- 1State Key Laboratory of Marine Resource Utilization in South China Sea, Hainan University, Haikou, China
- 2Guangxi Key Laboratory of Aquatic Genetic Breeding and Healthy Aquaculture, Guangxi Academy of Fisheries Sciences, Nanning, China
Pearl oyster (Pinctada fucata martensii) is the main species cultured for marine pearls in the world. A breeding program was carried out for desirable production traits, including high growth rate, and a fast-growing selective strain of pearl oysters was established. In the current study, we compared the growth characteristics between a selective strain and a cultured population of P. f. martensii in Beihai, Guangxi Province, China. Large size (SL) and small size (SS) individuals of the selective strain were selected, and the differences of physiological and metabolic indexes, such as feeding, respiration, excretion, and enzyme activities between SL and SS and cultured population (CL), were also compared. The results showed that at the age of 6 months, pearl oysters of the selective strain were 14.61% larger than CL, and the proportion of SL (30–40 mm) was 59%, which was two times higher than CL (28%). SL with a rapid growth rate had a high clearance rate (CR), and the CR of SL was about 1.8 times higher than that of CL and 5 times higher than that of SS. In addition, the activities of digestive enzymes (amylase, pepsin, and lipase) and growth-related carbonic anhydrase enzymes in SL were higher than those in the other two groups (p < 0.05). SS with a slow growth rate had higher oxygen consumption (OCR) and ammonia excretion (AER) rates than SL and CL (p < 0.05). Our results suggest that the rapid growth of the selective strain P. f. martensii can be attributed to increased energy intake and reduced energy consumption.
Introduction
The pearl oyster, Pinctada fucata martensii, belonging to the family Pteriidae (Pterioida, Bivalvia), is naturally distributed in countries along the west coast of the Pacific Ocean, such as China, Japan, Australia, and the Philippines (Wang et al., 2004). P. f. martensii is the primary species cultured for marine pearls in China and Japan (Wang et al., 2011a). The pearl oyster industry has become one of the most important mariculture industries in several southern provinces of China, including Guangdong, Guangxi, and Hainan (Wang et al., 2004; Deng et al., 2009a, 2011; He et al., 2021; Xu et al., 2021). However, the conditions are changing due to the slow growth and mass mortalities of oysters (Deng et al., 2011; Gu et al., 2011). Pearl production dropped sharply in recent years, and the cultivate industry was dealt a huge blow. A selective breeding program toward P. f. martensii was carried out in 2000. A rapid growth selective strain was obtained through hybrid breeding with the Indian population as the male parent and the Chinese Sanya population as the female parent (Wang et al., 2000, 2004). After seven generations of selection, remarkable genetic gains for growth rate and a high proportion of high-quality pearls were achieved in the selected strain (Wang et al., 2011b).
Specific mechanisms associated with fast growth rate in bivalves include the efficacies of processes of food acquisition and metabolic rates in resting or active states (Bayne, 1999, 2000; Bayne et al., 1999; Tamayo et al., 2011, 2014, 2015). Physiological processes related to these mechanisms include clearance rate (CR), oxygen consumption rate (OCR), ammonia excretion rate (AER), and enzyme activities. Therefore, ingestion and energy metabolism of some commercial bivalve species have received considerable attention (Ibarrola et al., 2017; Zhang et al., 2018). Individuals might exhibit faster growth due to increased energy acquisition and maintenance or growth costs (Bayne et al., 1999; Meyer and Manahan, 2010; Tamayo et al., 2011), and this explanation is applicable for other bivalve larval stages (Pace et al., 2006; Tamayo et al., 2014). Furthermore, in bivalves, the process of biological mineralization is closely related to shell growth. Carbonic anhydrase is one of the most important indicators of biological mineralization (Medaković, 2000; Cardoso et al., 2019).
Processes related to energy utilization and growth efficiency of mollusks are largely controlled by genetics, such as in oysters (Bayne et al., 1999; Parker et al., 2010; Zhao et al., 2019b), abalone (Gonzalez et al., 2010), and green-lipped mussels (Ibarrola et al., 2017). These theories provide a feasible scheme for improving the growth characteristics of bivalves (Newkirk, 1980; Evans and Langdon, 2006; Schöne et al., 2021). Numerous studies have confirmed effective improvement in commercial traits of bivalve species through selective experiments (Deng et al., 2009b; Gu et al., 2011; Li et al., 2011; Zhang et al., 2018). Physiological differences between improved selected and non-selected groups were explored (Bayne et al., 1999; Zhang et al., 2018). However, the relationship between fast-growth and physiological factors of P. f. martensii has not been documented. Therefore, the study aimed to figure out the physiological processes related to fast-growth, including CR (energy acquisition), OCR and AER (metabolic rate), and digestive enzyme and carbonic anhydrase activities in P. f. martensii, determine the physiological parameters leading to the existence of differences in the growth potential of the selective strain, and explore the mechanism of selected strain of fast-growing P. f. martensii from a physiological point of view.
Materials and Methods
Pearl Oyster Collection
On June 10, 2020, two different stocks, A and B, of P. f. martensii were selected to conduct mass selection experiments at a shellfish hatchery in Beihai, Guangxi Province, China. Stock A descended from the fast-growing selective strain. Stock B was a cultured population without artificial selection. For the two stocks, 50 individuals were selected as parents in each group, and the two groups were inseminated to obtain progeny. Larval rearing was similar to the process previously described by Zheng et al. (2004) and Deng et al. (2011). After 30 d, juveniles from each group were taken from the polyethylene bags and raised in coastal areas suspended on a raft. The two groups were cultured in the same area located at Beihai, China (21°26′-21°55′ N, 108°50′-109°47′ E).
The polyethylene bags were cleaned periodically, and the oyster shell height was measured monthly. On November 10, 2020, a total of 270 randomly selected individuals from each group were taken to the laboratory and used in a physiological experiment. Pearl oysters of the selected strain were divided into two groups (SL, shell height 37.56 ± 9.01 mm; SS, shell height 10.01 ± 0.31 mm) based on shell height. Large-sized individuals from the cultured population (CL, shell height 36.76 ± 9.88 mm) were selected to compare the physiological differences between the populations (inter-population) (Table 1).
From December 10, 2020, the oyster shell height was measured for 6 months. A total of 100 oysters of the selective strain and cultured population were randomly selected to determine the size-frequency distribution (Figure 1).
