. Science 314, 787–791.
Wu, X., Bezerra, T. C., Van Gansbeke, D., and Moens, T. (2019). Natural stable isotope ratios and fatty acid profiles of estuarine tidal flat nematodes reveal very limited niche overlap among co-occurring species. PeerJ 7:e7864. doi: 10.7717/peerj.7864
Zapata, S. C., Travaini, A., Delibes, M., and Martínez-Peck, R. (2005). Food habits and resource partitioning between grey and culpeo foxes in southeastern Argentine Patagonia. Stud. Neotrop. Fauna Environ. 40, 97–103. doi: 10.1080/01650520500129836
Zhang, Y., Lu, H., and Bargmann, C. I. (2005). Pathogenic bacteria induce aversive olfactory learning in Caenorhabditis elegans. Nature 438, 179–184. doi: 10.1038/nature04216
Keywords: resource partitioning, balanced diet hypothesis, bacteria, taxis, fitness, interspecific interactions
Citation: Guden RM, Derycke S and Moens T (2021) A Multi-Faceted Approach to Understand How Resource Diversity Can Mediate the Coexistence of Cryptic Marine Nematode Species. Front. Mar. Sci. 8:777425. doi: 10.3389/fmars.2021.777425
Received: 15 September 2021; Accepted: 19 November 2021;
Published: 08 December 2021.
Edited by:
Bernardo Antonio Perez Da Gama, Fluminense Federal University, BrazilReviewed by:
Punyasloke Bhadury, Indian Institute of Science Education and Research Kolkata, IndiaRodrigo Riera, University of Las Palmas de Gran Canaria, Spain
Copyright © 2021 Guden, Derycke and Moens. This is an open-access article distributed under the terms of the Creative Commons Attribution License (CC BY). The use, distribution or reproduction in other forums is permitted, provided the original author(s) and the copyright owner(s) are credited and that the original publication in this journal is cited, in accordance with accepted academic practice. No use, distribution or reproduction is permitted which does not comply with these terms.
*Correspondence: Rodgee Mae Guden, cm9kZ2VlbWFlLmd1ZGVuQHVnZW50LmJl