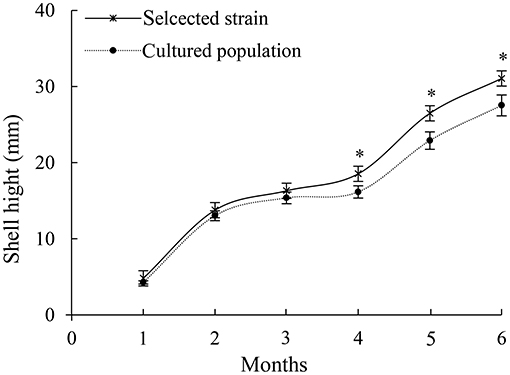
Figure 1. Growth of shell height of selective strain and cultured population pearl oysters. *p < 0.05.
Experimental Design
After 24 h of starvation, the SL, SS, and CL oysters were placed in 100 L buckets, with 30 individuals per bucket. Each group was replicated thrice and domesticated in the laboratory for 7 d. Pearl oysters were fed twice a day with a microalgae diet of Isochrysis galbana (3 × 104 cells/mL). Water temperature and salinity were maintained at 19 ± 0.5°C and 30 ± 0.5‰, respectively. Seawater was stored and aerated after precipitation and sand filtration before each experiment.
Physiological Parameters Determination
CR
After a week of acclimation, 10 pearl oysters from each of the three groups (SL, SS, and CL) were randomly collected and placed in 1 L beakers. Each treatment was replicated thrice. One blank beaker with no pearl oysters was set as the control group for each treatment. Pearl oysters were fed with I. galbana (6 × 104 cells mL−1). The experiment lasted for 3 h, and the concentration of algae was calculated under a microscope by a hemocytometer.
CR (clearance rate) is defined as the volume of water cleared of algal cells per hour. The value of CR (L/h) was estimated following the equation (Zhang et al., 2018):
where V = volume (L) of beaker, t = duration of the measurement (h), C0 = concentration of unicellular algae at initial time, and Ct = concentration of unicellular algae at t time. Cell concentrations were measured by direct counting with a haemocytometer. Sd = variable coefficient of the control group unicellular algae. W = dry weight of the pearl oyster soft tissues.
Metabolism Measurements
Closed-chamber respiration methods were employed to determine oxygen consumption rate (OCR) and ammonia excretion rate (AER). Six pearl oysters were placed in 3 L glass respiration chamber at test SL, SS, and CL, which was aerated for at least 1 h to reach oxygen saturation before the measurement. Each group was replicated thrice and one blank chamber with no oysters was used as the control. The experiment lasted for 3 h. Water samples were collected by siphoning, and dissolved oxygen (DO) was measured at the start and end of the experiment. DO was measured by the YSI (5331) polarographic electrode coupled with a micro-oxymeter (Yellow Springs, OH, USA). Ammonia determination was done by the sodium hypobromite method (Gu et al., 2020). The DO and ammonia of blank seawater were carried out and then subtracted from the experimental units to correct for autogenic trends. DO was not <4.2 mg/L (more than 70% of total DO) in test vessels at the end of the experiment. Ammonia accumulation did not exceed the maximum values of ammonium concentration (0.04 mg/L).
OCR (mg/h) was calculated using the following equation:
The initial and final concentrations of DO are expressed as DO0 and DOt, t = duration of measurement (h), V = volume of the chamber (L), and W= dry weight of soft tissues.
AER (mg/L) was measured using the following equation:
The initial and final concentrations of AER are expressed as N0 and Nt, t = duration of measurement (h), V = volume of the chamber (L), and W = dry weight of the soft tissues.
The oxygen: nitrogen (O/N) atomic ratio was used to estimate the proportion of protein in relation to lipids or carbohydrates for metabolism, which was calculated as follows:
Digestive Enzyme and Carbonic Anhydrase Activities
Before the experiment, three groups of pearl oysters were starved for 1 d. Ten oysters were randomly selected from each group. The visceral mass was removed from each oyster by vivisection on a liquid nitrogen cold plate, rapidly frozen, and stored at −80°C. The visceral mass was shredded with a tissue mashing instrument, and then the analytical balance was used to weigh visceral mass (0.01 g), diluted 10 times with sterilized normal saline, and centrifuged at 5,000 rpm for 10 min. Then, each supernatant was collected in an Eppendorf tube and stored at 4°C. Amylase, lipase, pepsin, and carbon acid anhydride enzyme activities were determined using commercial assay kits (Nanjing Jian-cheng Institute, Nanjing, China).
Amylase activity was determined by the method described by Vega-Villasante et al. (1993), using starch as a substrate. The samples were read spectrophotometrically at 540 nm, and results were expressed in units per milligram of protein (U/mg protein). Lipase activity was determined by the method described by Li et al. (2020), using β-naphthyl-caprylate as a substrate. The activity was evaluated spectrophotometrically at 540 nm, and the specific activity was expressed in units per milligram of protein (U/mg protein). Pepsin activity was determined by the method described by Li et al. (2020), using hydrolyzed proteins to produce amino acids containing phenols as a substrate. The samples were read spectrophotometrically at 660 nm, and results were expressed in units per milligram of protein (U/mg protein). Carbon acid anhydride enzyme activities were measured using the classical phenol red method (Roy et al., 2012). The samples were read spectrophotometrically at 450 nm, and results were expressed in units per milligram of protein (U/g protein).
Statistical Analysis
The results are presented as mean ± standard deviation (S.D.). The differences in physiological measurements among different categories were analyzed with one-way analysis of variance (ANOVA) to determine the significant differences among the treatments at p < 0.05. If the main effects were significant, Tukey's multiple range test was used to compare the mean values (p < 0.05) between individual treatments. Analysis was conducted using SPSS statistics 20.0 software (IBM, Armonk, NY, USA).
Results
Growth Rate Comparisons
In 6 months, selective breeding pearl oysters had a higher phenotypic (shell height) mean than the cultured population (Figure 1). At the age of 6 months, the average shell height was 3.9 mm (14.61%), which was higher than the cultured population (p < 0.05). The cultured population had a higher value of phenotypic variation (CV) (Table 2). In the selective strain (Figure 1), the proportion of large size (30–40 mm) individuals was higher (59%) than the control group (28%). The proportion of 20–30 mm pearl oysters was the highest among the cultured population, accounting for 60% (Figure 2).
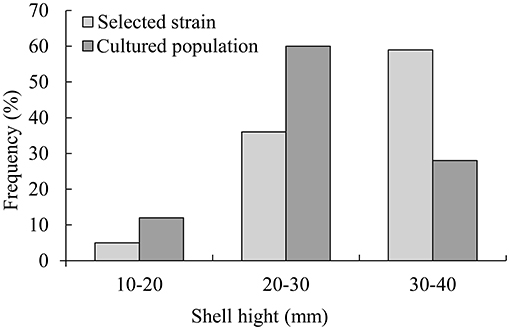
Figure 2. Size distribution of selective strain and cultured population pearl oysters at 6-month-old.
Differences in Physiological Performance
CR
CRs of pearl oysters of three groups are shown in Figure 3A. SL had a higher CR than the other two groups (p < 0.05) (Table 3). The value was about 1.8 times higher than CL and 5 times higher than SS.
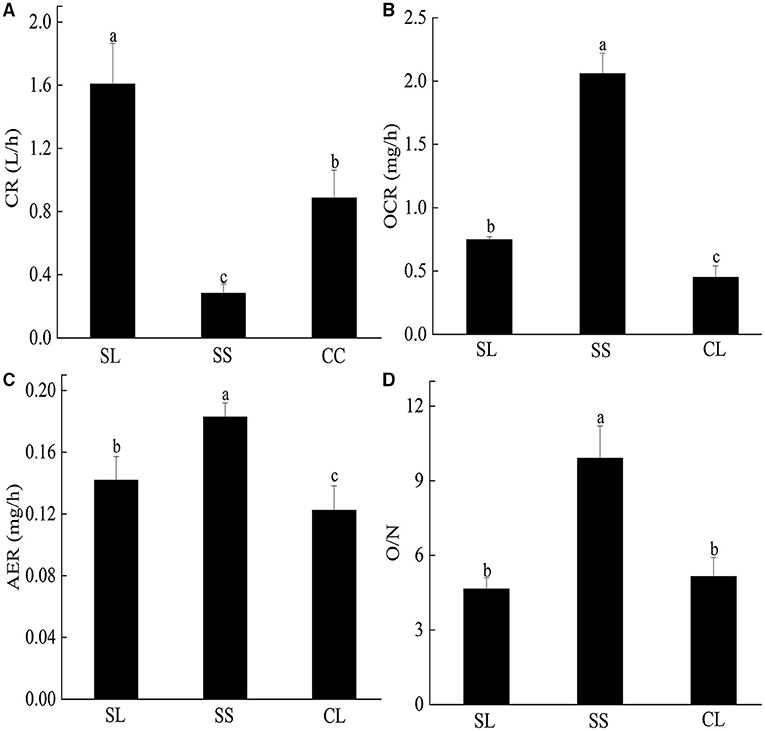
Figure 3. (A–D) The clearance rate, oxygen consumption rate, ammonia excretion rate, and O/N rate of P. f. martensii in SL, CL, and SS groups. Means ± S.D. are presented. n = 3 replicate per treatment. Different letters represent significant differences (p < 0.05).
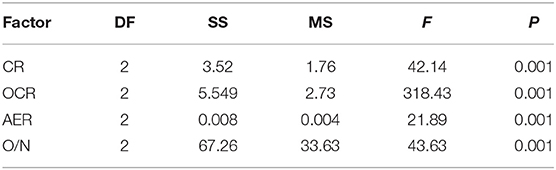
Table 3. Analysis of variance (ANOVA) test of clearance rate, oxygen consumption rate, ammonia excretion rate, and O/N rate of P. f. martensii in the three groups.
OCR and AER
There were significant differences in OCR and AER between the SL and SS and CL (Figures 3B,C). ANOVA test showed that weight traits were significantly correlated with the changes of AER and OCR (p < 0.05) (Table 3). The AER and OCR decreased as the dry weight of soft tissues increased, and there was a negative correlation between them. The AER and OCR of SS were significantly higher than that in SL (p < 0.05). Under the same specifications, the AER and OCR in SL were significantly higher than that in CL (p < 0.05).
Comparison of Enzyme Activities
Enzyme activities of the three groups are presented in Figure 4. For the three digestive enzymes, the activities of SL were significantly higher than those of SS and CL (p < 0.05). Amylase activity of SL was about 10 times higher than that of SS and 1.7 times higher than that of CL (Figure 4A). Although the specifications of CL and SS differ greatly, there was no significant difference in lipase activity between them (Figure 4B). When comparing the pepsin activity, significant differences were observed among three groups, and the activity in SL was about 3 times higher than that of SS (Figure 4C). For carbonic anhydrase, the enzyme activity in SL and CL was significantly higher than that in SS (p < 0.05) (Figure 4D).
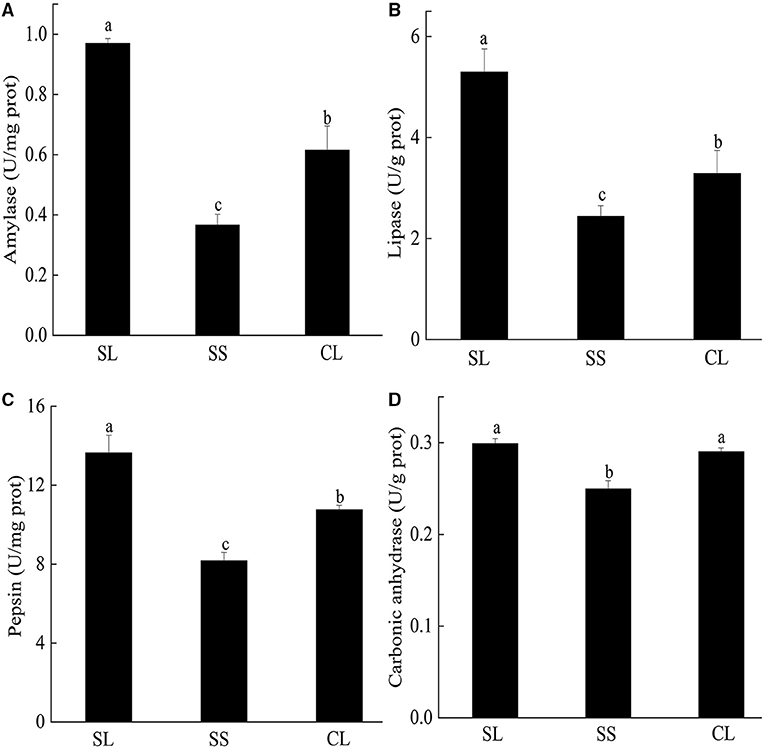
Figure 4. (A–D) Specific activities of amylase, pepsin, lipase, and carbonic anhydrase activities in the visceral mass of P. f. martensii. Means ± S.D. are presented. n = 3 replicate per treatment. Different letters represent significant differences (p < 0.05).
Discussion
In the present study, we compared the growth differences between the selective strain of P. f. martensii and the control group, and results showed that the growth rate of selective strain was significantly improved (14.61%) (p < 0.05), which was consistent with the previous study on 1-year-old selective strain pearl oyster (15.31%) (Wang et al., 2011a). The proportion of large-size (30–40 mm) oysters in the selected strain was two times higher than the cultured population. In addition, the median of shell height of the selected strain was larger, and the coefficient of variation was smaller, indicating that the cumulative multi-generation selection breeding improved the growth rate and reduced the phenotypic differences of the young generations (Wang et al., 2011b).
The differences of growth rate or survival were mainly caused by physiological variety, such as feeding, respiration, or enzyme activities (Bayne, 2000; Tamayo et al., 2014; Zhang et al., 2018; Zhao et al., 2019a, 2020). Feeding, respiration, and excretion are the most important basic forms of metabolism in organisms. In this study, we examined the difference of feeding and metabolism between the selected strain and cultured population of P. f. martensii and identified the mechanism responsible for the improved growth performance in the selective breeding of pearl oysters.
In this study, mean CR values in P. f. martensii ranged from 0.4 to 1.6 (L/g*h), which were close to the values of Tapes decussatus and banded carpet-shell clams Paphia rhomboïdes (Savina and Pouvreau, 2004), but comparatively lower than the values of the Indo-Pacific mytilid Brachidontes pharaonis (Sara et al., 2008) and clams Ruditapes decussatus (Sobral and Widdows, 1997). Inter-tidal species living in highly variable habitats should seize an immediate advantage from every profitable environmental situation allowing them to acquire energy to be allocated to growth and reproduction (Wang et al., 2015a). Pearl oysters showed a relatively low food acquisition speed, which explained that the species lived in an invariable condition. This hypothesis was proven by a study, which reported that ribbed mussel (Geukensia demissa) under subtidal zone conditions exhibited lower CR than intertidal conspecific (Charles and Newell, 1997). P. f. martensii is a species distributed in the subtidal zone (Wang et al., 2004). CR of the selected strain pearl oysters with a highly homologous genetic basis was significantly different, indicating that feeding rates were highly correlated with growth within species (Vladimirova et al., 2003). In the comparison between the selective strain and cultured population, CR values of the same size were also significantly different, which showed a reduced ecological footprint of the selective strain oysters (Hall et al., 2020). The results were similar to Tamayo et al. (2012), who found that the rapid growth of R. philippinarum showed a significantly higher CR than slow-growing ones, irrespective of temperature. Correlations between growth and CR have been published in several marine bivalves (Sousa et al., 2011; Tamayo et al., 2015; Zhang et al., 2018). The individuals with rapid growth had relatively higher clearance rate and absorption rate and lower metabolic cost (Hall et al., 2020). Bivalves may maximize energy for growth through rapid feeding. This hypothesis was supported by Bayne et al. (1999), who reported that rapid growth in Sydney rock oysters (Saccostrea commercialism) was positively related to increased food acquisition.
OCR is a crucial physiological parameter that reflects the energy metabolism level of aerobic respiration (Zhang et al., 2018). AER is considered an index to evaluate the tolerance of bivalves to environmental stress (Nie et al., 2017). In this study, OCR values ranged from 0.45 to 2.30 (mg/g*h), and AER values ranged from 0.07 to 0.19 (mg/g*h), which were different from the results reported by Wang et al. (2009). These differences may be caused by different temperatures, as temperature is a fundamental factor influencing energy metabolism in marine bivalves (Tamayo et al., 2015). Wang et al. (2009) also confirmed that OCR and AER of P. f. martensii were positively associated with temperature. The fast-growing selective strain of pearl oysters was obtained through generations of intensive mass selection. Therefore, the genetic basis was highly homologous (Wang et al., 2011a). According to the current study (showed in Figure 3), OCR and AER of SS pearl oysters were comparatively higher than SL. Such metabolic behavior was previously reported in R. philippinarum (Tamayo et al., 2011, 2012) and oyster (C. gigas) (Zhang et al., 2018), indicating that the size of an organism was closely associated with the metabolic rate (Kang et al., 2010). Generally, metabolic rate of the small specification was significantly faster than large ones, including the larval stage (Beiras and Camacho, 1994; Kang et al., 2010; Xu et al., 2020). Higher metabolic rate in SS was associated with a smaller deficiency in heterozygote frequencies, a genetic condition imposing raised metabolic costs associated with elevated protein turnover rates (Hawkins et al., 1989; Hawkins, 1995; Tremblay et al., 1998; Myrand et al., 2002). As shown in Figure 3, both OCR and AER of metabolism were significantly higher in SL and SS than CL (p < 0.05). A similar metabolism behavior was reported in the hard clam (Mercenaria mercenaria), where the selective bred of hard clam showed a 33% increment in their standard OCR compared to the wild individuals (Pernet et al., 2006). It suggests that the differences in growth and metabolism of P. f. martensii are caused by genetic variation, and the genes that control growth are pleiotropic or closely linked to the genes determining physiological traits (Wang et al., 2009; Zhang et al., 2018).
O/N represents the ratio of protein to fat and carbohydrate catabolism in organism (Mayzaud, 1976). If the energy is completely supplied by protein, the O/N value is 7. If the energy is supplied by protein and fat, the ratio is 24. If the energy is completely supplied by fat or carbohydrate, the ratio will become infinite (Mayzaud, 1976). For SL and CL, the main energy supply was protein, followed by fat and carbohydrate, while the proportion of protein oxidation energy supply decreased for SS in this study. The results were consistent with R. philippinarum (Nie et al., 2017).
For energy utilization during the metabolic process, various complex organic compounds are hydrolyzed to monomeric subunits, including glucose, fatty acid, and amino acids (Boetius and Felbeck, 1995). Marine molluscs obtain multiple nutritional requirements by utilizing various digestive enzymes, such as amylase, lipase, trypsin, pepsin, and lysozyme, to catalyze and decompose different types of substrates (Weel, 1961; Wang et al., 2015b; Martínez-Montaño et al., 2018; Kong et al., 2019). In this study, we observed three typical digestive enzymes (amylase, lipase, and pepsin) in P. f. martensii. The types and activities of enzymes were different from those reported by Wang et al. (2010), for example, the value of amylase activity was comparatively lower than in their studies. The difference in the type and level of digestive enzymatic activity may be due to differences in diet (Fernández-Reiriz et al., 2001; Labarta et al., 2002; Alberto et al., 2020). In the experiment, we found no cellulase activity in any of the test tubes. During the experiment, the enzyme activity was examined to reduce the types of error in the feed. All groups were fed I. galbana for domestication, which was considered common feed for bivalves (Cheng et al., 2020). I. galbana has a naked cell without cell walls, but cellulase is related to the digestion of cell walls (Cheng et al., 2020), indicating the absence of cellulase in pearl oysters (P. f. martensii). In addition, digestive enzyme activity was strongly correlated with body size. A study on Aulacomya ater showed that increasing body-size increased specific amylase activity (Ibarrola et al., 2012). However, increasing body-size promoted a significant reduction in protease activity in M. chilensis, chorus mussel (Choromytilus chorus), and A. ater (Ibarrola et al., 2012). In the current study, the activities of three digestive enzymes were higher in large individuals (SL and CL) than SS, indicating a relatively higher food processing capacity in the gut of fast-growing pearl oysters (Tamayo et al., 2012). Metabonomics and transcriptome studies have showed that fast-growing pearl oysters (P. f. martensii) exhibited higher digestion, anabolic ability, and osmotic regulation ability than the slow-growing group (Hao et al., 2019).
The successive selection of fast-growing bivalves may have positive effects on hydrolytic activities of gland tissues and enzyme activities in digestive glands, which are closely correlated with the digestive capacities of bivalves (Ibarrola et al., 2000; Tamayo et al., 2015; Zhang et al., 2018). Studies on bivalves had confirmed that a significant degree of genetic control was exerted over parameters influencing energy utilization and growth efficiency, including oysters (Bayne et al., 1999; Parker et al., 2010) and green-lipped mussels (Ibarrola et al., 2017). By comparing first-generation yellow shell color and cultivated stocks of P. f. martensii, the digestive enzyme activity was effectively increased through breeding (Wang et al., 2010). We also obtained similar results for digestive enzyme activity.
Carbonic anhydrase, one of the major biocatalysts for carbon capture and storage, is involved in shell formation processes in bivalves. The activity of carbonic anhydrase in the mantle tissue of European abalone (Haliotis tuberculate) was closely related to the formation of shell structure (prismatic and nacreous layers) (Roy et al., 2012). Carbonic anhydrase plays a key role in regulating mineralized structures through calcium carbonate crystal formation (Cardoso et al., 2019). In the internal selected strain, SL had a higher carbonic anhydrase activity than SS, indicating that larger pearl oysters had a higher calcium carbonate formation and shell mineralization. Studies on blue mussels (M. edulis) have confirmed that carbonic anhydrase activity is essential for rapid shell development (Medaković, 2000). Wilbur and Anderson (1950) also reported that enzyme content might increase with the development of shellfish.
Conclusion
In summary, pearl oysters (P. f. martensii) from the fast-growing selective strain had more efficient food ingestion, food digestion, and energy absorption compared to the cultured population. In the inter selective strain, the OCR of SS was nearly 3 times higher than that of SL, and the AER of SS was 1.5 times higher than that of SL, which proved that a combination of fast feeding and reduced metabolic costs would enable SL to increase their growth rate. In order to identify growth-related regulatory genes, further investigation is required to compare gene expression in contrasting physiological phenotypes.
Data Availability Statement
The original contributions presented in the study are included in the article/Supplementary Material, further inquiries can be directed to the corresponding author/s.
Ethics Statement
The animal study was reviewed and approved by the Committee and Laboratory Animal Department of Hainan University. Written informed consent was obtained from the owners for the participation of their animals in this study.
Author Contributions
AW and CL designed the experiment. BY and ML performed the experiment. ZG analyzed the experimental data and draw diagrams. XZ wrote the paper. SY revised the paper. All authors contributed to the article and approved the submitted version.
Funding
This research was supported financially by the National Key Research and Development Program of China (2018YFD0900704), the National Natural Science Foundation of China (1772847), and the Talent Development Program of Hainan Province (HD-YSZX-202011).
Conflict of Interest
The authors declare that the research was conducted in the absence of any commercial or financial relationships that could be construed as a potential conflict of interest.
Publisher's Note
All claims expressed in this article are solely those of the authors and do not necessarily represent those of their affiliated organizations, or those of the publisher, the editors and the reviewers. Any product that may be evaluated in this article, or claim that may be made by its manufacturer, is not guaranteed or endorsed by the publisher.
Supplementary Material
The Supplementary Material for this article can be found online at: https://www.frontiersin.org/articles/10.3389/fmars.2021.770702/full#supplementary-material
References
Alberto, P. R., Gabriela, M. A., Regina, E. G., Gabriela, M. C., Dariel, T. R., and Cristina, E. F. (2020). Seaweed single cell detritus effects on the digestive enzymes activity and microbiota of the oyster Crassostrea gigas. J. Appl. Phycol. 32, 3481–3493. doi: 10.1007/s10811-020-02167-4
Bayne, B. L. (1999). Physiological components of growth differences between individual oysters (Crassostrea gigas) and a comparison with Saccostrea commercialis. Physiol. Biochem. Zool. 72:705. doi: 10.1086/316714
Bayne, B. L. (2000). Relations between variable rates of growth, metabolic costs and growth efficiencies in individual Sydney rock oysters (Saccostrea commercialis). J. Exp. Mar. Bio. Ecol. 251, 185–203. doi: 10.1016/S0022-0981(00)00211-2
Bayne, B. L., Svensson, S., and Nell, J. A. (1999). The physiological basis for faster growth in the sydney rock oyster, Saccostrea commercialis. Biol. Bull–US. 197, 377–387. doi: 10.2307/1542792
Beiras, R., and Camacho, A. P. (1994). Influence of food concentration on the physiological energetics and growth of Ostrea edulis larvae. Mar. Biol. 120, 427–435. doi: 10.1007/bf00680217
Boetius, A., and Felbeck, H. (1995). Digestive enzymes in marine invertebrates from hydrothermal vents and other reducing environments. Mar. Biol. 122, 105–113. doi: 10.1007/BF00349283
Cardoso, J. C. R., Ferreira, V., Zhang, X., Anjos, L., Félix, R. C., Batista, F. M., et al. (2019). Evolution and diversity of alpha-carbonic anhydrases in the mantle of the Mediterranean mussel (Mytilus galloprovincialis). Sci. Rep. 9:10400. doi: 10.1038/s41598-019-46913-2
Charles, F., and Newell, R. (1997). Digestive physiology of the ribbed mussel Geukensia demissa (Dillwyn) held at different tidal heights. J. Exp. Mar. Biol. Ecol. 209, 201–213. doi: 10.1016/S0022-0981(96)02694-9
Cheng, P., Zhou, C., Chu, R., Chang, T., Xu, J., Ruan, R., et al. (2020). Effect of microalgae diet and culture system on the rearing of bivalve mollusks: Nutritional properties and potential cost improvements. Algal. Research. 51:102076. doi: 10.1016/j.algal.2020.102076
Deng, Y., Du, X., and Wang, Q. (2009a). Selection for fast growth in the chinese pearl oyster, pinctada martensii: response of the first generation line. J. World. Aquacult. Soc. 40, 843–847. doi: 10.1111/j.1749-7345.2009.00307.x
Deng, Y., Fu, S., Du, X., and Wang, Q. (2009b). Realized heritability and genetic gain estimates of larval shell length in the chinese pearl oyster pinctada martensii at three different salinities. N. Am. J. Aquacult. 71, 302–306. doi: 10.1577/A08-024.1
Deng, Y., Yu, Z., Du, X., Wang, Q., and Fu, S. (2011). Growth performance and physiological parameters of the second generation selected and control groups of pinctada martensii. Acta. Oceanol. Sin. 30, 120–125. doi: 10.1007/s13131-011-0112-9
Evans, S., and Langdon, C. (2006). Direct and indirect responses to selection on individual body weight in the Pacific oyster (Crassostrea gigas). Aquaculture 261, 546–555. doi: 10.1016/j.aquaculture.2006.07.037
Fernández-Reiriz, M., Labarta, U., Navarro, J., and Velasco, A. (2001). Enzymatic digestive activity in Mytilus chilensis (Hupé 1854) in response to food regimes and past feeding history. J. Comp. Physiol. B. 171, 449–456. doi: 10.1007/s003600100194
Gonzalez, G. G., Brokordt, K. B., and Winkler, F. E. (2010). Repeatability of physiological traits in juvenile Pacific abalone, Haliotis discus hannai. Mar. Biol. 157, 2195–2203. doi: 10.1007/s00227-010-1485-6
Gu, Z., Shi, Y., Wang, Y., and Wang, A. (2011). Heritable characteristics in the pearl oyster Pinctada martensii: comparisons of growth and shell morphology of Chinese and Indian populations, and reciprocal crosses. J. Shellfish Res. 30, 241–246. doi: 10.2983/035.030.0207
Gu, Z., Wei, H., Cheng, F., Wang, A., and Liu, C. (2020). Effects of air exposure time and temperature on physiological energetics and oxidative stress of winged pearl oyster (Pteria penguin). Aquacult. Rep. 17:100384. doi: 10.1016/j.aqrep.2020.100384
Hall, S. A., Méthé, D., Stewart-Clark, S. E., Clark, K. F., and Tremblay, R. (2020). Comparison of absorption efficiency and metabolic rate between wild and aquaculture oysters (Crassostrea virginica). Aquaculture. Rep. 16:100263. doi: 10.1016/j.aqrep.2019.100263
Hao, R., Du, X., Yang, C., Deng, Y., Zheng, Z., and Wang, Q. (2019). Integrated application of transcriptomics and metabolomics provides insights into unsynchronized growth in pearl oyster Pinctada fucata martensii. Sci. Total Environ. 666, 46–56. doi: 10.1016/j.scitotenv.2019.02.221
Hawkins, A. J. S. (1995). Effects of temperature change on ectotherm metabolism and evolution: Metabolic and physiological interrelations underlying the superiority of multi-locus heterozygotes in heterogeneous environments. J. Therm. Biol. 20, 23–33. doi: 10.1016/0306-4565(94)00023-C
Hawkins, A. J. S., Widdows, J., and Bayne, B. L. (1989). The relevance of whole-body protein metabolism to measured costs of maintenance and growth in Mytilus edulis. Physiol. Zool. 62, 745–763. doi: 10.1086/physzool.62.3.30157925
He, G., Liu, X., Xu, Y., Liang, J., Deng, Y., Zhang, Y., et al. (2021). Repeated exposure to simulated marine heatwaves enhances the thermal tolerance in pearl oysters. Aquat. Toxicol. 239:105959. doi: 10.1016/j.aquatox.2021.105959
Ibarrola, I., Arambalza, U., Navarro, J. M., Urrutia, M. B., and Navarro, E. (2012). Allometric relationships in feeding and digestion in the Chilean mytilids Mytilus chilensis (Hupé), Choromytilus chorus (Molina) and Aulacomya ater (Molina): A comparative study. J. Exp. Mar. Biol. Ecol. 426–427, 18–27. doi: 10.1016/j.jembe.2012.05.012
Ibarrola, I., Etxeberria, M., Iglesias, J. I. P., Urrutia, M. B., and Angulo, E. (2000). Acute and acclimated digestive responses of the cockle Cerastoderma edule (L.) to changes in the food quality and quantity II. Enzymatic, cellular and tissular responses of the digestive gland. J. Exp. Mar. Biol. Ecol. 21, 181–198. doi: 10.1016/s0022-0981(00)00233-1
Ibarrola, I., Hilton, Z., and Ragg, N. L. C. (2017). Physiological basis of inter-population, inter-familiar and intra-familiar differences in growth rate in the green-lipped mussel Perna canaliculus. Aquaculture 479, 544–555. doi: 10.1016/j.aquaculture.2017.06.031
Kang, K. H., Haemgoong, P., Kim, Y. H., Seon, S. C., and Zhou, B. (2010). Filtration and oxygen consumption rates on various growth stages of Scapharca broughtonii spat. Aquaculture. Res. 39, 195–199. doi: 10.1111/j.1365-2109.2007.01879.x
Kong, H., Wu, F., Jiang, X., Wang, T., Hu, M., Chen, J., et al. (2019). Nano-TiO2 impairs digestive enzyme activities of marine mussels under ocean acidification. Chemosphere 237:124561. doi: 10.1016/j.chemosphere.2019.124561
Labarta, U., Fernández-Reiriz, M. J., Navarro, J. M., and Velasco, A. (2002). Enzymatic digestive activity in epifaunal (Mytilus chilensis) and infaunal (Mulinia edulis) bivalves in response to changes in food regimes in a natural environment. Marine Biol. 140, 669–676. doi: 10.1007/s00227-001-0742-0
Li, C., Liu, E., Li, T., Wang, A., Liu, C., and Gu, Z. (2020). Suitability of three seaweeds as feed in culturing strawberry conch Strombus luhuanus. Aquaculture 519:734761. doi: 10.1016/j.aquaculture.2019.734761
Li, Q., Wang, Q., Liu, S., and Kong, L. (2011). Selection response and realized heritability for growth in three stocks of the Pacific oyster Crassostrea gigas. Fisheries. Sci. 77, 643–648. doi: 10.1007/s12562-011-0369-0
Martínez-Montaño, E., Uriarte, I., Rosas, C., Amthauer, R., Romero, A., and Farías, A. (2018). Replacing live feed with formulated diets in juvenile Patagonian red octopus (Enteroctopus megalocyathus). Aquacult. Nutr. 24, 633–643. doi: 10.1111/anu.12589
Mayzaud, P. (1976). Respiration and nitrogen excretion of zooplankton. IV. The influence of starvation on the metabolism and the biochemical composition of some species. Mar. Biol. 37, 47–58. doi: 10.1007/BF00386778
Medaković, D. (2000). Carbonic anhydrase activity and biomineralization process in embryos, larvae and adult blue mussels Mytilus edulis L. Helgol. Mar. Res. 54, 1–6. doi: 10.1007/s101520050030
Meyer, E., and Manahan, D. T. (2010). Gene expression profiling of genetically determined growth variation in bivalve larvae (Crassostrea gigas). J. Exp. Biol. 213:749. doi: 10.1242/jeb.037242
Myrand, B., Tremblay, R., and Sévigny, J. M. (2002). Selection against blue mussels (Mytilus edulis L.) homozygotes under various stressful conditions. J. Hered. 93, 238–248. doi: 10.1093/jhered/93.4.238
Newkirk, G. F. (1980). Review of the genetics and the potential for selective breeding of commercially important bivalves. Aquaculture 19, 209–228. doi: 10.1016/0044-8486(80)90045-9
Nie, H. T., Huo, Z. M., Hou, X. L., Chen, Y., Yang, F., Yan, X. W., et al. (2017). Comparison study on the effect of temperature and salinity on oxygen consumption and ammonia excretion in zebra strain and wild ruditapes philippinarum. Acta. Hydrobiol. Sinica 41, 121–126.
Pace, D. A., Marsh, A. G., Leong, P. K., Green, A. J. D, and Hedgecock Manahan, D. T. (2006). Physiological bases of genetically determined variation in growth of marine invertebrate larvae: A study of growth heterosis in the bivalve Crassostrea gigas. J. Exp. Mar. Biol. Ecol. 335, 188–209. doi: 10.1016/j.jembe.2006.03.005
Parker, L. M., Ross, P. M., and O'Connor, W. A. (2010). Comparing the effect of elevated pCO2 and temperature on the fertilization and early development of two species of oysters. Mar. Biol. 157, 2435–2452. doi: 10.1007/s00227-010-1508-3
Pernet, F., Tremblay, R., Gionet, C., and Landry, T. (2006). Lipid remodeling in wild and selectively bred hard clams at low temperatures in relation to genetic and physiological parameters. J. Exp. Biol. 209, 4663–4675. doi: 10.1242/jeb.02581
Roy, N. L., Marie, B., Gaume, B., Guichard, N., Delgado, S., Zanella-Cléon, I., et al. (2012). Identification of two carbonic anhydrases in the mantle of the european abalone Haliotis tuberculata (Gastropoda, Haliotidae): Phylogenetic Implications. J. Exp. Zool. Part. B. 318, 353–367. doi: 10.1002/jez.b.22452
Sara, G., Romano, C., Widdows, J., and Staff, F. J. (2008). Effect of salinity and temperature on feeding physiology and scope for growth of an invasive species (Brachidontes pharaonis-mollusca: bivalvia) within the Mediterranean sea. J. Exp. Mar. Biol. Ecol. 363, 130–136. doi: 10.1016/j.jembe.2008.06.030
Savina, M., and Pouvreau, S. (2004). A comparative ecophysiological study of two infaunal filter-feeding bivalves: Paphia rhombodes and Glycymeris glycymeris. Aquaculture 239, 289–306. doi: 10.1016/j.aquaculture.2004.05.029
Schöne, B. R., Huang, X., Zettler, M. L., Zhao, L., Mertz-Kraus, R., Jochum, K. P., et al. (2021). Mn/Ca in shells of Arctica islandica (Baltic Sea) – A potential proxy for ocean hypoxia? Estuar. Coast Shelf S 251:107257. doi: 10.1016/j.ecss.2021.107257
Sobral, P., and Widdows, J. (1997). Effects of elevated temperatures on the scope for growth and resistance to air exposure of the clam Ruditapes decussatus (L.), from southern Portugal. Sci. Mar. 61:1128. doi: 10.1023/A:1018435711128
Sousa, J., Matias, D., Joaquim, S., Ben-Hamadou, R., and Leitão, A. (2011). Growth variation in bivalves: New insights into growth, physiology and somatic aneuploidy in the carpet shell Ruditapes decussatus. J. Exp. Mar. Biol. Ecol. 406, 46–53. doi: 10.1016/j.jembe.2011.06.001
Tamayo, D., Ibarrola, I., Cigarria, J., and Navarro, E. (2015). The effect of food conditioning on feeding and growth responses to variable rations in fast and slow growing spat of the Manila clam (Ruditapes philippinarum). J. Exp. Mar. Biol. Ecol. 471, 92–103. doi: 10.1016/j.jembe.2015.05.017
Tamayo, D., Ibarrola, I., and Navarro, E. (2012). Thermal dependency of clearance and metabolic rates in slow and fast growing spat of Manila clam Ruditapes philippinarum. Comp. Biochem. Physiol. A. Mol. Integr. Physiol. 163, 893–904. doi: 10.1007/s00360-013-0764-1
Tamayo, D., Ibarrola, I., Urrutia, M. B., and Navarro, E. (2011). The physiological basis for inter-individual growth variability in the spat of clams (Ruditapes philippinarum). Aquaculture 321, 113–120. doi: 10.1016/j.aquaculture.2011.08.024
Tamayo, D., Ibarrola, I., Urrutxurtu, I., and Navarro, E. (2014). Physiological basis of extreme growth rate differences in the spat of oyster (Crassostrea gigas). Mar. Biol. 161, 1627–1637. doi: 10.1007/s00227-014-2447-1
Tremblay, R., Myrand, B., Sevigny, J. M., Blier, P., and Guderley, H. (1998). Bioenergetic and genetic parameters in relation to susceptibility of blue mussels, Mytilus edulis (L.) to summer mortality. J. Exp. Mar. Biol. Ecol. 221, 27–58. doi: 10.1016/S0022-0981(97)00114-7
Vega-Villasante, F., Nolasco, H., and Civera, R. (1993). The digestive enzymes of the pacific brown shrimp penaeus californiensis.: I—properties of amylase activity in the digestive tract. Comp. Biochem. Phys. B. 106, 547–550. doi: 10.1016/0305-0491(93)90130-W
Vladimirova, I. G., Kleimenov, S. Y., and Radzinskaya, L. I. (2003). The relation of energy metabolism and body weight in bivalves (Mollusca: Bivalvia). Biol. Bull. Russ. Acad. Sci. 30, 392–399. doi: 10.1023/A:1024822225406
Wang, A., Deng, F., Zhang, X., Yan, B., and Zhang, M. (2000). RAPD Analysis on genetic diversity of Pinctada martensii Dunker. Wuhan University J. 46, 467–470.
Wang, A., Shi, Y., and Zhou, Z. (2004). Morphological trait parameters and their correlations of the first generation from matings and crosses of geographical populations of Pinctada martensii (Dunker). Mar. Fish. Res. 25, 39–45.
Wang, A., Wang, Y., Gu, Z., Li, S., Shi, Y., Guo, X., et al. (2011a). Development of expressed sequence tags from the pearl oyster, Pinctada martensii Dunker. Mar. Biotechnol. 13, 275–283. doi: 10.1007/s10126-010-9296-9
Wang, J., Zhao, L., Liu, J., Wang, H., and Xiao, S. (2015a). Effect of potential probiotic Rhodotorula benthica D30 on the growth performance, digestive enzyme activity and immunity in juvenile sea cucumber Apostichopus japonicus. Fish. Shellfish. Immun. 43, 330–336. doi: 10.1016/j.fsi.2014.12.028
Wang, Q., Deng, Y., Du, X., Fu, S., and Lu, Y. (2011b). Realized heritability and genetic gains of three generations for superior growth in the pearl oyster Pinctada martensii. Acta Ecol. Sinica 31, 108–111. doi: 10.1016/j.chnaes.2010.12.001
Wang, Q., Zhang, S., Du, X., Deng, Y., and Huang, R. (2009). Comparison of oxygen consumption rate and NH3 excretion rate of the first-generation yellow shell color and control groups at different temperatures and salinities. J. Fish. China 33, 790–796.
Wang, Q., Zhang, S., Du, X., Deng, Y., and Huang, R. (2010). A comparative analysis of digestive enzyme activities of first generation yellow shell color and cultivated stocks of Pinctada martensii at suitable growth temperatures. J. Fish. Sci. China. 6, 1351–1356. doi: 10.3724/SP.J.1011.2010.01351
Wang, Y., Li, L., Hu, M., and Lu, W. (2015b). Physiological energetics of the thick shell mussel Mytilus coruscus exposed to seawater acidification and thermal stress. Sci. Total. Environ. 514, 261–272. doi: 10.1016/j.scitotenv.2015.01.092
Weel, P. B. V. (1961). The comparative physiology of digestion in molluscs. Am. Zool. 1, 245–252. doi: 10.1093/icb/1.2.245
Wilbur, K. M., and Anderson, N. G. (1950). Carbonic anhydrase and growth in the oyster and busycon. Biol. Bull. 98, 19–24. doi: 10.2307/1538594
Xu, C., Li, Q., and Chong, J. (2020). Combined effect of temperature, salinity, and rearing density on the larval growth of the black shell strain and wild population of the Pacific oyster Crassostrea gigas. Aquacult. Int. 28, 1–13. doi: 10.1007/s10499-019-00465-4
Xu, Y., Zhang, Y., Liang, J., He, G., Liu, X., Zheng, Z., et al. (2021). Impacts of marine heatwaves on pearl oysters are alleviated following repeated exposure. Mar. Pollut. Bull. 173:112932. doi: 10.1016/j.marpolbul.2021.112932
Zhang, J., Li, Q., Liu, S., and Yu, H. (2018). The effect of temperature on physiological energetics of a fast-growing selective strain and a hatchery population of the Pacific oyster (Crassostrea gigas). Aquac. Res. 49, 2844–2851. doi: 10.1111/are.13747
Zhao, L., Liu, L., Liu, B., Liang, J., Lu, Y., and Yang, F. (2019a). Antioxidant responses to seawater acidification in an invasive fouling mussel are alleviated by transgenerational acclimation. Aquat. Toxicol. 217:105331. doi: 10.1016/j.aquatox.2019.105331
Zhao, L., Shirai, K., Tanaka, K., Milano, S., Higuchi, T., Murakami-Sugihara, N., et al. (2020). A review of transgenerational effects of ocean acidification on marine bivalves and their implications for sclerochronology. Estuar. Coast Shelf S 235:106620. doi: 10.1016/j.ecss.2020.106620
Zhao, L., Zuykov, M., Tanaka, K., Shirai, K., Anderson, J., McKindsey, C. W., et al. (2019b). New insight into ligh-enhanced calcification in mytilid mussels, Mytilus sp., infected with photosynthetic algae Coccomyxa sp.: δ13C value and metabolic carbon record in shells. J. Exp. Mar. Biol. Ecol. 520:151211. doi: 10.1016/j.jembe.2019.151211
Keywords: pearl oyster, selective strain, physiological, feeding, metabolism
Citation: Zhang X, Ye B, Gu Z, Li M, Yang S, Wang A and Liu C (2021) Comparison in Growth, Feeding, and Metabolism Between a Fast-Growing Selective Strain and a Cultured Population of Pearl Oyster (Pinctada fucata martensii). Front. Mar. Sci. 8:770702. doi: 10.3389/fmars.2021.770702
Received: 04 September 2021; Accepted: 12 October 2021;
Published: 30 November 2021.
Edited by:
Liqiang Zhao, Guangdong Ocean University, ChinaReviewed by:
Pengzhi Qi, Zhejiang Ocean University, ChinaChuangye Yang, Guangdong Ocean University, China
Linda Adzigbli, University of Rostock, Germany
Copyright © 2021 Zhang, Ye, Gu, Li, Yang, Wang and Liu. This is an open-access article distributed under the terms of the Creative Commons Attribution License (CC BY). The use, distribution or reproduction in other forums is permitted, provided the original author(s) and the copyright owner(s) are credited and that the original publication in this journal is cited, in accordance with accepted academic practice. No use, distribution or reproduction is permitted which does not comply with these terms.
*Correspondence: Aimin Wang, aimwang@163.com; Chunsheng Liu, lcs5113@163.com
†These authors have contributed equally to this work